- 1Department of Environmental Science, Policy, and Management, University of California, Berkeley, Berkeley, CA, United States
- 2Department of Fisheries, Animal, and Veterinary Sciences, University of Rhode Island, Kingston, RI, United States
- 3Plant Soil Interactions, Division Agroecology and Environment, Agroscope, Zurich, Switzerland
- 4Department of Plant and Microbial Biology, University of Zurich, Zurich, Switzerland
- 5Department of Social, Economic and Geographical Sciences, The James Hutton Institute, Aberdeen, United Kingdom
- 6SAW-B (Solidarité des Alternatives Wallonnes et Bruxelloises), Monceau-sur-Sambre, Belgium
- 7Department of Zoology, Biodiversity Research Centre and Institute for Resources, Environment and Sustainability, University of British Columbia, Vancouver, BC, Canada
Humanity faces a triple threat of climate change, biodiversity loss, and global food insecurity. In response, increasing the general adaptive capacity of farming systems is essential. We identify two divergent strategies for building adaptive capacity. Simplifying processes seek to narrowly maximize production by shifting the basis of agricultural production toward centralized control of socially and ecologically homogenized systems. Diversifying processes cultivate social-ecological complexity in order to provide multiple ecosystem services, maintain management flexibility, and promote coordinated adaptation across levels. Through five primarily United States focused cases of distinct agricultural challenges—foodborne pathogens, drought, marginal lands, labor availability, and land access and tenure—we compare simplifying and diversifying responses to assess how these pathways differentially enhance or degrade the adaptive capacity of farming systems in the context of the triple threat. These cases show that diversifying processes can weave a form of broad and nimble adaptive capacity that is fundamentally distinct from the narrow and brittle adaptive capacity produced through simplification. We find that while there are structural limitations and tradeoffs to diversifying processes, adaptive capacity can be facilitated by empowering people and enhancing ecosystem functionality to proactively distribute resources and knowledge where needed and to nimbly respond to changing circumstances. Our cases suggest that, in order to garner the most adaptive benefits from diversification, farming systems should balance the pursuit of multiple goals, which in turn requires an inclusive process for active dialogue and negotiation among diverse perspectives. Instead of locking farming systems into pernicious cycles that reproduce social and ecological externalities, diversification processes can enable nimble responses to a broad spectrum of possible stressors and shocks, while also promoting social equity and ecological sustainability.
Introduction
Climate change, biodiversity loss, and global food insecurity present an Anthropocene triple threat for humanity (Kremen and Merenlender, 2018). The current global agrifood system contributes to the triple threat by emitting 23% of global greenhouse gases (IPCC, 2019), reducing biodiversity (Dainese et al., 2019), displacing traditional foodways and knowledge (Altieri, 1999; Hoover, 2017; White, 2017), and contributing to the decline of rural communities (Carolan, 2016). Although farmers have always dealt with climatic, ecological, socioeconomic, and political challenges that test their ability to continue farming, these long-standing “normal” challenges will be transformed, predominantly for the worse, by the novel shocks and stressors emanating from the triple threat (Figure 1 and Box 1). These threats and challenges partly arise from and are exacerbated by the well-known social and environmental externalities generated by industrialized agricultural systems (Kremen and Merenlender, 2018). In order to reduce social inequity and environmental destruction, and adapt to an increasingly uncertain future, there is growing consensus that our agricultural system must undergo systemic, transformative change (McIntyre et al., 2010; International Panel of Experts on Sustainable Food Systems, 2018; IPCC, 2019). Transformation can occur rapidly, or can emerge from incremental progress along context-specific transition pathways that influence adaptive capacity (Box 1), or the ability to respond flexibly and effectively to changing circumstances (Wilson, 2007; Tomich et al., 2011; Anderson et al., 2019; Chhetri et al., 2019). The scale and scope of response matters, and varies along a spectrum ranging from simply coping with the impacts of shocks and stressors in the moment to re-imagining and reconfiguring the structural conditions and drivers that give rise to those shocks and stressors (Van Noordwijk et al., 2020). As such, farmers, agricultural service providers, academics, and policymakers must consider not only how transformation pathways address present social and environmental problems, but also how they build, do not build, or undermine the capacity to adapt to rapidly changing and unexpected biophysical and social challenges into the future.
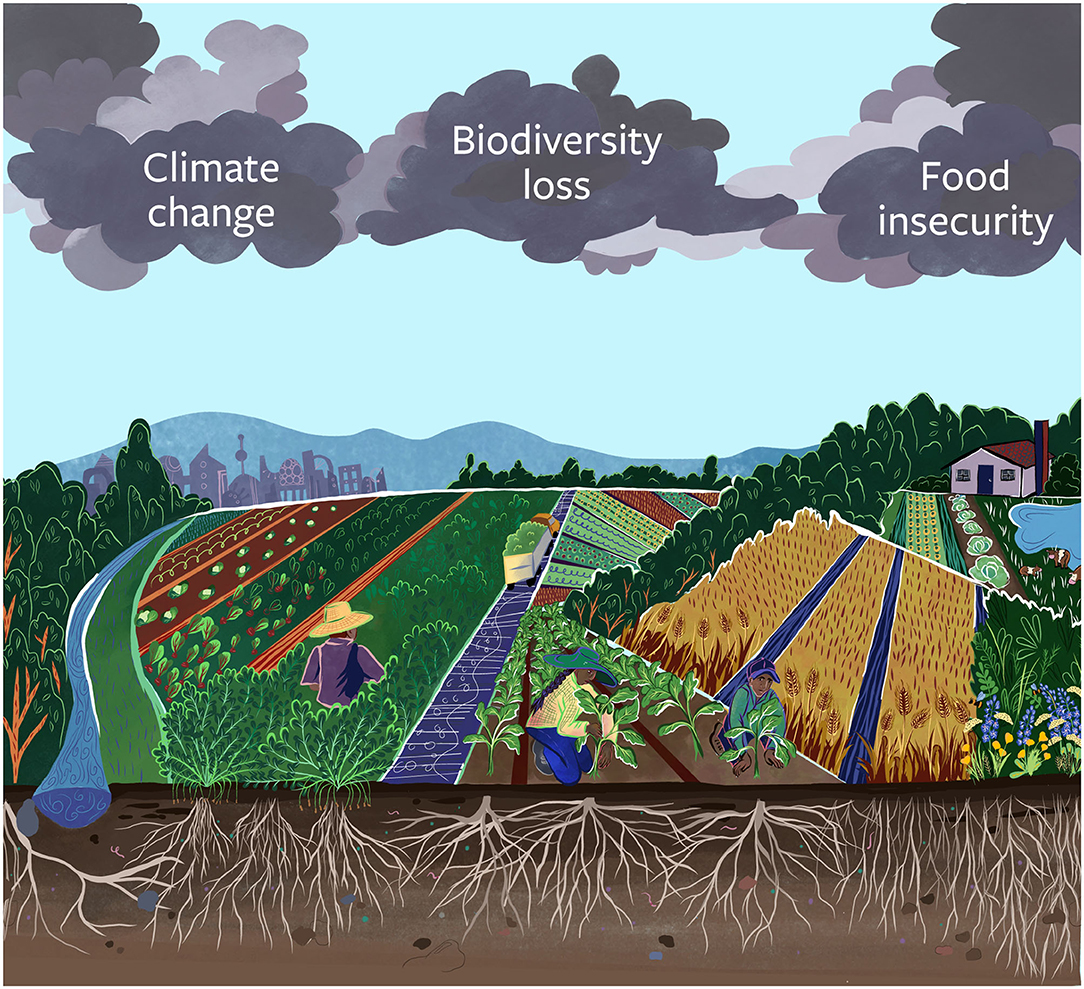
Figure 1. The Anthropocene “triple threat”—climate change, biodiversity loss, and global food insecurity—interact in ways that will exacerbate long-standing climatic, ecological, socioeconomic, and political challenges for agriculture and food systems. The process through which social-ecological systems build adaptive capacity to these looming threats shapes future adaptation and transformation possibilities.
Box 1. Definitions of key terms.
For the purposes of this paper, we define several terms to distinguish between different types of pressures that influence farming systems:
•Threats operate at a global scale to drive change in the focal social-ecological system, often exacerbating challenges
•Stressors and shocks are temporally or spatially localized manifestations of threats. Stressors are persistent changes in “slow variables” that create gradual or chronic pressure on farming systems over time, while shocks are sudden changes in “quick variables” that create acute pressure on farming systems
•Challenges are “normal” pressures faced by agriculturalists, such as accessing enough water, land, and labor to produce crops
Response to these pressures and their impacts can, broadly speaking, form two divergent pathways toward adaptive capacity:
•Simplifying processes seek to narrowly maximize production by shifting the basis of agricultural production toward centralized control of socially and ecologically homogenized systems
•Diversifying processes cultivate social-ecological complexity in order to provide multiple ecosystem services, maintain management flexibility, and promote coordinated adaptation across levels
•Processes comprise the knowledges, strategies, practices, policies, and technologies that can alter farming systems across levels
•Pathways describe the directionality and continuity of a suite of processes that are situated in broader social and ecological contexts, wherein historical actions shape future possibilities
Many proposed approaches to increase adaptive capacity are goal-oriented, without an explicit focus on process. For example, “climate-smart agriculture” defines clear goals— to simultaneously increase yields, improve resilience to climate change, and reduce greenhouse emissions (Lipper et al., 2014)—yet appears agnostic with regard to the pathway taken to achieve those goals. Sustainable intensification similarly emphasizes optimizing stable productivity, which could be pursued through many routes ranging from narrowly increasing input use efficiency to completely redesigning agricultural systems (Campbell et al., 2014; Pretty, 2018). Approaches like these, which isolate the ends from the means, fail to differentiate how all the tools in the toolbox function socially, and avoid crucial processual questions: How do various strategies differentially distribute benefits and costs of adaptation? In what ways do different processes influence vulnerability or resilience of social and ecological functions beyond farm productivity? Who controls access to these tools, and who is excluded? No approach to adaptation can constitute a coherent strategy without also addressing these questions.
To address this gap, we propose a process-oriented approach to adaptation rooted in strategies of diversification. Drawing upon the paradigm of agroecology, the theory of diversified farming systems (DFS) proposes that adoption of biodiversity-enhancing practices can increase the magnitude and stability of ecosystem services and simultaneously reduce or eliminate the need for external inputs, reduce negative externalities, and increase positive on-farm outcomes (Kremen et al., 2012; Rosa-Schleich et al., 2019). Networking experiential and scientific knowledge helps farmers flexibly employ different suites of management practices to fit their situated goals and constraints (Vandermeer and Perfecto, 2017). Recent interdisciplinary scholarship further links DFS success to the diversity of disciplines, practitioners, markets, ideas, and cultures in farming systems through the knowledge density required to productively manage biodiversity in a specific place and time (Timmermann and Félix, 2015; Dumont et al., 2016; Carlisle et al., 2019a). Other scholarship has also synthesized how the diversification transition can happen, and the barriers and opportunities that exist across different institutional scales (International Panel of Experts on Sustainable Food Systems, 2016, 2018).
We expand upon the DFS framework in two important ways. First, while previous DFS work focused on biodiversification and managerial diversification at farm and landscape levels, we weave in further dimensions of cultural, economic, epistemic, and organizational diversity across multiple social relational levels from the farm enterprise to national policies (Carlisle, 2014). Thus, in addition to indicators in genetic, crop, ecosystem, functional, and managerial diversity, we include diversification of societal goals, market channels, governance arrangements, knowledge production infrastructures, and social networks. Second, we distinguish the state of being diversified from the process of diversification, which represents iterative socio-ecological transition pathways and can broaden individual and collective participation in sustainable adaptation irrespective of scale or starting point.
This paper frames a Research Topic on Diversifying Farming Systems for Adaptive Capacity in Frontiers in Sustainable Food Systems by: (a) Briefly reviewing the ways that climate change, biodiversity loss, and food insecurity impact the adaptive capacity of agricultural systems; (b) Contrasting the implications for equity and sustainability of diversifying as opposed to simplifying processes for building adaptive capacity; (c) Analyzing these processes through five cases that exemplify ongoing challenges compounded by the triple threat; and (d) Presenting a novel framework to explore how diversifying processes influence adaptive capacity to shocks and stressors emanating from the triple threat. Moreover, our framework explicitly considers whether and in what ways diversifying pathways can lead to the emergence of different qualities of adaptive capacity that also enhance sustainability and equity more broadly.
Background
The Anthropocene Triple Threat
Climate change already impacts farmers around the world. The increase in frequency and intensity of weather extremes (e.g., droughts, heat waves, hurricanes, and floods), together with the spread of novel diseases and pests, altered growing seasons, and fewer chill hours, reduces crop yields in many regions and increases environmental degradation such as nitrogen pollution and soil erosion (Bowles et al., 2018; IPCC, 2019). The complex interaction of acute shocks and chronic stressors produces both discrete events that can lead to abrupt agroecosystem collapse, like widespread crop failure, and damage to farming infrastructure, as well as continuous deterioration that gradually undermines productivity and resilience to acute shocks (Tomich et al., 2011). Directly and indirectly, climate change also impacts livelihoods by inducing rural migration, reducing food security, worsening inequalities, and spurring resource conflict, to name a few examples (Hsiang et al., 2013; Burrows and Kinney, 2016; Nawrotzki et al., 2017; Jha et al., 2018). Although mitigation remains critical, adaptation imperative. In order to respond to ongoing and future climate change already incurred from past emissions, farmers must find long-term solutions suitable to new climatic norms (Steffen et al., 2018; IPCC, 2019).
Biodiversity is rapidly declining across the globe (Dainese et al., 2019; IPBES, 2019), altering ecosystem functions and jeopardizing ecosystem services that are essential for human well-being (Hooper et al., 2005). The alarming rate of species loss through extinction is compounded by dramatically declining biomass of taxa like insects and birds (Hallmann et al., 2017; Wagner, 2020). Some of the primary drivers of biodiversity loss are habitat loss and fragmentation, as well as chemical pollution from industrial agriculture (Dainese et al., 2019). Global agricultural simplification has also eroded crop genetic diversity, which is critical for adaptive crop breeding (Jackson et al., 2013; Veteto and Carlson, 2014; Zimmerer and de Haan, 2017) and productivity in marginal environments (Altieri, 1999). Some studies have shown that certain ecosystem services can persist with merely a few species under ideal conditions (Kleijn et al., 2015). But many more species are required when considering additional services, larger spatial or temporal scales, and variable environments (Kremen, 2005; Isbell et al., 2011, 2017; Reich et al., 2012). Increasing biodiversity in agricultural landscapes can help these systems maintain multiple critical functions, such as pest control and protection of water quality, in the face of climate change (Bowles et al., 2018; Kremen and Merenlender, 2018).
Confronting these momentous environmental changes (Figure 1), it is essential to produce food in ways that sustainably and equitably assure the basic human right to food (De Schutter, 2011). Globally, two billion people experience moderate or severe food insecurity, including uncertainty about obtaining food and compromising quality or quantity of food consumed, a number that is rapidly rising with the COVID-19 pandemic (FAO, 2020). Healthy ecosystems and rural livelihoods are integrally linked to food security (Chappell, 2018). While strategies such as sustainable intensification focus on maximizing productivity and reducing environmental externalities (Garnett et al., 2013; Rockström et al., 2017), they fail to address the underlying inequities that cause food insecurity and the ways in which capital-intensive “solutions” exacerbate social and ecological vulnerabilities (International Panel of Experts on Sustainable Food Systems, 2018), which perversely undermines the human right to food. Food insecurity is an issue of access, not production. The world currently produces enough food to feed all of humanity (Patel and Moore, 2017; Chappell, 2018), but a large portion is either wasted, used for animal feed, or used to manufacture non-food products such as biofuels (Cassidy et al., 2013). Globally, access to food continues to be grossly unequal (Patel and Moore, 2017), and food insecurity is linked to the erosion of agricultural sovereignty, local foodways, experiential knowledge, and farming livelihoods, as well as land degradation, particularly in many regions of the Global South (Altieri and Toledo, 2011; Wittman, 2011; Edelman, 2014). To fully realize the human right to food, agricultural systems must maintain critical ecosystem services while also meeting the intertwined challenges of access, adequacy, acceptability, appropriateness, and agency (Chappell, 2018).
The triple threat of climate change, biodiversity loss, and global food insecurity intersect to exacerbate the challenges farmers and ranchers already face (Table 1). For example, climate change increases the intensity and frequency of droughts, while diminished biodiversity limits ecological management options to cope with drought, and the combined effects ripple and magnify through synchronized markets, reducing global food security.
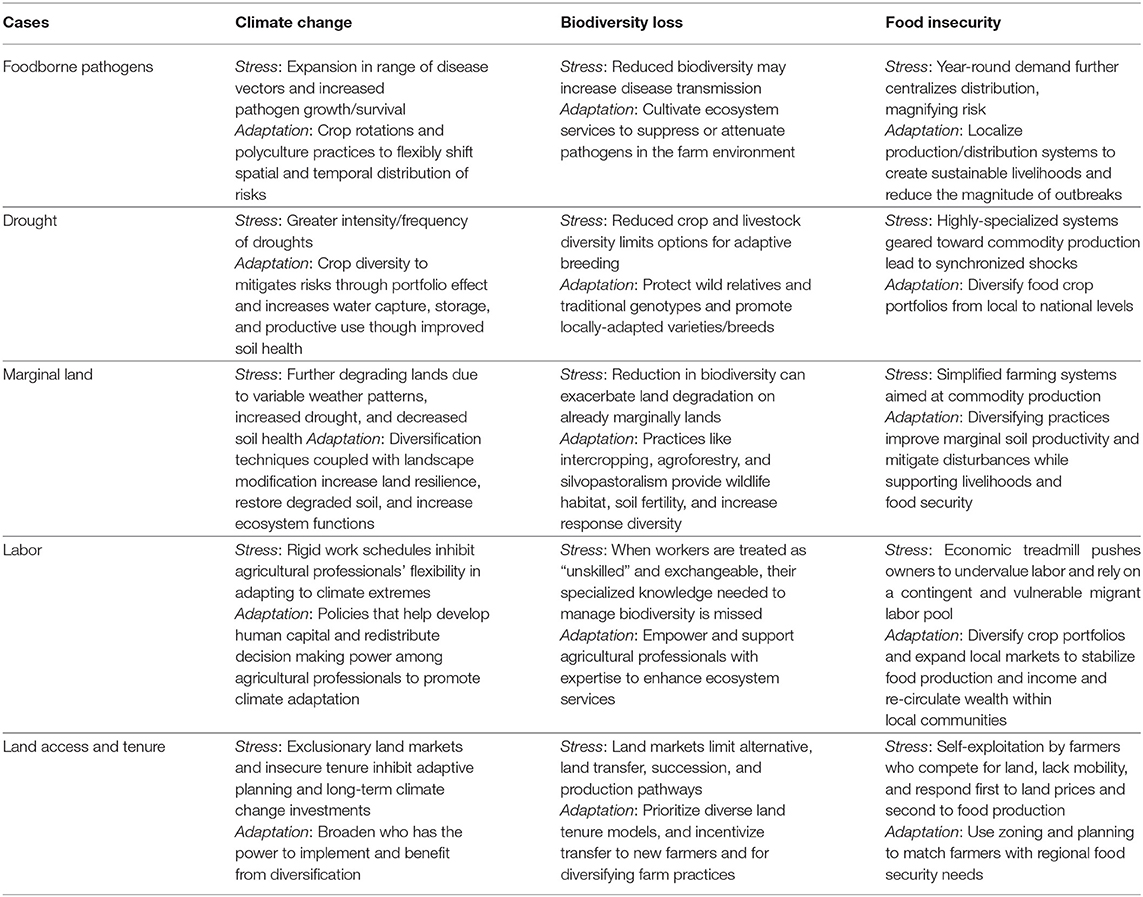
Table 1. Increased stresses from, and potential diversifying adaptations to, the triple threat for each of the five cases.
Defining Adaptive Capacity
Adaptive capacity is the ability to adapt to changing circumstances (Engle, 2011). In much of the literature, adaptive capacity is used specifically in the context of climate change (McLeman and Hunter, 2010; Liverman, 2015), but the concept also accommodates other types of change. Adaptive capacity, vulnerability, and resilience are highly interrelated concepts (Gallopín, 2006) that all describe how changes affect a system in terms of susceptibility and responses to change. The vulnerability of a system to a particular stress or shock is widely accepted to be a function of (1) the sensitivity and exposure of that system to the perturbation and (2) the response capacity, described as the system's ability to cope, resist, adapt, recover, or take advantage of the opportunities arising from the consequences of the perturbation (Smit and Wandel, 2006). Adaptive capacity is sometimes seen as interchangeable with response capacity (Smit and Wandel, 2006; IPCC, 2019), but others recognize adaptive capacity as a broader concept (Gallopín, 2006), since specific adaptations may actually influence the sensitivity or exposure of a system to particular perturbations, or increase a system's resilience (Walker et al., 2004). A concept with roots in ecology, resilience has traditionally been defined as the extent to which systems can absorb a perturbation while remaining in, or returning to, a state with essentially the same structure, function, identity, and feedbacks (Gunderson and Holling, 2001; Walker et al., 2004; Folke, 2006). Resilience has been extended to include possibilities of transformation to other stable states with more desirable attributes (Folke et al., 2010), which is crucial in our understanding of adaptive capacity that encompasses transformation.
Although scholars often apply these concepts to either social or biophysical dimensions, an interconnected social-ecological system is the most relevant analytical unit in agricultural systems (Folke, 2006; Gallopín, 2006). We thus define agricultural adaptive capacity as the extent to which agricultural systems can respond to the triple threat in ways that, at a minimum, preserve core social-ecological functions, and which ideally make progress toward greater equity and sustainability. Connections between adaptive capacity and sustainability are well-captured in the term “sustainagility,” which emphasizes the need for agile responses to unforeseen change while also considering sustainability tradeoffs across multiple levels (Jackson et al., 2010). Conceptualizing agricultural systems as complex social-ecological systems captures the reciprocal interactions between people and the environment and can be defined at multiple levels (e.g., an individual farm or household, a community, a region etc.). Since the adaptive capacity of each level depends on levels below and above and can vary in time and space, a multidimensional perspective is essential for understanding adaptive capacity. Adaptive capacity must be conceptualized as an emergent property of social-ecological systems. It cannot be broken down into component parts or studied in isolation. For instance, at the scale of a farm, adaptive capacity emerges from the collective, intertwined relationships happening on the farm and in the surrounding landscapes and communities. Moreover, the qualities of adaptive capacity that emerge vary depending on the social-ecological processes of the system from which it emerges. More diverse and inclusive processes, for example, may be better able to create qualities of adaptive capacity that include components of social justice and sustainability.
Strengthening the adaptive capacity of agricultural systems depends on several factors (Darnhofer et al., 2010). Adaptive capacity encompasses both proactive and reactive responses to change, reducing vulnerability, and increasing resilience to a particular stressor (Engle, 2011). Proactive measures depend not only on the ability to anticipate what might happen in the future, but also on the ability to learn from past experiences and from other examples of what has and has not worked in similar circumstances (Fazey et al., 2007; Darnhofer et al., 2010; Engle, 2011). Yet since the future may not have a prolog in the past, and since changes and impacts may be varied and uncertain, flexibility is also key to strengthening adaptive capacity (Darnhofer et al., 2010). Diversity enables flexibility by increasing options for adaptation in the face of stressors, and also lowers vulnerability by helping to spread risks. Finally, adaptive capacity also depends on the resource base available (e.g., agrobiodiversity; Jackson et al., 2010), and the human, economic, and social capital needed to make use of it.
Simplifying vs. Diversifying Pathways for Adaptive Capacity
Adaptation strategies can be based on simplifying processes or diversifying processes. Farmers and farming systems may follow either set of processes in seeking to adapt to changing biophysical and socioeconomic conditions, which form divergent but directionally reinforcing pathways. Adaptation pathways embed changing conditions and response processes within broader social-ecological contexts, wherein historical actions shape future possibilities (Wyborn et al., 2015). Although defining approaches to adaptive capacity along a single axis cannot capture the full complexity involved, we believe these broadly divergent pathways provide a useful heuristic that can be adjusted to specific contexts.
Simplifying Pathways
Around the globe and across various kinds of agriculture, simplifying processes iteratively shift the basis of agricultural production from complex ecological systems toward centralized control of socially and ecologically homogenized systems (Vandermeer et al., 1998), although the extent varies by biome, availability of capital assets, agroecological knowledge, and sociopolitical organization (Jackson et al., 2012). Against the perennial challenges of variable environments and markets, simplifying “fixes” promise greater control and scalability in agriculture (Henke, 2008). Simplifying farming systems are characterized by (1) high-yielding crop and livestock varieties dependent on non-renewable, synthetic inputs manufactured off-farm (i.e., seeds, agrichemicals, equipment), and (2) increasingly concentrated markets, both for those upstream inputs to agriculture and for downstream markets for agricultural products (Block, 1990). Such processes result in greater specialization and uniformity in ecologies, landscapes, technologies, labor practices, and knowledge across large scales.
Simplifying processes offer short-term benefits to some growers, generally those who can access capital-intensive technologies, inputs, and other resources that grant them temporary production advantages over their market competitors. However, that advantage fades as other farmers either follow suit or exit agriculture, setting up the next cycle of a “technological treadmill” (Cochrane, 1993) and locking farmers into dependence on purchased proprietary inputs (Busch, 2010). Many farmers do not choose simplifying processes per-se, but are compelled to simplify in order to compete in a globalized economy shaped by the interlocking forces of market concentration, land consolidation, and crop and livestock homogenization (International Panel of Experts on Sustainable Food Systems, 2017). Concentration of market shares for agricultural inputs (e.g., machinery or agrichemicals) and products (food, fiber, and fuel) occurs through horizontal and vertical integration, in which a few firms steadily buy up their competitors and/or their suppliers (Hendrickson and Heffernan, 2002; Howard, 2016). Consolidation of farm and land ownership occurs both as farmers become locked into a downward economic spiral—in which they must take on debt to purchase increasingly capital-intensive inputs in the face of steadily shrinking profit margins—and through farmland financialization, in which non-farmers use new forms of financial investment to profit from farmland. This process drives a trend toward increasing farmer tenancy and absentee land ownership, which siphons wealth away from rural communities and limits the range of viable farm business models (Cochrane, 1993; Hendrickson and Heffernan, 2002; Bernstein, 2010; Howard, 2016; Fairbairn et al., 2021). Homogenization refers to the rapid decline of crop and livestock diversity across both farm and landscape scales due to specialization in commodity crops for global markets (Khoury et al., 2014); increasing concentration of the global seed market (Howard, 2020); and privatization of plant genetic resources (Kloppenburg, 2005; Montenegro de Wit, 2017b). These interlocking forces result in more homogenous landscapes characterized by the widespread cultivation of just a few varieties of crops or livestock and severe reduction of natural habitats.
In essence, these simplifying forces produce many losers and a few winners, exacerbating inequity in farming systems. In the US, for example, owner-operated farms have declined in number over the last century, especially for Black farmers (White, 2018), as concentration, consolidation, and homogenization have disadvantaged small and midsize farmers (De Master, 2018). While some farms grow larger and more profitable, benefiting more from government subsidies and bailouts, the majority of small and mid-sized farms, especially those operated by farmers of color, struggle to survive. Meanwhile, the remaining larger farms tend to become inflexibly integrated into fixed national and international supply chains, rendering the food system less flexible and adaptable to dramatic market changes. As food crises caused by the COVID-19 epidemic illustrate, the vulnerability of long supply chains and centralized food distribution channels renders this highly simplified system vulnerable (Heinberg, 2020; Ransom et al., 2020).
As agriculturalists respond to the triple threat, existing economic structures, production philosophies, capital-intensive technologies, public policies, and physical infrastructure associated with simplification processes create a strong predisposition to continue down a simplifying pathway (International Panel of Experts on Sustainable Food Systems, 2016). For those few already benefiting from the status quo, these structures may provide additional opportunities. Yet these lock-ins also constrain adaptation choices and reduce farm-level flexibility for everybody, adding further weight to the forces of simplification.
Diversifying Pathways
As explained in the Introduction, diversifying processes offer farmers an alternative pathway (Wezel et al., 2020). By strategically managing biodiversity and landscapes to increase the magnitude and range of ecosystem services flowing to and from agriculture (Zhang et al., 2007), diversifying processes leverage “nature's technologies” that rely on common-pool resources rather than capital-intensive technologies subject to privatization. Diversifying farming systems requires place-based knowledge of agroecosystems and context-specific innovations derived from collaboration among traditional, experiential, and multi-disciplinary scientific sources of knowledge. Diversifying processes may also promote more inclusively networked systems where alternatives to the vertically integrated supply chain model can flourish (International Panel of Experts on Sustainable Food Systems, 2016), eschewing trends toward concentration, consolidation, and homogenization of farming systems.
Research Questions and Objectives
Building on prior work showing the potential of diversified farming systems to improve social-ecological outcomes of agriculture (Kremen et al., 2012), we explore what happens when farming systems adapt to the triple threat through diversifying pathways as opposed to simplifying pathways. This exploration is motivated by several questions: What properties and qualities of adaptive capacity emerge from diversifying as compared to simplifying processes across different challenges? How might diversifying processes promote sustainability and equity across multiple levels, scales, and functions simultaneously? What challenges and opportunities might manifest through diversifying farming systems? What are key knowledge gaps for understanding how diversifying processes affect adaptive capacity?
Our objective is to address these questions through structured analyses of five cases of challenges in which farming systems struggle to adapt to the triple threat under different types of shocks and stressors (Box 1): living with foodborne pathogens, weathering drought, farming marginal land, dignifying labor, and enhancing land access and tenure (Figure 2). We selected these cases to represent challenges that range across the social-ecological spectrum and based on our expertise and research experience as participants in the Diversified Farming Systems Research Group at the University of California, Berkeley. Each case is presented primarily in the context of US agriculture, though the challenges discussed are common to farming systems worldwide. We analyze each challenge area according to a four-point framework:
1) reviewing the potential for the triple threat to exacerbate each farming challenge;
2) describing simplifying pathway trends for that challenge;
3) comparing those trends to the potential for diversifying pathways to enhance adaptive capacity to the challenge;
4) identifying barriers to diversifying pathways.
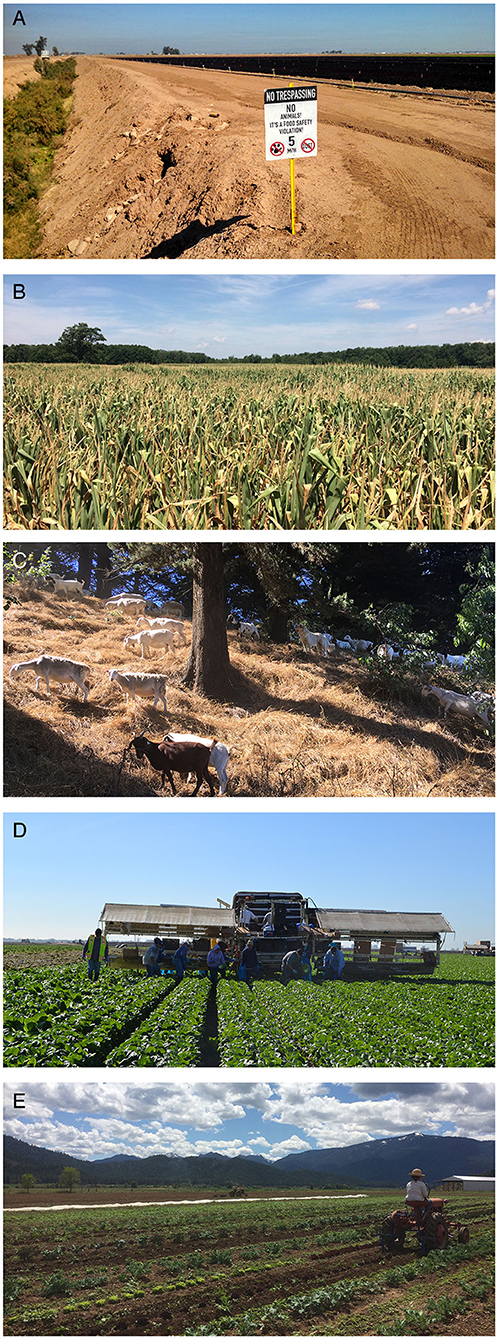
Figure 2. Five cases of challenges in which farming systems must adapt to the triple threat: (A) Pathogens: A no-trespassing sign at the edge of a lettuce field in California, warning, “No animals! It's a food safety violation!” Many crop farms maintain bare-ground buffers around field borders, actively stripped of vegetation, in an attempt to control foodborne pathogen risks. Photo: Patrick Baur; (B) Maize showing symptoms of drought stress grows in a field in southern Ontario, Canada during a drought in summer 2016. Photo: Leah Renwick; (C) Marginal lands: Rotating livestock, like goats, on marginal land can, if managed appropriately to their context, diversify livelihoods and provide ecosystem services like fire fuel load reduction. Photo: Margiana Petersen-Rockney; (D) Labor: Farmworkers who harvest crops like this lettuce are disproportionately impacted by shocks and stressors like heat waves and COVID19, which exacerbate the inequities and risks they already bear. Photo: Patrick Baur; (E) Land access: New-entrant and socially disadvantaged farmers are often more likely to adopt diversifying farming practices, but consistently cite land access and tenure as their greatest barriers to success. Photo: Margiana Petersen-Rockney.
We do not expect most readers to read every case. Rather, we present a diverse palette of cases as self-contained applications of the framework from which readers may selectively choose according to their interests before continuing to the Discussion. For quick reference and ease of comparison, we also direct readers to our two summary tables: Table 1 summarizes our findings on increased stresses from, and potential diversifying adaptations to, the triple threat for each challenge; Table 2 summarizes our findings on simplifying processes and opportunities for, and barriers to, diversification for each challenge.
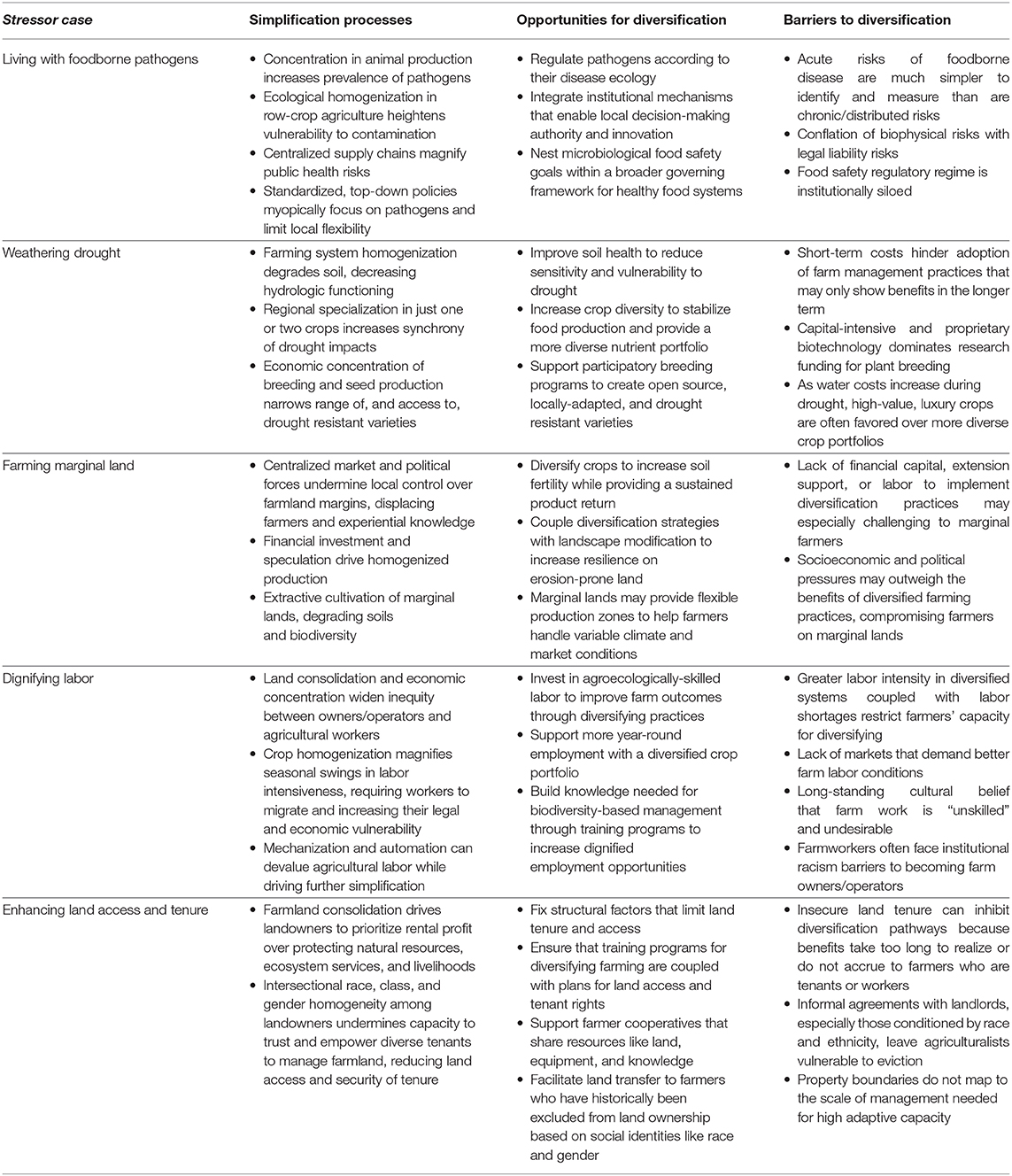
Table 2. Simplification processes, opportunities for and barriers to diversifying processes through which farming systems could strengthen agricultural adaptive capacity.
Case Studies
Living With Foodborne Pathogens
Background: The Triple Threat Increases Microbial Food Safety Risk
While risks from zoonotic diseases have long been associated with animal production systems (Sofos, 2008; Karesh et al., 2012; Rahman et al., 2020), and especially concentrated animal feeding operations (Gilchrist et al., 2007), over the past decade, foodborne human pathogens have newly emerged as a significant challenge for vegetable and fruit agriculture. In the United States, for example, repeated major outbreaks of foodborne illness—most recently several outbreaks of Shiga-toxigenic E. coli (STEC) associated with romaine lettuce (Marshall et al., 2020)—have been linked to in-field contamination of fresh produce crops (Bennett et al., 2018; Li et al., 2018; Turner et al., 2019). Outbreaks can cause significant human morbidity and mortality but also result in second-order shocks to farmers through lost sales, damage to market reputation, and lawsuits (Baur et al., 2017). Moreover, recurring outbreaks induce governments and private industry to introduce precautionary measures (Lytton, 2019), creating a persistent regulatory stressor on farmers to eliminate environmental sources of potential pathogenic risk (Karp et al., 2015a). The triple threat heightens microbial food safety risk (Table 1). Climate change may exacerbate foodborne infectious disease risks through multiple mechanisms, such as altered temperature and moisture patterns that directly influence pathogen growth and survival, as well as shifts in the distribution of disease vectors that may introduce foodborne pathogens to novel human populations (Tirado et al., 2010; Hellberg and Chu, 2015; Lake and Barker, 2018). At the same time, emerging evidence also suggests that, at least in some systems, biodiversity loss can lead to higher likelihood of disease transmission by increasing the relative abundance of species most competent to host and transmit pathogens (Keesing et al., 2010; Ostfeld and Keesing, 2012; Civitello et al., 2015; Mendoza et al., 2019), particularly at local scales (Halliday and Rohr, 2019). Compounding these potential trends, there is rising demand for year-round fresh produce to meet the requirements for nutritional food security. Yet the US food system depends on a very few major sites of production to supply this demand, leading to more intense pressure on the already consolidated, and hence vulnerable (Hendrickson, 2015), regions that specialize in vegetable, fruit, and nut crops. This leads to further centralization of distribution systems and magnification of cross-contamination and outbreak risks (DeLind and Howard, 2008; Stuart and Worosz, 2012). As described below, current simplifying trends in produce agriculture may make these farming systems more vulnerable to foodborne human pathogen stress (Table 2).
Simplifying Pathway Trends: Heightened Vulnerability and Magnified Risks
Many human pathogens that contaminate fruit and vegetable crops originate in the guts of cattle and poultry (Heredia and García, 2018). Concentrating animals in densely-populated locations, such as feedlots, may heighten the prevalence and transmission risk of pathogens such as STEC, Salmonella, and Campylobacter (Valcour, 2002; Frank et al., 2008; Gast et al., 2017; Poulsen et al., 2018). Simplified livestock diets may further accentuate this risk. For example, cattle eating grain-heavy diets have been shown to shed more STEC than do cattle eating diverse, forage-based diets (Callaway et al., 2003, 2009). Likewise, homogenization may increase the vulnerability of plants to pathogenic contamination originating from livestock. Monocrop fields tend to support lower levels of soil and vegetative biodiversity, which impairs ecosystem services, such as microbial competition or physical filtration, that may mitigate the transfer of human pathogens to crops (Karp et al., 2015b, 2016; Sellers et al., 2018; Jones et al., 2019).
The policy response to the risks magnified by concentrated and homogenous production environments has largely followed a simplifying process fixated on increasing technological and regulatory controls (Ansell and Baur, 2018). In the context of a siloed US policy system (Broad Leib and Pollans, 2019; Baur, 2020), such controls drive further ecological and social simplification in agriculture, leading to a self-reinforcing cycle of crisis-and-reform (Baur et al., 2017). On the ecological side, the narrowly precautionary stance embedded within food safety controls reinforces homogenization. In the absence of definitive proof to the contrary, both natural habitat (e.g., riparian vegetation) and managed beneficial vegetation (e.g., hedgerows) are presumed to be risky because they provide habitat for animals that might vector pathogens onto the field (Olimpi et al., 2019). There is thus strong incentive to “purify” farmland (DuPuis, 2015, p. 111–124) by physically separating cultivated fields from biodiverse ecosystems, leading to more biologically homogenous agriculture (Beretti and Stuart, 2008; Stuart, 2009; Baur et al., 2016; Olimpi et al., 2019).
On the socioeconomic side, this pernicious cycle also reinforces concentration and consolidation through several mechanisms. First, food safety precautions require money, time, and labor, but farmers rarely receive a corresponding price premium to offset this cost. In addition, the relative cost of compliance is higher for smaller scale as compared to larger-scale farm operations (Astill et al., 2018; Bovay et al., 2018), driving further farm consolidation due to imposed competitive disadvantage (DuPuis, 2015; Karp et al., 2015a; Olimpi et al., 2019). Second, food safety's precautionary stance disincentivizes rotation, polyculture, or integrated livestock practices, because complex agricultural management techniques multiply the burden on farmers to prove that such techniques are safe (Olimpi et al., 2019). Third, food safety standards are generally set by experts external to the target agricultural system with minimal design input by the farmers who must then implement those standards (Baur et al., 2017; Verbruggen, 2017). This top-down decision-making structure concentrates power and adopts a homogenous risk management system that rewards simplified farming systems and limits local flexibility and adaptation. Fourth, myopic focus on producing crops free from human pathogens obscures interrelationships among multiple agricultural functions and objectives (McMahon, 2013; Broad Leib and Pollans, 2019), undermining the capacity of farms to adapt to the novel food safety challenges posed by the triple threat. In these ways, the simplifying process of adaptation to pathogenic risks—based on a model of control designed for factories rather than agroecosystems (Karp et al., 2015a)—forms a pernicious feedback loop that iteratively renders farming systems more vulnerable to the challenges posed by foodborne pathogens (Table 2).
Diversifying Pathway Opportunities and Barriers to Increasing Adaptive Capacity: Harnessing Ecosystem Services and Distributing Authority to Mitigate Risks
This case reveals three areas of opportunity to enhance adaptive capacity toward foodborne human pathogens by diversifying farming systems that grow fresh fruits and vegetables (Table 2), with the goal of enabling specific adaptations to the triple threat such as those posited in Table 1. First, if farmers and regulators recognize the role that high biological diversity—at the farm and landscape level—might play in mitigating foodborne pathogen risks, then research effort could be directed to identify and validate novel management options for cultivating pathogen-suppressing ecosystem services (Karp et al., 2015b; Olimpi et al., 2019). For example, emerging evidence suggests that managing healthy soils for biodiverse microbial and insect communities with practices like maintaining soil cover and high above-ground diversity may effectively mitigate pathogenic strains of E. coli in feces (Jones et al., 2019). Second, integrating institutional mechanisms that allow for nested, multi-level standard-setting could help equalize decision-making authority between farmers and external experts and permit greater flexibility and innovation, especially for producers with less access to scientific expertise (Olimpi et al., 2019). An example would be for national regulatory agencies to delegate standard-setting and monitoring authority to smallholder cooperatives, which would be responsible for governing day-to-day food safety risks among their membership. Third, at the policy level, an opportunity exists to shift toward a perspective that accepts that pathogens are endemic to their host systems, and thus cannot simply be eliminated from the farm environment. Such an adjustment of perspective would allow diversification of food safety objectives beyond simply controlling the points of contamination where pathogen meets edible crop to also minimize the genesis of dangerous pathogens (e.g., in high-density, confined animal feeding systems) and limit their risk-factor multipliers (e.g., through centralized processing facilities) (Stuart and Worosz, 2012; Broad Leib and Pollans, 2019). To date, these opportunities remain largely unexplored (see Appendix 1).
The primary barrier to diversifying opportunities for ecological management of pathogens at the farm scale originates with the simplifying assumption, tacitly held by powerful market and regulatory actors, that the presence of natural ecosystems near fields automatically increases food safety risk (Olimpi et al., 2019). Efforts to ease this barrier through further agroecological research into pathogen disease ecologies are hindered by the mingling of perceptions about biophysical and legal liability risks in informing food safety decisions (Baur et al., 2017). In turn, the fragmentation of food safety governance into uncoordinated institutional silos, in the US at least (GAO, 2017), complicates any effort to overcome the preceding barriers. For example, in the US, microbial food safety for animal products is regulated separately from fruits and vegetables, while both regimes operate independently of regulatory agencies charged with overseeing other safety concerns such as pesticide risks or occupational hazards to farmworkers (Broad Leib and Pollans, 2019). Due to fragmentation and siloing, there is a general failure to acknowledge the dampening effects that microbial food safety efforts impose on attempts to manage agriculture adaptively for other goals, including those that affect public health (Table 2).
In summary, the simplifying pathway seeks standardized methods to control the spread of foodborne pathogens without addressing the growing vulnerabilities to pathogenic risks created through operational concentration, agroecological homogenization, and supply chain centralization. A diversifying pathway, in contrast, would seek to (a) reduce those vulnerabilities by creating strategic heterogeneity in operations, agroecological systems, and supply chains and (b) promote local resilience through ecosystem services that regulate pathogen disease ecologies and by increasing local decision-making authority to innovate place-specific mitigation strategies.
Weathering Drought
Background: Droughts Will Increase in Intensity and Frequency
In the coming decades, climate change will further increase the intensity and frequency of droughts in agricultural landscapes, especially in temperate regions (Hatfield et al., 2011; Trenberth et al., 2014), impacting farmers' immediate ability to grow crops, raise livestock, and sustain their livelihoods. These impacts will have rippling effects throughout the food system. As an example, in 2012 a widespread and intense drought across two-thirds of the continental United States reduced corn yields by 25%, which was accompanied by a 53% global price spike (Boyer et al., 2013). Dramatic declines in crop biodiversity further worsen the impacts of drought by reducing variation in how crops respond, i.e., response diversity (Elmqvist et al., 2003; Laliberté et al., 2010).
Drought acts not only as an acute shock but can also become a long-term stressor for agriculture depending on the drought's duration. Both irrigated and rainfed agroecosystems are affected when water availability becomes limited by scarcity or policy. For instance, the 2011–2016 drought in California, where most crops are irrigated, caused estimated losses of 21,000 jobs and $2.7 billion in agricultural output in 2015 alone (Howitt et al., 2015). Economic losses would have been far greater if farmers had not switched to groundwater for irrigation, though this in turn led to substantial groundwater overdraft and land subsidence (Faunt et al., 2016). Climate change-driven reductions in the snowpack that recharges groundwater exacerbate these overdraws (Pathak et al., 2018). Although new policies in California that regulate future groundwater withdrawal may reduce overdraft (Harter, 2015), this example highlights how the biophysical impacts of climate change can interact with policy change to create or exacerbate complex, multi-dimensional stressors for farmers (Table 1).
Simplifying Pathway Trends: Simplification Has Increased Vulnerability to Drought
The vulnerability to drought that results from simplifying processes (Table 2) is exemplified in the Corn Belt of the central United States, where intensive rainfed commodity corn and soybean production suffered at the center of the 2012 drought. Over the course of the last century, significant homogenization of farming systems occurred in response to federal and state policies and increasing downward economic pressures from rampant concentration of input suppliers and grain processors (Philpott, 2020), resulting in farms that now almost exclusively grow corn and soybeans rather than the small grains, hay and integrated animal pasture they once also produced (Brown and Schulte, 2011; Liebman and Schulte, 2015). Reductions in crop diversity, disintegration of crop and livestock production, and other concurrent changes in management have led to widespread soil degradation (Karlen et al., 1994; O'Brien et al., 2020), including declining soil organic matter and topsoil erosion, which in turn undermines hydrologic functioning critical for rainfed systems. At wider scales, specialization in just these two crops coupled with increasing climatic sensitivity of corn production increase regional sensitivity to drought (Lobell et al., 2014, 2020; Ortiz-Bobea et al., 2018). While the particularities of this simplification process are unique to the U.S. Corn Belt, similarly homogenized farming systems across the world increase the potential for globally synchronized climatic shocks that threaten food production (Tigchelaar et al., 2018). Genetic engineering of drought- and heat-resistant crop genotypes—one of the most commonly recommended strategies for addressing the projected increase in drought severity (Hu and Xiong, 2014; Ortiz-Bobea and Tack, 2018; Ortiz-Bobea et al., 2018; Tigchelaar et al., 2018)—will further entrench the trajectory of simplification by increasing reliance on proprietary and capital-intensive biotechnology. Despite substantial investments, genotypes engineered for drought resistance show only modest improvement, if any, over decentralized, traditional breeding approaches for drought resistance (Gilbert, 2014). Widespread use of these genotypes may in turn impact ongoing declines in crop genetic diversity if only a few engineered crop varieties displace a multitude of other varieties.
Other capital-intensive responses to water limitations exist, but may come with unexpected tradeoffs that also reinforce simplification pathways (Table 2). In irrigated cropping systems, field-level investments in purchased inputs like drip irrigation and water sensors can potentially reduce exposure to drought by increasing water use efficiency. In the absence of policy and institutional support for resource conservation, however, such investments can also lead to tradeoffs for soil health, such as decreased soil aggregation (Schmidt et al., 2018) and higher water consumption on a regional basis (Grafton et al., 2018). The latter phenomenon is an example of Jevon's paradox, which can occur when increased irrigation efficiency at the field level incentivizes farmers to switch to higher-value, but more water-intensive, crops, thereby causing an overall increase in water use at a regional level. Relatively expensive capital upgrades, like water sensor networks, often accompany simplifying strategies, as they are better suited to large-scale production of uniform crops. Drought itself may reduce the diversity of crops grown due to water shortage and commodity prices. For example, high value, luxury crops like wine grapes may be favored during drought over food staples with lower value, like rice (Bradsher, 2008). These shifts in production have the potential not only to affect global food supply and potentially exacerbate food insecurity, but also to push farmers along a simplification pathway.
Diversifying Pathway Opportunities and Barriers to Increasing Adaptive Capacity: Improve Soil and Increase Crop Diversity at Multiple Scales
Diversifying pathways can reduce exposure and vulnerability to drought while also providing other benefits (Table 2) and adaptations to the triple threat (Table 1). Cropping system diversification is one process that reduces impacts from drought, likely mediated through soil improvements (Lotter et al., 2003; Gaudin et al., 2015). For example, long-term evidence across multiple sites in the U.S. and Canadian Corn Belt showed that rotational diversification reduced corn yield losses by 14 to 90% in various drought years (Bowles et al., 2020). In general, improving soil's capacity to capture, store, and supply water to crops and forage increases resistance to droughts, especially in rainfed systems. Field-scale diversification practices, like cover cropping, crop rotation, application of organic amendments, and reduced soil disturbance, often increase soil organic matter (Marriott and Wander, 2006; McDaniel et al., 2014) and the abundance and diversity of soil organisms (Bender et al., 2016; Bowles et al., 2018) along with soil water holding capacity, infiltration, and porosity (Basche and DeLonge, 2017, 2019). Empirical evidence supporting our robust theoretical understanding of how these improvements increase crop performance under water limitation is only just emerging (Gaudin et al., 2015; Gil et al., 2017; Solorio et al., 2017; Bowles et al., 2020).
Increasing crop diversity at multiple scales, from intercropping to whole farms to regional scales, can also reduce drought risk in ways other than changes to soil (Lin, 2011; Renwick et al., 2020). Increasing the diversity of crops grown at the farm-scale to include ones that differ in their water use, drought tolerance, or phenology helps reduce risks to farm-level yield and income through a “portfolio effect” (Helmers et al., 2001, Isbell et al., 2017). Reflecting this principle at broader scales, recent work shows that greater crop diversity provides a more diverse set of human nutrients and stabilizes food production at the national scale (Renard and Tilman, 2019). Breeding new crop varieties with greater drought resistance can also be a diversifying strategy, if the result of breeding programs expands rather than contracts genetic diversity. Participatory, decentralized breeding programs that develop open source, locally adapted drought-resistant varieties are a promising development (Gilbert, 2014), though significant legal, cultural, and social network transformations are needed to sustain a seed commons in a global seed market dominated by multinational corporations (Montenegro de Wit, 2017a).
Farmers face several barriers to diversification as a strategy for adapting to drought (Table 2). Short-term costs hinder adoption of farm management practices that may only show benefits in the longer term (DeVincentis et al., 2020). For example, while growing cover crops clearly provides several long-term benefits for agroecosystems, their establishment entails both short-term fixed costs like seeds, field operations and labor, and potential risks like disruption to planting or harvest contract schedules (Jackson et al., 2004). Policy, market, and research and development structures currently incentivize and retrench low cropping system diversity while failing to support diversification strategies (Mortensen and Smith, 2020, this special issue). Programs that provide incentives for farmers to adopt diversification practices, like California's Healthy Soils Program, can reduce barriers related to fixed costs, but may not be enough to address opportunity costs of high-value crop production. Another barrier is that diversification practices are knowledge-intensive (Carlisle et al., 2019a) and cannot be applied in a “plug and play” manner as in simplifying technological approaches like applying non-renewable fertilizers. For instance, in especially arid climates, cover crops can compete with the cash crop for water and must be carefully managed to avoid a net loss of water (Bodner et al., 2007). Even when examples of successful diversifying management practices exist in such regions, perceptions of the challenges by farmers and technical assistance providers can be a barrier to adoption.
In summary, simplifying pathways are primarily comprised of capital-intensive, large-scale technological fixes that help well-resourced farms survive acute drought crises without taking steps to reverse the crop homogenization and seed concentration trends that produce chronic vulnerability to drought. A diversifying pathway, in contrast, would seek regional resilience by promoting local-scale, and more accessible, solutions through investment in soil health, crop diversity, and participatory breeding programs.
Farming Marginal Land
Background: Shifting Boundaries of Land on the Margins
Farmers across the globe—especially those with limited access to markets, financial resources, infrastructure, and natural resources like water—have always sought innovative ways to extend production onto the margins and boundaries of arable land (Kumar et al., 2015; Calderón et al., 2018). While the definition of marginal land is highly contingent and reflective of shifting, context-specific, and interconnected biophysical and political-economic processes (CGIAR Technical Advisory Committee, 2000), in common usage these lands are often characterized by low or compromised soil quality, suboptimal precipitation or temperature, rugged or steep topography, and low or inter-annually irregular productivity (Kang et al., 2013; Peter et al., 2017). As climate change shifts the boundaries of arability, more land will be pushed toward this “marginal” category (Reed and Stringer, 2016). Simultaneously, increasing farmland consolidation limits land access and pushes smallholder farmers into regions of relatively poor fertility (Naranjo, 2012). Biodiversity loss has the potential to decrease both in situ ecosystem service provisioning and ecological response diversity, exacerbating the economic and ecological marginalization of these lands and those who rely on them (Table 1).
Simplifying Pathway Trends: Extracting Value From Marginalized Land and Those Who Farm It
Marginalizing certain lands and conflating marginal lands with the people who use them (CGIAR Technical Advisory Committee, 2000), have served to simplify agricultural landscapes and communities by promoting the replacement of complex local knowledge-based agricultural systems with homogenized commodity crop production (McNeely and Schroth, 2006; McMichael, 2012; Naranjo, 2012). The growing trend of farmland financialization in the United States offers an example of how so-called marginal lands continue to be leveraged to justify the simplification of farming systems (Table 2). Financial institutions often seek marginal farmland, for example land with low soil quality and little annual rainfall, for speculative investment. And this often removes that land from the hands of local farmers (Fairbairn et al., 2021). Studies from around the world suggest that marginal lands can be used for bioenergy crops (Helliwell, 2018; Koide et al., 2018), livestock production (Hall, 2018), or removed from cultivation for restoration and conservation (Merckx and Pereira, 2015). These uses can simplify or diversify farming systems, depending on how they are implemented and by whom. Many existing studies disregard the ways in which top-down approaches to transition marginal lands to more capitally productive uses can take marginal lands out of local community control or smallholder cultivation, and in the process displace resource-poor or subsistence farmers (Wells et al., 2018), exacerbating food insecurity and potentially forcing intensive cultivation into sensitive ecological areas.
Transitioning marginal lands to intensive cultivation can have devastating ecological and social consequences. Capital-intensive or technocratic approaches, which function to simplify production, can at least temporarily increase the productive potential of marginal lands, but in doing so often exacerbate underlying stressors and vulnerabilities. For example, the west side of the San Joaquin Valley in California is agriculturally constrained by salinization, selenium contamination, low groundwater availability, and impermeable clays (Ohlendorf, 1989; Garone, 1998, 2011). Despite these challenges, this landscape has been developed into one of the highest-output agricultural regions in the United States, mainly due to massive irrigation projects. Such projects remain highly controversial and have led to environmental and social harms including further-depleted aquifers, land subsidence, greater salinization, rapid die-off of flora and fauna at the Kesterson Reservoir, and increased concentration of simplified agricultural operations (Ohlendorf, 1989; Garone, 1998, 2011).
Diversifying Pathway Opportunities and Barriers to Increasing Adaptive Capacity: Tools to Mitigate Against Social and Ecological Stressors
Diversifying farming practices may allow farmers to farm productively on marginal lands by mitigating ecological stressors, including acute disturbances such as weather extremes, while also helping to restore degraded soil or mitigate inherent soil limitations (Table 2; Altieri, 2002). Crop diversification is a foundational agroecological technique that has helped farmers cope with the stressors they face on marginal lands and is key for adapting to the triple threat (Table 1). Selecting for drought-tolerant cultivars, for example, has increased climate resilience in the water-limited southwestern United States (Elias et al., 2018). Crop rotation or intercropping may also improve soil fertility while providing a low but sustained return (Ewel and Hiremath, 1998; Mader, 2002). On steeper, erosion-prone lands, coupling diversification practices with landscape modification like terracing can increase resilience (e.g., Bocco and Napoletano, 2017). For example, Nicaraguan farms on steep slopes that employed agroforestry and cover cropping for at least 3–5 years were more resilient to Hurricane Mitch's impacts (Holt-Giménez, 2002). Introducing perennial crops and livestock onto marginal lands can also improve agroecosystem functioning, ameliorate extreme weather impacts, improve soil fertility (Speakman, 2018), sustain economic returns (Peter et al., 2017; Rois-Díaz et al., 2018), and conserve cultural landscapes (Vries et al., 2015; Zanten et al., 2016).
For marginal lands, diversification practices are especially important to mitigate against social stressors, such as food insecurity. Several studies on marginal lands farmed by resource-poor farmers have found that diversification of agricultural production is co-linked to food security and diet diversity at the household level (Kumar et al., 2015; Calderón et al., 2018), although notable exceptions exist (Sibhatu et al., 2015). For example, Oyarzun et al. (2013) found a weak but significantly positive correlation between the number of species of crops grown by smallholders in the Ecuadorian highlands and dietary diversity within families. The data suggests this relationship results from the positive correlation between on-farm agrobiodiversity and consumption of on-farm products. Families with less agrobiodiversity consumed more off-farm foods and had lower overall diet diversity.
In some cases, marginal lands could provide opportunities for diversifying farming systems, especially in regions with low land values (Table 2). For example, Gabriel et al. (2009) found that lower quality land, often with lower land values, is likely to predispose farmers to convert to organic farming, which then encourages other nearby farmers to convert to organic. In other cases, without financial capital, knowledge, extension support, or accessible labor, diversifying marginal lands poses challenges to farmers, such as implementation costs of diversification practices (Altieri, 1999; Iles and Marsh, 2012). If farms are marginal to the dominant political, economic, and market system due to their small size or production methods, it may not be possible to maintain farmer livelihoods even with diversified agroecological techniques (Naranjo, 2012). For example, there may simply not be a market for crops grown at relatively low volumes, posing a severe economic barrier for farmers seeking to diversify on marginal lands (Sharma, 2011; Naranjo, 2012). Thus, market limitations (e.g., demands and infrastructural limitations) may further limit the adoption of diversifying farming practices on marginal lands (Sharma, 2011; Naranjo, 2012).
In summary, the simplifying pathway seeks to increase productivity on marginal lands by enrolling them in commodity crop markets that promise cash flow but may over-exploit these fragile ecosystems and undermine local food sovereignty. A diversifying pathway, in contrast, might seek to empower local communities to utilize marginal lands as flexible production zones from which a variety of farm products can be derived to complement, rather than compete with, the production portfolio of neighboring farmland.
Dignifying Labor
Background: A Double Crisis of Agricultural Labor
Agriculture in the United States faces a labor crisis. Agricultural workers are poorly paid, with few legal protections, while also facing challenging working conditions, including exposure to toxic chemicals, dangerous physical demands, extreme heat, and social hazards that threaten their health and well-being (Shreck et al., 2006; Holmes, 2013; Castillo et al., 2021). Simultaneously, due to demographic trends and migration policies at the federal level, employers face shortages and instability of labor supply (Martin et al., 2016). This situation affects critical tasks including planting, cultivating, and harvesting, which threatens food production and farm profitability and undermines farmers' ability to both adapt to climate change and use diversified farming practices. Finding ways to ensure stable, healthy, and dignified farm livelihoods that sustain sufficient food production will become more difficult as the triple threat intensifies.
Additional stressors from climate change exacerbate the inequities and risks that farmworkers already bear (Table 1). Extreme events such as heat waves can cause significant health consequences and socio-economic hardship for workers—while also potentially disrupting farm operations (Castillo et al., 2021). When workers continue working in extreme heat, they can suffer both short- and long-term negative health consequences such as dizziness, heatstroke, and chronic kidney disease (Fleischer et al., 2013; Stoecklin-Marois et al., 2013). A recent occupational safety rule in California requires farmworkers to take paid rest for at least 10 min every 2 h when temperatures reach 95°F (observations by co-author Castillo). Farm managers, however, often ignore this policy, resulting in income losses to a population already among the lowest paid in the United States (ibid). Drought can also adversely affect agricultural workers, for example, by causing the loss of work or increased travel time to alternative work sites if cropland is fallowed (ibid). More broadly, extreme weather events reduce worker productivity and availability, with potential negative impacts on rural economies and food production (Kjellstrom et al., 2009; Hsiang, 2010), especially when coupled with direct impacts of extreme weather on crop productivity. Farmers and farming systems must adapt to these labor challenges, either through simplifying pathways that replace labor with capital-intensive inputs (notably agricultural chemicals and new technologies whose use encourages monoculture farming methods), or through diversifying pathways that improve farm working conditions around multiple crops and farm biodiversity, by emphasizing knowledge-intensive management, offering employment stability, and valuing farmworker skills.
Simplifying Pathway Trends: Labor Shortages Are a Product of Agricultural Simplification
Consolidation of farm and farmland ownership not only hinders new-entry access to agriculture (see section Enhancing Land Access and Tenure), but also further cements the social divide between owners/operators and agricultural workers. This divide results in the devaluation of farm work, leading to a negative feedback loop in which only the most economically vulnerable workers seek employment in agriculture, which in turn leads to further disinvestment in improving farm labor wages and working conditions. A simplifying approach to this challenge perceives only a labor shortage, to be remedied by either finding new populations of workers to exploit (e.g., through migrant farmworker programs) or obviating the need for farm workers in the first place (e.g., through mechanization).
The United States, like most market economies, has opened its borders to migrant agricultural laborers (Pfeffer, 1983; Weiler et al., 2016). Historically, US immigration policies have resulted in a flow of low-wage migrant laborers from successive geographic regions, each arriving with few legal rights or protections, and who are subject to high rates of wage theft, sexual harassment and assault, and other forms of violence based on their race, gender, immigration status, and economic positionality (Walker, 2004). With a steady supply of migrant, cheap, and right-less labor, farm owners have had little incentive to internalize the production risks that these laborers have borne (Pfeffer, 1983). If, for example, drought devastates a crop one year, farmers can readily lay off migratory workers, whereas they must absorb the costs of repaying loans for an expensive harvester that is depreciating and losing value. However, in recent years, the agricultural workforce has aged (Hertz, 2019), partly due to slowed immigration from Mexico and partly because immigrant farmworkers often encourage their children to enter other careers (Martin et al., 2017). The Trump Administration targeted undocumented farmworkers for deportation, creating a fearful atmosphere that further deterred immigration (Goldbaum, 2019). An aging labor force is also more susceptible to injuries and health problems (Varney, 2017).
In the face of harsh working conditions and poor pay, migrant farm workers in the post-World War II era have tended to exit agriculture for other sectors that offer greater economic opportunity as soon as they are able. The importation of immigrant agricultural labor is therefore always in a race with the steady outflow of farm workers. US farmers have thus sought a more lasting solution: replacing human labor with machine labor. Beginning in the 1920s, mechanization for greater scales of efficiency has progressively made substantial inroads into commodity crops like wheat, cotton, tomatoes, and corn, significantly reducing demand for labor in these sectors (Schmitz and Moss, 2015). Since the 1950s, other crop sectors, such as row crops and nut orchards, have been mechanized to varying degrees. This has reinforced the simplifying tendency of farms to specialize in monoculture fields or orchards and grow larger in size (Fitzgerald, 2008). Yet many capital-intensive farms remain only partially mechanized and still rely on numerous seasonal human workers to carry out critical farming activities. For example, harvesting strawberries or romaine lettuce is not mechanized (Price, 2019). Indeed, within California, acreage of labor-intensive crops has increased due to growing market demand for these products over the past 30 years, increasing agricultural labor demand (Martin et al., 2016). Crop homogenization means that demand for labor is very seasonal: particularly at harvest, workers must constantly move between regions where specific crops are picked for a few weeks at a time. This pressure to migrate internally further increases their legal and economic vulnerability.
Facing a labor shortage, employers may be forced to spend additional time finding farmworkers, offer better pay and working conditions, or reduce production by leaving land fallow or crops unharvested (Kitroeff and Mohan, 2017; Morris, 2017). Well-capitalized farming interests may respond by re-committing to keep the labor force available, cheap, mobile, and disposable with few rights, resources, or recognition (Mitchell, 1996). If they choose a simplifying pathway, employers in the US are more likely to seek increased mechanization and automation to replace workers in labor-intensive tasks. A current example is the heavy investment in developing robotic strawberry harvesters to replace human pickers (Seabrook, 2019). Historically, many crops have proven difficult to mechanize, but with growing labor scarcity, technology developers are redoubling efforts to combine cameras, GPS, 3-dimensional mapping, and other technologies to substitute for worker dexterity and intelligence.
The overall effect of the automation trend on diversification is unclear. Adopting automated tools that replace human workers can decouple diversified farms from an unstable labor supply, potentially leading to increased farm stability. Small scale automation could improve working conditions and health outcomes, by eliminating dangerous tasks and paying fewer, but more ecologically skilled, workers better (Price, 2019). But widespread reliance on capital-intensive automation—which incurs high upfront financial costs and may take many years to pay off—may reinforce simplification processes and lock farms into inflexible production regimes that cannot nimbly adapt to novel stressors. Robots and other expensive automation technologies require further simplifying farming systems into even larger fields with uniform crops to operate efficiently (Seabrook, 2019). Ultimately, then, automation to replace labor could diminish farm economic stability. Additionally, adoption of automation can make it harder for smaller scale or more diversified farms to compete economically by driving down production costs even further.
Diversifying Pathway Opportunities and Barriers to Increasing Adaptive Capacity
The extent to which diversifying farms in the US can build adaptive capacity through their labor to maintain production and adjust to environmental changes is largely unexplored empirically. Farmers' ability to adopt labor-friendly measures to overcome current labor shortages and reduce worker exposure to climate-related extremes is also uncertain. In principle, increasing crop diversity and focusing on developing agroecological management skills rather than capital-intensive inputs such as automation could potentially increase opportunities for both workers and new-entry farmers (Table 2; Carlisle et al., 2019a,b). Working conditions may improve incrementally in more diversified systems due to reductions in chemical exposure, greater mental stimulation leading to increased job satisfaction, and more possibilities for year-round employment from diverse cropping and livestock systems that spread peak labor needs more evenly across seasons (Shreck et al., 2006; Bacon et al., 2012; Timmermann and Félix, 2015). This could make agriculture more attractive to younger workers, thus expanding the labor pool. It may also allow diversifying farmers to stabilize their production in conditions where simplifying farmers struggle to find enough labor. Critically, having workers who are experienced in observing and managing farm conditions can directly strengthen a farm's adaptive capacity (Hammond et al., 2013).
However, simply adopting diversification practices—in the absence of changes to the overall socio-economic environment—likely has limited potential to improve farm labor conditions at either the systemic or individual scales. Research on labor in organic agriculture in the United States has demonstrated that a greater number of jobs in the organic sector does not necessarily translate into more socially equitable jobs (Shreck et al., 2006). Organic farmworkers may still face conditions of poor pay, food insecurity, and lack of access to housing and health care, especially where farmers feel they must compete with conventional producers on price to gain entry to key markets (Guthman, 2004). Recent European research further supports this observation. By comparing working conditions for farm owners and workers across agroecological, organic, and conventional farms in Belgium, Dumont and Baret (2017) concluded that several practices—including increased crop diversity of both winter and summer crops and opportunities for laborers to participate in a variety of tasks from production to marketing— were necessary, but not sufficient, to support better working conditions for both farm employers and workers.
How pursuing diversifying pathways affects farmer responses to extreme weather events is also unclear. The impact of heat waves on farmworkers may be mitigated through measures such as providing accessible water stations, longer and more frequent rests, shaded rest areas, and adjusting harvesting schedules to avoid the highest temperatures (Stoecklin-Marois et al., 2013). Adopting these measures requires a labor-friendly farm operator. However, it is not clear whether there is a correlation between agroecological diversification and equalization of the historical power differential between farm employers and farmworkers. Diversifying farms may still not recognize workers as knowledgeable agricultural experts or share decision-making power between managers and workers (Dumont and Baret, 2017; International Panel of Experts on Sustainable Food Systems, 2018). As a result, workers can remain at the bottom of a management hierarchy. This relationship remains under-studied in the context of farm diversification. Moreover, without structural changes in markets, policies, and institutions that prevent farm owners and managers from exploiting their workers, efforts to ecologically diversify farms could actually impose further harmful burdens on farmworkers: for example, diversifying practices could require more physically intensive labor without empowering workers or improving work conditions.
While jobs in simplified farming systems may be undesirable because of the poor conditions, low pay, physical danger, and even stigma, jobs in more diverse and complex farming systems may be more socially desirable, requiring a high degree of recognized skill and knowledge (Carlisle et al., 2019a,b). If wages properly reflect the greater human capital required to diversify, then employers would need to pay these ecologically skilled workers a higher wage, which could be partly offset by reduced costs of external farm inputs and greater market value of products (Carlisle et al., 2019a). However, many farms that adopt diversification practices are smaller in scale with fewer financial resources than their larger market competitors, leaving these farm operators struggling to pay both themselves and their workers and unable to provide higher wages (Harrison and Getz, 2015; Dumont and Baret, 2017). To internalize social and ecological externalities in a diversifying system will require markets and buyers that demand better labor conditions and reward early adopters with higher prices for their products.
In summary, the simplifying pathway responds to labor problems by seeking either more exploitable workers or to replace farmworkers with machines—both processes further the devaluation of labor produced by widening inequity between farm owners/operators and agricultural workers. A diversifying pathway, in contrast, could seek to restore the value and dignity of farm work through recognition of, and investment in, the agroecological skills necessary for ecologically-based farm management.
Enhancing Land Access and Tenure
Background: Access to Land Shapes Adaptation Potential
Our final case focuses on how farmland tenure and access, primarily in the United States, shape pathways for adaptation. The economic factors driving land use are increasingly divorced from the day-to-day operational decisions of working farms. Each year, more land is taken out of food production for other uses—e.g., energy production, residential development, or conservation reserves—while farmland that remains in agriculture is increasingly purchased by non-farmers as a capital investment (Fairbairn et al., 2021). These trends make it harder for farmers to own and access farmland, reducing their control over its dispensation. Decreased land access, tenure, and control by farmers is expected to exacerbate food insecurity and disincentivize sustainable farm practices (Trauger, 2014; Borras et al., 2015), although targeted policies may mitigate this tenure effect in some cases (Leonhardt et al., 2021). Furthermore, land access and tenure are consistently cited as the greatest barriers to the establishment of new-entrant farmers who would otherwise bring the skills, aspirations, and labor necessary for agricultural diversification (Beckett and Galt, 2014; Carlisle et al., 2019b). Heightened climate change risks coupled with biodiversity loss of ecosystem service providers will exacerbate barriers to entry for new-entrant farmers (Carlisle et al., 2019b).
Simplifying Pathway Trends: Capital-Intensive Solutions Align With Simplified Land Tenure
Capital-intensive solutions to the triple threat, like climate-smart agriculture or sustainable intensification, tend to favor simplified land tenure regimes (Table 1). These strategies for adaptation align with centralized decision making and consolidated land ownership. Certain high-value crop regimes dictate the “highest and best use” of farmland, further inhibiting adoption of conservation-based practices, especially those that may rely on high crop diversity (Guthman, 2004, 2019). Regional trends like natural gas development in Pennsylvania (Malin and De Master, 2016), biofuel investments in the US Midwest (White and Selfa, 2013), or corporate CAFO development in Illinois' corn belt (Ashwood et al., 2014) can put pressure on farmers—whether they lease or own the land—to maximize their production value (i.e., plant only the highest-value crops) to pay debts or to hold on to the land when it could be sold for more lucrative uses or to investment firms and hedge funds. Becoming locked-in to narrow goals of yield and profit by the ever-rising value of land itself, farmers face significant structural barriers to diversifying, limiting their potential to enhance adaptive capacity (Table 2).
Land access is shaped by structural factors that influence land tenure and complicate farmers' ability to diversify. Recent research has examined the role of race, ethnicity, and gender-based factors in determining inequities in farmland access and tenure (Calo and De Master, 2016; Minkoff-Zern, 2019). Exclusionary policies shape land ownership trends in the US, such that most farmland is owned by white males (Horst and Marion, 2019), a trend that grows stronger with increasing farm size and wealth (USDA Census, 2017).
As land tenure regimes continue to simplify—particularly as farmland is accumulated by distant owners interested in land as an asset (Fairbairn, 2020) and those who work on the land are disenfranchised tenant farmers—we are likely to see greater homogenization in management regimes. In this context, the characteristics of actors (class, ethnic background, motivation) who have capacity to make decisions becomes less diverse. More importantly, the ability of tenant farmers to influence changes to the landscape diminishes, as they follow prescribed production pathways that allow them to meet the conditions of their lease. Under such tenure regimes, the capacity for field and landscape level diversification shrinks.
Access to land is mediated by social mechanisms beyond property regimes that determine the ways in which agriculturalists can actually derive benefit from land and to what extent (Ribot and Peluso, 2003). This suggests that having title to land does not guarantee that the holder will be able to gain full benefit from the lands' total capacity. Instead, potential land use options are constrained by structural factors, such as food safety regulations (Olimpi et al., 2019), water rights (Calo and De Master, 2016), or neighboring land uses (e.g., pesticide drift). Building agroecosystems through diversification often takes years, at which point those benefits may be realized by the landlord if the farmer was renting, or the next owner. In other words, land tenure does not necessarily determine who might benefit from even farm-scale diversification. Despite the fact that simply identifying who owns land in itself is not enough to understand whether a farmer will seek to diversify, the majority of related research to date focuses on the relationship between land ownership and farm practices.
Diversifying Pathway Trends: Support New Entrant Farmers and Alternative Land Access Structures
When considering diversifying farming systems, it is important to consider farmers themselves as an axis of diversity who bring, as social network theorists posit, innovation and new ideas introduced at the margins of networks (Granovetter, 2005). The ability of farmers to build adaptive capacity through diversification, therefore, relies not only on access to land, but also on the ability to build and use their knowledge of their land (Table 2).
Research indicates that new-entrant and socially disadvantaged farmers (e.g., women, immigrants, racial/ethnic minorities, and young farmers) may be more willing and likely to adopt diversifying farming practices (Deaton et al., 2018). Many immigrant farmers in the US have agroecological expertise and experience using diversifying farming practices that could improve adaptive practices to a wide range of conditions (Shava et al., 2010; Minkoff-Zern, 2019). Carney (2020) points to the cultural knowledge and social memories of Afrodescendant smallholder farming systems that have “long prioritized agrobiodiversity and agroecological practices.” Latinx farmers not only provide the vast majority of US agricultural labor today, but also bring expertise and diversification values (Minkoff-Zern, 2018). It is therefore crucial—for both equity and sustainability—to expand land access for new-entrant and socially disadvantaged farmers.
While most funding to support new-entrant and socially disadvantaged farmers in the United States has focused on farmer education and training programs, these have little impact on the adoption of diversified farming practices if farmers do not have the agency to implement them due to limited land access or insecure tenure (Calo, 2018). Training programs that, alternatively, decentralize expertise and enhance farmer networks—such as farmer-to-farmer learning networks—can help overcome land access issues by sharing knowledge and building solidarity and collaborative relationships (Holt-Giménez, 2006; Bacon et al., 2012; Carlisle, 2016). Additionally, governments can incentivize land transfer programs. Agricultural conservation easements, for example, are an important tool to lower the price of farmland, making it more affordable to new-entrant farmers, and farmers enrolled in the federal Conservation Reserve Program can receive an additional 2 years of government payments if they rent or sell that land to new-entrant farmers (Carlisle et al., 2019b). Programs like these should be expanded, protections from corporate capture put in place, and new entry and socially disadvantaged farmers prioritized (Calo and Petersen-Rockney, 2018).
As fewer farmers own the land that they farm, there are some promising signs that non-operator landlords—from private conservation-minded individuals, to conservation non-profits, to government agencies—increasingly recognize the ecosystem services that diversifying farming systems can provide, like managed grazing to improve endangered species habitat or reduce fuel load in fire-prone areas (Plieninger et al., 2012). Landowners can make lease agreements that specify the use of conservation practices, and can choose to prioritize farmers who manage their farm enterprises in ways that benefit the land, and farmers can negotiate more favorable lease terms or prices in exchange for providing these services to landowners, thus improving the land and/or generating ecosystem service payments through healthy soils or carbon farming programs (Ribaudo et al., 2010; Iles and Marsh, 2012; Ma et al., 2012; Petrzelka et al., 2013). Education and outreach programs for landowners regarding conservation practices can also be effective at increasing incorporation of diversified farming practices into agreements. One innovative example from the US Midwest tailors conservation programs for women non-operator landowners, who own half of the farmland but participate less in conservation decisions than non-operator landlord men (Wells and Eells, 2011). Following women-only field training in conservation practices, women non-operator landowners were substantially more likely to participate in decisions with tenants to implement conservation practices (Sreenviasan, 2020). Novel institutional opportunities to shift leasing norms are also emerging, for example through agricultural land trusts and agricultural easements that maintain land in agriculture into perpetuity.
Alternative ownership structures, like grower cooperatives—in which producers own a collective stake in the farm business—may facilitate diversification pathways and lead to greater adaptive capacity. For example, in the southern US, the Federation of Southern Cooperatives began reenergizing the cooperative farming model that had been popular among Black farmers in the late nineteenth century and early twentieth century to facilitate the sharing of experiences and expenses and slow the tide of farmland loss among Black farmers (White, 2018). Farming cooperatives, equipment cooperatives, and farm incubator programs all provide institutional mechanisms for farmers to share resources, like specialized equipment such as seed drills, which can be prohibitively expensive for individual farmers to purchase, posing a barrier to implementing diversifying practices like reduced tillage (Carlisle et al., 2019b).
Because biodiversity-enhancing strategies are best managed by coalitions of land managers working at a landscape scale (Brodt et al., 2008), diversifying farming systems offers opportunities to coordinate land managers in regionally scaled networks. In the 1990s, with government support, the grassroots Landcare movement in Australia motivated thousands of farmers to form local groups to cooperate in conservation projects, like controlling invasive species or managing soil erosion (Curtis and De Lacy, 1996; Sobels et al., 2001). Similarly, US farmers and ranchers often coordinate management efforts informally through “norms of neighborliness” (Yung and Belsky, 2007) or formally through coordinated ranch management planning for habitat restoration goals (Petersen-Rockney, in progress).
In summary, the simplifying pathway limits long-term adaptive capacity by failing to address the significant barriers that insecure land tenure poses to adopting sustainable farming practices, and that limited land access poses to diverse new-entrant farmers. A diversifying pathway, in contrast, would seek to secure tenure and expand land access through both innovative resource-sharing mechanisms and legal and policy reforms, foundational for the emergence of just and sustainable adaptive capacity.
Discussion: Diversifying Farming Systems for Adaptive Capacity
Using the same integrated four-point framework (see section Research Questions and Objectives and Tables 1, 2), we have contrasted the properties and qualities of adaptive capacity that emerge from simplifying vs. diversifying adaptation pathways for five widely varying agricultural challenges. Synthesizing insights across these five cases illuminates the potential for diversifying strategies to preserve and, ideally, enhance core social-ecological functioning in the face of climate change, biodiversity loss, and global food insecurity (Figure 3 and Table 1). Diversifying processes, we argue, weave a form of broad and nimble adaptive capacity that is fundamentally distinct from the narrow and brittle adaptive capacity produced through simplification. Diversifying processes also demonstrate potential to enhance equity and sustainability. Yet our analysis reveals some cross-cutting barriers to diversification, such as exclusionary land tenure regimes and lack of available markets for diverse farm products. To give a specific example, marginal land may be drought-prone, and those farming it more likely to be disenfranchised with insecure land tenure (section Farming Marginal Land). While there are barriers, there are also synergies and positive feedback dynamics that can arise among the ecological, managerial, economic, scientific, and institutional opportunities to diversify farming systems (Figure 3 and Table 2). Finally, we acknowledge the limitations of our approach and analysis, and suggest new research directions to fill key gaps in our understanding of the potential to diversify farming systems for adaptive capacity.
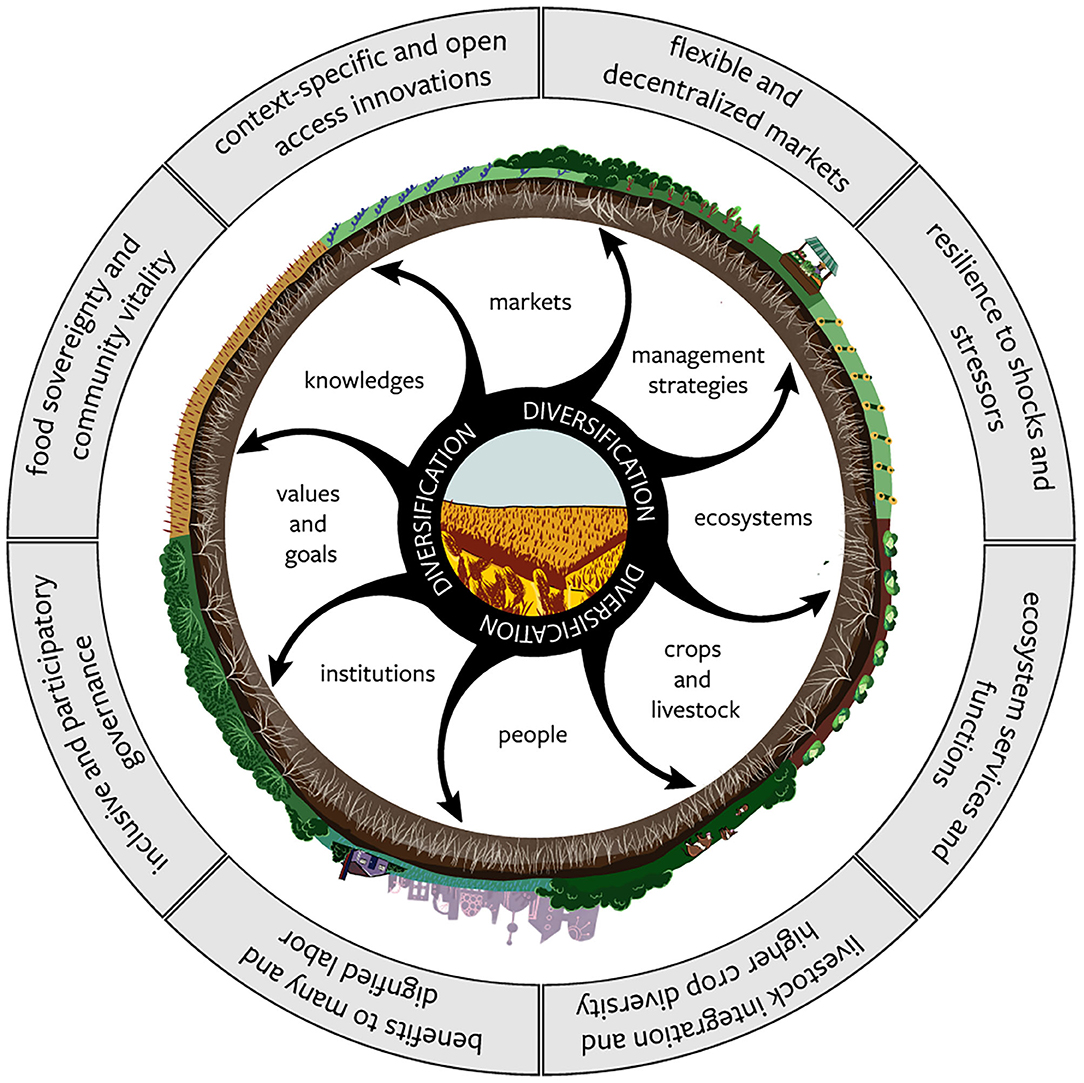
Figure 3. As farming systems move from a simplified state (center of wheel) to a more diversified state (outer ring of wheel) along multiple dimensions (spokes of the wheel) adaptive capacity can emerge. But the wheel will only “turn well” when multiple dimensions grow together and interact, i.e., progress along only one or two dimensions will not be sufficient to create these properties.
Simplifying Farming Systems Leads to Narrow and Brittle Adaptive Capacity
Stressors and shocks of various kinds will force farm management responses that, under current structural conditions, are likely to further simplify farming systems via greater reliance on proprietary capital-intensive inputs and concentrated markets. As noted earlier, while the majority of farms will struggle to survive, some well-capitalized farms will likely prosper under these conditions. Yet even those limited benefits may be vulnerable as the triple threat intensifies. At the system level, across our five cases we observe that the intensity of stressors and the magnitude of potential shocks increase as farms become locked-in to simplifying pathways of production that are expected to hold constant across space and time.
As the five cases highlighted, although simplifying pathways may appear to increase adaptive capacity to field-level shocks or stressors when those problems are considered in isolation, they often prove to be brittle responses to the complex and intersecting challenges of the Anthropocene. Simplification strategies can sometimes “resolve” field-level challenges and stabilize yields of specific crops under a narrow set of predictable conditions (Swift et al., 2004). However, these fixes may be prone to sudden catastrophic failure, inasmuch as when they break, they break big. For example, when the food safety system does experience a local lapse in control, and some pathogenic contamination is able to enter the supply chain, the combination of centralized processing and long-distance distribution can send the entire system into shock, i.e., a pathogen outbreak (section Living With Foodborne Pathogens). As another example, farmland financialization may improve irrigation infrastructure that can boost yields on marginal land in the short term, or allow for planting high-value perennial crops, but those gains will fade as climate change brings multi-year droughts unprecedented in modern times, leading to depletion of groundwater resources and increased soil salinity (sections Weathering Drought and Farming Marginal Land). Regional stressors like droughts and labor shortages can also cause synchronized production shocks that destabilize entire markets, especially when farms specialize in only a handful of commodities and supply chains (Table 2). With sufficient access to capital and institutional support structures, simplified farms can respond to singular stressors or shocks in isolation. A few well-resourced farms may, for example, be able to track market trends closely and quickly replace low-demand crops with high-demand/value alternatives, as some produce growers have done in response to shifting consumer demands in the COVID-19 pandemic (Yaffe-Bellany and Corkery, 2020). Yet the COVID-19 pandemic has highlighted how brittle our current food system is. Supply chains have broken under the strain of rapid consumer and labor shifts, forcing dairy farmers to dump their milk down the drain, meat producers to cull animals when the only facilities that can process their products shut down, produce farmers to let their crops rot in the field, and farmworkers to face the impossible choice between unemployment (and financial ruin) and risking life-threatening illness on the job (Ransom et al., 2020). In summary, apparent gains through simplification represent a form of temporary and “highly optimized tolerance” that makes the system inflexible to novel or compounded stressors and shocks (Janssen et al., 2007).
The kind of adaptive capacity pursued through simplification is also narrow, in two senses. First, by focusing on maximizing production of a limited number of commodities by fewer and fewer firms, simplification narrows the range of ecological and social functions sought to be preserved through adaptation, in part because they are not prioritized in the first place (Kloppenburg, 2005; Khoury et al., 2014; Montenegro de Wit, 2017b; Howard, 2020). Planting a single high-yielding and drought-tolerant patented crop variety, for example, may increase yields during a moderate drought, but fail during more severe droughts or other extreme weather (e.g., floods or high winds) in the absence of other adaptive practices (section Weathering Drought). Second, the benefits of simplification accrue to a narrow slice of people—a privileged minority—while production risks associated with simplification are often externalized into the public sphere through programs like commodity price supports and subsidized crop insurance (Graddy-Lovelace and Diamond, 2017), and onto the bodies of farmworkers and low-income and majority-black-and-brown communities who are disproportionately exposed to dangerous work and pollutants (Harrison, 2006; Fleischman and Franklin, 2017). For instance, the capacity for market flexibility following COVID-19 mentioned above does not account for farmworker health risks as an externality (Chang and Holmes, 2020). In general, the narrowing of adaptive responses leads to further inequity in distributions of benefits and burdens as, in the earlier example of patented drought-tolerant varieties, owners of the germplasm, land, and other inputs profit while farmers and farmworkers bear the burdens of risks like harvest failures (section Dignifying Labor).
Our cases show that a rigid social hierarchy exacerbates exploitation and produces further externalities (section Dignifying Labor), centralized regulatory systems disproportionately burden small scale agriculturalists (section Living With Foodborne Pathogens), and an inflexible property regime leads to gradual consolidation of land over time, cutting off access to socioeconomically disadvantaged agriculturalists (sections Farming Marginal Land and Enhancing Land Access and Tenure). In sum, fewer people benefit in fewer ways from the kind of narrow adaptive capacity that emerges through further simplification.
That simplifying processes produce narrow and brittle adaptive capacity is borne out by mounting evidence that agricultural simplification itself is a major cause of the Anthropocene triple threat. As long as the underlying structural paradigms of our dominant global food system persist, without specific attention to the processes by which they meet their goals of “incentivizing and enabling intensification” (Campbell et al., 2014), these approaches portend further entrenchment of simplified agriculture. Additionally, simplified farming systems must be homogenous over large spatial and temporal scales to allow for standardization, which leads to greater agroecosystem sensitivity to environmental stressors (Lobell et al., 2014; Ortiz-Bobea et al., 2018; Renard and Tilman, 2019). To the extent that “diversity and redundancy are an insurance against uncertainty and surprise,” simplification not only exacerbates climate change and biodiversity loss, but also impairs effective responses to these stressors (Darnhofer et al., 2010). Moving toward further simplification in reaction to these challenges will likely undermine the processes by which a more sustainable and equitable adaptive capacity to the triple threat could emerge.
Diversifying Farming Systems Leads to Broad and Nimble Adaptive Capacity
We also asked specifically how diversifying processes might promote sustainability and equity across multiple levels, scales, and functions simultaneously. Our cases show that pursuing diversification pathways through multiple social and ecological domains can lead to a virtuous cycle in which multiple beneficial qualities can emerge. The cycle, however, will only ‘turn well’ when multiple dimensions grow together and interact, i.e., progress along only one or two dimensions will not be sufficient to create these properties (Figure 3). For example, while adopting diversifying agroecological field management practices can increase resilience to climate shocks and stressors at the farm scale, that adaptation alone cannot defuse global threats or build systemic adaptive capacity (Holt-Giménez et al., 2021). Diversifying farming systems can support the emergence of a broader, more robust, sustainable, and equitable adaptive capacity. Adaptive capacity that emerges from diversifying farming systems can enable nimble responses to a broad spectrum of possible, even not-yet-imagined, stressors and shocks. Instead of locking farming systems into pernicious cycles that reproduce social and ecological externalities, systemic commitment to social equity and ecological sustainability becomes the self-reinforcing conditions from which adaptive capacity emerges. Greater social equity leads to better balance between the priorities of privileged and vulnerable populations (reducing social externalities), while ecologically sustainable management seeks balance between present and future needs (reducing environmental externalities). Both equity and sustainability bolster the capacity to recognize localized stressors early, enabling more rapid and precise reallocation of resources and expertise to mitigate those stressors before they amplify into systemic shocks. Equity and sustainability are thus both prerequisite conditions for high adaptive capacity in farming systems, and our cases suggest that both qualities can emerge from diversifying processes.
The ability to envision and prioritize multiple goals appears across our cases as an essential condition for diversification. It is critical to value farming system functions beyond maximizing yield and profit to include conserving biodiversity, supporting community vitality, strengthening food security and sovereignty, enhancing justice and equity, and increasing non-commodity ecosystem services to and from agriculture (Maier and Shobayashi, 2001; Van Huylenbroeck et al., 2007; Renting et al., 2009). Agriculture can, and should, support a wide range of benefits. Multifunctional agricultural systems emphasize social and environmental values and components of farming activities beyond private or individual values. Intentionally promoting multiple and accessible functions facilitates the emergence of system properties that underlie adaptive capacity. For instance, when diversifying practices adopted at landscape scales promote soil-based ecosystem services like water capture and storage, agroecosystems can better cope with droughts and more farmers can access this benefit. Furthermore, adaptive capacity may itself be an explicit goal, either as a generic proactive preparation for uncertain future challenges or as a specific catalyzing agent for other goals, such as conserving biodiversity amidst increasingly forceful food safety pressures.
Whether in response to crisis, slow-simmering changes in consciousness, shifting social-ecological circumstances, or other triggers, when decision-makers and farmers recognize and act on multiple goals they often take diversification steps across ecological, social, and economic dimensions (Atwell et al., 2009; Carlisle, 2014; Roesch-McNally et al., 2018). Although structural forces and macro-scale factors still constrain adaptation (Stuart and Gillon, 2013; Roesch-McNally et al., 2018), the resulting diversification creates options and flexibility in responding to stressors and shocks, spreads risks, and increases response diversity—all of which facilitate the emergence of adaptive capacity.
Balancing the pursuit of multiple goals requires an inclusive process for active dialogue and iterative negotiation among diverse and potentially diverging perspectives. For example, dignifying farm labor will not be achieved through ecological diversification alone, but also requires reconfiguring hierarchical relationships among workers, managers, and owners. Conceptualizing social-ecological function to encompass multiple provisioning and service goals simultaneously not only widens the scope of potential solutions and the set of metrics by which to evaluate those solutions (International Panel of Experts on Sustainable Food Systems, 2016), but also allows for redistributing decision-making power, agency, and voices from owners and managers to frontline workers.
The levels at which stressors and shocks first manifest or can best be managed—such as regional labor markets or climates—do not align with a hierarchical understanding of farmers as individual actors, or even actors subject to hierarchical relations of power such as landowner vs. renter, farm manager vs. worker, or individuals vs. states or institutions. For example, drought is often felt at a regional scale while groundwater recharge is best managed at a watershed or landscape scale. Farmworkers may have more direct contact with changing field conditions, but may not have the authority to make timely management decisions. Additionally, rigid hierarchies also manifest in social networks that can be exclusionary, protecting static notions of culture and antiquated management norms (Naranjo, 2012; Bidwell et al., 2013; Davidson, 2016). Rigid hierarchies therefore limit system adaptation to specific levels with little recognition that farming systems are deeply interconnected through biophysical and socioeconomic ties. Instead, farming systems require a high degree of coordination and cooperation across levels in order for adaptive capacity to emerge.
Systemic diversification may provide a pathway to iteratively dismantle the lock-ins that perpetuate simplification and associated hierarchy. Greater biological diversity and access to more diverse and redundant market channels and community connections, for example, equips agricultural actors with management options to nimbly navigate through stressors like droughts or supply chain disruptions from foodborne pathogens or pandemics. Moreover, a dynamic operation may better attract skilled workers, who then add their human capital to further bolster its adaptive capacity. In contrast to the rigid hierarchies characteristic of simplifying pathways, our cases suggest that adaptive capacity can be facilitated by empowering people and enhancing ecosystem functionality to proactively distribute resources and knowledge where needed and nimbly respond to changing circumstances.
Challenges and Opportunities That Arise With Diversification
While our cases highlight a broad range of diversifying opportunities, they also reveal tradeoffs, limitations, complexities, and uncertainties that can manifest through diversifying farming systems, as well as the unexpected opportunities that can be harnessed from change. One important limitation is that enhancing ecological diversity alone is insufficient to promote sustainable and equitable adaptive capacity. Ecological diversification cannot be practiced widely without addressing the environmental, cultural, political, economic, institutional, and technological factors that constrain farmers' capacity to diversify at the farm level. Examples of these factors from our cases include the ubiquity of access to labor, land, and markets in mediating farmers' capacity to increase crop diversity or substitute on-farm ecosystem services for external capital-intensive inputs (e.g., sections Weathering Drought and Farming Marginal Land). Our cases demonstrate how building adaptive capacity requires diversifying processes across all of these dimensions—from diverse institutional arrangements to better manage foodborne pathogens (section Living With Foodborne Pathogens), to increasing land access and tenure for new entry farmers (section Enhancing Land Access and Tenure), to diversifying open-source technologies and broadening who is considered a holder of knowledge to enhance marginal lands and protect cultural landscapes (section Farming Marginal Land).
Diversification as a general strategy across these many dimensions seems to face a “chicken-and-egg” dilemma: no single diversifying step definitively comes first. Rather many steps likely need to be made iteratively and in concert with one another (Figure 3); otherwise, diversifying along one dimension may pose a tradeoff with another dimension. For example, increasing crop diversity in the absence of reforms for farmworker protection and compensation may actually further entrench hierarchical and exploitative labor relations (section Dignifying Labor). On the other hand, this lack of clearly ordered steps may open many entry points for farmers and other decision-makers to begin diversifying from any starting point and along any dimension, no matter how simplified their farming system is to begin with (Figure 3). We see a pressing need to consider opportunities for structurally diversifying farming systems, such as the nested regulatory system suggested for enhancing adaptive capacity to foodborne pathogens or supply chain flexibility in demand and distribution to better absorb the harmful economic impacts of drought events (Table 2 and Appendix 1). Opportunities for local diversification exists, but will require systemic support from higher levels to reach their full potential to enhance sustainable and equitable adaptive capacity.
Another challenge arises from the complexity that diversification intentionally introduces into agri-food systems. As the complexity of a system increases, so does uncertainty about the specific outcome of any given event (e.g., a decision or disturbance), especially at fine-grained resolution. Furthermore, stochasticity, non-linearities, and surprises increase- despite our increased ability to use complex models- the ability to apply mechanistic thinking decreases (Preiser et al., 2018). Complex adaptive systems also tend toward dynamic equilibria, suggesting that rather than seeking persistent adherence to a desired state, adaptive capacity more humbly seeks ephemeral balance points that are ever-changing or fluctuating (Gunderson and Holling, 2001; Allen et al., 2014). Adaptively managing complex systems also means tolerating uncertainty and accepting a loss of short-term, precise control in favor of long-term, “fuzzy” stability in realizing system goals. Whether a community is responding to unfair labor conditions, a farmer to a severe weather event, or lawmakers to new disease ecologies, every adaptive “solution” is contingent, provisional, and temporary, subject to change as circumstances shift, dialogue progresses, and new insights are learned. When the outcomes of an action are unknown or uncertain, cycles of targeted experimentation, observation, learning, reflecting, and adjustment are key (Allen et al., 2011), as are participatory conversations that more fully represent the range of ways to frame problems and solutions and weigh the potential distribution of burdens and benefits (Jasanoff, 2003).
In our cases, farmers face ongoing challenges to balance on-farm biodiversity with the potential transmission of pathogens onto growing crops (section Living With Foodborne Pathogens), work to improve land and ecosystem resilience on marginal lands while maintaining production that supports livelihoods (section Farming Marginal Land), and manage labor shortages while addressing more labor-intensive practices associated with ecologically based farming practices (section Dignifying Labor). Adaptive capacity therefore does not aim to “fix problems” that farmers face, but to enable self-organization such that farming systems minimize challenges to begin with and can functionally persist, and even improve, through change (Vandermeer and Perfecto, 2017). For example, diversifying pathways for weathering drought —e.g., soil management practices that improve water capture and storage— do not immediately deliver water when a drought occurs, but if these field management practices are in place and supported by secure land tenure, diverse markets, and workers valued for their knowledge, the farm will likely be less negatively impacted by drought and may even be able to harness new cropping or marketing opportunities.
Knowledge Gaps and Future Research Directions
Scholars, practitioners, and policy makers can use the conceptual framework presented in this article (Figure 3) to describe, evaluate, and guide efforts to diversify farming systems in order to enhance their adaptive capacity. We identified research needs for each case presented (Appendix 1), which articles in this special issue begin to address. We invite future research that utilizes our framework, and tests its utility, in assessing how diversifying farming systems can nurture adaptive capacity to climate change, biodiversity loss, and food insecurity, as well as novel shocks and stressors. The COVID-19 pandemic, for example, is already transforming long-standing challenges such as labor shortages and exacerbating systemic threats like global food insecurity.
Many questions remain about potential institutional, market, and policy reforms that could increase adaptive capacity by diversifying farming systems while also promoting equity and sustainability. Case studies and comparisons of policy instruments, legal tools, and equitable decision-making structures can, for example, help us understand the conditions under which diversification can be pursued or continued on marginal lands without compromising resource-poor farmers or sensitive ecosystem needs (section Farming Marginal Land). Likewise, exploring how to de-silo the US food safety regulatory regime would enable situating food safety goals within a broader governing framework for just and healthy food systems (section Living With Foodborne Pathogens). Studies that explore how to manage landscapes collectively are crucial to promoting the emergence of adaptive capacity at the landscape and community levels (section Enhancing Land Access and Tenure).
Future work that is situated in specific social and ecological contexts, including research outside the US, can highlight opportunities for public policy and social movements to incentivize and increase adaptive capacity by diversifying farming systems. For example, empirical studies that document how soil improvements made through diversified farming practices affect drought responses will need to consider a myriad of environmental, social, and economic factors to understand context-specific tradeoffs, leverage synergies, and mitigate risks (section Weathering Drought). To assess whether agricultural diversification reduces or enhances adaptive capacity in the case of farm labor, research must grapple with considerable agronomic and socioeconomic heterogeneity (section Dignifying Labor). The challenge of land access and tenure highlights the need for regionally contextualized understandings of who has the power to implement diversifying land management pathways (see Calo, 2020, in this special issue). Geographies outside the US, for example, illustrate a wide variety of socio-legal land tenure contexts, such as communal lands and sovereign sub-territories, that demand analysis beyond landlord-tenant dynamics (section Enhancing Land Access and Tenure).
Diversifying farming systems and building adaptive capacity requires promoting diversity in scholars and scholarship. This “thickening” of the legitimacy of agroecological knowledge must include work conducted by people and in ways not generally recognized as conventional scientists and science (Montenegro de Wit and Iles, 2016). Diverse knowledge bearers and ways of knowing (Haraway, 1988) invite more collaborative, transdisciplinary, and participatory research (Méndez et al., 2013) that can “take better account of the world” in particular times and places (Fortmann, 2008)—a prerequisite for context-specific diversification pathways that can build real-world equitable and sustainable adaptive capacity. Applied, participatory agroecological research is necessary to, for example, identify key drought-related issues, find innovative and appropriate practices, and select species that are context-specific in their ability to tolerate and alleviate drought stress (section Weathering Drought). Employing our conceptual framework, future research on farm labor should, for example, include perspectives from people who work on farms in the process of appropriately scaling and co-designing technologies that support diversification while also protecting farmworkers by alleviating physically dangerous work (section Dignifying Labor).
A diversifying farming systems research agenda must also analyze the institutionalized and entrenched power hierarchies that limit the potential for diversification strategies to benefit food and farm workers as well as environments. This includes interrogating legacies within our own research institutions that have facilitated simplification, from the expropriation of Indigenous land (Lee and Atone, 2020) to ongoing privatization of public resources and knowledge (Hightower, 1972; Kloppenburg, 2005). Research and development that encourages simplifying pathways dominate our public and private institutions, at least in the United States, leaving diversifying pathways largely sidelined and ignored (Miles et al., 2017). Limited resources to conduct sustainable agriculture research remains a significant obstacle to addressing the gaps outlined in this article (DeLonge et al., 2020). Research is needed to understand precisely how to shift resources and energy away from simplifying and toward diversifying farming systems simultaneously in both our research institutions and the broader agri-food system in ways that support actors in the process of transitioning pathways (see, for example, sections Living With Foodborne Pathogens and Weathering Drought).
Our conceptual framework highlights the conditions under which farming systems can cultivate forms of adaptive capacity with qualities congruent with principles of equity and sustainability. Future studies should also consider how diversifying farming systems and emergent adaptive capacity can resist market capture and dilution, learning from past efforts in organics (Guthman, 2004) and fair trade (Jaffee, 2014). Simultaneously, there may be transition opportunities for those who employ diversifying farming systems practices (such as agroforestry or cover cropping) to benefit from market-based funding mechanisms, like payment for ecosystem services programs or market premiums. Identifying inflection points when diversifying farming systems can overcome barriers, even turning them into opportunities, in response to global change will continue to be important for moving on a pathway toward a more just, sustainable, and agroecological food system that benefits a broad range of actors.
Limitations of Framework
We have outlined a descriptive framework to appraise agri-food system challenges, impacts of the Anthropocene triple threat, and the potential for sustainable and equitable adaptive capacity to emerge from diversifying farming systems. This framework can provide the foundation for developing future analytical and intervention-oriented tools to facilitate the emergence of adaptive capacity through diversification. The complexity and heterogeneity that arises from diversification, which is so crucial to adaptive capacity, complicates efforts to model or predict how these systems respond to global threats (Morton, 2007). This framework is not intended to specify precisely what should be analyzed or define the units of analysis. These points will need to be developed for each context-specific study before comparisons, evaluations, experiments, interventions, or other research processes are pursued in future work. While this is certainly a limitation of this paper, it is so by design—diversification and adaptation to context are also foundational processes in the type of reflexive research needed to diversify farming systems.
Most participants in this group conduct research in the United States and other high-income country contexts, and only a few of us have direct experience as farmers or practitioners. Most of our specific cases are situated in particular US contexts, with some resonant global examples used. We invite future work exploring the emergence of adaptive capacity from diversifying farming systems and the applicability of the framework to other contexts. Recognizing that these biases limit the scope of our assessments, we recommend that future work in this area seek to represent a broader and more inclusive range of interdisciplinary knowledge, multi-stakeholder expertise, and social network innovation and exchange within situated local contexts (Iles and Marsh, 2012).
Conclusion
Rather than fixed states, diversification and simplification are ongoing and iterative adaptation pathways in farming systems. Building on this conceptual extension, we presented a novel framework that integrates social and ecological systems to analyze the properties of adaptive capacity that emerge through simplifying as opposed to diversifying pathways. Applying our framework to five distinct cases of stressors in the agri-food system, we have found that:
1. Simplifying processes, though high performing under narrow metrics of productivity, lead toward a narrow and brittle adaptive capacity marked by exacerbated long-term vulnerability to the triple threat of climate change, biodiversity loss, and food insecurity.
2. Diversifying processes, though their benefits may not accrue directly or immediately, lead toward broad and nimble adaptive capacity marked by long-term and equitably distributed resilience to the shocks and stresses emanating from the triple threat.
3. Both simplifying and diversifying processes are self-reinforcing, forming “vicious” or “virtuous” cycles, respectively.
4. Diversifying farming systems means pursuing multiple goals in concert across multiple levels and domains simultaneously (ecological, structural, social, cultural, economic, etc.) with attention to potential tradeoffs.
5. Diversifying farming systems offers a pathway to embrace complexity and uncertainty, which is particularly useful in times of global shocks (e.g., pandemic) and change (e.g., long-term droughts).
Diversifying farming systems provides opportunities to increase adaptive capacity in various ways—including via different processes, magnitudes, and rates of adoption—that are ecologically and culturally appropriate to their specific environmental, social, political, and market contexts. The framework we have outlined invites ongoing participation of individuals, communities, and institutions—irrespective of scale or starting point—to evaluate and enhance the qualities of adaptive capacity that emerge from divergent adaptation pathways in farming systems. Understood as an ongoing process, diversifying pathways can be an inclusive space to “call in” producers, policy makers, and other actors to take steps toward enhancing adaptive capacity to pressing global threats. We hope that diversifying farming systems to increase adaptive capacity can help build a more representative coalition of farmers and support emancipatory agroecology movements, adding to the well-developed scholarship and practice of agroecology.
Author Contributions
MP-R conceived and presented the idea and wrote the initial draft. MP-R, PB, and TB developed the framework, literature review, theory, co-wrote iterative drafts, edited all cases, coordinated meetings, facilitated discussions, and managed contributions. PB and PS drafted the foodborne pathogen case. MM, YS, SB, JO, and TB drafted the drought case. AG, KD, CK, MP, and MP-R drafted the marginal land case. AI, FC, and AD drafted the labor case. AC, MP-R, KD, and JL drafted the land access and tenure case. AI and CK edited the entire article and assisted in conceptual refinement. MM, KE, and MP provided helpful edits to the full text. AG created Figure 1 and Figure 3 with conceptual development by MP-R, PB, and TB. TB and MP-R developed Figure 2. PB led the development of Box 1, Tables 1, 2 with TB, AI, and MP-R. All authors discussed the broad ideas as part of the Diversified Farming Systems Center weekly meetings during Spring 2019 at the University of California, Berkeley, were invited to edit the full manuscript, and approved publication.
Funding
TB, AI, CK, FC, PB, JO, KE, and KD acknowledge USDA AFRI Grant # 2019-67019-29537 and NSF Coupled Natural Humans Systems Grant #1824871. FC acknowledges University of California Health Initiative of the Americas and the University of California Program Alianza UCMX. TB and MM acknowledge USDA AFRI Grant # 2017-67013-26254. MP-R acknowledge NSF GRFP #2018255596.
Conflict of Interest
The authors declare that the research was conducted in the absence of any commercial or financial relationships that could be construed as a potential conflict of interest.
Acknowledgments
We would like to thank the Center for Diversified Farming Systems at the Berkeley Food Institute for bringing these authors together. We would like to thank Milton Stookey for assistance with references.
Supplementary Material
The Supplementary Material for this article can be found online at: https://www.frontiersin.org/articles/10.3389/fsufs.2021.564900/full#supplementary-material
References
Allen, C. R., Angeler, D. G., Garmestani, A. S., Gunderson, L. H., and Holling, C. S. (2014). Panarchy: theory and application. Ecosystems 17, 578–589. doi: 10.1007/s10021-013-9744-2
Allen, C. R., Fontaine, J. J., Pope, K. L., and Garmestani, A. S. (2011). Adaptive management for a turbulent future. J. Environ. Manage. Adapt. Manage. Nat. Resour. 92, 1339–1345. doi: 10.1016/j.jenvman.2010.11.019
Altieri, M. A. (1999). The ecological role of biodiversity in agroecosystems. Agric. Ecosyst. Environ. 74, 19–31. doi: 10.1016/S0167-8809(99)00028-6
Altieri, M. A. (2002). Agroecology: the science of natural resource management for poor farmers in marginal environments. Agric. Ecosyst. Environ. 93, 1–24. doi: 10.1016/S0167-8809(02)00085-3
Altieri, M. A., and Toledo, V. M. (2011). The agroecological revolution in Latin America: rescuing nature, ensuring food sovereignty and empowering peasants. J. Peasant Stud. 38, 587–612. doi: 10.1080/03066150.2011.582947
Anderson, C. R., Bruil, J., Chappell, M. J., Kiss, C., and Pimbert, M. P. (2019). From transition to domains of transformation: getting to sustainable and just food systems through agroecology. Sustainability 11:5272. doi: 10.3390/su11195272
Ansell, C., and Baur, P. (2018). Explaining trends in risk governance: how problem definitions underpin risk regimes. Risk Hazards Crisis Public Policy 9, 397–430. doi: 10.1002/rhc3.12153
Ashwood, L., Diamond, D., and Thu, K. (2014). Where's the farmer? Limiting liability in midwestern industrial hog production. Rural Sociol. 79, 2–27. doi: 10.1111/ruso.12026
Astill, G., Minor, T., Calvin, L., and Thornsbury, S. (2018). Before Implementation of the Food Safety Modernization Act's Produce Rule: A Survey of U.S. Produce Growers. AgEcon Search. USDA.
Atwell, R. C., Schulte, L. A., and Westphal, L. M. (2009). Linking resilience theory and diffusion of innovations theory to understand the potential for perennials in the US corn belt. Ecol. Soc. 14:30. doi: 10.5751/ES-02787-140130
Bacon, C. M., Getz, C., Kraus, S., Montenegro, M., and Holland, K. (2012). The social dimensions of sustainability and change in diversified farming systems. Ecol. Soc. 17:41. doi: 10.5751/ES-05226-170441
Basche, A., and DeLonge, M. S. (2017). The impact of continuous living cover on soil hydrologic properties: a meta-analysis. Soil Sci. Soc. Am. J. 81, 1179–1190. doi: 10.2136/sssaj2017.03.0077
Basche, A., and DeLonge, M. S. (2019). Comparing infiltration rates in soils managed with conventional and alternative farming methods: a meta-analysis. PLoS ONE 14:e0215702. doi: 10.1371/journal.pone.0215702
Baur, P. (2020). When farmers are pulled in too many directions: comparing institutional drivers of food safety and environmental sustainability in California agriculture. Agric. Human Values 37, 1175–1194. doi: 10.1007/s10460-020-10123-8
Baur, P., Driscoll, L., Gennet, S., and Karp, D. (2016). Inconsistent food safety pressures complicate environmental conservation for California produce growers. California Agric. 70, 142–151. doi: 10.3733/ca.2016a0006
Baur, P., Getz, C., and Sowerwine, J. (2017). Contradictions, consequences and the human toll of food safety culture. Agric. Human Values 34, 713–728. doi: 10.1007/s10460-017-9772-1
Beckett, J., and Galt, R. E. (2014). Land trusts and beginning farmers' access to land: exploring the relationships in coastal California. J. Agric. Food Syst. Commun. Dev. 4, 19–35. doi: 10.5304/jafscd.2014.042.008
Bender, S. F., Wagg, C., and van der Heijden, M. G. A. (2016). An underground revolution: biodiversity and soil ecological engineering for agricultural sustainability. Trends Ecol. Evol. 31, 440–452. doi: 10.1016/j.tree.2016.02.016
Bennett, S. D., Sodha, S. V., Ayers, T. L., Lynch, M. F., Gould, L. H., and Tauxe, R. V. (2018). Produce-associated foodborne disease outbreaks, USA, 1998–2013. Epidemiol. Infect. 146, 1397–1406. doi: 10.1017/S0950268818001620
Beretti, M., and Stuart, D. (2008). Food safety and environmental quality impose conflicting demands on central coast growers. California Agric. 62, 68–73. doi: 10.3733/ca.v062n02p68
Bidwell, D., Dietz, T., and Scavia, D. (2013). Fostering knowledge networks for climate adaptation. Nat. Clim. Chang. 3, 610–611. doi: 10.1038/nclimate1931
Bocco, G., and Napoletano, B. M. (2017). The prospects of terrace agriculture as an adaptation to climate change in latin America. Geogr. Compass 11:e12330. doi: 10.1111/gec3.12330
Bodner, G., Loiskandl, W., and Kaul, H. P. (2007). Cover crop evapotranspiration under semi-arid conditions using FAO dual crop coefficient method with water stress compensation. Agric. Water Manag. 93, 85–98. doi: 10.1016/j.agwat.2007.06.010
Borras, S., Franco, J., and Suárez, S. M. (2015). Land and food sovereignty. Third World Q. 36, 600–617. doi: 10.1080/01436597.2015.1029225
Bovay, J., Ferrier, P., and Zhen, C. (2018). Estimated Costs for Fruit and Vegetable Producers to Comply With the Food Safety Modernization Act's Produce Rule. AgEcon Search. USDA.
Bowles, T. M., Atallah, S. S., Campbell, E. E., Gaudin, A. C. M., Wieder, W. R., and Grandy, A. S. (2018). Addressing agricultural nitrogen losses in a changing climate. Nat. Sustain. 1, 399–408. doi: 10.1038/s41893-018-0106-0
Bowles, T. M., Mooshammer, M., Socolar, Y., Calderón, F., Cavigelli, M. A., Culman, S. W., et al. (2020). Long-term evidence shows that crop-rotation diversification increases agricultural resilience to adverse growing conditions in North America. One Earth 2, 284–293. doi: 10.1016/j.oneear.2020.02.007
Boyer, J. S., Byrne, P., Cassman, K. G., Cooper, M., Delmer, D., Greene, T., et al. (2013). The U.S. drought of 2012 in perspective: a call to action. Global Food Security 2, 139–43. doi: 10.1016/j.gfs.2013.08.002
Brodt, S., Klonsky, K., Jackson, L., Brush, S., and Smukler, S. (2008). Factors affecting adoption of hedgerows and other biodiversity-enhancing features on farms in California, USA. Agrofor. Syst. 76, 195–206. doi: 10.1007/s10457-008-9168-8
Brown, P. W., and Schulte, L. A. (2011). Agricultural landscape change (1937–2002) in three townships in Iowa, USA. Landsc. Urban Plan. 100, 202–212. doi: 10.1016/j.landurbplan.2010.12.007
Burrows, K., and Kinney, P. L. (2016). Exploring the climate change, migration and conflict nexus. Int. J. Environ. Res. Public Health 13:443. doi: 10.3390/ijerph13040443
Busch, L. (2010). Can fairy tales come true? The surprising story of neoliberalism and world agriculture: neoliberalism and world agriculture. Sociol. Ruralis 50, 331–351. doi: 10.1111/j.1467-9523.2010.00511.x
Calderón, C. I., Jerónimo, C., Praun, A., Reyna, J., Castillo, I. D. S., León, R., et al. (2018). Agroecology-based farming provides grounds for more resilient livelihoods among smallholders in western guatemala. Agroecol. Sustain. Food Syst. 42, 1128–1169. doi: 10.1080/21683565.2018.1489933
Callaway, T. R., Carr, M. A., Edrington, T. S., Anderson, R. C., and Nisbet, D. J. (2009). Diet, Escherichia coli O157:H7, and cattle: a review after 10 years. Curr. Issues Mol. Biol. 11:67. doi: 10.21775/cimb.011.067
Callaway, T. R., Elder, R. O., Keen, J. E., Anderson, R. C., and Nisbet, D. J. (2003). Forage feeding to reduce preharvest Escherichia coli populations in cattle, a review. J. Dairy Sci. 86, 852–860. doi: 10.3168/jds.S0022-0302(03)73668-6
Calo, A. (2018). How knowledge deficit interventions fail to resolve beginning farmer challenges. Agric. Hum. Values 35, 367–381. doi: 10.1007/s10460-017-9832-6
Calo, A. (2020). ‘Who has the power to adapt?’ Frameworks for resilient agriculture must contend with the power dynamics of land tenure. Front. Sustain. Food Syst. 3:5. doi: 10.3389/fsufs.2020.555270
Calo, A., and De Master, K. (2016). After the incubator: factors impeding land access along the path from farmworker to proprietor. J. Agric. Food Syst. Commun. Dev. 2, 11–127. doi: 10.5304/jafscd.2016.062.018
Calo, A., and Petersen-Rockney, M. (2018). What Beginning Farmers Need Most in the Next Farm Bill: Land, 6. Available online at: https://food.berkeley.edu/wp-content/uploads/2018/08/BFI-Beginning-Farmers-Policy-Brief.pdf
Campbell, B. M., Thornton, P., Zougmoré, R., Van Asten, P., and Lipper, L. (2014). Sustainable intensification: what is its role in climate smart agriculture? Curr. Opin. Environ. Sustain. 8, 39–43. doi: 10.1016/j.cosust.2014.07.002
Carlisle, L. (2014). Diversity, flexibility, and the resilience effect: lessons from a social-ecological case study of diversified farming in the northern great plains, USA. Ecol. Soc. 19:45. doi: 10.5751/ES-06736-190345
Carlisle, L. (2016). Factors influencing farmer adoption of soil health practices in the united states: a narrative review. Agroecol. Sustain. Food Syst. 40, 583–613. doi: 10.1080/21683565.2016.1156596
Carlisle, L., Montenegro de Wit, M., DeLonge, M. S., Calo, A., Getz, C., Ory, J., et al. (2019b). Securing the future of US agriculture: the case for investing in new entry sustainable farmers. Elem. Sci. Anth. 7:17. doi: 10.1525/elementa.356
Carlisle, L., Montenegro de Wit, M., DeLonge, M. S., Iles, A., Calo, A., Getz, C., et al. (2019a). Transitioning to sustainable agriculture requires growing and sustaining an ecologically skilled workforce. Front. Sustain. Food Syst. 3:96. doi: 10.3389/fsufs.2019.00096
Carney, J. A. (2020). Subsistence in the plantationocene: dooryard gardens, agrobiodiversity, and the subaltern economies of slavery. J. Peasant Stud. 1–25. doi: 10.1080/03066150.2020.1725488
Cassidy, E. S., West, P. C., Gerber, J. S., and Foley, J. A. (2013). Redefining agricultural yields: from tonnes to people nourished per hectare. Environ. Res. Lett. 8:034015. doi: 10.1088/1748-9326/8/3/034015
Castillo, F., Sanchez, A., Gilless, J. K., and Wehner, M. (2021). “The impact of heat waves on agricultural labor productivity and output,” in Extreme Events and Climate Change: A Multidisciplinary Approach, eds F. Castillo, M. Wehner, and D. A. Stone (Hoboken, NJ: John Wiley & Sons, Inc.), 11–21.
CGIAR Technical Advisory Committee (2000). CGIAR Research Priorities for Marginal Lands. Available online at: https://cgspace.cgiar.org/handle/10947/332
Chang, V. L., and Holmes, S. M. (2020). US Food Workers Are in Danger. That Threatens All of Us. The Guardian, April 14, 2020, sec. Opinion.
Chappell, M. J. (2018). Beginning to End Hunger. Available online at: https://www.ucpress.edu/book/9780520293090/beginning-to-end-hunger
Chhetri, N., Stuhlmacher, M., and Ishtiaque, A. (2019). Nested Pathways to Adaptation. Environ. Res. Commun.1:015001. doi: 10.1088/2515-7620/aaf9f9
Civitello, D. J., Cohen, J., Fatima, H., Halstead, N. T., Liriano, J., McMahon, T. A., et al. (2015). Biodiversity inhibits parasites: broad evidence for the dilution effect. Proc. Natl. Acad. Sci. U.S.A. 112, 8667–8671. doi: 10.1073/pnas.1506279112
Cochrane, W. (1993). The Development of American Agriculture: A Historical Analysis. University of Minnesota Press.
Curtis, A., and De Lacy, T. (1996). Landcare in Australia: does it make a difference? J. Environ. Manage 46, 119–137. doi: 10.1006/jema.1996.0011
Dainese, M., Martin, E. A., Aizen, M. A., Albrecht, M., Bartomeus, I., Bommarco, R., et al. (2019). A global synthesis reveals biodiversity-mediated benefits for crop production. Sci. Adv. 5:eaax0121. doi: 10.1126/sciadv.aax0121
Darnhofer, I., Bellon, S., Dedieu, B., and Milestad, R. (2010). Adaptiveness to enhance the sustainability of farming systems. A review. Agron. Sustain. Dev. 30, 545–555. doi: 10.1051/agro/2009053
Davidson, D. (2016). Gaps in agricultural climate adaptation research. Nat. Clim. Change 6:433. doi: 10.1038/nclimate3007
De Master, K. (2018). New inquiries into the agri-cultures of the middle. Cult. Agric. Food Environ. 40, 130–135. doi: 10.1111/cuag.12219
De Schutter, O. (2011). The right of everyone to enjoy the benefits of scientific progress and the right to food: from conflict to complementarity. Hum. Rights Q. 33, 304–350. doi: 10.1353/hrq.2011.0020
Deaton, B. J., Lawley, C., and Nadella, K. (2018). Renters, landlords, and farmland stewardship. Agric. Econ. 49, 521–531. doi: 10.1111/agec.12433
DeLind, L. B., and Howard, P. H. (2008). Safe at any scale? Food scares, food regulation, and scaled alternatives. Agric. Hum. Values 25, 301–317. doi: 10.1007/s10460-007-9112-y
DeLonge, M., Robbins, T., Basche, A. D., and Haynes-Maslow, L. (2020). The state of sustainable agriculture and agroecology research and impacts: a survey of U.S. scientists. J. Agric. Food Syst. Commun. Dev. 9, 159–184. doi: 10.5304/jafscd.2020.092.009
DeVincentis, A. J., Solis, S. S., Bruno, E. M., Leavitt, A., Gomes, A., Rice, S., et al. (2020). Using cost-benefit analysis to understand adoption of winter cover cropping in California's specialty crop systems. J. Environ. Manage 261:110205. doi: 10.1016/j.jenvman.2020.110205
Dumont, A., and Baret, P. (2017). Why working conditions are a key issue of sustainability in agriculture? A comparison between agroecological, organic and conventional vegetable systems J. Rural Stud. 56, 1–228. doi: 10.1016/j.jrurstud.2017.07.007
Dumont, A. M., Vanloqueren, G., Stassart, P. M., and Baret, P. V. (2016). Clarifying the socioeconomic dimensions of agroecology: between principles and practices. Agroecol. Sustain. Food Syst. 40, 24–47. doi: 10.1080/21683565.2015.1089967
DuPuis, E. M. (2015). Dangerous Digestion: The Politics of American Dietary Advice. University of California Press.
Edelman, M. (2014). Food sovereignty: forgotten genealogies and future regulatory challenges. J. Peasant Stud. 41, 959–978. doi: 10.1080/03066150.2013.876998
Elias, E., Reyes, J., Steele, C., and Rango, A. (2018). diverse landscapes, diverse risks: synthesis of the special issue on climate change and adaptive capacity in a hotter, drier Southwestern United States. Clim. Change 148, 339–353. doi: 10.1007/s10584-018-2219-x
Elmqvist, T., Folke, C., Nyström, M., Peterson, G., Bengtsson, J., Walker, B., et al. (2003). Response diversity, ecosystem change, and resilience. Front. Ecol. Environ. 1, 488–494. doi: 10.1890/1540-9295(2003)001[0488:RDECAR]2.0.CO;2
Engle, N. L. (2011). Adaptive Capacity and Its Assessment. Global Environ. Change 21, 647–656. doi: 10.1016/j.gloenvcha.2011.01.019
Ewel, J. J., and Hiremath, A. J. (1998). Nutrient Use Efficiency and the Management of Degraded Lands. International Scientific Publications, Ecology Today: An Anthology of Contemporary Ecological Research, 199–215. Available online at: https://people.clas.ufl.edu/jackewel/files/ewel-hiremath-nutrient-use-eco-today-1998.pdf
Fairbairn, M. (2020). Fields of Gold: Financing the Global Land Rush. Ithaca; London: Cornell University Press.
Fairbairn, M., LaChance, J., De Master, K. T., and Ashwood, L. (2021). In vino veritas, in aqua lucrum: Farmland investment, environmental uncertainty, and groundwater access in California's Cuyama Valley. Agric. Hum. Values 38, 285–299. doi: 10.1007/s10460-020-10157-y
FAO (2020). Assessing Food Insecurity: Different Numbers, Different Objectives. Available online at: http://www.fao.org/3/cb0165en/cb0165en.pdf
Faunt, C. C., Sneed, M., Traum, J., and Brandt, J. T. (2016). Water availability and land subsidence in the Central Valley, California, USA. Hydrogeol. J. 24, 675–684. doi: 10.1007/s10040-015-1339-x
Fazey, I., Fazey, J. A., Fischer, J., Sherren, K., Warren, J., Noss, R. F., et al. (2007). Adaptive capacity and learning to learn as leverage for social–ecological resilience. Front. Ecol. Environ. 5, 375–380. doi: 10.1890/1540-9295(2007)5[375:ACALTL]2.0.CO;2
Fitzgerald, D. K. (2008). Every Farm a Factory: The Industrial Ideal in American Agriculture. Yale University Press.
Fleischer, N. L., Tiesman, H. M., Sumitani, J., Mize, T., Amarnath, K. K., Bayakly, A. R., et al. (2013). Public health impact of heat-related illness among migrant farmworkers. Am. J. Prev. Med. 44, 199–206. doi: 10.1016/j.amepre.2012.10.020
Fleischman, L., and Franklin, M. (2017). Fumes Across the Fence-Line: The Health Impacts of Air Pollution From Oil and Gas Facilities on African American Communities. National Association for the Advancement of Colored People, Clean Air Task Force. Available online at: http://arks.princeton.edu/ark:/88435/dsp011z40kw51m
Folke, C. (2006). Resilience: the emergence of a perspective for social–ecological systems analyses. Glob. Environ. Change16, 253–267. doi: 10.1016/j.gloenvcha.2006.04.002
Folke, C., Carpenter, S. R., Walker, B., Scheffer, M., Chapin, T., and Rockström, J. (2010). Resilience thinking: integrating resilience, adaptability and transformability. Ecol. Soc. 15:20. doi: 10.5751/ES-03610-150420
Fortmann, L. (2008). Participatory Research in Conservation and Rural Livelihoods: Doing Science Together. Available online at: https://www.wiley.com/en-us/Participatory+Research+in+Conservation+and+Rural+Livelihoods%3A+Doing+Science+Together-p-9781405176798
Frank, C., Kapfhammer, S., Werber, D., Stark, K., and Held, L. (2008). Cattle density and shiga toxin-producing Escherichia Coli infection in Germany: increased risk for most but not all serogroups. Vecto. Borne Zoonot. Dis. 8, 635–644. doi: 10.1089/vbz.2007.0237
Gabriel, D., Carver, S. J., Durham, H., Kunin, W. E., Palmer, R. C., Sait, S. M., et al. (2009). The spatial aggregation of organic farming in england and its underlying environmental correlates. J. Appl. Ecol. 46, 323–333. doi: 10.1111/j.1365-2664.2009.01624.x
Gallopín, G. C. (2006). Linkages between vulnerability, resilience, and adaptive capacity. Glob. Environ. Change 16, 293–303. doi: 10.1016/j.gloenvcha.2006.02.004
GAO (2017). Food Safety: A National Strategy Is Needed to Address Fragmentation in Federal Oversight, no. GAO-17-74. Available online at: https://www.gao.gov/products/GAO-17-74
Garnett, T., Appleby, M. C., Balmford, A., Bateman, I. J., Benton, T. G., Bloomer, P., et al. (2013). Sustainable intensification in agriculture: premises and policies. Science 341, 33–34. doi: 10.1126/science.1234485
Garone, P. (1998). The tragedy at Kesterson reservoir: a case study in environmental history and a lesson in ecological complexity. Environs Environ. Law Policy J. 22:107.
Garone, P. (2011). The Fall and Rise of the Wetlands of California's Great Central Valley. University of California Press.
Gast, R. K., Guraya, R., Jones, D. R., Anderson, K. E., and Karcher, D. M. (2017). Frequency and duration of fecal shedding of Salmonella enteritidis by experimentally infected laying hens housed in enriched colony cages at different stocking densities. Front. Vet. Sci. 4:20. doi: 10.3389/fvets.2017.00047
Gaudin, A. C. M., Tolhurst, T. N., Ker, A. P., Janovicek, K., Tortora, C., Martin, R. C., et al. (2015). Increasing crop diversity mitigates weather variations and improves yield stability. PLoS ONE 10:e0113261. doi: 10.1371/journal.pone.0113261
Gil, J. D. B., Cohn, A. S., Duncan, J., Newton, P., and Vermeulen, S. (2017). The resilience of integrated agricultural systems to climate change. Wiley Interdisc. Rev. Clim. Change 8:e461. doi: 10.1002/wcc.461
Gilchrist, M. J., Greko, C., Wallinga, D. B., Beran, G. W., Riley, D. G., and Thorne, P. S. (2007). The potential role of concentrated animal feeding operations in infectious disease epidemics and antibiotic resistance. Environ. Health Perspect. 115, 313–316. doi: 10.1289/ehp.8837
Goldbaum, C. (2019, March 18). Trump crackdown unnerves immigrants, and the farmers who rely on them. The New York Times. New York, NY.
Graddy-Lovelace, G., and Diamond, A. (2017). From supply management to agricultural subsidies—and back again? The US farm bill and agrarian (in) viability. J. Rural Stud. 50, 70–83. doi: 10.1016/j.jrurstud.2016.12.007
Grafton, R. Q., Williams, J., Perry, C. J., Molle, F., Ringler, C., Steduto, P., et al. (2018). The paradox of irrigation efficiency. Science 361, 748–750. doi: 10.1126/science.aat9314
Granovetter, M. (2005). The impact of social structure on economic outcomes. J. Econ. Perspect. 19, 33–50. doi: 10.1257/0895330053147958
Gunderson, L. H., and Holling, C. S. (2001). Panarchy: Understanding Transformations in Human and Natural Systems. Washington, DC: Island press.
Guthman, J. (2004). Agrarian Dreams: The Paradox of Organic Farming in California. University of California Press.
Guthman, J. (2019). Wilted. Available online at: https://www.ucpress.edu/book/9780520305281/wilted
Hall, S. J. G. (2018). A novel agroecosystem: beef production in abandoned farmland as a multifunctional alternative to rewilding. Agric. Syst. 167, 10–16. doi: 10.1016/j.agsy.2018.08.009
Halliday, F. W., and Rohr, J. R. (2019). Measuring the shape of the biodiversity-disease relationship across systems reveals new findings and key gaps. Nat. Commun. 10:5032. doi: 10.1038/s41467-019-13049-w
Hallmann, C. A., Sorg, M., Jongejans, E., Siepel, H., Hofland, N., Schwan, H., et al. (2017). More than 75 percent decline over 27 years in total flying insect biomass in protected areas. PLoS ONE 12:e0185809. doi: 10.1371/journal.pone.0185809
Hammond, B., Berardi, G., and Green, R. (2013). Resilience in agriculture: small- and medium-sized farms in Northwest Washington state. Agroecol. Sustain. Food Syst. 37, 316–339. doi: 10.1080/10440046.2012.746251
Haraway, D. (1988). Situated knowledges: the science question in feminism and the privilege of partial perspective. Feminist Stud. 14, 575–599. doi: 10.2307/3178066
Harrison, J. (2006). ‘Accidents’ and invisibilities: scaled discourse and the naturalization of regulatory neglect in California's pesticide drift conflict. Polit. Geogr. 25, 506–529. doi: 10.1016/j.polgeo.2006.02.003
Harrison, J., and Getz, C. (2015). Farm size and job quality: mixed-methods studies of hired farm work in California and Wisconsin. Agric. Hum. Values 32, 617–634. doi: 10.1007/s10460-014-9575-6
Harter, T. (2015). California's agricultural regions gear up to actively manage groundwater use and protection. California Agric. 69, 193–201. doi: 10.3733/ca.E.v069n03p193
Hatfield, J. L., Boote, K. J., Kimball, B. A., Ziska, L. H., Izaurralde, R. C., Ort, D., et al. (2011). Climate impacts on agriculture: implications for crop production. Agron. J. 103, 351–370. doi: 10.2134/agronj2010.0303
Heinberg, R. (2020). Fraying food system may be our next crisis. Resilience. Available online at: https://www.resilience.org/stories/2020-04-23/fraying-food-system-may-be-our-next-crisis/
Hellberg, R. S., and Chu, E. (2015). Effects of climate change on the persistence and dispersal of foodborne bacterial pathogens in the outdoor environment: a review. Crit. Rev. Microbiol. 42, 548–572. doi: 10.3109/1040841X.2014.972335
Helliwell, R. (2018). Where did the marginal land go? Farmers perspectives on marginal land and its implications for adoption of dedicated energy crops. Energy Policy 117, 166–172. doi: 10.1016/j.enpol.2018.03.011
Helmers, G. A., Yamoah, C. F., and Varvel, G. E. (2001). Separating the impacts of crop diversification and rotations on risk. Agron. J. 93, 1337–1340. doi: 10.2134/agronj2001.1337
Hendrickson, M., and Heffernan, W. (2002). Concentration of Agricultural Markets. National Farmers Union.
Hendrickson, M. K. (2015). Resilience in a concentrated and consolidated food system. J. Environ. Stud. Sci. 5, 418–431. doi: 10.1007/s13412-015-0292-2
Henke, C. (2008). Cultivating Science, Harvesting Power: Science and Industrial Agriculture in California. Cambridge, MA: MIT Press.
Heredia, N., and García, S. (2018). Animals as sources of food-borne pathogens: a review. Anim. Nutr. 4, 250–255. doi: 10.1016/j.aninu.2018.04.006
Hertz, T. (2019). U.S. Hired Farm Workforce Is Aging. USDA ERS. Available online at: https://www.ers.usda.gov/amber-waves/2019/may/us-hired-farm-workforce-is-aging/
Hightower, J. (1972). Hard Tomatoes, Hard Times: The Failure of the Land Grant College Complex. Washington, DC: Agribusiness Accountability Project.
Holmes, S. (2013). Fresh Fruit, Broken Bodies: Migrant Farmworkers in the United States. University of California Press.
Holt-Giménez, E. (2002). Measuring farmers' agroecological resistance after Hurricane mitch in Nicaragua: a case study in participatory, sustainable land management impact monitoring. Agric. Ecosyst. Environ. 93, 87–105. doi: 10.1016/S0167-8809(02)00006-3
Holt-Giménez, E. (2006). Campesino a Campesino: Voices from Latin America's Farmer to Farmer Movement for Sustainable Agriculture. Oakland, CA: Food First Books.
Holt-Giménez, E., Shattuck, A., and Van Lammeren, I. (2021). Thresholds of resistance: agroecology, resilience and the agrarian question. J. Peasant Stud. 1–19. doi: 10.1080/03066150.2020.1847090
Hooper, D. U., Chapin, F. S., Ewel, J. J., Hector, A., Inchausti, P., Lavorel, S., et al. (2005). Effects of biodiversity on ecosystem functioning: a consensus of current knowledge. Ecol. Monogr. 75, 3–35. doi: 10.1890/04-0922
Hoover, E. (2017). ‘You can’t say you're sovereign if you can't feed yourself': defining and enacting food sovereignty in american indian community gardening. Am. Indian Cult. Res. J. 41, 31–70. doi: 10.17953/aicrj.41.3.hoover
Horst, M., and Marion, A. (2019). Racial, ethnic and gender inequities in farmland ownership and farming in the U.S. Agric. Hum. Values 36, 1–16. doi: 10.1007/s10460-018-9883-3
Howard, P. H. (2016). Concentration and Power in the Food System: Who Controls What We Eat? Vol. 3. New York, NY: Bloomsbury Publishing.
Howard, P. H. (2020). “How corporations control our seeds,” in Bite Back: People Taking on Corporate Food and Winning, eds K. De Master, and S. Jayaraman (Oakland, CA: University of California, Press) 15.
Howitt, R. E., MacEwan, D., Medellín-Azuara, J., Lund, J. R., and Sumner, A. (2015). Economic Analysis of the 2015 Drought for California Agriculture, 16 Pp. Centre for Watershed Science, University of California, Davis.
Hsiang, S. M. (2010). Temperatures and cyclones strongly associated with economic production in the Caribbean and Central America. Proc. Natl. Acad. Sci. U.S.A. 107, 15367–15372. doi: 10.1073/pnas.1009510107
Hsiang, S. M., Burke, M., and Miguel, E. (2013). Quantifying the influence of climate on human conflict. Science 341:1235367. doi: 10.1126/science.1235367
Hu, H., and Xiong, L. (2014). Genetic engineering and breeding of drought-resistant crops. Annu. Rev. Plant Biol. 65, 715–741. doi: 10.1146/annurev-arplant-050213-040000
Iles, A., and Marsh, R. (2012). Nurturing diversified farming systems in industrialized countries: how public policy can contribute. Ecol. Soc. 17:42. doi: 10.5751/ES-05041-170442
International Panel of Experts on Sustainable Food Systems, (2016). Uniformity to Diversity. Available online at: http://www.ipes-food.org/_img/upload/files/UniformityToDiversity_FULL.pdf (accessed February 4, 2021).
International Panel of Experts on Sustainable Food Systems, (2017). Too Big to Feed, IPES-Food Full Report. Available online at: http://www.ipes-food.org/_img/upload/files/Concentration_FullReport.pdf (accessed February 4, 2021).
International Panel of Experts on Sustainable Food Systems, (2018). Breaking Away from Industrial Food Farming Systems: Seven Case Studies of Agroecological Transition. Available online at: http://www.ipes-food.org/_img/upload/files/CS2_web.pdf (accessed February 4, 2021).
IPBES (2019). Global Assessment Report on Biodiversity and Ecosystem Services of the Intergovernmental Science-Policy Platform on Biodiversity and Ecosystem Services. IPBES Secretariat.
IPCC (2019). Special Report on Climate Change and Land. Available online at: https://www.ipcc.ch/srccl/ (accessed February 4, 2021).
Isbell, F., Calcagno, V., Hector, A., Connolly, J., Harpole, W. S., Reich, P. B., et al. (2011). High plant diversity is needed to maintain ecosystem services. Nature 477, 199–202. doi: 10.1038/nature10282
Isbell, F., Gonzalez, A., Loreau, M., Cowles, J., Díaz, S., Hector, A., et al. (2017). Linking the influence and dependence of people on biodiversity across scales. Nature 546, 65–72. doi: 10.1038/nature22899
Jackson, L. E., Pulleman, M. M., Brussaard, L., Bawa, K. S., Brown, G. G., Cardoso, I. M., et al. (2012). Social-ecological and regional adaptation of agrobiodiversity management across a global set of research regions. Glob. Environ. Chang. 22, 623–639. doi: 10.1016/j.gloenvcha.2012.05.002
Jackson, L. E., Ramirez, I., Yokota, R., Fennimore, S. A., Koike, S. T., Henderson, D. M., et al. (2004). On-farm assessment of organic matter and tillage management on vegetable yield, soil, weeds, pests, and economics in California. Agric. Ecosyst. Environ. 103, 443–463. doi: 10.1016/j.agee.2003.11.013
Jackson, L. E., van Noordwijk, M., Bengtsson, J., Foster, W., Lipper, L., Pulleman, M., et al. (2010). Biodiversity and agricultural sustainagility: from assessment to adaptive management. Curr. Opin. Environ. Sustain. 2, 1–8. doi: 10.1016/j.cosust.2010.02.007
Jackson, M., Ford-Lloyd, B., and Parry, M. (2013). Plant Genetic Resources and Climate Change. CABI.
Jaffee, D. (2014). Brewing Justice: Fair Trade Coffee, Sustainability, and Survival. University of California Press.
Janssen, M. A., Anderies, J. M., and Ostrom, E. (2007). Robustness of social-ecological systems to spatial and temporal variability. Soc. Nat. Resour. 20, 307–322. doi: 10.1080/08941920601161320
Jasanoff, S. (2003). Technologies of humility: citizen participation in governing science. Minerva 41, 223–244. doi: 10.1023/A:1025557512320
Jha, C. K., Gupta, V., Chattopadhyay, U., and Sreeraman, B. A. (2018). Migration as adaptation strategy to cope with climate change: a study of farmers' migration in rural India. Int. J. Clim. Change Strateg. Manag. 10, 121–141. doi: 10.1108/IJCCSM-03-2017-0059
Jones, M. S., Fu, Z., Reganold, J. P., Karp, D. S., Besser, T. E., Tylianakis, J. M., et al. (2019). Organic farming promotes biotic resistance to foodborne human pathogens. J. Appl. Ecol. 56, 1117–1127. doi: 10.1111/1365-2664.13365
Kang, S., Post, W., Wang, D., Nichols, J., Bandaru, V., and West, T. (2013). Hierarchical marginal land assessment for land use planning. Land Use Policy 30, 106–113. doi: 10.1016/j.landusepol.2012.03.002
Karesh, W. B., Dobson, A., Lloyd-Smith, J. O., Lubroth, J., Dixon, M. A., Bennett, M., et al. (2012). Ecology of zoonoses: natural and unnatural histories. Lancet 380, 1936–1945. doi: 10.1016/S0140-6736(12)61678-X
Karlen, D. L., Varvel, G. E., Bullock, D. G., and Cruse, R. M. (1994). Crop rotations for the 21st century. Adv. Agron. 53, 1–45. doi: 10.1016/S0065-2113(08)60611-2
Karp, D. S., Baur, P., Atwill, E. R., De Master, K., Gennet, S., Iles, A., et al. (2015a). The in unintended ecological and social impacts of food safety regulations California's Central Coast Region. Bioscience 65, 1173–1183. doi: 10.1093/biosci/biv152
Karp, D. S., Gennet, S., Kilonzo, C., Partyka, M., Chaumont, N., Atwill, E. R., et al. (2015b). Comanaging fresh produce for nature conservation and food safety. Proc. Natl. Acad. Sci. U.S.A. 112, 11126–11131. doi: 10.1073/pnas.1508435112
Karp, D. S., Moses, R., Gennet, S., Jones, M. S., Joseph, S., M'Gonigle, L. K., et al. (2016). Agricultural practices for food safety threaten pest control services for fresh produce. J. Appl. Ecol. 53, 1402–1412. doi: 10.1111/1365-2664.12707
Keesing, F., Belden, L. K., Daszak, P., Dobson, A., Harvell, C. D., Holt, R. D., et al. (2010). Impacts of biodiversity on the emergence and transmission of infectious diseases. Nature 468, 647–652. doi: 10.1038/nature09575
Khoury, C. K., Bjorkman, A. D., Dempewolf, H., Ramirez-Villegas, J., Guarino, L., Jarvis, A., et al. (2014). Increasing homogeneity in global food supplies and the implications for food security. Proc. Natl. Acad. Sci. U.S.A. 111, 4001–4006. doi: 10.1073/pnas.1313490111
Kitroeff, N., and Mohan, G. (2017). Wages rise on california farms. americans still don't want the job. Los Angeles Times.
Kjellstrom, T., Holmer, I., and Lemke, B. (2009). Workplace heat stress, health and productivity – an increasing challenge for low and middle-income countries during climate change. Glob. Health Action 2:2047. doi: 10.3402/gha.v2i0.2047
Kleijn, D., Winfree, R., Bartomeus, I., Carvalheiro, L. G., Henry, M., Isaacs, R., et al. (2015). Delivery of crop pollination services is an insufficient argument for wild pollinator conservation. Nat. Commun. 6:7414. doi: 10.1038/ncomms8414
Kloppenburg, J. R. (2005). First the Seed: The Political Economy of Plant Biotechnology. University of Wisconsin Press.
Koide, R. T., Nguyen, B. T., Skinner, R. H., Dell, C. J., Adler, P. R., Drohan, P. J., et al. (2018). Comparing biochar application methods for switchgrass yield and C sequestration on contrasting marginal lands in Pennsylvania, USA. Bioenergy Res. 11, 784–802. doi: 10.1007/s12155-018-9940-1
Kremen, C. (2005). Managing ecosystem services: what do we need to know about their ecology?: Ecology of ecosystem services. Ecol. Lett. 8, 468–479. doi: 10.1111/j.1461-0248.2005.00751.x
Kremen, C., Iles, A., and Bacon, C. (2012). Diversified farming systems: an agroecological, systems-based alternative to modern industrial agriculture. Ecol. Soc. 17:44. doi: 10.5751/ES-05103-170444
Kremen, C., and Merenlender, A. M. (2018). Landscapes that work for biodiversity and people. Science 362:aau6020. doi: 10.1126/science.aau6020
Kumar, N., Harris, J., and Rawat, R. (2015). If they grow it, will they eat and grow? Evidence from Zambia on agricultural diversity and child undernutrition. J. Dev. Stud. 51, 1060–1077. doi: 10.1080/00220388.2015.1018901
Lake, I. R., and Barker, G. C. (2018). Climate change, foodborne pathogens and illness in higher-income countries. Curr. Environ. Health Rep. 5, 187–196. doi: 10.1007/s40572-018-0189-9
Laliberté, E., Wells, J. A., DeClerck, F., Metcalfe, D. J., Catterall, C. P., Queiroz, C., et al. (2010). Land-use intensification reduces functional redundancy and response diversity in plant communities. Ecol. Lett. 13, 76–86. doi: 10.1111/j.1461-0248.2009.01403.x
Leonhardt, H., Braito, M., and Penker, M. (2021). Why do farmers care about rented land? Investigating the context of farmland tenure. J. Soil Water Conserv. 76, 89–102. doi: 10.2489/jswc.2021.00191
Li, M., Baker, C. A., Danyluk, M. D., Belanger, P., Boelaert, F., Cressey, P., et al. (2018). Identification of biological hazards in produce consumed in industrialized countries: a review. J. Food Prot. 81, 1171–1186. doi: 10.4315/0362-028X.JFP-17-465
Liebman, M., and Schulte, L. A. (2015). Enhancing agroecosystem performance and resilience through increased diversification of landscapes and cropping systems. Elementa Sci. Anthropocene 3, 1–7. doi: 10.12952/journal.elementa.000041
Lin, B. B. (2011). Resilience in agriculture through crop diversification: adaptive management for environmental change. Bioscience 61, 183–193. doi: 10.1525/bio.2011.61.3.4
Lipper, L., Thornton, P., Campbell, B. M., Baedeker, T., Braimoh, A., Bwalya, M., et al. (2014). Climate-smart agriculture for food security. Nat. Clim. Change 4, 1068–1072. doi: 10.1038/nclimate2437
Liverman, D. (2015). “Reading climate change and climate governance as political ecologies,” in: The Routledge Handbook of Political Ecology, eds G. Bridge, T. Perreault, and J. McCarthy (New York, NY: Routledge), 303–319.
Lobell, D. B., Deines, J. M., and Tommaso, S. D. (2020). Changes in the drought sensitivity of US maize yields. Nature Food 1, 729–735. doi: 10.1038/s43016-020-00165-w
Lobell, D. B., Roberts, M. J., Schlenker, W., Braun, N., Little, B. B., Rejesus, R. M., et al. (2014). Greater sensitivity to drought accompanies maize yield increase in the U.S. Midwest. Science 344, 516–519. doi: 10.1126/science.1251423
Lotter, D. W., Seidel, R., and Liebhardt, W. (2003). The performance of organic and conventional cropping systems in an extreme climate year. Am. J. Altern. Agric. 18, 146–154. doi: 10.1079/AJAA200345
Lytton, T. D. (2019). Outbreak: Foodborne Illness and the Struggle for Food Safety. University of Chicago Press.
Ma, S., Swinton, S. M., Lupi, F., and Jolejole-Foreman, C. (2012). Farmers' willingness to participate in payment-for-environmental-services programmes. J. Agric. Econ. 63, 604–626. doi: 10.1111/j.1477-9552.2012.00358.x
Mader, P. (2002). Soil fertility and biodiversity in organic farming. Science 296, 1694–1697. doi: 10.1126/science.1071148
Maier, L., and Shobayashi, M. (2001). Multifunctionality: Towards an Analytical Framework. Organization for Economic.
Malin, S., and De Master, K. T. (2016). A devil's bargain: rural environmental injustices and hydraulic fracturing on Pennsylvania's farms. J. Rural Stud. 47, 278–290. doi: 10.1016/j.jrurstud.2015.12.015
Marriott, E. E., and Wander, M. M. (2006). Total and labile soil organic matter in organic and conventional farming systems. Soil Sci. Soc. Am. J. 70, 950–959. doi: 10.2136/sssaj2005.0241
Marshall, K. E., Hexemer, A., Seelman, S. L., Fatica, M. K., Blessington, T., Hajmeer, M., et al. (2020). Lessons learned from a decade of investigations of shiga toxin-producing Escherichia coli outbreaks linked to leafy greens, United States and Canada. Emerging Infect. Dis. 26, 2319–2328. doi: 10.3201/eid2610.191418
Martin, P., Hooker, B., Akhtar, M., and Stockton, M. (2016). How many workers are employed in California agriculture? California Agric. 71, 30–34. doi: 10.3733/ca.2016a0011
Martin, P., Hooker, B., and Stockton, M. (2017). Employment and earnings of California farmworkers in 2015. California Agric. 72, 107–113. doi: 10.3733/ca.2017a0043
McDaniel, M. D., Tiemann, L. K., and Grandy, A. S. (2014). Does agricultural crop diversity enhance soil microbial biomass and organic matter dynamics? A meta-analysis. Ecol. Appl. 24, 560–570. doi: 10.1890/13-0616.1
McIntyre, B. D., Herren, H. R., Wakhungu, J., and Watson, R. T. (2010). Agriculture at a crossroads: synthesis report of the International Assessment of Agricultural Knowledge, Science, and Technology for Development (IAASTD). Exp. Agric. 46, 115–16. doi: 10.1017/S0014479709990676
McLeman, R. A., and Hunter, L. M. (2010). Migration in the context of vulnerability and adaptation to climate change: insights from analogues. Wiley Interdisc. Rev. Clim. Change 1, 450–461. doi: 10.1002/wcc.51
McMahon, M. (2013). What food is to be kept safe and for whom? Food-safety governance in an unsafe food system. Laws 2, 401–427. doi: 10.3390/laws2040401
McMichael, P. (2012). The land grab and corporate food regime restructuring. J. Peasant Stud. 39, 681–701. doi: 10.1080/03066150.2012.661369
McNeely, J. A., and Schroth, G. (2006). Agroforestry and biodiversity conservation – traditional practices, present dynamics, and lessons for the future. Biodivers. Conserv. 15, 549–554. doi: 10.1007/s10531-005-2087-3
Méndez, V. E., Bacon, C. M., and Cohen, R. (2013). Agroecology as a transdisciplinary, participatory and action-oriented approach. Agroecol. Sustain. Food Syst. 37, 3–18. doi: 10.1080/10440046.2012.736926
Mendoza, H., Rubio, A. V., García-Peña, G. E., Suzán, G., and Simonetti, J. A. (2019). does land-use change increase the abundance of zoonotic reservoirs? Rodents Say Yes. Eur. J. Wildl. Res. 66:6. doi: 10.1007/s10344-019-1344-9
Merckx, T., and Pereira, H. M. (2015). Reshaping agri-environmental subsidies: from marginal farming to large-scale rewilding. Basic Appl. Ecol. 16, 95–103. doi: 10.1016/j.baae.2014.12.003
Miles, A., DeLonge, M. S., and Carlisle, L. (2017). Triggering a positive research and policy feedback cycle to support a transition to agroecology and sustainable food systems. Agroecol. Sustain. Food Syst. 41, 855–879. doi: 10.1080/21683565.2017.1331179
Minkoff-Zern, L.-A. (2018). Race, immigration and the agrarian question: farmworkers becoming farmers in the United States. J. Peasant Stud. 45, 389–408. doi: 10.1080/03066150.2017.1293661
Minkoff-Zern, L.-A. (2019). The New American Farmer: Immigration, Race, and the Struggle for Sustainability. Cambridge, MA: MIT Press.
Mitchell, D. (1996). The Lie of the Land. University of Minnesota Press. Available online at: https://www.upress.umn.edu/book-division/books/the-lie-of-the-land
Montenegro de Wit, M. (2017a). Beating the bounds: how does ‘open source’ become a seed commons? J. Peasant Stud. 46, 44–79. doi: 10.1080/03066150.2017.1383395
Montenegro de Wit, M. (2017b). Stealing into the wild: conservation science, plant breeding and the makings of new seed enclosures. J. Peasant Stud. 44, 169–212. doi: 10.1080/03066150.2016.1168405
Montenegro de Wit, M., and Iles, A. (2016). Toward thick legitimacy: creating a web of legitimacy for agroecology. Elem. Sci. Anth. 4, 115–139. doi: 10.12952/journal.elementa.000115
Morris, C. (2017). California Crops Rot as Immigration Crackdown Creates Farmworker Shortage. Fortune.
Mortensen, D. A., and Smith, R. G. (2020). Confronting barriers to cropping system diversification. Front. Sustain. Food Syst. 4:564197. doi: 10.3389/fsufs.2020.564197
Morton, J. F. (2007). The impact of climate change on smallholder and subsistence agriculture. Proc. Natl. Acad. Sci. U.S.A. 104, 19680–19685. doi: 10.1073/pnas.0701855104
Naranjo, S. (2012). Enabling food sovereignty and a prosperous future for peasants by understanding the factors that marginalise peasants and lead to poverty and hunger. Agric. Human Values 29, 231–246. doi: 10.1007/s10460-011-9345-7
Nawrotzki, R. J., DeWaard, J., Bakhtsiyarava, M., and Ha, J. T. (2017). Climate shocks and rural-urban migration in mexico: exploring nonlinearities and thresholds. Clim. Change 140, 243–258. doi: 10.1007/s10584-016-1849-0
O'Brien, P. L., Hatfield, J. L., Dold, C., Kistner-Thomas, E. J., and Wacha, K. M. (2020). Cropping pattern changes diminish agroecosystem services in North and South Dakota, USA. Agron. J. 112, 1–24. doi: 10.1002/agj2.20001
Ohlendorf, H. M. (1989). “Bioaccumulation and effects of selenium in wildlife,” in Selenium in Agriculture and the Environment, ed L. W. Jacobs (Hoboken, NJ: John Wiley and Sons, Ltd), 133–177.
Olimpi, E. M., Baur, P., Echeverri, A., Gonthier, D., Karp, D. S., Kremen, C., et al. (2019). Evolving food safety pressures in California's central coast region. Front. Sustain. Food Syst. 3:102. doi: 10.3389/fsufs.2019.00102
Ortiz-Bobea, A., Knippenberg, E., and Chambers, R. G. (2018). Growing climatic sensitivity of U.S. agriculture linked to technological change and regional specialization. Sci. Adv. 4:eaat4343. doi: 10.1126/sciadv.aat4343
Ortiz-Bobea, A., and Tack, J. (2018). Is another genetic revolution needed to offset climate change impacts for us maize yields? Environ. Res. Lett. 13:124009. doi: 10.1088/1748-9326/aae9b8
Ostfeld, R. S., and Keesing, F. (2012). Effects of host diversity on infectious disease. Annu. Rev. Ecol. Evol. Syst. 43, 157–182. doi: 10.1146/annurev-ecolsys-102710-145022
Oyarzun, P. J., Borja, R. M., Sherwood, S., and Parra, V. (2013). Making sense of agrobiodiversity, diet, and intensification of smallholder family farming in the Highland Andes of Ecuador. Ecol. Food Nutr. 52, 515–541. doi: 10.1080/03670244.2013.769099
Patel, R., and Moore, J. W. (2017). A History of the World in Seven Cheap Things: A Guide to Capitalism, Nature, and the Future of the Planet. Oakland, CA: University of California Press.
Pathak, T. B., Maskey, M. L., Dahlberg, J. A., Kearns, F., Bali, K. M., and Zaccaria, D. (2018). Climate change trends and impacts on California agriculture: a detailed review. Agronomy 8:25. doi: 10.3390/agronomy8030025
Peter, B. G., Mungai, L. M., Messina, J. P., and Snapp, S. S. (2017). Nature-based agricultural solutions: scaling perennial grains across Africa. Environ. Res. 159, 283–290. doi: 10.1016/j.envres.2017.08.011
Petrzelka, P., Ma, Z., and Malin, S. (2013). The elephant in the room: absentee landowner issues in conservation and land management. Land Use Policy 30, 157–166. doi: 10.1016/j.landusepol.2012.03.015
Pfeffer, M. (1983). Social Origins of Three Systems of Farm Production in the United States - ProQuest. Available online at: https://search.proquest.com/openview/5d485d7c526f24e915c11fbbf2f863e6/
Philpott, T. (2020). Perilous Bounty: The Looming Collapse of American Farming and How We Can Prevent It. Bloomsbury Publishing.
Plieninger, T., Ferranto, S., Huntsinger, L., Kelly, M., and Getz, C. (2012). Appreciation, use, and management of biodiversity and ecosystem services in California's working landscapes. Environ. Manage 50, 427–440. doi: 10.1007/s00267-012-9900-z
Poulsen, M. N., Pollak, J., Sills, D. L., Casey, J. A., Rasmussen, S. G., Nachman, K. E., et al. (2018). Residential proximity to high-density poultry operations associated with campylobacteriosis and infectious diarrhea. Int. J. Hyg. Environ. Health 221, 323–333. doi: 10.1016/j.ijheh.2017.12.005
Preiser, R., Biggs, R., De Vos, A., and Folke, C. (2018). Social-ecological systems as complex adaptive systems. Ecol. Soc. 23:46. doi: 10.5751/ES-10558-230446
Pretty, J. (2018). Intensification for redesigned and sustainable agricultural systems. Science 362:eaav0294. doi: 10.1126/science.aav0294
Price, B. (2019). The Political Economy of Mechanization in U.S. Agriculture. New York, NY: CRC Press.
Rahman, M. T., Sobur, M. A., Islam, M. S., Ievy, S., Hossain, M. J., El Zowalaty, M. E., et al. (2020). Zoonotic diseases: etiology, impact, and control. Microorganisms 8:1405. doi: 10.3390/microorganisms8091405
Ransom, E., DuPuis, M., and Worosz, M. R. (2020). Why Farmers Are Dumping Milk Down the Drain and Letting Produce Rot in Fields. The Conversation. (New York, NY: Routledge).
Reed, M. S., and Stringer, L. (2016). Land Degradation, Desertification and Climate Change. New York, NY: Routledge.
Reich, P. B., Tilman, D., Isbell, F., Mueller, K., Hobbie, S. E., Flynn, D. F. B., et al. (2012). Impacts of biodiversity loss escalate through time as redundancy fades. Science 336, 589–592. doi: 10.1126/science.1217909
Renard, D., and Tilman, D. (2019). National food production stabilized by crop diversity. Nature 571, 257–260. doi: 10.1038/s41586-019-1316-y
Renting, H., Rossing, W. A. H., Groot, J. C. J., Van der Ploeg, J. D., Laurent, C., Perraud, D., et al. (2009). Exploring multifunctional agriculture. A review of conceptual approaches and prospects for an integrative transitional framework. J. Environ. Manag. Multifunct. Agric. 90, S112–S123. doi: 10.1016/j.jenvman.2008.11.014
Renwick, L. L. R., Kimaro, A. A., Hafner, J. M., Rosenstock, T. S., Gaudin, A. C. M., and Ahmed, S. (2020). Maize-pigeonpea intercropping outperforms monocultures under drought. Front. Sustain. Food Syst. 4. doi: 10.3389/fsufs.2020.562663
Ribaudo, M., Greene, C., Hansen, L., and Hellerstein, D. (2010). Ecosystem services from agriculture: steps for expanding markets. Ecol. Econ. 69, 2085–2092. doi: 10.1016/j.ecolecon.2010.02.004
Ribot, J. C., and Peluso, N. L. (2003). A theory of access*. Rural Sociol. 68, 153–181. doi: 10.1111/j.1549-0831.2003.tb00133.x
Rockström, J., Williams, J., Daily, G., Noble, A., Matthews, N., Gordon, L., et al. (2017). Sustainable intensification of agriculture for human prosperity and global sustainability. Ambio 46, 4–17. doi: 10.1007/s13280-016-0793-6
Roesch-McNally, G. E., Arbuckle, J. G., and Tyndall, J. C. (2018). Barriers to implementing climate resilient agricultural strategies: the case of crop diversification in the US corn belt. Glob. Environ. Change 48, 206–215. doi: 10.1016/j.gloenvcha.2017.12.002
Rois-Díaz, M., Lovric, N., Lovric, M., Ferreiro-Domínguez, N., Mosquera-Losada, M. R., den Herder, M., et al. (2018). Farmers' reasoning behind the uptake of agroforestry practices: evidence from multiple case-studies across Europe. Agrofor. Syst. 92, 811–828. doi: 10.1007/s10457-017-0139-9
Rosa-Schleich, J., Loos, J., Mußhoff, O., and Tscharntke, T. (2019). Ecological-economic trade-offs of diversified farming systems – a review. Ecol. Econ. 160, 251–263. doi: 10.1016/j.ecolecon.2019.03.002
Schmidt, J. E., Peterson, C., Wang, D., Scow, K. M., and Gaudin, A. C. M. (2018). Agroecosystem tradeoffs associated with conversion to subsurface drip irrigation in organic systems. Agric. Water Manag. 202, 1–8. doi: 10.1016/j.agwat.2018.02.005
Schmitz, A., and Moss, C. B. (2015). Mechanized Agriculture: Machine Adoption, Farm Size, and Labor Displacement. Available online at: https://mospace.umsystem.edu/xmlui/handle/10355/48143
Sellers, L. A., Long, R. F., Jay-Russell, M. T., Li, X., Atwill, E. R., Engeman, R. M., et al. (2018). Impact of field-edge habitat on mammalian wildlife abundance, distribution, and vectored foodborne pathogens in adjacent crops. Crop Protect. 108, 1–11. doi: 10.1016/j.cropro.2018.02.005
Sharma, H. R. (2011). Crop diversification in himachal pradesh: patterns, determinants and challenges. Indian J. Agric. Econ. 66, 97–114. doi: 10.22004/ag.econ.204738
Shava, S., Krasny, M. E., Tidball, K. G., and Zazu, C. (2010). Agricultural knowledge in urban and resettled communities: applications to social–ecological resilience and environmental education. Environ. Educ. Res. 16, 575–589. doi: 10.1080/13504622.2010.505436
Shreck, A., Getz, C., and Feenstra, G. (2006). Social sustainability, farm labor, and organic agriculture: findings from an exploratory analysis. Agric. Hum. Values 23, 439–449. doi: 10.1007/s10460-006-9016-2
Sibhatu, K. T., Krishna, V. V., and Qaim, M. (2015). Production diversity and dietary diversity in smallholder farm households. Proc. Natl. Acad. Sci. U.S.A. 112, 10657–10662. doi: 10.1073/pnas.1510982112
Smit, B., and Wandel, J. (2006). Adaptation, adaptive capacity and vulnerability. Glob. Environ. Change 16, 282–292. doi: 10.1016/j.gloenvcha.2006.03.008
Sobels, J., Curtis, A., and Lockie, S. (2001). The role of landcare group networks in rural Australia: exploring the contribution of social capital. J. Rural Stud. 17, 265–276. doi: 10.1016/S0743-0167(01)00003-1
Sofos, J. N. (2008). Challenges to meat safety in the 21st century. Meat Sci. 78, 3–13. doi: 10.1016/j.meatsci.2007.07.027
Solorio, S. F. J., Wright, J., Franco, M. J. A., Basu, S. K., Sarabia, S. L., Ramírez, L., et al. (2017). “Silvopastoral systems: best agroecological practice for resilient production systems under dryland and drought conditions,” in Quantification of Climate Variability, Adaptation and Mitigation for Agricultural Sustainability, eds M. Ahmed and C. O. Stockle (Cham: Springer International Publishing), 233–250.
Speakman, D. (2018). Growing at the margins: adaptation to severe weather in the marginal lands of the British Isles. Weather Clim. Soc. 10, 121–136. doi: 10.1175/WCAS-D-16-0113.1
Sreenviasan, H. (2020). How women in Iowa conservation are leading farmland efforts. PBS NewsHour. Available online at: https://www.pbs.org/newshour/show/how-women-in-iowa-are-leading-farmland-conservation-efforts
Steffen, W., Rockström, J., Richardson, K., Lenton, T. M., Folke, C., Liverman, D., et al. (2018). Trajectories of the earth system in the anthropocene. Proc. Natl. Acad. Sci. U.S.A. 115, 8252–8259. doi: 10.1073/pnas.1810141115
Stoecklin-Marois, M., Hennessy-Burt, T., Mitchell, D., and Schenker, M. (2013). Heat-related illness knowledge and practices among california hired farm workers in THE MICASA study. Ind. Health 51, 47–55. doi: 10.2486/indhealth.2012-0128
Stuart, D. (2009). Constrained choice and ethical dilemmas in land management: environmental quality and food safety in California agriculture. J. Agric. Environ. Ethics 22, 53–71. doi: 10.1007/s10806-008-9129-2
Stuart, D., and Gillon, S. (2013). Scaling up to address new challenges to conservation on US farmland. Land Use Policy 31, 223–236. doi: 10.1016/j.landusepol.2012.07.003
Stuart, D., and Worosz, M. R. (2012). Risk, anti-reflexivity, and ethical neutralization in industrial food processing. Agric. Hum. Values 29, 287–301. doi: 10.1007/s10460-011-9337-7
Swift, M. J., Izac, A.-M. N., and van Noordwijk, M. (2004). Biodiversity and ecosystem services in agricultural landscapes—are we asking the right questions? Agric. Ecosyst. Environ. 104, 113–134. doi: 10.1016/j.agee.2004.01.013
Tigchelaar, M., Battisti, D. S., Naylor, R. L., and Ray, D. K. (2018). Future warming increases probability of globally synchronized maize production shocks. Proc. Natl. Acad. Sci. U.S.A. 115, 6644–6649. doi: 10.1073/pnas.1718031115
Timmermann, C., and Félix, G. F. (2015). Agroecology as a VEHICLE for contributive justice. Agric. Hum. Values 32, 523–538. doi: 10.1007/s10460-014-9581-8
Tirado, M. C., Clarke, R., Jaykus, L. A., McQuatters-Gollop, A., and Frank, J. M. (2010). Climate change and food safety: a review. Food Res. Int. 43, 1745–1765. doi: 10.1016/j.foodres.2010.07.003
Tomich, T. P., Brodt, S., Ferris, H., Galt, R., Horwath, W. R., Kebreab, E., et al. (2011). Agroecology: a review from a global-change perspective. Annu. Rev. Environ. Resour. 36, 193–222. doi: 10.1146/annurev-environ-012110-121302
Trauger, A. (2014). Toward a political geography of food sovereignty: transforming territory, exchange and power in the liberal sovereign state. J. Peasant Stud. 41, 1131–1152. doi: 10.1080/03066150.2014.937339
Trenberth, K. E., Dai, A., van der Schrier, G., Jones, P. D., Barichivich, J., Briffa, K. R., et al. (2014). Global warming and changes in drought. Nat. Clim. Change 4, 17–22. doi: 10.1038/nclimate2067
Turner, K., Moua, C. N., Hajmeer, M., Barnes, A., and Needham, M. (2019). Overview of leafy greens–related food safety incidents with a California link: 1996 to 2016. J. Food Prot. 82, 405–414. doi: 10.4315/0362-028X
USDA Census (2017). USDA Census Full Report. Available online at: https://www.nass.usda.gov/Publications/AgCensus/2017/index.php#full_report
Valcour, J. E. (2002). Associations between indicators of livestock farming intensity and incidence of human shiga toxin-producing Escherichia coli infection. Emerg. Infect. Dis. 8, 252–257. doi: 10.3201/eid0803.010159
Van Huylenbroeck, G., Vandermeulen, V., Mettepenningen, E., and Verspecht, A. (2007). Multifunctionality of agriculture: a review of and definitions, evidence instruments. Liv. Rev. Landsc. Res. 1:3. doi: 10.12942/lrlr-2007-3
Van Noordwijk, M., Speelman, E., Hofstede, G. J., et al. (2020). Sustainable agroforestry landscape management. Land 9, 1–38. doi: 10.3390/land9080243
Vandermeer, J., and Perfecto, I. (2017). Ecological Complexity and Agroecology. 1st ed. Abingdon; Oxon; New York, NY: Routledge.
Vandermeer, J., van Noordwijk, M., Anderson, J., Ong, C., and Perfecto, I. (1998). Global change and multi-species agroecosystems: concepts and issues. Agric. Ecosyst. Environ. 67, 1–22. doi: 10.1016/S0167-8809(97)00150-3
Verbruggen, P. (2017). “Hybridization of food governance: an analytical framework,” in Hybridization of Food Governance, eds P. Verbruggen and T. Havinga (Northampton, MA: Edward Elgar Publishing), 1–28.
Veteto, J. R., and Carlson, S. B. (2014). Climate change and apple diversity: local perceptions from appalachian North Carolina. J. Ethnobiol. 34, 359–382. doi: 10.2993/0278-0771-34.3.359
Vries, M., de van Middelaar, C. E., and de Boer, I. J. M. (2015). Comparing environmental impacts of beef production systems: a review of life cycle assessments. Livest. Sci. 178, 279–288. doi: 10.1016/j.livsci.2015.06.020
Wagner, D. L. (2020). Insect declines in the anthropocene. Annu. Rev. Entomol. 65, 457–480. doi: 10.1146/annurev-ento-011019-025151
Walker, B., Holling, C. S., Carpenter, S. R., and Kinzig, A. (2004). Resilience, adaptability and transformability in social–ecological systems. Ecol. Soc. 9:5. doi: 10.5751/ES-00650-090205
Walker, R. (2004). The Conquest of Bread: 150 Years of California Agribusiness. London and New York: New Press.
Weiler, A. M., Otero, G., and Wittman, H. (2016). Rock stars and bad apples: moral economies of alternative food networks and precarious farm work regimes. Antipode 48, 1140–1162. doi: 10.1111/anti.12221
Wells, B., and Eells, J. (2011). One size does not fit all: customizing conservation to a changing demographic. J. Soil Water Conserv. 66, 136A−139A. doi: 10.2489/jswc.66.5.136A
Wells, G. J., Stuart, N., Furley, P. A., and Ryan, C. M. (2018). Ecosystem service analysis in marginal agricultural lands: a case study in Belize. Ecosyst. Serv. 32, 70–77. doi: 10.1016/j.ecoser.2018.06.002
Wezel, A., Herren, B. G., Kerr, R. B., Barrios, E., Gonçalves, A. L. R., and Sinclair, F. (2020). Agroecological principles and elements and their implications for transitioning to sustainable food systems. A review. Agron. Sustain. Dev. 40. doi: 10.1007/s13593-020-00646-z
White, M. (2017). Collective agency and community resilience: a theoretical framework to understand agricultural resistance. J. Agric. Food Syst. Commun. Dev. 4, 17–21. doi: 10.5304/jafscd.2017.074.014
White, M. (2018). Freedom Farmers: Agricultural Resistance and the Black Freedom Movement. Chapel Hill, NC: UNC Press Books.
White, S. S., and Selfa, T. (2013). Shifting lands: exploring kansas farmer decision-making in an era of climate change and biofuels production. Environ. Manage 51, 379–391. doi: 10.1007/s00267-012-9991-6
Wittman, H. (2011). Food sovereignty: a new rights framework for food and nature? Environ. Soc. 2, 87–105. doi: 10.3167/ares.2011.020106
Wyborn, C., Yung, L., Murphy, D., and Williams, D. (2015). Situating adaptation: how governance challenges and perceptions of uncertainty influence adaptation in the Rocky Mountains. Reg. Environ. Change 15, 669–682. doi: 10.1007/s10113-014-0663-3
Yaffe-Bellany, D., and Corkery, M. (2020, April 11). Dumped milk, smashed eggs, plowed vegetables: food waste of the pandemic. The New York Times. Business.
Yung, L., and Belsky, J. M. (2007). Private property rights and community goods: negotiating landowner cooperation amid changing ownership on the rocky mountain front. Soc. Nat. Resour. 20, 689–703. doi: 10.1080/08941920701216586
Zanten, H. H. E., van Mollenhorst, H., Klootwijk, C. W., van Middelaar, C. E., and de Boer, I. J. M. (2016). Global food supply: land use efficiency of livestock systems. Int. J. Life Cycle Assess. 21, 747–758. doi: 10.1007/s11367-015-0944-1
Zhang, W., Ricketts, T. H., Kremen, C., Carney, K., and Swinton, S. M. (2007). Ecosystem services and dis-services to agriculture. Ecol. Econ. 64, 253–260. doi: 10.1016/j.ecolecon.2007.02.024
Keywords: diversified farming systems, marginal land, land access, farm labor, food safety, drought, adaptive capacity, equity
Citation: Petersen-Rockney M, Baur P, Guzman A, Bender SF, Calo A, Castillo F, De Master K, Dumont A, Esquivel K, Kremen C, LaChance J, Mooshammer M, Ory J, Price MJ, Socolar Y, Stanley P, Iles A and Bowles T (2021) Narrow and Brittle or Broad and Nimble? Comparing Adaptive Capacity in Simplifying and Diversifying Farming Systems. Front. Sustain. Food Syst. 5:564900. doi: 10.3389/fsufs.2021.564900
Received: 22 May 2020; Accepted: 11 February 2021;
Published: 15 March 2021.
Edited by:
Stacy Michelle Philpott, University of California, Santa Cruz, United StatesReviewed by:
Celia A. Harvey, Monteverde Institute, Costa RicaMeine van Noordwijk, World Agroforestry Centre (ICRAF), Indonesia
Copyright © 2021 Petersen-Rockney, Baur, Guzman, Bender, Calo, Castillo, De Master, Dumont, Esquivel, Kremen, LaChance, Mooshammer, Ory, Price, Socolar, Stanley, Iles and Bowles. This is an open-access article distributed under the terms of the Creative Commons Attribution License (CC BY). The use, distribution or reproduction in other forums is permitted, provided the original author(s) and the copyright owner(s) are credited and that the original publication in this journal is cited, in accordance with accepted academic practice. No use, distribution or reproduction is permitted which does not comply with these terms.
*Correspondence: Margiana Petersen-Rockney, margiana@berkeley.edu
†Co-lead authors