- 1Department of Entomology, College of Agriculture, Purdue University, West Lafayette, IN, United States
- 2Department of Entomology, College of Agricultural Sciences, The Pennsylvania State University, University Park, PA, United States
For most of the last two decades, insect pest management in key grain and oilseed crops has relied heavily on an insurance-based approach. This approach mandates a suite of management tactics prior to planting and in the absence of pest data. Because there is little flexibility for using these tactics individually, most producers have adopted this full suite of practices despite mounting evidence that some components do not provide consistent benefits. In North America in particular, this preventive approach to insect pest management has led to steep increases in use of neonicotinoid insecticides and subsequent increases in neonicotinoids in soil and water within crop fields and beyond. These increases have been accompanied by a host of non-target effects that have been most clearly studied in pollinators and insect natural enemies. Less attention has been given to the effects of this practice upon the many thousands of aquatic insect species that are often cryptic and offer negligible, or undefined, clear benefits to humans and their commerce. A survey of the literature reveals that the non-target effects of neonicotinoids upon these aquatic species are often as serious as for terrestrial species, and more difficult to address. By focusing upon charismatic insect species that provide clearly defined services, we are likely dramatically under-estimating the effects of neonicotinoids upon the wider environment. Given the mounting evidence base demonstrating that the pest management and crop yield benefits of this approach are negligible, we advocate for a return to largely-abandoned IPM principles as a readily accessible alternative path.
Introduction
Insect pest management using chemical insecticides predates the industrial revolution, with uses of lead arsenate and Paris Green (Pedigo and Rice, 2014) being early examples of invaluable, if unsophisticated and heavy-handed, approaches to keep insect pests at bay. While our arsenal of pest management chemistries has been refined over time, the vast majority of our chemical insecticides are still non-specific, with broad activity on a wide range of target and non-target organisms. Some classes of insecticides have emerged that are more selective (e.g., insect-growth regulators, Bt toxins), but the expectation of “selective insecticides” that was articulated in one of the original descriptions of IPM (Stern et al., 1959) largely remains elusive in modern pest management. Given our current insecticide options, it remains a challenge to strike the delicate balance between safeguarding food production and human health while avoiding deleterious effects upon the wider environment—these are commonly grouped under the inclusive term “non-target effects.” The goal of this paper is to consider whether current agricultural use of neonicotinoids, the most popular class of insecticides in the world, is achieving this balance. For this assessment, we focus primarily on U.S. agriculture where most of the principal crops are routinely grown from neonicotinoid-treated seed. We review neonicotinoid use patterns, their potential for effective pest management and subsequent yield benefits. We then discuss the potential for non-target effects. Because neonicotinoids are highly water soluble, aquatic environments have proven to be an environmental sink for these compounds (Figure 1); we discuss some of the observed non-target impacts of neonicotinoids upon aquatic communities, and whether these negative effects matter in a broader context. Finally, we outline remedial steps for how the situation can be improved in the short and longer term.
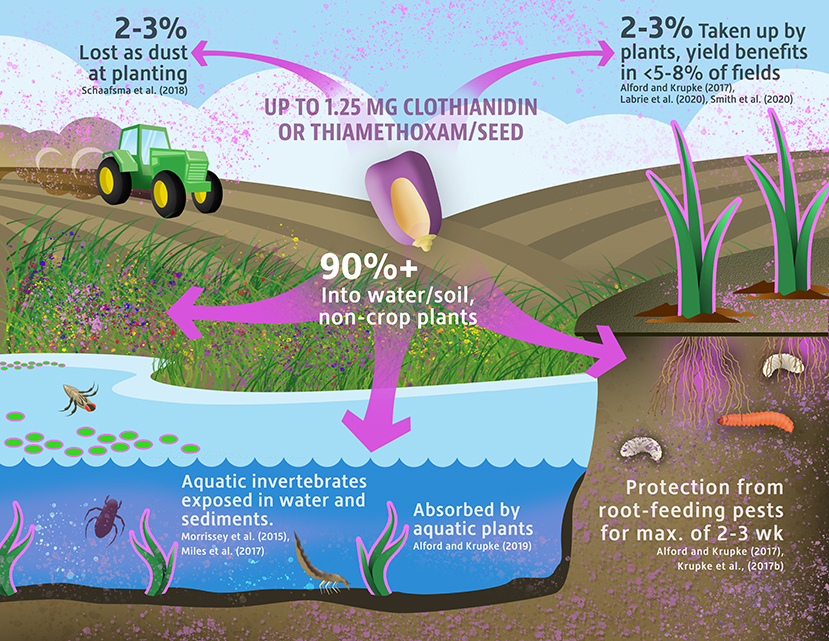
Figure 1. A schematic representation of the environmental fate and transport routes of neonicotinoid active ingredients applied to crop seeds.
Neonicotinoid use Patterns and Potential for Targeted Pest Management
Surveying the current insecticide landscape in the United States reveals that a single class of compounds, the neonicotinoids, clearly stands above the others in terms of both the prevalence of use on the landscape and in terms of their potential toxicity to insects (DiBartolomeis et al., 2019; Douglas et al., 2020). Compared to older classes of insecticides, neonicotinoids were originally touted as being more selective because of their systemic nature (i.e., it was thought that only insects feeding on the crop would be exposed) and their apparently lower toxicity to mammals and other vertebrates (Han et al., 2018), although recent data indicate that the latter should be re-evaluated (Berheim et al., 2019; Eng et al., 2019; Wu et al., 2020).
The U.S. is the top user of neonicotinoids, by a wide margin, and the majority of use is as coatings on seeds of annual crops, principally corn, soybeans, cotton, and wheat (Douglas et al., 2015; DiBartolomeis et al., 2019). Although recent estimates of use rates on these crops are not publicly available (the United States Geological Survey discontinued estimates in 2015), we know these compounds are used on virtually every hectare of corn planted in the United States, and that the amount of active ingredient applied to corn seeds doubled between 2011 and 2014 (Tooker et al., 2017). There are also high rates of use on seeds of soybeans, cotton and many other crop species (Douglas et al., 2015, 2020). It is important to note that these trends have been documented even as transgenic hybrids—Bt corn and cotton—have increasingly dominated the market, successfully reducing outbreaks of key pests of these crops (Dively et al., 2018) and even decreasing regional pest populations (Hutchison et al., 2010; Bohnenblust et al., 2014). Notably, however, the adoption rates of both Bt crops and neonicotinoid seed coatings have not corresponded with any new or increased pest threat. In fact, current insecticide use rates far exceed the <50% of corn acres and <10% of soybean acres that were typically treated with an insecticide from the 1950s to the 1990s (Osteen and Fernandez-Cornejo, 2013), when patterns of insecticide use were dictated primarily by the same key pests that are now largely controlled by transgenic hybrids. There are no longitudinal datasets to cite on pest trends over that time, but recent reviews reflect that most of the target pests listed for neonicotinoid seed treatments in registration and marketing materials were never common, are not more common now, and usually do not cause significant yield loss when they are present (Bredeson and Lundgren, 2015; Hesler et al., 2018; Sappington et al., 2018; Labrie et al., 2020; Smith et al., 2020). In other words, rather than “replacing” older insecticide classes with neonicotinoids, the seed-treatment approach fostered by neonicotinoids has created an entirely new and exceptionally convenient way to deliver insecticides without regard for documentation or monitoring of actual pest infestations on the most widely grown commodities in the U.S.
Before understanding how neonicotinoids may affect non-target organisms, it is useful to review how and why they were rapidly and thoroughly adopted in virtually every cropping system where insect pest management is required. Neonicotinoids are extremely water soluble, meaning that once in aqueous solution, they will readily move “upward” within xylem tissues from roots into newer aboveground plant tissues. Initially, this systemic characteristic was hailed as a breakthrough, and a “perfect” approach to pest management (Elbert et al., 2008; Jeschke et al., 2011). In theory, applying a water-soluble insecticide on or near a plant or seed, offers both immediate protection of the most vulnerable stages of the plant's life cycle and lasting insurance, as it is taken up into plant tissues and can protect plants from future pest attacks. This mode of action meant that, despite the very high and broad toxicity of neonicotinoids to most insects, the initial marketing was credibly able to claim that any organisms that are not pests—for example, insects and other arthropods merely resting or crawling along treated plants, living in the soil or water nearby, or flying by planted fields—would be unaffected. As long as they did not feed upon crop plants, any benign or beneficial organisms were free to live on or near neonicotinoid treated plants with no adverse effects. Even though similar approaches had been attempted (albeit on a much more limited scale) with carbamate insecticides and found to have undesirable non-target effects upon birds (Elliott et al., 1996), this seed-based approach was framed as a quantum leap, and a true paradigm shift and improvement from driftable liquid or powdered insecticides that are sprayed over entire fields. The low mammalian toxicity of neonicotinoids and enhanced worker safety were further selling points. Propelled by these perceived advantages, and rapid registration approvals in virtually every crop and ornamental system in North America, neonicotinoids rapidly became as ubiquitous as any insecticide class in history; neonicotinoids are currently deployed as seed coatings, liquid applications and occasionally as granular applications (Elbert et al., 2008) to a range of landscape, garden, and agricultural crops. Although restrictions in other jurisdictions have been imposed, use rates continue to rise each year in the U.S., providing an ongoing test case of this approach, with an ever-growing dataset to review. We qualitatively review some of these data here, both in terms of how well these compounds are delivering on their early pest management promise, but also in terms of their limiting effects on certain non-pest organisms that appear to have been largely overlooked during the registration and regulation processes.
Realized Potential of Neonicotinoid-Treated Seeds for Pest Management and Yield Protection in Field Studies
If a specific pest management tool provides sufficiently high benefits to agriculture or society at large, some level of collateral damage to the surrounding environment may be deemed acceptable. Non-target effects are somewhat inevitable, and perhaps to be expected, in agricultural systems that rely heavily upon large, intensive monocultures and regular deployment of a suite of pesticides. It is striking, then, that a survey of the literature reveals no convincing or consistent demonstrations of economic benefits associated with neonicotinoid use in grain and oilseed crops. Small-plot field studies throughout North America (Seagraves and Lundgren, 2012; Bredeson and Lundgren, 2015; Krupke et al., 2017a,b; North et al., 2018), meta-analyses of trials in soybeans over multiple U.S. states (Mourtzinis et al., 2019), and multi-year larger scale trials conducted across dozens of commercial corn and soybean fields (Labrie et al., 2020; Smith et al., 2020) have all failed to detect clear or consistent economic benefits attributable to this widespread approach to pest management. Indeed, detailed surveys of pest infestations over several years in intensive corn and soybean producing area of Quebec and Ontario, Canada found that neonicotinoids had potential to protect yield in <5–8% of cases (Labrie et al., 2020; Smith et al., 2020). Populations of target pests were also documented in these studies and were low (often zero)—leading the authors to conclude that no pest management benefit was realized since pests were not present at damaging levels. It is notable that, unlike the situations reflected by meta-analyses of aquatic organisms (Chagnon et al., 2015; Sánchez-Bayo et al., 2016; Wolfram et al., 2018), there are no datasets showing long-term population trends for any of the pest species targeted by neonicotinoids. It is reasonable to suggest that, given the ubiquity of the seed treatment approach in North America over some 15 years, pest pressures may be expected to have dramatically declined. One of the experimental hurdles to carrying out such work is that there is no “untreated” refuge for the pest species targeted in the US, as almost all annual, or row crops, are treated every year. Despite this lack of biological or agronomic rationale, rates of active ingredient applied to crop seeds have steadily increased (Douglas et al., 2015; DiBartolomeis et al., 2019). Similarly, there are no data to support the hypothesis that novel pest pressures or changing economic considerations, such as commodity prices, are driving increasing rates of neonicotinoid active ingredient/seed. Given this lack of quantifiable justification, it seems likely that the trends of adding progressively more active ingredient on crop seeds is driven chiefly by considerations other than farmer or market demand or any readily quantifiable agricultural considerations. If pest pressures or economic considerations were driving higher application rates, it would be easier to reconcile some of the non-target effects of releasing progressively more neonicotinoids into the environment each year.
The Fate of Neonicotinoids From Seed Coatings
The very properties that make neonicotinoids ideally suited for use as seed treatments—high water solubility and the potential for xylem transport in plant tissues—ensure that the majority of the neonicotinoid applied to crop seeds will, inevitably, wind up elsewhere (Hladik et al., 2014; Main et al., 2014; Long and Krupke, 2016; Mogren and Lundgren, 2016; Alford and Krupke, 2017, 2019). Chronicling the movement of these compounds from the seed coating, into the crop plant, and beyond, is a logical starting point in assessing the potential for effects upon non-target lands, waterways, and the organisms that live there.
Nowhere are neonicotinoids deployed more widely and thoroughly than annual production of corn, or maize in the United States, where virtually every non-organic seed grown for grain production is treated with thiamethoxam and/or clothianidin, and less commonly, imidacloprid (Douglas et al., 2015). During planting of corn seeds, neonicotinoids and other active ingredients are abraded from the seed coat and exhausted via pneumatic planting equipment typically used in modern North American planting operations. This dust moves out into the surrounding landscape (Krupke et al., 2012, 2017b; Stewart et al., 2014; Schaafsma et al., 2018), and presents a route of exposure via insecticide drift that has resulted in reports of honey bee mortality in multiple jurisdictions (summarized in Krupke and Long, 2015). Using honey bee foraging radii as a parameter, this airborne movement of active ingredient was found to have potential for lethal and sub-lethal effects extending hundreds of meters beyond each planted field, resulting in potential for lethal exposure of non-target organisms, in this case foraging honey bees (Krupke et al., 2017b). Although the environmental fate of dispersed particulates was not quantified in this study, these exposures are likely to be acute, in terms of toxicity, and relatively short in duration. One estimate concluded that planting of treated seeds typically results in 2–3% of the active ingredient applied to seeds being abraded and dispersed during planting, although the authors note that this percentage may range as high as 12% of the applied active ingredient (Schaafsma et al., 2018). Several potential solutions to address the release of this insecticide-laden dust have been proposed by the research groups referenced above and others (Biocca et al., 2017), and policies to reduce this non-target exposure route have been implemented in Canada (Health Canada, 2015). However, there are no indications that this route of exposure has been addressed or mitigated to any meaningful extent in the United States, where the majority of neonicotinoid treated seeds are planted even though initial reports first surfaced almost a decade ago (Krupke et al., 2012).
Once corn seeds are planted, concentrations of neonicotinoid active ingredients exhibit a typical exponential decay pattern, whereby there is a rapid initial uptake by plants following germination and movement into growing tissues, followed by a rapid decline (Alford and Krupke, 2017); the same pattern is found in rice treated with imidacloprid (Iwaya et al., 1998). This results in a potential “pest protection window” of a maximum of 2–3 weeks following planting. A similar pattern has been documented in soybeans, another key commodity planted using neonicotinoid-treated seeds (Krupke et al., 2017a). In fact, this pattern may be expected from any water-soluble compound—once plant tissues are saturated, any neonicotinoids remaining on or near the treated plant would be expected to demonstrate an affinity for unsaturated aqueous compartments, and not readily enter the plant. However, one surprising corollary of these results is the inefficiency associated with the seed-treatment approach; in corn, a maximum of 1.34% of active ingredient was recovered at any period in plant tissues, with a total of <5% of the initial application rate recovered from plants during the entire growing season. This raises obvious questions about the fate of the remaining active ingredient. Initial registration documents for both thiamethoxam and clothianidin reflect soil stability and persistence inherent in these compounds. Similarly, imidacloprid uptake by treated plants does not account for more than 2–20% of the applied amount (Goulson, 2013) with the remainder found in field soils and accumulating after successive yearly applications (Jones et al., 2014), effectively resulting in a reservoir of insecticide that may leach into surrounding waterways over months or years.
While not the only metric used to assess leaching risk, the Groundwater Ubiquity Score (GUS; Gustafson, 1989) relates the compound's soil organic carbon-water partitioning coefficient (Koc), and its half life (DT50) and assigns low, medium, and high leaching potentials to respective GUS values of <1.8, 1.8–2.8, and >2.8. Clothianidin and thiamethoxam have GUS values of 5.43–6.98 and 1.84–4.25, respectively, based upon respective Koc values of 60 and 68.4 (Alford and Krupke, 2017). These values reflect that both compounds are likely to be found in both ground and surface waters. Confining pesticide applications to the target crop and plant is a long-standing, elusive goal that was thought to be at least partially addressed by the seed treatment approach. However, we now know that neonicotinoid seed treatments can and do typically move beyond the planted field (Hladik et al., 2014; Samson-Robert et al., 2014; Morrissey et al., 2015; Chretien et al., 2017; Miles et al., 2017), and that leaching does occur when these compounds are used under field conditions (e.g., potatoes: Huseth and Groves, 2014; sugar beets: Wettstein et al., 2016). In one study, concentrations in tile-drained groundwaters peaked within several weeks following corn planting (Alford and Krupke, 2019). Similar results were found in no-till fields without tile drains, but this study also revealed that active ingredients with lower solubility left fields more slowly (Frame et al., accepted). In studies cited above (Samson-Robert et al., 2014; Alford and Krupke, 2019), spikes in detections of neonicotinoids from seed treatment in surface puddles and groundwater in and near fields corresponded with rainfall events, meaning that exposures are likely to be inconsistent, or pulsed, throughout the growing season and are likely to be most directly experienced by aquatic insects and other organisms in habitats that receive water running off of fields. This presents a challenge in replicating these exposures—that may include a mix of acute and chronic exposures—in controlled lab or semi-field settings.
Neonicotinoid Intersections With Aquatic Communities
It is unsurprising that non-target effects have been documented for a range of organisms and systems, given the rapid, widespread “adoption” of neonicotinoid insecticides, albeit often without a clear consent from buyers (e.g., around 30% of farmers were not aware that insecticides were on their seeds; Hitaj et al., 2020). However, most research on non-target effects has focused upon honey bees and other pollinators, both in terms of documenting exposure routes, and lethal and sub-lethal effects. Indeed, it is clear that honey bees are exposed to neonicotinoid insecticides through a range of exposure routes (Wood and Goulson, 2017). However, we argue that because honey bees are an intensively managed, mobile, and resilient species, living in colonies of tens of thousands, honey bee health metrics are likely to dramatically underestimate the risk to more sensitive organisms with life histories that are more easily disrupted by exposure to a water-soluble insecticide. With mounting evidence showing that a significant portion of applied neonicotinoids end up in waterways, we would be wise to direct more attention toward the effects these water-soluble insecticides have upon aquatic environments.
While water-soluble insecticides are arguably the best place to start in assessing mortality factors for aquatic insects, we note also that there are important additional and overlapping stressors upon aquatic insect populations that may confound attempts to track the effect of neonicotinoids on aquatic systems (Beketov and Liess, 2008). As neonicotinoid adoption has increased, so have levels of other stressors associated with agricultural intensification, including habitat loss and climate change (Verberk et al., 2016; Cavallaro et al., 2018; Jourdan et al., 2019; Baranov et al., 2020). There are other challenges in tracking the effects of neonicotinoids in aquatic systems, largely due to aquatic insects' often-cryptic nature (many live under rocks, leaf debris, or within sediment) and a dearth of basic natural-history information or population data. This lack of baseline data for species diversity and abundance, relatively sparse quantitative data on aquatic insect sensitivity to neonicotinoids (e.g., Van den Brink et al., 2016; Rico et al., 2018; Macaulay et al., 2019) and a shortage of published protocols for rearing and maintaining colonies of aquatic insects, further exacerbate the challenge of quantifying the degree and effects of pulsed neonicotinoid exposures on the wide range of organisms living in aquatic environments.
This dearth of data highlights that trends in science research and education are not immune to economics: the demonstrable benefit that pollinators have for the agricultural food supply has led to a plethora of funding opportunities and research interest aimed at documenting threats to pollinator health, and the recent explosion of literature on this topic reflects this emphasis.
For most aquatic invertebrates, however, although they are key components of aquatic systems, the benefits for the public good are not as easily quantified and research funding options for studying them are consequently more limited. In short, many of these organisms have little readily demonstrable utility to the public. They are generally uncharismatic, often drab in color, and live under rocks, dead leaves, or in the mud. A layperson could be forgiven for having little interest or curiosity about these organisms. However, there is no doubt that they contribute to food-web stability and provide functional redundancy in the community (Polis and Strong, 1996) and unsurprisingly, a recent review of the data that is available clearly outlined the pervasive, negative effects of neonicotinoids upon aquatic systems (Sánchez-Bayo et al., 2016).
In response to threats of this nature, researchers have urged regulatory authorities and the agricultural community to embrace other approaches for pest management, ranging from stopping the sale of neonicotinoid insecticides altogether, to a more thorough implementation of pest management approaches that rely upon an IPM framework (Sánchez-Bayo et al., 2016). In recent years, neonicotinoid use in some jurisdictions has been curtailed via regulation, or stopped altogether, notably in the European Union (Stokstad, 2018). Conversely, in the U.S., use rates have continued to rise rapidly over the same time period (DiBartolomeis et al., 2019; Douglas et al., 2020). There is no reason to expect this trend to abate, and a recent review of pesticide concentrations in U.S. surface waters found that corresponding levels of neonicotinoids in these systems are increasing and are expected to continue to do so (Wolfram et al., 2018). A meta-analysis of published studies focusing on the effects of environmental neonicotinoids upon non-target terrestrial arthropods found that neonicotinoids negatively affected all performance variables measured (Main et al., 2018). The purpose of this paper is not to repeat aspects of these reviews, but rather to point out that some of the ecosystem-level effects that these and other authors have warned about are coming into clearer focus in the U.S. and elsewhere, while crop yield benefits remain elusive—providing even more impetus for necessary change.
A striking example of an ecosystem-wide influence of neonicotinoids emerged recently from Japan. In this example, neonicotinoid runoff from rice production into a large lake caused drastic and long-term reductions in key species of midge and zooplankton, which are prey of eels and smelts, important fish species for local fishermen. With large reductions in their food source due to neonicotinoids, the eel and smelt fisheries collapsed, with severe consequences for local economies (Yamamuro et al., 2019). This story is instructive for at least two reasons. First, introduction of imidicloprid into the system quickly decreased zooplankton populations, and continued presence of neonicotinoids in the water and their time-cumulative toxicity (Tennekes and Sánchez-Bayo, 2013) kept their populations low. This continual depression of invertebrate populations, with effects that ripple through higher trophic levels, should be expected in regions where neonicotinoids are routinely used—each growing season releases a new dose of the insecticides into the environment, potentially chronically suppressing sensitive invertebrate populations with potential repercussions for invertebrates that rely on these invertebrate populations (e.g., Hallmann et al., 2014; Chen et al., 2019). Second, while midge populations in this example would have been expected to be sensitive to neonicotinoids in water (Cavallaro et al., 2018; United States Environmental Protection Agency, 2019), based on limited toxicity work with aquatic crustaceans the zooplankton (a copepod species) would not have been expected to be highly sensitive to neonicotinoids (Sánchez-Bayo, 2006). Yamamuro et al. (2019) discuss the role of multiple active ingredients of neonicotinoids in the unexpected toxicity, but their data clearly show the decline of zooplankton began when just one neonicotinoid (imidacloprid) was being sold in the region. More recent research indicates that many aquatic species are more sensitive to neonicotinoids than the model toxicological organisms often used by regulatory authorities to assess potential harms to aquatic ecosystems. One notable example is the crustacean, Daphnia magna, which despite being orders of magnitude more tolerant of neonicotinoid insecticides than many aquatic insects (Raby et al., 2018), is the most frequently used aquatic invertebrate for aquatic toxicity testing and regulatory (i.e., U.S. Environmental Protection Agency) submission packages. This suggests that ecological thresholds for neonicotinoid concentrations in water must be lowered to avoid unanticipated impacts from acute and chronic exposure (Morrissey et al., 2015); there is a clear rationale for including additional, and possibly more sensitive species, in future assessments (Roessink et al., 2013). Chronic exposure is a particularly important aspect of the aquatic toxicological picture that is difficult to mimic experimentally with compounds, like neonicotinoids, that are systemic, persistent, and re-inundate the water supply constantly at sub-lethal levels while triggering cumulative toxicity (Tennekes and Sánchez-Bayo, 2013).
Ecosystem-Wide Effects of Insecticide use: What's Past is Prolog
Sixty years ago, we were confronted with similar stories, but with different players; environmentalists, including author Rachel Carson, were alarmed by the negative ecosystem-wide effects of synthetic pesticides, especially the organochlorine insecticide, DDT. One of the key themes that made Carson's Silent Spring so influential was the connection she drew between environmental and human health (Carson, 1962). A recent example from Africa illustrates this connection clearly and highlights the likelihood of unanticipated ecological disruptions when pesticides enter aquatic systems. This study found that relatively low concentrations of the neonicotinoid imidacloprid and the organophosphate diazinon reduced abundance of some of the more sensitive aquatic insect species, leading to larger aquatic snail populations because of reduced competition for food and less predation from insect species (Becker et al., 2020). Snails are intermediate hosts for parasitic flatworms that cause schistosomiasis in human populations, and their greater abundance increased the likelihood of flatworm transmission to the local population (Becker et al., 2020). Remarkably, Carson predicted these sorts of outcomes when insecticides are overused. She vividly described a scene from salt marshes treated with insecticides where snails were the only visible living animals, and explicitly warned of the risk to human health resulting from this abundance of snails, relating this to schistosomiasis outbreaks (Carson, 1962). While this human health issue may not be a large concern for individuals considering neonicotinoid use in the U.S., this example does show how humans, and not just wildlife, can be victims of subtle insecticide-driven changes in invertebrate community structure. Similar outcomes have been found in agricultural systems, where neonicotinoid-driven changes in the invertebrate predator community can reduce crop productivity (Douglas et al., 2015).
In Chapter 7 of Silent Spring, when lamenting the “needless havoc” on wildlife wrought by indiscriminate insecticide use, Carson wrote: “The entomologist, whose specialty is insects, is not so qualified by training, and is not psychologically disposed to look for undesirable side effects of his control programs.” This is an unflattering, one-dimensional generalization of entomologists from the 1950s and early 1960s—rigid and myopic scientists focused on exterminating insect populations with no regard for non-target effects. Put into the perspective of that era, however, this is perhaps understandable as the responses of the Entomological Society of America to Carson reflected an initial hostility to her message (Krupke et al., 2007). This 2007 review of the response to Carson's work reflected that: “Today, we may be hard pressed to find a knowledgeable entomologist or toxicologist who would argue for a return to the widespread application of broad-spectrum, persistent pesticides,” and yet, this is precisely the situation we find ourselves in today.
Synthesis
There is no doubt that the advances in chemistry and synthetic insecticides that emerged from World War II extended into everyday life to benefit farmers and consumers besieged by insect pests. Notably, USDA and its entomologists embraced and promoted insecticide use, with officials repeatedly assuring that there would be little or no cost to wildlife if the insecticides were used according to USDA recommendations (Mart, 2015). Then, as now, the trust in the environmental safety of proper insecticide use was believed to be justified at the time. But, thankfully, science is progressive by nature and new hypotheses and the tools to test them emerge continually. Through the work of entomologists, ecologists, wildlife biologists, toxicologists and other scientists, we learned that non-target effects were not only present, but pervasive. We now know that indiscriminate, often prophylactic, insecticide use can have significant non-target effects. In the current landscape, ample research has demonstrated that these effects are ubiquitous in association with widespread neonicotinoid use. Indeed, we are unaware of any recent reports of increases in abundances of aquatic species in agricultural regions of the U.S. where neonicotinoids are common. Interestingly, a recent meta-analysis focusing on the phenomenon of insect declines found that freshwater insect populations were actually increasing in abundance by about 15% between the 1960s and 2005, likely due to improvements in water quality (van Klink et al., 2020). However, because neonicotinoid use has increased most dramatically since 2006 (Douglas et al., 2015; Tooker et al., 2017), their potential influence on aquatic communities would not have been included in the data used in this meta-analysis.
Importantly, compared to the 1960s, we now have a better grasp of the ecological effects of food web simplification. Decades of theoretical and empirical work has demonstrated that removing the easily overlooked, apparently inconsequential species from terrestrial food webs can result in unexpected shifts in primary productivity and consumer performance (e.g., Polis and Strong, 1996; Scheu et al., 1999). However, neonicotinoids present a particularly thorny problem without a historical comparator; the persistent, ubiquitous and highly water-soluble nature of neonicotinoids has resulted in an ongoing logistical hurdle in finding truly “untreated” control sites for rigorous experimental work. We do know that in aquatic food webs, exposure of communities to very low concentrations of insecticides can have unpredictable and lingering effects on species abundance, phenology and ecosystem function (e.g., litter decomposition) with influences that ripple up through trophic levels to influence the whole ecosystem (Liess and Ohe, 2005; Sánchez-Bayo et al., 2016; Cavallaro et al., 2018; Yamamuro et al., 2019; Becker et al., 2020). If current trends continue, the fundamentals of ecology ensure that eroding the foundations of aquatic systems will eventually manifest in negative consequences for game fish, birds, and other species that do have clear monetary value.
Although we cannot ignore the trend toward monetizing and commoditizing insects and their activities in terms of ecosystem services (Losey and Vaughan, 2006), this approach leaves us with blind spots regarding many aquatic insects, which are difficult to place into a clear economic framework, but we know to be critical for ecosystem function (Suter and Cormier, 2015). Until fishery collapses (Yamamuro et al., 2019), bird declines (Li et al., 2020), or human disease outbreak (Becker et al., 2020) belatedly sound an alarm, we remain largely unaware of these effects. We argue that in the case of neonicotinoid seed treatments, where the benefits of the approach are elusive and inconsistent, and the negative effects on aquatic systems are pervasive, the case is clear and the need for changing the trajectory is evident.
Concluding Thoughts
Contrary to popular belief, insecticide use in the U.S. continued to increase after Silent Spring, and those increases continue today (Mart, 2015). In the U.S., where Bt corn is rightly hailed as a target-specific and largely environmentally benign breakthrough in terms of reducing pesticide use (Dively et al., 2018), it is not common knowledge that virtually every kernel of Bt corn is treated with at least one neonicotinoid insecticide, and at steadily increasing levels (Douglas et al., 2020; Hitaj et al., 2020). It is also not widely reported that: (1) these insecticides target a suite of secondary pests that have historically been of only minor and sporadic economic importance, (2) are effective in a relatively short window of a maximum of 14–21 days following planting (Alford and Krupke, 2019), and that (3) most of the acres planted with Bt hybrids that include a neonicotinoid seed treatment are also likely to receive one or more applications of pyrethroid insecticides (United State Geological Survey National Water-Quality Assessment Project, 2020). These are underlying mechanisms by which use of insecticides in the U.S., and the presence of toxic residues across the landscape, have increased dramatically in recent years.
Aquatic, benthic invertebrates are almost certainly the “canary in the coal mine” when it comes to chronicling the non-target effects of ubiquitous, highly mobile and water-soluble neonicotinoid insecticides (Chagnon et al., 2015; Morrissey et al., 2015; Sánchez-Bayo et al., 2016; Miles et al., 2017; Main et al., 2018). These organisms have been steeped in steadily increasing concentrations of neonicotinoids in watersheds across the country for well over a decade, and it is possible that some of the documentable effects have been lost with time. We argue that as effects come to light, they are not counterbalanced by convincing arguments based upon increasing insect pest pressures, novel pest threats, or increases in yield common fiber or food production. If any slowing of this trend is to occur, there is an urgent need to begin quantifying effects on these poorly studied species before the ecological consequences of their disappearance has catastrophic effects on higher trophic levels (e.g., Hallmann et al., 2014; Li et al., 2020). At the upper-most trophic levels, we have increasing documentation of neonicotinoids in our food and drinking water (Klarich et al., 2017), and studies are underway to determine what effects, if any, these exposures may have for human health (Cimino et al., 2017; Chang et al., 2018; Craddock et al., 2019).
The intense focus on neonicotinoid impacts upon honey bee and pollinator health, while it has resulted in a vast repository of novel scientific literature, has not bent the curve of neonicotinoid use across the landscape. On the contrary, as mentioned above, use rates in the U.S. have increased even as evidence of environmental harms has mounted (Douglas et al., 2015; Tooker et al., 2017). While there are advocates in both the public and private sectors for continuation of the status quo, in terms of ongoing intensification of insecticide use to protect crop yield, these claims are not supported by trends in pest damage or rigorous economic analyses. As Rachel Carson's initial documentation demonstrated, the effects of unchecked, prophylactic insecticide use often reveal themselves in unexpected ways. We submit that looking in and near crop fields and other terrestrial environments for non-target effects of neonicotinoid insecticides is likely to miss most of the story. In hindsight it seems clear that, far from an unexpected consequence, the erosion of aquatic ecosystems is entirely predictable when faced with steadily increasing concentrations of a broadly toxic, highly soluble and virtually ubiquitous class of insecticides.
Aquatic systems are a cornerstone for a wide range of economically significant fisheries, recreational activities, and are also treasured by citizens for their aesthetic value. We now know that they are the main environmental sink for neonicotinoid insecticides. This may present a new potential lever to effect change that is urgently needed in how we approach pest management in our largest commodities. Perhaps most importantly, we outline above the mounting evidence that this change can be accomplished without reducing yields and influencing the food supply. Although there is undoubtedly a need in the U.S. and elsewhere for wholesale changes in regulatory policy to address current and future unintended environmental consequences of pesticide use (Brühl and Zaller, 2019), a strategic approach to implement immediate change already exists: integrated pest management (IPM). If IPM is not re-embraced and use of neonicotinoids (and other pesticides) continues along the current trajectory, aquatic communities will continue to degrade (Beketov et al., 2013).
IPM was developed over 50 years ago as a response to a suite of non-target effects (Stern et al., 1959), but remains all but abandoned in modern pest management in oilseed and grain crops grown in the U.S., notably corn, even while pest pressures are at or near historic lows (Hutchison et al., 2010; Bohnenblust et al., 2014; Sappington et al., 2018; Tinsley et al., 2018; Veres et al., 2020). It is worthwhile to note that consistent plant breeding efforts have led to many plant commodities, including corn, that are more durable and competitive than ever (Duvick, 2005), yet this aspect of plant tolerance is largely ignored in modern pest management (Peterson et al., 2018) as we use ever-increasing levels of insecticides, in terms of insect killing power, than ever before (DiBartolomeis et al., 2019; Douglas et al., 2020). These trends are evident despite recent data demonstrating that an IPM approach offers clear economic advantages over the neonicotinoid seed treatment approach, for example, in the case of the soybean aphid, a key pest of US soybean production (Krupke et al., 2017a).
Given the increases in land devoted to agricultural production since the 1950's, and the more potent and persistent insecticide tools of the modern era, there is abundant rationale to re-introduce the IPM approach—including the notion of simply not applying pesticides where pest densities are not sufficient to cause economic harm. This requires that monitoring of pests and use of thresholds—critical elements of IPM—be re-introduced to protect natural enemies communities that can provide biological control, one of the original goals of IPM (Stern et al., 1959) that neonicotinoids work directly against (e.g., Douglas et al., 2015; Douglas and Tooker, 2016). In the case of many pests targeted by neonicotinoid seed treatments, these thresholds have not been revisited in decades, likely partly a result of both their low incidence and the ubiquity of preventative, prophylactic neonicotinoid seed treatment use. In the U.S., there are no modern datasets documenting where, or when, many of these pests are abundant, or if they are present at all, although recent analyses in Canadian corn and soybean production systems found remarkably few pests and a consequent limited pest-management benefit of neonicotinoid-treated seed (Labrie et al., 2020; Smith et al., 2020). These are readily accessible areas for future research in the U.S. that can give agricultural producers additional confidence in reducing their reliance on insecticides. Moreover, recent research has revealed that practices that form the core of conservation agriculture (e.g., no-till farming, cover crops, animal-based fertilizers, increased diversity) can, among other benefits, improve predator populations and associated top-down control; therefore, embracing these approaches, along with IPM, can help build biological control in fields and decrease reliance on insecticides (Schipanski et al., 2014; Lundgren and Fausti, 2015; Tamburini et al., 2016; Rowen et al., 2019, 2020; Busch et al., 2020; Tooker et al., 2020; Wyckhuys et al., 2020).
In the meantime, however, there is abundant evidence that the routine use of neonicotinoids on every hectare, every year, in many key commodities is an approach with mounting costs and few benefits. Considered within the backdrop of the multitude of environmental grand challenges facing our society (National Academies of Sciences, 2019), this issue stands out simply because the economic and food/feed production benefits of the current practice have been so difficult to document—this should be among the more solvable problems on the board, About 60 years ago, Carson (1962) documented a similar imbalance of costs and benefits, and that imbalance was addressed with regulation that has proven insufficient for dealing with the current ubiquity of neonicotinoid seed coatings. As the data reviewed above demonstrate, continuing along the current trajectory will result in mounting negative environmental consequences, with no consistent, demonstrable benefits. We submit that by ignoring the data, regulatory authorities and industry alike are unwittingly building a stronger case that neonicotinoid insecticides, and future offerings, must be more strongly regulated to prevent these entirely avoidable outcomes.
Author Contributions
CK initiated the contribution and developed the initial framework of the article. CK and JT contributed equally to writing the article. Both authors contributed to the article and approved the submitted version.
Funding
Funding for this project came in part from the College of Agriculture at Purdue University via the National Institute of Food and Agriculture and Hatch Appropriations under Accession #1009386 and from the College of Agricultural Sciences at Penn State via the National Institute of Food and Agriculture and Hatch Appropriations under Project #PEN04606 and Accession #1009362.
Conflict of Interest
The authors declare that the research was conducted in the absence of any commercial or financial relationships that could be construed as a potential conflict of interest.
Acknowledgments
The authors thank to the editors for inviting our contribution to this themed issue, the three reviewers for their constructive comments that improved quality of the manuscript, and to N. Sloff for assistance with Figure 1. We were grateful to K. Pearsons and S. Shepherd for constructive reviews of an earlier version of the manuscript.
References
Alford, A., and Krupke, C. H. (2017). Translocation of the neonicotinoid seed treatment clothianidin in maize. PLoS ONE 12:e0173836. doi: 10.1371/journal.pone.0173836
Alford, A., and Krupke, C. H. (2019). Movement of the neonicotinoid seed treatment clothianidin into groundwater, aquatic plants, and insect herbivores. Environ. Sci. Technol. 53, 14368–14376. doi: 10.1021/acs.est.9b05025
Baranov, V., Jourdan, J., Pilotto, F., Wagner, R., and Haase, P. (2020). Complex and nonlinear climate-driven changes in freshwater insect communities over 42 years. Conserv. Biol. 34, 1241–1251. doi: 10.1111/cobi.13477
Becker, J. M., Ganatra, A. A., Kandie, F., Mühlbauer, L., Ahlheim, J., Brack, W., et al. (2020). pesticide pollution in freshwater paves the way for schistosomiasis transmission. Sci. Rep. 10, 1–13. doi: 10.1038/s41598-020-60654-7
Beketov, M. A., Kefford, B. J., Schäfer, R. B., and Liess, M. (2013). Pesticides reduce regional biodiversity of stream invertebrates. Proc. Nat. Acad. Sci. U.S.A. 110, 11039–11043. doi: 10.1073/pnas.1305618110
Beketov, M. A., and Liess, M. (2008). Acute and delayed effects of the neonicotinoid insecticide thiacloprid on seven freshwater arthropods. Environ. Toxicol. Chem. 27, 461–470. doi: 10.1897/07-322R.1
Berheim, E. H., Jenks, J. A., Lundgren, J. G., Michel, E. S., Grove, D., and Jensen, W. F. (2019). Effects of neonicotinoid insecticides on physiology and reproductive characteristics of captive female and fawn white-tailed deer. Sci. Rep. 9:4534. doi: 10.1038/s41598-019-40994-9
Biocca, M., Pochi, D., Fanigliulo, R., Gallo, P., Pulcini, P., Marcovecchio, F., et al. (2017). Evaluating a filtering and recirculating system to reduce dust drift in simulated sowing of dressed seed and abraded dust particle characteristics. Pest Manag. Sci. 73, 1134–1142. doi: 10.1002/ps.4428
Bohnenblust, E. W., Breining, J. A., Shaffer, J. A., Fleischer, S. J., Roth, G. W., and Tooker, J. F. (2014). Current European corn borer, Ostrinia nubilalis, injury levels in the northeastern United States and the value of Bt field corn. Pest Manag. Sci. 70, 711–1719. doi: 10.1002/ps.3712
Bredeson, M. M., and Lundgren, J. G. (2015). Thiamethoxam seed treatments have no impact on pest numbers or yield in cultivated sunflowers. J. Econ. Entomol. 108, 2665–2671. doi: 10.1093/jee/tov249
Brühl, C. A., and Zaller, J. G. (2019). Biodiversity decline as a consequence of an inappropriate environmental risk assessment of pesticides. Front. Environ. Sci. 7:177. doi: 10.3389/fenvs.2019.00177
Busch, A. K., Douglas, M. R., Malcolm, G. M., Karsten, H. D., and Tooker, J. F. (2020). A high-diversity/IPM cropping system fosters beneficial arthropod populations, limits invertebrate pests, and produces competitive maize yields. Agric. Ecosyst. Environ. 292:106812. doi: 10.1016/j.agee.2019.106812
Cavallaro, M. C., Liber, K., Headley, J. V., Peru, K. M., and Morrissey, C. A. (2018). Community-level and phenological responses of emerging aquatic insects exposed to 3 neonicotinoid insecticides: an in situ wetland limnocorral approach. Environ. Toxicol. Chem. 37, 2401–2412. doi: 10.1002/etc.4187
Chagnon, M., Kreutzweiser, D., Mitchell, E. D., Morrissey, C. A., Noome, D. A., and Van der Sluijs, J. P. (2015). Risks of large-scale use of systemic insecticides to ecosystem functioning and services. Environ. Sci. Pollut. Res. 22, 119–134. doi: 10.1007/s11356-014-3277-x
Chang, C. H., MacIntosh, D., Lemos, B., Zhang, Q., and Lu, C. (2018). Characterization of daily dietary intake and the health risk of neonicotinoid insecticides for the U.S. population. J. Agric. Food Chem. 66, 10097–10105. doi: 10.1021/acs.jafc.8b02982
Chen, Y., Zang, L., Liu, M., Zhang, Z., Shen, G., Du, W., et al. (2019). Ecological risk assessment of the increasing use of the neonicotinoid insecticides along the east coast of China. Environ. Internat. 127, 550–557. doi: 10.1016/j.envint.2019.04.010
Chretien, F., Giroux, I., Theriault, G., Gagnon, P., and Corriveau, J. (2017). Surface runoff and subsurface tile drain losses of neonicotinoids and companion herbicides at edge-of-field. Environ. Pollut. 224, 255–264. doi: 10.1016/j.envpol.2017.02.002
Cimino, A. M., Boyles, A. L., Thayer, K. A., and Perry, M. J. (2017). Effects of neonicotinoid pesti-cide exposure on human health: a systematic review. Environ. Health Perspect. 125, 155–162. doi: 10.1289/EHP515
Craddock, H. A., Huang, D., Turner, P. C., Quiros-Alcala, L., and Payne-Sturges, D. C. (2019). Trends in neonicotinoid pesticide residues in food and water in the United States, 1999–2015. Environ. Health 18:7. doi: 10.1186/s12940-018-0441-7
DiBartolomeis, M., Kegley, S., Mineau, P., Radford, R., and Klein, K. (2019). An assessment of acute insecticide toxicity loading (AITL) of chemical pesticides used on agricultural land in the United State. PLoS ONE 14:e0220029. doi: 10.1371/journal.pone.0220029
Dively, G. P., Venugopal, D., Bean, D., Whalen, J., Holmstrom, K., Kuhar, T. P., et al. (2018). Regional pest suppression associated with widespread Bt maize adoption benefits vegetable growers. Proc. Natl. Acad. Sci. U.S.A. 115, 3320–3325. doi: 10.1073/pnas.1720692115
Douglas, M. R., Rohr, J. R., and Tooker, J. F. (2015). Neonicotinoid insecticide travels through a soil food chain, disrupting biological control of non-target pests and decreasing soybean yield. J. Appl. Ecol. 52, 250–260. doi: 10.1111/1365-2664.12372
Douglas, M. R., Sponsler, D. B., Lonsdorf, E. V., and Grozinger, C. M. (2020). County-level analysis reveals a rapidly shifting landscape of insecticide hazard to honey bees (Apis mellifera) on US farmland. Sci. Rep. 10:797. doi: 10.1038/s41598-019-57225-w
Douglas, M. R., and Tooker, J. F. (2016). Meta-analysis reveals that neonicotinoid seed treatments and pyrethroids have similar negative effects on abundance of arthropod natural enemies. PeerJ 4:e2776. doi: 10.7717/peerj.2776
Duvick, D. N. (2005). The contribution of breeding to yield advances in maize (Zea mays L.). Adv. Agron. 86, 83–145. doi: 10.1016/S0065-2113(05)86002-X
Elbert, A., Haas, M., Springer, B., Thielert, W., and Nauen, R. (2008). Applied aspects of neonicotinoid uses in crop protection. Pest Manag. Sci. 64, 1099–1105. doi: 10.1002/ps.1616
Elliott, J. E., Langelier, K. M., Mineau, P., and Wilson, L. K. (1996). Poisoning of bald eagles and red-tailed hawks by carbofuran and fensofothion in the Fraser Delta of British Columbia, Canada. J. Wildlife Dis. 32, 486–491. doi: 10.7589/0090-3558-32.3.486
Eng, M. L., Stutchbury, B. J. M., and Morrissey, C. A. (2019). A neonicotinoid insecticide reduces fueling and delays migration in songbirds. Science 365, 1177–1180. doi: 10.1126/science.aaw9419
Frame, S. T., Pearsons, K. A., Elkin, K. R., Saporito, L. S., Preisendanz, H. E., and Karsten, H. D. (accepted). Assessing surface subsurface transport of neonicotinoid insecticides from crop fields. J. Environ. Qual.
Goulson, D. (2013). Review: an overview of the environmental risks posed by neonicotinoid insecticides. J. Appl. Ecol. 50, 977–987. doi: 10.1111/1365-2664.12111
Gustafson, D. I. (1989). Groundwater ubiquity score: A simple method for assessing pesticide leachability. Environ. Toxicol. Chem. 8, 339–357. doi: 10.1002/etc.5620080411
Hallmann, C., Foppen, R., van Turnhout, C., deKroon, H., and Jongejans, E. (2014). Declines in insectivorous birds are associated with high neonicotinoid concentrations. Nature 511, 341–343. doi: 10.1038/nature13531
Han, W., Tian, Y., and Shen, X. (2018). Human exposure to neonicotinoid insecticides and the evaluation of their potential toxicity: an overview. Chemosphere 192, 59–65. doi: 10.1016/j.chemosphere.2017.10.149
Health Canada (2015). Requirement When Using Treated Corn/Soybean Seed. Available online at: https://www.canada.ca/en/health-canada/services/consumer-product-safety/reports-publications/pesticides-pest-management/fact-sheets-other-resources/pollinator-treated-seed/treated-corn-soybean-seed.html (accessed October 26, 2020).
Hesler, L., Allen, K. C., Luttrell, R. G., Sappington, T. W., and Papiernik, S. K. (2018). Early-season pests of soybean in the United States and factors that affect their risk of infestation. J. Integr. Pest Manag. 9:19. doi: 10.1093/jipm/pmx028
Hitaj, C., Smith, D. J., Code, A., Wechsler, S., Esker, P. D., and Douglas, M.R. (2020). Sowing uncertainty: what we do and don't know about the planting of pesticide-treated seed. BioScience 70, 390–403. doi: 10.1093/biosci/biaa019
Hladik, M. L., Kolpin, D. W., and Kuivila, K. M. (2014). Widespread occurrence of neonicotinoids in streams in a high corn and soybean producing region USA. Environ. Pollut. 193, 189–196. doi: 10.1016/j.envpol.2014.06.033
Huseth, A. S., and Groves, R. L. (2014). Environmental fate of soil applied neonicotinoid insecticides in an irrigated potato agroecosystem. PLoS ONE 9:e97081. doi: 10.1371/journal.pone.0097081
Hutchison, W. D., Burkness, E. C., Mitchell, P. D., Moon, R. D., Leslie, T. W., Fleischer, S. J., et al. (2010). Areawide suppression of European corn borer with Bt maize reaps savings to non-Bt maize growers. Science 330, 222–225. doi: 10.1126/science.1190242
Iwaya, K., Maruyama, M., Nakanishi, H., and Kurogochi, S. (1998). Concentration of imidacloprid in rice plants and biological effect on Nilaparvata lugens. J. Pesticide Sci. 23, 419–421. doi: 10.1584/jpestics.23.419
Jeschke, P., Nauen, R., Schindler, M., and Elbert, A. (2011). Overview of the status and global strategy for neonicotinoids. J. Agric. Food Chem. 59, 2897–2908. doi: 10.1021/jf101303g
Jones, A., Harrington, P., and Turnbull, G. (2014). Neonicotinoid concentrations in arable soils after seed treatment applications in preceding years. Pest Manag. Sci. 70, 1780–1784. doi: 10.1002/ps.3836
Jourdan, J., Baranov, V., Wagner, R., Plath, M., and Haase, P. (2019). Elevated temperatures translate into reduced dispersal abilities in a natural population of an aquatic insect. J. Anim. Ecol. 88, 1498–1509. doi: 10.1111/1365-2656.13054
Klarich, K. L., Pflug, N. C., DeWald, E. M., Hladik, M. L., Kolpin, D. W., Cwiertny, D. M., et al. (2017). Occurrence of neonicotinoid insecticides in finished drinking water and fate during drinking water treatment. Environ. Sci. Technol. Lett. 5, 168–173. doi: 10.1021/acs.estlett.7b00081
Krupke, C. H., Alford, A. M., Cullen, E. M., Hodgson, E. W., Knodel, J. J., McCornack, B., et al. (2017a). Assessing the value and pest management window provided by neonicotinoid seed treatments for management of soybean aphid (Aphis glycines Matsumura) in the Upper Midwestern United States. Pest Manag. Sci. 73, 2184–2193. doi: 10.1002/ps.4602
Krupke, C. H., Holland, J. D., Long, E. Y., and Eitzer, B. D. (2017b). Planting of neonicotinoid-treated maize poses risks for honey bees and other non-target organisms over a wide area without consistent crop yield benefit. J. Appl. Ecol. 54, 1449–1458. doi: 10.1111/1365-2664.12924
Krupke, C. H., Hunt, G., Eitzer, B., Andino, G., and Given, K. (2012). Multiple routes of pesticide exposure for honey bees living near agricultural fields. PLoS ONE 7:e29268. doi: 10.1371/journal.pone.0029268
Krupke, C. H., and Long, E.Y. (2015). Intersections between neonicotinoid seed treatments and honey bees. Curr. Opin. Insect. Sci. 10, 8–13. doi: 10.1016/j.cois.2015.04.005
Krupke, C. H., Prasad, R., and Anelli, C. (2007). A noisy response to a silent spring: entomology's response to Rachel Carson. Am. Entomol. 53, 16–25. doi: 10.1093/ae/53.1.16
Labrie, G., Gagnon, A. È., Vanasse, A., Latraverse, A., and Tremblay, G. (2020). Impacts of neonicotinoid seed treatments on soil-dwelling pest populations and agronomic parameters in corn and soybean in Quebec (Canada). PLoS ONE 15:e0229136. doi: 10.1371/journal.pone.0229136
Li, Y., Miao, R., and Khanna, M. (2020). Neonicotinoids and decline in bird biodiversity in the United States. Nat. Sustain. doi: 10.1038/s41893-020-0582-x
Liess, M., and Ohe, P. C. V. D. (2005). Analyzing effects of pesticides on invertebrate communities in streams. Environ. Toxicol. Chem. 24, 954–965. doi: 10.1897/03-652.1
Long, E. L., and Krupke, C. H. (2016). Non-cultivated plants present a season-long route of pesticide exposure for honey bees. Nat. Commun. 7:11629. doi: 10.1038/ncomms11629
Losey, J. E., and Vaughan, M. (2006). The economic value of ecological services provided by insects. BioScience 56, 311–323. doi: 10.1641/0006-3568(2006)56[311:TEVOES]2.0.CO;2
Lundgren, J. G., and Fausti, S. W. (2015). Trading biodiversity for pest problems. Sci. Adv. 1:e1500558. doi: 10.1126/sciadv.1500558
Macaulay, S. J., Hageman, K. J., Alumbaugh, R. E., Lyons, S. M., Piggott, J. J., and Matthaei, C. D. (2019). Chronic toxicities of neonicotinoids to nymphs of the common New Zealand Mayfly Deleatidium spp. Environ. Toxicol. Chem. 38, 2459–2471. doi: 10.1002/etc.4556
Main, A. R., Headley, J. V., Peru, K. M. Michel, N. L., Cessna, A. J., and Morrissey, C. A. (2014). Widespread use and frequent detection of neonicotinoid insecticides in wetlands of Canada's Prairie Pothole Region. PLoS ONE 9:e92821. doi: 10.1371/journal.pone.0092821
Main, A. R., Webb, E. B., Goyne, K. W., and Mengel, D. (2018). Neonicotinoid insecticides negatively affect performance measures of non-target terrestrial arthropods: a meta-analysis. Ecol. Appl. 28, 1232–1244. doi: 10.1002/eap.1723
Mart, M. (2015). Pesticides, a Love Story: America's Enduring Embrace of Dangerous Chemicals. Lawrence, KS: University Press of Kansas.
Miles, J. C., Hua, J., Sepulveda, M. S., Krupke, C. H., and Hoverman, J. T. (2017). Effects of clothianidin on aquatic communities: Evaluating the impacts of lethal and sublethal exposure to neonicotinoids. PLoS ONE 12:e0174171. doi: 10.1371/journal.pone.0174171
Mogren, C., and Lundgren, J. (2016). Neonicotinoid-contaminated pollinator strips adjacent to cropland reduce honey bee nutritional status. Sci. Rep. 6:29608. doi: 10.1038/srep29608
Morrissey, C. A., Mineau, P., Devries, J. H., Sanchez-Bayo, F., Liess, M., Cavallaro, M. C., et al. (2015). Neonicotinoid contamination of global surface waters and associated risk to aquatic invertebrates: a review. Environ. Int. 74, 291–303. doi: 10.1016/j.envint.2014.10.024
Mourtzinis, S., Krupke, C. H., Esker, P. D., Varenhorst, A., Arneson, N. J., Bradley, C. A., et al. (2019). Neonicotinoid seed treatments of soybean provide negligible benefits to US farmers. Nat. Sci. Rep. 9:11207. doi: 10.1038/s41598-019-47442-8
National Academies of Sciences Engineering and Medicine. (2019). Environmental Engineering for the 21st Century: Addressing Grand Challenges. Washington, DC: The National Academies Press.
North, J. H., Gore, J., Catchot, A. L., Stewart, S. D., Lorenz, G. M., Musser, F. R., et al. (2018). Value of neonicotinoid insecticide seed treatments in mid-south corn (Zea mays) production systems. J. Econ. Entomol. 111, 187–192. doi: 10.1093/jee/tox278
Osteen, C. D., and Fernandez-Cornejo, J. (2013). Economic and policy issues of U.S. agricultural pesticide use trends. Pest Manag. Sci. 69, 1001–1025. doi: 10.1002/ps.3529
Pedigo, L. E., and Rice, M. E. (2014). Entomology and Pest Management, 6th Edn. Long Grove, IL: Waveland Press Inc.
Peterson, R. K. D., Higley, L. G., and Pedigo, L. P. (2018). Whatever happened to IPM? Am. Entomol. 64, 146–150, doi: 10.1093/ae/tmy049
Polis, G. A., and Strong, D. R. (1996). Food web complexity and community dynamics. Am. Nat. 147, 813–846. doi: 10.1086/285880
Raby, M., Nowierski, M., Perlov, D., Zhao, X., Hao, C., Poirier, D. G., et al. (2018). Acute toxicity of 6 neonicotinoid insecticides to freshwater invertebrates. Environ. Toxicol. Chem. 37, 1430–1445. doi: 10.1002/etc.4088
Rico, A., Arenas-Sánchez, A., Pasqualini, J., García-Astillero, A., Cherta, L., Nozal, L., et al. (2018). Effects of imidacloprid and a neonicotinoid mixture on aquatic invertebrate communities under Mediterranean conditions. Aquat. Toxicol. 204, 130–143. doi: 10.1016/j.aquatox.2018.09.004
Roessink, I., Merga, L. B., Zweers, H. J., and Van den Brink, P. J. (2013). The neonicotinoid imidacloprid shows high chronic toxicity to mayfly nymphs. Environ. Toxicol. Chem. 32, 1096–1100. doi: 10.1002/etc.2201
Rowen, E., Tooker, J. F., and Blubaugh, C. K. (2019). Managing fertility with animal waste to promote arthropod pest suppression. Biol. Control 134, 130–140. doi: 10.1016/j.biocontrol.2019.04.012
Rowen, E. K., Regan, K. H., Barbercheck, M. E., and Tooker, J. F. (2020). Is tillage beneficial or detrimental for insect and slug management? A meta-analysis. Agric. Ecosyst. Environ. 294:106849. doi: 10.1016/j.agee.2020.106849
Samson-Robert, O., Labrie, G., Chagnon, M., and Fournier, V. (2014). Neonicotinoid-contaminated puddles of water represent a risk of intoxication for honey bees. PLoS ONE 9:e108443. doi: 10.1371/journal.pone.0108443
Sánchez-Bayo, F. (2006). Comparative acute toxicity of organic pollutants and reference values for crustaceans. I. Branchiopoda, Copepoda and Ostracoda. Environ. Pollut. 139, 385–420. doi: 10.1016/j.envpol.2005.06.016
Sánchez-Bayo, F., Goka, K., and Hayasaka, D. (2016). Contamination of the aquatic environment with neonicotinoids and its implication for ecosystems. Front. Environ. Sci. 4:71. doi: 10.3389/fenvs.2016.00071
Sappington, T. W., Hesler, L. S., Allen, C., Luttrell, R. G., and Papiernik, S. K. (2018). Prevalence of sporadic insect pests of seedling corn and factors affecting risk of infestation. J. Integr. Pest Manag. 9:1. doi: 10.1093/jipm/pmx020
Schaafsma, A. W., Limay-Rios, V., and Forero, L. G. (2018). The role of field dust in pesticide drift when pesticide-treated maize seeds are planted with vacuum-type planters. Pest Manag. Sci. 74, 323–331. doi: 10.1002/ps.4696
Scheu, S., Theenhaus, A., and Jones, T. H. (1999). Links between the detritivore and the herbivore system: effects of earthworms and Collembola on plant growth and aphid development. Oecologia 119, 541–551. doi: 10.1007/s004420050817
Schipanski, M. E., Barbercheck, M., Douglas, M. R., Finney, D. M., Haider, K., Kaye, J. P., et al. (2014). A framework for evaluating ecosystem services provided by cover crops in agroecosystems. Agric. Syst. 125, 12–22. doi: 10.1016/j.agsy.2013.11.004
Seagraves, M. P., and Lundgren, J. G. (2012). Effects of neonicitinoid seed treatments on soybean aphid and its natural enemies. J. Pest Sci. 85, 125–132. doi: 10.1007/s10340-011-0374-1
Smith, J. L., Baute, T. S., and Schaafsma, A. W. (2020). Quantifying early-season pest injury and yield protection of insecticide seed treatments in corn and soybean production in Ontario, Canada. J. Econ. Entomol. 113, 2197–2212. doi: 10.1093/jee/toaa132
Stern, V., Smith, R., van den Bosch, R., and Hagen, K. (1959). The integration of chemical and biological control of the spotted alfalfa aphid: the integrated control concept. Hilgardia 29, 81–101. doi: 10.3733/hilg.v29n02p081
Stewart, S. D., Lorenz, G. M., Catchot, A. L., Gore, J., Cook, D., Skinner, J., et al. (2014). Potential exposure of pollinators to neonicotinoid insecticides from the use of insecticide seed treatments in the mid-southern United States. Environ. Sci. Technol. 48, 9762–9769. doi: 10.1021/es501657w
Stokstad, E. (2018). European Union expands ban of three neonicotinoid insecticides. Science. doi: 10.1126/science.aau0152
Suter, G. W., and Cormier, S. M. (2015). Why care about aquatic insects: uses, benefits, and services. Integr. Environ. Assess. Manag. 11, 188–194. doi: 10.1002/ieam.1600
Tamburini, G., De Simone, S., Sigura, M., Boscutti, F., and Marini, L. (2016). Conservation tillage mitigates the negative effect of landscape simplification on biological control. J. Appl. Ecol. 53, 233–241. doi: 10.1111/1365-2664.12544
Tennekes, H. A., and Sánchez-Bayo, F. (2013). The molecular basis of simple relationships between exposure concentration and toxic effects with time. Toxicology 309, 39–51. doi: 10.1016/j.tox.2013.04.007
Tinsley, N. A., Spencer, J. L., Estes, R. E., Estes, K. A., Kaluf, A. L., Isard, S. A., et al. (2018). Multi-year surveys reveal significant decline in western corn rootworm densities in Illinois soybean fields. Am. Entomol. 64, 112–119. doi: 10.1093/ae/tmy024
Tooker, J. F., Douglas, M. R., and Krupke, C. H. (2017). Neonicotinoid seed treatments: limitations and compatibility with integrated pest management. Agric. Environ. Lett. 2, 1–5. doi: 10.2134/ael2017.08.0026
Tooker, J. F., O'Neal, M. E., and Rodriguez-Saona, C. (2020). Balancing disturbance and conservation in agroecosystems to improve biological control. Annu. Rev. Entomol. 65, 81–100. doi: 10.1146/annurev-ento-011019-025143
United State Geological Survey National Water-Quality Assessment Project. (2020). Available online at: https://water.usgs.gov/nawqa/pnsp/usage/maps/.
United States Environmental Protection Agency (2019). Aquatic Life Benchmarks and Ecological Risk Assessments for Registered Pesticides. Available online at: https://www.epa.gov/pesticide-science-and-assessing-pesticide-risks/aquatic-life-benchmarks-and-ecological-risk#benchmarks (accessed July 27, 2020).
Van den Brink, P. J., Van Smeden, J. M., Bekele, R. S., Dierick, W., De Gelder, D. M., Noteboom, M., et al. (2016). Acute and chronic toxicity of neonicotinoids to nymphs of a mayfly species and some notes on seasonal differences. Environ. Toxicol. Chem. 35, 128–133. doi: 10.1002/etc.3152
van Klink, R., Bowler, D. E., Gongalsky, K. B., Swengel, A. B., Gentile, A., and Chase, J. M. (2020). Meta-analysis reveals declines in terrestrial but increases in freshwater insect abundances. Science 368, 417–420. doi: 10.1126/science.aax9931
Verberk, W. C., Durance, I., Vaughan, I. P., and Ormerod, S. J. (2016). Field and laboratory studies reveal interacting effects of stream oxygenation and warming on aquatic ectotherms. Glob. Change Biol. 22, 1769–1778. doi: 10.1111/gcb.13240
Veres, A., Wyckhuys, K. A. G., Kiss, J., Tóth, F., Burgio, G., Pons, X., et al. (2020). An update of the Worldwide Integrated Assessment (WIA) on systemic pesticides. Part 4: alternatives in major cropping systems. Environ. Sci. Pollut. Res. 27, 29867–29899. doi: 10.1007/s11356-020-09279-x
Wettstein, F. E., Kasteel, R., Garcia Delgado, M. F., Hanke, I., Huntscha, S., Balmer, M. E., et al. (2016). Leaching of the neonicotinoids thiamethoxam and imidacloprid from sugar beet seed dressings to subsurface tile drains. J. Agric. Food Chem. 64, 6407–6415. doi: 10.1021/acs.jafc.6b02619
Wolfram, J., Stehle, S., Bub, S., Petschick, L. L., and Schulz, R. (2018). Meta-analysis of insecticides in United States surface waters: status and future implications. Environ. Sci. Technol. 52, 14452–14460. doi: 10.1021/acs.est.8b04651
Wood, T. J., and Goulson, D. (2017). The environmental risks of neonicotinoid pesticides: a review of the evidence post 2013. Environ. Sci. Pollut. Res. 24, 17285–17325. doi: 10.1007/s11356-017-9240-x
Wu, C. H., Lin, C. L., Wang, S. E., and Lu, C. W. (2020). Effects of imidacloprid, a neonicotinoid insecticide, on the echolocation system of insectivorous bats. Pest. Biochem. Physiol. 163, 94–101. doi: 10.1016/j.pestbp.2019.10.010
Wyckhuys, K. A., Lu, Y., Zhou, W., Cock, M. J., Naranjo, S. E., Fereti, A., et al. (2020). Ecological pest control fortifies agricultural growth in Asia–Pacific economies. Nat. Ecol. Evol. 4, 1522–1530. doi: 10.1038/s41559-020-01294-y
Keywords: aquatic insects, corn, maize, neonicotinoid, non-target effects, seed treatment
Citation: Krupke CH and Tooker JF (2020) Beyond the Headlines: The Influence of Insurance Pest Management on an Unseen, Silent Entomological Majority. Front. Sustain. Food Syst. 4:595855. doi: 10.3389/fsufs.2020.595855
Received: 17 August 2020; Accepted: 06 November 2020;
Published: 09 December 2020.
Edited by:
Kris A. G. Wyckhuys, Chinese Academy of Agricultural Sciences (CAAS), ChinaReviewed by:
Francisco Sánchez-Bayo, The University of Sydney, AustraliaGenevieve Labrie, Centre de Recherche Agroalimentaire de Mirabel, Canada
Michael Bredeson, Ecdysis Foundation, United States
Copyright © 2020 Krupke and Tooker. This is an open-access article distributed under the terms of the Creative Commons Attribution License (CC BY). The use, distribution or reproduction in other forums is permitted, provided the original author(s) and the copyright owner(s) are credited and that the original publication in this journal is cited, in accordance with accepted academic practice. No use, distribution or reproduction is permitted which does not comply with these terms.
*Correspondence: Christian H. Krupke, Y2tydXBrZUBwdXJkdWUuZWR1