- 1Department of Biochemistry, Microbiology and Biotechnology, Kenyatta University, Nairobi, Kenya
- 2Institute of Life Sciences, Scuola Superiore Sant'Anna, Pisa, Italy
- 3Department of Biological Sciences, Chuka University, Chuka, Kenya
Smallholder agroecosystems play a key role in the world's food security providing more than 50% of the food produced globally. These unique agroecosystems face a myriad of challenges and remain largely unsupported, yet they are thought to be a critical resource for feeding the projected increasing human population in the coming years. The new challenge to increase food production through agricultural intensification in shrinking per capita arable lands, dwindling world economies, and unpredictable climate change, has led to over-dependence on agrochemical inputs that are often costly and hazardous to both human and animal health and the environment. To ensure healthy crop production approaches, the search for alternative ecofriendly strategies that best fit to the smallholder systems have been proposed. The most common and widely accepted solution that has gained a lot of interest among researchers and smallholder farmers is the use of biological agents; mainly plant growth promoting microorganisms (PGPMs) that provide essential agroecosystem services within a holistic vision of enhancing farm productivity and environmental protection. PGPMs play critical roles in agroecological cycles fundamental for soil nutrient amelioration, crop nutrient improvement, plant tolerance to biotic and abiotic stresses, biocontrol of pests and diseases, and water uptake. This review explores different research strategies involving the use of beneficial microorganisms, within the unique context of smallholder agroecosystems, to promote sustainable maintenance of plant and soil health and enhance agroecosystem resilience against unpredictable climatic perturbations.
Introduction
Biological soil fertility restoration techniques within the smallholder agroecosystems, in combination with other agronomic management practices, would provide the much-needed solutions for revitalizing the declining global food production (Raimi et al., 2017). Beneficial soil microbiota such as plant growth promoting microorganisms (PGPMs), comprising of specific groups of bacteria and fungi, provide essential agroecosystem services that support plant growth (Rouphael and Colla, 2020) and ameliorates soil productivity (Santos et al., 2019). PGPMs maintain key agroecological cycles fundamental for soil nutrient enrichment, crop nutrient improvement, plant tolerance to biotic and abiotic stresses, biocontrol of pests and diseases, and water uptake enhancement (Lobo et al., 2019; Goswami and Deka, 2020). They are actively involved in healthy plant development and growth through secretion of hormonal growth regulators, and resistance induction against phytopathogens (Dakora et al., 2015). Besides, versatile PGPMs could be used to bioremediate polluted fields and increase the land available for production as in the case of heavy metals polluted soils (Gouda et al., 2018). These agroecosystem services are primarily important in supporting crop production in smallholder agroecosystems, which are characteristically defined by limited resource inputs.
PGPMs promote plant growth and productivity through various direct and indirect approaches. Several direct mechanisms have been established through previous studies and can be broadly classified into phytostimulants (Babalola and Glick, 2012), biofertilizers (Kalayu, 2019), rhizomediators, or stress regulators (Stamenković et al., 2018). Indirect mechanisms mainly occur in form of biocontrol of phytopathogens through competition for nutrients, enzymatic lysis, antibiosis (Köhl et al., 2019), secretion of volatile organic compounds (VOCs) (Sun and Tang, 2013), and triggering of antioxidative defense mechanism (Sandhya et al., 2010; Malik et al., 2020) and induced systemic resistance (ISR) response in the host plant (Heil and Bostock, 2002). PGPM biofertilizers promote plant growth by enhancing nutrient availability to the plants and the most studied pathways include N fixation (Ahemad and Kibret, 2014; Fukami et al., 2018b), P and K solubilization (Sharma et al., 2013; Soumare et al., 2020), S oxidation, Fe and C sequestration (Kannahi and Senbagam, 2014; Velivelli et al., 2014). PGPMs enhance the availability of P, K, Zn, Se, and Fe in the soil through biochemical processes such as solubilization, chelation, mineralization, oxidation and reduction reactions (Ahmed and Holmström, 2014; Velivelli et al., 2014; Rouphael and Colla, 2020). PGPMs are also known to secrete phytohormones such as auxins (Lin and Xu, 2013; Azizoglu, 2019), cytokinin, abscisic acid, ethylene, brassinosteroids, jasmonic acid, salicylic acid, strigolactones, and gibberellins (Goswami and Deka, 2020; Saad et al., 2020) that act as plant growth stimulators and stress controllers.
The PGPMs functionality and vigor, however, depend on intrinsic soil properties, environmental and agronomic management factors. Nutrient availability, soil pH, water, temperature, crop genotype, and cultural management are some of the key drivers determining the survival and function of PGPMs in the soil (Gouda et al., 2018; Gupta et al., 2019). For maximum farm benefits to be realized in a highly heterogenous smallholder systems (Njeru et al., 2020), tradeoffs in balancing the highlighted determinants have to be considered and appropriate farm management practices are ought to be carried out. For instance, the choice of crop species and diversity is critical in stimulating specific plant-microbe interactions and consequently the intended output (Orrell and Bennett, 2013; Saad et al., 2020). The authors suggest the use of multiple cropping systems that promote synergy and minimize the yield gap between the potential and realized production, a phenomenon that is commonly seen in smallholder systems. Additional methods efficient in augmenting indigenous soil PGPMs can be integrated to enhance microbial bio-functionality. These include the use of organic amendments such as farmyard manure and vermicompost (Mosa et al., 2018; Koskey et al., 2020), biotechnological approaches such as plant breeding (Bakker et al., 2012), crop management practices such as agroforestry, rotation, intercropping, cover cropping, and practicing reduced soil disturbance (Ventorino et al., 2012; Hontoria et al., 2019; Elagib and Al-Saidi, 2020).
The increasing demand among smallholder farmers to cut input costs and the need for sustainable nutrient management practices is driving the growing adoption and use of microbial-rich fertilizers in smallholder setups (Raimi et al., 2017). Commercial microbial inoculants (commonly used as biofertilizers or bioenhancers) containing single species or multiple strains of rhizobia, Pseudomonas spp., Azotobacter spp., Bacillus spp., Trichoderma spp., Aspergillus spp., and Glomus spp. (Figure 1) have been largely used in smallholder agroecosystems for crop production (Koskey et al., 2017; Bargaz et al., 2018; Adeyemi et al., 2019). Previous field researches carried out in different agroecosystems around the world have reported varying levels of successes on the use of PGPMs to support crop performance quantitatively and qualitatively (Pellegrino et al., 2012; Mishra et al., 2019; Saad et al., 2020). However, little has been done focusing on the use of PGPMs to address various challenges facing smallholder agroecosystems in the context of changing climatic conditions. Therefore, this review explores different available strategies involving the use of beneficial microorganisms as biofertilizers, within the unique context of smallholder agroecosystems, to promote sustainable maintenance of plant and soil health, and enhance agroecosystem resilience against unpredictable climatic perturbations.
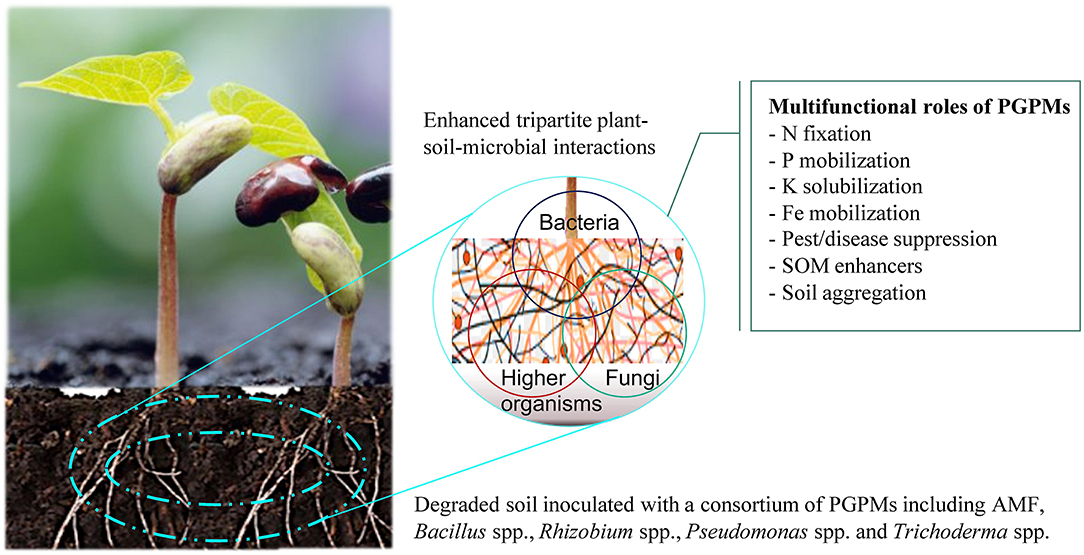
Figure 1. Plant growth promoting microorganisms (PGPMs) play a significant role in provision of beneficial ecosystem services in resource-limiting smallholder farming systems. Together with crops such as legumes (Phaseolus vulgaris L.), they form multifunctional interactions that enhance nutrient availability and uptake, pest and disease suppression, soil organic matter (SOM) accumulation and formation of soil aggregates, that collectively increase crop productivity.
Roles and Challenges of Smallholder Agroecosystems in Global Food Security
Eradicating hunger, poverty, and food insecurity while ensuring sustainable use of natural resources for agriculture, as highlighted in the United Nations Sustainable Development Goals (SDGs), is paramount in a world faced with a myriad of economic, social, political, and environmental challenges (Pérez-Escamilla, 2017). Globally, ~700 million people have no access to sufficient food while about 2 billion people face nutritional deficiencies, of which about 50% of the food insecure individuals are from Asia, 35% from Africa, and 10% from Latin America (FAO, 2018). This is likely to increase due to the current global health and economic crisis due to the Coronavirus disease 2019 (Covid-19) pandemic. Smallholder agroecosystems, predominantly found in developing countries, are considered critical food security resources that will support food production for the increasing human population in the coming years. Currently, it is estimated that smallholder agroecosystems account for more than 50% of the food produced globally (Herrero et al., 2017). In Africa, they contribute about 75% of the total crop production and 50% of the animal products (Nyambo et al., 2019) and, thus, are significantly involved in rural poverty reduction, economic development, and food security. However, compared to large-scale profit-driven systems, smallholder agroecosystems have limited land size, stringent financial resources, low market sharing, and product range, thus, are faced with more risks and vulnerabilities (Kuivanen et al., 2016; Herrero et al., 2017).
The productivity of smallholder agroecosystems largely depends on the services naturally provided by the ecosystem such as soil fertility, nutrient cycling, water availability, pest control, and pollination (Altieri et al., 2012). Farmers' decision and selection of their appropriate agronomic management practices affect the extent of agroecosystem functioning. External pressures such as poverty, unreliable climatic conditions, and farmer's need for immediate satisfaction exert pressure on land use and cause negative impacts on the ecosystem (IFAD and UNEP, 2013). Agricultural intensification coupled with the use of harmful agrochemical inputs has negatively impacted on smallholder agroecosystems (Bationo et al., 2012). Their long-term sustainability in the face of new challenges such as the shrinking per capita arable lands, emerging diseases, dwindling world economies, and unpredictable climate change, is on the balance. Notwithstanding their benefits, economic and policy marginalization, low investment support, and the increasing land fragmentation of small farms threaten their contribution to global food security, leaving many farmers vulnerable (IFAD and UNEP, 2013). The rising environmental awareness, depletion of natural resources, and human health nutritional concerns have led to a paradigm shift among the farmers from over-dependence on agrochemical inputs to the use of ecofriendly biological agents for agricultural production (Herrero et al., 2017; Alori and Babalola, 2018). To increase the use and adoption of biological agents in smallholder agroecosystems, more robust integrated pathways that encourage food production based on local innovations, practices, and resources should be established. Tapsoba et al. (2020) emphasize that smallholder farmers should be involved in re-designing agricultural production. This way, farmers are likely to integrate new techniques into their current farm management practices to meet their agrosociological and economic needs. The authors warn that some agricultural initiatives may remain localized and isolated despite evidence of success in other agroecosystems. It is, therefore, necessary to have the right network of stakeholders on a territorial scale to support agricultural initiatives and their implementation.
Use of PGPM Inoculants in Enhancing the Productivity of Smallholder Agroecosystems; Opportunities and Challenges
Use of Nitrogen Fixing PGPMs as Biofertilizers
Nitrogen (N) is one of the essential elements required by plants for proper growth, development, and productivity, and plays a pivotal role in various structural, biochemical, and physiological processes (Giller et al., 2019). Therefore, to achieve good crop productivity and quality, N application in form of nitrogenous-based fertilizers or amendments is inevitable. The production of inorganic N fertilizer through the Haber-Bosch industrial chemical process revolutionized agriculture and significantly increased crop production. However, there are serious human health, economic, and environmental concerns raised on the excessive and continuous use of chemically derived inorganic N fertilizers (Reddy and Saravanan, 2013), and hence the introduction of N biofertilizer formulations as a viable and sustainable alternative. Biological nitrogen fixation (BNF) is a process that naturally involves legumes and rhizobia symbionts, and/or plants and a group of free-living PGPMs known as diazotrophs (Giller et al., 2019). Through the BNF, inert atmospheric N2 gas is converted via a series of enzymatically regulated complex reaction mechanisms into N containing organic compounds utilizable by the plants (Gupta et al., 2019). In symbiotic association, nodule forming rhizobia produces nitrogenase enzyme complex in the presence of leghemoglobin molecules and convert N2 into ammonium and nitrate ions which are readily absorbed by the plants. In return, plant hosts the bacteria inside the root nodules and provide photosynthates such as C that rhizobia uses as an energy source (Wang et al., 2013; Choudhary and Varma, 2017).
In smallholder farming systems, the use of microbial inoculants containing diazotrophs and symbiotic PGPMs is on the rise. Major groups of N-fixing bacteria commonly used include Rhizobium spp., Azorhizobium spp., Mesorhizobium spp., Bradyrhizobium spp., Thiobacillus spp., Azospirillum spp., Sinorhizobium spp., Clostridium spp., Azotobacter spp., Cyanobacteria, and Frankia spp. (Yeager et al., 2005; Mus et al., 2018; Raimi et al., 2019). It has been demonstrated that inoculating legumes with a single or a consortium of N-fixing bacteria improves soil fertility, plant growth, yield, and nutrition quality (Kawaka et al., 2014; Mabrouk et al., 2018; Menge et al., 2018). Inoculation also enhances root development, nodulation, water stress tolerance, and suppresses pathogenic infestation (Koskey et al., 2017; Alori and Babalola, 2018; Musyoka et al., 2020). Private sectors, research institutions and universities have partnered with smallholder farmers in delivering efficient inoculants. For instance, N2-Africa, a multi-stakeholder project, actively researched on N-fixing rhizobia strains and developed inoculants for use by African smallholder farmers in the production of soybean, common bean, chickpea, ground nut, and faba bean (Giller et al., 2019). In Kenya, the University of Nairobi in collaboration with the Microbiological Resources Center Network (MIRCEN) partnered with MEA Fertilizer Ltd to produce Biofix®, a cheap Rhizobium based bioinoculant for use in the cultivation of legumes (Odame, 1997). In South Africa, BioControl Products SA (Pty) Ltd produces Azospirillum based N-fixing bioinoculants such as Azo-N® and Azo-N Plus® for cultivating grain and cover crop legumes (Raimi et al., 2017). Currently, more bacterial species are being identified for use as potential N-fixing bioinoculants (Ouma et al., 2016; Koskey et al., 2018; Gabasawa, 2020; Musyoka et al., 2020). Most of these trials have shown promising results under greenhouse-controlled conditions. Repeated field trials should be done to ascertain their performance under different ecological conditions of smallholder agroecosystems.
Generally, BNF can supply more than half of the plant N needs and can significantly reduce the use and overdependence on external chemical N fertilizers in agriculture (Bado et al., 2018). For instance, in Australia, diazotrophic N-fixation is estimated to provide the annual N demand of 20–80 kg N ha−1 year−1 for perennial grasses (Gupta et al., 2019). In Ghana, symbiotic N fixation is estimated to provide up to 16–145 kg N ha−1 year−1 for legumes (Kermah et al., 2018). Therefore, BNF could reduce substantially the use of additional basal or top-dresser inorganic N fertilizers and thus cutting the input cost for the smallholder farmers. BNF contribution and N quantification within smallholder agroecosystems, however, remains poorly understood due to the high cost of resources and technical expertise needed, and difficulty to implement at the grassroot level (Mhango et al., 2017; Bado et al., 2018). Despite a large and diverse genetic pool of N-fixing bacteria (Giller et al., 2019) and legume species suitable for different African agroclimatic conditions (Kebede, 2020), their utilization in promoting soil fertility and plant growth has not been achieved. Hence, new sustainable methods that are affordable, simpler, rapid, and easier to implement in smallholder setups should be developed to fill the aforementioned gap.
Use of Nutrient (P, K, Fe) Solubilizing and Mobilizing Microorganisms as Biofertilizers
Soil nutrients such as phosphorus (P), potassium (K), and iron (Fe) often limit plant growth and development because of their low solubility in the soil (Giovannini et al., 2020). They are firmly fixed and are not readily available for plant uptake, and their shortage could be detrimental to healthy growth and physiological development of the plant (Parani and Saha, 2012). Smallholder farmers rely on the external application of inorganic P-fertilizers whose efficiency declines in the presence of too much rainfall. Granular P-inorganic fertilizers precipitate to form metal-cation complexes in rainy tropical ecosystems and thus become unavailable for plant use (Dissanayaka et al., 2018). Most soils of East and West Africa experience N, P, and K deficiency (Bationo et al., 2012). Therefore, the use of low-cost P and K solubilizing and mobilizing microorganisms that take part in P and K geo-cycles would be of paramount importance to alleviate soil nutrient deficiency and losses. They mineralize organic P and K through a series of complex enzymatic and hydrolytic reactions (Thakur et al., 2014) and also secrete organic acids such as gluconic, lactic, and oxalic acids that hydrolyze inorganic P compounds found in the soil (Sharma et al., 2013). Figure 2 shows the effect of maize (Zea mays L.) and cowpea [Vigna unguiculata (L.) Walp.] inoculation with P solubilizing bacteria (PSB) under controlled greenhouse conditions. There was an improved growth of maize and cowpea plants inoculated with PSB compared to the un-inoculated controls. Similarly, field studies have reported an enhanced growth, yield and improved nutritional values on crops inoculated with PSBs (Kalayu, 2019; Soumare et al., 2020). The most commonly used P and K solubilizing microorganisms (PSMs) include bacteria such as Pseudomonas spp., Enterobacter spp., Burkholderia spp., and Bacillus spp., and fungi such as Penicillium spp., Trichoderma spp., and Aspergillus spp. (Aseri et al., 2009; Sangeeth et al., 2012; Selvi et al., 2017). Although these studies evidently show increase in P solubilization when a single or a combination of bacteria species are used, the mechanism of action leading to synergism in delivering P to the plants remains unclear.
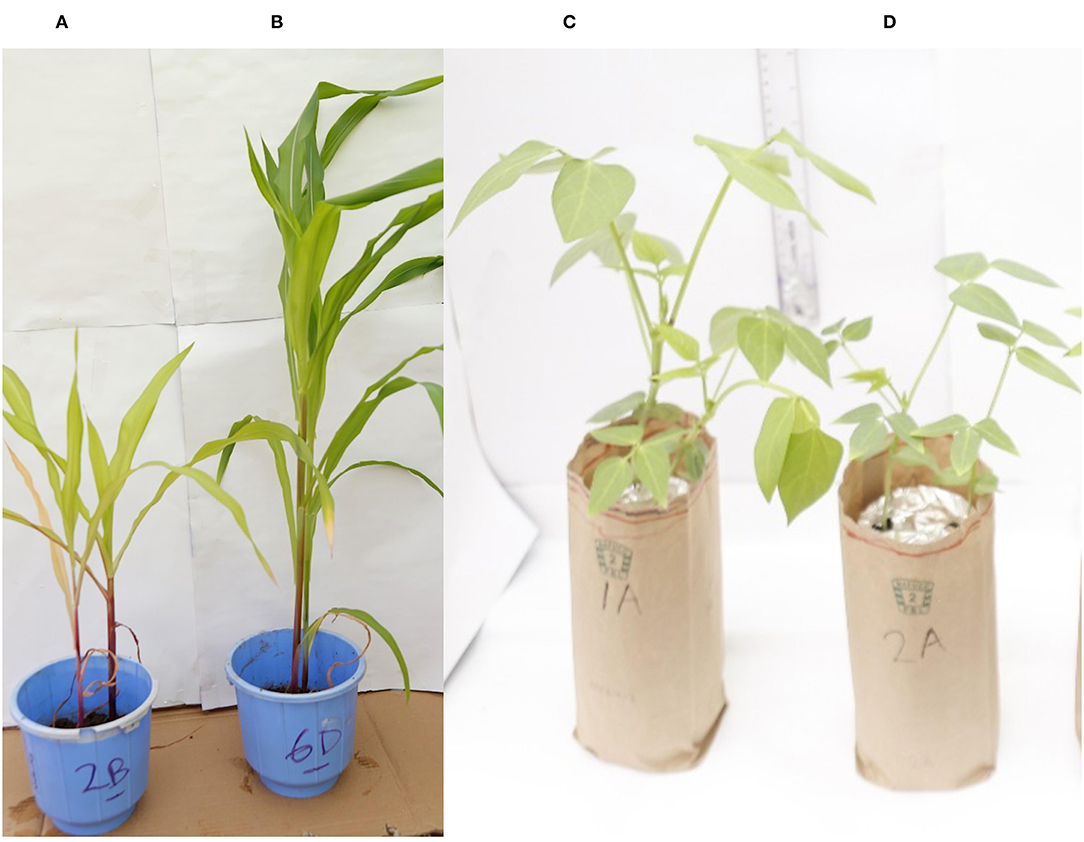
Figure 2. Application of phosphate solubilizing bacteria (PSB) enhances P availability without disturbing the soil biochemical composition, improves plant growth, photosynthetic activity, crop nutritional values, and yield. The photo shows a greenhouse experiment carried out by the Kenyatta University FLAIR research students on the effect of PSB inoculation on Zea mays L. and Vigna unguiculata (L.) Walp. (A) Un-inoculated Zea mays L. plant, (B) PSB inoculated Zea mays. L plant, (C) PSB inoculated Vigna unguiculata (L.) Walp, and (D) Un-inoculated Vigna unguiculata (L.) Walp. Inoculated plants showed improved growth and enhanced photosynthetic activity.
Arbuscular mycorrhizal fungi (AMF) are also well-known for P mobilization and solubilization and this has led to the development of mycorrhizal inoculants (Tabassum et al., 2017). AMF colonize the roots of nearly 90% of the terrestrial plants and increase the plant root surface area for the absorption of nutrients and water. Primarily, AMF actively participates in P and K mobilization and solubilization and this has been demonstrated both in the greenhouse and in the field with various crops including cereals, legumes, vegetables, fruits and trees (Wu et al., 2005; Njeru et al., 2017; Avio et al., 2018). The most commonly used AMF inoculants include Funneliformis mosseae, Glomus etunicatum, and Rhizophagus irregularis (Giovannini et al., 2020; Musyoka et al., 2020). Iron starvation in the soil causes a specific group of plants and soil microbes to secrete siderophores, iron specific chelating molecules, that play a vital role in iron transportation and regulating its bioavailability (Novo et al., 2018). Multifunctional biofertilizers containing Bacillus subtilis, Pseudomonas aeruginosa, Penicillium chrysogenum, and Streptomyces griseus not only secrete ferric ion-specific chelating biomolecules but also stimulate antagonistic actions against rhizosphere pathogens (Ahmed and Holmström, 2014; Kannahi and Senbagam, 2014).
In Kenya, smallholder farmers utilize Rhizatech® inoculant (Table 1) containing a combination of three AMF species produced and distributed by Dudu Tech Ltd company (Faye et al., 2020). In South Africa, Mycoroot (Pty) Ltd produces a number of Mycoroot® branded AMF inoculants that solubilize P, K, Cu, Zn, and Fe, and improve plant tolerance against abiotic stresses. Organo® biofertilizer produced by Amka Products (Pty) Ltd, South Africa, contains Bacillus spp., Enterobacter spp., Pseudomonas spp., Stenotromonas spp., and Rhizobium spp (Raimi et al., 2017). These PGPMs secrete siderophores and multifunctional plant growth promoting hormones such as gibberellin, IAA, and cytokinin (Saad et al., 2020). Pseudomonas fluorescens, produced by BioControl Products SA (Pty) Ltd as NAT-P®, and Bacillus subtilis produced by Ag-Chem Africa SA (Pty) Ltd as B-RUS®, are some of the commonly used multifunctional inoculants available for farmers (Raimi et al., 2017). In addition to P-K-solubilization, they produce siderophores that bind iron (Fe3+) suppressing its availability to phytopathogens, and indole acetic acid (IAA) responsible for stimulating root growth and plant cell elongation (Parani and Saha, 2012).
Despite their novel potential use as agro-inputs, various biotic and abiotic factors may mask the performance of several upcoming commercial bioinoculants in delivering nutrients to the plant (Wahid et al., 2020). Comparative studies on their performance in various smallholder agroecosystems characterized by varying soil typologies should be assessed. Recent studies have suggested that a second generation of multi-trait bioinoculants should be developed based on specific biostimulatory synergism of different PGPMs (Rouphael and Colla, 2020). However, little work has been done to adequately understand the synergistic role of AMF, P-solubilizing bacteria, and siderophore producers and how smallholder farming conditions affect their functional roles. Without scientific experiments to answer these considerations, the economic benefits in the use of bioinoculants in smallholder setups will remain elusive.
PGPMs in Enhancing the Adaptation of Crops to Abiotic Stresses
Crop production in rain-fed smallholder agroecosystems is mainly limited by various abiotic stresses that interfere with the genetic regulation of key cellular pathways in plants and severely affect the plant's physiological functioning and morphology (Sindhu et al., 2020). High temperatures, water stress, salinity, and floods are some of the important abiotic plant stressors experienced by smallholder farmers in SSA and may cause up to 70% of crop yield losses (Bationo et al., 2012). As the average global temperatures increase, the risk of widespread desertification heightens and this could traverse across many developing nations, hitting on the majority of the vulnerable smallholder farmers the hardest. The search for new plant breeds that could cope up with the stressors is a long-drawn and costly process considering the unique crop diversity of smallholder agroecosystems. Exploiting the unique environment-tolerant properties of microorganisms, their huge genetic diversity, and interaction with various plants could be crucial in addressing the management of abiotic stress in agriculture (Grover et al., 2011).
Agroforestry is an important practice central to climate change mitigation, soil and water conservation, energy and food sources. There is evidence that farmer-managed agroforestry is responsible for the significant increase in food production, tree diversity, and the greening trends in the Sahel region of Senegal, Niger, and Mali (Elagib and Al-Saidi, 2020). The choice of tree species used in agroforestry depends on various environmental, social, and economic factors. Recently, many development programs in collaboration with the local farmers of the Sahel region have engaged to combat the rising desertification through the use of leguminous trees such as acacia (Sileshi et al., 2020). Legumes such as Acacia seyal, A. senegal, and A. albida have successfully demonstrated their ability to interact with the indigenous AMF and rhizobia species of the Sahel region of West Africa and are considered potential agents for carbon sequestration and land restoration in the region (Fofana et al., 2020). Based on these and other evidence, integration of biofertilization and optimization of agroforestry techniques in the context of an integrated fight against desertification should be considered. The search for potential microbial candidates for ameliorating various abiotic stresses, restoring soil fertility, and enhancing crop productivity should be done in areas vulnerable to the effects of climate change (Goswami and Deka, 2020).
PGPMs exhibiting growth-promoting and stress-tolerant traits such as secretion of volatile organic compounds (VOCs), osmoprotectants (proline, glutamate, trehalose), siderophores, gibberellic and IAA production, P-solubilization, and exopolysaccharides (EPS) (Table 2) could be ideal for use in dryland agroecosystems (Grover et al., 2011; Gouda et al., 2018). For instance, in semiarid regions of India, EPS-producing drought-tolerant strains of Pseudomonas spp. with various plant-growth inducing traits, osmoregulation, and antioxidant properties on maize have been identified (Sandhya et al., 2010). EPS cement and stabilizes soil aggregates together creating a biofilm that increases water retention and regulates nutrient and water flow within the plant roots (Grover et al., 2011). In Brazil, an impressive soybean yield enhancement and increased tolerance to water stress have been reported through co-inoculation with Azospirillum brasilense and Bradyrhizobium japonicum (Hungria et al., 2015). PGPMs such as Pseudomonas spp., Burkholderia spp., Funneliformis mosseae, Enterobacter spp., and Rhizophagus irregularis stimulate osmolyte regulation mechanisms that control plant cell wall integrity and induce plant tolerance to water and salinity stresses (Agami et al., 2016; Gouda et al., 2018).
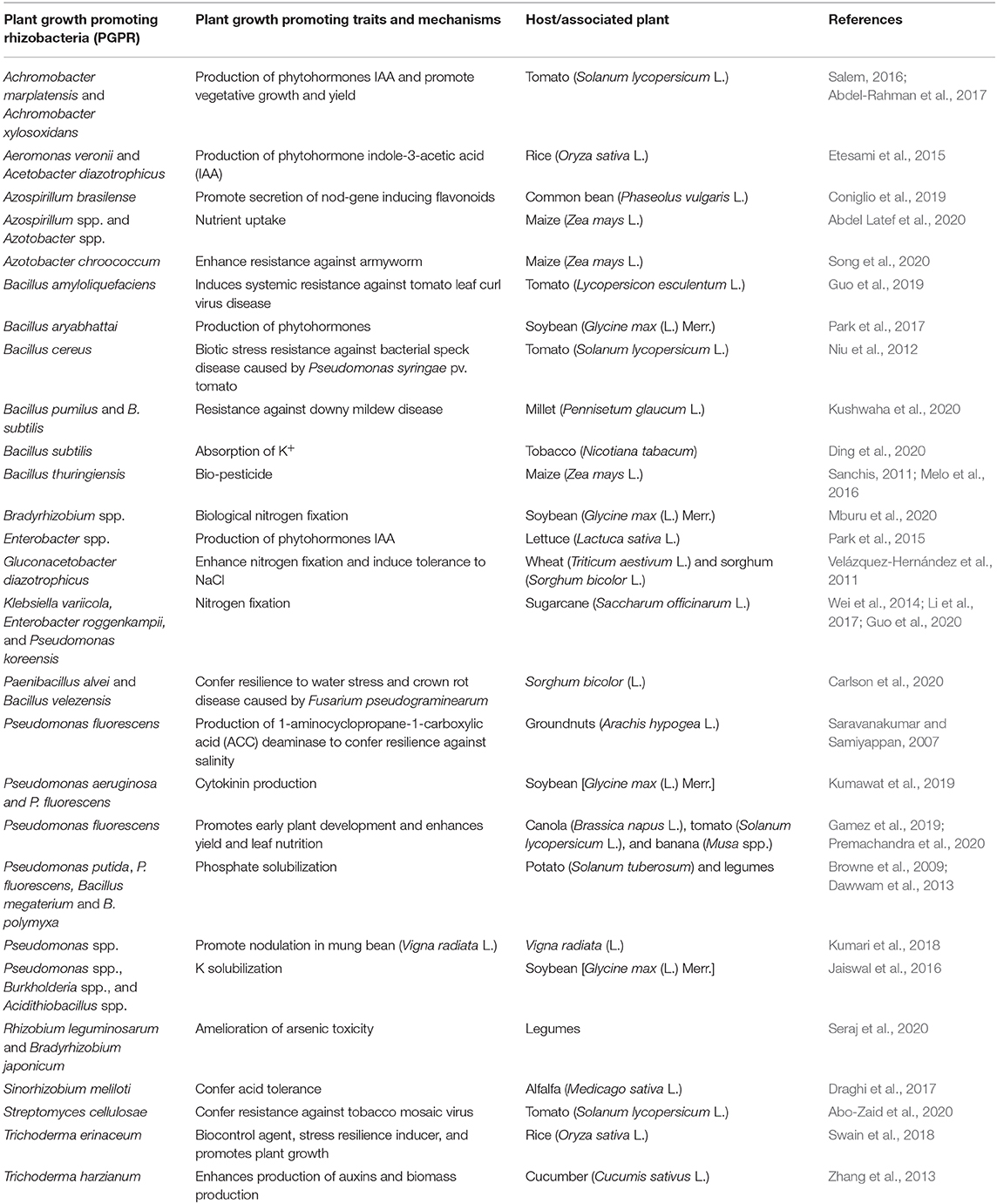
Table 2. Plant growth promoting rhizobacteria (PGPR) and their plant growth promoting traits and mechanisms.
High soil salinity, caused by excessive water evaporation and accumulation of chloride salts such as NaCl and MgCl2, negatively affect soil microbial biomass, seed germination, and plant development via osmotic potential or ion-specific damage mechanisms (Sindhu et al., 2020). In Uganda, smallholder farmers use Symbion vam plus® biofertilizer produced by T. Stanes and Company Ltd, and contains Bacillus megaterium, Glomus spp., and Gigaspora spp. that improve salinity tolerance and bioavailability of nutrients such as Fe, Cu, Zn, and P (Mukhongo et al., 2016). Gururani et al. (2013) reported increased potato (Solanum tuberosum) tuberization, enhanced tolerance to salt, drought and heavy metal stresses upon inoculation with two Bacillus spp. that induce changes in the expression of reactive oxygen species (ROS), scavenging enzymes, and proline content. Oxidative stress is commonly associated with drought, salinity and high-temperature conditions and also during plant-pathogen interaction. Fukami et al. (2018a) reported an enhanced induced systemic tolerance (IST) on maize against salinity stress following co-inoculation with Azospirillum brasilense and Rhizobium tropici that significantly affected antioxidant enzymes and proline content in the leaves.
The area of multi-microbial combinations to equip plants with abiotic stress tolerance is still equivocal and involves many genes of which some have not been identified yet. Thus, further research at the gene regulation level should elucidate the superior performance or lack of additive or synergistic effects that are observed when a combination of certain PGPMs are applied in the field (Ouma et al., 2016; Njeru et al., 2017). Understanding the complex plant-microbe interactions, stress tolerance, response, and adaptation as influenced by the changing soil and environmental factors will be important. Advanced biotechnological tools for identifying the potential microbial candidates with abiotic stress-tolerant properties should be employed and tested for their efficiency under different smallholder agroecosystems.
Use of Microorganisms in Suppression of Pests and Diseases
Evidence-based concerns against the use of synthetic chemical pesticides are increasingly pushing for the need to develop environmentally friendly pest and disease management strategies. Teratogenic and carcinogenic effects of chemical pesticides have been well-documented (Nicolopoulou-Stamati et al., 2016; Bonner and Alavanja, 2017). Notwithstanding the injurious effects, the development of synthetic pesticides is a complex process and requires rigorous regulatory approval demands. Besides, they are often costly and beyond the reach of the resource-strained smallholder farmers that contribute immensely to global food security (Mburu et al., 2016; Constantine et al., 2020). In smallholder agroecosystems, pests and diseases cause agricultural losses ranging from 45 to 100% (McDonald and Stukenbrock, 2016), depending on the infestation level. Fall armyworm alone can potentially cause losses of up to 13 billion USD in the smallholding family units of Sub-Saharan Africa (Harrison et al., 2019). Other than significantly limiting yields, most fungal pathogens are well-known producers of mycotoxins that negatively impact human health (Zhou et al., 2018). Development of alternative green technologies in pest and pathogen control is a need of the hour that should be fast-tracked to boost crop production that could feed the growing human population with minimalist disturbance to the already shrunk natural ecosystems. Microorganisms have been widely used as biological control agents (BCAs) for a long time and have been established to antagonize and suppress destructive entomopathogens in several ways (Köhl et al., 2019).
Most bacterial BCAs are of the genus Bacillus, with Bacillus thuringiensis being the most widely used bacterial biocontrol agent against common fungal pathogens and insects. Its derivatives are found in over 70% of bacterial biopesticides (Melo et al., 2016; Liu et al., 2019). Agrobacterium, Arthrobacter, Burkholderia, Azotobacter, Rhizobium, Serratia, Thiobacillus, and Pseudomonas are other bacteria genera with antibiotic attributes in-vitro and in-vivo (Saxena et al., 2000; Raaijmakers et al., 2002). Bacterial biopesticides, like most BCAs, are environmentally friendly and are inexpensive to develop and can be as effective as synthetic pesticides (McDonald and Stukenbrock, 2016; Köhl et al., 2019). Pseudomonas fluorescens has been established to be as effective in controlling the root decay agent Aphanomyces cochlioides in sugar beet as the commercial fungicides (Kristek et al., 2006). Most fungal BCAs are of the genera Aspergillus, Penicillium, Beauveria, Metarhizium, and Trichoderma (Abbey et al., 2019; McGuire and Northfield, 2020). Trichoderma species have been extensively studied for their antagonism against common soil-borne pathogens such as Rhizoctonia and Fusarium (Haldar and Sengupta, 2015; Köhl et al., 2019). Most destructive arthro-pests have also been successfully suppressed in smallholder agroecosystems through the use of Steinernema and Heterorhabditis nematodes (Arthurs and Heinz, 2006), baculoviruses and protozoa, such as Nosema (Sarwar, 2015; Hatting et al., 2019). BCAs act against pests and pathogens in several established ways, which can be direct or indirect. Understanding the modes of action of BCAs are integral in determining their efficacy in field conditions since in-vitro antagonism is not often reflected in-vivo (Köhl et al., 2019).
Direct mechanisms of antagonism involve parasitism, antibiosis, and predation (Figure 3). Mycoparasitism is the primary mode of action of most strains of Trichoderma and Clonostachys spp. against fungal pathogens (Abbey et al., 2019). Bdellovibrio bacteriovorus is a biocontrol agent unique in its ability to invade and derive nutrients from the cytoplasmic contents of other pathogenic gram-negative bacteria (McNeely et al., 2017). The direct antagonistic mechanisms of microbial BCAs are aided by the agents' ability to secrete cell wall degrading enzymes; chitinases, proteases, cellulases, glucanases, esterases, and catalases (Alori and Babalola, 2018). These hydrolytic enzymes facilitate the penetration of pathogen's cell wall and pest's tissues. Bacillus thuringiensis, the prime entomopathogenic bacteria, produces endotoxins that disrupt insect cell structures, inducing osmotic cell lysis that causes significant ion leakage and functional integrity loss (Melo et al., 2016; Azizoglu, 2019). Steinernema and Heterorhabditis nematodes secrete lytic enzymes that enable them to invade and release bacteria into the insect's haemocoel (Arthurs and Heinz, 2006). The infected insects consequently die of septicaemia. Trichoderma asperellum, Trichoderma virens, Trichoderma atroviride, and Trichoderma harzianum, are well-known to possess a high level of chitinolytic activity against common soil-borne pathogens such as Fusarium, Aspergillus, Rhizoctonia, and Puccinia (Panwar et al., 2014; Abbey et al., 2019). At humidity of at least 60%, BCA Ampelomyces germinates its pycnidia on host surfaces and penetrates powdery mildew hyphae resulting in cytoplasm degeneration (Kiss, 2008).
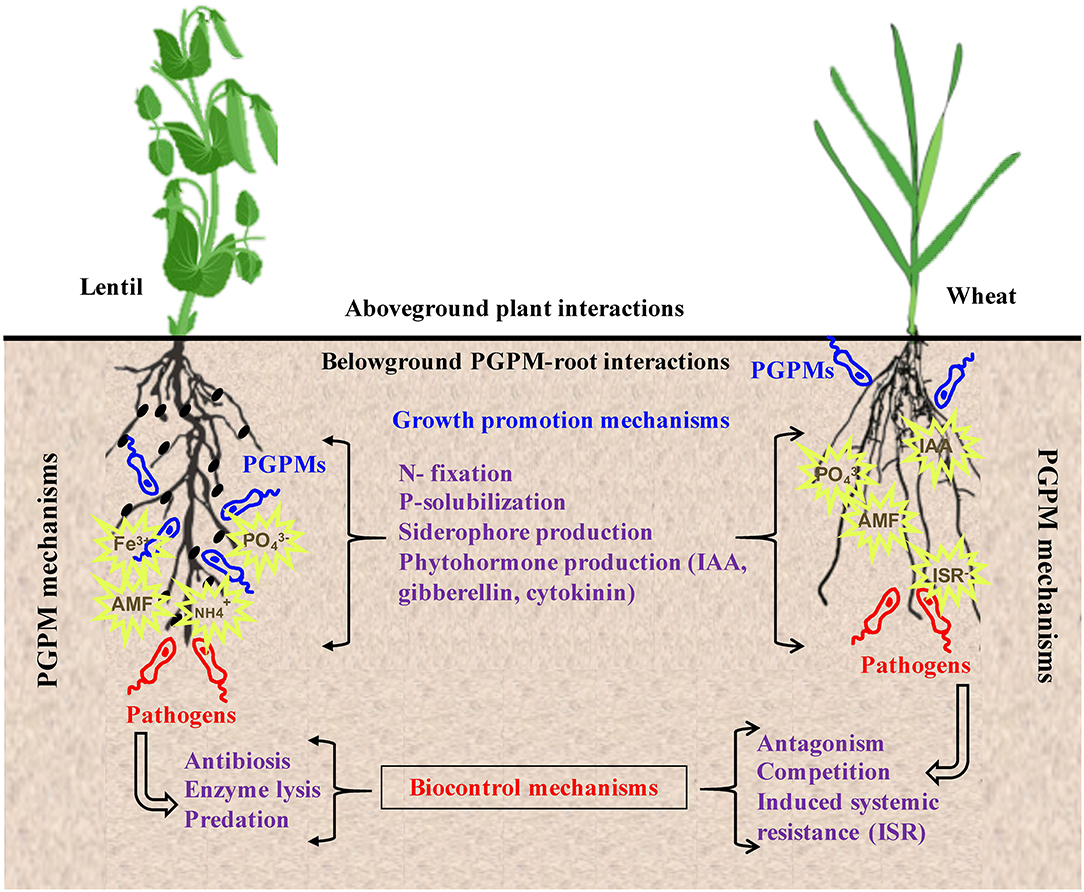
Figure 3. PGPMs are considered models of biotechnological interest in agronomy. Their positive effects on crops can be direct as in the case of stimulating plant growth by enhancing nutrient acquisition via mechanisms such as N fixation, P solubilization, siderophore production, and secretion of phytohormones. Indirect methods are mainly in form of biocontrol of plant pest and diseases which is achieved through antibiosis, antagonism, competition, predation, enzymatic lysis, and induction of plant systemic resistance.
Other than parasitism, most BCAs also suppress pathogens directly through the production of antibiotic compounds that impede the proliferation of the target pathogens. Bassiacridin and beauvericin produced by Beauveria have insecticidal property against entomopathogens (McGuire and Northfield, 2020); bioactive lipopeptides produced by Bacillus subtilis necrotizes insect epithelial cells causing death (Melo et al., 2016; Liu et al., 2019). Microbial antagonists also suppress pathogens through indirect mechanisms, notably through competition and induced systemic resistance (Heil and Bostock, 2002). Most antagonist microbes possess aggressive colonization ability suppressing the pathogens hindering their establishment through competition. This mode of action is incredibly effective in controlling necrotrophic pathogens that require exogenous nutrients for their establishment (Tewari et al., 2019). Trichoderma strains can produce siderophores and out-compete pathogens for iron, which is essential for the pathogens' normal physiology (Ahmed and Holmström, 2014). Some secondary metabolites produced by Trichoderma strains are associated with systemic resistance in Lycopersicon esculentum against Leptosphaeria macularis and Botrytis cinerea (Abbey et al., 2019). It is imperative to note that the antagonistic mechanisms are complex, and microbial BCAs may suppress a pathogen through several mechanisms. Synergistic effects of co-inoculation of certain BCAs have led to higher efficacy in field conditions (Köhl et al., 2019), which can be explored further in smallholder agroecosystems for more significant results.
Microbial Functional Identity vs. Functional Diversity in Delivering Agroecosystem Services
Soil microbial communities mediate nearly every biogeochemical process occurring on earth crust controlling the functionality of an ecosystem (Escalas et al., 2019). Their ubiquitous nature, diversity richness, and ability to establish multiple interactions with higher organisms and among themselves, make them the best candidates in delivery of essential agroecosystem services (Brussaard et al., 2007). Understanding the importance of having a specific functional species or group of PGPMs here referred to as “functional identity” or “functional diversity,” respectively (definitions adapted from Barberi, 2015) is not well-established in smallholder agroecosystems. This drives the rising research demand for soil biodiversity in the quest for the delivery of beneficial agroecosystem services. In the previous decades, most of the commercial inoculants contained a single microbial species or strain targeting a specific crop genotype (Kaminsky et al., 2019). Economically, this no longer favors the smallholder agroecosystems that are nowadays characterized by a wide range of crop production.
Currently, through research, various microbial species and strain combinations have been produced targeting a broad range of crop species depending on the market requirement and species compatibility. For instance, SumaGrow® bioinoculant (Table 1) produced by Bio Soil Enhancers Inc. (USA) contains a consortium of polyfunctional PGPMs comprising of N fixers, P mobilizers and solubilizers, micronutrient mobilizers, growth hormones, and organic humic acid, and enhance growth and yield in a wide range of crops including vegetables, cereals, legumes, trees, and fruits (Preininger et al., 2018). Co-inoculation or mixed inoculation of diverse multifunctional microbial groups as a single inoculant could maximize the chances of strain functional performance (Escalas et al., 2019) in particular the functional biodiversity effect when functional identity effect is suppressed. For instance, a mixture of PGPMs that enhance P solubilization (e.g., Bacillus spp.), phytopathogenic biocontrol (e.g., Pseudomonas spp.), BNF (e.g., Rhizobium spp.), and phytohormone production (e.g., Azospirillum spp.) could have synergistic or additive functional biodiversity effect on crops (Hungria et al., 2015; Rashid et al., 2016). However, the performance of co-inoculation is not always the case as its efficiency is affected by several factors including strain compatibility, concentration ratios, inoculation methods, plant genotypes, soil factors, and environmental conditions at the time of application (Kaminsky et al., 2019). Considering all these factors and the changing climatic conditions, the performance of the current inoculants may not be guaranteed in the near future. Thus, research should be done to deepen the understanding of complex interactions associated with mixing various inoculants to come up with new formulations for use with different crops. Efficient delivery methods of inoculant application in the context of the changing climate should also be investigated.
PGPMs Contribution to Crop Yield and Nutritional Quality
Studies have shown that there are significant benefits of using PGPM inoculants as biofertilizers or biostimulants in crop production (Chavoshi et al., 2018; Aliyu et al., 2019; Santos et al., 2019). A global meta-analysis of biofertilizer efficiency in enhancing crop nutrients and yields showed an average of 16 % increase in yield of all crops, with legumes showing a superior response to inoculation (Schütz et al., 2018). The beneficial effects of bioinoculation could be more in nutrient-limited soil conditions which reflects the case of SSA smallholder agroecosystems. According to Singh et al. (2017), Rhizobium biofertilizer inoculum when applied in semiarid and arid environments can supplement nutrient requirement in legumes and hence improving crop yield. Chavoshi et al. (2018) reported a maximum biomass accumulation of 7,985 kg ha−1 in red beans and a higher water use efficiency after inoculation with Bio-P and Bio-K fertilizers in limited water resources. In desert areas of the Sahel region, double inoculation of indigenous rhizobia and AMF isolates improved the survival rate and growth of Vachellia seyal (acacia) plantations (Fofana et al., 2020). The authors attribute the improved plant growth to the adaptation of the native bioinoculants to the pedoclimatic conditions of the region and the microbial synergism in delivering plant nutrients under water and heat stress conditions. The fact that PGPM inoculants mitigate water stress in crops and enhance crop health and productivity ensures that there is consistency in yields for smallholder farmers.
The use of effective PGPMs enhances leaf photosynthesis, seed quality traits, and yield of legumes such as Phaseolus vulgaris L.) (Iriti et al., 2019). High-quality agricultural products are easily marketable and fetch high prices, therefore, bringing more fortune to smallholder farmers resulting in improved livelihoods. The choice and selection of superior native strains over exotic strains is often encouraged (Aliyu et al., 2019). For instance, the application of native strains of Bacillus spp. as PGPM in the cultivation of cumin (Cuminum cyminum Linn.) increased the seed oil content and yields compared to uninoculated control (Mishra et al., 2019). Studies have revealed that it is economical for smallholder farmers to apply polyfunctional microbial inoculants with multiple plant growth-promoting traits such as P solubilization, N fixation, and biocontrol compared to the use of single-trait inoculants (Reddy and Saravanan, 2013). However, some studies favor the promotion of functional identity over functional diversity when dealing with specific crop genotypes and environments (Njeru et al., 2017). Further studies to elucidate this disparity should be done in the context of smallholder agroecosystems paying attention to proper management of microbial inoculants as this could significantly affect microbial functioning, abundance, and effectiveness.
The global demand for healthy food and fiber is expected to rise by 2050 to 70% (Singh and Trivedi, 2017). The increase in food demand needs to be satisfied from the existing arable land, which is already under pressure from the rising human population, harsh climatic conditions, and the decline in soil fertility and water availability. In addition, there is a need to safeguard farm produce from emerging and re-emerging pests and diseases. Despite the success of chemically associated conventional farming practices in increasing agricultural productivity, their future reliability is on the balance due to various health concerns arising from food contamination and disease resistance (Alori and Babalola, 2018). Harnessing natural resources such as plant-associated microbiome (PAM) could be one of the most effective strategies to improve agricultural productivity and future food security in a more sustainable way. The PAM technology has a better potential to minimize environmental hazards and increase food quality and quantity while lessening resource inputs compared to the conventional farm practices. Additional plant-microbiome discoveries and technological improvements are emerging and embracing a shift in the paradigm toward next-generation microbial applications could lead to better food production systems (Nezhad et al., 2015). In situ plant-microbiome engineering, high throughput sequencing, and plant breeding will be integral to enhancing the understanding and development of efficient microbial inoculants. Evidence shows that more attention has been given to rhizosphere and root microbiota that play a key role in plant productivity (Goswami and Deka, 2020; Nuzzo et al., 2020; Sousa et al., 2020), while other plant sections such as leaf, stem, seeds, and flowers remains largely unexplored. Yet, they could be playing important roles in plant defense system and response against abiotic and biotic stress (Singh and Trivedi, 2017).
Agronomic Management Practices and Interventions That Optimize PGPM Functionality
Modern farming encourages the integration of bio-inoculants with other farm management practices and this has been shown to have complementary and synergistic effects in improving growth and yield quality characteristics in apple fruit farming (Mosa et al., 2018). The positive association between agronomic management practices and ecosystem functioning could be exploited to enhance soil fertility amelioration and plant productivity (Costanzo and Bàrberi, 2014). One possible approach is to increase the above-ground litter and below-ground root traits that host and provide energy to the PGPMs responsible for decomposition and nutrient recycling (Bakker et al., 2012). This approach encourages farmers to utilize agronomic practices that increase genetic, species, and habitat diversity within the field scale hence increasing farm's overall productivity and agroecosystem resilience (Moonen and Bàrberi, 2008; Mburu et al., 2016). Conserving a high diversity of indigenous microbial functional communities in the soil ensures continuous maintenance of critical soil functions amidst the changing climatic conditions. This could provide production-related insurance to the farmers on ecosystem productivity and stability upon any ecological perturbations (Yachi and Loreau, 1999; Shanafelt et al., 2015).
Multiple cropping systems coupled with rotational practices is demonstrated to sequester more carbon to the soil (Hontoria et al., 2019). Obligate PGPMs, that would not survive without a plant host, utilize carbon as the sole energy source (Ventorino et al., 2012). Therefore, farmers should critically choose cropping systems that favor carbon sequestration in order to conserve the functioning of beneficial obligate microbial communities. However, for maximum benefits to be achieved in multiple cropping systems, choosing complementary plant genotypes known to host multiple beneficial PGPMs would be ideal. For instance, intercropping of cereals with legumes such as lentils, faba bean, and chickpea, which are considered (Faucon et al., 2017; Lazzaro et al., 2018). Cereal-legume intercropping system creates a microclimate that regulates heat stress, moisture, wind stress, weed and pest infestation (Lazzaro et al., 2018). The use of PGPMs can enhance the interspecific plant-microbial interactions in intercropping system (Figure 3). The addition of organic amendments such as vermicompost, manure, and biochar that are rich in PGPMs and nutrients would help to sustain high energy demanding soil processes and promotes microbial decomposition and nutrient recycling (Cobb et al., 2018; Nyamwange et al., 2018; Koskey et al., 2020). Non-chemical weeding and pest control methods promote the build-up of beneficial plant-soil biodiversity which are considered the main drivers of an ecosystem (Marzaioli et al., 2010). On the contrary, intensive cultivation, a common practice in smallholder agroecosystems, reduces soil biodiversity, organic matter, and increase CN ratio, therefore, reducing the overall microbial functionality (Ventorino et al., 2012). Therefore, a change in cultivation practices to more sustainable agroecological practices in smallholder systems would be inevitable if food security is to be realized.
Plants are considered naturally as selective agents that continuously shape rhizospheric soil microbiome and rhizosphere engineering is slowly emerging as a new research field to potentially address crop production (Haldar and Sengupta, 2015). AMF are important ecosystem promoters and are recognized as key elements in low-input agroecosystems; however, their structural composition, diversity and performance are highly influenced by plant genotype (López-García et al., 2017). Certain plant taxonomic families are known to be poor AMF hosts while others such as legumes are known to be excellent AMF hosts. A few plant taxa are non-host. Currently, plants are selectively bred to produce root exudates that enhance rhizosphere plant-microbiome interactions and possibly promote agroecosystem sustainability and productivity (Bakker et al., 2012). To embrace this strategy and apply in smallholder agroecosystems, it will require farmers no infrastructural changes other than the selection of their preferred crop cultivars or species bred to enhance root exudation. However, more knowledge in this area should be generated to deepen the understanding of their mechanisms, pros and cons, cost-benefit analysis, and their applicability to resource-strained smallholder farmers. Enhancing farmers' knowledge of agroecosystem functionality would optimize soil fertility restoration successes, agroecosystem sustainability, and crop productivity.
Development of Microbial Inocula for Smallholder Farmers
Currently, there is a rising global market demand for microbial inoculants that can be used as biofertilizers or biostimulants in crop production (Lobo et al., 2019). Bioinoculants contain one or more PGPMs (bacteria, algae, or fungi) packaged in a carrier material. A carrier material refers to the delivery “vehicle” that is packaged to transfer the bioinoculum to the plant rhizosphere. It determines the form (either liquid or solid), shelf life, and the application or delivery methods of the microbial inoculant (Reddy and Saravanan, 2013). According to Soumare et al. (2020), a good microbial inoculum should be packaged in a carrier material that provides an optimum microenvironment (pH, water, and carbon content) for microorganisms, maintain longer shelf life and microbial viability without the need for a special storage facility. Besides, the carrier material should be cost-effective, readily available, eco-friendly, acquiescent to nutrient supplement, and not harmful to the user (Alori and Babalola, 2018). It is interesting to note that very few studies have focused on the selection and development of carrier material and their effect on bio-inoculum as most studies emphasize on the performance of microbial strains (Herrmann and Lesueur, 2013). Encapsulation of bioinoculants is a newly emerging technique that utilizes polymer beads to enclose one or more microbial species. This technique allows the incorporation of other organic bio-effectors such as humic acid and strigolactones, protects the microbial life from desiccation, and allows slow release of the components into the soil (Gouda et al., 2018). Despite its biotechnological potentials as bioinoculant carriers, nano- and micro-encapsulation methods have not been exploited commercially particularly by entities targeting smallholder systems (Herrmann and Lesueur, 2013). Therefore, with advancing technologies, more studies that could lead to the development of versatile carrier inoculants suitable for use in smallholder agroecosystems should be done.
The fact that the majority of microbial inoculants in SSA are imported (Babalola and Glick, 2012) raises a question if they are tailored to match the varying smallholder farmers' agroecosystems, shelf-life needs, local storage, and soil conditions. A short inoculant shelf-life constraints inoculant supply chain and significantly reduces inoculant reliability, viability, and availability (Deaker et al., 2012). Inoculant viability determines the success of its use and continuous adoption by the farmers, who are in most cases production-oriented rather than agroecology conservationists. Therefore, effective bioinoculants should be able to competitively and successfully establish themselves in the soil within the shortest time possible amidst the presence of already established native microbes. Various studies have tried to unveil the fate of microbial inoculum and their effect on the native microbial communities upon their introduction into the soil (Sharma et al., 2012). For instance, Nuzzo et al. (2020) demonstrated that some PGPM formulations have no impact on plant growth but significantly affect the diversity and structure of native microbial communities. On the contrary, PGPM inoculation improved plant growth but had no influence on species diversity and richness of native microbes in the host plant roots (Piromyou et al., 2011). These inconsistencies are likely to be common in smallholder agroecosystems and, therefore, calls for selection and development of microbial strains that interact well with native microbial communities.
Soil conditions such as pH, presence of organic matter, water availability, and other physicochemical properties affect the infectivity of microbial inoculants (Njeru et al., 2020; Saad et al., 2020). Some PGPM inoculants can confer resilience against such harsh local environmental conditions (Agami et al., 2016). This explains why microbial inoculants are recommended even in soils with less water and where most nutrients are immobile (Sindhu et al., 2020). In some instances, seed companies and researchers have solved this challenge by producing crop cultivars that customarily favor colonization of specific PGPMs under a wide range of soil conditions (Faye et al., 2020). Through this approach, Gitonga et al. (2021) investigated how organic and conventional smallholder farming systems and soybean cultivars (promiscuous vs. non-promiscuous) affect native Bradyrhizobium spp. diversity. Similarly, Sinong et al. (2021) found P-solubilizing microbial isolates that could be exploited to enhance the growth of two rice cultivars under low-input cultivation than conventional practices. The authors aimed at optimizing smallholder agroecosystem at the farm level that potentially harbor diverse soil microbiota that enhances crop productivity.
In other cases, seed manufacturers have partnered with farmers to establish ‘custom seed inoculation’ where on farmer's request, seeds are inoculated with specific PGPMs by the manufacturer prior to packaging and delivery to the farmers for planting (Deaker et al., 2012). These two approaches can be easily replicated in smallholder agroecosystems, but more research and partnerships on bioprospecting for effective PGPMs compatible with various local crop cultivars should be initiated. Researchers should keep in mind the need for new PGPM inoculants adapted to the current and incoming stressful climatic conditions as the future performance of the inoculants currently in the market may not be guaranteed.
Challenges of Widespread Utilization of PGPMs in Smallholder Agroecosystems
Notwithstanding their importance in upscaling agriculture, the use of PGPM inoculants in smallholder settings is largely unaccountable (Oruru and Njeru, 2016) and more research should focus on quantifying their use, adoption, and their overall effect on soil, crops, and farmers' livelihood. Farmers are used to the application of ‘blanket’ solutions in solving their day-to-day field challenges and the adoption of particular techniques providing specific solutions in certain locations should be encouraged. Industrially, there is a challenge in the production of bioinoculants that could be used for a broad range of crops grown in geographically and climatically diverse territories (Santos et al., 2019). PGPMs, unlike the broad-spectrum agrochemicals, are highly selective in their use and only target specific plant hosts. Their viability is short and the cost of maintaining PGPMs during storage is very high especially in rural setups where electricity and modern storage facilities are limiting resources (Tabassum et al., 2017). Thus, the search for innovative microbial solutions based on farmers' needs should be done with geographical considerations, increasing episodes of climatic and environmental stresses.
The risk of toxicity arising from inoculant contamination is high if proper quality control standards and storage measures are not taken into consideration. Raimi et al. (2019) reported 67% of the South African biofertilizers, analyzed through sequencing, showed high levels of toxins and contaminants affecting the quality of the inoculants, therefore, jeopardizing their potential benefits. The intentional movement of bioinoculants containing various exotic microbial species or strains to new agroecosystems is growing, but the possible negative ecological consequence of their introduction is poorly understood (Schwartz et al., 2006). This may lead to unintended negative invasion and the establishment of microbes in new agroecosystems. Non-sterile and contaminated inoculum can result in the emergence of phytopathogens that are parasitic to the native PGPMs and plants and can potentially cause significant crop losses. Controlling the cases of invasive species would be costly to the farmers and thus, the need for inoculation particularly with imported inoculants should first be carefully evaluated and where possible, the use of high-quality local indigenous species should be recommended. In some of the African countries where economic and technological policies have been put in place to support the use of biofertilizers, there is hardly any evidence of the successful implementation of the approaches. This failure is linked to financial misappropriation, policy management and lack of investment interest among the stakeholders (Abdullah and Samah, 2013). Additionally, unreliable climatic conditions, variation in soil factors and poor agronomic management practices need to be addressed especially in the SSA region where the impact of bio-inoculation would be profound if fully adopted (Ngetich et al., 2012).
Future Perspectives
The increasing demand for safe food and better nutrition, advancing research technologies, and interest in sustainable agriculture has further renewed global interest on PGPM bioinoculants. For instance, by 2019, China registered more than 800 inoculant related patents while India surpassed 100 patents (Santos et al., 2019). Therefore, it is expected that, through advanced research innovations, more bioinoculants will be produced in the following years. The effects of climate change present a major challenge to industrial bioinoculant producers, and research on PGPMs that are more effective under a broader range of stressful conditions and induce plant tolerance would increase. The challenges on microbial shelf life, storage and viability losses should be addressed and new technologies of seed coating that deliver stable formulations able to withstand harsh storage conditions should be developed (Bargaz et al., 2018). Plant breeders, seed producers, and farmers could overcome these challenges through the initiation of “tailor-made” products that could address specific challenges. However, further scientific research and economic benefit analysis in this area should be done.
More knowledge and deeper understanding are needed on how agronomic practices under changing climatic conditions affect the composition, abundance, and bio-functionality of PGPMs in delivering multiple agroecosystem services. Farmers need to know how PGPM communities are managed at spatial and temporal scale to promote synergies, effectiveness, and avoid trade-offs. Fostering proximity to smallholder farmers in redesigning agroecosystems and policy making should be prioritized. This can be achieved by involving them in-field research demonstrations, data collection, reporting, and policy recommendation drafting (IFAD and UNEP, 2013). These approaches will enhance farmers' knowledge and technological capacity in the use of bio-inoculants, therefore, ensuring a continuous adoption of techniques that take into account their local ecological conditions and knowledge.
Conclusion
The use of PGPMs as biostimulants, biofertilizers, or biocontrol agents by smallholder farmers has substantially grown owing to their impressive performance, economic benefits, and environmental safety associated with their use. They provide beneficial agroecosystem services such as soil nutrient amelioration, crop nutrient, and yield improvement, plant tolerance to biotic and abiotic stresses, biocontrol of pests and diseases, and water uptake. The adoption of PGPMs in smallholder agroecosystems is on the rise with the increasing number of patents and new inoculants being observed in developing countries. Multisectoral research on the use of PGPMs involving smallholder farmers is encouraged and its output should capture the aims of both the “productionist” and “agroecologist” paradigms. More knowledge on the effects of climate change and agronomic practices on the bio-functionality of PGPMs in delivering multiple agroecosystem services should be generated. Robust technologies are needed to enhance the PGPM production and most importantly to improve the effectiveness, stability, and reliability of the products under environmentally stressing conditions. Putting into consideration the collective experience, needs, and indigenous knowledge of smallholder farmers, PGPM inoculation could be a key pillar in addressing SDG 2 goal of ending hunger, promoting food security, and environmental sustainability.
Author Contributions
GK drafted the outline structure and prepared the manuscript with contributions from SM and RA. EN and JM provided technical guidelines and reviews during manuscript preparation. All authors approved the final draft of the manuscript for submission.
Funding
This work was supported by The Future Leaders–African Independent Researchers (FLAIR) Fellowship Programme, which is a partnership between the African Academy of Sciences and the Royal Society funded by the UK Government's Global Challenges Research Fund (Grant No. FLR/R1/190944). The Scuola Superiore Sant'Anna, Pisa, Italy and the Deutscher Akademischer Austauschdienst (DAAD) funded the Ph.D., scholarships of GK and SM, respectively.
Conflict of Interest
The authors declare that the research was conducted in the absence of any commercial or financial relationships that could be construed as a potential conflict of interest.
Acknowledgments
The authors acknowledge the smallholder farmers from Tharaka Nithi County, Kenya and the Kenyatta University FLAIR research team (Nairobi) led by EN for their collaboration and in provision of supporting materials included in this manuscript.
References
Abbey, J. A., Percival, D., Abbey L.ord, Asiedu, S. K., Prithiviraj, B., and Schilder, A. (2019). Biocontrol science and technology biofungicides as alternative to synthetic fungicide control of grey mould (Botrytis cinerea)-prospects and challenges. Biocontrol Sci. Technol. 29, 241–262. doi: 10.1080/09583157.2018.1548574
Abdel Latef, A. A. H., Abu Alhmad, M. F., Kordrostami, M., Abo–Baker, A. B. A. E., and Zakir, A. (2020). Inoculation with azospirillum lipoferum or azotobacter chroococcum reinforces maize growth by improving physiological activities under saline conditions. J. Plant Growth Regul. 39, 1293–1306. doi: 10.1007/s00344-020-10065-9
Abdel-Rahman, H. M., Salem, A. A., Moustafa, M. M. A., and Hoda, El-Garhy, A. S. (2017). A novice Achromobacter sp. EMCC1936 strain acts as a plant-growth-promoting agent. Acta Physiol. Plant. 39:61. doi: 10.1007/s11738-017-2360-6
Abdullah, F. A., and Samah, B. A. (2013). Factors impinging farmers' use of agriculture technology article in asian social science. Asian Soc. Sci. 9, 120–124. doi: 10.5539/ass.v9n3p120
Abo-Zaid, G. A., Matar, S. M., and Abdelkhalek, A. (2020). Induction of plant resistance against tobacco mosaic virus using the biocontrol agent streptomyces cellulosae isolate actino 48. Agronomy 10, 1–16. doi: 10.3390/agronomy10111620
Adeyemi, N. O., Atayese, M. O., Olubode, A. A., and Akan, M. E. (2019). Effect of commercial arbuscular mycorrhizal fungi inoculant on growth and yield of soybean under controlled and natural field conditions. J. Plant Nutr. doi: 10.1080/01904167.2019.1685101
Agami, R., Abd El Khalek Abd El Mola, I., A, A. R., Medani, R., Abd El-Mola, I., and Taha, R. (2016). Exogenous application with plant growth promoting rhizobacteria (PGPR) or proline induces stress tolerance in basil plants (Ocimum basilicum L.) exposed to water stress. Int. J. Environ. Agric. Res. 2, 78–92. Available online at: https://www.researchgate.net/publication/308984083
Ahemad, M., and Kibret, M. (2014). Mechanisms and applications of plant growth promoting rhizobacteria: current perspective. J. King Saud Univ. Sci. 26, 1–20. doi: 10.1016/j.jksus.2013.05.001
Ahmed, E., and Holmström, S. J. M. (2014). Siderophores in environmental research: roles and applications. Microb. Biotechnol. 7, 196–208. doi: 10.1111/1751-7915.12117
Aliyu, I. A., Yusuf, A. A., and Atta, A. (2019). Evaluation of indigenous rhizobial isolates in search for candidate strain for commercial production. Bayero J. Pure Appl. Sci. 11:33. doi: 10.4314/bajopas.v11i1.5S
Alori, E. T., and Babalola, O. O. (2018). Microbial inoculants for improving crop quality and human health in Africa. Front. Microbiol. 9:2213. doi: 10.3389/fmicb.2018.02213
Altieri, M. A., Funes-Monzote, F. R., and Petersen, P. (2012). Agroecologically efficient agricultural systems for smallholder farmers: contributions to food sovereignty. Agron. for Sust. Dev. 32, 1–13. doi: 10.1007/s13593-011-0065-6
Arthurs, S., and Heinz, K. M. (2006). Biocontrol science and technology evaluation of the nematodes steinernema feltiae and thripinema nicklewoodi as biological control agents of western flower thrips frankliniella occidentalis infesting chrysanthemum. Biocontrol Sci. Technol. 16, 141–155. doi: 10.1080/09583150500258545
Aseri, G. K., Jain, N., and Tarafdar, J. C. (2009). Hydrolysis of organic phosphate forms by phosphatases and phytase producing fungi of arid and semi arid soils of India. Am. Euras. J. Agric. Environ. Sci. 5, 564–570. Available online at: https://www.researchgate.net/publication/278679570
Avio, L., Turrini, A., Giovannetti, M., and Sbrana, C. (2018). Designing the ideotype mycorrhizal symbionts for the production of healthy food. Front. Plant Sci. 9:1089. doi: 10.3389/fpls.2018.01089
Azizoglu, U. (2019). Bacillus thuringiensis as a biofertilizer and biostimulator: a mini-review of the little-known plant growth-promoting properties of Bt. Curr. Microbiol. 76, 1379–1385. doi: 10.1007/s00284-019-01705-9
Babalola, O. O., and Glick, B. R. (2012). The use of microbial inoculants in African agriculture: current practice and future prospects. J. Food Agric. Environ. 10, 540–549. https://www.researchgate.net/publication/260934217
Bado, B. V., Sedogo, M., Lompo, F., and Maman Laminou, S. M. (2018). Biological nitrogen fixation by local and improved genotypes of cowpea in burkina faso (West Africa): total nitrogen accumulated can be used for quick estimation. Adv. Agric. 2018, 1–8. doi: 10.1155/2018/9641923
Bakker, M. G., Manter, D. K., Sheflin, A. M., Weir, T. L., and Vivanco, J. M. (2012). Harnessing the rhizosphere microbiome through plant breeding and agricultural management. Plant Soil 360, 1–13. doi: 10.1007/s11104-012-1361-x
Barberi, P. (2015). Functional biodiversity in organic systems: the way forward? Sust Agric Res. 4:26. doi: 10.5539/sar.v4n3p26
Bargaz, A., Lyamlouli, K., Chtouki, M., Zeroual, Y., and Dhiba, D. (2018). Soil microbial resources for improving fertilizers efficiency in an integrated plant nutrient management system. Front. Microbiol. 9:1606. doi: 10.3389/fmicb.2018.01606
Bationo, A., Hartemink, A., Lungu, O., Naimi, M., Okoth, P., Smaling, E., et al. (2012). “Knowing the African soils to improve fertilizer recommendations,” in Improving Soil Fertility Recommendations in Africa Using the Decision Support System for Agrotechnology Transfer (DSSAT), eds J. Kihara, D. Fatondji, J. Jones, G. Hoogenboom, R. Tabo, and A. Bationo (Dordrecht: Springer), 19–42. doi: 10.1007/978-94-007-2960-5_3
Bonner, M. R., and Alavanja, M. C. R. (2017). Pesticides, human health, and food security. Food Energy Secur. 6, 89–93. doi: 10.1002/fes3.112
Browne, P., Rice, O., Miller, S. H., Burke, J., Dowling, D. N., Morrissey, J. P., et al. (2009). Superior inorganic phosphate solubilization is linked to phylogeny within the pseudomonas fluorescens complex. Appl. Soil Ecol. 43, 131–138. doi: 10.1016/j.apsoil.2009.06.010
Brussaard, L., de Ruiter, P. C., and Brown, G. G. (2007). Soil biodiversity for agricultural sustainability. Agric. Ecosyst. Environ. 121, 233–244. doi: 10.1016/j.agee.2006.12.013
Carlson, R., Tugizimana, F., Steenkamp, P. A., Dubery, I. A., Hassen, A. I., and Labuschagne, N. (2020). Rhizobacteria-induced systemic resilience in Sorghum bicolor (L.) moench against Fusarium pseudograminearum crown rot under drought stress conditions. Biol. Control 151:104395. doi: 10.1016/j.biocontrol.2020.104395
Celador-Lera, L., Jiménez-Gómez, A., Menéndez, E., and Rivas, R. (2018). “Biofertilizers based on bacterial endophytes isolated from cereals: potential solution to enhance these crops,” in Role of Rhizospheric Microbes in Soil: Stress Management and Agricultural Sustainability, Vol. 1 (Singapore: Springer), 175–203. doi: 10.1007/978-981-10-8402-7_7
Chavoshi, S., Nourmohamadi, G., Madani, H., Abad, H. H. S., and Fazel, M. A. (2018). The effects of biofertilizers on physiological traits and biomass accumulation of red beans (Phaseolus vulgaris cv.Goli) under water stress. Iran. J. Plant Physiol. 8, 2555–2562. doi: 10.22034/IJPP.2018.543427
Choudhary, D. K., and Varma, A. (2017). “Nitrogenase (a Key Enzyme): Structure and Function,” in Rhizobium Biology and Biotechnology. Soil Biology, eds A. Hansen, D. Choudhary, P. Agrawal, and A. Varma (Cham: Springer), 293–307. doi: 10.1007/978-3-319-64982-5_14
Cobb, A. B., Wilson, G. W. T., Goad, C. L., and Grusak, M. A. (2018). Influence of alternative soil amendments on mycorrhizal fungi and cowpea production. Heliyon 4:e00704. doi: 10.1016/j.heliyon.2018.e00704
Coniglio, A., Mora, V., Puente, M., and Cassán, F. (2019). “Azospirillum as biofertilizer for sustainable agriculture: azospirillum brasilense AZ39 as a model of PGPR and field traceability,” in Microbiol Probiotics for Agricultural Systems, eds D. Zúñiga-Dávila, F. González-Andrés, and E. Ormeño-Orrillo (Cham: Springer), 45–70. doi: 10.1007/978-3-030-17597-9_4
Constantine, K. L., Kansiime, M. K., Mugambi, I., Nunda, W., Chacha, D., Rware, H., et al. (2020). Why don't smallholder farmers in Kenya use more biopesticides? Pest Manage. Sci. 76, 3615–3625. doi: 10.1002/ps.5896
Costanzo, A., and Bàrberi, P. (2014). Functional agrobiodiversity and agroecosystem services in sustainable wheat production. A review. Agron. Sust. Dev. 34, 327–348. doi: 10.1007/s13593-013-0178-1
Dakora, F. D., Matiru, V. N., and Kanu, A. S. (2015). Rhizosphere ecology of lumichrome and riboflavin, two bacterial signal molecules eliciting developmental changes in plants. Front. Plant Sci. 6:700. doi: 10.3389/fpls.2015.00700
Dawwam, G. E., Elbeltagy, A., Emara, H. M., Abbas, I. H., and Hassan, M. M. (2013). Beneficial effect of plant growth promoting bacteria isolated from the roots of potato plant. Ann. Agric. Sci. 58, 195–201. doi: 10.1016/j.aoas.2013.07.007
Deaker, R., Hartley, E., and Gemell, G. (2012). Conditions affecting shelf-life of inoculated legume seed. Agriculture 2, 38–51. doi: 10.3390/agriculture2010038
Ding, B., Zhang, X., Xu, Y., An, L., Liu, X., and Su, Q. (2020). The bacterial potassium transporter gene MbtrkH improves K+ uptake in yeast and tobacco. PLoS ONE 15:e036246. doi: 10.1371/journal.pone.0236246
Dissanayaka, D. M. S. B., Plaxton, W. C., Lambers, H., Siebers, M., Marambe, B., and Wasaki, J. (2018). Molecular mechanisms underpinning phosphorus-use efficiency in rice. Plant Cell Environ. 41, 1483–1496. doi: 10.1111/pce.13191
Draghi, W. O., María, P., Del, F., Barsch, A., Albicoro, F. J., Lozano, M. J., et al. (2017). A metabolomic approach to characterize the acid-tolerance response in Sinorhizobium meliloti. Metabolomics. 1, 71.
Egamberdiyeva, D. (2007). The effect of plant growth promoting bacteria on growth and nutrient uptake of maize in two different soils. Appl. Soil Ecol. 36, 184–189. doi: 10.1016/j.apsoil.2007.02.005
Elagib, N. A., and Al-Saidi, M. (2020). Balancing the benefits from the water–energy–land–food nexus through agroforestry in the Sahel. Sci. Total Environ. 742:140509. doi: 10.1016/j.scitotenv.2020.140509
Escalas, A., Hale, L., Voordeckers, J. W., Yang, Y., Firestone, M. K., Alvarez-Cohen, L., et al. (2019). Microbial functional diversity: from concepts to applications. Ecol. Evolut. 9, 12000–12016. doi: 10.1002/ece3.5670
Etesami, H., Alikhani, H. A., and Hosseini, H. M. (2015). Indole-3-acetic acid (IAA) production trait, a useful screening to select endophytic and rhizosphere competent bacteria for rice growth promoting agents. MethodsX. 2, 72–78. doi: 10.1016/j.mex.2015.02.008
FAO (2018). Tracking Progress on Food and Agriculture-Related SDG Indicators A Report on the Indicators Under FAO Custodianship. Available online at: http://www.fao.org/sdg-progress-report/en/
Faucon, M. P., Houben, D., and Lambers, H. (2017). Plant functional traits: soil and ecosystem services. Trends Plant Sci. 22, 385–394. doi: 10.1016/j.tplants.2017.01.005
Faye, A., Stewart, Z. P., Ndung'u-Magiroi, K., Diouf, M., Ndoye, I., Diop, T., et al. (2020). testing of commercial inoculants to enhance p uptake and grain yield of promiscuous soybean in Kenya. Sustainability 12:3803. doi: 10.3390/su12093803
Fofana, B., Sacande, M., Blagna, F., Dibloni, T. O., Compaore, E., Sanon, K. B., et al. (2020). Boosting land restoration success in the great green wall through the use of symbiotic microorganisms for propagated tree seedlings. Int. J. Biol. Chem. Sci. 14, 110–125. doi: 10.4314/ijbcs.v14i1.10
Fukami, J., Cerezini, P., and Hungria, M. (2018a). Azospirillum: benefits that go far beyond biological nitrogen fixation. AMB Express 8:73. doi: 10.1186/s13568-018-0608-1
Fukami, J., Ollero, F. J., de la Osa, C., Megías, M., and Hungria, M. (2018b). Co-inoculation of maize with azospirillum brasilense and rhizobium tropici as a strategy to mitigate salinity stress. Funct. Plant Biol. 45, 328. doi: 10.1071/FP17167
Gabasawa, A. I. (2020). “Prospects for developing effective and competitive native strains of rhizobium inoculants in Nigeria,” in Current Microbiological Research in Africa, eds A. Abia and G. Lanza (Cham: Springer), 223–256. doi: 10.1007/978-3-030-35296-7_9
Gamez, R., Cardinale, M., Montes, M., Ramirez, S., Schnell, S., and Rodriguez, F. (2019). Screening, plant growth promotion and root colonization pattern of two rhizobacteria (pseudomonas fluorescens Ps006 and Bacillus amyloliquefaciens Bs006) on banana cv. Williams (Musa acuminata Colla). Microbiol. Res. 220, 12–20. doi: 10.1016/j.micres.2018.11.006
Giller, K. E., Kanampiu, F., Hungria, M., and Vanlauwe, B. (2019). The role of nitrogen fixation in African smallholder agriculture. Agric. Ecosyst. Environ. 285:106601. doi: 10.1016/j.agee.2019.106601
Giovannini, L., Palla, M., Agnolucci, M., Avio, L., Sbrana, C., Turrini, A., et al. (2020). Arbuscular mycorrhizal fungi and associated microbiota as plant biostimulants: research strategies for the selection of the best performing inocula. Agronomy 10:106. doi: 10.3390/agronomy10010106
Gitonga, N. M., Njeru, E. M., Cheruiyot, R., and Maingi, J. M. (2021). Genetic and morphological diversity of indigenous bradyrhizobium nodulating soybean in organic and conventional family farming systems. Front. Sust. Food Syst. 4:606618. doi: 10.3389/fsufs.2020.606618
Goswami, M., and Deka, S. (2020). Plant growth-promoting rhizobacteria—alleviators of abiotic stresses in soil: a review. Pedosphere 30, 40–61. doi: 10.1016/S1002-0160(19)60839-8
Gouda, S., Kerry, R. G., Das, G., Paramithiotis, S., Shin, H. S., and Patra, J. K. (2018). Revitalization of plant growth promoting rhizobacteria for sustainable development in agriculture. Microbil. Res. 206, 131–140. doi: 10.1016/j.micres.2017.08.016
Grover, M., Ali, S. Z., Sandhya, V., Rasul, A., and Venkateswarlu, B. (2011). Role of microorganisms in adaptation of agriculture crops to abiotic stresses. World J. Microbiol. Biotechnol. 27, 1231–1240. doi: 10.1007/s11274-010-0572-7
Guo, D. J., Singh, R. K., Singh, P., Li, D. P., Sharma, A., Xing, Y. X., et al. (2020). Complete genome sequence of enterobacter roggenkampii ED5, a nitrogen fixing plant growth promoting endophytic bacterium with biocontrol and stress tolerance properties, isolated from sugarcane root. Front. Microbiol. 11:580081. doi: 10.3389/fmicb.2020.580081
Guo, Q., Li, Y., Lou, Y., Shi, M., Jiang, Y., Zhou, J., et al. (2019). Bacillus amyloliquefaciens Ba13 induces plant systemic resistance and improves rhizosphere microecology against tomato yellow leaf curl virus disease. Appl. Soil Ecol. 137, 154–166. doi: 10.1016/j.apsoil.2019.01.015
Gupta, V. V. S. R., Zhang, B., Penton, C. R., Yu, J., and Tiedje, J. M. (2019). Diazotroph diversity and nitrogen fixation in summer active perennial grasses in a mediterranean region agricultural soil. Front. Mol. Biosci. 6:115. doi: 10.3389/fmolb.2019.00115
Gururani, M. A., Upadhyaya, C. P., Baskar, V., Venkatesh, J., Nookaraju, A., and Park, S. W. (2013). Plant growth-promoting rhizobacteria enhance abiotic stress tolerance in solanum tuberosum through inducing changes in the expression of ROS-scavenging enzymes and improved photosynthetic performance. J. Plant Growth Regul. 32, 245–258. doi: 10.1007/s00344-012-9292-6
Haldar, S., and Sengupta, S. (2015). Plant-microbe cross-talk in the rhizosphere: insight and biotechnological potential. Open Microbiol. J. 9, 1–7. doi: 10.2174/1874285801509010001
Harrison, R. D., Thierfelder, C., Baudron, F., Chinwada, P., Midega, C., Schaffner, U., et al. (2019). Agro-ecological options for fall armyworm (Spodoptera frugiperda JE Smith)management: Providing low-cost, smallholder friendly solutions to an invasive pest. J. Environ. Manage. 243, 318–330. doi: 10.1016/j.jenvman.2019.05.011
Hatting, J. L., Moore, S. D., and Malan, A. P. (2019). Microbial control of phytophagous invertebrate pests in South Africa: current status and future prospects. J. Invertebr. Pathol. 165, 54–66. doi: 10.1016/j.jip.2018.02.004
Heil, M., and Bostock, R. M. (2002). Induced systemic resistance (ISR) against pathogens in the context of induced plant defences. Ann. Bot. 89, 503–512. doi: 10.1093/aob/mcf076
Herrero, M., Thornton, P. K., Power, B., Bogard, J. R., Remans, R., Fritz, S., et al. (2017). Farming and the geography of nutrient production for human use: a transdisciplinary analysis. Lancet Planet. Health 1, e33–e42. doi: 10.1016/S2542-5196(17)30007-4
Herrmann, L., and Lesueur, D. (2013). Challenges of formulation and quality of biofertilizers for successful inoculation. Appl. Microbiol. Biotechnol. 97, 8859–8873. doi: 10.1007/s00253-013-5228-8
Hontoria, C., García-González, I., Quemada, M., Roldán, A., and Alguacil, M. M. (2019). The cover crop determines the AMF community composition in soil and in roots of maize after a ten-year continuous crop rotation. Sci. Total Environ. 660, 913–922. doi: 10.1016/j.scitotenv.2019.01.095
Hungria, M., Nogueira, M. A., and Araujo, R. S. (2015). Soybean Seed Co-Inoculation with Bradyrhizobium spp. and Azospirillum brasilense: A New Biotechnological Tool to Improve Yield and Sustainability. American Journal of Plant Sciences. 6, 811–817. doi: 10.4236/ajps.2015.66087
IFAD UNEP (2013). Smallholders, Food Security and the Environment. Available online at: https://www.ifad.org/documents/38714170/39135645/smallholders_report.pdf/133e8903-0204-4e7d-a780-bca847933f2e
Iriti, M., Scarafoni, A., Pierce, S., Castorina, G., and Vitalini, S. (2019). Soil application of effective microorganisms (EM) maintains leaf photosynthetic efficiency, increases seed yield and quality traits of bean (Phaseolus vulgaris L.) plants grown on different substrates. Int. J. Mol. Sci. 20:2327. doi: 10.3390/ijms20092327
Jaiswal, D. K., Verma, J. P., Prakash, S., Meena, V. S., and Meena, R. S. (2016). “Potassium as an important plant nutrient in sustainable agriculture: a state of the art,” in Potassium Solubilizing Microorganisms for Sustainable Agriculture, eds V. Meena, B. Maurya, J. Verma, and R. Meena (Springer), 21–29. doi: 10.1007/978-81-322-2776-2_2
Jiménez-Gómez, A., Celador-Lera, L., Fradejas-Bayón, M., and Rivas, R. (2017). Plant probiotic bacteria enhance the quality of fruit and horticultural crops. AIMS Microbiol. 3, 483–501. doi: 10.3934/microbiol.2017.3.483
Kalayu, G. (2019). Phosphate solubilizing microorganisms: Promising approach as biofertilizers. Int. J. Agron. 2019:4917256. doi: 10.1155/2019/4917256
Kaminsky, L. M., Trexler, R. V., Malik, R. J., Hockett, K. L., and Bell, T. H. (2019). The Inherent conflicts in developing soil microbial inoculants. Trends Biotechnol. 37, 140–151. doi: 10.1016/j.tibtech.2018.11.011
Kannahi, M., and Senbagam, N. (2014). Studies on siderophore production by microbial isolates obtained from rhizosphere soil and its antibacterial activity. J. Chem. Pharmaceut. Res. 6, 1142–1145. Available online at: http://jocpr.com/vol6-iss4-2014/JCPR-2014-6-4-1142-1145.pdf
Kavoo-Mwangi, A. M., Kahangi, E. M., Ateka, E., Onguso, J., Mukhongo, R. W., Mwangi, E. K., et al. (2013). Growth effects of microorganisms based commercial products inoculated to tissue cultured banana cultivated in three different soils in Kenya. Appl. Soil Ecol. 64, 152–162. doi: 10.1016/j.apsoil.2012.12.002
Kawaka, F., Dida, M. M., Opala, P. A., Ombori, O., Maingi, J., Osoro, N., et al. (2014). Symbiotic Efficiency of Native Rhizobia Nodulating Common Bean (Phaseolus vulgaris L. ) in Soils of Western Kenya. Int. Sch. Res. Notices 2014, 1–8. doi: 10.1155/2014/258497
Kebede, E. (2020). Grain legumes production and productivity in Ethiopian smallholder agricultural system, contribution to livelihoods and the way forward. Cogent Food Agric. 6:1722353. doi: 10.1080/23311932.2020.1722353
Kermah, M., Franke, A. C., Adjei-Nsiah, S., Ahiabor, B. D. K., Abaidoo, R. C., and Giller, K. E. (2018). N2-fixation and N contribution by grain legumes under different soil fertility status and cropping systems in the Guinea savanna of northern Ghana. Agric. Ecosyst. Environ. 261, 201–210. doi: 10.1016/j.agee.2017.08.028
Khalid, M., Hassani, D., Bilal, M., Asad, F., and Huang, D. (2017). Influence of bio-fertilizer containing beneficial fungi and rhizospheric bacteria on health promoting compounds and antioxidant activity of Spinacia oleracea L. Botan. Stud. 58:35. doi: 10.1186/s40529-017-0189-3
Kiss, L. (2008). Intracellular mycoparasites in action: interactions between powdery mildew fungi and ampelomyces. Br. Mycol. Soc. Sympo. Ser. 27, 37–52. doi: 10.1016/S0275-0287(08)80045-8
Köhl, J., Kolnaar, R., and Ravensberg, W. J. (2019). Mode of action of microbial biological control agents against plant diseases: relevance beyond efficacy. Front. Plant Sci. 10:845. doi: 10.3389/fpls.2019.00845
Koskey, G., Avio, L., Lazzaro, M., Pellegrini, F., Sbrana, C., Turrini, A., et al. (2020). Smart use of microbial-rich vermicomposting to enhance tripartite plant-microbe-soil interactions. EGU Gen. Assemb. 2020, 1–2. doi: 10.5194/egusphere-egu2020-3345
Koskey, G., Mburu, S. W., Kimiti, J. M., Ombori, O., Maingi, J. M., and Njeru, E. M. (2018). Genetic characterization and diversity of rhizobium isolated from root nodules of mid-altitude climbing bean (Phaseolus vulgaris L.) varieties. Front. Microbiol. 9:968. doi: 10.3389/fmicb.2018.00968
Koskey, G., Mburu, S. W., Njeru, E. M., Kimiti, J. M., Ombori, O., and Maingi, J. M. (2017). Potential of native rhizobia in enhancing nitrogen fixation and yields of climbing beans (Phaseolus vulgaris L.) in contrasting environments of eastern Kenya. Front. Plant Sci. 8:443. doi: 10.3389/fpls.2017.00443
Kristek, S., Kristek, A., Guberac, V., and Stanisavljevi,ć, A. (2006). Effect of bacterium Pseudomonas fluorescens and low fungicide dose seed treatments on parasite fungus Aphanomyces cochlioides and sugar beet yield and. Plant Soil Environ. 52, 314–320. doi: 10.17221/3447-PSE
Kuivanen, K. S., Alvarez, S., Michalscheck, M., Adjei-Nsiah, S., Descheemaeker, K., Mellon-Bedi, S., et al. (2016). Characterising the diversity of smallholder farming systems and their constraints and opportunities for innovation: a case study from the Northern Region, Ghana. NJAS Wagen. J. Life Sci. 78, 153–166. doi: 10.1016/j.njas.2016.04.003
Kumari, P., Meena, M., Gupta, P., Dubey, M. K., Nath, G., and Upadhyay, R. S. (2018). Plant growth promoting rhizobacteria and their biopriming for growth promotion in mung bean (Vigna radiata (L.) R. Wilczek). Biocatal. Agric. Biotechnol. 16, 163–171. doi: 10.1016/j.bcab.2018.07.030
Kumawat, K. C., Sharma, P., Sirari, A., Singh, I., Gill, B. S., Singh, U., et al. (2019). Synergism of Pseudomonas aeruginosa (LSE-2) nodule endophyte with Bradyrhizobium sp. (LSBR-3) for improving plant growth, nutrient acquisition and soil health in soybean. World J. Microbiol. Biotechnol. 35:47. doi: 10.1007/s11274-019-2622-0
Kushwaha, P., Kashyap, P. L., Kuppusamy, P., Srivastava, A. K., and Tiwari, R. K. (2020). Functional characterization of endophytic bacilli from pearl millet (Pennisetum glaucum) and their possible role in multiple stress tolerance. Plant Biosyst. 154, 503–514. doi: 10.1080/11263504.2019.1651773
Lazzaro, M., Costanzo, A., and Bàrberi, P. (2018). Single vs multiple agroecosystem services provided by common wheat cultivar mixtures: weed suppression, grain yield and quality. Field Crops Res. 221, 277–297. doi: 10.1016/j.fcr.2017.10.006
Li, H.-B., Singh, R. K., Singh, P., Song, Q.-Q., Xing, Y.-X., Yang, L.-T., et al. (2017). Genetic diversity of nitrogen-fixing and plant growth promoting pseudomonas species isolated from sugarcane rhizosphere. Front. Microbiol. 8:1268. doi: 10.3389/fmicb.2017.01268
Lin, L., and Xu, X. (2013). Indole-3-acetic acid production by endophytic streptomyces sp. En-1 isolated from medicinal plants. Curr. Microbiol. 67, 209–217. doi: 10.1007/s00284-013-0348-z
Liu, X., Cao, A., Yan, D., Ouyang, C., Wang, Q., and Li, Y. (2019). Overview of mechanisms and uses of biopesticides. Int. J. Pest Manage. 24, 1–8. doi: 10.1080/09670874.2019.1664789
Lobo, C. B., Juárez Tomás, M. S., Viruel, E., Ferrero, M. A., and Lucca, M. E. (2019). Development of low-cost formulations of plant growth-promoting bacteria to be used as inoculants in beneficial agricultural technologies. Microbiol. Res. 219, 12–25. doi: 10.1016/j.micres.2018.10.012
López-García, Á., Varela-Cervero, S., Vasar, M., Öpik, M., Barea, J. M., and Azcón-Aguilar, C. (2017). Plant traits determine the phylogenetic structure of arbuscular mycorrhizal fungal communities. Mol. Ecol. 26, 6948–6959. doi: 10.1111/mec.14403
Mabrouk, Y., Hemissi, I., Salem, I., Ben, M. S., Saidi, M., and Belhadj, O. (2018). “Potential of rhizobia in improving nitrogen fixation and yields of legumes,” in Symbiosis, ed E. C. Rigobelo (London: InTech), 107–119. doi: 10.5772/intechopen.73495
Malik, A., Mor, V. S., Tokas, J., Punia, H., Malik, S., Malik, K., et al. (2020). “Biostimulant-treated seedlings under sustainable agriculture: a global perspective facing climate change. Agronomy 11:14. doi: 10.3390/agronomy11010014
Marzaioli, R., D'Ascoli, R., and De Pascale, R. F. A. R. (2010). Soil quality in a Mediterranean area of Southern Italy as related to different land use types. Appl. Soil Ecol. 44, 205–212. doi: 10.1016/j.apsoil.2009.12.007
Masso, C., Mukhongo, R. W., Thuita, M., Abaidoo, R., Ulzen, J., Kariuki, G., et al. (2016). “Biological inoculants for sustainable intensification of agriculture in Sub-Saharan Africa smallholder farming systems,” in Climate Change and Multi-Dimensional Sustainability in African Agriculture, eds R. Lal, D. Kraybill, D. Hansen, B. Singh, T. Mosogoya, and L. Eik (Cham: Springer), 639–658. doi: 10.1007/978-3-319-41238-2_33
Mburu, S. W., Koskey, G., Kimiti, J. M., Ombori, O., Maingi, J. M., and Njeru, E. M. (2016). Agrobiodiversity conservation enhances food security in subsistence-based farming systems of Eastern kenya. Agric. Food Secur. 5:19. doi: 10.1186/s40066-016-0068-2
Mburu, S. W., Koskey, G., Njeru, E. M., Ombori, O., Maingi, J. M., and Kimiti, J. M. (2020). Differential response of promiscuous soybean to local diversity of indigenous and commercial bradyrhizobium inoculation under contrasting agroclimatic sones. Int. J. Plant Product. doi: 10.1007/s42106-020-00117-1
McDonald, B. A., and Stukenbrock, E. H. (2016). Rapid emergence of pathogens in agro-ecosystems: global threats to agricultural sustainability and food security. Philos. Trans. Royal Soc. B Biol. Sci. 371, 20160026. doi: 10.1098/rstb.2016.0026
McGuire, A. V., and Northfield, T. D. (2020). Tropical occurrence and agricultural importance of beauveria bassiana and metarhizium anisopliae. Front. Sust. Food Syst. 4:6. doi: 10.3389/fsufs.2020.00006
McNeely, D., Chanyi, R. M., Dooley, J. S., Moore, J. E., and Koval, S. F. (2017). Biocontrol of Burkholderia cepacia complex bacteria and bacterial phytopathogens by Bdellovibrio bacteriovorus. Can. J. Microbiol. 63, 350–358. doi: 10.1139/cjm-2016-0612
Melo, A. L., de, A., Soccol, V. T., and Soccol, C. R. (2016). Bacillus thuringiensis: mechanism of action, resistance, and new applications: a review. Crit. Rev. Biotechnol. 36, 317–326. doi: 10.3109/07388551.2014.960793
Menge, E. M., Njeru, E. M., Koskey, G., and Maingi, J. (2018). Rhizobial inoculation methods affect the nodulation and plant growth traits of host plant genotypes: a case study of common bean Phaseolus vulgaris L. germplasms cultivated by smallholder farmers in Eastern Kenya. Adv. Agric. Sci. 6, 77–94. Available online at: https://www.researchgate.net/publication/328886447
Mhango, W. G., Snapp, S., and Kanyama-Phiri, Y. (2017). Biological nitrogen fixation and yield of pigeonpea and groundnut: quantifying response on smallholder farms in northern Malawi. Afr. J. Agric. Res. 12, 1385–1394. doi: 10.5897/AJAR2017.12232
Mishra, B. K., Lal, G., Sharma, Y. K., Kant, K., Saxena, S. N., and Dubey, P. N. (2019). Effect of microbial inoculants on cumin (Cuminum cyminum Linn.) growth and yield. Int. J. Seed Spices 53, 53–56. Available online at: http://isss.ind.in/userfiles/file/2019~new/Untitled-9.pdf
Misra, M., Sachan, A., and Sachan, S. G. (2020). “Current aspects and applications of biofertilizers for sustainable agriculture,” in Plant Microbiomes for Sustainable Agriculture (Cham: Springer), 445–473. doi: 10.1007/978-3-030-38453-1_15
Moonen, A. C., and Bàrberi, P. (2008). Functional biodiversity: an agroecosystem approach. Agric. Ecosyst. Environ. 127, 7–21. doi: 10.1016/j.agee.2008.02.013
Mosa, W. F. A. E.-G., Paszt, L. S., Frac, M., Trzciński, P., Treder, W., and Klamkowski, K. (2018). The role of biofertilizers in improving vegetative growth, yield and fruit quality of apple. Hortic. Sci. 45, 173–180. doi: 10.17221/101/2017-HORTSCI
Mukhongo, R. W., Tumuhairwe, J. B., Ebanyat, P., AbdelGadir, A. A. H., Thuita, M., and Masso, C. (2017). Combined application of biofertilizers and inorganic nutrients improves sweet potato yields. Front. Plant Sci. 8:219. doi: 10.3389/fpls.2017.00219
Mukhongo, R. W., Tumuhairwe, J. B., Ebanyat, P., AbdelGadir, A. H., Thuita, M., and Masso, C. (2016). Production and use of arbuscular mycorrhizal fungi inoculum in sub-Saharan Africa: challenges and ways of improving. Int. J. Soil Sci. 11, 108–122. doi: 10.3923/ijss.2016.108.122
Mus, F., Alleman, A. B., Pence, N., Seefeldt, L. C., and Peters, J. W. (2018). Exploring the alternatives of biological nitrogen fixation. Metallomics 10, 523–538. doi: 10.1039/C8MT00038G
Musyoka, D. M., Njeru, E. M., Nyamwange, M. M., and Maingi, J. M. (2020). Arbuscular mycorrhizal fungi and bradyrhizobium co-inoculation enhances nitrogen fixation and growth of green grams (Vigna radiata L.) under water stress. J. Plant Nutr. 43, 1036–1047. doi: 10.1080/01904167.2020.1711940
Nezhad, H. M., Shafiabadi, J., Hussain, M. A., and Nezhad, M. H. (2015). Microbial resources to safeguard future food security. Adv. Food Technol. Nutr. Sci. 1, 8–13. doi: 10.17140/AFTNSOJ-SE-1-102
Ngetich, F. K., Shisanya, C. A., Mugwe, J., Mucheru-Muna, M., and Mugendi, D. (2012). “The potential of organic and inorganic nutrient sources in sub-saharan african crop farming systems,” in Soil Fertility Improvement and Integrated Nutrient Management - A Global Perspective, ed J. K. Whalen (InTech), 135–159. Available online at: www.intechopen.com
Nicolopoulou-Stamati, P., Maipas, S., Kotampasi, C., Stamatis, P., and Hens, L. (2016). Chemical pesticides and human health: the urgent need for a new concept in agriculture. Front. Public Health 4:148. doi: 10.3389/fpubh.2016.00148
Niu, D.-D., Wang, C.-J., Guo, Y.-H., Jiang, C.-H., Zhang, W.-Z., Wang, Y.-P., et al. (2012). The plant growth-promoting rhizobacterium Bacillus cereus AR156 induces resistance in tomato with induction and priming of defence response. Biocont. Sci. Technol. 22, 991–1004. doi: 10.1080/09583157.2012.706595
Njeru, E. M., Bocci, G., Avio, L., Sbrana, C., Turrini, A., Giovannetti, M., et al. (2017). Functional identity has a stronger effect than diversity on mycorrhizal symbiosis and productivity of field grown organic tomato. Euro. J. Agron. 86, 1–11. doi: 10.1016/j.eja.2017.02.007
Njeru, E. M., Muthini, M., Muindi, M. M., Ombori, O., Nchore, S. B., Runo, S., et al. (2020). “Exploiting arbuscular mycorrhizal fungi-rhizobia-legume symbiosis to increase smallholder farmers' crop production and resilience under a changing climate,” in Climate Impacts on Agricultural and Natural Resource Sustainability in Africa, eds B. Singh, A. Safalaoh, N. Amuri, L. Eik, B. Sitaula, and R. Lal (Cham: Springer), 471–488. doi: 10.1007/978-3-030-37537-9_27
Novo, L. A. B., Castro, P. M. L., Alvarenga, P., and da Silva, E. F. (2018). “Plant growth-promoting rhizobacteria-assisted phytoremediation of mine soils,” in Bio-Geotechnologies for Mine Site Rehabilitation, eds M.N.V. Prasad, P.J. de Campos Favas, and S.K. Maiti (Amsterdam: Elsevier Inc.), 281–295. doi: 10.1016/B978-0-12-812986-9.00016-6
Nuzzo, A., Satpute, A., Albrecht, U., and Strauss, S. L. (2020). Impact of soil microbial amendments on tomato rhizosphere microbiome and plant growth in field soil. Microbiol. Ecol. 80, 398–409. doi: 10.1007/s00248-020-01497-7
Nyambo, D. G., Luhanga, E. T., and Yonah, Z. Q. (2019). A review of characterization approaches for smallholder farmers: towards predictive farm typologies. Sci. World J. 2019:6121467. doi: 10.1155/2019/6121467
Nyamwange, M. M., Njeru, E. M., Mucheru-Muna, M., and Ngetich, F. (2018). Soil management practices affect arbuscular mycorrhizal fungi propagules, root colonization and growth of rainfed maize. AIMS Agric. Food 3, 120–134. doi: 10.3934/agrfood.2018.2.120
Odame, H. (1997). Biofertilizer in Kenya: Research, production and extension dilemmas. Biotechnol. Dev. Mon. 30, 20–23. Available online at: http://www.biotech-monitor.nl/3009.htm
Orrell, P., and Bennett, A. E. (2013). How can we exploit above-belowground interactions to assist in addressing the challenges of food security? Front. Plant Sci. 4:432. doi: 10.3389/fpls.2013.00432
Oruru, M. B., and Njeru, E. M. (2016). Upscaling arbuscular mycorrhizal symbiosis and related agroecosystems services in smallholder farming systems. BioMed Res. Int. 2016, 1–12. doi: 10.1155/2016/4376240
Ouma, E. W., Asango, A. M., Maingi, J., and Njeru, E. M. (2016). Elucidating the potential of native rhizobial isolates to improve biological nitrogen fixation and growth of common bean and soybean in smallholder farming systems of Kenya. Int. J. Agronomy. 2016:4569241. doi: 10.1155/2016/4569241
Panwar, V., Aggarwal, A., Singh, G., Saharan, M. S., Verma, A., Sharma, I., et al. (2014). Efficacy of foliar spray of Trichoderma isolates against Fusarium graminearum causing head blight of wheat. J. Wheat Res. 6, 59–63. Available online at: http://epubs.icar.org.in/ejournal/index.php/JWR
Parani, K., and Saha, B. K. (2012). Prospects of using phosphate solubilizing pseudomonas as bio fertilizer. Eur. J. Biol. Sci. 4, 40–44. doi: 10.5829/idosi.ejbs.2012.4.2.63117
Park, J. M., Radhakrishnan, R., Kang, S. M., and Lee, I. J. (2015). IAA producing Enterobacter sp. I-3 as a potent bio-herbicide candidate for weed control: a special reference with lettuce growth inhibition. Ind. J. Microbiol. 55, 207–212. doi: 10.1007/s12088-015-0515-y
Park, Y. G., Mun, B. G., Kang, S. M., Hussain, A., Shahzad, R., Seo, C. W., et al. (2017). Bacillus aryabhattai SRB02 tolerates oxidative and nitrosative stress and promotes the growth of soybean by modulating the production of phytohormones. PLoS ONE 12:e0173203. doi: 10.1371/journal.pone.0173203
Pellegrino, E., Turrini, A., Gamper, H. A., Caf,à, G., Bonari, E., Young, J. P. W., et al. (2012). Establishment, persistence and effectiveness of arbuscular mycorrhizal fungal inoculants in the field revealed using molecular genetic tracing and measurement of yield components. New Phytol. 194, 810–822. doi: 10.1111/j.1469-8137.2012.04090.x
Pérez-Escamilla, R. (2017). Food security and the 2015–2030 sustainable development goals: from human to planetary health. Curr. Dev. Nutr. 1:e000513. doi: 10.3945/cdn.117.000513
Piromyou, P., Buranabanyat, B., Tantasawat, P., Tittabutr, P., Boonkerd, N., and Teaumroong, N. (2011). Effect of plant growth promoting rhizobacteria (PGPR) inoculation on microbial community structure in rhizosphere of forage corn cultivated in Thailand. Euro. J. Soil Biol. 47, 44–54. doi: 10.1016/j.ejsobi.2010.11.004
Preininger, C., Sauer, U., Bejarano, A., and Berninger, T. (2018). Concepts and applications of foliar spray for microbial inoculants. Appl. Microbiol. Biotechnol. 102, 7265–7282. doi: 10.1007/s00253-018-9173-4
Premachandra, D., Hudek, L., Enez, A., Ballard, R., Barnett, S., Franco, C. M. M., et al. (2020). Assessment of the capacity of beneficial bacterial inoculants to enhance canola (brassica napus l.) growth under low water activity. Agronomy 10:1449. doi: 10.3390/agronomy10091449
Raaijmakers, J. M., Vlami, M., and de Souza, J. T. (2002). Antibiotic production by bacterial biocontrol agents. Antonie Leeuwenhoek. 81, 537–547. doi: 10.1023/A:1020501420831
Raimi, A., Adeleke, R., and Roopnarain, A. (2017). Soil fertility challenges and Biofertiliser as a viable alternative for increasing smallholder farmer crop productivity in sub-Saharan Africa. Cogent Food Agric. 3:1400933. doi: 10.1080/23311932.2017.1400933
Raimi, A., Ezeokoli, O., and Adeleke, R. (2019). High-throughput sequence analysis of bacterial communities in commercial biofertiliser products marketed in South Africa: an independent snapshot quality assessment. 3 Biotech 9:108. doi: 10.1007/s13205-019-1643-6
Rashid, M. I., Mujawar, L. H., Shahzad, T., Almeelbi, T., Ismail, I. M. I., and Oves, M. (2016). Bacteria and fungi can contribute to nutrients bioavailability and aggregate formation in degraded soils. Microbiol. Res. 183, 26–41. doi: 10.1016/j.micres.2015.11.007
Reddy, C. A., and Saravanan, R. S. (2013). Polymicrobial multi-functional approach for enhancement of crop productivity. Adv. Appl. Microbiol. 82, 53–113. doi: 10.1016/B978-0-12-407679-2.00003-X
Rivera, J. D., Lemus, R. W., Gipson, M. L., and Gipson, R. G. (2015). Effects of polymicrobial bioinoculant on yield, quality and in situ digestibility of sorghum sudangrass in south mississippi cover crops. Agrotechnology 5:1. doi: 10.4172/2168-9881.1000136
Rouphael, Y., and Colla, G. (2020). Editorial: biostimulants in agriculture. Front. Plant Sci. 11:40. doi: 10.3389/fpls.2020.00040
Rowe, H., Brown, C., and Claassen, V. P. (2007). Comparisons of mycorrhizal responsiveness with field soil and commercial inoculum for six native montane species and bromus tectorum. Restor. Ecol. 15, 44–52. doi: 10.1111/j.1526-100X.2006.00188.x
Saad, M. M., Eida, A. A., and Hirt, H. (2020). Tailoring plant-associated microbial inoculants in agriculture: a roadmap for successful application. J. Exp. Bot. 71, 3878–3901. doi: 10.1093/jxb/eraa111
Salem, A. A. (2016). Potential acting of a novice Achromobacter xylosoxidans strain as a plant growth-promoting factor. Ann. Agric. Sci. 54, 63–76. doi: 10.21608/assjm.2016.103911
Sanchis, V. (2011). From microbial sprays to insect-resistant transgenic plants: History of the biospesticide Bacillus thuringiensis: A review. Agron. Sust. Dev. 31, 217–231. doi: 10.1051/agro/2010027
Sandhya, V., Ali, S. Z., Grover, M., Reddy, G., and Venkateswarlu, B. (2010). Effect of plant growth promoting Pseudomonas spp. on compatible solutes, antioxidant status and plant growth of maize under drought stress. Plant Growth Regul. 62, 21–30. doi: 10.1007/s10725-010-9479-4
Sangeeth, K. P., Bhai, R. S., and Srinivasan, V. (2012). Paenibacillus glucanolyticus, a promising potassium solubilizing bacterium isolated from black pepper (Piper nigrum L.) rhizosphere. J. Spices Aromat Crops 21, 118–124. Available online at: www.indianspicesociety.in/josac/index.php/josac
Santos, M. S., Nogueira, M. A., and Hungria, M. (2019). Microbial inoculants: reviewing the past, discussing the present and previewing an outstanding future for the use of beneficial bacteria in agriculture. AMB Express 9, 1–13. doi: 10.1186/s13568-019-0932-0
Saravanakumar, D., and Samiyappan, R. (2007). ACC deaminase from pseudomonas fluorescens mediated saline resistance in groundnut (Arachis hypogea) plants. J. Appl. Microbiol. 102, 1283–1292. doi: 10.1111/j.1365-2672.2006.03179.x
Sarwar, M. (2015). Biopesticides: an effective and environmental friendly insect-pests inhibitor line of action. Int. J. Eng. Adv. Res. Technol. 1, 10–15. Available online at: http://www.academia.edu/download/49520237/IJEART01108.pdf
Saxena, A. K., Pal, K. K., and Tilak, K. V. B. R. (2000). “Bacterial biocontrol agents and their role in plant disease management,” in Biocontrol Potential and its Exploitation in Sustainable Agriculture, eds R.K. Upadhyay, K.G. Mukerji, and B.P. Chamola (Boston MA: Springer), 25–37. doi: 10.1007/978-1-4615-4209-4_3
Schütz, L., Gattinger, A., Meier, M., Müller, A., Boller, T., Mäder, P., et al. (2018). Improving crop yield and nutrient use efficiency via biofertilization—a global meta-analysis. Front. Plant Sci. 8:2204. doi: 10.3389/fpls.2017.02204
Schwartz, M. W., Hoeksema, J. D., Gehring, C. A., Johnson, N. C., Klironomos, J. N., Abbott, L. K., et al. (2006). The promise and the potential consequences of the global transport of mycorrhizal fungal inoculum. Ecol. Lett. 9, 501–515. doi: 10.1111/j.1461-0248.2006.00910.x
Selvi, K., Paul, J., Vijaya, V., and Saraswathi, K. (2017). Analyzing the efficacy of phosphate solubilizing microorganisms by enrichment culture techniques. Biochem. Mol. Biol. J. 3:1. doi: 10.21767/2471-8084.100029
Seraj, M. F., Rahman, T., Lawrie, A. C., and Reichman, S. M. (2020). Assessing the plant growth promoting and arsenic tolerance potential of Bradyrhizobium japonicum CB1809. Environ. Manage. 66, 930–939. doi: 10.1007/s00267-020-01351-z
Shanafelt, D. W., Dieckmann, U., Jonas, M., Franklin, O., Loreau, M., and Perrings, C. (2015). Biodiversity, productivity, and the spatial insurance hypothesis revisited. J. Theor. Biol. 380, 426–435. doi: 10.1016/j.jtbi.2015.06.017
Sharma, S., Gupta, R., Dugar, G., and Srivastava, A. K. (2012). “Impact of application of biofertilizers on soil structure and resident microbial community structure and function,” in Bacteria in Agrobiology: Plant Probiotics, ed D. Maheshwari (Berlin: Springer), 65–77. doi: 10.1007/978-3-642-27515-9_4
Sharma, S. B., Sayyed, R. Z., Trivedi, M. H., and Gobi, T. A. (2013). Phosphate solubilizing microbes: Sustainable approach for managing phosphorus deficiency in agricultural soils. SpringerPlus. 2:587. doi: 10.1186/2193-1801-2-587
Sileshi, G. W., Teketay, D., Gebrekirstos, A., and Hadgu, K. (2020). “Sustainability of faidherbia albida-based agroforestry in crop production and maintaining soil health,” in Agroforestry for Degraded Landscapes, eds J.C. Dagar, S.R. Gupta, and D. Teketay (Singapore: Springer), 349–369. doi: 10.1007/978-981-15-6807-7_12
Sindhu, S., Dahiya, A., Gera, R., and Sindhu, S. S. (2020). Mitigation of abiotic stress in legume-nodulating rhizobia for sustainable crop production. Agric. Res. 9, 444–459. doi: 10.1007/s40003-020-00474-3
Singh, B. K., and Trivedi, P. (2017). Microbiome and the future for food and nutrient security. Microbiol. Biotechnol. 10, 50–53. doi: 10.1111/1751-7915.12592
Singh, S. K., Pathak, R., and Pancholy, A. (2017). Role of Root Nodule Bacteria in Improving Soil Fertility and Growth Attributes of Leguminous Plants Under Arid and Semiarid Environments. Cham: Springer. doi: 10.1007/978-3-319-64982-5_4
Sinong, G. F., Yasuda, M., Nara, Y., Lee, C. G., Dastogeer, K. M. G., Tabuchi, H., et al. (2021). Distinct root microbial communities in nature farming rice harbor bacterial strains with plant growth-promoting traits. Front. Sust. Food Syst. 4:629942. doi: 10.3389/fsufs.2020.629942
Song, Y., Liu, J., and Chen, F. (2020). Azotobacter chroococcum inoculation can improve plant growth and resistance of maize to armyworm, MYTHIMNA separata even under reduced nitrogen fertilizer application. Pest Manage. Sci. 76, 4131–4140. doi: 10.1002/ps.5969
Soumare, A., Boubekri, K., Lyamlouli, K., Hafidi, M., Ouhdouch, Y., and Kouisni, L. (2020). From isolation of phosphate solubilizing microbes to their formulation and use as biofertilizers: status and needs. Front. Bioeng. Biotechnol. 7:425. doi: 10.3389/fbioe.2019.00425
Sousa, R. M. S., Mendes, L. W., Antunes, J. E. L., Oliveira, L. M., de, S., Sousa, A. M. de, C. B., et al. (2020). Diversity and structure of bacterial community in rhizosphere of lima bean. Appl. Soil Ecol. 102:103490. doi: 10.1016/j.apsoil.2019.103490
Stamenković, S., Beškoski, V., Karabegović, I., Lazić, M., and Nikolić, N. (2018). Microbial fertilizers: A comprehensive review of current findings and future perspectives. Span. J. Agric. Res. 16:2192. doi: 10.5424/sjar/2018161-12117
Sun, X. G., and Tang, M. (2013). Effect of arbuscular mycorrhizal fungi inoculation on root traits and root volatile organic compound emissions of Sorghum bicolor. South Afr. J. Bot. 88, 373–379. doi: 10.1016/j.sajb.2013.09.007
Swain, H., Adak, T., Mukherjee, A. K., Mukherjee, P. K., Bhattacharyya, P., Behera, S., et al. (2018). Novel Trichoderma strains isolated from tree barks as potential biocontrol agents and biofertilizers for direct seeded rice. Microbiol. Res. 214, 83–90. doi: 10.1016/j.micres.2018.05.015
Tabassum, B., Khan, A., Tariq, M., Ramzan, M., Iqbal Khan, M. S., Shahid, N., et al. (2017). Bottlenecks in commercialisation and future prospects of PGPR. Appl. Soil Ecol. 121, 102–117. doi: 10.1016/j.apsoil.2017.09.030
Tapsoba, P. K., Aoudji, A. K. N., Kabore, M., Kestemont, M. P., Legay, C., and Achigan-Dako, E. G. (2020). Sociotechnical context and agroecological transition for smallholder farms in benin and Burkina Faso. Agronomy 10, 1–34. doi: 10.3390/agronomy10091447
Tewari, S., Shrivas, V. L., Hariprasad, P., and Sharma, S. (2019). “Harnessing endophytes as biocontrol agents,” in Plant Health Under Biotic Stress (Sigapore: Springer), 189–218. doi: 10.1007/978-981-13-6040-4_10
Thakur, D., Kaushal, R., and Shyam, V. (2014). Phosphate solubilising microorganisms: role in phosphorus nutrition of crop plants-A review. Agric. Rev. 35:159. doi: 10.5958/0976-0741.2014.00903.9
Velázquez-Hernández, M. L., Baizabal-Aguirre, V. M., Cruz-Vázquez, F., Trejo-Contreras, M. J., Fuentes-Ramírez, L. E., Bravo-Patiño, A., et al. (2011). Gluconacetobacter diazotrophicus levansucrase is involved in tolerance to NaCl, sucrose and desiccation, and in biofilm formation. Arch. Microbiol. 193, 137–149. doi: 10.1007/s00203-010-0651-z
Velivelli, S. L. S., Sessitsch, A., and Prestwich, B. D. (2014). The role of microbial inoculants in integrated crop management systems. Potato Res. 57, 291–309. doi: 10.1007/s11540-014-9278-9
Ventorino, V., De Marco, A., Pepe, O., Virzo, A., Santo, D., and Moschetti, G. (2012). “Impact of innovative agricultural practices of carbon sequestration on soil microbial community,” in Carbon Sequestration in Agricultural Soils, ed A. Piccolo (Berlin: Springer), 145–177. doi: 10.1007/978-3-642-23385-2_6
Wahid, F., Fahad, S., Danish, S., Adnan, M., Yue, Z., Saud, S., et al. (2020). Sustainable management with mycorrhizae and phosphate solubilizing bacteria for enhanced phosphorus uptake in calcareous soils. Agriculture 10, 1–14. doi: 10.3390/agriculture10080334
Wang, L., Zhang, L., Liu, Z., Zhao, D., Liu, X., Zhang, B., et al. (2013). “A minimal nitrogen fixation gene cluster from Paenibacillus sp. WLY78 enables expression of active nitrogenase in Escherichia coli. PLoS Genet. 9, 1–10. doi: 10.1371/annotation/1e9bcb70-265a-4383-abf4-3466d144d56e
Wei, C. Y., Lin, L., Luo, L. J., Xing, Y. X., Hu, C. J., Yang, L. T., et al. (2014). Endophytic nitrogen-fixing Klebsiella variicola strain DX120E promotes sugarcane growth. Biol. Fertil. Soils 50, 657–666. doi: 10.1007/s00374-013-0878-3
Wu, S. C., Cao, Z. H., Li, Z. G., Cheung, K. C., and Wong, M. H. (2005). Effects of biofertilizer containing N-fixer, P and K solubilizers and AM fungi on maize growth: a greenhouse trial. Geoderma 125, 155–166. doi: 10.1016/j.geoderma.2004.07.003
Yachi, S., and Loreau, M. (1999). Biodiversity and ecosystem productivity in a fluctuating environment: the insurance hypothesis. Proc. Natl. Acad. Sci. 96, 1463–1468. doi: 10.1073/pnas.96.4.1463
Yeager, C. M., Northup, D. E., Grow, C. C., Barns, S. M., and Kuske, C. R. (2005). Changes in nitrogen-fixing and ammonia-oxidizing bacterial communities in soil of a mixed conifer forest after wildfire. Appl. Environ. Microbiol. 71, 2713–2722. doi: 10.1128/AEM.71.5.2713-2722.2005
Zhang, F., Yuan, J., Yang, X., Cui, Y., Chen, L., Ran, W., et al. (2013). Putative Trichoderma harzianum mutant promotes cucumber growth by enhanced production of indole acetic acid and plant colonization. Plant Soil 368, 433–444. doi: 10.1007/s11104-012-1519-6
Keywords: plant growth promoting microorganisms, biocontrol agents, microbial inoculants, smallholder agroecosystems, soil fertility, food security
Citation: Koskey G, Mburu SW, Awino R, Njeru EM and Maingi JM (2021) Potential Use of Beneficial Microorganisms for Soil Amelioration, Phytopathogen Biocontrol, and Sustainable Crop Production in Smallholder Agroecosystems. Front. Sustain. Food Syst. 5:606308. doi: 10.3389/fsufs.2021.606308
Received: 14 September 2020; Accepted: 30 March 2021;
Published: 29 April 2021.
Edited by:
Duraisamy Saravanakumar, The University of the West Indies St. Augustine, Trinidad and TobagoReviewed by:
Naeem Khan, University of Florida, United StatesPraveen Guleria, DAV University, India
Copyright © 2021 Koskey, Mburu, Awino, Njeru and Maingi. This is an open-access article distributed under the terms of the Creative Commons Attribution License (CC BY). The use, distribution or reproduction in other forums is permitted, provided the original author(s) and the copyright owner(s) are credited and that the original publication in this journal is cited, in accordance with accepted academic practice. No use, distribution or reproduction is permitted which does not comply with these terms.
*Correspondence: Ezekiel Mugendi Njeru, bmplcnUuZXpla2llbEBrdS5hYy5rZQ==