- Mycorrhizal Research Laboratory, Department of Biochemistry and Microbiology, Rhodes University, Grahamstown, South Africa
The potato (Solanum tuberosum L.) is an important tuber crop with high dietary value that could potentially help to alleviate malnutrition and hunger in Africa. However, production is expensive, with high fertilizer and pesticide demands that lead to environmental pollution, and tillage practices that negatively affect soil structure. Microorganisms of different types have increasingly been found to be useful as biofertilizers, and arbuscular mycorrhizal (AM) fungi are an important crop symbiont. AM fungi have been shown to increase tolerance of crop plants to drought, salinity and disease by facilitating water and nutrient acquisition and by improving overall soil structure. However, the establishment and maintenance of the symbioses are greatly affected by agricultural practices. Here, we review the benefits that AM fungi confer in potato production, discuss the role and importance of mycorrhiza helper bacteria, and focus on how AM fungal diversity and abundance can be affected by conventional agricultural practices, such as those used in potato production. We suggest approaches for maintaining AM fungal abundance in potato production by highlighting the potential of conservation tillage practices augmented with cover crops and crop rotations. An approach that balances weed control, nutrient provision, and AM fungal helper bacterial populations, whilst promoting functional AM fungal populations for varying potato genotypes, will stimulate efficient mycorrhizal interventions.
Introduction
The potato (Solanum tuberosum L.), also known as the “Hidden Treasure,” is the most important non-cereal crop in the world (Ezekiel et al., 2013). In Africa, it is ranked 4th after wheat, maize and rice (Ezekiel et al., 2013; Dreyer, 2017). According to FAOSTAT (2020a), potato production within the continent rose by 90% between 2002 and 2018 (Figure 1), spanning 42 African countries on an estimated 1.9 million ha of arable land (FAOSTAT, 2020a). This contrasts with Europe, where there has been a downward trend in potato production from 221 million tons in 1961 to 105 million tons in 2018 (FAOSTAT, 2020a). In Africa, potato production is carried out by small-holder and commercial farmers, for household consumption and exportation, respectively (Gildemacher et al., 2009; Haverkort et al., 2013). The production and processing of potatoes provides employment for many in Africa, whilst the capacity to export them offers opportunities to earn foreign currency for economic development. In developing countries such as those in Africa, potatoes are mainly produced for their carbohydrate content. Potatoes can provide 130 Kcal of energy per individual every day (Ezekiel et al., 2013) with 100 g of baked potato (with its skin) providing 390 kJ of energy (Zaheer and Akhtar, 2016). Since one plant can produce many tubers, the potato provides more energy per plant compared to other staple crops (Ezekiel et al., 2013). The potato also contains no fat but has a considerable amount of protein, which is necessary for human growth and development (Wijesinha-Bettoni and Mouillé, 2019). The protein content is, however, dependent on variety, there being over 5000 potato varieties with varying degrees of nutritional value (Burlingame et al., 2009). This genetic diversity facilitates successful cultivation of potatoes in various agro-ecological regions across the continent (Dreyer, 2017), making it unique compared to other less diverse crops (Pathak et al., 2017).
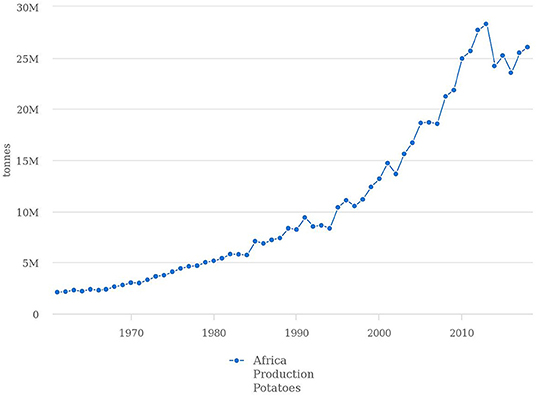
Figure 1. Potato production from 1961–2018, which shows an increased demand over the years. In 2018, 26,041,721 tons of potatoes were produced. The most dramatic change of 90% was realized between 2002 and 2018 (FAOSTAT, 2020a).
Africa is home to 260 million undernourished people, 90% being in Sub-Saharan Africa (FAO, 2019). Potatoes provide vitamins C, B6 and E, iron (Fe), magnesium (Mg), phosphorus (P), potassium (K) and thiamine, which are all vital for human nutrition (Andre et al., 2014; Zaheer and Akhtar, 2016). They provide dietary fiber (through the potato skin), carotenoids, flavonoids, folate and phenolic compounds (Burlingame et al., 2009; Zaheer and Akhtar, 2016). These confer anti-diabetic and anti-cancer properties, in addition to helping to combat chronic skin inflammations and hepatic damage. They have also been reported to reduce the toxicity of cholesterol oxidation products, the incidence of hypertension, heart diseases and neurodegenerative diseases, among others (Duthie et al., 2000; Singh et al., 2008; Burlingame et al., 2009; Arun et al., 2015; Yang et al., 2015). As a major component of the diets of many people of Africa and others worldwide, the potato can contribute toward the Sustainable Development Goal 2, which strives to “End hunger, achieve food security and improve nutrition and promote sustainable agriculture” by 2030 (FAO et al., 2019).
Various initiatives have been set up to promote efficient and high yield potato production, particularly in sub-Saharan Africa. Prominent among them is the CGIAR project for Root, Tuber and Banana (RTB) research, which was initiated to provide high-quality seed potato for planting (Harahagazwe et al., 2018). Other initiatives include non-profit organizations such as the African Potato Association and Potatoes South Africa.
Production of large quantities of high-quality potatoes to meet these goals requires the intensive application of fertilizers and pesticides. This threatens biodiversity by selecting for certain organisms at the expense of others, and human health due to point source pollution, particularly where runoff can reach water sources. Thus, current potato production systems are unsustainable with the expansion of Africa's population (United Nations Department of Economic Social Affairs Population Division., 2017). However, the soil already contains a diversity of beneficial microorganisms that can facilitate plant development through their role as biofertilizers (Barea et al., 2005), including bacteria, protozoa and fungi (Barea et al., 2005), many of which offer much more sustainable solutions. Primary amongst these are AM fungi, as they are major crop symbionts, however, as with all living organisms, they too are affected by human activity. An understanding of AM fungal symbiosis, and adaptation of management practices to ensure AM fungal biodiversity, is key to accruing their many invaluable agricultural benefits. This paper reviews current advances in understanding regarding the benefits that AM fungi can provide in potato production and discusses the maintenance of AM fungi through a more conservative agricultural approach for sustainable potato production.
Arbuscular Mycorrhizal Fungi
Arbuscular mycorrhizal (AM) fungi are ubiquitous soil microbes forming obligatory biotrophic symbiotic associations with the roots of more than 80% of all vascular plant families (Besserer et al., 2006). Environmental history places their existence from the Ovicadan era and they are believed to have been the facilitators of land invasion by plants (Redecker et al., 2000). These fungi belong to the phylum Glomeromycota and order Glomerales (Pathak et al., 2017). AM fungal species are functionally diverse and their ability to form symbioses is largely dependent on their ability to acquire food from the host, whether they confer the required benefits to the host and whether the host genotype allows AM fungi to complete their life cycles (Feddermann et al., 2010; Pathak et al., 2017).
AM fungi are known to be ancient asexual organisms. The hyphae are aseptate and coenocytic (Parniske, 2008). They undergo anastomosis, which is the association of hyphae followed by genetic reshuffling that results in the development of new progeny (Chagnon, 2014). Anastomosis occurs intraspecifically and between related species (Parniske, 2008; Chagnon, 2014). It has been suggested that AM fungi can reproduce sexually, however, scientific research should be undertaken to validate this claim (Yildirir et al., 2020).
The AM fungal life cycle is composed of 3 main stages, namely the asymbiotic, presymbiotic and symbiotic stages.
Asymbiotic Stage
AM fungi begin as resting spores, which, depending on species, can be from 50–100 μm (or more) in diameter with thick cell walls. They lie in a conservatory state awaiting a host. Though resting, it has been proven that many metabolic and morphological processes occur during this stage. If the spores have the right moisture content and are under optimum temperatures, they can germinate for 2–3 weeks. They achieve this by utilizing their carbohydrate and triacylglyceride energy reserves (Requena et al., 2007). It was experimentally identified that trehalose is the main carbohydrate found in AM fungal spores and this is utilized during the first 5 days of spore germination, followed by the subsequent utilization of lipids (Bécard et al., 1991). During germination, the hyphae develop, forming germ tubes (Requena et al., 2007) (Figure 2). Numerous nuclei formed by cell division can be observed under the microscope at this stage (Bonfante and Perotto, 1995). If no host is recognized, germination arrests and the cytoplasm retracts with consequential hyphal retraction (Requena et al., 2007). This arrest occurs before the food reserves have been depleted, allowing the spores to proceed with germination a few more times to increase their chances of finding a host (Bago et al., 2000).
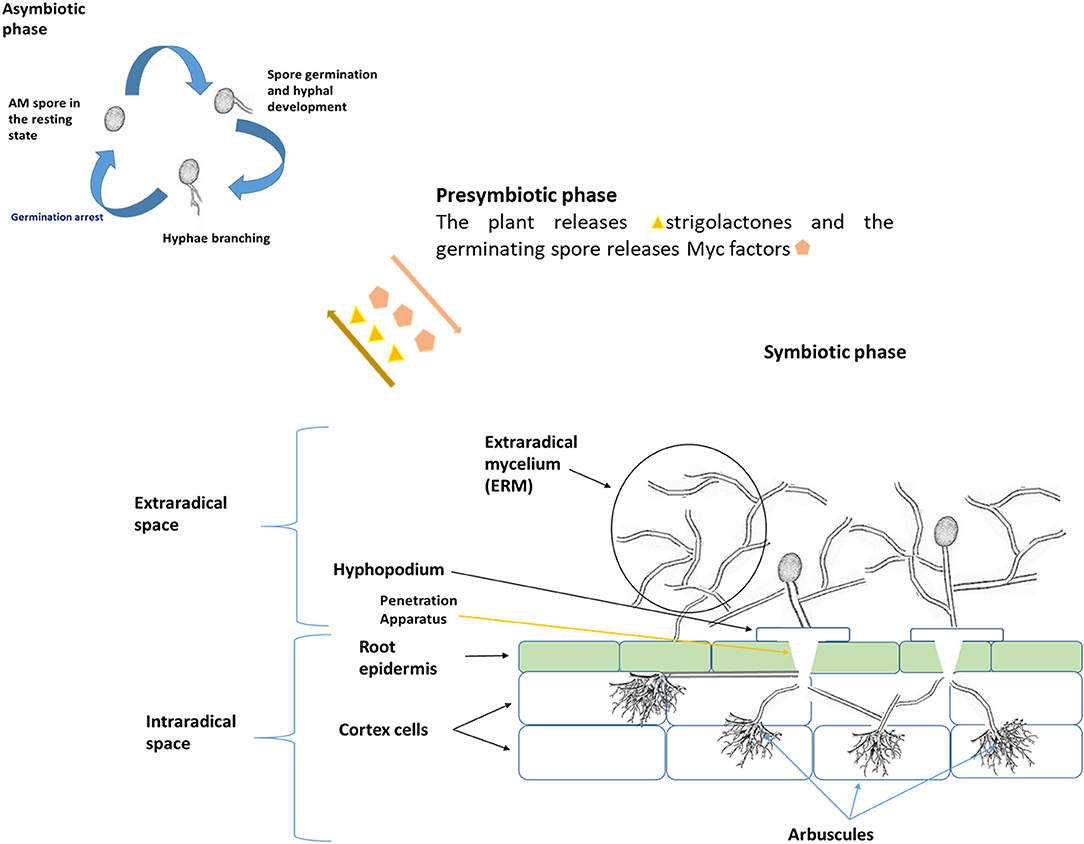
Figure 2. The asymbiotic stage involves spore germination without host associations. The spore utilizes stored reserves of trehalose and lipids to extend its hyphae. In the absence of a host, the hyphae retract, and the spore returns to its resting state. However, when in proximity to a symbiotic host, the spore with extending hyphae moves into the pre-symbiotic stage. In this stage, to encourage further hyphal branching, the plant exudes stigolactones (yellow triangles). In turn, AM fungi release Myc factors (pink pentagons). These chemicals prepare both the plant and AM fungi for association. In the symbiotic phase, the AM fungal hyphae develop a hyphopodium that allows it to rest on the plant root epidermal cells. The development of a PPA in the epidermal cells creates an invagination which guides the growing hyphae past the epidermis into the cortex cells. From the cortex cells, the hyphae branch into tree-like structures called arbuscules. It is through these arbuscules that nutrients are delivered past the periarbuscular membrane where all nutrient transporters are present.
Presymbiotic Stage
Once the spore or plant roots with spores are near a host plant's roots, various genetic, metabolic and signaling cascades are initiated. The plant releases the phytohormone strigolactone, which triggers AM fungal activity. In turn, AM fungi release lipochito-oligosaccharides commonly referred to as Myc factors (Harrison, 2012). These chemicals initiate an AM fungi-host genetic and metabolic coordination which encourages hyphal differentiation and extension toward the host roots (Chen et al., 2018).
Symbiotic Phase
After the presymbiotic phase the hypha forms an appressorium, called the hyphopodium, on root epidermal cells (Parniske, 2008). The root epidermal cells begin an internal rearrangement process, pushing the nucleus to one corner and initiating the formation of a penetration apparatus (PPA) that induces invagination of the epidermal cell right below the hyphopodium (Siciliano et al., 2007). The PPA, consisting of the endoplasmic reticulum and microtubules, surrounds this invagination (Siciliano et al., 2007). The Myc factors likely influence the development of the PPA (Harrison, 2012). The hypha moves into the cortex cell through this invagination. Pumplin et al. (2010) and Feddermann et al. (2010) reported that Myc factors also induce the expression of Vapyrin and PAM1 genes that allow cortex cell penetration. As the hypha continues to penetrate, it branches off into tree-like structures called the arbuscules (Parniske, 2008) (Figure 2). The cell wall of AM fungus is separated from the plant cell firstly by the periabuscular space, followed by the periarbuscular membrane (PAM) (Parniske, 2008). VAMP721d and VAMP721e proteins have been identified to play a major role in PAM development (Ivanov et al., 2012). This AM fungal and plant symbiosis induces the lysolipid lyso-phosphatidylcholine that in turn induces the expression of various transporter genes, such as the Solanum tuberosum Phosphate transporter 3 and 4 (StPT3 and StPT4), for phosphate transport on the PAM (Drissner et al., 2007). In the symbiotic exchange, AM fungi acquire carbon and lipids for their own development. Continuous symbiosis leads to AM fungal growth and sporulation.
Symbiotic Benefits
Enhanced Nutrient Uptake, Plant Growth, and Yield
Potato production relies heavily on the consistent use of fertilizers to provide all the 3 major nutrients, potassium (K), nitrogen (N), and phosphorus (P) (Jenkins and Mahmood, 2003). Lack of P results in stunted growth whilst a lack of N and K result in reduced tuber yield (Potatoes South Africa, 2020). Most researchers report that P is the more rate-limiting nutrient, as it influences the uptake of nutrients such as N (nitrogen uptake stops within days of P deficiency), iron (Fe), zinc (Zn), and magnesium (Mg) (Rufty et al., 1990; Skinner and Matthews, 1990; Fageria, 2001). Regardless of relative importance, all macronutrients diffuse slowly in the soil toward plant root hairs, creating a zone of depletion (Shuab et al., 2017). This coupled with the fact that potato plants have sparse and shallow root hair systems, results in a lack of optimum nutrients at any given time unless heavy fertilization ensues (Yamaguchi, 2002). The drawback is that fertilizers are expensive and often not available to the small-scale or subsistence farmers. In addition, heavy fertilizer application does not always guarantee high mineral nutrient efficiency (Brentrup and Pallière, 2010). AM fungi, on the other hand, can, through their extraradical mycelium, reach soil depths which root hairs cannot (Davies et al., 2005). Their thin hyphae increase the surface area for absorption and cover the gap between the potato root hairs and a nutrient sufficient zone (Davies et al., 2005). Under soil nutrient limiting conditions, AM fungi increase nutrient uptake and make it available to the plant from the arbuscular apoplast via the expression of numerous transporters located on the PAM (Genre et al., 2008). AM fungi are capable of absorbing P and presenting it as the more soluble form, orthophosphate (Pi, H2), which the potato plant can absorb through AM fungal induced expression of the StPT3 gene (Rausch et al., 2001; Smith and Smith, 2011). This absorption is facilitated by H+ATPase activity (Gianinazzi-Pearson et al., 2000). AM fungi can also assist the potato in acquiring inorganic N present in the soil in the form of and through the nitrate reductase pathway and glutamine synthetase pathways, respectively (Jin et al., 2005; Shuab et al., 2017). They can also assimilate amino acids, such as arginine, via their extraradical mycelium and transport them intracellularly, where the amino acids are broken down via these pathways to make available N (Jin et al., 2005). Copper (Cu), Zn, Mg, Fe, and K are also readily absorbed in AM plants (Shuab et al., 2017). The result of this nutrient absorption is enhanced potato growth and development. Inoculations with Rhizophagus irregularis and Glomus proliferum (*Rhizophagus prolifer–currently accepted AM fungal generic and species names according to Index Fungorum are shown in brackets were applicable) were shown to increase potato biomass, tuber ratio, tuber size and potato plant growth in low P conditions (Davies et al., 2005; Liu et al., 2018). Increased tuber size, which translated to increased yields over two seasons in mycorrhizal inoculated potato, was also observed (McArthur and Knowles, 1993; Douds et al., 2007). Potato tuber diameter, root length and shoot P content, as well as root and shoot fresh weight, increased when inoculated with G. intraradices (R. intraradices) and G. mosseae (Funneliformis mosseae) (Lone et al., 2015). Similar results were observed by Vosátka and Gryndler (2000). The ability of AM fungi to assimilate nutrients lessens the requirement for fertilizer use.
Microtubers are becoming the most common potato planting material. AM fungi have been found to have a positive effect on their growth and development, increasing microtuber fresh weight, which reduces transplantation loses (Cheng et al., 2008). Once in soil substrate inoculated with AM fungi, the developed minituber produces an increased tuber number regardless of whether the plants are potted or field-grown (Cheng et al., 2008). The AM fungal association, therefore, increases seed potato production (Nurbaity et al., 2019). AM fungi continue to influence plant growth even after the fungi die, as fungal necromass has been shown to stimulate increases in crop biomass (Jansa et al., 2020). It has been suggested that this may be caused by active AM fungal biomolecules present in the necromass and/or the stimulation of other plant development promoting microorganisms within the soil (Jansa et al., 2020).
Successful use of AM fungi in potato production was demonstrated in 231 field trials in Europe and North America (Hijri, 2016). Inoculation of potato seed with Rhizophagus irregularis DAOM 197198 produced a marketable yield of 42.2 tons/ha compared to 38.2 tons/ha in the uninoculated control, thus improving yield by 3.9 tons/ha (Hijri, 2016). However, the ability of the potato to access nutrients and to grow and produce high yields depends largely on the type of mycorrhiza and whether the inoculum is a pure strain or a mixture of strains (Cheng et al., 2008). In the Ecuadorian Andes, inoculation with Rhizophagus irregularis DAOM 197198 showed no significant impact on yield (Loján et al., 2017). This highlights the particular importance of AM fungal biogeography. Effective functionality is also highly influenced by inoculation techniques, interaction with indigenous background AM fungi and soil nutrient status, all of which may affect yield potential of potato crops significantly (Loján et al., 2017).
Tolerance to Salinity
Soil salinity is determined by the concentration of soluble salts in the soil (Kapoor et al., 2013), and ions which contribute to high salinity include sodium ions (Na+), calcium ions (Ca2+), chloride ions (Cl−), bicarbonate anions (), carbonate ions (), strontium ions (Sr 2+), silicon dioxide (SiO2), molybdenum (Mo), and barium ions (Ba2+), with NaCl being the most soluble salt (Hu and Schmidhalter, 2002; Munns and Tester, 2008; Kapoor et al., 2013). Sources of these salts mainly include ocean deposits and the weathering of rocks, as well as some sources of irrigation water (Munns and Tester, 2008). It is undeniable that water sources such as dams may also contain these salts in high concentrations, as many are close to industries where industrial effluent is not adequately purified before deposition into water bodies (Woolard and Irvine, 1995). Across Africa, farmers rely on irrigation and rainfall for potato production (Hijmans, 2003). Reliance on irrigation poses a threat because of the potential introduction of excessive salts into fields if the water is polluted, in addition to the accumulation of salts contributed by leachate post excessive fertilizer application (Han et al., 2015; Parihar et al., 2015).
The osmotic imbalance induced by high salinity results in stunted growth, leading to a 20% decrease in yield in irrigated crops (Porcel et al., 2012). Salinity reduces photosynthesis, leads to cellular dehydration, nutritional disorders and alters protein and lipid metabolic processes (Evelin et al., 2012). These lead to increased senescence, early plant death, reduced shoot and root length, increased radical oxygen species and an overall reduction in biomass in the potato plant (Khenifi et al., 2011; Jaarsma et al., 2013). AM fungi have been shown in previous experiments to relieve plants of salinity stress by increasing osmotic regulation through plant accumulation of osmolytes such as internal metabolites (proline, betaines) and sugars (fructose, trehalose) to prevent salt dehydration (Porcel et al., 2012; Kapoor et al., 2013). As examples, inoculation with Claroideoglomus etunicatum resulted in increased proline levels in maize roots under high salt conditions (Estrada et al., 2013b), and inoculation of Zea mays with R. intraradices led to increased stomatal conductance and reduced electrolyte leakage, which promoted photosynthesis (Estrada et al., 2013a). Under salt stress, AM fungi can increase uptake of N and Mg, where the latter is an important component of the chlorophyll molecule (Kapoor et al., 2013). It can also maintain Na+:K+ leaf ratios, which are vital for photosynthesis (Evelin et al., 2019). Xu et al. (2018) showed that Glomus tortuosum (Sieverdingia tortuosa) in Zea mays increased chlorophyll content and stomatal conductance of the plant, which improved photosynthesis under saline conditions. AM fungi can selectively remove Na+ and compartmentalize it in vacuoles (Porcel et al., 2016). The ability of Claroideoglomus etunicatum to induce NaCl compartmentalization was also observed in rice, and this led to reduced shoot Na+ levels (Porcel et al., 2016). In addition, Glomus intraradices (Rhizophagus intraradices) was shown to increase the supply of macro and micro-nutrients, whilst avoiding the uptake of NaCl ions (Evelin et al., 2012). Uptake of P increases uptake of micronutrients such as Zn and Cu, and with Calcium (Ca), reduces damage to DNA by Reactive Oxygen Species (ROS) attack, and as these nutrients are vital components of molecular processes (Liu et al., 2000; Kapoor et al., 2012), thus increase plant biomass. The host plant upregulates strigolactone production which enhances AM fungal symbiosis and consequentially overall stress alleviation (Aroca et al., 2013).
Tolerance to Drought
Increased temperatures attributable to global climate change have led to persistent drought spells in Africa, and thus to increased crop losses. This is of concern as the potato plant does not thrive under hot and dry conditions. This is because water makes up almost 90–95% of the plant tissue, whilst the tubers comprise 70–85% water (Potatoes South Africa, 2020). Averaging at 60 cm, the relatively small potato root system renders it unable to adapt to drought (Haverkort and Verhagen, 2008). Water is essentially required at every developmental stage of the potato (Potatoes South Africa, 2020), making the crop heavily reliant on irrigation and rainfall. It has been predicted that, without any climatic mitigation, potato yields will decline by ~50% across Africa by 2055 (Raymundo et al., 2018).
Drought stress results in tuber deformation, early senescence, and reduced growth yields (Potatoes South Africa, 2020). While water deficiency also interferes with the AM fungal cell cycle, some species tolerate water-deficient environments and can thrive (Stahl and Christensen, 1991). Plants tend to release strigolactone when under drought stress, and this acts as a signal to AM fungi (Aroca et al., 2013). Under drought conditions, AM fungi, through their hyphae, can extend into 100 m g−1 of soil and scavenge for water and nutrients, subsequently making it available to the plant (Evelin et al., 2019). AM fungal interactions stimulate adventitious root development by reducing root tip meristem activity (Bahadur et al., 2019). This improved root architecture together with AM fungal hyphae increases water and nutrient availability, which stimulates plant growth. As water deficiency causes osmotic stress that limits cellular expansion for growth, the accumulation of osmolytes can also relieve this stress (Bahadur et al., 2019). The accumulation of proline was noted in potatoes after inoculation with R. intraradices and C. etunicatum (Khosravifar et al., 2020). Reduced leaf water potential and increased tuber yield were also noted in this study. Other mechanisms of AM fungal induced drought tolerance include increased conductivity and transpiration, both of which improve water uptake (Kapoor et al., 2013). In addition, hormones such as abscisic acid (ABA) are released to help relieve the plant and further enhance AM symbiosis (Kumar and Verma, 2018). Inoculation of Zea mays with Rhizophagus irregularis induced plant rehydration, improved water efficiency, increased proline production, and increased root ABA production (Zhao et al., 2015; Quiroga et al., 2017). A further consequence of drought is the increased release of ROS, and AM fungi have been found to stimulate plant production of ROS scavengers and enzymatic antioxidants (Bahadur et al., 2019). Potatoes have been shown to deal with ROS through the induction of catalase (CAT), peroxidase and ascorbate peroxidase, though levels of these enzymes were highly dependent on the cultivar (Adavi et al., 2020). Fungal mycelia improve soil aggregation, which enhances water holding capacity of the soil, thus making water available to the potato root hairs (Rillig and Mummey, 2006). The contribution of AM fungi toward drought and salinity tolerance in plants is summarized in Figure 3.
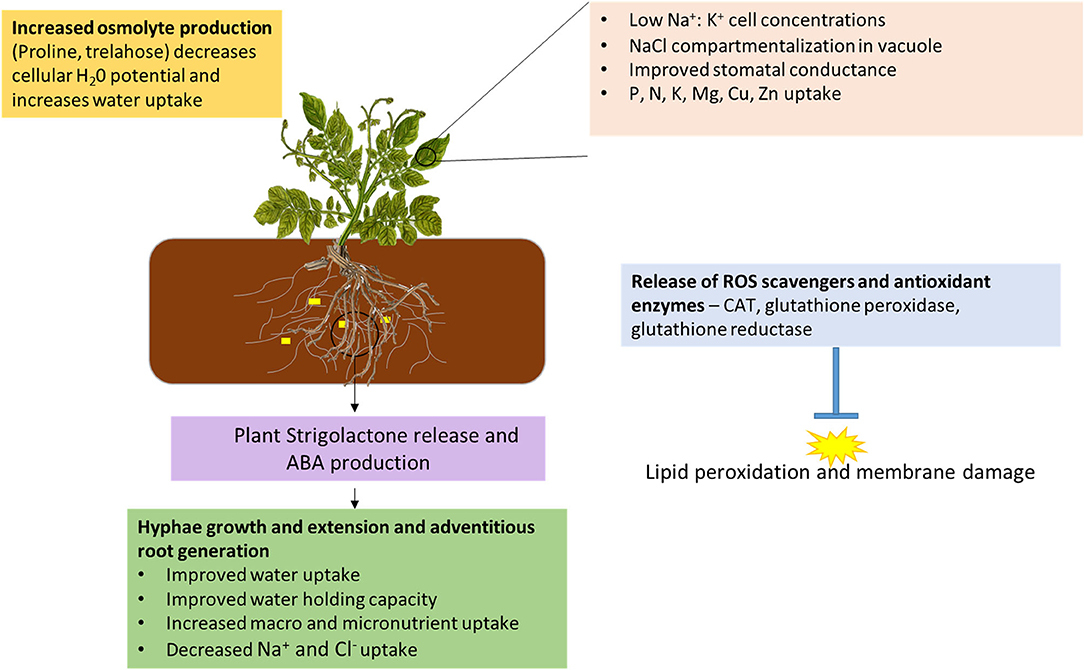
Figure 3. AM fungi mediated crop tolerance to drought and salinity. Upon drought and salinity perception in the root area, plants release strigolactone (yellow rectangles) which strengthens AM fungi-crop symbiosis. AM fungi induce ABA release by plants, which also strengthens AM fungal associations. The extending hyphae provide plants with water relief as well as nutrient uptake. Under saline conditions AM fungi further discriminate NaCl uptake. In both the roots and shoots, anti-ROS compounds and antioxidant enzymes are produced to maintain membrane integrity and stop protein, enzyme, and DNA damage, as well as alteration of plant metabolic processes. In the leaves, plants accumulate osmolytes, which lower plant osmotic potential and allow water movement from the soil into the plant. Nutrient accumulation and improved stomatal conductance increase photosynthesis and therefore plant biomass. The plant cells are also capable of compartmentalizing NaCl to reduce salinity induced drought.
Tolerance to Plant Pathogens and Diseases
The threat to potato production is a reality as increased temperatures and high humidity shorten microbial life cycles and allow potato pathogens to thrive. Potato virus Y (PVY) strain PVYNTN, Fusarium wilt, Verticillium, Late blight, Rhizoctonia, Early blight, bacterial wilt, common scab, and Erwinia blackleg are amongst the numerous viral, fungal and bacterial diseases that affect the potato, with fungal diseases being most prominent (Potatoes South Africa, 2020). The use of pesticides is costly both financially and environmentally, leading to pesticide residues and environmental pollution.
AM fungi can interfere with plant-pathogen interactions through the induction of beneficial microbe-associated molecular patterns (Van Wees et al., 2008). These involve the activation of induced systemic resistance at a mild level to prime the crop (Van Wees et al., 2008). Various hormones such as jasmonic acid, salicylic acid, ethylene and abscisic acid, auxin and toxins cross communicate to prime for resistance (Wasternack and Hause, 2013). These provide the crop with tolerance to pathogens. Such observations were made by Gallou et al. (2011) through the upregulation of Pathogenesis related 1 and 2 (PR1 and PR2) genes prior to Phytophthora infestans inoculation. “The PR1 gene is linked to the jasmonic acid pathway in Solanum species, whilst the PR2 gene expresses β-1,3-glucanase protein for the salicylic acid-dependent pathway” (Gallou et al., 2011). The Salicylate pathway triggers systemic resistance after infection with biotrophs, post initial attack, whilst the jasmonate pathway is induced by necrosis (Beckers and Spoel, 2006).
In other experiments, Fusarium dry rot, the most important postharvest potato disease caused by nine fusarium species, was reduced by 20–90% on potato minitubers in the presence of AM fungi, with effects being passed on to progeny (Niemira et al., 1996; Potatoes South Africa, 2020). The ability of AM fungi to reduce postharvest loses is critical. Fusarium species are also known to result in the production of mycotoxins which are harmful when consumed. The use of Glomus irregulare (Rhizophagus irregularis) inhibited the growth of F. sambucinum and consequential trichothecene 4, 15-diacetoxyscirpenol (mycotoxin) production, illustrating the potential use of AM fungi as an antimicrobial and anti-mycotoxin agent (Ismail et al., 2013). In a study by O'Herlihy et al. (2003), Phytophthora infestans, regarded as the most notorious potato pathogen, was reduced by growing mycorrhizal potatoes. The effects of AM fungi are not limited only to the roots, but also prevail aboveground. AM fungi colonization reduced the severity of shoot and crown rot disease caused by Rhizoctonia solani, whilst increasing nutrient uptake, tuber fresh weight and decreasing mortality (Yao et al., 2002). Similar observations were made by Larkin (2008) when a mix of AM fungi resulted in reduced stem canker and black scurf in the potato crop. AM fungi also act by alleviating H2O2 in PVY stressed potato plants (Deja-Sikora et al., 2020). They can upregulate defense mechanisms upon herbivore attack against disease vectors such as aphids (Rizzo et al., 2020), as in the case with PVY. AM fungi have also been reported to contain silencing RNA, enabling negative regulation of pathogen gene expression and thus conferring plant protection (Silvestri et al., 2020).
AM fungi associate with other microorganisms in a synergistic fight against pathogens. Pseudomonas species associated with AM fungal spores have shown inhibition of Verticillium dahliae and Erwinia carotovora, both of which are potato pathogens (Bharadwaj et al., 2008). In the rhizosphere, AM fungal interactions with rhizobacteria in potato fields have been shown to lead to the production of antibiotics and enzymes that act as antimicrobials against potato pathogens (Sessitsch et al., 2004). Baradar et al. (2015) showed that the presence of rhizobacteria and iron decreased R. solani disease progression and increased potato biomass. AM fungi also interact with other soil pathogens such as nematodes. By influencing nematode hatching, they could provide an effective way to reduce disease severity or stop infection caused by nematodes (Schouteden et al., 2015).
It seems that the response of AM fungi to stress is to improve the general health of the host plant through various processes, to enable it to fight against biotic and abiotic stress. This may be verified by the fact that systemic acquired resistance hormones also interact with auxins and gibberellins during biotic stress (Wasternack and Hause, 2013). An important factor to note, however, is that AM fungi exhibit increased effectiveness when the soil into which they are inoculated is free from disease. An experiment which combined solarization and use of AM fungi reported a reduction in diseased tubers compared to when either solarisation or AM fungi were applied alone (Ngakou et al., 2006). It is also vital to determine which AM fungal species interacts best with which potato cultivar, as these have been observed to be important variables (Yao et al., 2002). AM fungal effectiveness in disease control is also dependent on pathogen virulence and mycorrhizal potential (Azcón-Aguilar and Barea, 1997). AM fungi and the benefits they provide to the potato plant are summarized in Table 1 below.
Improved Soil Health
Soil structure is a complex encompassment of soil aggregates, pore space, as well as biota (Rillig and Mummey, 2006). AM fungi contribute to the development of soil particles by driving their hyphae into soil beds and breaking them down into smaller pieces (Miller and Jastrow, 2000). Their soil penetration allows nutrients and water to be drawn from deep within the earth (Davies et al., 2005). AM fungi do not compete for soil C as they are supplied with C as a trade-off from their symbiotic hosts, allowing soil C enrichment (Rillig, 2004). Aggregates also comprise non-organic matter, and the proportion of soil metals affect soil structure and fertility. AM fungi can play an important role in limiting the concentration of xenobiotics and heavy metals in the soil through bioremediation (Li et al., 2018). They also add to the pool of decomposing matter through their senescing hyphae (Miller and Jastrow, 2000). AM fungal interactions with other microorganisms in the rhizosphere and mycorhizosphere enrich the microbiome and increase nutrient availability in the soil, for example, by facilitating the proliferation of N2-fixing bacteria, which increases N in the soil (Jeffries et al., 2003; Johansson et al., 2004).
AM fungi release glomalin, a sticky substance that forms hydrophobic interactions with the soil particles (Miller and Jastrow, 2000; Rillig and Steinberg, 2002; Vlček and Pohanka, 2020). This causes aggregation of clay, silt and other particles in the soil and brings together nutrients and biota (Miller and Jastrow, 2000). The unique fungal extraradical mycelia act together like a net that firmly holds this enriched structure together (Rillig and Mummey, 2006). The mycelial net increases soil water holding capacity, limits leaching and indirectly improves soil aeration (Rillig and Mummey, 2006). This enriched soil structure (Miller and Jastrow, 2000) and improved soil health around the root area, induced by the presence of AM fungi, ultimately translates to healthier potato crop growth and helps to achieve maximum yield.
Soil Management and Maintaining Mycorrhizal Biodiversity
Soil Tillage
Various crop management systems significantly affect the AM fungal composition and association, shaping AM fungal communities. Tillage is a practice often used by farmers and involves the mechanical turning of soil by disc plows to de-weed, increase aeration, reduce pathogens and enhance decomposition in agricultural systems (Kabir, 2005). Tillage can be conventional or conservative (Kabir, 2005). Conventional tillage (CT) consists mainly of numerous tillage cycles which are followed by fertilizer and pesticide application (Kabir, 2005). Costa et al. (2017) demonstrated that deep tillage, which goes beyond conventional tillage by 50 cm, improves potato yield by reducing soil compaction, allowing aeration, nutrient flow and tuber expansion. Whilst this practice initially appears beneficial, it destroys soil structure long term. It affects the physical, chemical and biological cohesion associated with the AM fungal network (Wang et al., 2020). This is because AM fungi are mainly found in the topsoil where they make symbiotic associations with their hosts (Kabir, 2005). Soil disturbance can dislodge the fungi from their hosts. It interrupts the physical interaction between mycelium and soil particles. Conventional tillage reduces mycelium density as well as field glomalin (Kabir et al., 1998; Avio et al., 2013). It alters AM fungal diversity within the soil as some AM fungal species are permanently eradicated (Castillo et al., 2006; Brito et al., 2012; Säle et al., 2015). This undoubtedly shifts the microbiome, as with the movement of organic and inorganic material within the soil, macro and micronutrient supply will be diluted (Borie et al., 2006; Roger-Estrade et al., 2010).
Importantly, it appears that AM fungi are differentially vulnerable to disturbance (Brito et al., 2012). This implies that tillage induces some kind of selection pressure, and it is therefore vital to understand what degree of disturbance each tillage practice creates (Brito et al., 2012). AM fungal populations can be sustained by practicing conservation tillage, which involves less intensive, reduced tillage (RT), or no tillage at all (NT). This practice maintains crop soil surface cover, which can act as mulch for improved soil fertility (Kabir, 2005). A lack of disturbance allows AM fungi to proliferate, leading to increased hyphal lengths, spore richness, and increased glomalin concentrations (Borie et al., 2006; Säle et al., 2015).
Overall, it is important to look holistically at the advantages brought about by the practice of conservation agriculture. The success of conservation tillage is highly dependent on the ability to control weeds, pests and ensure efficient nutrient delivery (Morse, 1999). Whilst AM fungal populations are increased, there is a need to understand how integrated methods can be used to control weeds and pests. If reduced tillage is used, a type of tilling mechanism is required that does not affect AM fungi, but aims for the success of conservation agriculture (Roger-Estrade et al., 2010). Most conservation tillage systems are augmented by crop rotations, the use of cover crops and a more organic approach to farming to improve the success rate of the practice. Cover crops control weeds and can be a source of organic matter after their destruction (Morse, 1999). Some experiments utilizing RT with manure and cover crops have been shown to increase potato yield when compared to CT, without any quality compromises (Larney et al., 2016). Hou and Li (2018) demonstrated that NT combined with straw mulch increased potato marketable yield and tuber production. In another experiment, NT was carried out in low lying areas after a rice rotation and drainage (Sarangi et al., 2018). Potatoes grown on this drained paddy soil had enough moisture to sustain germination. The use of straw as cover eliminated weeds whilst the use of compost manure enriched the soil and reduced fertilizer application. The straw also served as mulch, which reduced irrigation requirements. No pesticides were used, and these methods led to the production of high-quality potato tubers (Sarangi et al., 2018).
Agrochemical Applications
Pesticide Application
A sustainable approach to agriculture requires an appreciation of the effects of pesticides and fertilizers on the environment, human health and the microbiome. Though pesticide usage in Africa is only 2%, this percentage is high considering the percentage of utilized cultivatable land (FAOSTAT, 2020b). Most pesticides are abused, often without the use of proper formulations. Fumigation affects soil microorganisms (Collins et al., 2006). Only about 0.1% of pesticides reach the target pests, whilst the rest pollutes the soil, influencing microorganism profiles including that of AM fungi (Meena et al., 2020).
There are various reports regarding the effect of pesticides on AM fungi. In a review, Hage-Ahmed et al. (2019) reported that some AM fungal spores only germinate after the active pesticide ingredient has worn off, whilst other AM fungal species seem to have developed abilities to cope with pesticide residues. However, the harmful effects of pesticides have been observed even in lower pesticide concentrations than those applied in the field (de Novais et al., 2019). Kjøller and Rosendahl (2000) and Kling and Jakobsen (1997) showed that fungicides had a deleterious effect on alkaline phosphatase and succinate dehydrogenase activity in extraradical hyphae. The malfunctioning of these enzymes affects AM survival as well as P supply to the plant. Fungicides also induce abnormal hyphal branching, reduce the hyphal length and diminish anastomosis, which is pivotal in the life cycle of AM fungi, and consequentially AM fungal -plant symbiosis (de Novais et al., 2019). In experiments involving pesticides against Rhizotonia solani, azoxystrobin affected AM fungal extraradical development and spore formation whilst flutolanil affected arbuscular formation (Buysens et al., 2015). In these experiments, the lack of spore formation hindered any sort of AM fungus–host symbiosis, whilst a lack of arbuscules compromises the plant by limiting the potential for transfer of nutrients to the plant. In the same study, however, the use of pencycuron targeted only the pathogen, with no effect on AM fungi (Buysens et al., 2015). This experiment is a clear demonstration of the different effects of the various active ingredients. Ipsilantis et al. (2012) showed that even biopesticides such as azadirachtin (extracted from neem seeds) had persistent deleterious effects in the field. This means that any antagonistic effects against other favorable soil microorganisms, such as AM fungi, should be considered during biopesticide formulation. Fungicide coated seeds of maize had no adverse effects on AM fungal colonization, suggesting that this could be a strategy for employing fungicides (depending on the mechanism of action of the fungicide) without affecting AM fungal diversity (Cameron et al., 2017).
The application of herbicides and their interaction with AM fungi can be varied. AM fungi were reported to be able to remove atrazine from the soil and relieve maize from chemical stress (Huang et al., 2007). However, when nicosulfuron was tested, the AM fungal population decreased due to a cumulative effect of the active ingredient in the soil (Karpouzas et al., 2014). Glyphosate-based herbicides were also shown to have a deleterious effect on AM fungi infection rates in maize grown in pasteurized soil (Savin et al., 2009). Therefore, the mode and the rate of application of chemicals, the active ingredient(s), the chemistry and the microbiome all have significant impacts on the maintenance of AM fungal populations (Hage-Ahmed et al., 2019).
Fertilizer Application
Only about 50% of N and about 15–20% of P fertilizers, respectively, are taken up by crops (Smil, 1999; Roy et al., 2006). This means that fertilizer is applied in excess of demand. Excessive fertilization may not affect AM fungal diversity or structure, but it limits AM fungal colonization, as the crop has sufficient available nutrients for growth (Higo et al., 2020). Savin et al. (2009) reported that applying N and P reduced AM fungal infection in maize. Reports of low AM infectivity in potatoes upon fertilizer application were also reported by Loit et al. (2018). Lin et al. (2012) showed that, contrary to initial thinking, fertilizers affected AM fungal diversity. AM fungal populations can, however, be maintained by using lower fertilizer concentrations. Ziane et al. (2017) reported that 50% NPK application and AM fungal inoculation allowed crops to reach optimal yield. Ultimately, organic farming can be used to avoid the adverse effects of synthetic fertilizers and at the same time promote AM fungal populations, as it was observed that organic farming increased microbial richness (Lupatini et al., 2017; Loit et al., 2018). In addition, pyrolysis in organic farming produces compounds that act positively on AM fungal growth and development, as well as promoting the growth of other beneficial microbes (Gryndler et al., 2009).
Monoculture, Selection of Cultivars, and Fallow Periods
Monoculture of the potato crop drastically reduces crop yield. This is because the practice severely reduces soil microbial diversity, decreasing soil fertility, and potentially increasing the incidence of disease (Liu et al., 2014). To successfully improve or maintain high crop yield, it is advisable to practice crop rotations/intercropping. Legumes encourage the proliferation of nitrogen-fixing bacteria, which increases soil N availability, whilst AM fungi associating crops influence AM fungal community composition with the aforementioned benefits. Regarding AM fungal diversity and crop rotations, it is important to note that AM fungal host compatibility depends on the host genotype and therefore differs from crop to crop (Feddermann et al., 2010; Wu et al., 2013). Arbuscular mycorrhizal response increases from grasses to legumes, then to wheat, with maize being the most responsive, whilst the non-mycorrhizal plants in the Brassicaceae and Chenopodiaceae families are unresponsive (Plenchette et al., 2005). For instance, intercropping maize–potato increases AM fungal biomass, faba-bean—potato, increases the presence of functional AM fungal species, and rotating potatoes with peas, beans and alfalfa generally increases mycorrhizal infectivity (Plenchette et al., 2005; Ma et al., 2016). On the other hand, although Trifolium pratense is used as a nitrogen-fixing crop, its rhizospheric AM fungal infectivity in potato roots is low, whilst the non-leguminous Leucanthemum vulgare Lam. promotes the growth of AM fungi that efficiently colonize potato roots (Bharadwaj et al., 2007). Therefore, the use of strongly mycorrhizal plants can increase potato yields under crop rotation. Shared diseases should also be considered in the selection of cultivars, as crop rotation with crops that share the same diseases allows disease proliferation and increases crop losses from one rotation to the next.
Fallowing is a term for the use of a resting period that allows soil recovery, and this can be undertaken after periods of monoculture. Whilst perennial farming is detrimental to the soil, it has been suggested that extensive fallow periods are often detrimental to AM fungi (Plenchette et al., 2005). This is probably because of decreased AM fungal activity for long periods in the absence of a host plant and reduced microbes to maintain AM fungal activity (Plenchette et al., 2005). However, once crops are reintroduced in the correct order, AM fungal activity can be increased. Short periods of fallowing with cover crops may also be an alternative to crop rotations and intercropping for monoculture crops, to maintain AM fungal diversity.
Mycorrhizospheric Interactions With Other Soil Microbes
Arbuscular mycorrhizal fungi influence the microbiome by changing soil pH and exuding stimulatory or inhibitory substances that create an environment for AM fungal establishment and plant colonization over time (Johansson et al., 2004; Larkin, 2008; Budi et al., 2012). Bacteria found in association with AM fungi have been isolated from AM fungal spore surfaces, hyphae and cytoplasm, with Gram-positive bacteria having strict associations with the spore surface (Lecomte et al., 2011; Cruz and Ishii, 2012; Selvakumar et al., 2016). These bacteria degrade the hyaline wall layer through the release of hydrolytic enzymes and inhibit AM fungal anti-germination compounds (Roesti et al., 2005). This facilitates spore germination (Roesti et al., 2005), initiating the AM fungus life cycle. They are also capable of forming biofilms by stacking themselves around the spore and hyphae, as well as on the surface of plant root epidermal cells, protecting the fungus and the plant (Manchanda et al., 2017). An example of this protection is the induction of bacterial aiiA gene expression against the activation of E. carotovora virulent genes in infected potato plants (Dong et al., 2000). Bacteria also exude chitinases and cellulases, which assist penetration of root epidermis by AM fungal hyphae, and confer defense against pathogens (Bonfante and Perotto, 1995; Bharadwaj et al., 2008). Examples of spore associating bacteria that conferred resistance to R. solani, E. carotovora, Verticillium dahliae and Phytophthora infestans were found on G. mosseae (Funneliformis mosseae) and G. intraradice (R. intraradices) and include Bacillus subtilis, P. putida biotype A, and S. maltophilia, among others (Bharadwaj et al., 2008). In symbiotic response to this association, AM fungi supply chitin, polysaccharides, carboxylates, formate, and acetate for bacterial growth (Toljander et al., 2007 and Selvakumar et al., 2016; Zhang et al., 2016). It was also found that AM fungi induced bacterial proteolytic activity to assist the bacteria in the utilization of amino acids (Selvakumar et al., 2016).
Arbuscular mycorrhizal fungi interact synergistically with rhizobia and other phosphate solubilising bacteria that make P available for AM fungal uptake and transport to the plant (Toro et al., 1997). The solubilising bacteria can moderate their population to accommodate AM fungi, allowing AM fungal establishment on host plants (Toro et al., 1997). Glomus mosseae (Funneliformis mosseae) and G. fasciculatum (Rhizophagus fasciculatus) interaction with P solubilizing Pseudomonas and Bacillus resulted in an overall increase in P absorption and increased potato plant chlorophyll content (Hassani et al., 2014). The P absorbed by AM fungi activates nodule formation and nitrogenase expression in N2-fixing bacteria (Barea et al., 1992). This, in turn, makes N available for plant uptake, resulting in increased plant biomass and macronutrient accumulation in the host and therefore a high carbon pool for the fungi (Barea et al., 1992) and other microorganisms. Experiments with Azotobacter DSM-281, Bacillus PTCC-1020 and Glomus intraradices (Rhizophagus intraradices) in potato production led to increased minituber number and size, which translated to increased yields (Otroshy et al., 2013). Overall, AM fungi and microbiome associations lead to crop improvement. Vosátka and Gryndler (2000) observed that Glomus fistulosum (Claroideoglomus claroideum) and Bacillus subtilis associations resulted in increased minituber numbers and total minituber weight. Glomus mosseae (Funneliformis mosseae) with P. fluorescens F140 and G. intraradices (R. intraradices) with P. fluorescens T17−4 lead to increased potato root colonization and increased potato plant biomass (Baradar et al., 2015). Strenotrophomonas maltophilia and Pseudomonas putida biotype B induced indole acetic acid production, which leads to increased potato plant growth (Bharadwaj et al., 2008). AM fungi also associate with other non-mycorrhizal saprophytic fungi and these synergize the availability of soil nutrients for plant growth and enhance plant immunity (Saldajeno and Hyakumachi, 2011). These multipartite associations greatly contribute to plant development and growth. Table 2 below is a summary of AM fungi and associated bacteria and their synergistic effect on potato development.
The ability of a plant to have and maintain multiple microbial associations maximizes the benefits accruing to the plant. To conserve these interactions, it is important to understand which type of AM fungi associate with any specific staple crop, and which microorganisms associate harmoniously with the dominant AM fungal species for each crop. It is not sufficient simply to know the existing microorganisms, but it is also necessary to evaluate which cropping systems and crop associations enhance and maintain such synergies.
Recommendations for Commercial and Small-Scale Crop Production
While the purchase of AM fungus inoculum is expensive, managing existing populations may be the best approach for acquiring benefits conferred by symbionts. Conventional practices which utilize tillage, monoculture, pesticides, and intense fertilization interfere with soil microbial dynamics, negatively affecting AM fungal symbiosis, resulting in diluted micronutrient supplies and leading to poor soil structure. Adopting conservation agricultural systems would favor AM fungal and microbial proliferation in the soil. We suggest the use of conservation systems that utilize organic fertilizer (for example seaweed extracts and animal manure), cover crops, crop rotations and no-tillage. Crops with high AM fungal responses such as maize, wheat and legumes should be utilized in crop rotations with potatoes. We also highlight that symbiosis is affected by both AM fungal and potato genotype, and thus it would not be enough to have a practice that focuses only on the AM fungal population. Thus, it would be appropriate for the potato-growing industry to collaborate with academia in researching the extent to which different conservation farming practices affect potato AM fungal populations, and thus influence functional AM fungi against potato genotypes. This would encourage natural selection for functionally beneficial AM fungi, either individually or in a consortium, for any potato genotype. Since agro-ecological zones vary across Africa, differentially influencing symbiosis, we encourage in-country research for the identification of applicable conservation agricultural practices suited to these ecological zones. Furthermore, since AM fungi and other soil microorganisms such as bacteria are interdependent, suitable conservation practices should allow greater exploitation of such interactions.
Conclusion
Potato production is vital for food security within the African continent and increasing population numbers will soon give rise to further increase in demand. This pressure will most likely result in intensified conventional farming practices. However, from this review of current research into the growing of potatoes, it is clear that the use of arbuscular mycorrhizal fungi and other potential symbionts can help to promote sustainable agriculture, whilst at the same time producing high quality and high yielding potato crops, presenting a potentially highly advantageous, less damaging and more sustainable alternative. This would be reliant on the ability to maintain mycorrhizal populations and associates, requiring the practice of conservation agriculture that will increase the diversity and populations of AM fungal species, while positively selecting for AM fungi most functional with chosen potato cultivars. This can be done using crop rotations with favorable crop species, less tillage and the use of organic manure, which will increase AM fungal proliferation and that of AM fungus associated microorganisms, among others. Ultimately, the conservation practice should be able to balance nutrient provision, weed control and water usage, whilst promoting functional AM fungi and maintaining soil microbial dynamics. To benefit from AM fungal intervention, research in Africa should focus on the individual ecological zones to identify the conservation agriculture approach and the varying potato genotypes and symbionts best suited to local conditions. Such systems employing effective mycorrhizal interventions could promote sustainable potato production across Africa.
Author Contributions
VC drafted the review and JD read, edited, and approved the review.
Conflict of Interest
The authors declare that the research was conducted in the absence of any commercial or financial relationships that could be construed as a potential conflict of interest.
Acknowledgments
The authors would like to acknowledge the support of Mrs. Margot Brooks, Rhodes University, in assisting with the editing of the manuscript. The authors are grateful to the German Academic Exchange Service (DAAD 57511427) and the Department of Biochemistry and Microbiology, Rhodes University for their support.
Abbreviations
ABA, Abscisic acid; AM, Arbuscular mycorrhizal; CT, conventional tillage; NT, no-tillage; PAM, Periarbuscular membrane; PPA, Penetration Apparatus; ROS, Reactive Oxygen Species; RT, Reduced Tillage.
References
Adavi, Z., Tadayon, M. R., Razmjoo, J., and Ghaffari, H. (2020). Antioxidant enzyme responses in potato (Solanum tuberosum) cultivars colonized with Arbuscular Mycorrhizas. Potato Res. 63, 291–301. doi: 10.1007/s11540-019-09440-1
Andre, C. M., Legay, S., Iammarino, C., Ziebel, J., Guignard, C., Larondelle, Y., et al. (2014). The potato in the human diet: a complex matrix with potential health benefits. Potato Res. 57, 201–214. doi: 10.1007/s11540-015-9287-3
Aroca, R., Ruiz-Lozano, J. M., Zamarreño, Á. M., Paz, J. A., García-Mina, J. M., Pozo, M. J., et al. (2013). Arbuscular mycorrhizal symbiosis influences strigolactone production under salinity and alleviates salt stress in lettuce plants. J. Plant Physiol. 170, 47–55. doi: 10.1016/j.jplph.2012.08.020
Arun, K. B., Chandran, J., Dhanya, R., Krishna, P., Jayamurthy, P., and Nisha, P. (2015). A comparative evaluation of antioxidant and antidiabetic potential of peel from young and matured potato. Food Biosci. 9, 36–46. doi: 10.1016/j.fbio.2014.10.003
Avio, L., Castaldini, M., Fabiani, A., Bedini, S., Sbrana, C., Turrini, A., et al. (2013). Impact of nitrogen fertilization and soil tillage on arbuscular mycorrhizal fungal communities in a Mediterranean agroecosystem. Soil Biol. Biochem. 67, 285–294. doi: 10.1016/j.soilbio.2013.09.005
Azcón-Aguilar, C., and Barea, J. M. (1997). Arbuscular mycorrhizas and biological control of soil-borne plant pathogens–an overview of the mechanisms involved. Mycorrhiza 6, 457–464. doi: 10.1007/s005720050147
Bago, B., Pfeffer, P. E., and Shachar-Hill, Y. (2000). Carbon metabolism and transport in arbuscular mycorrhizas. Plant Physiol. 124, 949–958. doi: 10.1104/pp.124.3.949
Bahadur, A., Batool, A., Nasir, F., Jiang, S., Mingsen, Q., Zhang, Q., et al. (2019). Mechanistic insights into arbuscular mycorrhizal fungi-mediated drought stress tolerance in plants. Int. J. Mol. Sci. 20:4199. doi: 10.3390/ijms20174199
Baradar, A., Saberi-Riseh, R., Sedaghati, E., and Akhgar, A. (2015). Effect of some bacteria and iron chelators on potato colonization by arbuscular mycorrhiza fungi inoculated by Rhizoctonia. Indian J. Sci. Technol. 8, 1–5. doi: 10.17485/ijst/2015/v8i19/76216
Barea, J. M., Azcon, R., and Azcón-Aguilar, C. (1992). “21 Vesicular-arbuscular mycorrhizal fungi in nitrogen-fixing systems,” in Methods in Microbiology, eds Vol. 24, eds R. Norris, D. J. Read, and A. K. Varma (London: Academic Press), 391–416.
Barea, J. M., Pozo, M. J., Azcon, R., and Azcon-Aguilar, C. (2005). Microbial co-operation in the rhizosphere. J. Exp. Bot. 56, 1761–1778. doi: 10.1093/jxb/eri197
Bécard, G., Doner, L. W., Rolin, D. B., Douds, D. D., and Pfeffer, P. E. (1991). Identification and quantification of trehalose in vesicular-arbuscular mycorrhizal fungi by in vivo13C NMR and HPLC analyses. New Phytol. 118, 547–552. doi: 10.1111/j.1469-8137.1991.tb00994.x
Beckers, G. J. M., and Spoel, S. H. (2006). Fine-tuning plant defence signalling: salicylate versus jasmonate. Plant Biol. 8, 1–10. doi: 10.1055/s-2005-872705
Besserer, A., Puech-Pagès, V., Kiefer, P., Gomez-Roldan, V., Jauneau, A., Roy, S., et al. (2006). Strigolactones stimulate arbuscular mycorrhizal fungi by activating mitochondria. PLoS Biol. 4:e226. doi: 10.1371/journal.pbio.0040226
Bharadwaj, D. P., Lundquist, P. O., and Alström, S. (2007). Impact of plant species grown as monocultures on sporulation and root colonization by native arbuscular mycorrhizal fungi in potato. Appl. Soil Ecol. 35, 213–225. doi: 10.1016/j.apsoil.2006.04.003
Bharadwaj, D. P., Lundquist, P. O., and Alström, S. (2008). Arbuscular mycorrhizal fungal spore-associated bacteria affect mycorrhizal colonization, plant growth and potato pathogens. Soil Biol. Biochem. 40, 2494–2501. doi: 10.1016/j.soilbio.2008.06.012
Bonfante, P., and Perotto, S. (1995). Tansley review No. 82. strategies of arbuscular mycorrhizal fungi when infecting host plants. New Phytol. 130, 3–21. doi: 10.1111/j.1469-8137.1995.tb01810.x
Borie, F., Rubio, R., Rouanet, J. L., Morales, A., Borie, G., and Rojas, C. (2006). Effects of tillage systems on soil characteristics, glomalin and mycorrhizal propagules in a chilean ultisol. Soil Tillage Res. 88, 253–261. doi: 10.1016/j.still.2005.06.004
Brentrup, F., and Pallière, C. (2010). “Nitrogen use efficiency as an agro-environmental indicator,” in Proceedings of the OECD Workshop on Agri-Environmental Indicators (Leysin) 23–26.
Brito, I., Goss, M. J., de Carvalho, M., Chatagnier, O., and van Tuinen, D. (2012). Impact of tillage system on arbuscular mycorrhiza fungal communities in the soil under Mediterranean conditions. Soil Tillage Res. 121, 63–67. doi: 10.1016/j.still.2012.01.012
Budi, S. W., Bakhtiar, Y., and May, N. L. (2012). Bacteria associated with arbuscula mycorrhizal spores gigaspora margarita and their potential for stimulating root mycorrhizal colonization and neem (Melia azedarach Linn) seedling growth. Microbiol. Indones. 6, 6–6. doi: 10.5454/mi.6.4.6
Burlingame, B., Mouillé, B., and Charrondiere, R. (2009). Nutrients, bioactive non-nutrients and anti-nutrients in potatoes. J. Food Comp. Anal. 22, 494–502. doi: 10.1016/j.jfca.2009.09.001
Buysens, C., De Boulois, H. D., and Declerck, S. (2015). Do fungicides used to control Rhizoctonia solani impact the non-target arbuscular mycorrhizal fungus Rhizophagus irregularis? Mycorrhiza 25, 277–288. doi: 10.1007/s00572-014-0610-7
Cameron, J. C., Lehman, R. M., Sexton, P., Osborne, S. L., and Taheri, W. I. (2017). Fungicidal seed coatings exert minor effects on arbuscular mycorrhizal fungi and plant nutrient content. Agron. J. 109, 1005–1012. doi: 10.2134/agronj2016.10.0597
Castillo, C. G., Rubio, R., Rouanet, J. L., and Borie, F. (2006). Early effects of tillage and crop rotation on arbuscular mycorrhizal fungal propagules in an ultisol. Biol. Fertil. Soils 43, 83–92. doi: 10.1007/s00374-005-0067-0
Chagnon, P. L. (2014). Ecological and evolutionary implications of hyphal anastomosis in arbuscular mycorrhizal fungi. FEMS Microbiol. Ecol. 88, 437–444. doi: 10.1111/1574-6941.12321
Chen, M., Arato, M., Borghi, L., Nouri, E., and Reinhardt, D. (2018). Beneficial services of arbuscular mycorrhizal fungi–from ecology to application. Front. Plant Sci. 9:1270. doi: 10.3389/fpls.2018.01270
Cheng, Y., Dengsha, B., Sun, L., Feldmann, F., and Gu, F. (2008). “Utilization of arbuscular mycorrhizal fungi during production of micropropagated potato Solanum tuberosum,” in Mycorrhiza Works, eds F. Feldmann, Y. Kapulnik, and J. Baar, 47–59.
Collins, H. P., Alva, A., Boydston, R. A., Cochran, R. L., Hamm, P. B., McGuire, A., et al. (2006). Soil microbial, fungal, and nematode responses to soil fumigation and cover crops under potato production. Biol. Fertil. Soils 42, 247–257. doi: 10.1007/s00374-005-0022-0
Costa, C. F., Melo, P. C., Guerra, H. P., and Ragassi, C. F. (2017). Soil properties and agronomic attributes of potato grown under deep tillage in succession of grass species. Horticul. Brasileira. 35, 75–81. doi: 10.1590/s0102-053620170112
Cruz, A. F., and Ishii, T. (2012). Arbuscular mycorrhizal fungal spores host bacteria that affect nutrient biodynamics and biocontrol of soil-borne plant pathogens. Biol. Open 1, 52–57. doi: 10.1242/bio.2011014
Davies, F. T., Calderón, C. M., and Huaman, Z. (2005). Influence of arbuscular mycorrhizae indigenous to peru and a flavonoid on growth, yield, and leaf elemental concentration of yungay'potatoes. HortScience 40, 381–385. doi: 10.21273/HORTSCI.40.2.381
de Novais, C. B., Avio, L., Giovannetti, M., de Faria, S. M., Siqueira, J. O., and Sbrana, C. (2019). Interconnectedness, length and viability of arbuscular mycorrhizal mycelium as affected by selected herbicides and fungicides. Appl. Soil Ecol. 143, 144–152. doi: 10.1016/j.apsoil.2019.06.013
Deja-Sikora, E., Kowalczyk, A., Trejgell, A., Szmidt-Jaworska, A., Baum, C., Mercy, L., et al. (2020). Arbuscular mycorrhiza changes the impact of potato virus Y on growth and stress tolerance of Solanum tuberosum L. in vitro. Front. Microbiol. 10:2971. doi: 10.3389/fmicb.2019.02971
Dong, Y. H., Xu, J. L., Li, X. Z., and Zhang, L. H. (2000). AiiA, an enzyme that inactivates the acylhomoserine lactone quorum-sensing signal and attenuates the virulence of Erwinia carotovora. Proc. Natl. Acad. Sci. U.S.A. 97, 3526–3531. doi: 10.1073/pnas.97.7.3526
Douds, D. D Jr., Nagahashi, G., Reider, C., and Hepperly, P. R. (2007). Inoculation with arbuscular mycorrhizal fungi increases the yield of potatoes in a high P soil. Biol. Agric. Horticult. 25, 67–78. doi: 10.1080/01448765.2007.10823209
Dreyer, H. (2017). Towards sustainable potato production: partnering to support family farmers in Africa. Potato Res. 60, 237–238. doi: 10.1007/s11540-018-9354-7
Drissner, D., Kunze, G., Callewaert, N., Gehrig, P., Tamasloukht, M. B., Boller, T., et al. (2007). Lyso-phosphatidylcholine is a signal in the arbuscular mycorrhizal symbiosis. Science 318, 265–268. doi: 10.1126/science.1146487
Duthie, G. G., Duthie, S. J., and Kyle, J. A. (2000). Plant polyphenols in cancer and heart disease: implications as nutritional antioxidants. Nutr. Res. Rev. 13, 79–106. doi: 10.1079/095442200108729016
Estrada, B., Aroca, R., Barea, J. M., and Ruiz-Lozano, J. M. (2013a). Native arbuscular mycorrhizal fungi isolated from a saline habitat improved maize antioxidant systems and plant tolerance to salinity. Plant Sci. 201, 42–51. doi: 10.1016/j.plantsci.2012.11.009
Estrada, B., Aroca, R., Maathuis, F. J., Barea, J. M., and Ruiz-lozano, J. M. (2013b). Arbuscular mycorrhizal fungi native from a Mediterranean saline area enhance maize tolerance to salinity through improved ion homeostasis. Plant Cell Environ. 36, 1771–1782. doi: 10.1111/pce.12082
Evelin, H., Devi, T. S., Gupta, S., and Kapoor, R. (2019). Mitigation of salinity stress in plants by arbuscular mycorrhizal symbiosis: current understanding and new challenges. Front. Plant Sci. 10:470. doi: 10.3389/fpls.2019.00470
Evelin, H., Giri, B., and Kapoor, R. (2012). Contribution of Glomus intraradices inoculation to nutrient acquisition and mitigation of ionic imbalance in NaCl-stressed Trigonella foenum-graecum. Mycorrhiza 22, 203–217. doi: 10.1007/s00572-011-0392-0
Ezekiel, R., Singh, N., Sharma, S., and Kaur, A. (2013). Beneficial phytochemicals in potato—a review. Food Res. Int. 50, 487–496. doi: 10.1016/j.foodres.2011.04.025
Fageria, V. D. (2001). Nutrient interactions in crop plants. J. Plant Nutr. 24, 1269–1290. doi: 10.1081/PLN-100106981
FAO IFAD, UNICEF, WFP and WHO. (2019). The State of Food Security and Nutrition in the World 2019. Safeguarding Against Economic Slowdowns And Downturns (Rome, FAO).
FAO. (2019). Transforming the World Through Food and Agriculture. Available online at: http://www.fao.org/3/ca5299en/ca5299en.pdf (accessed March 2020).
FAOSTAT. (2020a). Data from Crops Production. Available online at: http://www.fao.org/faostat/en/#data (accessed October 5, 2020).
FAOSTAT. (2020b). Data from Pesticides Use. Available online at: http://www.fao.org/faostat/en/#data/RP (accessed November 10, 2020).
Feddermann, N., Duvvuru Muni, R. R., Zeier, T., Stuurman, J., Ercolin, F., Schorderet, M., et al. (2010). The PAM1 gene of petunia, required for intracellular accommodation and morphogenesis of arbuscular mycorrhizal fungi, encodes a homologue of VAPYRIN. Plant J. 64, 470–481. doi: 10.1111/j.1365-313X.2010.04341.x
Gallou, A., Mosquera, H. P. L., Cranenbrouck, S., Suárez, J. P., and Declerck, S. (2011). Mycorrhiza induced resistance in potato plantlets challenged by Phytophthora infestans. Physiol. Mol. Plant Pathol. 76, 20–26. doi: 10.1016/j.pmpp.2011.06.005
Genre, A., Chabaud, M., Faccio, A., Barker, D. G., and Bonfante, P. (2008). Prepenetration apparatus assembly precedes and predicts the colonization patterns of arbuscular mycorrhizal fungi within the root cortex of both Medicago truncatula and Daucus carota. Plant Cell. 20, 1407–1420. doi: 10.1105/tpc.108.059014
Gianinazzi-Pearson, V., Arnould, C., Oufattole, M., Arango, M., and Gianinazzi, S. (2000). Differential activation of H+-ATPase genes by an arbuscular mycorrhizal fungus in root cells of transgenic tobacco. Planta 211, 609–613. doi: 10.1007/s004250000323
Gildemacher, P. R., Kaguongo, W., Ortiz, O., Tesfaye, A., Woldegiorgis, G., Wagoire, W. W., et al. (2009). Improving potato production in Kenya, Uganda and Ethiopia: a system diagnosis. Potato Res. 52, 173–205. doi: 10.1007/s11540-009-9127-4
Gryndler, M., Hršelová, H., Cajthaml, T., Havránková, M., Rezáčová, V., Gryndlerová, H., et al. (2009). Influence of soil organic matter decomposition on arbuscular mycorrhizal fungi in terms of asymbiotic hyphal growth and root colonization. Mycorrhiza 19, 255–266. doi: 10.1007/s00572-008-0217-y
Hage-Ahmed, K., Rosner, K., and Steinkellner, S. (2019). Arbuscular mycorrhizal fungi and their response to pesticides. Pest Manag. Sci. 75, 583–590. doi: 10.1002/ps.5220
Han, J., Shi, J., Zeng, L., Xu, J., and Wu, L. (2015). Effects of nitrogen fertilization on the acidity and salinity of greenhouse soils. Environ. Sci. Pollut. Res. 22, 2976–2986. doi: 10.1007/s11356-014-3542-z
Harahagazwe, D., Andrade-Piedra, J. L., Parker, M., and Schulte-Geldermann, E. (2018). Current Situation of Rapid Multiplication Techniques for Early Generation Seed Potato Production in Sub-Saharan Africa. Lima: CGIAR Research Program on Roots, Tubers and Bananas.
Harrison, M. J. (2012). Cellular programs for arbuscular mycorrhizal symbiosis. Curr. Opin. Plant Biol. 15, 691–698. doi: 10.1016/j.pbi.2012.08.010
Hassani, F., Ardakani, M., Asgharzade, A., Paknezhad, F., and Hamidi, A. (2014). Efficiency of mycorrhizal fungi and phosphate solubilizing bacteria on phosphorus uptake and chlorophyll index in potato plantlets. Int. J. Biosci. 4, 244–251. doi: 10.12692/ijb/4.1.244-251
Haverkort, A. J., Franke, A. C., Engelbrecht, F. A., and Steyn, J. M. (2013). Climate change and potato production in contrasting South African agro-ecosystems 1. Effects on land and water use efficiencies. Potato Res. 56, 31–50. doi: 10.1007/s11540-013-9230-4
Haverkort, A. J., and Verhagen, A. (2008). Climate change and its repercussions for the potato supply chain. Potato Res. 51:223. doi: 10.1007/s11540-008-9107-0
Higo, M., Azuma, M., Kamiyoshihara, Y., Kanda, A., Tatewaki, Y., and Isobe, K. (2020). Impact of phosphorus fertilization on tomato growth and arbuscular mycorrhizal fungal communities. Microorganisms 8:178. doi: 10.3390/microorganisms8020178
Hijmans, R. J. (2003). The effect of climate change on global potato production. Am. J. Potato Res. 80, 271–279. doi: 10.1007/BF02855363
Hijri, M. (2016). Analysis of a large dataset of mycorrhiza inoculation field trials on potato shows highly significant increases in yield. Mycorrhiza 26, 209–214. doi: 10.1007/s00572-015-0661-4
Hou, X., and Li, R. (2018). Potato tuber yields in semi-arid environments are increased by tillage and mulching practices. Agron. J. 110, 2641–2651. doi: 10.2134/agronj2017.10.0606
Hu, Y., and Schmidhalter, U. (2002). “Limitation of salt stress to plant growth,” in Plant Toxicology, eds B. Hock, C. F. Elstner (New York, NY: Marcel Dekker Inc), 91–224.
Huang, H., Zhang, S., Shan, X. Q., Chen, B. D., Zhu, Y. G., and Bell, J. N. B. (2007). Effect of arbuscular mycorrhizal fungus (Glomus caledonium) on the accumulation and metabolism of atrazine in maize (Zea mays L.) and atrazine dissipation in soil. Environ. Pollut. 146, 452–457. doi: 10.1016/j.envpol.2006.07.001
Ipsilantis, I., Samourelis, C., and Karpouzas, D. G. (2012). The impact of biological pesticides on arbuscular mycorrhizal fungi. Soil Biol. Biochem. 45, 147–155. doi: 10.1016/j.soilbio.2011.08.007
Ismail, Y., McCormick, S., and Hijri, M. (2013). The arbuscular mycorrhizal fungus, glomus irregulare, controls the mycotoxin production of Fusarium sambucinum in the pathogenesis of potato. FEMS Microbiol. Lett. 348, 46–51. doi: 10.1111/1574-6968.12236
Ivanov, S., Fedorova, E. E., Limpens, E., De Mita, S., Genre, A., Bonfante, P., et al. (2012). Rhizobium–legume symbiosis shares an exocytotic pathway required for arbuscule formation. Proc. Natl. Acad. Sci. U.S.A. 109, 8316–8321. doi: 10.1073/pnas.1200407109
Jaarsma, R., de Vries, R. S., and de Boer, A. H. (2013). Effect of salt stress on growth, Na+ accumulation and proline metabolism in potato (Solanum tuberosum) cultivars. PLoS ONE 8:e60183. doi: 10.1371/journal.pone.0060183
Jansa, J., Šmilauer, P., Borovička, J., Hršelová, H., Forczek, S. T., Slámová, K., et al. (2020). Dead rhizophagus irregularis biomass mysteriously stimulates plant growth. Mycorrhiza 30, 63–77. doi: 10.1007/s00572-020-00937-z
Jeffries, P., Gianinazzi, S., Perotto, S., Turnau, K., and Barea, J. M. (2003). The contribution of arbuscular mycorrhizal fungi in sustainable maintenance of plant health and soil fertility. Biol. Fertil. Soils 37, 1–16. doi: 10.1007/s00374-002-0546-5
Jenkins, P. D., and Mahmood, S. (2003). Dry matter production and partitioning in potato plants subjected to combined deficiencies of nitrogen, phosphorus and potassium. Ann. Appl. Biol. 143, 215–229. doi: 10.1111/j.1744-7348.2003.tb00288.x
Jin, H., Pfeffer, P. E., Douds, D. D., Piotrowski, E., Lammers, P. J., and Shachar-Hill, Y. (2005). The uptake, metabolism, transport and transfer of nitrogen in an arbuscular mycorrhizal symbiosis. New Phytol. 168, 687–696. doi: 10.1111/j.1469-8137.2005.01536.x
Johansson, J. F., Paul, L. R., and Finlay, R. D. (2004). Microbial interactions in the mycorrhizosphere and their significance for sustainable agriculture. FEMS Microbiol. Ecol. 48, 1–13. doi: 10.1016/j.femsec.2003.11.012
Kabir, Z. (2005). Tillage or no-tillage: impact on mycorrhizae. Canad. J. Plant Sci. 85, 23–29. doi: 10.4141/P03-160
Kabir, Z., O'halloran, I. P., Widden, P., and Hamel, C. (1998). Vertical distribution of arbuscular mycorrhizal fungi under corn (Zea mays L.) in no-till and conventional tillage systems. Mycorrhiza 8, 53–55.
Kapoor, R., Evelin, H., Mathur, P., and Giri, B. (2013). “Arbuscular mycorrhiza: approaches for abiotic stress tolerance in crop plants for sustainable agriculture,” in Plant Acclimation to Environmental Stress, eds N. Tuteja and S. Singh Gill (New York, NY: Springer), 359–401. doi: 10.1007/978-1-4614-5001-6_14
Karpouzas, D. G., Papadopoulou, E., Ipsilantis, I., Friedel, I., Petric, I., Udikovic-Kolic, N., et al. (2014). Effects of nicosulfuron on the abundance and diversity of arbuscular mycorrhizal fungi used as indicators of pesticide soil microbial toxicity. Ecol. Indic. 39, 44–53. doi: 10.1016/j.ecolind.2013.12.004
Khenifi, M. L., Boudjeniba, M., and Kameli, A. (2011). Effects of salt stress on micropropagation of potato (Solanum tuberosum L.). Afr. J. Biotechnol. 10, 7840–7845. doi: 10.5897/AJB10.982
Khosravifar, S., Farahvash, F., Aliasgharzad, N., Yarnia, M., and Khoei, F. R. (2020). Effects of different irrigation regimes and two arbuscular mycorrhizal fungi on some physiological characteristics and yield of potato under field conditions. J. Plant Nutr. 43, 2067–2079. doi: 10.1080/01904167.2020.1758133
Kjøller, R., and Rosendahl, S. (2000). Effects of fungicides on arbuscular mycorrhizal fungi: differential responses in alkaline phosphatase activity of external and internal hyphae. Biol. Fertil. Soils 31, 361–365. doi: 10.1007/s003749900180
Kling, M., and Jakobsen, I. (1997). Direct application of carbendazim and propiconazole at field rates to the external mycelium of three arbuscular mycorrhizal fungi species: effect on 32 P transport and succinate dehydrogenase activity. Mycorrhiza 7, 33–37.
Kumar, A., and Verma, J. P. (2018). Does plant—microbe interaction confer stress tolerance in plants: a review? Microbiol. Res. 207, 41–52. doi: 10.1016/j.micres.2017.11.004
Larkin, R. P. (2008). Relative effects of biological amendments and crop rotations on soil microbial communities and soilborne diseases of potato. Soil Biol. Biochem. 40, 1341–1351. doi: 10.1016/j.soilbio.2007.03.005
Larney, F. J., Pearson, D. C., Blackshaw, R. E., Lupwayi, N. Z., and Lynch, D. R. (2016). Conservation management practices and rotations for irrigated processing potato in southern alberta. Am. J. Potato Res. 93, 50–63. doi: 10.1007/s12230-015-9487-z
Lecomte, J., St-Arnaud, M., and Hijri, M. (2011). Isolation and identification of soil bacteria growing at the expense of arbuscular mycorrhizal fungi. FEMS Microbiol. Lett. 317, 43–51. doi: 10.1111/j.1574-6968.2011.02209.x
Li, J., Sun, Y., Jiang, X., Chen, B., and Zhang, X. (2018). Arbuscular mycorrhizal fungi alleviate arsenic toxicity to medicago sativa by influencing arsenic speciation and partitioning. Ecotoxicol. Environ. Saf. 157, 235–243. doi: 10.1016/j.ecoenv.2018.03.073
Lin, X., Feng, Y., Zhang, H., Chen, R., Wang, J., Zhang, J., et al. (2012). Long-term balanced fertilization decreases arbuscular mycorrhizal fungal diversity in an arable soil in North China revealed by 454 pyrosequencing. Environ. Sci. Technol. 46, 5764–5771. doi: 10.1021/es3001695
Liu, A., Hamel, C., Hamilton, R. I., Ma, B. L., and Smith, D. L. (2000). Acquisition of Cu, Zn, Mn and Fe by mycorrhizal maize (Zea mays L.) grown in soil at different P and micronutrient levels. Mycorrhiza 9, 331–336. doi: 10.1007/s005720050277
Liu, C., Ravnskov, S., Liu, F., Rubæk, G. H., and Andersen, M. N. (2018). Arbuscular mycorrhizal fungi alleviate abiotic stresses in potato plants caused by low phosphorus and deficit irrigation/partial root-zone drying. J. Agric. Sci. 156, 46–58. doi: 10.1017/S0021859618000023
Liu, X., Zhang, J., Gu, T., Zhang, W., Shen, Q., and Yin, S. (2014). Microbial community diversities and taxa abundances in soils along a seven-year gradient of potato monoculture using high throughput pyrosequencing approach. PLoS ONE 9:e86610. doi: 10.1371/journal.pone.0086610
Loit, K., Soonvald, L., Kukk, M., Astover, A., Runno-Paurson, E., Kaart, T., et al. (2018). The indigenous arbuscular mycorrhizal fungal colonisation potential in potato roots is affected by agricultural treatments. Agronomy Res. 16, 510–522. doi: 10.15159/ar.18.063
Loján, P., Senés-Guerrero, C., Suárez, J. P., Kromann, P., Schüßler, A., and Declerck, S. (2017). Potato field-inoculation in ecuador with rhizophagus irregularis: no impact on growth performance and associated arbuscular mycorrhizal fungal communities. Symbiosis 73, 45–56. doi: 10.1007/s13199-016-0471-2
Lone, R., Shuab, R., Sharma, V., Kumar, V., Mir, R., and Koul, K. K. (2015). Effect of arbuscular mycorrhizal fungi on growth and development of potato (Solanum tuberosum) plant. Asian J. Crop Sci. 7, 233–243. doi: 10.3923/ajcs.2015.233.243
Lupatini, M., Korthals, G. W., de Hollander, M., Janssens, T. K., and Kuramae, E. E. (2017). Soil microbiome is more heterogeneous in organic than in conventional farming system. Front. Microbiol. 7:2064. doi: 10.3389/fmicb.2016.02064
Ma, K., Yang, G. L., Ma, L., Wang, C. M., Wei, C. H., Dai, X. H., et al. (2016). Effects of intercropping on soil microbial communities after long-term potato monoculture. Acta Ecol Sin. 36, 2987–2995. doi: 10.5846/stxb201412072425
Manchanda, G., Singh, R. P., Li, Z. F., and Zhang, J. J. (2017). “Mycorrhiza: creating good spaces for interactions,” in Mycorrhiza-Function, Diversity, State of the Art, eds A. Varma, R. Prasad, and N. Tuteja (Cham: Springer), 39–60.
McArthur, D. A., and Knowles, N. R. (1993). Influence of species of vesicular-arbuscular mycorrhizal fungi and phosphorus nutrition on growth, development, and mineral nutrition of potato (Solanum tuberosum L.). Plant Physiol. 102, 771–782. doi: 10.1104/pp.102.3.771
Meena, R. S., Kumar, S., Datta, R., Lal, R., Vijayakumar, V., Brtnicky, M., et al. (2020). Impact of agrochemicals on soil microbiota and management: a review. Land 9:34. doi: 10.3390/land9020034
Miller, R. M., and Jastrow, J. D. (2000). “Mycorrhizal fungi influence soil structure,” in Arbuscular Mycorrhizas: Physiology and Function, eds Y. Kapulnik, and D. D. Douds Jr (Dordrecht: Springer), 3–18.
Morse, R. D. (1999). No-till vegetable production—its time is now. Horttechnology 9, 373–379. doi: 10.21273/HORTTECH.9.3.373
Munns, R., and Tester, M. (2008). Mechanisms of salinity tolerance. Annu. Rev. Plant Biol. 59, 651–681. doi: 10.1146/annurev.arplant.59.032607.092911
Ngakou, A., Megueni, C., Nwaga, D., Mabong, M. R., Djamba, F. E., and Gandebe, M. (2006). Solanum tuberosum (L.) responses to soil solarization and arbuscular mycorrhizal fungi inoculation under field conditions: growth, yield, health status of plants and tubers. Middle-East J. Sci. Res. 1, 23–30.
Niemira, B. A., Hammerschmidt, R., and Safir, G. R. (1996). Postharvest suppression of potato dry rot (Fusarium sambucinum) in prenuclear minitubers by arbuscular mycorrhizal fungal inoculum. Am. Potato J. 73, 509–515.
Nurbaity, A., Hamdani, J. S., and Rahayu, R. P. (2019). “December. Effect of arbuscular mycorrhizal fungi and different composition of growing medium on growth and production of potato seed cultivars Medians in Inceptisols Jatinangor,” in IOP Conference Series: Earth and Environmental Science, Vol. 393 (Bandung: IOP Publishing), 012052.
O'Herlihy, E. A., Duffy, E. M., and Cassells, A. C. (2003). The effects of arbuscular mycorrhizal fungi and chitosan sprays on yield and late blight resistance in potato crops from microplants. Folia Geobot. 38, 201–207. doi: 10.1007/BF02803152
Otroshy, M., Naeem, A. H., Soleymani, A., Bazrafshan, A. H., Khodaee, S. M. M., and Struik, P. C. (2013). Efficiency of plant growth-promoting rhizobacteria and fungi on enhancement of growth and minituber production of tissue-cultured potato cultivars (Solanum tuberosum). World Sci. J. 1, 38–52. Available online at: https://edepot.wur.nl/285811
Parihar, P., Singh, S., Singh, R., Singh, V. P., and Prasad, S. M. (2015). Effect of salinity stress on plants and its tolerance strategies: a review. Environ. Sci. Pollut. Res. 22, 4056–4075. doi: 10.1007/s11356-014-3739-1
Parniske, M. (2008). Arbuscular mycorrhiza: the mother of plant root endosymbioses. Nat. Rev. Microbiol. 6, 763–775. doi: 10.1038/nrmicro1987
Pathak, D., Lone, R., and Koul, K. K. (2017). “Arbuscular Mycorrhizal fungi (AMF) and plant growth-promoting Rhizobacteria (PGPR) association in potato (Solanum tuberosum L.): a brief review,” in Probiotics and Plant Health, eds V. Kumar, M. Kumar, S. Sharma, and R. Prasad (Singapore: Springer), 401–420.
Plenchette, C., Clermont-Dauphin, C., Meynard, J. M., and Fortin, J. A. (2005). Managing arbuscular mycorrhizal fungi in cropping systems. Canad. J. Plant Sci. 85, 31–40. doi: 10.4141/P03-159
Porcel, R., Aroca, R., Azcon, R., and Ruiz-Lozano, J. M. (2016). Regulation of cation transporter genes by the arbuscular mycorrhizal symbiosis in rice plants subjected to salinity suggests improved salt tolerance due to reduced Na+ root-to-shoot distribution. Mycorrhiza 26, 673–684. doi: 10.1007/s00572-016-0704-5
Porcel, R., Aroca, R., and Ruiz-Lozano, J. M. (2012). Salinity stress alleviation using arbuscular mycorrhizal fungi. a review. Agronomy Sustain. Dev. 32, 181–200. doi: 10.1007/s13593-011-0029-x
Potatoes South Africa (2020). Multimedia Guide to Potato Production in South Africa. Available online at: https://www.nbsystems.co.za/potato/ (accessed April 2020).
Pumplin, N., Mondo, S. J., Topp, S., Starker, C. G., Gantt, J. S., and Harrison, M. J. (2010). Medicago truncatula Vapyrin is a novel protein required for arbuscular mycorrhizal symbiosis. Plant J. 61, 482–494. doi: 10.1111/j.1365-313X.2009.04072.x
Quiroga, G., Erice, G., Aroca, R., Chaumont, F., and Ruiz-Lozano, J. M. (2017). Enhanced drought stress tolerance by the arbuscular mycorrhizal symbiosis in a drought-sensitive maize cultivar is related to a broader and differential regulation of host plant aquaporins than in a drought-tolerant cultivar. Front. Plant Sci. 8:1056. doi: 10.3389/fpls.2017.01056
Rausch, C., Daram, P., Brunner, S., Jansa, J., Laloi, M., Leggewie, G., et al. (2001). A phosphate transporter expressed in arbuscule-containing cells in potato. Nature 414, 462–465. doi: 10.1038/35106601
Raymundo, R., Asseng, S., Robertson, R., Petsakos, A., Hoogenboom, G., Quiroz, R., et al. (2018). Climate change impact on global potato production. Eur. J. Agronomy 100, 87–98. doi: 10.1016/j.eja.2017.11.008
Redecker, D., Kodner, R., and Graham, L. E. (2000). Glomalean fungi from the ordovician. Science 289, 1920–1921. doi: 10.1126/science.289.5486.1920
Requena, N., Serrano, E., Ocón, A., and Breuninger, M. (2007). Plant signals and fungal perception during arbuscular mycorrhiza establishment. Phytochemistry 68, 33–40. doi: 10.1016/j.phytochem.2006.09.036
Rillig, M. C. (2004). Arbuscular mycorrhizae, glomalin, and soil aggregation. Can. J. Soil Sci. 84, 355–363. doi: 10.4141/S04-003
Rillig, M. C., and Mummey, D. L. (2006). Mycorrhizas and soil structure. New Phytol. 171, 41–53. doi: 10.1111/j.1469-8137.2006.01750.x
Rillig, M. C., and Steinberg, P. D. (2002). Glomalin production by an arbuscular mycorrhizal fungus: a mechanism of habitat modification? Soil Biol. Biochem. 34, 1371–1374. doi: 10.1016/S0038-0717(02)00060-3
Rizzo, E., Sherman, T., Manosalva, P., and Gomez, S. K. (2020). Assessment of local and systemic changes in plant gene expression and aphid responses during potato interactions with arbuscular mycorrhizal fungi and potato aphids. Plants 9:82. doi: 10.3390/plants9010082
Roesti, D., Ineichen, K., Braissant, O., Redecker, D., Wiemken, A., and Aragno, M. (2005). Bacteria associated with spores of the arbuscular mycorrhizal fungi Glomus geosporum and Glomus constrictum. Appl. Environ. Microbiol. 71, 6673–6679. doi: 10.1128/AEM.71.11.6673-6679.2005
Roger-Estrade, J., Anger, C., Bertrand, M., and Richard, G. (2010). Tillage and soil ecology: partners for sustainable agriculture. Soil Tillage Res. 111, 33–40. doi: 10.1016/j.still.2010.08.010
Roy, R. N., Finck, A., Blair, G. J., and Tandon, H. L. S. (2006). Plant nutrition for food security. a guide for integrated nutrient management. FAO Fertilizer Plant Nutr. Bull. 16:368.
Rufty, T. W., MacKown, C. T., and Israel, D. W. (1990). Phosphorus stress effects on assimilation of nitrate. Plant Physiol. 94, 328–333. doi: 10.1104/pp.94.1.328
Saldajeno, M. G. B., and Hyakumachi, M. (2011). “Arbuscular mycorrhizal interactions with rhizobacteria or saprotrophic fungi and its implications to biological control of plant diseases,” in Mycorrhizal Fungi: Soil, Agriculture and Environmental Implications, ed S. M. Fulton (New York, NY: Nova Science Publishers, Inc.), 1–28.
Säle, V., Aguilera, P., Laczko, E., Mäder, P., Berner, A., Zihlmann, U., et al. (2015). Impact of conservation tillage and organic farming on the diversity of arbuscular mycorrhizal fungi. Soil Biol. Biochem. 84, 38–52. doi: 10.1016/j.soilbio.2015.02.005
Sarangi, S. K., Maji, B., Digar, S., Mahanta, K. K., Sharma, P. C., and Mainuddin, M. (2018). Zero tillage potato cultivation. Indian Farm. 68, 23–26.
Savin, M. C., Purcell, L. C., Daigh, A., and Manfredini, A. (2009). Response of mycorrhizal infection to glyphosate applications and P fertilization in glyphosate-tolerant soybean, maize, and cotton. J. Plant Nutr. 32, 1702–1717. doi: 10.1080/01904160903150941
Schouteden, N., De Waele, D., Panis, B., and Vos, C. M. (2015). Arbuscular mycorrhizal fungi for the biocontrol of plant-parasitic nematodes: a review of the mechanisms involved. Front. Microbiol. 6:1280. doi: 10.3389/fmicb.2015.01280
Selvakumar, G., Krishnamoorthy, R., Kim, K., and Sa, T. M. (2016). Genetic diversity and association characters of bacteria isolated from arbuscular mycorrhizal fungal spore walls. PLoS ONE 11:e0160356. doi: 10.1371/journal.pone.0160356
Sessitsch, A., Reiter, B., and Berg, G. (2004). Endophytic bacterial communities of field-grown potato plants and their plant-growth-promoting and antagonistic abilities. Can. J. Microbiol. 50, 239–249. doi: 10.1139/w03-118
Shuab, R., Lone, R., and Koul, K. K. (2017). Influence of arbuscular mycorrhizal fungi on storage metabolites, mineral nutrition, and nitrogen-assimilating enzymes in potato (Solanum tuberosum L.) plant. J. Plant Nutr. 40, 1386–1396. doi: 10.1080/01904167.2016.1263317
Siciliano, V., Genre, A., Balestrini, R., Cappellazzo, G., Pierre, J. G. M., and Bonfante, P. (2007). Transcriptome analysis of arbuscular mycorrhizal roots during development of the prepenetration apparatus. Plant Physiol. 144, 1455–1466. doi: 10.1104/pp.107.097980
Silvestri, A., Turina, M., Fiorilli, V., Miozzi, L., Venice, F., Bonfante, P., et al. (2020). Different genetic sources contribute to the small RNA population in the arbuscular mycorrhizal fungus gigaspora margarita. Front. Microbiol. 11:395. doi: 10.3389/fmicb.2020.00395
Singh, N., Kamath, V., Narasimhamurthy, K., and Rajini, P. S. (2008). Protective effect of potato peel extract against carbon tetrachloride-induced liver injury in rats. Environ. Toxicol. Pharmacol. 26, 241–246. doi: 10.1016/j.etap.2008.05.006
Skinner, P. W., and Matthews, M. A. (1990). A novel interaction of magnesium translocation with the supply of phosphorus to roots of grapevine (Vitis vinifera L.). Plant Cell Environ. 13, 821–826. doi: 10.1111/j.1365-3040.1990.tb01098.x
Smil, V. (1999). Nitrogen in crop production: an account of global flows. Glob. Biogeochem. Cycles 13, 647–662. doi: 10.1029/1999GB900015
Smith, S. E., and Smith, F. A. (2011). Roles of arbuscular mycorrhizas in plant nutrition and growth: new paradigms from cellular to ecosystem scales. Annu. Rev. Plant Biol. 62, 227–250. doi: 10.1146/annurev-arplant-042110-103846
Stahl, P. D., and Christensen, M. (1991). Population variation in the mycorrhizal fungus Glomus mosseae: breadth of environmental tolerance. Mycol. Res. 95, 300–307. doi: 10.1016/S0953-7562(09)81238-0
Toljander, J. F., Lindahl, B. D., Paul, L. R., Elfstrand, M., and Finlay, R. D. (2007). Influence of arbuscular mycorrhizal mycelial exudates on soil bacterial growth and community structure. FEMS Microbiol. Ecol. 61, 295–304. doi: 10.1111/j.1574-6941.2007.00337.x
Toro, M., Azcon, R., and Barea, J. (1997). Improvement of arbuscular mycorrhiza development by inoculation of soil with phosphate-solubilizing rhizobacteria to improve rock phosphate bioavailability ((sup32) P) and nutrient cycling. Appl. Environ. Microbiol. 63, 4408–4412.
United Nations Department of Economic and Social Affairs Population Division (2017). World Population Prospects: The 2017 Revision, Key Findings and Advance Tables. Working Paper No. ESA/P/WP/248. New York, NY: United Nations Department of Economic and Social Affairs Population Division.
Van Wees, S. C., Van der Ent, S., and Pieterse, C. M. (2008). Plant immune responses triggered by beneficial microbes. Curr. Opin. Plant Biol. 11, 443–448. doi: 10.1016/j.pbi.2008.05.005
Vlček, V., and Pohanka, M. (2020). Glomalin–an interesting protein part of the soil organic matter. Soil Water Res. 15, 67–74. doi: 10.17221/29/2019-SWR
Vosátka, M., and Gryndler, M. (2000). Response of micropropagated potatoes transplanted to peat media to post-vitro inoculation with arbuscular mycorrhizal fungi and soil bacteria. Appl. Soil Ecol. 15, 145–152. doi: 10.1016/S0929-1393(00)00090-1
Wang, Z., Li, Y., Li, T., Zhao, D., and Liao, Y. (2020). Conservation tillage decreases selection pressure on community assembly in the rhizosphere of arbuscular mycorrhizal fungi. Sci. Total Environ. 710:136326. doi: 10.1016/j.scitotenv.2019.136326
Wasternack, C., and Hause, B. (2013). Jasmonates: biosynthesis, perception, signal transduction and action in plant stress response, growth and development. an update to the 2007 review in Annals of Botany. Ann. Botany 111, 1021–1058. doi: 10.1093/aob/mct067
Wijesinha-Bettoni, R., and Mouillé, B. (2019). The contribution of potatoes to global food security, nutrition and healthy diets. Am. J. Potato Res. 96, 139–149. doi: 10.1007/s12230-018-09697-1
Woolard, C. R., and Irvine, R. L. (1995). Treatment of hypersaline wastewater in the sequencing batch reactor. Water Res. 29, 1159–1168. doi: 10.1016/0043-1354(94)00239-4
Wu, F., Wang, W., Ma, Y., Liu, Y., Ma, X., An, L., et al. (2013). Prospect of beneficial microorganisms applied in potato cultivation for sustainable agriculture. Afr. J. Microbiol. Res. 7, 2150–2158. doi: 10.5897/AJMR12x.005
Xu, H., Lu, Y., and Tong, S. (2018). Effects of arbuscular mycorrhizal fungi on photosynthesis and chlorophyll fluorescence of maize seedlings under salt stress. Emirates J. Food Agric. 199–204. doi: 10.9755/ejfa.2018.v30.i3.1642
Yamaguchi, J. (2002). Measurement of root diameter in field-grown crops under a microscope without washing. Soil Sci. Plant Nutr. 48, 625–629. doi: 10.1080/00380768.2002.10409248
Yang, G., Cheon, S. Y., Chung, K. S., Lee, S. J., Hong, C. H., Lee, K. T., et al. (2015). Solanum tuberosum L. cv Jayoung epidermis extract inhibits mite antigen-induced atopic dermatitis in NC/Nga mice by regulating the Th1/Th2 balance and expression of filaggrin. J. Med. Food. 18, 1013–1021. doi: 10.1089/jmf.2014.3338
Yao, M., Tweddell, R., and Desilets, H. (2002). Effect of two vesicular-arbuscular mycorrhizal fungi on the growth of micropropagated potato plantlets and on the extent of disease caused by Rhizoctoniasolani. Mycorrhiza 12, 235–242. doi: 10.1007/s00572-002-0176-7
Yildirir, G., Kokkoris, V., and Corradi, N. (2020). Parasexual and sexual reproduction in arbuscular mycorrhizal fungi: room for both. Trends Microbiol. 28, 517−519. doi: 10.1016/j.tim.2020.03.013
Zaheer, K., and Akhtar, M. H. (2016). Potato production, usage, and nutrition—a review. Crit. Rev. Food Sci. Nutr. 56, 711–721. doi: 10.1080/10408398.2012.724479
Zhang, L., Xu, M., Liu, Y., Zhang, F., Hodge, A., and Feng, G. (2016). Carbon and phosphorus exchange may enable cooperation between an arbuscular mycorrhizal fungus and a phosphate-solubilizing bacterium. New Phytol. 210, 1022–1032. doi: 10.1111/nph.13838
Zhao, R., Guo, W., Bi, N., Guo, J., Wang, L., Zhao, J., et al. (2015). Arbuscular mycorrhizal fungi affect the growth, nutrient uptake and water status of maize (Zea mays L.) grown in two types of coal mine spoils under drought stress. Appl. Soil Ecol. 88, 41–49. doi: 10.1016/j.apsoil.2014.11.016
Keywords: potato, symbiotic benefits, drought and salinity, soil management, mycorrhizospheric interactions, chemicals and fertilizers, sustainability
Citation: Chifetete VW and Dames JF (2020) Mycorrhizal Interventions for Sustainable Potato Production in Africa. Front. Sustain. Food Syst. 4:593053. doi: 10.3389/fsufs.2020.593053
Received: 09 August 2020; Accepted: 23 November 2020;
Published: 21 December 2020.
Edited by:
Duraisamy Saravanakumar, The University of the West Indies St. Augustine, Trinidad and TobagoReviewed by:
Deepranjan Sarkar, Banaras Hindu University, IndiaIfigeneia Mellidou, Hellenic Agricultural Organisation (HAO), Greece
Copyright © 2020 Chifetete and Dames. This is an open-access article distributed under the terms of the Creative Commons Attribution License (CC BY). The use, distribution or reproduction in other forums is permitted, provided the original author(s) and the copyright owner(s) are credited and that the original publication in this journal is cited, in accordance with accepted academic practice. No use, distribution or reproduction is permitted which does not comply with these terms.
*Correspondence: Joanna F. Dames, ai5kYW1lcyYjeDAwMDQwO3J1LmFjLnph