- 1Department of Mechanical Engineering, University of Missouri, Columbia, MO, United States
- 2Department of Biomedical, Biological and Chemical Engineering, University of Missouri, Columbia, MO, United States
- 3Division of Food Systems and Bioengineering (Food Science and Nutrition), University of Missouri, Columbia, MO, United States
- 4Division of Plant Sciences, University of Missouri, Columbia, MO, United States
While the world is facing food and nutritional challenges leading to the multifaceted burden of malnutrition (underweight and overweight), there is a need to sustainably diversify and explore underutilized crops. Climate-resilient crops, which have the potential to withstand climate crises, have drought resistance, and provide healthy foods with essential vitamins and minerals. Ancient seed grains like amaranth, millets, and sorghum are highly nutritious seed grains that are underutilized, and there is a need for comprehensive research into their properties. This study will specifically investigate amaranth alongside barnyard, finger, kodo, little, pearl, proso millets, and sorghum. Physical and structural properties of the ancient seed grains can provide useful data for storage and food processing. The angle of repose, porosity, and water activity of the grains varied from 19.3° to 23.9°, 3.6 to 17.4%, and 0.533 to 0.660 at 25.5°C, respectively. Additionally, Scanning Electron Microscopy (SEM) was used to observe the surface characteristics and overall shape of each grain. SEM images of the millets shows the impact of dehulling on the surface morphology of the grains (little, barnyard, proso, and kodo millets). This calls for research and development of novel food processing technologies to minimize loss and damage during processing of climate-resilient crops.
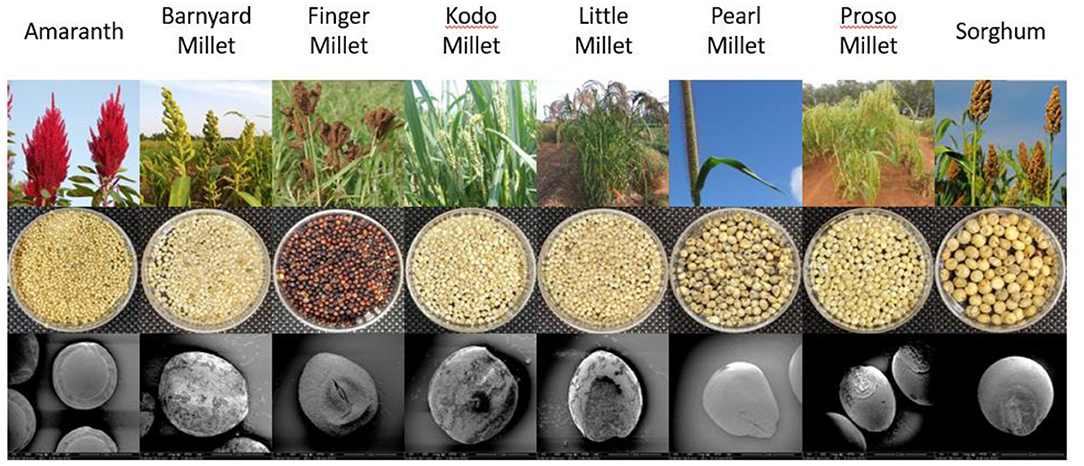
Graphical Abstract. Underutilized Ancient Grains: potentials to develop novel foods to improve the health of people and planet.
Introduction
The bold and transformative 2030 Agenda for Sustainable Development was officially launched in September 2015 by 193 member states of the United Nations. The 2030 agenda calls on countries to begin efforts to achieve the 17 Sustainable Development Goals (SDGs) over the next 10 years (United Nations, 2020). SDG 2 focuses on Zero Hunger, to achieve food security, improved nutrition, and promote sustainable agriculture. Food and nutrition security are interconnected and in order to achieve them in the near future, there is a need to explore underutilized crops as food sources leading to diversification of healthy diets to feed 9 billion population in 2050. Millets are a group of small nutritious seed grains that are primarily grown in Africa and Asia. Figure 1 shows production of millets by geographical region and year. The various millets represent both cold and warm season crops that can be suited for dry and wet conditions, depending upon the species. Pearl millet, which originated in Africa, is suited for hot and dry climates with little rainfall but also tolerates hot and wet climates. By contrast, proso millet is from a colder climate, originating from Central and Eastern Asia, so it performs better in cooler areas. Millets generally reach maturity faster than crops like maize or wheat. Foxtail and proso millet can mature in 60–90 days while pearl and finger millet can mature in up to 100–110 days (Myers, 2018b). Millet uses vary from being processed into infant formula, fermented foods, flour, breads, beer, and snacks and can be prepared in a multitude of ways. Millets are a highly underutilized seed grain in the Western world, and in the US, they are often used as animal feed or cover crops to promote healthy soil (Myers, 2018b).
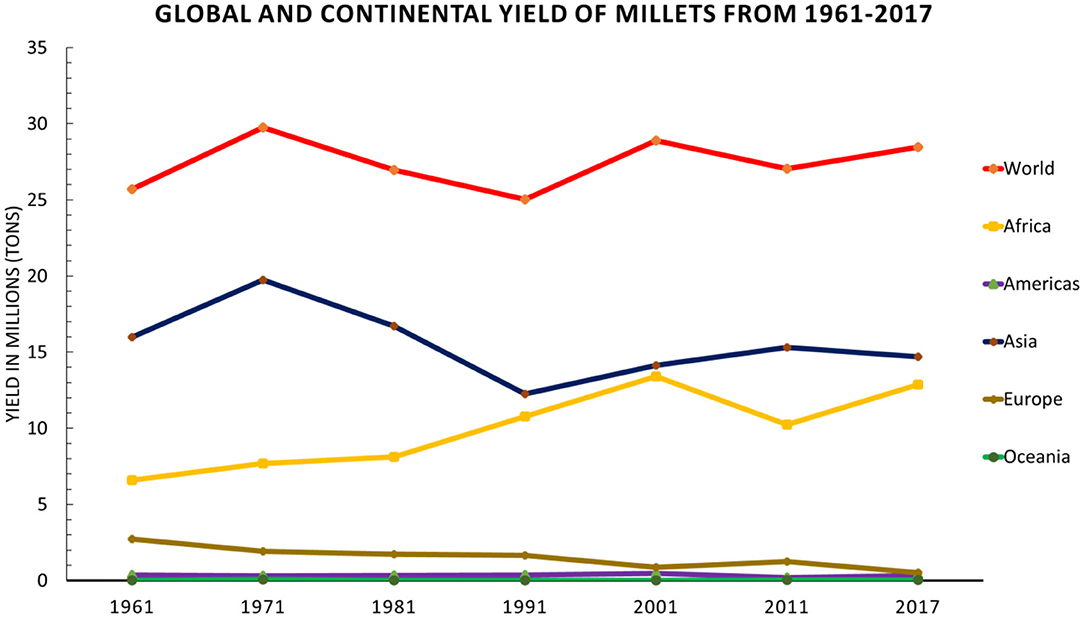
Figure 1. Global and Continental Yield of Millets from 1961 to 2017 (FAOSTAT, 2019).
Amaranth is another ancient cereal grain that was first utilized by humans 6,000 years ago and was once an important crop to the Aztecs. Like millet, amaranth has drought resistance and includes many different varieties that are adaptable to a wide range of environments (Myers, 2018a). The leaves of the vegetable amaranth plant can be boiled and consumed like spinach, while the grain amaranth can be popped, ground into flour, or turned into many ready-to-eat products, such as millets (Robinson, 1986). In the US, amaranth has gained popularity in commercial products like sandwich bread, breakfast cereals, granola bars, and crackers (Myers, 2018a).
Millets and amaranth have the potential to help address climate change challenges (Saxena et al., 2018) and the need to find alternative crops to feed the growing population with nutritious food. To utilize and popularize these forgotten grains, novel value-added food product development, optimization, and research is required in this area. Ancients seed grains are gaining popularity among millennials and Gen Z for their low-calorie and low-fat contents. An ideal way to introduce consumers to an unfamiliar ingredient, like millet or amaranth, is to develop a familiar product with this new food ingredient. They also have potentials to be used in food fortification as natural alternatives due to rich micronutrient profile and create a new demand for millet and amaranth-based foods.
The health benefits of ancient seed grains are numerous, as there are several different varieties and each with its own strengths. All varieties of millets and amaranth are naturally free from gluten and have a lower caloric content than rice and wheat. Millets are also lower in carbohydrates than rice, which can help prevent the development of diabetes and obesity which has become a serious problem in our modern world. Pearl and proso millet are higher in protein than all other millets as well as rice. However, they both have a lower protein content than amaranth, (Valcárcel-Yamani and Caetano da Silva Lannes, 2012). Barnyard millet has a higher amount of fiber compared to rice, wheat, and other millets, which aids in digestion and nutrient retention. Finger millet contains a third of the daily recommended calcium intake, which is the most significant percentage of any essential mineral found in millets (Saleh et al., 2013). Other high percentages of essential minerals include iron in barnyard millet, zinc in foxtail and barnyard millets, niacin in proso millet, and riboflavin in barnyard millet.
Amaranth has a high protein content and contains almost twice as much protein as maize. Although the total protein content is not as high as a legume's, amaranth can be consumed as a source of “complete” protein because it has an ideal balance of the nine essential amino acids (Myers, 2018a). A more complete comparison between millets, amaranth, rice, and wheat can be seen in Table 1.
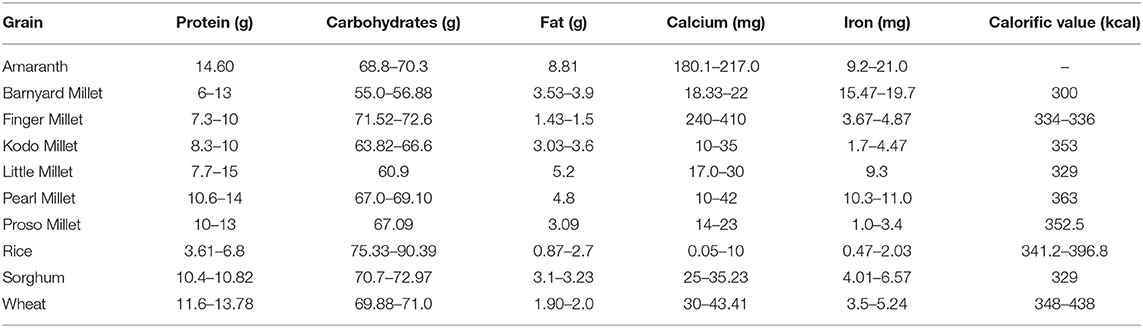
Table 1. Nutritional Comparison of Millets, Amaranth, Rice, and Wheat (per 100 g) (Valcárcel-Yamani and Caetano da Silva Lannes, 2012; Saleh et al., 2013; Kumar et al., 2018; Nithiyanantham et al., 2019).
Hidden Hunger is a worldwide issue (deficiency due to vitamins and minerals): specifically, it is the double burden of malnutrition (stunting/underweight and obesity/overweight), which can affect any age, gender, or nationality. Children deficient in iron and zinc are shown to have stunted growth, impaired immune function, and irreversible neurological and psychomotor impairments (Mehta et al., 2017). Efforts to solve this crisis have recently included the biofortification of pearl millet in India, Govindaraj et al. (2019) have attempted to genetically improve cultivars of pearl millet to increase the densities of iron and zinc. There is a need to specifically design food processing equipment for ancient seed grains, develop new value-added food products, and expand the market needs for diversification of healthy diets.
The physical and structural properties of grains are critical and impact food processing parameters that are essential in the design of equipment for handling, harvesting, processing, and storing of grains (Baryeh, 2002). The shape of the grains is important in the design of sorting and sizing machines (Jain and Bal, 1997). Water activity, bulk density, true density, and porosity are important parameters to consider in the designing of storage structures for grains (Singh et al., 2010). The angle of repose is an important measure in the designing of storage, processing, and transporting structures (Bhadra et al., 2017). Scanning Electron Microscopy (SEM) is used to identify microstructural features of grains that would be otherwise hard to differentiate with human visual observations. The present study is investigating the physical and structural properties of barnyard millet, finger millet, kodo millet, little millet, pearl millet, proso millet, sorghum, and amaranth as an observational exercise to establish a physical characterization method. This information can help researchers and food industries explore the usage of the underutilized ancient seed grains in the development of new sustainable diets.
Materials and Methods
Materials
Ancient Grains
The amaranth sample was provided by Dr. Rob Myers (Division of Plant Sciences, Columbia, MO), from his 2018 harvest of the Crimson Glow cultivar. The barnyard, little, kodo, and proso millets were obtained from Manna Foods (Southern Health Foods Pvt. Ltd, Chennai, India). The finger, pearl, and sorghum millets were supplied by Babco Foods International, LLC (Bridgewater, NJ 08807). All grain samples were stored at room temperature. The reasons for using commercial samples were based on the accessibility of raw materials and food availability to consumers/food start-up company interested to utilize ancient seed grains for new product development. Packaged food materials are subjected to food quality standards before entering commercial markets. Thus, for this study, we used commercially available ancient seed grains samples currently available for human consumption as an observational exercise to establish a physical characterization method. Table 2 shows the whole grain image (photographic representation) used in this study along with information related to the geography of domestication, major producers of ancient seed grains.
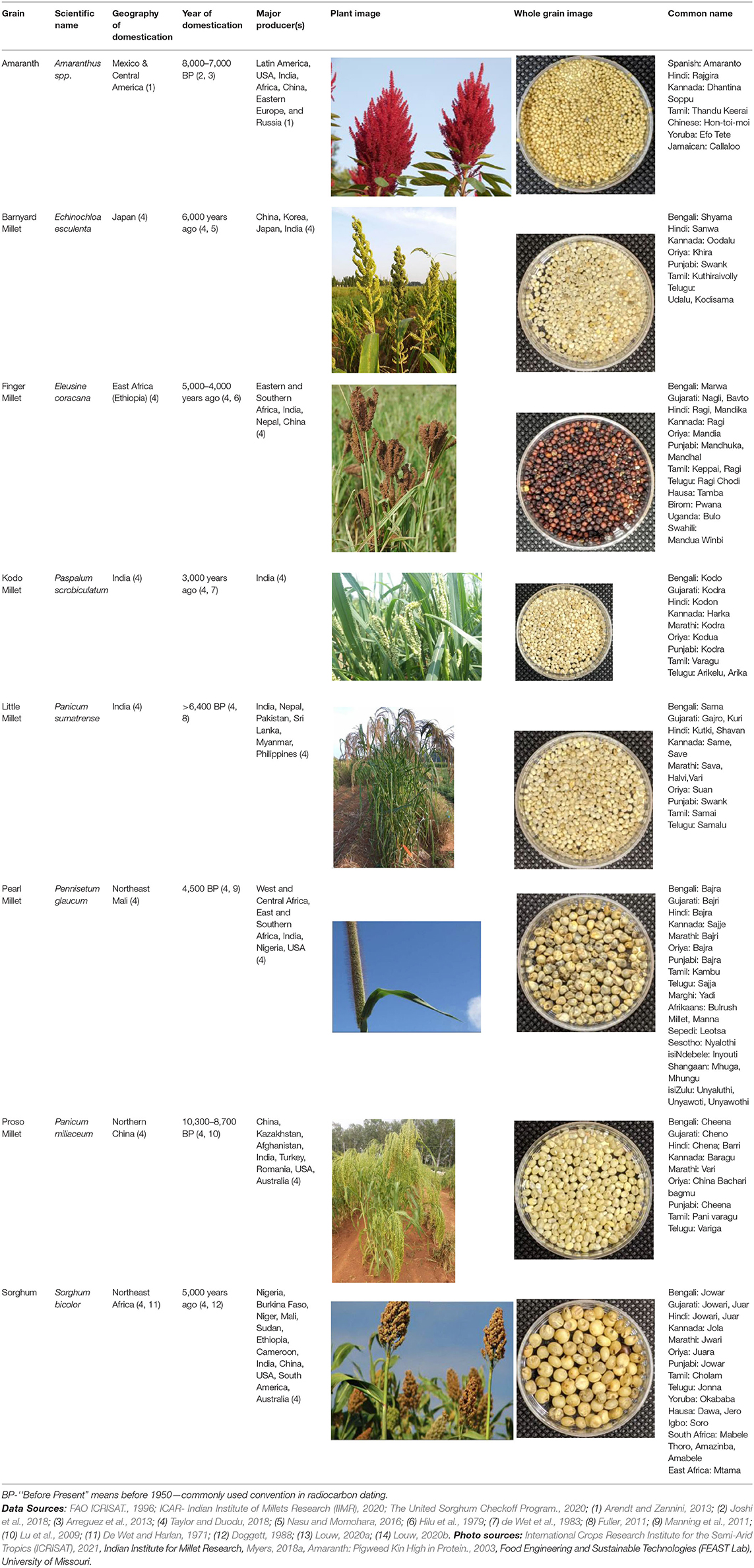
Table 2. Geography of domestication, major producers, and photographic representation of Ancient seed grains.
Methods
Angle of Repose
The angle of repose was calculated by filling a cylinder with grain that was then slowly lifted to create a conical mound (Akaaimo and Raji, 2006). The base diameter and the height of the cone were measured, and the following equation was used to calculate the angle of repose:
where ϕ is the angle of repose, H is the height, and d is the diameter. The measurements were replicated (n = 10) times and the average value was reported. A plastic cylinder of 37 mm diameter was used. This value is an important property because it can impact the design of storage containers and processing equipment due to the differences in each grain.
Bulk Density
The bulk density of grains was measured using two methods: the water displacement method and mass/volume relationship. The variability between both methods was compared. The water displacement method was done by filling a 25 ml graduated cylinder with 15 ml of water, 4.5 grams of each grain was added, and the volume of water displaced was measured. For the mass/volume method bulk density was measured using the procedure described by Mariotti et al. (2006). Four gram of each grain was poured into a 25 ml graduated cylinder gently tapped 10 times and the volume was noted. The ratio of the mass of the sample of grain to its total volume was then calculated (n = 5). The results are expressed in Kg/m3.
True Density
The true density of the millets was measured using a Quantachrome Ultrapycnometer 1000 (Anton Paar, Graz, Austria). In total, 4.5 grams were weighed out and placed in the small cell for measurement. The equipment was operated using the multi-run setting with 5 runs and a standard deviation of 0.5%. The final three runs were averaged and reported by the equipment. This was repeated three times, each with a different sample of 4.5 grams to account for the variability in grain size and shape.
Color
The color of each millet sample was measured using a Konica Minolta Chroma Meter CR-400/410 (Konica Minolta, Chiyoda, Tokyo, Japan). The device utilizes an L*, a*, b* color space system to quantify the color of an object. L* represents lightness and darkness, a* represents redness when positive and greenness when negative, and b* represents yellowness when positive and blueness when negative. The device was calibrated using a white sample provided by the manufacturer. For each sample, a container was filled, and the meter was placed on the sample. Five measurements were taken (n = 5), with the average value reported.
Porosity
The porosity of the millet grains was calculated using the true density values determined by the pycnometer and the experimental bulk density with the following equation.
Scanning Electron Microscopy
The internal and external structure of each millet type was determined using a Quanta 600F ESEM Scanning Electron Microscope located at the Electron Microscopy Core at the University of Missouri—Columbia. The grains were prepared by coating them with Platinum. Comparative analysis of multiple ancient seed grains at 5 KV power between 20 and 50X magnification.
Shape
The shape of each grain was determined by evaluating the SEM images and using the data characterizations McDonough and Rooney (1989) determined to classify the different shapes of pearl millet they observed. These shape types include lanceolate, obovate, hexagonal, and globose.
Water Activity
The water activity was measured by the Aqua Lab Cx-2 Water Activity Meter (Decagon Devices, Inc., Pullman, Washington 99163). The equipment determines the water activity of a sample by measuring the relative humidity of the sample when the sample water vapor pressure and air water vapor pressure reach equilibrium. The sample cups were filled less than half full and 5 measurements were made per sample. The average values were reported.
Statistical Analysis
A one-way ANOVA was conducted for all experiments. The number of replicates varies for different experiments with a minimum of (n = 3) for color, (n = 10) for the angle of repose, and (n = 5) for true density and water activity. All data were analyzed using SAS™ software, Version 9.4 of the System for Windows 10.
Results and Discussion
Angle of Repose
While designing food processing equipment and storage systems, the angle of repose is an important physical parameter to be estimated for each grain. An ancient grain with a high angle of repose will pile higher and require more energy to transport than a grain with a lower angle of repose. The angle of repose varied from 19.3° to 23.9° with kodo millet having the smallest angle and proso millet with the largest angle. The angle of repose values is shown in Figure 2. The base measurements for kodo, sorghum, little, finger, barnyard, and proso were not significantly different from each other. The height measurements for little, barnyard, and amaranth were not significantly different from each other, while proso, little, barnyard, amaranth, finger, sorghum, and kodo were not significantly different from each other. The values for base, height, and angle are presented in Table 3.
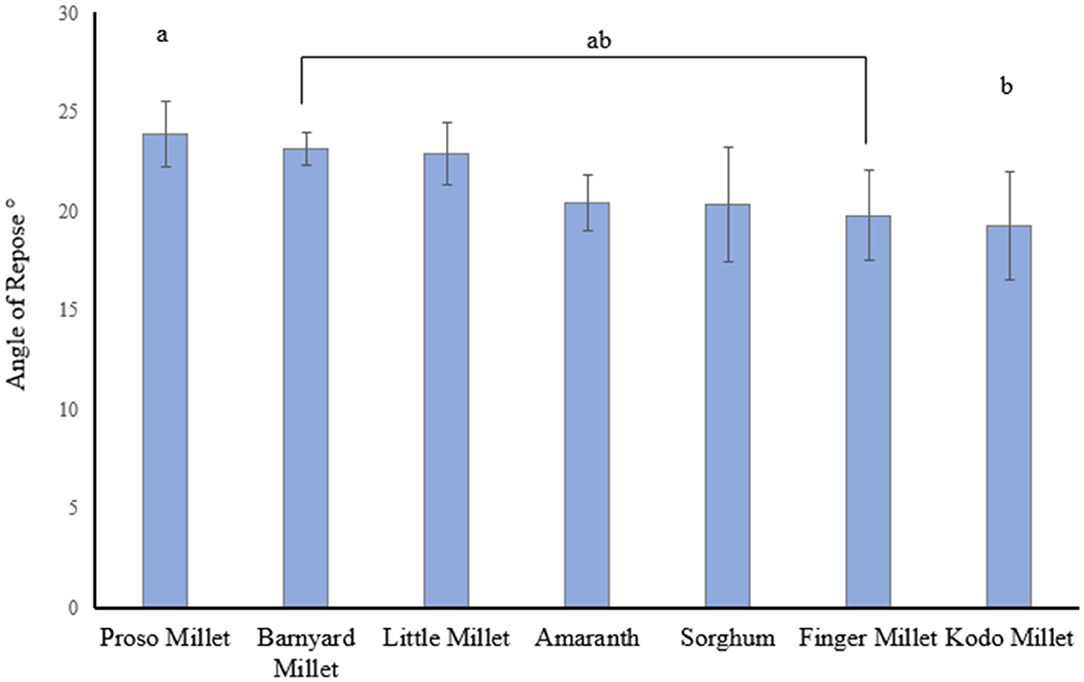
Figure 2. Angle of Repose for Ancient Grains. Mean values not connected by the same letters are significantly different (Tukey's HSD, P < 0.05).
Previous studies on the angle of repose use two main methods: a cylindrical method that was employed in this study and a “box” method. This method uses a plywood box with two plates, one fixed and the other unfixed. The box is filled with the samples and then either gradually inclined until a slope is formed or the front panel is quickly removed to allow a slope to form. Rough rice (Varnamkhasti et al., 2008), popcorn kernels (Karababa, 2006), and pearl millet (Baryeh, 2002) used this “box” method. These values are reported to vary from 37° to 38°, 25.3° to 30.8°, and 34.4° to 49°, respectively.
Soybean (Davies and El-Okene, 2009) has been found using the same method this study uses, along with Prosopis africana seeds (Akaaimo and Raji, 2006). The reported values vary with respect to moisture content from 30° to 51° and 21.57° to 22.71°, respectively. This study uses the cylindrical method as opposed to the box method because large amounts of grains are typically stored in cylindrical silos, so the values obtained would be the most applicable.
Bulk Density
Bulk density's values of the grains ranged from 1,153 to 1,408 kg/m3 when using the water displacement method and 728.16–828.72 Kg/m3 with the mass/volume method. The values of bulk density for both methods followed the same trend for the grains with sorghum showing the least bulk density at 1,153 Kg/m3 for the water displacement method and 728.16 Kg/m3 for mass/volume method and kodo millet showing the highest bulk density at 1,408 Kg/m3 for water displacement method and 828.72 Kg/m3 for mass/volume method.
Sorghum, while the largest in appearance, had the lowest bulk density, and kodo millet had the highest bulk density. Previous studies done on the bulk density of minor millets include a study by Subramanian and Viswanathan (2003), which employed a mass/volume method. A container of known volume was filled to the top with grain which was weighed. The bulk density was calculated as a ratio of the mass of grains to the volume of the container. In the study by Subramanian and Viswanathan (2003), the millet with the greatest bulk density was barnyard millet, at 888.7 kg m−3 and little millet had the lowest bulk density, at 748.1 kg m−3. Similarly, the bulk density of soybeans (Kibar and Öztürk, 2008) and popcorn kernels (Karababa, 2006) have been previously found with similar values. Both use a similar method of filling a 1,000 mL container full of the respective samples which were then weighed. A ratio of sample mass to container volume was calculated. The bulk density of soybeans varied from 719 and 766 kg · m−3, while the popcorn kernels varied from 703 to 771 kg ·m−3. The distributions of bulk density are shown in Figure 3.
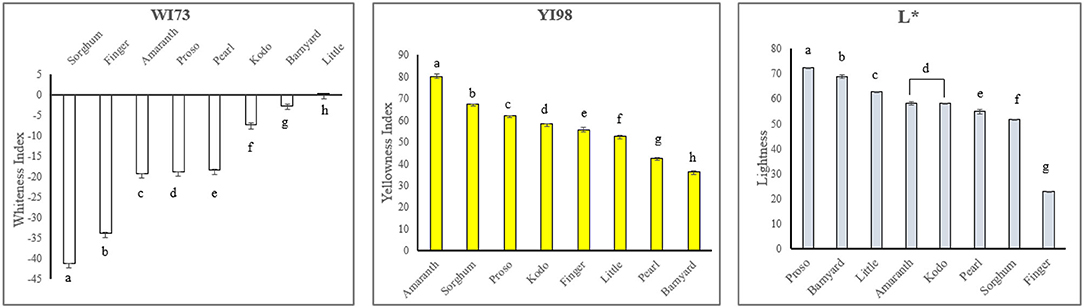
Figure 3. Distribution of Whiteness Index(E313) (73), Yellowness Index(E313) (98), and Lightness (L*) values of Ancient seed grains. For each chart, mean values not connected by the same letter are significantly different (Tukey's HSD, P < 0.05).
The observed difference in the values expressed in the water displacement method and the mass/volume method may be due to the presence of air spaces between and/or within the grains (Manger, 1966). Bulk density calculation using the mass/volume method includes the volume of the grains, open and closed pores, and the interparticle voids (Lorraine and and Flint, 2002) resulting in a higher volume and lower density. In the water displacement method, water is absorbed by the grains and it adheres to the pores of the grains and between the grains resulting in a lower volume of water displaced and a larger bulk density value.
Two methods for bulk density measurements were analyzed in this study to observe which one would be more suitable for the ancient grains of smaller size profiles. The comparison results are presented in Table 4. In this study, we establish the water displacement method was not ideal for bulk density measurement of ancient grains of smaller size profile. The presence of pore spaces in the grains leads to the loating of gains on the water surface. As a result, the mass/volume method was determined to be more suitable for the bulk density measurement of ancient grains.
True Density
The true density of the grains, as shown in Table 4, varied from 1390.17 to 1459.4 kg · m−3 with amaranth having the lowest true density and kodo have the largest. The masses of proso, barnyard, little, kodo, and amaranth could be grouped and were not significantly different from each other, while amaranth, little, kodo, and sorghum can be grouped and were also not significantly different from each other. The volume of each grain was significantly different from each other, except for sorghum and proso. The density of each grain was significantly different from each other.
Previous work finding the true density of soybean and rough rice has been done using the toluene displacement method. Kibar and Öztürk (2008) used the liquid displacement method outlined by Baryeh (2001) and Abalone et al. (2004), with toluene instead of water because the soybeans would absorb that substance less than it would water. Varnamkhasti et al. (2008) also found the true density of rough rice by using the toluene displacement method outlined by Mohsenin (1986).
The true density of soybeans varied from 905 to 983 kg · m−3. The true density of rough rice of two different cultivars were 1269.1 and 1193.43 kg · m−3. While the method of this study differed from these two studies, the results are comparable. The true density of amaranth is most similar to the true density of the densest rough rice cultivar determined by Varnamkhasti et al. (2008).
Color
Significant results of the color analysis show that the finger millet was the darkest, having the most redness and blueness of all of the grains. Oppositely, proso millet was the lightest, having the most yellowness and greenness of all of the grains. These values are shown in Table 3. The whiteness and yellowness indices were measured, WI(E313-73) and YI(E313-98), respectively. Deviation from whiteness can be perceived as yellowness, which is not always desirable in food. It is important to establish a base value for each grain in order to identify when one has spoiled, or in a comparison of grains like millets which can be very similar to the eye, it can be a way to differentiate between types. Barnyard millet had the greatest whiteness value, and proso millet had the highest yellowness value. The whiteness and yellowness of each grain were significantly different from each other, respectively. The L* value of each grain was significantly different from each other except for amaranth and kodo. The a* values for ancient grains were significantly different from each other except for proso and little. The b* values for each grain were all significantly different from each other. The distributions of whiteness and yellowness indices Whiteness Index(E313-73) and Yellowness Index(E313-98) and L* values are presented in Figure 3.
Porosity
The porosity, as shown in Table 4, varied from 3.6 to 17.4%, for water displacement method, and 44.5–48.1% with mass/volume method. Kodo millet and sorghum having the least and most porosity, respectively from both methods. The porosities of two rough rice cultivars were found to be 56.98 and 60.4% (Varnamkhasti et al., 2008). The porosity of popcorn kernels decreased from 42.56 to 40.87% when the moisture content of the kernels increased from 8.85 to 17.12% (Karababa, 2006). The porosity of Prosopis africana seed varied from 21.57 to 22.71% at 11% mc (Akaaimo and Raji, 2006).
Scanning Electron Microscopy
The scanning electron microscopy images shown in Table 5 reveal the unique surface characteristics of each grain. Finger millet has a highly textured surface with numerous round protrusions over its entire surface compared to the rest of the grains. Sorghum and pearl millet appear to be relatively smooth. Barnyard millet, kodo millet, little millet, and proso millet all appear to have a rough surface. Amaranth is the only grain that has a distinct separation in its surface, with an outer ring around the grain itself. This is a unique structural feature present in amaranth grains. Wild-type rice is shown to have wax crystals on the outer surface, which gives it a similar appearance to finger millet (Bai et al., 2015). This is the first study to consolidate and report the structural morphology of the ancient grains: amaranth, millets, and sorghum using SEM micrographs.
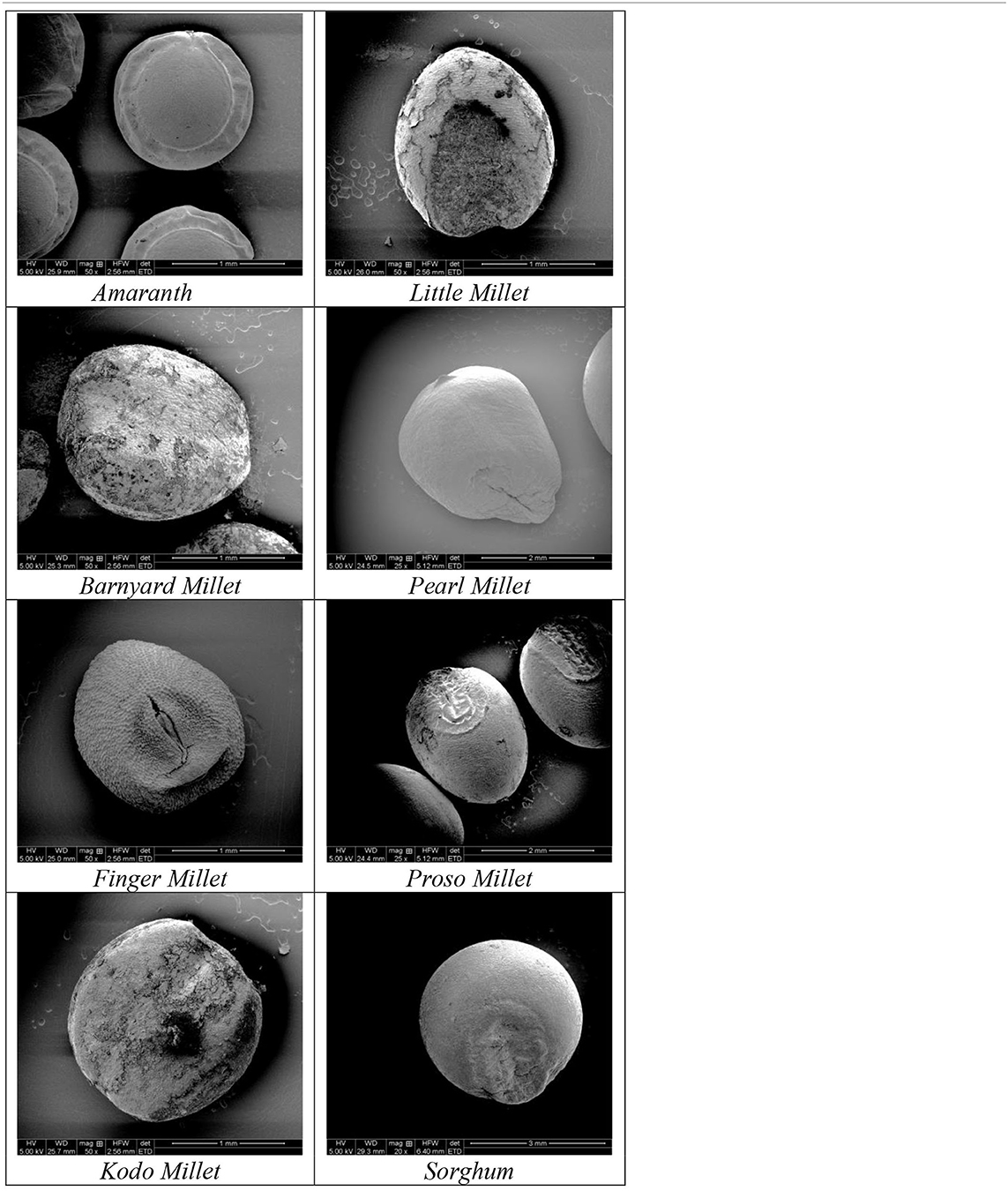
Table 5. Scanning Electron Microscopic (SEM) images of ancient grains: Amaranth, Millets, and Sorghum.
The surface features shown by the SEM photos can be explained due to the mechanical dehulling processes that the grains must undergo to be used as edible food and for further food processing. Little, barnyard, proso, and kodo millets must be dehulled before consuming. While dehulling, the grains experience shearing forces which cause the rough, irregular surfaces. The scanning electron microscopic images of the millets (Table 5) shows the impact of dehulling on the surface morphology of the grains. This is an important observation, as surface morphology affects various physical characterization, including the angle of repose, coefficient of friction, and porosity. This is the first report that provides a microscopic comparison of the surface morphology of ancient seed grains, which would be helpful to advance research related to millets.
Shape and Size
The ancient grains in this study were categorized in three out of the four shape types determined by McDonough and Rooney (1989). Amaranth, barnyard millet, kodo millet, and sorghum were globose in appearance, while finger millet, little millet, and proso millet were obovate. Pearl millet was the only grain with a hexagonal shape.
In increasing order, the size of the grains starts at the smallest with amaranth, followed by finger millet, little millet, barnyard millet, kodo millet, proso millet, pearl millet, and sorghum being the largest.
Baryeh (2002) observed lower bulk density with larger grains. Also showed that millet grains with a lower moisture content had a higher bulk density. Similar to Baryeh (2002), we also found that sorghum, having the larger grain size had lower bulk density using the water displacement method (1,153 ) compared to ancient seed grains with smaller size like amaranth, finger millet, little millet, and barnyard millet (1,324–1,326 ). While comparing the porosity data for sorghum (17.4%) and with other ancient seed grains (amaranth, finger, little, barnyard, kodo, and proso millets) the porosity range was between 3.6 and 8.5%. Similar trends were found for bulk density and porosity using mass/volume method. This could be explained by the fact that smaller grains could fill larger volumes by compactly packing themselves, filling interparticle voids, and minimizing porosity.
Water Activity (aw)
Water activity is an important food safety parameter. Water activity value ranges from 1.0 (pure water) to 0.0 (complete dry conditions). The water activity valve provides an indicator of microorganisms that can grow by utilizing the water in the food samples (ancient seed grains). Higher the water activity, bacteria, yeast, and mold can grow resulting in food spoilage during storage. Food products with a water activity below 0.60 have extended shelf-life during storage.
Water activity varied from 0.533 at a temperature of 25.5°C to 0.660 at a temperature of 25.3°C, with finger millet having the lowest water activity and sorghum having the greatest. The remaining values are shown in Table 3. This study did not study the effect of water activity based on different moisture content. The water activity was measured for all samples upon opening the sealed packaging from the suppliers. Amaranth, barnyard, and pearl can be grouped and were not significantly different from each other in terms of water activity. Likewise, kodo, sorghum, amaranth, and barnyard can be grouped. The water activity of amaranth and little, little and pearl, and kodo and barnyard were not significantly different from each other.
Conclusions
The results of this study show that while the millets appear very similar to the human eye, they all vary with respect to the physical and structural properties. The potential for ancient seed grains to be grown in North America is far greater than what is currently being realized. They are commonly assumed to be useful only in developing countries, but field trials in Missouri and other locations show amaranth and millets to have good adaptation in temperate areas of North America (Myers, 2018b). Further research and breeding could help improve their potential even further, including improved yield and nutritional factors. Parts of the Midwestern and Great Plains areas of the U.S. are particularly viable areas to produce amaranth and other ancient seed grains. This, combined with the minimal water required to grow ancient seed grains like millet and amaranth compared to corn and wheat, could contribute to the growing effort to reduce freshwater consumption during climate crisis.
From the physical and structural characterization study, sorghum had a larger appearance, size, and the lowest bulk density, while kodo millet had the highest bulk density. Kodo millet had less porosity, while sorghum had high porosity from both density measurement methods. This is the first report that provides microstructural comparison clearly showing the impact of mechanical dehulling on the surface morphology of the ancient seed grains, which is invisible to human visual observation. Little, barnyard, proso, and kodo millets must be dehulled to make the seeds edible for human consumption. This study reports important microstructural observations that could directly impact physical characterization, including the angle of repose, coefficient of friction, porosity, and development of food processing equipment.
Color analysis shows that the finger millet was the darkest, having the most redness, and blueness of all the ancient seed grains. Barnyard millet had the greatest whiteness value (L), and proso millet had the highest yellowness value. The whiteness and yellowness of each ancient seed grain were significantly different from each other. This is an important food processing parameter to consider while developing new food products, ingredients from millets and would be of interest to food industries and plant-based food start-up companies.
This observational study establishes the methodology for physical characterizations of ancient grains. Further investigation on specific genetic traits, the effect of moisture content on the physio-chemical properties for different ancient seed grains is recommended. Since each ancient seed gain exhibits different physical and structural properties, further research, including chemical, functional properties, and nutrigenomics of the ancient seed grains for defined genetic lines, into environmental growth conditions is recommended to develop new products with enhanced health benefits. New value-added functional/nutraceutical food products can be formulated using single seed grains or the combination of amaranth as well as barnyard, finger, kodo, little, pearl, proso millets, and sorghum to balance macro/micronutrient profiles.
To develop sustainable food processing technologies to utilize climate-resilient crops and improve agri-business, economic activity, and support for farming communities, a partnership between different stakeholders in food systems is critical. We conclude that ancient climate-resilient seed grains like millets, sorghum, and amaranth can lead to diversification of sustainable diets and have a positive impact to improve the health of people and the planet.
Data Availability Statement
The original contributions presented in the study are included in the article/Supplementary Material, further inquiries can be directed to the corresponding author/s.
Author Contributions
SG contributed to the formal analysis, investigation, visualization, and writing (original draft, reviewing, and editing). RM was responsible for the resources and the writing (review and editing). KK carried out the conceptualization, resource management, methodology, visualization, writing (review and editing), supervision, funding acquisition, and project administration. All authors contributed to the article and approved the submitted version.
Funding
This work was supported by USDA Hatch Funds (MO-HAFE0003) Food Engineering and Sustainable Technologies (FEAST), University of Missouri.
Conflict of Interest
The authors declare that the research was conducted in the absence of any commercial or financial relationships that could be construed as a potential conflict of interest.
Acknowledgments
We would like to thank the University of Missouri Electron Microscopy Core for being inclusive and providing training to Undergraduate Students to use the Scanning Electron Microscope for analysis of ancient seed grains. We would like to thank Ms. Mercy Oghenerukewe Nani for the analysis and identifying local names for millets from the African continent context.
References
International Crops Research Institute for the Semi-Arid Tropics (ICRISAT) (2021). Retrieved from: http://exploreit.icrisat.org/profile/Small%20millets/187 (accessed May 19, 2021).
Abalone, R., Cassinera, A., Gaston, A., and Lara, M. A. (2004). Some physical properties of amaranth seeds. Biosyst. Eng. 89, 109–117. doi: 10.1016/j.biosystemseng.2004.06.012
Akaaimo, D. I., and Raji, A. O. (2006). Some physical and engineering properties of prosopis africana seed. Biosyst. Eng. 95, 197–205. doi: 10.1016/j.biosystemseng.2006.06.005
Amaranth: Pigweed Kin High in Protein. (2003). Retrieved from: https://www.auri.org/2003/07/amaranth/ (accessed October 2, 2019).
Arendt, E. K., and Zannini, E. (2013). Cereal Grains for the Food and Beverage Industries. Cambridge: Elsevier.
Arreguez, G. A., Martínez, J. G., and Ponessa, G. (2013). Amaranthus hybridus L. ssp. hybridus in an archaeological site from the initial mid-Holocene in the Southern Argentinian Puna. Quatern. Int. 307, 81–85. doi: 10.1016/j.quaint.2013.02.035
Bai, J., Zhu, X., Wang, Q., Zhang, J., Chen, H., and Dong, G.. (2015). Rice TUTOU1 encodes a suppressor of cAMP receptor-like protein that is important for actin organization and panicle development. Plant Physiol. 169, 1179–1191. doi: 10.1104/pp.15.00229
Baryeh, E. A. (2001). Physical properties of bambara groundnuts. J. Food Eng. 47, 321–326. doi: 10.1016/S0260-8774(00)00136-9
Baryeh, E. A. (2002). Physical properties of millet. J. Food Eng. 51, 39–46. doi: 10.1016/S0260-8774(01)00035-8
Bhadra, R., Casada, M. E., Thompson, S. A., Boac, J. M., Maghirang, R. G., Montross, M. D., et al. (2017). Field-observed angles of repose for stored grain in the United States. Appl. Eng. Agric. 33, 131–137. doi: 10.13031/aea.11894
Davies, R. M., and El-Okene, A. M. (2009). Moisture-dependent physical properties of soybeans. Int. Agrophys. 23, 299–230.
de Wet, J. M., Brink, D. E., Rao, K. P., and Mengesha, M. H. (1983). Diversity in kodo millet, Paspalum scrobiculatum. Econ. Bot. 37, 159–163.
De Wet, J. M. J., and Harlan, J. R. (1971). The origin and domestication of Sorghum bicolor. Econ. Bot. 25, 128–135.
FAO ICRISAT. (1996). The World Sorghum and Millet Economies: Facts, Trends and Outlook. Retrieved from: http://www.fao.org/3/W1808E/w1808e0c.htm (accessed October 2, 2019).
FAOSTAT. (2019). Crops. Retrieved from: http://www.fao.org/faostat/en/#data/QC (accessed October 2, 2019).
Fuller, D. Q. (2011). Finding plant domestication in the Indian subcontinent. Curr. Anthropol. 52, S347–S362. doi: 10.1086/658900
Govindaraj, M., Rai, K. N., Kanatti, A., Rao, A. S., and Shivade, H. (2019). Nutritional security in drylands: fast-track intra-population genetic improvement for grain iron and zinc densities in pearl millet. Front. Nutr. 6:74. doi: 10.3389/fnut.2019.00074
Hilu, K. W., De Wet, J. M. J., and Harlan, J. R. (1979). Archaeobotanical studies of Eleusine coracana ssp. coracana (finger millet). Am. J. Bot. 66, 330–333.
ICAR- Indian Institute of Millets Research (IIMR) (2020). Retrieved from: https://millets.res.in/ (accessed May 19, 2021).
Joshi, D. C., Sood, S., Hosahatti, R., Kant, L., Pattanayak, A., Kumar, A., et al. (2018). From zero to hero: the past, present and future of grain amaranth breeding. Theor. Appl. Genet. 131, 1807–1823. doi: 10.1007/s00122-018-3138-y
Karababa, E. (2006). Physical properties of popcorn kernels. J. Food Eng. 72, 100–107. doi: 10.1016/j.jfoodeng.2004.11.028
Kibar, H., and Öztürk, T. (2008). Physical and mechanical properties of soybean. Int. Agrophys. 22, 239–244.
Kumar, A., Tomer, V., Kaur, A., Kumar, V., and Gupta, K. (2018). Millets: a solution to agrarian and nutritional challenges. Agric. Food Sec. 7, 1–15. doi: 10.1186/s40066-018-0183-3
Lorraine, F., and Flint, A. (2002). Porosity. Methods Soil Anal. 4, 241–254. doi: 10.2136/sssabookser5.4.c11
Louw, M. (2020a). Sorghum in South Africa South African Indigenous Grains. Available online at: https://southafrica.co.za/sorghum-in-south-africa.html (accessed September 25, 2020).
Louw, M. (2020b). What is Pearl Millet? South African Indigenous Grains. Available online at: https://southafrica.co.za/what-is-pearl-millet.html (accessed September 25, 2020).
Lu, H., Zhang, J., Liu, K. B., Wu, N., Li, Y., Zhou, K., et al. (2009). Earliest domestication of common millet (Panicum miliaceum) in East Asia extended to 10,000 years ago. Proc. Natl. Acad. Sci. U.S.A. 106, 7367–7372. doi: 10.1073/pnas.0900158106
Manger (1966). Method-dependent values of bulk, grain, and pore volume as related to observed porosity. Geological Survey Bulletin. doi: 10.3133/b1203D
Manning, K., Pelling, R., Higham, T., Schwenniger, J. L., and Fuller, D. Q. (2011). 4500-Year old domesticated pearl millet (Pennisetum glaucum) from the Tilemsi Valley, Mali: new insights into an alternative cereal domestication pathway. J. Archaeol. Sci. 38, 312–322. doi: 10.1016/j.jas.2010.09.007
Mariotti, M., Alamprese, C., Pagani, M. A., and Lucisano, M. (2006). Effect of puffing on ultrastructure and physical characteristics of cereal grains and flours. J. Cereal Sci. 43, 47–56. doi: 10.1016/j.jcs.2005.06.007
McDonough, C. M., and Rooney, L. W. (1989). Structural characteristics of Pennisetum Americanum (pearl millet) using scanning electron and fluorescence microscopy. Food Struct. 8, 137–149.
Mehta, S., Finkelstein, J. L., Venkatramanan, S., Huey, S. L., Udipi, S. A., Ghugre, P., et al. (2017). Effect of iron and zinc-biofortified pearl millet consumption on growth and immune competence in children aged 12–18 months in India: study protocol for a randomized controlled trial. BMJ Open 7:e017631. doi: 10.1136/bmjopen-2017-017631
Mohsenin, N. N. (1986). Physical Properties of Plant and Animal Materials, 2nd Edn. New York, NY: Gordon and Breach Science Publishers.
Myers, R. (2018a). Amaranth: An Ancient 3 Grain and Exceptionally Nutritious Food. Columbia, MO: Harvest Road Publishing.
Myers, R. (2018b). Growing Millets for Grain, Forage or Cover Crop Use. University of Missouri Extension Bulletin G4164. Retrieved from: https://extension.missouri.edu/publications/g4164 (accessed May 19, 2021).
Nasu, H., and Momohara, A. (2016). The beginnings of rice and millet agriculture in prehistoric Japan. Quatern. Int. 397, 504–512. doi: 10.1016/j.quaint.2015.06.043
Nithiyanantham, S., Kalaiselvi, P., Mahomoodally, M. F., Zengin, G., Abirami, A., and Srinivasan, G. (2019). Nutritional and functional roles of millets-A review. J. Food Biochem. 43:e12859. doi: 10.1111/jfbc.12859
Robinson, R. G. (1986). Amaranth, Quinoa, Ragi, Tef, and Niger: Tiny Seeds of Ancient History and Modern Interest. University of Minnesota Station Bulletin 571 AD-SB-2949. Retrieved from: https://hdl.handle.net/11299/139533 (accessed May 19, 2021).
Saleh, A. S. M., Zhang, Q., Chen, J., and Shen, Q. (2013). Millet grains: nutritional quality, processing, and potential health benefits. Compreh. Rev. Food Sci. Food Saf. 12, 281–295. doi: 10.1111/1541-4337.12012
Saxena, R., Vanga, S. K., Wang, A., Orsat, A., and Raghavan, V. (2018). Millets for food security in the context of climate change: A review. Sustainability 10:2228. doi: 10.3390/su10072228
Singh, K. P., Mishra, H. N., and Saha, S. (2010). Moisture-dependent properties of barnyard millet grain and kernel. J. Food Eng. 96, 598–606. doi: 10.1016/j.jfoodeng.2009.09.007
Subramanian, S., and Viswanathan, R. (2003). Thermal properties of minor millet grains and flours. Biosyst. Eng. 84, 289–296. doi: 10.1016/S1537-5110(02)00222-2
Taylor, J., and Duodu, K. G., (eds.). (2018). Sorghum and Millets: Chemistry, Technology, and Nutritional Attributes. Cambridge, MA: Elsevier.
The United Sorghum Checkoff Program. (2020). All About Sorghum. Retrieved from: https://www.sorghumcheckoff.com/all-about-sorghum (accessed October 2, 2019).
United Nations (2020). The Sustainable Development Agenda. Available online at: https://www.un.org/sustainabledevelopment/development-agenda/ (accessed May 19, 2021).
Valcárcel-Yamani, B., and Caetano da Silva Lannes, S. (2012). Applications of Quinoa (Chenopodium Quinoa Willd.) and Amaranth (Amaranthus Spp.) and their influence in the nutritional value of cereal based foods. Food Public Health 2, 265–275. doi: 10.5923/j.fph.20120206.12
Keywords: millet, scanning electron microscope, food and nutrition security, sustainable diets, SDG #2, ancient grains, UN sustainable development goal
Citation: Geisen S, Krishnaswamy K and Myers R (2021) Physical and Structural Characterization of Underutilized Climate-Resilient Seed Grains: Millets, Sorghum, and Amaranth. Front. Sustain. Food Syst. 5:599656. doi: 10.3389/fsufs.2021.599656
Received: 27 August 2020; Accepted: 20 April 2021;
Published: 10 June 2021.
Edited by:
José Antonio Teixeira, University of Minho, PortugalReviewed by:
Vetriventhan Mani, International Crops Research Institute for the Semi-Arid Tropics (ICRISAT), IndiaSean Mayes, University of Nottingham, United Kingdom
Copyright © 2021 Geisen, Krishnaswamy and Myers. This is an open-access article distributed under the terms of the Creative Commons Attribution License (CC BY). The use, distribution or reproduction in other forums is permitted, provided the original author(s) and the copyright owner(s) are credited and that the original publication in this journal is cited, in accordance with accepted academic practice. No use, distribution or reproduction is permitted which does not comply with these terms.
*Correspondence: Kiruba Krishnaswamy, a3Jpc2huYXN3YW15a0B1bXN5c3RlbS5lZHU=