- 1Laboratory of Biology and Molecular Typing in Microbiology, Department of Biochemistry and Cell Biology, Faculty of Sciences and Techniques, University of Abomey-Calavi, Cotonou, Benin
- 2Food Security and Safety Niche, Faculty of Natural and Agricultural Sciences, North-West University, Mmabatho, South Africa
- 3Biomathematics and Forest Estimation Laboratory, Faculty of Agronomic Sciences, University of Abomey-Calavi, Cotonou, Benin
- 4Scientific Management Department of the Institute, National Institute of Agricultural Research of Benin, Cotonou, Benin
Maize plays an important role in agricultural production systems in all agro-ecological zones of Benin. Despite its importance, its production faces many constraints including soil fertility. One of the ecological technologies aimed at improving agricultural production is the use of soil microorganisms including arbuscular mycorrhizal fungi (AMF). This study aims to evaluate the effectiveness of Rhizophagus intraradices, an indigenous strain, on maize productivity in farmers' areas in the Research and Development (RD) sites of the North (Ouénou), Center (Miniffi), and South (Zouzouvou). Three maize producers were selected at each RD site, for nine maize producers. The experimental design was a randomized complete block of three treatments with three replications. The different treatments were (i) Control–farmer's practice, (ii) R. intraradices + 50% of the recommended dose of NPK and urea, and (iii) 100% of the recommended dose of NPK and urea. Soil samples from the different RD sites were taken at a depth of 0–20 cm before sowing for chemical analysis. The different growth parameters (height, crown diameter, and leaf area), grain yield, and endomycorrhizal infection of maize plants were evaluated. The results showed that the soils were moderately acidic (5.5 ≤ pH waters ≤ 6.8) and low in organic matter (0.95 ≤ 33 OM ≤ 1.17) regardless of the study area. The greater maize grain yield was recorded with application of 100% of the recommended dose of NPK and urea, and R. intraradices + 50% of the recommended dose of NPK and urea. In the RD sites at the South, Center, and North recorded with R. intraradices + 50% of recommended dose of NPK and urea, the grain yields of 1.9, 3.4, and 1.74 t/ha with an increase of 28, 38.21, and 13.21%, respectively, compared with farmer's practice. Mycorrhization frequencies in plants treated with Ri½ N15P15K15 vary between 37.44 and 51.67% in the three zones. The results of the current study have proven the potential use of R. intraradices in sustainable intensification of maize production in Benin.
Introduction
The decadent productivity of agricultural speculation, in general, and that of maize, in particular, in Benin, a West African country, could be justified by certain constraints including climate change, declining soil fertility illustrated by nitrogen and phosphorus deficiencies (Benbrahim et al., 2004; Balogoun et al., 2014) caused by poor farming practices such as slash-and-burn agriculture. In tropical and subtropical countries, phosphorus is essential because of its natural unavailability in soils (Hailemariam et al., 2013), and nitrogen (N) is a limiting factor for cereal crops (Batamoussi et al., 2014). In this context, the satisfaction of food needs, in general, and maize demands, in particular, will have to be based on productivity improvement. Thus, producers resort to intensive use of inputs, especially mineral fertilizers, which are leached and easily diluted into rivers, lakes, and streams with adverse consequences on the environment and the health of humans and animals. According to Alalaoui (2007), the prolonged use of mineral fertilizers without organic inputs leads to the depletion of soil organic matter, which is more sensitive to wind and rain erosion.
For these reasons, it is imperative to have an understanding of the processes underlying the bioavailability of soil nutrients to plants, as well as the soil–root interactions of microorganisms (Eisenhauer, 2017; Bi et al., 2018). One of the oldest and most widespread mutualistic associations of microorganisms concern symbiosis in which a particular soil fungi called arbuscular mycorrhizal fungi (AMF) colonize the roots of most (74%) of the terrestrial plant families (van der Heijden et al., 2015). These fungi belong to the family of glomeromycetes, which includes at least 313 characterized species. AMF are key elements of soil fertility (Bedini et al., 2018). Several examples suggest the use of AMF for the promotion of plant performance (growth, survival, and tolerance) because they improve nutrition (water and minerals), photosynthesis, protection against biotic and abiotic stresses, regulation of development processes (flowering, fruit formation, rooting, etc.), (Bedini et al., 2018), and participate in soil structuring (Alqarawi et al., 2014). However, the wide use of mycorrhizal inocula in agriculture remains a challenge due to their cost, variability, quality, and effects on the plant such as their incompatibility with high levels of phosphorus (P) in the soil (Usharani et al., 2014; Berruti et al., 2016).
However, knowledge and understanding of the mechanisms that govern the functioning of these AMF communities, particularly in poor tropical agrosystems where sustainable management of generally low soil nutrient resources, must take into account the benefits of indigenous microorganisms. Fortunately, with the advent of molecular biology, considerable advances in AMF identification and nomenclature have been noted (Oehl et al., 2011).
Although the importance of intraspecific plant diversity and AMF for ecosystem functioning has often been highlighted (Wall et al., 2015), the interactive influences on their respective and reciprocal performance are still not well-understood (Sendek et al., 2019).
Although work on AMF diversity and use is not legion in Benin (Tchabi et al., 2008; Balogoun et al., 2014), it is, nonetheless, oriented toward speculation other than maize in small geographical study areas and has mainly concerned exotic strains of AMF. However, Benin has indigenous strains of AMF in regions characterized by different types of climate and soils from North to South through the center and which could each present significant specificity to be taken into account in the analyses. The objective of this study is to evaluate the effect of the fungus Rhizophagus intraradices on maize growth and yield at three Research and Development (RD) sites in Benin.
Materials and Methods
Materials
The maize variety 2,000 SYNEE-W was used during the experimentation at the different sites where the trials were carried out. It is an extra-early variety, 75 days old, developed by the International Institute of Tropical Agriculture (IITA) and the Institut National des Recherches Agricoles du Bénin (INRAB). It is a variety that presents a good resistance to the rot of the stem, to the maize streak virus (MSV) of the Mastrevirus genus, to the American rust, to the helminthosporiose caused by the Cochliobolus heterostrophus fungus. In addition, it tolerates drought (MAEP, 2016).
The mycorrhizal inoculum of the Rhizophagus intraradices species used was isolated, identified, and characterized on the basis of morphological criteria (diameter, color, ornamentation, thickness of the spore wall) from the rhizosphere of maize soils in different agro-ecological zones of Benin (Aguégué et al., 2021). This mychorizial fungus was preserved at the Laboratory of Biology and Molecular Typing in Microbiology of Faculty of Sciences and Techniques of University of Abomey-Calavi (FAST/UAC). The inocula were produced and multiplied by associating spores of Rhizophagus intraradices with sorghum seedlings. Sorghum seeds were disinfected in a bleach solution (5%), then rinsed and soaked in sterile distilled water for 24 h. Sorghum plants were grown in glasshouses in pots containing sterilized substrate consisting of a mixture of clay and peat (2:1 v/v), for 4 months to ensure good sporulation of the strains. After 4 months of cultivation, the inoculum, consisting of spores and root fragment mixture, was collected (Rivera et al., 2003).
Study Area
The tests were installed on Research and Development sites respectively in the South at Zouzouvou (Commune of Djakotomey), in the Center at Miniffi (Commune of Dassa) and in the North at Ouénou (Commune of N'Dali), (Figure 1). In each of the zones, the trials were set up at three (3) different producers. The choice of sites was made taking into account the fact that they are the sites of Research and Development programs and that declining soil fertility is a priority constraint. The sites are flat with a maximum 2% slope and are not flooded.
Determination of Soil Chemical Parameters
Soil samples were taken at a depth of 0–20 cm (Adjanohoun et al., 2011) at various sites in the South (Zouzouvou), Center (Miniffi), and North (Ouénou). A 500-g composite sample was collected at each site prior to the installation of the experimental device. At each site, a mixture of soil samples was collected using an auger at a depth of 0–20 cm. Five (05) sampling points were randomly selected. Four (04) of the five (5) sampling points are each located on the four cardinal points (North–South–West–East). The fifth sampling point is located approximately at the junction of the four (04) preceding points. These samples were sent to the Laboratoire des Sciences du Sol, Eau et Environment (LSSEE) of the Institut National des Recherches Agricoles du Bénin (INRAB). These analyses consisted of the determination of pH (water), (by glass electrode in a soil/water ratio of 1/2.5), organic matter and carbon (Walkley and Black, 1934), assimilable phosphorus (Bray and Kurtz, 1945), total nitrogen (Kjedahl, 1883), and exchangeable bases by the method of Metson (1957) with ammonium acetate at a pH equal to 7.
Experimental Device
The plowing was done at a depth of 20 cm using a hoe on each site. Each 12.8 m2 (4 × 3.2 m) elementary plot had four lines of 4-m long. The trials were installed at three growers on each site. At each grower, the experimental design used was a completely randomized block of three treatments with three replicates. Each treatment had, thus, covered three elementary plots (1 plot × 3 replicates) separated by alleys 1-m wide. The treatments were defined as follows:
- T1: Farmer's practice (control);
- T2: Ri½ N15P15K15_Urea (R. intraradices + 50% of the recommended dose of NPK and urea);
- T3: N15P15K15_Urea (100% of the recommended dose of NPK and urea).
Farmer's practice technique in the present study is characterized by the application of the recommended dose of mineral fertilizer N15P15K15 on the 15th day after sowing and urea on the 45th day after sowing. However, for treatments T2 and T3, the mineral fertilizer N15P15K15 was applied on the day of sowing, and urea was applied on the 45th day after sowing. The recommended rate of mineral fertilizer for the maize crop used in this study is 200 kg ha−1 of N15P15K15 and 100 kg ha−1 of urea (46% N).
Sowing and Inoculation of Maize Seeds
Before sowing, the maize seeds were coated with the inoculum of Rhizuphagus intraradices. Seed coating was done according to the methodology described by Fernandez et al. (2000). A 1 kg of the inoculum was mixed with 600 ml of distilled water to obtain a paste to which 10 kg of seeds were added for mixing. The coated seeds were dried at room temperature for 12 h. Sowing was done in plots about 5-cm deep at a spacing of 0.80 × 0.40 m, i.e., a density of 31,250 plants/ha (Hernandez et al., 1995). On the day of sowing, a bottom manuring at the rate of 200 kg/ha of fertilizer (N15P15K15) was applied in accordance with the experimental protocol. Then, on the 40th day after sowing (DAS), maintenance manure consisting of urea (40% N) was applied in accordance with the experimental design. Data for the various growth, yield, and mycorrhization parameters were collected on the two central lines of the 6.4-m2 working plot at each site.
Evaluation of Growth Parameters
The height of the maize plants and the diameter at the collar of the plants were collected on 12 plants from the two central lines of each elementary plot, every 15 days from DAS until the 60th DAS on the different RD sites. The height of a maize plant was measured with a graduated ruler, the diameter was measured with a caliper at the plant collar, and the leaf area was estimated at the 60th DAS by the product of the length and width of the leaves multiplied by 0.75 (Ruget et al., 1996).
Evaluation of Grain Yield
Corn grain yield was assessed at harvest (80th DAS). Corn cobs were harvested, dispatched, and shelled per plant and per elementary plot. Grain moisture percentage was determined using a moisture meter (LDS-1F). The average grain yield values of the maize plants were determined by Equation 1 used by (Ferro Valdés et al. 2013):
Where,
R = the average maize grain yield in t ha−1;
P = the maize grain weight in kilograms (kg);
S = the harvest area in m2, and
% H = the grain moisture content in %.
Evaluation of Endo Mycorrhizal Infection of Maize Plant Roots
Corn root samples were collected at the 80th DAS. After staining with trypan blue according to the method described by Phillips and Hayman (1970), arbuscular mycorrhizal fungi associated with maize plant roots were observed with binoculars (XSP-BM-2CEA. 2013). Estimation of mycorrhizal infection of roots was carried out according to the intersection method described by Giovanetti and Mosse (1980). The rate of mycorrhization was estimated by two parameters of arbuscular mycorrhizal infection described by Trouvelot et al. (1986), namely: (i) mycorrhization frequency (F), which reflects the degree of infection of the root system, and (ii) mycorrhization intensity or absolute mycorrhization intensity (m), which expresses the portion of the colonized cortex in relation to the entire root system, calculated according to Equations 2, 3, respectively.
where
N is the number of fragments observed and
no is the number of fragments with no trace of mycorhization;
In Equation 3, n5, n4, n3, n2, and n1 are the numbers of fragments, respectively, noted in the five classes of infection marking the importance of mycorrhization, namely, 5: more than 95%; 4: from 50 to 95%; 3: 30 to 50%; 2: 1 to 30%; 1: 1% of the cortex.
Statistical Analysis
Data per site for all parameters assessed were collected. Mixed-effect linear models on longitudinal data were fitted to evaluate the effect of treatments and area on plant growth parameters (height and crown diameter). In each model, treatments and zones were considered fixed factors, and time was considered random. Adjusted averages were also calculated to represent trends in each growth parameter at the treatment and zone level. These analyses were performed using the nlme (for model fitting), and it means (for the calculation of adjusted averages) packages. Descriptive statistics were calculated for each growth parameter measured.
In order to assess the effect of treatments and zone on yield and leaf area, it was evaluated using a two-criteria analysis of variance (treatment and zone). The Shapiro-Wilk and Levene tests (Glèlè Kakaï et al., 2006) were performed to verify the conditions of normality and homoscedasticity of the data required for ANOVA. As the experimental design was balanced, the ANOVA type II test was adopted. Once the ANOVA test was significant, a pairwise comparison post-hoc test using the Tuckey's post-hoc test (Douglas and Fligner, 1991) was performed to assess the statistical differences in the means. In addition, descriptive statistics were calculated for each measured parameter. These analyses required the use of the dplyr and DescTools packages for the calculation of descriptive statistics, the ggplot2, and ggpur packages for the mustache boxes, the stats package for the Shapiro test and Levene test, the car package for the ANOVA, and the multcomp package for the pairwise comparison post-hoc test.
The significance threshold retained is 5%, and all the different tests were performed in R 4.0.2 software (R Core Team, 2020).
Results
Chemical Characteristics of the Soil
The soil chemistry characteristics of the R&D Sites are presented in Table 1. The soil water pH in Zouzouvou (pH = 5.6), Ouénou (pH = 5.5), and Minifi (pH = 6.2) is acidic. Organic matter varies between 0.95 and 1.17%, while assimilable phosphorus has a respective value of 2 ppm at Zouzouvou, 11.7 ppm at Miniffi, and 6.9 ppm at Ouénou. Exchangeable bases vary between 1.82 and 7.84 meq/100 g of soil.
Effect of the Mycorrhizal Fungus Rhizophagus intradices on the Growth of Maize Seedlings
The results of the analysis of variance show that the height variations observed at the plant level depend only on the area (p-value < 0.0001) and over time (Table 2).
The evolution of these average plant heights over time and by treatment are presented graphically by zone (Figure 2). From the figure, it appears that whatever the zone, the growth in height of the plants reaches its maximum around 60 days after sowing. The highest maize plant heights were observed in the south regardless of treatment. However, in the Center and North, the best heights were observed, respectively, at the level of plants treated with Ri½ N15P15K15_Urea (T2) and N15P15K15_Urea (T3), (Figure 2).
The results of the analysis of variance show that the variations in collar diameter observed at the plant level depend on the treatments (p-value < 0.001) and on the zone (p-value = 0.016). However, these variations over time do not depend on the treatments (p-value = 0.751) but rather on the zone (p-value < 0.001), (Table 2).
The curves of the evolution of the diameter at the collar of the plants over time and by treatment show these variations by zone (Figure 3). Thus, in general, and whatever the zone, the growth in diameter of the plants reaches its maximum around 60 days after sowing. The treatment Ri½ N15P15K15_Urea (T2) followed by N15P15K15_Urea (T3) induced the largest collar diameters (Figure 3) in the Center, while the largest collar diameter values were recorded with treatments Farmer's practice (T1) and N15P15K15_Urea (T3) in the South and North, respectively.
From the analysis in Figure 4, it appears that the plants in the southern zone perform best for most treatments. The results of the analysis of variance show a significant difference in the effects of the interaction between treatment and zone (Df = 8, p-value = < 0.001) on the leaf area of the plants. It can be deduced that the variation in plant leaf area per treatment depends on the experimental area. The Tuckey's test performed (Figure 4) shows the difference in performance between the treatments according to each zone. Thus, the treatment N15P15K15_Urea (T3) in the South zone gives the best performance. Next comes the treatment Ri½ N15P15K15_Urea (T2) and treatment Farmer's practice (T1) in the southern zone, followed by the treatment N15P15K15_Urea (T3) in the northern zone. Treatments Ri½ N15P15K15_Urea (T2), and Farmer's practice (T1) in the northern zone have similar performances as the treatment N15P15K15_Urea (T3) in the central zone. The treatment Farmer's practice (T1) in the central zone, gives a low performance.
Effect of the Mycorrhizal Fungus Rhizophagus intradices on Maize Grain Yield
The analysis in Figure 5 shows that the plants in the Southern and Central zone perform best for most treatments. The results of the analysis of variance show a significant difference in the effects of the interaction between treatment and zone (Df = 8, p-value = < 0.001) on plant grain yield. This suggests that the variation in plant grain yield per treatment depends on the experimental area. The Tuckey's test performed (Figure 5) shows the difference in performance between the treatments according to each zone. Thus, the treatment N15P15K15_Urea (T3) in the South and Central zone gives the best performance (3.3 t ha−1). Next comes the treatment Ri½ N15P15K15_Urea (T2), (2.8 t ha−1) and the treatment Farmer's practice (T1) in the South and Central zones. The treatment Ri½ N15P15K15_Urea (T2) in the North zone has similar performances as the treatment N15P15K15_Urea (T3). Of all the zones, the treatment Farmer's practice (T1) in the North zone gives the lowest performance (1.6 t ha−1).
Effect of the Mycorrhizal Fungus Rhizophagus intradices on the Mycorrhization of Maize Plant Roots
Mycorhization frequencies in plants treated with the treatment Ri½ N15P15K15_Urea (T2) vary between 37.44 and 51.67% in the three zones (Figure 6). Mycorhization intensity varies between 6.19 and 27.02%. It should be noted, however, that we did not observe mycorhization at the root level of the plants treated with the treatment Farmer's practice (T1) and the plants treated with the treatment N15P15K15_Urea (T3). The results of the analysis of variance of mycorhization intensity and frequency in the three study areas revealed a significant difference (p < 0.001) in mycorhization intensity between the study areas, while the frequency of mycorhization was similar in the different study areas. The contact of the line with a given arc represents the mean value, while the horizontal line indicates the median. Thus, the high intensity of mycorhization is observed in the South of Benin. The intensities of mycorhization in the Center and in the North are similar.
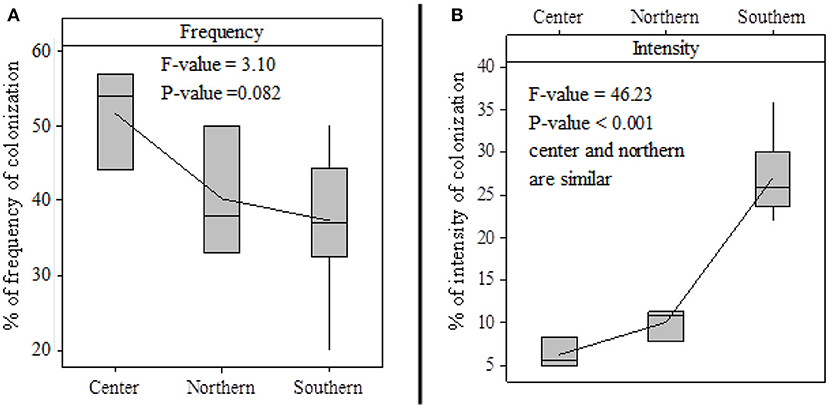
Figure 6. Boxplot of mycorrhization across study areas: frequency of colonization (A); intensity of colonization (B).
Discussion
The chemical characteristics of our study soils show that organic matter varies between 0.95 and 1.17%, while assimilable phosphorus had a respective value of 2 ppm in the South, 47.5 ppm in the Center, and 6.7 ppm in the North. Exchangeable bases varied between 1.82 and 7.84 meq/100 g of soil. The chemical characterizations show that the experimental soils in the South, Center, and North were acidic and moderately poor (Sys et al., 1993). Moreover, soil contents in organic matter, phosphorus, and exchangeable bases are good for an expression of the effects of NPK mineral fertilizers (Igué et al., 2015). The water pH of the study soils varies between 5.5 and 6.20, which shows that our study soils are acidic. According to Davet (1996), AMF are preponderant in acidic soils. The pH influences the activity of soil microorganisms that participate in the mineralization of organic matter as well as that of mycorrhizal fungi (Parent and Gagné, 2010). Coughlan et al. (2000) demonstrated a positive correlation of pH with the quality and quantity of intra-root colonization. They stated that mycorrhizal colonization is high at pH levels between 5 and 7, but low at pH levels around 4, as the adaptation of fungi to different pH levels varies between species. In soils with pH between 7 and 7.6 R. intraradices colonizes more the roots of plants to stimulate their growth.
The contribution of arbuscular mycorrhizal fungi had a significant effect on maize plant growth. Indeed, the application of Ri½ N15P15K15_Urea induced better growth of maize plants compared with the Farmer's practice and those receiving N15P15K15_Urea in Southern Benin, while in Central and Northern Benin, it was the Ri½ N15P15K15_Urea and N15P15K15_Urea treatments that generated the greatest growth variables. These results are in agreement with those obtained by Ndoye et al. (2016) in Senegal who revealed that inoculation with arbuscular mycorrhizal fungi (Glomus manihotis) significantly improved the growth (+28.5%) of fonio (Digitaria exilis Stapf) under semi-controlled conditions. Also, (Sánchez-Roque et al., 2016) also observed a positive effect of AMF inoculation on three pepper varieties.
As for corn grain yield, the results of the analysis of the means revealed a significant difference. Ri½ N15P15K15_Urea had generated the highest yields in the Center, while N15P15K15_Urea had induced the highest yields in the South. However, there was no significant difference between the three treatments in the North with low performance. Indeed, grain yields obtained in the South was 1.95 t ha−1 with the contribution of Ri½ N15P15K15_Urea. These grain yield values are 28% higher than the grain yield obtained from the plants treated with Farmers' practices (without AMF, with N15P15K15_Urea). Plants that received N15P15K15_Urea in Central Benin had an average yield of 2.5 t ha−1, and those that received Ri½ N15P15K15_Urea yielded around 3.4 t ha−1. In North Benin, there was no significant difference between the three treatments. These differences in yield are due to several factors such as the functional diversity of AMF and environmental conditions as so well-noted by (Walder and Van Der Heijden, 2015).
Grain yield was higher on ferruginous soil in the Center compared with yields in the North and South. This is explained by a good level of assimilable phosphorus (11.75 ppm), which was higher than in the ferruginous soils of the North (6.91 ppm) and ferralitic soils of the South (2 ppm) in this study. In addition, the soils of Central Benin have an organic matter rate of 1.16% and a pH in water (6.2) allowing a good expression of R. intraradices, which shows a good colonization of plant roots in soils with a pH between 7 and 7.6. Rivera et al. (2003) in Cuba, Assogba et al. (2017), and Aguégué et al. (2021) in Benin also observed a 35–50% increase in maize yields compared with the control (without AMF or mineral fertilizers) following the application of N15P15K15_Urea, which created a commercial strain. It should be noted that other factors such as temperature and pest attacks may explain the differences in yield observed from one area to another. According to the work of Hasanuzzaman et al. (2013), high temperatures in the northern zone of the country affected plant growth and development through mechanisms described by delayed growth rate, drop in biomass production, leaf and reproductive organ burning, leaf abscission and senescence, fruit damage and, in turn, yield reduction and cell death. Through symbiosis, AMF established mycorrhiza with their hosts (Nadeem et al., 2014; Zhang et al., 2017) and reduced drought-related consequences (Yooyongwech et al., 2016; Moradtalab et al., 2019). Thus, hosts benefited more often from increased access to nutrients with improved growth and yield (Hart et al., 2014; Liu et al., 2016; Chen et al., 2017). Also, the release of nitrogen from mineral fertilizers increased yield and its components (Torbert et al., 2001; Nyembo et al., 2012). Bakonyi and Csitári (2018) made the same observations, showing that AMF inoculation increased wheat grain yield from 7.52 to 8.17 t ha−1 in the same way as mineral fertilization (7.38 to 8.31 t ha−1).
The frequency of mycorhization of maize roots in this study was low. Root colonization of maize plants ranged from 6.19 to 27.02%. These values are low in comparison with the work of Rivera et al. (2003) and Tian et al. (2013), which showed a 76–80% colonization of maize roots as a result of the combination of arbuscular mycorrhizal fungi with the recommended half-dose of mineral fertilizer during and after moderate states of hydric stress. Ndoye et al. (2016) observed the highest mycorhization frequencies and intensities of fonio root mycorhization with G. aggregatum and R. irregularis. On the other hand, Incesu et al. (2015) observed higher rates of root colonization of Diospyros virginiana with R. irregularis and G. caledonium compared with other AMF species (G. etunicatum, Funneliformis mosseae, and G. clarium). However, it is important to note that above 12% mycorhization intensity, the benefits derived by the plant symbiote are not negligible (Diagne et al., 2013).
Conclusion
The results of this study showed that Ri½ N15P15K15_Urea had a positive impact on all the variables of grain growth and yield while reducing by half the use of mineral fertilizers at the different Research and Development Sites of Ouénou, Miniffi, and Zouzouvou. These results show that mycorhization of maize could play an important role in improving the growth and yield of maize plants and, thus, contribute to the development of methods that are environmentally friendly and guarantee sustainable agriculture in Benin. Further work is needed to better understand the behavior of AMF on maize growth and productivity, and soil fertility management in a large number of producers in order to make recommendations on the use of these fungus-based biofertilizers.
Data Availability Statement
The raw data supporting the conclusions of this article will be made available by the authors, without undue reservation.
Author Contributions
This work was carried out in collaboration with all authors. RA, SA, HSa, AK, NAg, and OA conducted the trial set-up, data collection, and harvesting. RA wrote the first draft of the manuscript and managed the bibliographical research. KS with RGK performed the statistical analysis. NAh, HSi, GD, AA, and LB-M wrote the protocol, managed the study analyses, and supervised the various activities. All authors read and approved the final manuscript.
Conflict of Interest
The authors declare that the research was conducted in the absence of any commercial or financial relationships that could be construed as a potential conflict of interest.
Publisher's Note
All claims expressed in this article are solely those of the authors and do not necessarily represent those of their affiliated organizations, or those of the publisher, the editors and the reviewers. Any product that may be evaluated in this article, or claim that may be made by its manufacturer, is not guaranteed or endorsed by the publisher.
Acknowledgments
The authors thank the National Institute of Agricultural Research of Benin, West Africa Agricultural Productivity Program (WAAPP) and Center National de Spécialisation-Maïs (CNS-Maïs) for funding this work.
References
Adjanohoun, A., Baba-Moussa, L., Glèlè kakaï, R., Allagbé, M., Yèhouénou, B., Gotoechan-Hodonou, H., et al. (2011). Caractérisation des rhizobactéries potentiellement promotrices de la croissance végétative du maïs dans différents agrosystèmes du Sud-Bènin. Int. J. Biol. Chem. Sci. 5, 433–444.
Aguégué, M. R., Nestor, R. A. A., Nadège, A. A., Pâcome, N. A., Sylvestre, A., Hafiz, S., et al. (2021). Efficacy of native strains of arbuscular mycorrhizal fungi on maize productivity on ferralitic soil in Benin. Agri. Res. 11, 1-9. doi: 10.1007/s40003-021-00602-7 [Epub ahead of print].
Alalaoui, A. C. (2007). Fertilisation minérale des cultures: les éléments fertilisants majeurs (Azote, Potassium et Phosphore). Bull. Mensuel Inform. Liaison 155, 1–4.
Alqarawi, A., Hashem, A., Abd_Allah, E., Alshahrani, T., and Huqail, A. (2014). Effect of salinity on moisture content, pigment system, and lipid composition in Ephedra alata Decne. Acta Biol. Hung. 65, 61–71. doi: 10.1556/ABiol.65.2014.1.6
Assogba, S., Noumavo, A. P., Dagbenonbakin, G., Agbodjato, A. N., Akpode, C., Koda, D. A., et al. (2017). Improvement of maize productivity (zea mays l.) By mycorrhizal inoculation on ferruginous soil in center of Benin. Int. J. Sustain. Agric. 4, 63-76. doi: 10.18488/journal.70.2017.43.63.76
Bakonyi, I., and Csitári, G. (2018). Response of winter wheat to arbuscular mycorrhizal fungal inoculation under farm conditions. Columella 5, 51–58. doi: 10.18380/SZIE.COLUM.2018.5.1.51
Balogoun, I., Saïdou, A., Ahoton, E. L., Amadji, G. L., Ahohuendo, C. B., and Adebo, J. B. (2014). Characterization of cashew based production system in the main growing areas in Benin. Agron. Afr. French 26, 9–22.
Batamoussi, M. H., Oga, C. A., Sèkloka, E., and Saïdou, A. (2014). Effects of different formulations of mineral fertilizers on the agronomic parameters of maize (Zea mays) in the climate change conditions of central Benin. Int. J. Adv. Sci. 4, 31–35.
Bedini, A., Mercy, L., Schneider, C., Franken, P., and Lucic-Mercy, E. (2018). Unraveling the initial plant hormone signaling, metabolic mechanisms and plant defence triggering the endomycorrhizal symbiosis behavior. Front. Plant Sci. 9:1800. doi: 10.3389/fpls.2018.01800
Benbrahim, K. F., Ismaili, M., Benbrahim, S. F., and Tribak, A. (2004). Problèmes de dégradation de l'environnement par la désertification et la déforestation: impact du phénomène au Maroc. Sci. Chang. Planét. Sécher. 15, 307–320.
Berruti, A., Lumini, E., Balestrini, R., and Bianciotto, V. (2016). Arbuscular mycorrhizal fungi as natural biofertlizers: let's benefit from past successes. Front. Microbiol. 6:1559. doi: 10.3389/fmicb.2015.01559
Bi, Y., Zhang, Y., and Zou, H. (2018). Plant growth and their root development after inoculation of arbuscular mycorrhizal fungi in coal mine subsided areas. Int. J. Coal Sci. Technol. 5, 47-53. doi: 10.1007/s40789-018-0201-x
Bray, R. H., and Kurtz, L. T. (1945). Determination of total, organic, and available forms of phosphorus in soils. Soil Sci. 59, 39–46.
Chen, S., Zhao, H., Zou, C., Li, Y., Chen, Y., Wang, Z., et al. (2017). Combined inoculation with multiple arbuscular mycorrhizal fungi improves growth, nutrient uptake and photosynthesis in cucumber seedlings. Front. Microbiol. 8:2516. doi: 10.3389/fmicb.2017.02516
Coughlan, A. P., Dalpé, Y., Lapointe, L., and Piché, Y. (2000). Soil pH-induced changes in root colonization, diversity, and reproduction of symbiotic arbuscular mycorrhizal fungi from healthy and declining maple forests. Can. J. For. Res. 30, 1543-1554. doi: 10.1139/x00-090
Davet, P. (1996). Vie microbienne du sol et production végétale. Editions Quae. Paris: Institut National de la Recherche Agronomique.
Diagne, N., Thioulouse, J., Sanguin, H., Prin, Y., Krasova-Wade, T., Sylla, S., et al. (2013). Ectomycorrhizal diversity enhances growth and nitrogen fixation of Acacia mangium seedlings. Soil Biol. Biochem. 57, 468–476. doi: 10.1016/j.soilbio.2012.08.030
Douglas, C. E., and Fligner, M. A. (1991). On distribution-free multiple comparisons in the one-way analysis of variance. Commun. Stat. Theory Methods 20, 127-139. doi: 10.1080/03610929108830487
Eisenhauer, N. (2017). Consumers control carbon. Nat. Ecol. Evol. 1, 1596-1597. doi: 10.1038/s41559-017-0352-y
Fernandez, F., Gómez, R., Vanegas, L. F., Martýnez, M. A., de la Noval, B. M., and Rivera, R. (2000). Producto inoculante micorrizógeno. Oficina Nacional de Propiedad Industrial.
Ferro Valdés, E. M., Chirino González, E., Márquez Serrano, M., Mirabal Báez, E., Ríos Labrada, H., Guevara Hernández, F., et al. (2013). Experiencias obtenidas en el desarrollo participativo de híbridos lineales simples de maíz (Zea mays, L.) en condiciones de bajos insumos agrícolas. Cult. Trop. 34, 61–69.
Giovanetti, M., and Mosse, B. (1980). An evaluation for measuring vesicular-arbuscular mycorrhizal infection in roots. New Phytol. 84, 489–500. doi: 10.1111/j.1469-8137.1980.tb04556.x
Glèlè Kakaï, R., Sodjinou, E., and Fonton, N. (2006). Conditions d'Application des Méthodes Statistiques Paramétriques: Application sur Ordinateur. Bénin: Bibliothèque Nationale, 86.
Hailemariam, M., Birhane, E., Asfaw, Z., and Zewdie, S. (2013). Arbuscular mycorrhizal association of indigenous agroforestry tree species and their infective potential with maize in the rift valley, Ethiopia. Agrofor. Syst. 87, 1261-1272. doi: 10.1007/s10457-013-9634-9
Hart, M. M., Gorzelak, M., Ragone, D., and Murch, S. J. (2014). Arbuscular mycorrhizal fungal succession in a long-lived perennial. Botany 92, 313–320. doi: 10.1139/cjb-2013-0185
Hasanuzzaman, M., Gill, S. S., and Fujita, M. (2013). “Physiological role of nitric oxide in plants grown under adverse environmental conditions,” in Plant Acclimation to Environmental Stress (New York, NY: Springer), 269-322. doi: 10.1007/978-1-4614-5001-6_11
Hernandez, J. A., Olmos, E., Corpas, F. J., Sevilla, F., and Del Rio, L. A. (1995). Salt-induced oxidative stress in chloroplasts of pea plants. Plant Sci. 105, 151–167. doi: 10.1016/0168-9452(94)04047-8
Igué, A. M., Adjanohoun, A., Aǐhou, C., and Mensah, G. A. (2015). Document Technique d'Information: Evaluation de l'état de la fertilité des sols champs des producteurs élites de maïs du Bénin. Dépôt légal N° 8116 du 09/09/2015. Trimestre: Bibliothèque Nationale (BN).
Incesu, M., Yepilðdlu, T., Cimen, B., and Yilmaz, B. (2015). Influences of different iron levels on plant growth and photosynthesis of W. Murcott mandarin grafted on two rootstocks under high pH conditions. Turk. J. Agric. For. 39, 838–844. doi: 10.3906/tar-1501-25
Kjedahl, J. (1883). Method for the Quantitative Determination of Nitrogen in Chemical Substances. Copenhague: Carlberq Laboratory.
Liu, A., Chen, S., Wang, M., Liu, D., Chang, R., Wang, Z., et al. (2016). Arbuscular mycorrhizal fungus alleviates chilling stress by boosting redox poise and antioxidant potential of tomato seedlings. J. Plant Growth Regul. 35, 109-120. doi: 10.1007/s00344-015-9511-z
MAEP (2016). Catalogue Béninois des Espèces et Variétés végétales (CaBEV), 2ème Edn. Ministère de l'Agriculture de l'Elevage et de la Peche; INRAB/DPVPPAAO/ProCAD/MAEP and CORAF/WAAPP, Dépôt légal N 8982 du 21 octobre 2016, Bibliothèque Nationale du Bénin, 4ème trimestre. Available online at: http://inrab.org/wp-content/uploads/2018/01/CaBEV-interactif-2.pdf (accessed January 25, 2021).
Metson, A. J. (1957). Methods of chemical analysis for soil survey samples. Soil Sci. 83:245. doi: 10.1097/00010694-195703000-00016
Moradtalab, N., Hajiboland, R., Aliasgharzad, N., Hartmann, T. E., and Neumann, G. (2019). Silicon and the association with an arbuscular-mycorrhizal fungus (Rhizophagus clarus) mitigate the adverse effects of drought stress on strawberry. Agronomy 9:41. doi: 10.3390/agronomy9010041
Nadeem, S. M., Ahmad, M., Zahir, Z. A., Javaid, A., and Ashraf, M. (2014). The role of mycorrhizae and plant growth promoting rhizobacteria (PGPR) in improving crop productivity under stressful environments. Biotechnol. Adv. 32, 429–448. doi: 10.1016/j.biotechadv.2013.12.005
Ndoye, F., Abdala, G. D., Moustapha, G., Diomacor, F., Adeline, B., Mame, O. S., et al. (2016). Réponse du fonio blanc (Digitaria exilis Stapf) à l'inoculation avec des champignons mycorhiziens à arbuscules en conditions semi-contrôlées. J. Appl. Biosci. 103, 9784–9799. doi: 10.4314/jab.v103i1.1
Nyembo, K. L., Useni, S. Y., Mpundu, M. M., Bugeme, M. D., Kasongo, L. E., and Baboy, L. L. (2012). Effect of increasing doses of the mineral fertilizer (NPKS and Urea) on maize (Zea mays L.) yield and profitability in Lubumbashi, Southeast of the DR Congo. J. Appl. Biosci. 59, 4286–4296.
Oehl, F., Alves a Silva, G., Goto, B. T., Costa Maia, L., and Sieverding, E. (2011). Glomeromycota: two new classes and a new order. Mycotaxon 116, 365-379. doi: 10.5248/116.365
Parent, L. E., and Gagné, G. (2010). Guide de référence en fertilisation. Centre de référence en agriculture et agroalimentaire du Québec.
Phillips, J. M., and Hayman, D. S. (1970). Improved procedures for clearing roots and staining parasitic and vesicular-arbuscular mycorrhizal fungi for rapid assessment of infection. Trans. Br. Mycol. Soc. 55, 158–IN18. doi: 10.1016/S0007-1536(70)80110-3
R Core Team (2020). R: A Language and Environment for Statistical Computing. Vienna: R Foundation for Statistical Computing.
Rivera, R., Fernández, F., Hernández-Jiménez, A., Martín, J. R., and Fernández, K. (2003). “El manejo efectivo de la simbiosis micorrízica, una vía hacia la agricultura sostenible,” in Estudio de caso, el Caribe, eds R. Y. Rivera, K. Fernández (La Habana: Ediciones Instituto Nacional de Ciencias Agrícolas), 1-42.
Ruget, F., Bonhomme, R., and Chartier, M. (1996). A simplified method for estimating the leaf area growth of field-grown maize from a reduced number of measurements. Agronomie 9, 553–561.
Sánchez-Roque, Y., Pérez-Luna, Y., Becerra-Lucio, A., Alvarez-Gutiérrez, P., Pérez-Luna, E., González-Mendoza, D., et al. (2016). Effect of arbuscular mycorrhizal fungi in the development of cultivars of Chili. Int. J. Adv. Agric. Res. 4, 10–15.
Sendek, A., Karakoç, C., Wagg, C., Domínguez-Begines, J., do Couto, G. M., van der Heijden, M. G., et al. (2019). Drought modulates interactions between arbuscular mycorrhizal fungal diversity and barley genotype diversity. Sci. Rep. 9, 1-15. doi: 10.1038/s41598-019-45702-1
Sys, C., Van Ranst, E., Debaveye, J., and Beenaert, F. (1993). Land Evaluation Part III. Crop Requirements. Agriculture publication, 166.
Tchabi, A., Coyne, D., Hountondji, F., Lawouin, L., Wiemken, A., and Oehl, F. (2008). Arbuscular mycorrhizal fungal communities in sub-Saharan Savannas of Benin, West Africa, as affected by agricultural land use intensity and ecological zone. Mycorrhiza 18, 181–195. doi: 10.1007/s00572-008-0171-8
Tian, H., Drijber, R. A., Li, X., Miller, D. N., and Wienhold, B. J. (2013). Arbuscular mycorrhizal fungi differ in their ability to regulate the expression of phosphate transporters in maize (Zea mays L.).Mycorrhiza 23, 507–514. doi: 10.1007/s00572-013-0491-1
Torbert, H. A., Potter, K. N., and Morrison, J. E. (2001). Tillage system, fertilizer nitrogen rate, and timing effect on corn yields in the Texas Blackland Prairie. Agron. J. 93, 1119–1124. doi: 10.2134/agronj2001.9351119x
Trouvelot, A., Kough, J. L., and Gianinazzi-Pearson, V. (1986). “Mesure du taux de mycorhization VA d'un syste‘me radiculaire. Recherche de methode d'estimation ayant une signification fonctionnelle,” in Physiological and Genetical Aspects of Mycorrhizae: Proceedings of the 1st European Symposium on Mycorrhizae (Dijon), 217–221.
Usharani, G., Sujitha, D., Sivasakthi, S., and Saranraj, P. (2014). Effect of arbuscular Mycorrhizal (AM) fungi (Glomus fasciculatum L.) for the improvement of growth and yield of maize (Zea mays L.). Centr. Eur. J. Exp. Biol. 3, 19–25.
van der Heijden, M. G., Martin, F. M., Selosse, M. A., and Sanders, I. R. (2015). Mycorrhizal ecology and evolution: the past, the present, and the future. New Phytol. 205, 1406-1423. doi: 10.1111/nph.13288
Walder, F., and Van Der Heijden, M. G. (2015). Regulation of resource exchange in the arbuscular mycorrhizal symbiosis. Nat. Plants 1, 1–7. doi: 10.1038/nplants.2015.159
Walkley, A., and Black, I. A. (1934). An examination of the Degtjareff method for determining soil organic matter, and a proposed modification of the chromic acid titration method. Soil Sci. 37, 29-38. doi: 10.1097/00010694-193401000-00003
Wall, D. H., Nielsen, U. N., and Six, J. (2015). Soil biodiversity and human health. Nature 528, 69-76. doi: 10.1038/nature15744
Yooyongwech, S., Samphumphuang, T., Tisarum, R., Theerawitaya, C., and Cha-Um, S. (2016). Arbuscular mycorrhizal fungi (AMF) improved water deficit tolerance in two different sweet potato genotypes involves osmotic adjustments via soluble sugar and free proline. Sci. Hortic. 198, 107–117. doi: 10.1016/j.scienta.2015.11.002
Keywords: Rhizophagus intraradices, farming environment, ecological systems, yield, maize, Benin
Citation: Aguégué RM, Assogba SA, Salami HAA, Koda AD, Agbodjato NA, Amogou O, Sina H, Salako KV, Ahoyo Adjovi NR, Dagbénonbakin G, Kakai RG, Adjanohoun A and Baba-Moussa L (2021) Organic Fertilizer Based on Rhizophagus intraradices: Valorization in a Farming Environment for Maize in the South, Center and North of Benin. Front. Sustain. Food Syst. 5:605610. doi: 10.3389/fsufs.2021.605610
Received: 12 September 2020; Accepted: 05 October 2021;
Published: 08 November 2021.
Edited by:
Duraisamy Saravanakumar, The University of the West Indies St. Augustine, Trinidad and TobagoReviewed by:
Luciana Maria Saran, São Paulo State University, BrazilEverlon Cid Rigobelo, São Paulo State University, Brazil
Copyright © 2021 Aguégué, Assogba, Salami, Koda, Agbodjato, Amogou, Sina, Salako, Ahoyo Adjovi, Dagbénonbakin, Kakai, Adjanohoun and Baba-Moussa. This is an open-access article distributed under the terms of the Creative Commons Attribution License (CC BY). The use, distribution or reproduction in other forums is permitted, provided the original author(s) and the copyright owner(s) are credited and that the original publication in this journal is cited, in accordance with accepted academic practice. No use, distribution or reproduction is permitted which does not comply with these terms.
*Correspondence: Lamine Baba-Moussa, bGFtaW5lc2FpZCYjeDAwMDQwO1lhaG9vLmZy