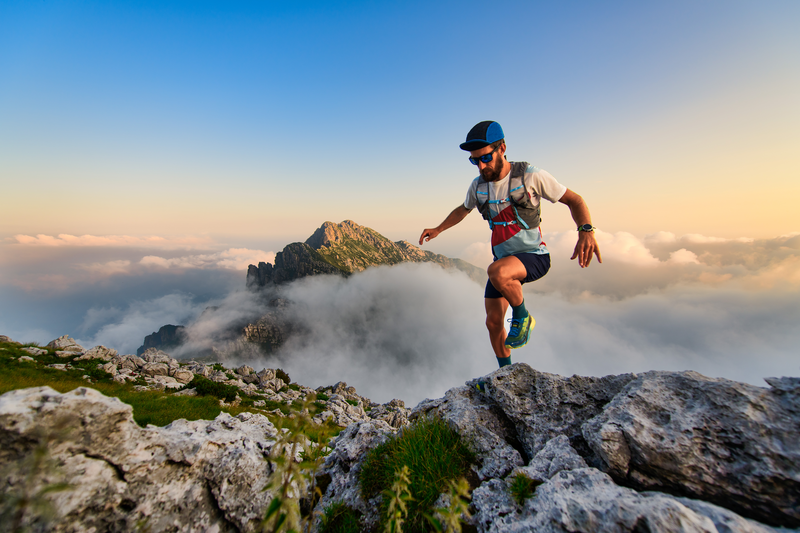
94% of researchers rate our articles as excellent or good
Learn more about the work of our research integrity team to safeguard the quality of each article we publish.
Find out more
ORIGINAL RESEARCH article
Front. Sustain. Food Syst. , 11 June 2021
Sec. Land, Livelihoods and Food Security
Volume 5 - 2021 | https://doi.org/10.3389/fsufs.2021.616033
This article is part of the Research Topic Soil Fertility Management for Sustainable Food Production in Sub-Saharan Africa View all 11 articles
Peanut forms a major component of the predominantly cereal-based farming systems in Northern Ghana. However, yields are low, prompting the need to evaluate the effects of phosphorus (PR) and rhizobium inoculant (IR) rates on growth, nodulation, and yield of peanut varieties. On-station and on-farm experiments were conducted to determine the interaction effects of three P rates (0, 30, and 60 kg P2O5/ha), three IR rates (0, 3, and 6 g/kg seed), and two peanut varieties [Chinese and Nkatie Savannah Agricultural Research Institute (SARI)] on growth, nodulation, and yield of peanut on Haplic Lixisols of Northern Ghana. Both experiments were conducted using a split-split plot design replicated three times for the on-station experiment and on six farmer's fields (on-farm experiment). In both experiments, combined application of 60 kg P2O5/ha and IR at 6 g/kg seed increased pod number in the Nkatie SARI and Chinese varieties compared to their control counterparts. PR × V interaction influenced growth, effective nodule number, and podding capacity with 60 kg P2O5/ha combined with Nkatie SARI to produce significantly higher values. The interaction of IR × V improved pod number, nodule number, and harvest index, such that inoculant at 6 g/kg seed combined with Nkatie SARI gave the best performance. PR × IR also had a significant interactive influence on peanut grain yield. Higher grain yields were recorded from 60 kg P2O5/ha in combination with 6 g/kg seed of rhizobium inoculant. Therefore, these results suggest that the use of P fertilizer at 60 kg/ha and rhizobium inoculant at 6 g/kg seed increase peanut productivity on Haplic Lixisols in Northern Ghana. However, it was prudent not to suggest any recommendations from the P rates in interaction with IR, since the result between the on-station and on-farm experiments appeared not consistent.
Peanut (Arachis hypogea L.) is among the three most important grain legumes in West Africa, which forms a major component of the predominantly cereal-based farming systems. It is primarily grown as food and cash income, but in the dry savannas of Western Africa, the haulms and husk after harvest are excellent sources of quality livestock feed (Adjei-Nsiah et al., 2018). According to the Food and Agriculture Organization (FAO, 2018) estimate for 2018, 28.5 million hectares of peanut were harvested worldwide, of which the West African sub-region harvested 7.1 million hectares. In Ghana, 95% of the total national production of peanut occurs in Northern Ghana (Ministry of Food and Agriculture, 2012). Despite this, peanut yield on farmers' fields is low due to several biotic and abiotic factors, three of which are low and declining soil fertility, high cost and/or unavailability of inputs (seed, fertilizer, an inoculant), and use of low-yielding varieties (Kolawole, 2012).
Phosphorus (P) is an important mineral nutrient that promotes the growth of leguminous crops to produce their own N sources. Therefore, P deficiency can negatively affect access to atmospheric N by legumes (Adjei-Nsiah et al., 2018). In confirming this, Asante et al. (2020) indicated that rhizobium activity and N2 fixation are negatively affected when the system lacks phosphorus (P) since P serves as an energy source for the rhizobia. Similarly, the requirement of P in nodulating legumes is higher compared to non-nodulating crops as it contributes significantly to root development (Tang et al., 2001), root nodulation (Nwaga and Ngo Nkot, 1998), and shoot uptake of N, P, and K (Ramesh et al., 1997) in legumes. Due to the important role played by P in plant growth, its addition to P-deficient soils leads to an increase in grain yield (Uchida, 2000). However, there are conflicting results on the optimum application rate of P on yield of peanut as some authors have reported higher yields at 60 kg P2O5/ha (Panwar and Naidu, 2002) while no significant effect on yield was recorded by Taruvinga (2014), when P rate was increased beyond 45 kg P2O5/ha. This has therefore renewed interest for further research.
Leguminous crops are also able to meet a significant portion of their nitrogen requirement when they are cultivated in the presence of effective rhizobia (Bekere and Hailemariam, 2012). It has been reported that leguminous plants with symbiotic nitrogen-fixing bacteria are able to fix about 15–210 kg/ha of nitrogen per season (Dakora and Keya, 1997). However, the effective functioning of rhizobia can be influenced by the availability of other soil nutrients, especially P. In confirming this, Asante et al. (2020) stated that rhizobium activity is reduced when the soil lacks phosphorus (P). Thus, in the presence of P, the inoculation of peanut with the appropriate rate of rhizobium inoculant can be a beneficial strategy to improve productivity.
In addition to phosphorus and rhizobium inoculant application, the grain yield of peanut may be increased through the cultivation of improved peanut varieties. According to Zhou et al. (2016), the identification and use of P-efficient legume genotypes is a sustainable P management strategy for enhancing yield and P use efficiency. While studies have shown that the use of improved varieties (Konlan et al., 2013) and the application of the appropriate rate of P fertilizer (Kamara et al., 2011) and rhizobium inoculant (Catroux et al., 2001) have the potential to improve the productivity of peanut, there is limited information on the interaction effects of variety, phosphorus rate, and rate of rhizobium inoculation on the yield of peanut varieties under on-farm conditions. Similarly, there is a gap in knowledge on the effect of P fertilizer and rhizobium inoculant rates on productivity of peanut varieties in Ghana. The need therefore arises to undertake a study on the comparative and interactive effects of P fertilization and rhizobium inoculation on productivity of peanut varieties to help inform measures that aim to increase productivity of the legume.
In this paper, we study the comparative and interactive effects of P fertilization and rhizobium inoculation on growth, nodulation, yield, and nutrient uptake in two peanut varieties on Haplic Lixisols in the Guinea savanna zone of Ghana.
The study was conducted under both on-station and on-farm conditions in the Guinea savanna agro-ecological zone of Ghana during the 2018 cropping season (July–October).
This was carried out at the experimental field of the Faculty of Agriculture, University for Development Studies (UDS), Nyankpala, in the northern region of Ghana. The site is located on latitude 9°25′N, longitude 0°59′W (Figure 1) and at an altitude of 183 m above sea level. The total annual rainfall received by the area ranges between 800 and 1,200 mm, which occurs from May to October, with a dry season characterized by harmattan winds occurring between October and April. The mean monthly temperatures range from 25°C in December to 38°C in April. The soil of the study area was Haplic Lixisols (International Union of Soil Sciences Working Group, 2014) with organic carbon of about 4.2 g/kg, total nitrogen of 4 g/kg, exchangeable potassium of 42.6 mg/kg, and available phosphorus of 6 mg/kg.
The on-farm experiment was carried out in two communities in the Guinea savanna agro-ecological zone of Ghana. These were at Gurumanchenyili (9°24′N, 1°0′W) and Zangbalun Fandu (9°36′N, 0°58′W) (Figure 1). The climate is warm, semi-arid tropical and has a unimodal rainfall of 800–1,200 mm, which increases from March until a maximum is reached in August/September. There is considerable variation between years in the time of onset, duration, and amount of rainfall. The vegetation is basically Guinea savanna which is interspersed with trees and grassland that are drought resistant. Bush fire is an annual event, which usually destroys the vegetation (Amoako et al., 2018). The fires are noted to result in substantial losses of plant nutrients, which has a negative impact on the livelihood of the people.
The experimental field was plowed and harrowed with a tractor, after which the field was laid out. The experimental design was a split-split plot arranged in a randomized complete block with three replications. The treatments were made up of three rhizobium inoculant rates (IR) (0, 3, and 6 g/kg seed) as the main plot factor, three P fertilizer rates (PR) (0, 30, and 60 kg P2O5/ha) as the sub-plot factor, and two peanut varieties (V) [Chinese and Nkatie Savannah Agricultural Research Institute (SARI)] as the sub-sub-plot factor. Variety was used as the sub-sub-plot, followed by P fertilization as the sub-plot and inoculant rate as the main plot to reduce potential error attributable to factor migration. Variety is the least migrating factor, followed by P, while the bacteria in the inoculant are the most mobile during the growing season. The inoculant rates were halved to understudy productivity of nodulation under a limited- or scarce-inoculant condition. The sub-plot size was 4 × 2.4 m, and an alley of 1 and 2 m separated sub-plots within and between replications, respectively. The sub-plot consisted of six rows, each 4 m long with an inter-row spacing of 40 cm and an intra-row spacing of 20 cm. The peanut was sown at two seeds per stand and thinned to one seedling per stand 2 weeks after planting.
The same experimental design, planting distance, and treatment combinations as used in the on-station experiment were used in the on-farm experiment. These included three rates of rhizobium inoculant, three rates of P fertilizer, and the two peanut varieties. The treatments were replicated on six farmers' fields across the two communities, thus one replication per farmer. To ensure minimal soil variation, farmers' fields with similar soil type, slope, and minimal shading with no history of peanut cultivation in previous years were selected.
Two groundnut varieties—Chinese, a spreading type with 100 days of maturity duration, and Nkatie SARI, an erect bunch habit with 110 days of maturity duration released by the Council for Scientific and Industrial Research (CSIR)—were used in the experiment. A commercial Bradyrhizobium inoculant product, SARIFIX, containing strain BR 3267 was obtained from the Savanna Agricultural Research Institute, Tamale, Ghana, for the experiment.
Seed inoculation was performed just before planting by weighing 1 kg of seeds into a plastic container and adding 10 ml of dissolved gum Arabic solution as a sticker. The seeds and gum Arabic solution were mixed thoroughly, and the respective mass of SARIFIX inoculant (strain BR 3267) at 10 g/kg seeds of a 100-g pack was applied to the seed and mixed thoroughly to ensure that all the seeds were effectively covered with the inoculant before planting.
Prior to treatment application and before planting, representative soil samples were collected from a depth of 0–20 cm using a soil auger. Similar sampling was done after harvest on each treatment plot. A composite was made from 30 samples (on-station experiment) and 10 samples (per farmer's field) collected randomly from different parts of the plot and thoroughly mixed, sub-sampled, air-dried, and then sieved with a 2-mm sieve. The sieved soil samples were analyzed at SARI for chemical and physical soil properties. The soil pH was determined according to the electrometric method. Organic carbon was analyzed by the Walkley–Black procedure as described by Nelson and Sommers (1982), total N by the Kjeldahl method as described by KIT (1984), P by the Bray-1 method as described by Bray and Kurtz (1945), and K by the flame photometer method as described by Hald (1947). Particle size distribution was carried out by the hydrometer method as described by Bouyoucos (1962).
At 8 weeks after sowing, data on growth (plant height) and nodulation assessment were collected for both on-station and on-farm experiments. The plant height of five peanut plants was measured from the two central rows of each plot. The average height of the five plants were then calculated for each plot. For nodulation assessment, five plants outside the two central rows of each plot were carefully uprooted and placed in a plastic bowl containing water for 20 min to loosen adhering soil on the roots. The nodules were then removed, counted, and dissected to determine effective nodules using the procedure described by Ishizawa and Toyoda (1955).
At physiological maturity, plants from the two middle rows of each sub-sub-plot (3.2 m2) were harvested, after which five plants were randomly selected from the harvested plants for each sub-sub-plot to determine their podding capacities (pod number and weight). The average number and weight of pods were then calculated for each sub-sub-plot, after which both plant and pods were added back to the harvested plants for each sub-sub-plot. The plants (whole plant with pod) from the harvested area (3.2 m2) were weighed with an electronic scale, after which the pods were removed and shelled. The seed was then weighed and subtracted from the initial weight (whole plant with pod) to obtain the haulm weight per plot. It was then expressed in kg/ha. Grain yield per hectare was determined by shelling the pods from the harvested area (3.2 m2) of each sub-sub-plot. The shelled seeds were then oven-dried at 65°C to a moisture of 13% to measure grain yield.
Plant nutrient analysis was not performed for the on-station experiment. At physiological maturity, a quadrat (0.5 m2) was placed outside the two central rows of each plot, and representative plants per each farmer's plot were harvested to determine the effects of PR, IR, and V on nutrient uptake. Plant shoots and roots from on-farm experiments were cut into pieces and then oven-dried at 70°C for 2 days to a constant weight. The oven-dried samples were then milled and analyzed chemically for N using the Kjeldahl method (Bremner and Muluvaney, 1982), P using the VV/VIS spectrophotometer through colorimetric determination, and K using the flame photometer (Myers et al., 1947). To determine biological nitrogen fixation (BNF), the reference crop used (maize) was sown on plots that received application rates of P and rhizobium inoculant as the peanut plots. Whole plants of both maize and peanut were sampled by treatment and prepared for the quantitative determination of total nitrogen, using the Kjeldahl method. The amount of nitrogen fixed (N%) in the plant was then calculated by using the nitrogen difference technique (Mweetwa et al., 2014; Anglade et al., 2015) as follows:
Q = N yield (groundnut) – N yield (maize) + [N soil (groundnut) – N soil (maize)]
where
Q = Quantity of legume N derived from N2 fixation
N yield (groundnut) = Total N in groundnut plant
N yield (maize) = Total N in maize plant
N soil (groundnut) = Soil mineral N for groundnut plots after harvest
N soil (maize) = Soil mineral N for maize plots after harvest.
The general linear model procedure of the Statistix 10 analytical package (Statistix, 2013) for Windows was used to analyse data from the two experiments. The Duncan multiple-range test was used to separate treatment means of significant difference at the 5% probability level.
Table 1 shows the physicochemical properties of soil for both experiments (on-station and on-farm). Generally, the soil at the study sites was sandy loam with a slightly acidic pH (Table 1). The rating for the soil was done according to the classification by Sela (2020). The organic carbon level was very low (1.05–1.09%), while available phosphorus was low (0.02–0.03%); similarly, the exchangeable potassium range of 0.04–0.06% for the on-farm sites was low. The exchangeable potassium value of 0.02% recorded for the on-station site was low. The total nitrogen recorded before planting at the sites was generally very low.
The IR × PR × V, likewise IR × PR and IR × V, was not significant on plant height. However, the PR × V and the effect of IR and V were significant on plant height (Table 2), such that PR at 60 kg P2O5/ha combined with the Chinese variety produced significantly higher values for plant height than did Nkatie SARI (Figure 2).
Table 2. Summary of ANOVA for variety (V), phosphorus (PR), and rhizobium inoculant rate (IR) effects on plant height, nodulation, yield, and yield components of groundnut in the on-station and on-farm experiments.
Figure 2. Interactive effect of P-rate (PR) and variety on plant height (cm) of groundnut at 8 weeks after planting (8 WAP). Bars with different letters (in horizontal direction) show significantly different means. Error bars represent standard error of mean.
IR × PR × V and IR × PR, IR × V, and PR × V were not significant on nodulation parameters. However, significant differences in nodule number per plant and effective nodule number per plant occurred among IR, PR, and V treatments. Inoculation or P application increased nodule number per plant by an average of 26 or 50%, respectively, compared with their control, while the use of Nkatie SARI over the Chinese variety increased nodule number per plant by 7% (Table 3). Applying inoculant or P alone increased significantly the effective number of nodules per plant by an average of 46 or 25%, respectively, compared to their control, while sowing with Nkatie SARI produced 29% more effective nodule number per plant than did the Chinese variety (Table 3).
Table 3. Plant height, nodulation, yield, and yield components of groundnut as affected by variety, phosphorus, and rhizobium inoculation rates in the on-station experiment.
The effects of IR, PR, and V and their interactions on podding capacity were not consistent. For pod number per plant, the effects of IR × PR × V, IR × PR, IR × V, and PR × V were significant (Table 2). Inoculation and P application either alone or in combination with variety increased significantly the pod number per plant compared with the control. However, inoculation and P application appeared to favor higher pod number in Nkatie SARI than in the Chinese variety (Figure 3). Conversely, pod weight followed a different trend as that for the pod number. The IR × PR × V interaction was not significant on pod weight; however, the effects of IR and PR contributed significantly to pod weight such that IR at 6 g/kg seed and PR at 60 kg P2O5/ha produced the heaviest pod weight compared with un-inoculated and un-applied control plots, respectively.
Figure 3. Interactive effect of P-rate (PR), inoculant rate (IR), and variety (V) on pod number of groundnut grown in Haplic Lixisols of northern Ghana. Bars with different letters (in horizontal direction) show significantly different means. Error bars represent standard error of mean.
IR × PR × V, IR × V, and PR × V were not significant on grain yield; however, significant differences in grain yield occurred among IR, PR, V, and IR × PR treatments (Table 2). Grain yield of IR at 3 and 6 g/kg seed combined with PR at 60 kg P2O5/ha increased significantly compared with the control (Figure 4). Grain yield was positively correlated with effective nodules and pod number (Table 5). These results indicate that when the number of effective nodules and pods increases, grain yield also increases.
Figure 4. Interactive effect of P-rate (PR) and inoculant rate (IR) on grain yield of groundnut grown in Haplic Lixisols of northern Ghana. Bars with different letters (in horizontal direction) show significantly different means. Error bars represent standard error of mean.
IR × PR × V, IR × PR, and PR × V were not significant on haulm weight and harvest index (HI); however, significant differences occurred among IR × V treatments (Table 2). IR at 6 g/kg seed had higher (P < 0.05) haulm weight and HI at all levels of peanut variety compared with other IR at all levels of peanut variety (Figure 5).
Figure 5. Interactive effect of Innoculant rate (IR) and variety (V) on halum weight (A) and harvest index (B) of groundnut grown in Haplic Lixisols of northern Ghana. Bars with different letters (in horizontal direction) show significantly different means. Error bars represent standard error of mean.
The IR × PR × V, IR × PR, PR × V, and IR × V as well as the effects of IR and PR did not affect (P > 0.05) plant height of peanut. However, the effect of V was significant on plant height, with Nkatie SARI recording higher (P < 0.05) plant height than the Chinese variety (Table 4).
Table 4. Plant height, nodulation, yield, and yield components of groundnut as affected by variety, phosphorus, and rhizobium inoculation rates in the on-farm experiment.
For nodulation parameters, significant differences in nodule number per plant and effective nodule number per plant occurred among IR × V and PR × V treatments, respectively (Table 2). Applying the inoculant at 6 g/kg seed to Nkatie SARI increased significantly the nodule number per plant by 13% compared with that for the non-inoculated Nkatie SARI plots. However, applying the inoculant at 3 g/kg seed appeared to favor the Chinese variety, as it increased (P < 0.05) nodule number per plant in the Chinese variety by 8 and 7% compared to IR at 6 g/kg seed and the non-inoculated Chinese variety, respectively (Figure 6A). The application of 30 kg P2O5/ha combined with Nkatie SARI increased (P < 0.05) effective nodule number compared with the control (Figure 6B). Furthermore, the effective nodule number per plant observed from Nkatie SARI at each level of P was higher than that of the Chinese variety at each level of P.
Figure 6. Interactive effect of Innoclant rate (IR) and variety (V) on nodule number per five plants (A) and; P-rate and variety on effective nodule number per five plants of groundnut (B) grown in Haplic Lixisols of northern Ghana. Bars with different letters (in horizontal direction) show significantly different means. Error bars represent standard error of mean.
The IR × PR × V, IR × PR, PR × V, and IR × V, likewise the effect of IR and PR, were not significant on pod number per plant; however, the effect of V significantly increased pod number per plant, with the Chinese variety recording higher (P < 0.05) pod number than did Nkatie SARI (Table 4). Pod weight was significantly influenced by PR × V (Table 2). PR at 60 kg P2O5/ha combined with the Chinese and Nkatie SARI varieties increased (P < 0.05) pod weight compared with the control (Figure 7).
Figure 7. Interactive effect of P-rate (PR) and inoculant rate (IR) on pod weight of groundnut grown in Haplic Lixisols of northern Ghana. Bars with different letters (in horizontal direction) show significantly different means. Error bars represent standard error of mean.
IR × PR × V, IR × V, and PR × V, likewise the effect of V, were not significant on grain yield; however, significant differences occurred among IR and PR treatments. The grain yield of IR at 6 g/kg seed and PR at 60 kg P2O5/ha contributed to higher (P < 0.05) grain yields, respectively. Furthermore, the lowest grain yields from both IR and PR were recorded in their respective controls (Table 4). Grain yield was positively correlated with pod weight (Table 5). This indicates that an increase in pod weight results in a corresponding increase in grain yield.
Table 5. Coefficient of correlation (r) among plant height, nodulation, yield, and yield components of groundnut in the on-station and on-farm experiments.
IR × PR × V, IR × V, and PR × V, likewise the effect of V, were not significant on both haulm weight and HI; however, the effects of IR and PR were significant on both haulm weight and HI (Table 4). The haulm weight of IR at 0 g/kg seed increased significantly compared with that of the inoculated plots. Conversely, the HI of IR at 0 g/kg seed reduced significantly compared with that of the inoculated plots (Table 4). Similarly, the effect of PR on haulm weight and HI followed a similar trend as that for IR. The highest (P < 0.05) haulm weight and the lowest HI were observed in the control (0 g/kg seed) (Table 4).
The IR, PR, and V interactions, likewise the effect of V, did not significantly contribute to N fixed in soil; conversely, the effects of IR and PR influenced N fixation in soil, with their respective controls performing poorly (Table 6).
Table 6. N fixation and N, P, and K uptake by groundnut as affected by variety, phosphorus, and rhizobium inoculation rates in the on-farm experiment.
The IR × PR × V, IR × PR, and IR × V were significant on N uptake in the shoot and root (Table 2). N uptake at the inoculant rate of 6 g/kg seed and P rate at 60 kg P2O5/ha combined with Nkatie SARI increased significantly compared to the control under both varieties (Figure 8). Furthermore, at all levels of IR × PR, uptake of N in the shoot and root appeared to be higher in Nkatie SARI than in the Chinese variety (Figure 8).
Figure 8. Interactive effect of P-rate (PR), inoculant rate (IR), and variety (V) on N uptake of groundnut grown in Haplic Lixisols of northern Ghana. Bars with different letters (in horizontal direction) show significantly different means. Error bars represent standard error of mean.
The IR × PR × V, PR × V, and IR × V were not significant on P uptake in the shoot and root; however, IR × PR interaction significantly improved P uptake (Table 2), such that the inoculant rate of 6 g/kg seed combined with P rate at 60 kg P2O5/ha gave the best performance (Figure 9). In contrast with the other levels of PR, 60 kg P2O5/ha at all levels of IR influenced higher (P < 0.05) uptake of P in the root and shoot (Figure 9).
Figure 9. Interactive effect of P-rate (PR) and inoculant rate (IR) on P uptake of groundnut grown in Haplic Lixisols of northern Ghana. Bars with different letters (in horizontal direction) show significantly different means. Error bars represent standard error of mean.
The IR × PR × V was not significant on K uptake in the shoot and root; however, the IR × PR, PR × V, and IR × V were significant on K uptake in the shoot and root. K uptake in the shoot and root of IR at 6 g/kg seed combined with PR at 60 kg P2O5/ha increased significantly compared with the control (Figure 10A).
Figure 10. Interactive effect of effects of P-rate (PR) and inoculant rate (IR) (A); P-rate (PR) and variety (V) (B); and inoculant rate (IR) and variety (V) (C) on K uptake of groundnut in Haplic Lixisols of northern Ghana. Bars with different letters (in horizontal direction) show significantly different means. Error bars represent standard error of mean.
The PR at 60 kg P2O5/ha combined with the Chinese variety significantly increased K uptake in the shoot and root compared with the control (Figure 10B). Conversely, the IR at 6 g/kg seed combined with the Nkatie SARI variety increased K uptake in the shoot and root significantly compared to the control (Figure 10C).
The significant interaction effect of PR × V on plant height as observed in the on-station experiment could possibly be due to the supply of plant nutrients to peanut varieties from P application. Several authors (Shiyam, 2010; Kabir et al., 2013) have reported on the positive influence of P on peanut growth and development. Similarly, Adjei-Nsiah et al. (2018) attributed rapid plant growth in soybean to P fertilization. Although the results of the on-farm experiment did not show any significant interaction effect on plant height, there was a significant difference in plant height among peanut varieties; the Nkatie SARI recorded higher plant height than the Chinese variety. The difference in plant height among the varieties could possibly be due to differences in genetic composition. In confirming this, Konlan (2010) observed significant differences in plant height among six peanut varieties (Adepa, Azivivi, Jenkaar, Kpanieli, Nkosour, and Manipintar) and attributed these differences to genetic factors. Similarly, Golakia et al. (2005) reported a significant difference in plant height between the Virginia runner type and the Spanish bunch type of peanut. However, the discrepancy in response of peanut plant height to inoculation and/or P application across experiments could be due to differences in prevailing soil conditions in the two experiments; although we did not assess soil temperature and moisture in our study, there is sufficient evidence that available soil moisture had a negative influence on peanut growth. In addition to soil nutrients and cultivar selection, germination, emergence, and plant vigor are primarily determined by the temperature and soil moisture in the seeding zone (Kumar et al., 2012).
The process of BNF heavily involves the formation of nodules. In this study, the nodule number and effective number of nodules were significantly influenced by IR × V and PR × V interactions, respectively, in the on-farm experiment. These results indicate that the ability to nodulate and further produce effective nodules is not only a genetic attribute but can also be influenced by the application of either P fertilizer or inoculant. In confirming this, several authors (Gentili et al., 2006; Lira et al., 2015) have reported on the significant influence of phosphorus and rhizobium inoculant on nodule and effective nodule formation in legumes. Similarly, Boddey et al. (2017) reported a higher nodule number in Northern Ghana due to the application of the Bradyrhizobium inoculant with P fertilizer. The study of Asante et al. (2020) on yield response of groundnut to inoculation and P application also supports this conclusion. In the on-station experiment, nodule and effective nodule formation did not differ significantly among IR, PR, and V interactions. This discrepancy in results between the on-farm and the on-station experiments suggests that, in the on-station experiment, the varieties responded similarly to inoculation and/or P application. This could be attributed to the low soil pH (4.9) at the on-station experiment (Table 1), which might have reduced phosphorus availability and impaired nitrogen fixation. This finding is affirmed by the report of Sela (2020) that the optimal pH range for enhanced phosphorus availability to crops is between 6.0 and 7.0.
The significant interaction effect of IR × PR × V on pod number per plant could possibly be due to the supply of nutrients by P and the introduction of effective rhizobia for peanut formation. The above interaction result indicated that peanut varieties require the application of both P and rhizobium inoculant to enhance its podding capacity. These results agree with earlier reports that inoculant combined with phosphorus fertilizer increased pod yield per plant more than the application of the inoculant or phosphorus fertilizer alone (Kyei-Boahen et al., 2017). Conversely, the interactive effect of IR, PR, and V did not affect (P < 0.05) pod weight. The failure of pod number to translate into pod weight could probably be due to drought conditions experienced during the pod filling period, which might have masked the positive influence of IR × PR on pod filling in the varieties. According to Martinson (2009), adequate soil moisture received during pod filling and seed formation periods results in rapid translocation of photosynthates to reproductive parts. PR × V influenced pod weight in the on-farm experiment. Statistically, Chinese and Nkatie SARI varieties combined with 60 kg P2O5/ha produced the highest pod weight. This increase in pod weight could possibly be due to enhanced stimulation of pod filling in such treatment combinations. In confirming this, Hernanadez and Cuevas (2003) recorded increased pod weight under 100 kg P2O5/ha application than the control (no P application).
The significant effect of IR × PR on grain yield in the on-station experiment could possibly be due to the positive interactive effect of the P fertilizer and rhizobium inoculant. The increase in grain yield with P fertilizer and rhizobium inoculant supports earlier findings that the combined application of P with rhizobium inoculant increased grain yield and nitrogenase activity as well as enhanced soil fertility (Fatima et al., 2007). Similarly, a study by Ulzen et al. (2018) reported significantly higher soybean grain yields from plots that received P and/or inoculation than those of the control plots. Accordingly, Ndakidemi and Semoka (2006) recommended that, for farmers who can afford P fertilization, its combined use with inoculants can further increase grain yield. In the on-farm experiment, grain yield did not differ significantly among IR, PR, and V interactions. It can be deduced that the IR and PR failed to play a complementary role with peanut varieties. This is corroborated by the insignificant response of P uptake (%) to IR × PR × V (Table 2). This observation contradicts research findings by Kyei-Boahen et al. (2017), where the application of P and inoculant affected (P < 0.05) grain yield of cowpea. Although there were no significant interaction effect on grain yield in the on-farm experiment, the effects IR and PR significantly affected grain yield. These results indicate that phosphorus and inoculant were the main deficient factors for peanut performance in the on-farm sites during this study, confirming earlier observations made by Stefanescu and Palanciuc (2000) that phosphorus and inoculation induced a pronounced effect on grain yield.
Haulm weight and HI were significantly affected by the interaction effect of IR × V in the on-station experiment. This could possibly be due to the supply of nutrients as enhanced by the application of rhizobium inoculant. The above interaction results indicated that higher dry matter accumulation in peanut requires the application of rhizobium inoculant. Amos et al. (2001) reported significantly higher dry matter accumulation, when rhizobium inoculation and P fertilizer were applied to common bean (Phaseolus vulgaris). In the on-farm experiment, haulm weight, and HI were significantly influenced by the effects of PR and IR. This result is in consonance with the findings by Emmanuel et al. (2021), who reported that the application of P and Bradyrhizobium inoculant increased dry matter of cowpea in savanna soils of Ghana. The application of either P fertilizer or inoculant most often influenced nitrogenase activity, and this nitrogen helps in vegetative plant growth, which might explain the significant effect of P and inoculant on haulm yield in this study.
N2 fixed in the soil was significantly enhanced by the effect of the phosphorus rate and rhizobium inoculant rate. This observation could be due to the availability of phosphorus needed by rhizobium for N2 fixation, affirming the importance of both in peanut production. This result agrees with the report of Adjei-Nsiah et al. (2018) who stated that N fixation is strongly influenced by the availability of both P and effective rhizobium strain. However, in this study, the average nitrogen stored in plants was 148% higher in plants compared with that in the soil.
The significant effect of IR × PR × V interaction on N uptake in shoots and roots could possibly be attributed to the adequate plant nutrients supply by both P fertilizer and inoculant to peanut. P application influenced the growth of plant roots while the introduction of rhizobia ensured that there were enough bacteria in the rhizosphere of the root to enhance formation of nodules for N fixation. Similar findings were reported by Rodelas et al. (1999) when they attributed N uptake in the shoots of fava beans to enhanced root development, which resulted in increased nutrient uptake.
The results from this study indicate that the combined application of phosphorus and inoculant affected P uptake in the shoots and roots, with the highest P rate and IR combining to influence larger uptake of P. The value recorded under the interaction of 6 g/kg seed and 60 kg P2O5/ha for P (0.73%) was higher than the sufficiency range for P (0.2–0.5%), as reported by Motsara and Roy (2008). This result suggests that a combined higher rate of P and inoculant application could lead to higher P uptake in the shoots and roots of peanuts. However, the results of our study contradict earlier reports by Taruvinga (2014) that higher P concentrations in the soil tend to “lock” and reduce the absorption and utilization of some soil nutrients.
The significant interaction effects of PR × V and IR × V on K uptake could possibly be due to the interactive effects of P and rhizobium to positively influence K uptake in the shoot and root. This concurs with earlier reports that P fertilizers and rhizobium inoculant may enhance root development and induce an increase in number of root hairs, thereby favoring nutrient uptake by exploring a larger volume of soils (Rodelas et al., 1999; Biswas et al., 2000). However, the observed values for K at all levels were not within the sufficiency or optimal range (1.0–5.0%) as described by Motsara and Roy (2008).
This study has revealed a variable response of peanut varieties to P fertilizer rates and rhizobium inoculant on Haplic Lixisols of the Guinea savanna zone of Ghana. P fertilizer rates combined with peanut varieties had a significant influence on growth, effective nodule number, and podding capacity. Nkatie SARI appears to have the potential to increase peanut productivity in P-deficient soils as it combined with 60 kg P2O5/ha to promote the best growth, nodulation, and podding capacity.
Peanut varieties treated with rhizobium inoculant improved pod number, nodule number, Haulm weight, and HI, with Nkatie SARI combined with inoculant at 6 g/kg seed giving the best performance. The interaction of IR × PR was significant on peanut grain yield. Higher grain yields were recorded in peanuts sown with P fertilizer rate at 60 kg P2O5/ha in combination with inoculant at 6 g/kg seed. This points to the fact that limited use of P fertilizers and inoculants by farmers within the study location is among the factors that have affected peanut productivity. Our results show that the use of P fertilizer and rhizobium inoculant has the potential to increase peanut productivity in areas with similar soil characteristics. However, since the result between the on-station and on-farm experiments appeared inconsistent, further studies are required to determine if the yield from this interaction is stable enough to form the basis of recommendation of this treatment combination to small-scale peanut farmers.
The original contributions presented in the study are included in the article/supplementary material, further inquiries can be directed to the corresponding author/s.
AR and JK designed the experiment and protocol. RN, AR, JK, and AB performed the data collection. RN and AB analyzed the data and interpreted the results. RN did the literature searches. AB wrote the manuscript. All authors read and approved the submitted version.
The authors declare that the research was conducted in the absence of any commercial or financial relationships that could be construed as a potential conflict of interest.
The reviewer AK declared a past co-authorship with one of the authors AB to the handling Editor.
Collaborative staff, field, and data collection support from the Department of Agronomy, University for Development Studies, was greatly appreciated.
Adjei-Nsiah, S., Alabi, B. U., Ahiakpa, J. K., and Kanampiu, F. (2018). Response of grain legumes to phosphorus application in the Guinea Savanna agro-ecological zone of Ghana. Agron. J. 110, 1–8. doi: 10.2134/agronj2017.11.0667
Amoako, E. E., Misana, S., Kranjac-Bersavijevic, G., Zizinga, A., and Ballu Duweija, A. (2018). Effect of seasonal burning on tree species in the Guinea savanna wood land, Ghana: implications for climate change mitigation. Appl. Ecol. Environ. Res. 16, 1935–1949. doi: 10.15666/aeer/1602_19351949
Amos, A. O., Ogendo, M., and Joshua, O. (2001). Response of common bean to Rhizobium inoculation and fertilizer. J. Food Technol. 6, 121–125. doi: 10.4314/jfta.v6i4.19303
Anglade, J., Billen, G., and Garnier, J. (2015). Relationships for estimating N2 fixation in legumes: incidence for N balance of legume-based cropping systems in Europe. Ecosphere 6, 37–41. doi: 10.1890/ES14-00353.1
Asante, M., Ahiabor, B. D. K., and Atakora, W. K. (2020). Growth, nodulation and yield response of groundnut (Arachis hypogea L.) as influenced by combined application of Rhizobium Inoculant and phosphorus in the Guinea savanna zone of Ghana. Int. J. Agron. 2020, 2–7. doi: 10.1155/2020/8691757
Bekere, W., and Hailemariam, A. (2012). Influences of inoculation methods and phosphorus levels on nitrogen fixation attributes and yield of soybean (Glycine max L.) at Haru, Western Ethiopia. Am. J. Plant Nutr. Fertil. Technol. 2, 45–55. doi: 10.3923/ajpnft.2012.45.55
Biswas, J. C., Ladha, J. K., and Dazzo, F. B. (2000). Rhizobium inoculation improves nutrient uptake and growth of lowland rice. Pak. J. Bot. 164, 1644–1650. doi: 10.2136/sssaj2000.6451644x
Boddey, R. M., Fosu, M., Atakora, W. K., Miranda, C. H., Boddey, L. H., Guimaraes, A. P., et al. (2017). Cowpea (Vigna unguiculata) crops in Africa can respond to inoculation with rhizobium. Exp. Agric. 53, 578–587. doi: 10.1017/S0014479716000594
Bouyoucos, G. J. (1962). Hydrometer method improved for making particle size analysis of soils. Agron. J. 53, 464–465. doi: 10.2134/agronj1962.00021962005400050028x
Bray, R. H., and Kurtz, L. T. (1945). Determination of total organic and available forms of phosphorus in soil. Soil Sci. 599, 39–45. doi: 10.1097/00010694-194501000-00006
Bremner, J. M., and Muluvaney, C. S. (1982). “Nitrogen-total,” in Methods of Soil Analysis Part 2: Chemical and Microbiological Properties, 2nd Edn., eds A. L. Page, R. H. Miller, and D. R. Keeney (Madison, WI: American Society of Agronomy and Soil Science), 593–624.
Catroux, G., Hartmann, A., and Revellin, C. (2001). Trends in Rhizobia inoculants production and use. Plant Soil 230, 21–30. doi: 10.1023/A:1004777115628
Dakora, F. D., and Keya, S. O. (1997). Contribution of legume nitrogen fixation to sustainable agriculture in Sub-Saharan Africa. Soil Biol. Biochem. 29, 809–817. doi: 10.1016/S0038-0717(96)00225-8
Emmanuel, O. C., Akintola, O. A., Tetteh, F. M., and Babalola, O. O. (2021). Combined application of inoculant, phosphorus and potassium enhances cowpea yield in Savanna Soils. Agronomy 11:15. doi: 10.3390/agronomy11010015
FAO (2018). FAO Agricultural Statistics. Retrieved from: www.FAOSTAT.org (accessed April 7 2020).
Fatima, Z., Zia, M., and Chaudhary, M. F. (2007). Interactive effect of Rhizobium strains and P on soybean yield, nitrogen fixation and soil fertility. Pakis. J. Bot. 39:255.
Gentili, F., Wall, L. G., and Huss-Danelle, K. (2006). Effects of Phosphorus and Nitrogen on Nodulation are Seen Already at the Stage of Early Cortical Cell Divisions in Alnus incana, Annals of Botany, 98, 309–315. doi: 10.1093/aob/mcl109
Golakia, P. R., Makne, V. G., and Monpara, B. A. (2005). Heritable variation and association in Virginia runner and Spanish bunch group of groundnut. Natl. J. Plant Improv. 7, 50–53.
Hald, P. M. (1947). The flame photometer for the measurement of sodium and potassium in biological materials. J. Biol. Chem. 167, 499–510. doi: 10.1016/S0021-9258(17)31003-7
Hernanadez, M., and Cuevas, F. (2003). The effect of inoculating with Arbuscular mycorrhiza and Radyrhizobium strains on soybean (Glycine max (l.) Merill) crop development. Cultivos Trop. 24, 19–21.
International Union of Soil Sciences Working Group (2014). World Reference Base for Soil Resources 2014 International Soil Classification System for Naming Soils and Creating Legends for Soil Maps. World Soil Resource Reports 106. Rome: FAO.
Ishizawa, S., and Toyoda, H. (1955). Comparative study on effective and ineffective nodules of leguminous plants. Soil Sci. Plant Nutr. 1, 47–48. doi: 10.1080/00380768.1955.10434369
Kabir, R., Yeasmin, S., Mominul-Islam, A. K. M., and Sarkar, M. D. (2013). Effect of phosphorus, calcium and boron on the growth and yield of groundnut (Arachis hypogea L.). Int. J. Biosci. Biotechnol. 5, 51–60.
Kamara, A. Y., Ekeleme, F., Kwari, J. D., Omoigu, L. O., and Chiloye, D. (2011). Phosphorus effect on growth and yield of groundnut varieties in the tropical savannas of northeast Nigeria. J. Trop. Agric. 49, 25–30.
KIT (1984). Analytical Methods of the Service Laboratory for Soil, Plant and Water Analysis. Part 1: Methods of Soil Analysis. Amsterdam: Soils Laboratory Staff, Koninklijk Istituut voor de Tropen (Royal Tropical Institute).
Kolawole, G. O. (2012). Effect of phosphorus fertilizer application on the performance of maize/soybean intercrop in the southern Guinea savanna of Nigeria. Archiv. Agron. Soil Sci. 58, 189–198. doi: 10.1080/03650340.2010.512723
Konlan, S. (2010). Groundnut varietal response to spacing in the Guinea savannah and forest zones of Ghana (Ph.D. thesis), Kwame Nkrumah University of Science and Technology, Kumasi, Ghana.
Konlan, S., Sarkodie-Addo, J., Asare, E., Adu-Dappah, H., and Kombiok, M. J. (2013). Groundnut (Arachis hypogea) varietal response to spacing in the humid forest zone of Ghana. ARPN J. Agric. Biol. Sci. 8, 642–651.
Kumar, U., Singh, P., and Boote, K. J. (2012). Effect of climate change factors on processes of crop growth and development and yield of groundnut (Arachis hypogea). Adv. Agron. 166, 41–69. doi: 10.1016/B978-0-12-394277-7.00002-6
Kyei-Boahen, S., Savala, C. E. N., Chikoye, D., and Abaidoo, R. C. (2017). Growth and yield responses of cowpea to inoculation and phosphorus fertilization in different environments. Front. Plant Sci. 8:646. doi: 10.3389/fpls.2017.00646
Lira, A. M. Jr., Nascimento, S. R. L., and Fracetto, M. G. G. (2015). Legume-rhizobia signal exchange: promiscuity and environmental effects. Front. Microbial 6:945. doi: 10.3389/fmicb.2015.00945
Martinson, S. (2009). Growth and yield performance of four groundnut varieties in response to seed size (M.Sc. thesis), Kwame Nkrumah University of Science and Technology, Kumasi, Ghana.
Ministry of Food and Agriculture (2012). Ghana Agriculture Facts and Figures. Ghana: Ministry of Food and Agriculture.
Motsara, M. R., and Roy, R. N. (2008). Guide to Laboratory Establishment. New Delhi: FAO Fertilizer and Plant Nutrition Bulletin.
Mweetwa, M. A., Mulenga, M., Mulilo, X., Nyulube, M., Banda, K. S. J., Kapulu, N., et al. (2014). Response of cowpea, soybean and groundnuts to non-indigenous legume inoculants. Sustain. Agric. Res. 3, 84–88. doi: 10.5539/sar.v3n4p84
Myers, A. T., Dtal, R. S., and Borland, J. W. (1947). The flame photometerin soil and plant analysis. Soil. Sci. Soc. Am. Proc. 12, 127–130.
Ndakidemi, P. A., and Semoka, J. M. R. (2006). Soil fertility survey in western Usambara mountains, northern Tanzania. Pedosphere 1692, 237–244. doi: 10.1016/S1002-0160(06)60049-0
Nelson, D. W., and Sommers, L. W. (1982). “Total carbon, organic carbon and organic matter,” in Methods of Soil Analyses 2. Chemical and Microbial Properties, eds A. L. Page, R. H. Miller, and D. R. Keeney (Madison, WI: American Society of Agronomy and Soil Science Society of America), 301–312.
Nwaga, D., and Ngo Nkot, L. (1998). In vitro acid tolerance of rhizobia isolated from Vigna unguiculata in cameroon in comparison with Bradyrhizobium japonicum. Cahiers Agic. 407–410.
Panwar, J. D. S., and Naidu, V. S. G. R. (2002). Recent advances in physiology and genetics of nodulation and nitrogen fixation in cereals. Adv. Plant Physio. 4, 373–382.
Ramesh, R., Shanthamalliah, M. R., Jayadeva, A. M., and Hiermath, R. R. (1997). Seed yield and nutrient uptake of groundnut as influenced by sources of phosphate, FYM and phosphate solubilizing microorganisms. Curr. Res. Bangalore Univ. 26, 2002–2004.
Rodelas, B., Lithgow, J. K., Wisniewski-Dye, F., Hardman, A., Wilkinson, A., Economou, A., et al. (1999). Analysis of quorum-sensing-dependent control of rhizosphere-expressed (rhi) genes in Rhizonium leguminosarum bv. Viciae. J. Bacteriol. 181, 3816–3823 doi: 10.1128/JB.181.12.3816-3823.1999
Sela, G. (2020). Fertilization and Irrigation: Theory and Best Practices, 2020 Edn. Hod Hasharon: Cropaia.
Shiyam, J. O. (2010). Growth and yield response of groundnut (Archis hypogea L.) to plant densities and phosphorus on an ultisol in Southeastern Nigeria. Libyan Agric. Res. Centre J. Int. 1, 211–214. doi: 10.4314/naj.v40i1-2.55549
Stefanescu, M., and Palanciuc, V. (2000). Efficiency of bacterial inoculation and mineral nitrogen and phosphorus fertilization in rainfed soybean. Roman. Agric. Res. 13, 75–83.
Tang, C., Hisinger, P., Drewn, J., and Jaillard, B. (2001). Phosphorus deficiency impairs early nodule functioning and enhances proton release in roots of Medicago truncatuta L. Ann. Bot. 88, 131–138. doi: 10.1006/anbo.2001.1440
Taruvinga, B. (2014). Nutritional and soil fertility benefits: Influece of fertilizers on groundnut (Arachis hypogea L.) yield and soil nitrogen contribution for smallholder farmers in Uganda (Thesis), Wageningen University, Wageningen, Netherlands.
Uchida, R. (2000). “Essential nutrients for plant growth: nutrient functions and deficiency symptoms,” in Plant Nutrient Management in Hawaii's Soils, Approaches for Tropical and Sub-Tropical Agriculture, eds J. A. Silva and R. Uchida (Hawaii: College of Tropical Agriculture and Human Resource, University of Hawaii at Manoa), 31–55.
Ulzen, J., Abaidoo, R. C., Ewusi-Mensah, N., and Masso, C. (2018). On-farm evaluation and determination of sources of variability of soybean response to Bradyrhizobium inoculation and phosphorus fertilizer in northern Ghana. Agric. Ecosyst. Environ. 267, 23–32. doi: 10.1016/j.agee.2018.08.007
Keywords: peanut varieties, phosphorus, inoculant, nitrogen fixation, grain yield
Citation: Naabe Yaro R, Rufai Mahama A, Kugbe JX and Berdjour A (2021) Response of Peanut Varieties to Phosphorus and Rhizobium Inoculant Rates on Haplic Lixisols of Guinea Savanna Zone of Ghana. Front. Sustain. Food Syst. 5:616033. doi: 10.3389/fsufs.2021.616033
Received: 10 October 2020; Accepted: 03 May 2021;
Published: 11 June 2021.
Edited by:
Ole Mertz, University of Copenhagen, DenmarkReviewed by:
Gabriel da Silva Medina, University of Brasilia, BrazilCopyright © 2021 Naabe Yaro, Rufai Mahama, Kugbe and Berdjour. This is an open-access article distributed under the terms of the Creative Commons Attribution License (CC BY). The use, distribution or reproduction in other forums is permitted, provided the original author(s) and the copyright owner(s) are credited and that the original publication in this journal is cited, in accordance with accepted academic practice. No use, distribution or reproduction is permitted which does not comply with these terms.
*Correspondence: Albert Berdjour, YmVyZGpvdXJqdW5pb3JAZ21haWwub3Jn
Disclaimer: All claims expressed in this article are solely those of the authors and do not necessarily represent those of their affiliated organizations, or those of the publisher, the editors and the reviewers. Any product that may be evaluated in this article or claim that may be made by its manufacturer is not guaranteed or endorsed by the publisher.
Research integrity at Frontiers
Learn more about the work of our research integrity team to safeguard the quality of each article we publish.