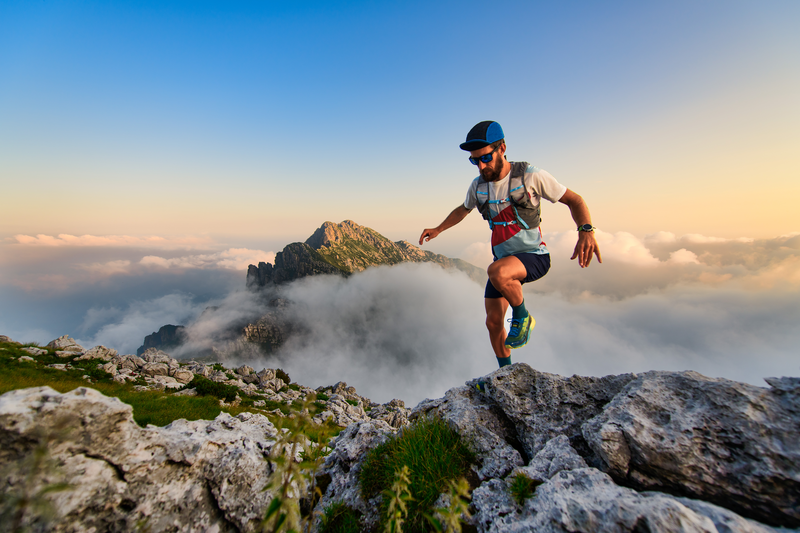
94% of researchers rate our articles as excellent or good
Learn more about the work of our research integrity team to safeguard the quality of each article we publish.
Find out more
ORIGINAL RESEARCH article
Front. Sustain. Food Syst. , 21 October 2020
Sec. Sustainable Food Processing
Volume 4 - 2020 | https://doi.org/10.3389/fsufs.2020.590597
This article is part of the Research Topic Sustainable Production of Bioactive Pigments View all 13 articles
Analysis of pulp and peels of Arabica coffee varieties with different external fruit color allowed the identification of 16 phenolic compounds using high-performance liquid chromatography with diode array detection and electrospray ionization multi-stage mass spectrometry (HPLC-DAD-ESI-MSn). Nine chlorogenic acids, three flavan-3-ols, the xanthone mangiferin, the flavonol rutin and two anthocyanins were tentatively identified and quantified. 5-O-Caffeoylquinic acid together with a putative (epi)catechin hexoside were the predominant phenolics detected in the coffee varieties analyzed in this work. Whereas, 3- and 4-caffeoylquinic acids, as well as 5-O-feruloylquinic acid were consistently found in higher quantities in the pulp than in the peels when individual varieties were compared, the opposite was found for the other phenolic compounds detected. Complementary, GC-MS after alkaline hydrolysis and trimethylsilylation permitted the identification of more than 30 constituents, including phenolic compounds and other benzenoids, caffeine, and diverse carboxylic acids. Detected anthocyanins were cyanidin-3-O-glucoside and cyanidin-3-O-rutinoside. Anthocyanin contents in orange-colored berries were lower than those in red fruits, while no anthocyanins were found in yellow-colored fruit. Among non-anthocyanin pigments, we found β-carotene and lutein in all varieties, along with other chloroplast-specific carotenoids in some accessions. In addition, saponification evidenced the presence of several xanthophyll esters. Both, chlorophyll a and b, were detected in the peels of all varieties, while only chlorophyll b was observed in the pulp. Thus, the color of yellow-peeled varieties is due to carotenoids, while that of orange and red-peeled varieties is due to both carotenoids as well as low and high levels of anthocyanins, respectively. Present results point out to the potential use of by-products of particular coffee varieties with differences in the external fruit color as a source of distinctive bioactive compounds, including anthocyanins and carotenoids, with health benefits.
By-products accruing during coffee processing represent more than 50% of the coffee fruit dry weight (Esquivel and Jiménez, 2012). Considering an estimated world production of about 171.2 million 60-kg bags for 2018/2019 (USDA, 2018), a significant amount of biomass, which has been mainly considered as “waste” material by the coffee industry, is currently discarded without further valorization. In contrast to dry coffee processing, the wet procedure usually allows the recovery of non-degraded peels and pulp (Esquivel and Jiménez, 2019), a very interesting source of valuable bioactives, whose identification and quantification have sluggishly started to promote their utilization for nutritional and pharmaceutical purposes. Taking advantage of these by-products will certainly benefit from further studies and from the development of new methodologies to improve their extraction (Esquivel and Jiménez, 2012; Heeger et al., 2017; Saini and Keum, 2018; Torres-Valenzuela et al., 2020).
Phenolic compounds and carotenoids are ubiquitous constituents of higher plants. While the profile and concentrations of the former have been studied in detail in coffee seeds and brew, knowledge about their presence in coffee by-products (peel and pulp) is more limited (reviewed by Esquivel and Jiménez, 2012). Older publications mention that coffee processing by-products contain several isomeric caffeoylquinic acids, dicaffeolyquinic acids, feruloylquinic acids, and epicatechin, considered as potent antioxidants (Ramirez-Martinez, 1988; Clifford and Ramirez-Martinez, 1991). In addition to the aforementioned constituents, caffeine, diverse quercetin glycosides (+)-catechin, and procyanidin di-, tri-, and tetra-mers have been reported in coffee husk (Mullen et al., 2013). Furthermore, the anthocyanins cyanidin-3-O-rutinoside and cyanidin-3-O-glucoside have been described (Prata and Oliveira, 2007), for which potential culinary applications have been proposed (Parra-Campos and Ordóñez-Santos, 2019). Regarding carotenoids, their contents have been only analyzed in whole berries of coffee, without separating the different fractions (i.e., pulp, peel, and bean), and their accumulation was associated with the expression of carotenoid biosynthetic genes (Simkin et al., 2010).
The chemical composition of coffee beans, used to prepare the coffee brew, varies depending on the genotype (Scholz et al., 2011; Tessema et al., 2011), which also seems to affect the composition of other fruit fractions (Ramirez-Martinez, 1988; Clifford and Ramirez-Martinez, 1991; González De Colmenares et al., 1994; Mullen et al., 2013). To the best of our knowledge, research on the compositional pattern of coffee by-products from different genotypes cultivated on the same site is scarce and has focused only on phenolic compounds (Ramirez-Martinez, 1988; Clifford and Ramirez-Martinez, 1991; Rodríguez-Durán et al., 2014), while carotenoids have not been considered. Aiming at increasing evidence about the potential use of coffee by-products as source of bioactive compounds, in this study we employed up-to-date analytical methods to characterize the phenolic compounds, including anthocyanins, as well as carotenoids in peels and pulp of five Costa Rican coffee (Coffea arabica L.) varieties growing at the same farm under the same agro-ecological conditions.
Fully ripe Coffea arabica L. berries of the varieties Caturra Rojo, Caturra Amarillo, Anaranjado, Catuaí, and Arábica were manually collected from a commercial coffee farm located in Tres Ríos, Cartago, Costa Rica (9°54′42″N 83°59′09″W). While Caturra Rojo, Catuaí, and Arábica have red peel color, the exocarp is yellow- and orange-colored in Caturra Amarillo and Anaranjado varieties, respectively. Chemical standards were obtained from Sigma Aldrich Chemie (Taufkirchen, Germany). All further reagents or solvents were purchased from VWR International (Darmstadt, Germany), at least of analytical or HPLC grade. Deionized water was used throughout.
CIE-L*a*b* color values from the peel of 10 freshly collected single fruits of each variety were measured at different positions using a colorimeter (Colorflex HunterLab, Reston, USA) operated by SpectraManager software. An observer angle of 10° and illuminant D65 were used. Hue angle () and chroma () were calculated from a* and b*.
After removing the seeds, peels and pulp were manually separated. Every sample was immediately frozen in liquid nitrogen and subsequently freeze-dried.
The protocol used for polyphenol extraction and analysis was modified from Kammerer et al. (2004), using 0.25 g of pulp and 0.5 g of freeze-dried peels. Polyphenols were extracted twice from each sample with MeOH/0.1% HCl (v/v), and the combined supernatants were evaporated to dryness in vacuo at 30°C. The residue was dissolved in 1 or 2 ml of deionized water (pH 3.0) for pulp and peels, respectively. Subsequently, samples were membrane-filtered (0.45 μm) into amber vials and stored at −20°C until analysis. For HPLC analysis, an Agilent HPLC series 1100 (Agilent, Waldbronn, Germany) equipped with ChemStation software, G1379A degasser, G1312A binary gradient pump, G1313A autosampler, G1316A column oven, and G1315B diode-array detector (DAD), was used. The separation was conducted with a Phenomenex (Torrance, CA, USA) C18 Synergi® Hydro-RP (150 × 3.0 mm i.d., 4 μm particle size) column with a C18 ODS guard column (4.0 × 2.0 mm i.d.) operated at 25°C. LC/MS analyses were performed with the above HPLC system coupled online to a Bruker Esquire 3000+ ion trap mass spectrometer (Bruker Daltonics, Bremen, Germany) operating with an ESI source in negative and positive ion mode for phenolics and anthocyanins, respectively, as detailed by Kammerer et al. (2004).
For the analysis of phenolic acids, the mobile phase consisted of a mixture of 2% (v/v) acetic acid in water (eluent A) and 0.5% acetic acid in water and MeOH (10:90, v/v; eluent B). The gradient used started with 10% eluent B increasing to 35% B in 35 min, ramping from 35 to 75% B in 20 min and 75 to 100% B in 2 min, afterwards 100% B isocratic (5 min), from 100 to 10% B (2 min), 10% B isocratic (5 min). Total run time was 69 min at a flow rate of 0.4 ml/min. The injection volume was 10 μl. Detection wavelengths were 280 and 320 nm (Kramer et al., 2012).
A mixture of 5% (v/v) formic acid (eluent A) and MeOH/water/formic acid (80/10/10, v/v/v, eluent B) was used for analysis of anthocyanins and the gradient used ramped from 10 to 14% B (5 min), 14 to 23% B (25 min), 23 to 32% B (15 min), 32 to 62% B (15 min), 62 to 100% B (5 min), 100% B isocratic (5 min), 100 to 10% B (5 min), 10% B isocratic (5 min). Total run time was 80 min. The injection volume was 4 μl. Monitoring was performed at 520 nm and a flow rate of 0.4 ml/min was used.
Compounds were quantified by integration of the peak areas at the respective wavelength using calibration curves of corresponding standard compounds or related reference compounds (5-O-caffeoylquinic acid, epicatechin, p-coumaric acid, proanthocyanidin B1, ferulic acid, and cyanidin-3-O-glucoside). Limits of detection (LOD) and limits of quantitation (LOQ) were calculated based on the regression parameters of the calibration curve. In addition, the identity of mangiferin and cyanidin-3-O-rutinoside was confirmed by using authentic standards.
Methanolic crude extracts obtained as detailed above were subjected to purification by solid phase extraction (SPE). Briefly, 0.25 g of freeze dried and milled peel and pulp, was extracted using 3 × 5 mL of MeOH/0.1% HCl. The crude extract was evaporated to dryness, re-dissolved in 1 ml of H2O and extracted with 5 × 2 mL of n-hexane for removal of lipids. The defatted extract was analyzed as detailed below or subjected to SPE as reported previously (Steingass et al., 2015).
GC-MS analysis was performed after alkaline hydrolysis of the methanolic crude extract and the SPE purified sample as detailed elsewhere (Steingass et al., 2015), including the following modifications. After the admixture of 3.5 mL of 1 M NaOH and 4.5 mg of ascorbic acid as an antioxidant (final concentration of 1%), the sample was stirred for 4 h at room temperature. Subsequently, a pH of 1.25 ± 0.1 was adjusted with 25% H2SO4, followed by liquid-liquid extraction with 3 × 2 mL of ethyl acetate. The combined ethyl acetate phase was dried with 2.5 g of Na2SO4 and evaporated to dryness. Successively, 4 × 3 mL of toluene evaporated in vacuo at 40°C was used to remove acetic acid. Then, the analytes were dissolved in 0.5 mL of water-free pyridine. An aliquot of 0.5 mL was transferred into a GC vial and 0.2 mL Sweeley reagent (10% v/v hexamethyldisilazane (HDMS): trimethylchlorosilane (TMCS) at a ratio of 2:1 v/v in pyridine) was admixed following heating to 45°C for 30 min. After centrifugation, the clear supernatant was analyzed by GC-MS.
GC-MS analysis was performed using a 6890 N gas chromatograph and a 5976 mass selective detector (both Agilent Technologies, Santa Clara, CA, USA). The injection volume applying the splitless mode was 1.0 μL. Chromatographic separation was achieved using a fused silica capillary column coated with 5% phenyl 95% polydimethylsiloxane (60 m × 0.25 mm i.d., film thickness df = 0.25 μm, HP-5 ms, Agilent J&W Columns, Santa Clara, CA, USA). The temperature program, source and transfer line temperature were set as reported previously (Steingass et al., 2015). The scan ranges were m/z 50–600 (scan frequency 2.7 Hz) between 4.6 and 35 min and m/z 50–800 (2.0 Hz) for the final segment, respectively.
Assignment of individual compounds was based on their electron impact (EI) mass spectra compared to Wiley 6N (Wiley and Sons, New York, NY, USA) and NIST 08 (National Institute of Standards and Technology, Gaithersburg, USA) libraries, linear retention indices calculated relative to n-alkanes (C8–C30), and authentic reference standards (Steingass et al., 2015).
Carotenoid extraction and HPLC-DAD-APcI-MSn analysis were performed using the instrumentation and system settings as described by Schweiggert et al. (2011).
For selected samples, saponification of carotenoid esters was performed as follows: Carotenoid extracts were evaporated to dryness in vacuo at 25°C, re-dissolved in 50 mL of petroleum ether, and subsequently 50 ml of methanolic 10% (w/v) KOH were added. The solutions were maintained in agitation at 300 rpm overnight under nitrogen atmosphere. After saponification, the organic phase was separated, washed twice with deionized water, evaporated to dryness, and prepared for HPLC analysis according to Schweiggert et al. (2011).
Identification of carotenoids was performed by comparing their UV/Vis and APcI(+)-MS1 spectra with data published previously (Britton, 1995; de Rosso and Mercadante, 2007; Maroneze et al., 2019).
Determination of significant differences between concentration means was carried out with two biological replicates using one-way analysis of variance, followed by Tukey's test for mean comparison, using the statistical program Statistica 6.0 (StatSoft, Tulsa, OK, USA).
A total of 14 non-anthocyanin phenolic compounds were tentatively identified in pooled pulp and peel samples of the coffee fruit varieties by means of their UV spectra and mass fragmentation as well as the comparison to literature data. Nine of these compounds were free hydroxycinnamic acids (chlorogenic acids), including each three isomeric mono- (compounds 1–3 in Table 1) and di-caffeoylquinic acids (compounds 10, 11, and 13), in addition to p-coumaroyl- and feruloylquinic acids (compounds 6, 7b, and 8). All of these compounds are commonly found in green coffee beans (Clifford et al., 2003; Perrone et al., 2008) and, with the exception of p-coumaroylquinic acid, have been reported to be present in coffee pulp as well (Campa et al., 2012; Mullen et al., 2013; da Silveira et al., 2020).
Table 1. HPLC retention times (tR), UV/Vis and ESI-MS spectral data of non-anthocyanin phenolic compounds from pooled samples of coffee peels and pulp.
The prevailing chlorogenic acid found in the peels and pulp of all five coffee varieties was 5-O-caffeoylquinic acid (Table 2). It displayed dimeric ions [2M-H]− (Table 1) as the most abundant species in the ESI(-)-MS1 spectrum. Similar to our results, Clifford and Ramirez-Martinez (1991) found higher concentration of 5-O-caffeoylquinic acid over the other chlorogenic acids measured in coffee pulp. This compound was differentiated from other caffeoylquinic acids by the distinctive fragmentation pattern (Table 1) according to Gras et al. (2016). Both, 3-O- and 4-O-caffeoylquinic acids (compounds 1 and 3 in Table 1 and Figure 1) also exhibited [M-H]− precursor ions at m/z 353 but displayed different mass fragmentations as described previously (Clifford et al., 2003; Weisz et al., 2009). The deprotonated molecules [M-H]− at m/z 337 and 367 and the unique fragmentation patterns were considered for the identification of peaks 6 and 8 as p-coumaroylquinic and feruloylquinic acids, possibly 5-O-p-coumaroylquinic acid and 5-O-feruloylquinic acid, respectively (Weisz et al., 2009) (Table 1). The former could be only quantified in the peels, while the latter solely in the pulp, without a clear pattern between genotypes (Table 2). Some variation in the contents of individual chlorogenic acids in the pulp and husks of different coffee varieties, like in this report (Table 2), was previously described by Ramirez-Martinez (1988), Clifford and Ramirez-Martinez (1991) and Mullen et al. (2013) as well. Moreover, the concentrations in our work are within the range of concentrations they reported in the wet-processed coffee husks.
Table 2. Concentration (mg/kg DM) of major phenolic compounds in pulp and peels of different coffee varietiesa.
Figure 1. Representative HPLC-DAD chromatogram showing the separation of non-anthocyanin phenolic compounds present in the peels of Caturra amarillo (yellow variety) monitored at 280 nm. For peak assignment, see Table 1.
Additional phenolic compounds identified in coffee pulp and peels in our work include the monomeric flavan-3-ols (epi)catechin hexoside (epi)catechin and a catechin dimer (peaks 4, 5, and 9 in Table 1, respectively). Monomeric and dimeric aglycone flavan-3-ol forms have been previously reported in Arabica coffee pulp (Ramirez-Martinez, 1988; González De Colmenares et al., 1994; Ramirez-Coronel et al., 2004).
The putative (epi)catechin hexoside (peak 4, Figure 1) was one of the most conspicuous peaks and was found at concentrations ranging from 1,030 to 2,410 mg/kg. Although few differences were observed among genotypes, the yellow cultivar showed the highest contents in the peels, while the red Catuaí pulp had the lowest ones in the same fraction (Table 2). This peak displayed a deprotonated molecule [M-H]− at m/z 451 and a base peak fragment ion at m/z 289 generated by the neutral loss of 162 amu, resembling a dehydrated hexose moiety according to Robbins et al. (2014). (Epi)catechin (peak 5, Figure 1) with a precursor ion [M-H]− at m/z 289 and a base peak product ion at m/z 245 ([M-H-CO2]−) (Karar et al., 2014) was quantified at lower contents than those found for the (epi)catechin hexoside in peels and pulp showing less amount in the pulp of the red genotype Caturra Rojo than in the pulp of other genotypes. Peak 9 (Figure 1) was identified as a catechin dimer with a precursor ion [M-H]− at m/z 577 and product ions at m/z 425, 407, and 289 being characteristic for B-type procyanidins (Sui et al., 2016; Rue et al., 2017). The presence of proanthocyanidins has been previously reported by Ramirez-Martinez (1988), Ramirez-Coronel et al. (2004), and Mullen et al. (2013) in the pulp of Coffea arabica. Catechin and epicatechin levels in husks have previously shown differences according to the coffee species (C. arabica and C. canephora) and cultivation place (Mexico, India, or China), with much lower concentrations than the ones being reported in our work (Mullen et al., 2013). Conversely, higher levels of epicatechin have been previously reported in the pulp of eight coffee cultivars (Ramirez-Martinez, 1988) than those measured in this study, while similar values for catechin were described in the same report.
Furthermore, the C-glycosylated xanthone mangiferin (peak 7a, Figure 1), previously described as a compound with high potential as cancer chemopreventive (Gold-Smith et al., 2016), was identified by mass spectral data and using the corresponding standard. However, mangiferin quantitation was omitted due to a coelution with a feruloylquinic acid according to the mass fragmentation signals. Consistent with the elution sequence reported by Mullen et al. (2013), this compound could be 4-O-feruloylquinic acid (7b). Mangiferin has predominantly been found in mango (Mangifera indica L.) bark and fruits (Berardini et al., 2004, 2005; Nong et al., 2005), although it has been reported in leaves and fruits of diverse coffee species as well (Talamond et al., 2008; Campa et al., 2012; Trevisan et al., 2016). Therefore, its quantification in the coffee fruit byproducts of genotypes with different peel colors deserves more attention.
Finally, the flavonol rutin [quercetin 3-O-(6-O-rhamnosyl-glucoside)] (peak 12, Table 1), with a precursor ion [M-H]− at m/z 609 and a base peak product ion at m/z 301 ([M-H-308]−) (Karar et al., 2014), was only detected and quantified in the fruit peels, but not in the pulp, of all studied coffee genotypes, predominantly in the orange one Anaranjado (Table 2). Previous works (Ramirez-Martinez, 1988; Heeger et al., 2017; Torres-Valenzuela et al., 2020) have reported lower levels of this compound in coffee husks. The fact that in our work rutin was only found in the peels could, at least partially, explain these differences to previous reports in which husk samples (containing pulp and peels mixed) were analyzed. The higher ratio of pulp to peels' mass could have caused a dilution in the rutin contents reported elsewhere. Because of the health beneficial properties of this flavonoid (antioxidant, antimicrobial, anti-inflammatory, anticancer, antidiabetic, antiallergic, etc.), there is an increasing interest in identifying new rutin sources, and residual biomass has been the focus of recent research (Sharma et al., 2013; Gullón et al., 2017; Junker-Frohn et al., 2019).
Two anthocyanins, namely cyanidin-3-O-glucoside and cyanidin-3-O-rutinoside (peaks 1 and 2 in Figure 2, respectively), previously reported as the prevailing coffee anthocyanins (Prata and Oliveira, 2007), were identified by using the corresponding authentic standards (Table 3). Both cyanidins displayed molecular ions [M]+ in the ESI(+)-MS1 spectra and product ions from the elimination of the sugar moieties. Chemical ionization dissociation (CID) of compound 1 with a [M]+ at m/z 449 resulted in a single fragment ion from the elimination of a dehydrated hexose at m/z 287 ([M-162]+) resembling cyanidin. CID of 2 with [M]+ at m/z 595 generated fragment ions at m/z 449 ([M-146]+) and 287 ([M-308]+) from the neutral losses of the deoxyhexosyl (146 amu) and the entire (deoxyhexosyl)hexosyl (308 amu) moiety, respectively (Faramarzi et al., 2015). Both anthocyanins were absent in the pulp of all varieties assessed and in the peel of the yellow coffee genotype. The concentrations of cyanidin-3-O-glucoside and of cyanidin-3-O-rutinoside in the orange sample amounted to 4.9 and 10.2 mg/kg, respectively. These values were clearly exceeded by the 15.9–22.8 and 62.9–97.7 mg/kg, respectively, as determined across all red-colored samples (Table 2), as can be expected according to the color of the peels. The same anthocyanins were detected previously in coffee husks and, likewise, cyanidin-3-O-rutinoside was detected in higher contents than cyanidin-3-O-glucoside (Prata and Oliveira, 2007; Murthy et al., 2012). Prevalence of cyanidin-3-O-rutinoside over cyanidin-3-O-glucoside has been consistently reported in several ripe palm fruits [e.g., Euterpe oleracea Mart., Euterpe edulis Mart. and Bactris guineensis (L.) H.E. Moore], although in much higher contents than the ones measured in coffee peels (Gordon et al., 2012; Vieira et al., 2017; Erşan et al., in press). Extraction of anthocyanins from the coffee fruit processing byproducts for their utilization in the food industry is under development and has a promising future (Murthy and Naidu, 2012; Punbusayakul et al., 2014; Parra-Campos and Ordóñez-Santos, 2019).
Figure 2. Representative HPLC-DAD chromatogram showing the separation of anthocyanins present in the peels of Caturra Rojo (red variety) monitored at 520 nm. For peak assignment, see Table 3.
Table 3. HPLC retention times (tR), UV/Vis and ESI-MS spectral data of anthocyanins from pooled samples of coffee peels and pulp.
GC-MS alkaline hydrolysis and trimethylsilylation was conducted to verify the compound assignments based on HPLC-DAD-ESI-MSn analysis (Table 4). Abundant peaks were assigned to the hydroxycinnamic acids (E)-caffeic and (E)-p-coumaric acid, most likely resulting from the hydrolytic cleavage of their esters with quinic acid (chlorogenic acids). Their corresponding (Z)-isomers were detected as minor constituents and may represent possible workup artifacts (Steingass et al., 2015). In addition, quinic acid detected in the hydrolyzed extract may derive from these hydroxycinnamoyl esters. Two late eluting compounds detected at a low abundance were identified as epicatechin and catechin, thus substantiating their identification by LC-MS (Table 1).
Table 4. GC-MS analysis of methanolic extracts from coffee peel and pulp after alkaline hydrolysis, liquid-liquid extraction with ethyl acetate and trimethylsilylation.
Moreover, caffeine and diverse low molecular weight benzenoids were detected such as benzoic acid and derived hydroxybenzoic acids (hydroxybenzoic, protocatechuic, and vanillic acid) in addition to benzaldehyde, benzyl alcohol, eugenol, and phloretic acid. Noteworthy, the presence of protocatechuic acid and caffeine in coffee pulp has been previously reported (Ramirez-Martinez, 1988; Clifford and Ramirez-Martinez, 1991).
Further constituents detected by GC-MS comprised carboxylic acids from the citric acid cycle (citric, cis-aconitic, succinic, fumaric, and malic acid) ubiquitously occurring in plants. Glyceric acid has been previously reported in brewed coffee (Bähre and Maier, 1996). The retention indices and mass spectra of the coffee constituents compiled in Table 4 represent a solid basis for continuative research and may be highly instrumental for a GC-MS based metabolite profiling, e.g., for the authentication of coffee varieties but also derived products.
In this work, carotenoids were detected in the coffee fruit peels and pulp (Table 5). The much lower absolute signal observed in the latter fraction did not allow to assess potential differences between genotypes and, therefore, results are exemplified with the pulp of the yellow Caturra variety (Table 5). Regarding the peels, comparable profiles were observed in the yellow and orange coffee varieties analyzed here, both showing the highest diversity of carotenoids. The red Caturra peels mainly showed lutein and β-carotene, but did not show quantifiable amounts neither of the xanthophylls violaxanthin and neoxanthin [both with protonated molecules [M+H]+ at m/z 601], nor of α-carotene. β-Carotene and α-carotene displayed protonated molecules [M+H]+ at m/z 537. The comparison of their distinctive Vis absorption spectra to those reported in literature (e.g., Britton, 1995) permitted assignment of these isobaric carotenes. Lutein and β-carotene were the major carotenoids detected in all varieties (peaks 4 and 8 in Figure 3, respectively). Lutein showed a protonated molecule [M+H]+ at m/z 569 and an abundant, characteristic in-source fragment [M+H-18]+ at 551, and UV/Vis absorption maxima of 420, 444, and 472 nm, which is in agreement with Britton (1995) and Rodriguez-Amaya (2001). Lutein was not baseline resolved from one of the two chlorophylls detected in the genuine extracts.
Table 5. HPLC retention times (tR), Vis absorption maxima, APcI(+)-MS data and qualitative carotenoid and chlorophyll profiles in the peels of three coffee varieties and in the pulp of cv. Caturra Amarillo.
Figure 3. Representative HPLC-DAD separation of carotenoids and chlorophylls in genuine and saponified extracts from the peels of the Anaranjado (orange) variety monitored at 450 nm. For peak assignment, see Table 5.
Chlorophylls a and b were identified in the ripe coffee peels of all varieties, while only the latter was present in the pulp. Presence of chlorophyll is not only evident in unripe coffee fruits because of the exocarp green color, but also due to their relevant photosynthetic capacity (Vaast et al., 2005). However, a progressive decrease in the chlorophyll content along ripening has been reported (Marín-López et al., 2003; de Castro and Marraccini, 2006). These primary photosynthetic pigments were identified in our work by their protonated molecules [M+H]+ at m/z 892 and 906, respectively, and their corresponding absorption maxima, according to Maroneze et al. (2019).
Table 5 compiles the criteria for identification of the remaining carotenoids and the corresponding references. Comparison of chromatograms of genuine and saponified samples showed disappearance of several peaks in the latter, which points out to the presence of some carotenoid esters (Figure 3). This merits further investigation, especially, considering the increased bioavailability of esterified carotenoids over the free forms (Hempel et al., 2017; Schweiggert and Carle, 2017; Chacón-Ordóñez et al., 2019).
To the best of our knowledge, this is the first report on the characterization of carotenoids in different coffee fruit tissues (viz., pulp, and peels). In a previous work aiming at studying the profile and expression of carotenoid biosynthetic genes during coffee fruit development, Simkin et al. (2010) have analyzed complete fruits instead. They found the same pattern of carotenoids and chlorophylls we are reporting here. Similar to our results, these authors also found lutein to be the prevailing carotenoid in the ripe coffee fruits (in both C. arabica and C. canephora species). The carotenoid profile of coffee peel and pulp resembled that of a (degraded) chloroplast. These organelles ubiquitously occurring in green plant tissues contain chlorophylls a and b, in addition to a highly preserved carotenoid pattern, with prevailing constituents being lutein (40–45%) and β-carotene (20–25%), as well as violaxanthin and neoxanthin (both 10–15%) (Schweiggert and Carle, 2017).
Lutein, together with zeaxanthin, are the only carotenoids of about 20–30 found in the human tissues, that accumulate in the macula lutea, which is the most sensitive region of the retina of humans and other primates. These carotenoids are believed to be important for the prevention of age-related macular degeneration, being a cause of impaired vision and blindness in elderly people. Furthermore, frequent lutein intake has been associated with improved cognitive functions like verbal fluency in senior citizens (Johnson, 2012; Eisenhauer et al., 2017). In addition, β-carotene, the other predominant carotenoid found in the peels and pulp of coffee berries according to results in Figure 3, is well-known for its provitamin A activity. Deficiency of this vitamin is an important drawback for deprived populations in various regions of the world and has been related to eye health issues and increased severity and death rates due to infectious diseases (Wiseman et al., 2017). Therefore, looking for affordable lutein and β-carotene sources has been the aim of considerable research, and coffee by-products might be considered for the food industry in the framework of the application of circular economy strategies. Further investigation regarding the effect of environmental and genetic conditions on carotenoid accumulation, as well as evaluation of extraction methods, to attain high recovery and reduce prospective antinutritional factors, are necessary to take advantage of this potential source of functional compounds (Janissen and Huynh, 2018; Kumar et al., 2018; Saini and Keum, 2018).
Color parameters of the peels are displayed in Table 6 and pictures of the studied coffee cherries are shown in Figure 4. In agreement with the visual perception, L* values corroborated higher lightness for yellow- and orange-colored fruits (L* of 43 and 37, resp.) when compared to the red ones (L* of 21–26). Moreover, yellow- and orange-colored coffee cherries also showed higher chroma (C*) values when compared to the other materials. As expected, hue (h°) values of 75 in the yellow colored berries agreed with the yellow tonalities (h° at ~70–90), orange colored fruits with hue values of ~56, also neared the expected orange tonalities (h° at ~40–70). Hue values ranging between 26 and 35 were measured for the red colored fruits, also in accordance with red tonalities (h° at ~10–30).
Figure 4. Photographs of the ripe coffee berries analyzed in this work: (A) Caturra Rojo (red), (B) Anaranjado (orange), (C) Catuaí Rojo (red), (D) Arábica (red), (E) Caturra amarillo (yellow).
The profile of anthocyanins, together with that of carotenoids, explains the observable colors of the coffee peels in the different genotypes. The relationship between both pigment groups in terms of quantity, profile and distribution in the different tissues has been pointed out as determinant for generating the observable color. This has been described previously in several tropical fruits such as bananas, cashew apples, mango, and nance (Schweiggert et al., 2016; Fu et al., 2018; Irías-Mata et al., 2018; Ranganath et al., 2018). In flowers (Lewis et al., 2003) and banana fruits (Fu et al., 2018), the deposition of anthocyanins in external cell layers has been documented, whereas carotenoids are sometimes located in more internal ones (sub-epidermis and mesophyll) and, thus, their yellow color might be less dominantly visible than the red color of anthocyanins. In any case, the color of anthocyanins often overlays with the color of carotenoids and, eventually, chlorophylls and, therefore, according to the pigments available, different colorations are noticeable. Knowing whether differential deposition in layers also occurs in the coffee peels might be the subject of further research. Nevertheless, clearly, the absence of anthocyanins in the yellow genotype (Caturra Amarillo) (Table 2) allows the sole appearance of a yellow color caused by lutein and β-carotene, the most conspicuous carotenoid peaks observed (Figure 3), and widely known for their yellow and orange colors, respectively (Meléndez-Martínez et al., 2007). Orange pigmentation in Zantedeschia (calla lily) and red pigmentation in bananas have been found to result from the visual appearance of having both pigment groups (carotenoids and anthocyanins) in different cell layers, and not because of their mixture (Lewis et al., 2003; Fu et al., 2018). Something similar might occur in the orange coffee variety (Anaranjado), a genotype that contains a lower concentration of anthocyanins compared to the red ones (Table 3). Nevertheless, in the red-colored varieties, the much higher concentration of anthocyanins apparently had totally masked the yellow background color of the more internal carotenoids, hiding their appearance, either simply due to their contents or because of their localization in the outer cell layers.
5-O-Caffeoylquinic acid together with a putative (epi)catechin hexoside were consistently the prevailing phenolic compounds among 16 detected in the pulp and peels of coffee berries belonging to different varieties with distinct exocarp color. Moreover, the xanthone mangiferin, a cancer chemopreventive agent, was found in all samples and its study deserves more attention. GC-MS analysis after alkaline hydrolysis and trimethylsilylation substantiated the assignment of the individual phenolic compounds by HPLC-DAD-ESI-MSn and, moreover, permitted the identification of additional plant metabolites, thus providing a solid basis for ongoing research. Anthocyanins were found in red-colored fruit peels and in lower contents in orange-colored ones, but were absent in the yellow-colored berries. On the other hand, carotenoid peel profiles can be divided into two groups, with the red genotype on the one side and the yellow and orange ones clustered together on the other. The latter showed additional carotenoid compounds to the ones found in the former. These results point out to the potential that coffee berry by-products have for their utilization as a source of health beneficial compounds, including natural pigments.
The raw data supporting the conclusions of this article will be made available by the authors, without undue reservation.
PE, EG, RC, and VJ designed research. MV, CS, MG, and RS conducted research. PE, MV, CS, RS, and VJ analyzed data. PE, MV, CS, EG, RC, RS, and VJ discussed the data. PE, MV, CS, RS, and VJ wrote the paper. All authors read and approved the final manuscript.
MV acknowledges support from the Baden-Württemberg Stiftung (Stuttgart, Germany), the Ministerio de Ciencia y Tecnología (MICITT), and the Consejo Nacional para Investigaciones Científicas y Tecnológicas (CONICIT) of Costa Rica. PE and VJ received financial support from the German Academic Exchange Service (DAAD) in form of short-time fellowships and gratefully acknowledge it. This work was partially supported by the University of Costa Rica under the research project VI-735-C0-750 and Fondo Restringido #6373.
The authors declare that the research was conducted in the absence of any commercial or financial relationships that could be construed as a potential conflict of interest.
Part of the results of this work were presented at the 24th International Conference on Coffee Science of the Association Scientifique Internationale du Café (ASIC), held in San José, Costa Rica on Nov. 12th−16th 2012 (Viñas et al., 2012).
Bähre, F., and Maier, H. G. (1996). Electrophoretic clean-up of organic acids from coffee for the GC/MS analysis. Fresenius J. Anal. Chem. 355, 190–193. doi: 10.1007/s0021663550190
Berardini, N., Carle, R., and Schieber, A. (2004). Characterization of gallotannins and benzophenone derivatives from mango (Mangifera indica L. cv. ‘Tommy Atkins') peels, pulp and kernels by high-performance liquid chromatography/electrospray ionization mass spectrometry. Rapid Commun. Mass Spectrom. 18, 2208–2216. doi: 10.1002/rcm.1611
Berardini, N., Fezer, R., Conrad, J., Beifuss, U., Carle, R., and Schieber, A. (2005). Screening of mango (Mangifera indica L.) cultivars for their contents of flavonol O- and xanthone C-glycosides, anthocyanins, and pectin. J. Agric. Food Chem. 53, 1563–1570. doi: 10.1021/jf0484069
Britton, G. (1995). “UV/visible spectroscopy,” in Carotenoids, eds G. Britton, S. Liaaen-Jensen, and H. Pfander (Basel: Birkhäuser Verlag AG), 13–62.
Campa, C., Mondolot, L., Rakotondravao, A., Bidel, L. P. R., Gargadennec, A., Couturon, E., et al. (2012). A survey of mangiferin and hydroxycinnamic acid ester accumulation in coffee (Coffea) leaves: biological implications and uses. Ann. Bot. 110, 595–613. doi: 10.1093/aob/mcs119
Chacón-Ordóñez, T., Carle, R., and Schweiggert, R. (2019). Bioaccessibility of carotenoids from plant and animal foods. J. Sci. Food Agric. 99, 3220–3239. doi: 10.1002/jsfa.9525
Clifford, M. N., Johnston, K. L., Knight, S., and Kuhnert, N. (2003). Hierarchical scheme for LC-MSn identification of chlorogenic acids. J. Agric. Food Chem. 51, 2900–2911. doi: 10.1021/jf026187q
Clifford, M. N., and Ramirez-Martinez, J. R. (1991). Phenols and caffeine in wet-processed coffee beans and coffee pulp. Food Chem. 40, 35–42. doi: 10.1016/0308-8146(91)90017-I
da Silveira, J. S., Mertz, C., Morel, G., Lacour, S., Belleville, M.-P., Durand, N., et al. (2020). Alcoholic fermentation as a potential tool for coffee pulp detoxification and reuse: analysis of phenolic composition and caffeine content by HPLC-DAD-MS/MS. Food Chem. 319:126600. doi: 10.1016/j.foodchem.2020.126600
de Castro, R. D., and Marraccini, P. (2006). Cytology, biochemistry and molecular changes during coffee fruit development. Braz. J. Plant Physiol. 18, 175–199. doi: 10.1590/S1677-04202006000100013
de Rosso, V. V., and Mercadante, A. Z. (2007). Identification and quantification of carotenoids, by HPLC-PDA-MS/MS, from Amazonian fruits. J. Agric. Food Chem. 55, 5062–5072. doi: 10.1021/jf0705421
Eisenhauer, B., Natoli, S., Liew, G., and Flood, V. (2017). Lutein and zeaxanthin—food sources, bioavailability and dietary variety in age-related macular degeneration protection. Nutrients 9:120. doi: 10.3390/nu9020120
Erşan, S., Berning, J. C., Esquivel, P., Jiménez, V. M., Carle, R., and May, B. (in press). Phytochemical mineral composition of fruits seeds of wild-growing Bactris guineensis (L.) H.E. Moore palms from Costa Rica. J. Food Compos. Anal. 103611. doi: 10.1016/j.jfca.2020.103611.
Esquivel, P., and Jiménez, V. M. (2012). Functional properties of coffee and coffee by-products. Food Res. Int. 46, 488–495. doi: 10.1016/j.foodres.2011.05.028
Esquivel, P., and Jiménez, V. M. (2019). “Valuable compounds in coffee by products,” in Green Extraction and Valorization of By-Products from Food Processing, eds F. J. Barba, E. Roselló Soto, M. Brncic, and J. M. Lorenzo (Boca Raton, FL: CRC Press), 277–292.
Faramarzi, S., Pacifico, S., Yadollahi, A., Lettieri, A., Nocera, P., and Piccolella, S. (2015). Red-fleshed apples: old autochthonous fruits as a novel source of anthocyanin antioxidants. Plant Foods Hum. Nutr. 70, 324–330. doi: 10.1007/s11130-015-0497-2
Fu, X., Cheng, S., Liao, Y., Huang, B., Du, B., Zeng, W., et al. (2018). Comparative analysis of pigments in red and yellow banana fruit. Food Chem. 239, 1009–1018. doi: 10.1016/j.foodchem.2017.07.046
Gold-Smith, F., Fernandez, A., and Bishop, K. (2016). Mangiferin and cancer: mechanisms of action. Nutrients 8:396. doi: 10.3390/nu8070396
González De Colmenares, N., Ramírez-Martínez, J. R., Aldana, J. O., and Clifford, M. N. (1994). Analysis of proanthocyanidins in coffee pulp. J. Sci. Food Agric. 65, 157–162. doi: 10.1002/jsfa.2740650206
Gordon, A., Cruz, A. P. G., Cabral, L. M. C., de Freitas, S. C., Taxi, C. M. A. D., Donangelo, C. M., et al. (2012). Chemical characterization and evaluation of antioxidant properties of Açaí fruits (Euterpe oleraceae Mart.) during ripening. Food Chem. 133, 256–263. doi: 10.1016/j.foodchem.2011.11.150
Gras, C. C., Bogner, H., Carle, R., and Schweiggert, R. M. (2016). Effect of genuine non-anthocyanin phenolics and chlorogenic acid on color and stability of black carrot (Daucus carota ssp. sativus var. atrorubens Alef.) anthocyanins. Food Res. Int. 85, 291–300. doi: 10.1016/j.foodres.2016.05.006
Gullón, B., Lú-,Chau, T. A., Moreira, M. T., Lema, J. M., and Eibes, G. (2017). Rutin: A review on extraction, identification and purification methods, biological activities and approaches to enhance its bioavailability. Trends Food Sci. Technol. 67, 220–235. doi: 10.1016/j.tifs.2017.07.008
Heeger, A., Kosińska-Cagnazzo, A., Cantergiani, E., and Andlauer, W. (2017). Bioactives of coffee cherry pulp and its utilisation for production of Cascara beverage. Food Chem. 221, 969–975. doi: 10.1016/j.foodchem.2016.11.067
Hempel, J., Fischer, A., Fischer, M., Högel, J., Bosy-Westphal, A., Carle, R., et al. (2017). Effect of aggregation form on bioavailability of zeaxanthin in humans: a randomised cross-over study. Br. J. Nutr. 118, 698–706. doi: 10.1017/S0007114517002653
Irías-Mata, A., Jiménez, V. M., Steingass, C. B., Schweiggert, R. M., Carle, R., and Esquivel, P. (2018). Carotenoids and xanthophyll esters of yellow and red nance fruits (Byrsonima crassifolia (L.) Kunth) from Costa Rica. Food Res. Int. 111, 708–714. doi: 10.1016/j.foodres.2018.05.063
Jaiswal, R., Karar, M. G. E., Gadir, H. A., and Kuhnert, N. (2014). Identification and characterisation of phenolics from Ixora coccinea L. (Rubiaceae) by liquid chromatography multi-stage mass spectrometry. Phytochem. Anal. 25, 567–576. doi: 10.1002/pca.2530
Janissen, B., and Huynh, T. (2018). Chemical composition and value-adding applications of coffee industry by-products: a review. Resour. Conserv. Recycl. 128, 110–117. doi: 10.1016/j.resconrec.2017.10.001
Johnson, E. J. (2012). A possible role for lutein and zeaxanthin in cognitive function in the elderly. Am. J. Clin. Nutr. 96, 1161S−1165S. doi: 10.3945/ajcn.112.034611
Junker-Frohn, L. V., Lück, M., Schmittgen, S., Wensing, J., Carraresi, L., Thiele, B., et al. (2019). Tomato's green gold: bioeconomy potential of residual tomato leaf biomass as a novel source for the secondary metabolite rutin. ACS Omega 4, 19071–19080. doi: 10.1021/acsomega.9b01462
Kammerer, D., Claus, A., Carle, R., and Schieber, A. (2004). Polyphenol screening of pomace from red and white grape varieties (Vitis vinifera L.) by HPLC-DAD-MS/MS. J. Agric. Food Chem. 52, 4360–4367. doi: 10.1021/jf049613b
Karar, M. G. E., Pletzer, D., Jaiswal, R., Weingart, H., and Kuhnert, N. (2014). Identification, characterization, isolation and activity against Escherichia coli of quince (Cydonia oblonga) fruit polyphenols. Food Res. Int. 65, 121–129. doi: 10.1016/j.foodres.2013.10.040
Kramer, M., Maksylewicz-Kaul, A., Barranski, R., Nothnagel, T., Carle, R., and Kammerer, D. (2012). Effects of cultivation year and growing location on the phenolic profile of differently coloured carrot cultivars. J. Appl. Bot. Food Qual. 85, 235–247.
Kumar, S. S., Swapna, T. S., and Sabu, A. (2018). “Coffee husk: a potential agro-industrial residue for bioprocess,” in Waste to Wealth, eds R. R. Singhania, R. A. Agarwal, R. P. Kumar, and R. K. Sukumaran (Singapore: Springer Singapore), 97–109. doi: 10.1007/978-981-10-7431-8_6
Lewis, D. H., Arathoon, H. S., Swinny, E. E., Huang, S. C., and Funnell, K. A. (2003). Anthocyanin and carotenoid pigments in spathe tissue from selected Zantedeschia hybrids. Acta Hortic. 624, 147–154. doi: 10.17660/ActaHortic.2003.624.19
Marín-López, S. M., Arcila-Pulgarín, J., Montoya-Restrepo, E. C., and Oliveros-Tascón, C. E. (2003). Cambios físicos y químicos durante la maduración del fruto de café Coffea arabica L. var. Colombia. Cenicafé 54, 208–225.
Maroneze, M. M., Zepka, L. Q., Lopes, E. J., Pérez-Gálvez, A., and Roca, M. (2019). Chlorophyll oxidative metabolism during the phototrophic and heterotrophic growth of Scenedesmus obliquus. Antioxidants 8:600. doi: 10.3390/antiox8120600
Meléndez-Martínez, A. J., Britton, G., Vicario, I. M., and Heredia, F. J. (2007). Relationship between the colour and the chemical structure of carotenoid pigments. Food Chem. 101, 1145–1150. doi: 10.1016/j.foodchem.2006.03.015
Mullen, W., Nemzer, B., Stalmach, A., Ali, S., and Combet, E. (2013). Polyphenolic and hydroxycinnamate contents of whole coffee fruits from China, India, and Mexico. J. Agric. Food Chem. 61, 5298–5309. doi: 10.1021/jf4003126
Murthy, P. S., Manjunatha, M., Sulochannama, G., and Naidu, M. M. (2012). Extraction, characterization and bioactivity of coffee anthocyanins. Eur. J. Biol. Sci. 4, 13–19. doi: 10.5829/idosi.ejbs.2012.4.1.6149
Murthy, P. S., and Naidu, M. M. (2012). Recovery of phenolic antioxidants and functional compounds from coffee industry by-products. Food Bioprocess Technol. 5, 897–903. doi: 10.1007/s11947-010-0363-z
Nong, C., He, W., Fleming, D., Pan, L., and Huang, H. (2005). Capillary electrophoresis analysis of mangiferin extracted from Mangifera indica L. bark and Mangifera persiciformis C.Y. Wu et T.L. Ming leaves. J. Chromatogr. B 826, 226–231. doi: 10.1016/j.jchromb.2005.09.006
Parra-Campos, A., and Ordóñez-Santos, L. E. (2019). Natural pigment extraction optimization from coffee exocarp and its use as a natural dye in French meringue. Food Chem. 285, 59–66. doi: 10.1016/j.foodchem.2019.01.158
Perrone, D., Farah, A., Donangelo, C. M., de Paulis, T., and Martin, P. R. (2008). Comprehensive analysis of major and minor chlorogenic acids and lactones in economically relevant Brazilian coffee cultivars. Food Chem. 106, 859–867. doi: 10.1016/j.foodchem.2007.06.053
Prata, E. R. B. A., and Oliveira, L. S. (2007). Fresh coffee husks as potential sources of anthocyanins. LWT Food Sci. Technol. 40, 1555–1560. doi: 10.1016/j.lwt.2006.10.003
Punbusayakul, N., Saim, N., Haiyee, Z. A., Subari, S. N. M., Yahya, N. I. A., Sarnkhuankaew, W., et al. (2014). Subcritical water extraction (SWE) of anthocyanin from Arabica coffee pulp. Sci. Lett. 8, 34–40. doi: 10.2174/22102892013040100176
Ramirez-Coronel, M. A., Marnet, N., Kolli, V. S. K., Roussos, S., Guyot, S., and Augur, C. (2004). Characterization and estimation of proanthocyanidins and other phenolics in coffee pulp (Coffea arabica) by thiolysis–high-performance liquid chromatography. J. Agric. Food Chem. 52, 1344–1349. doi: 10.1021/jf035208t
Ramirez-Martinez, J. R. (1988). Phenolic compounds in coffee pulp: Quantitative determination by HPLC. J. Sci. Food Agric. 43, 135–144. doi: 10.1002/jsfa.2740430204
Ranganath, K. G., Shivashankara, K. S., Roy, T. K., Dinesh, M. R., Geetha, G. A., Pavithra, K. C., et al. (2018). Profiling of anthocyanins and carotenoids in fruit peel of different colored mango cultivars. J. Food Sci. Technol. 55, 4566–4577. doi: 10.1007/s13197-018-3392-7
Robbins, K. S., Ma, Y., Wells, M. L., Greenspan, P., and Pegg, R. B. (2014). Separation and characterization of phenolic compounds from U.S. pecans by liquid chromatography–tandem mass spectrometry. J. Agric. Food Chem. 62, 4332–4341. doi: 10.1021/jf500909h
Rodríguez-Durán, L. V., Ramírez-Coronel, M. A., Aranda-Delgado, E., Nampoothiri, K. M., Favela-Torres, E., Aguilar, C. N., et al. (2014). Soluble and bound hydroxycinnamates in coffee pulp (Coffea arabica) from seven cultivars at three ripening stages. J. Agric. Food Chem. 62, 7869–7876. doi: 10.1021/jf5014956
Rue, E. A., Rush, M. D., and van Breemen, R. B. (2017). Procyanidins: a comprehensive review encompassing structure elucidation via mass spectrometry. Phytochem. Rev. 17, 1–16. doi: 10.1007/s11101-017-9507-3
Saini, R. K., and Keum, Y.-S. (2018). Carotenoid extraction methods: a review of recent developments. Food Chem. 240, 90–103. doi: 10.1016/j.foodchem.2017.07.099
Scholz, M. B. D. S., Figueiredo, V. R. G., Silva, J. V. N., and Kitzeberger, C. S. G. (2011). Atributos sensoriais e características físico-químicas de bebidas de cultivares de café do IAPAR. Coffee Sci. 6, 245–255.
Schweiggert, R. M., and Carle, R. (2017). Carotenoid deposition in plant and animal foods and its impact on bioavailability. Crit. Rev. Food Sci. Nutr. 57, 1807–1830. doi: 10.1080/10408398.2015.1012756
Schweiggert, R. M., Steingass, C. B., Mora, E., Esquivel, P., and Carle, R. (2011). Carotenogenesis and physico-chemical characteristics during maturation of red fleshed papaya fruit (Carica papaya L.). Food Res. Int. 44, 1373–1380. doi: 10.1016/j.foodres.2011.01.029
Schweiggert, R. M., Vargas, E., Conrad, J., Hempel, J., Gras, C. C., Ziegler, J. U., et al. (2016). Carotenoids, carotenoid esters, and anthocyanins of yellow-, orange-, and red-peeled cashew apples (Anacardium occidentale L.). Food Chem. 200, 274–282. doi: 10.1016/j.foodchem.2016.01.038
Sharma, S., Ali, A., Ali, J., Sahni, J. K., and Baboota, S. (2013). Rutin: therapeutic potential and recent advances in drug delivery. Expert Opin. Investig. Drugs 22, 1063–1079. doi: 10.1517/13543784.2013.805744
Simkin, A. J., Kuntz, M., Moreau, H., and McCarthy, J. (2010). Carotenoid profiling and the expression of carotenoid biosynthetic genes in developing coffee grain. Plant Physiol. Biochem. 48, 434–442. doi: 10.1016/j.plaphy.2010.02.007
Steingass, C. B., Glock, M. P., Schweiggert, R. M., and Carle, R. (2015). Studies into the phenolic patterns of different tissues of pineapple (Ananas comosus [L.] Merr.) infructescence by HPLC-DAD-ESI-MSn and GC-MS analysis. Anal. Bioanal. Chem. 407, 6463–6479. doi: 10.1007/s00216-015-8811-2
Sui, Y., Zheng, Y., Li, X., Li, S., Xie, B., and Sun, Z. (2016). Characterization and preparation of oligomeric procyanidins from Litchi chinensis pericarp. Fitoterapia 112, 168–174. doi: 10.1016/j.fitote.2016.06.001
Talamond, P., Mondolot, L., Gargadennec, A., de Kochko, A., Hamon, S., Fruchier, A., et al. (2008). First report on mangiferin (C-glucosyl-xanthone) isolated from leaves of a wild coffee plant, Coffea pseudozanguebariae (Rubiaceae). Acta Bot. Gallica 155, 513–519. doi: 10.1080/12538078.2008.10516130
Tessema, A., Alamerew, S., Kufa, T., and Garedew, W. (2011). Genetic diversity analysis for quality attributes of some promising Coffea arabica germplasm collections in Southwestern Ethiopia. J. Biol. Sci. 11, 236–244. doi: 10.3923/jbs.2011.236.244
Torres-Valenzuela, L. S., Ballesteros-Gómez, A., and Rubio, S. (2020). Supramolecular solvent extraction of bioactives from coffee cherry pulp. J. Food Eng. 278:109933. doi: 10.1016/j.jfoodeng.2020.109933
Trevisan, M. T. S., de Almeida, R. F., Soto, G., de Melo Virginio Filho, E., Ulrich, C. M., and Owen, R. W. (2016). Quantitation by HPLC-UV of mangiferin and isomangiferin in coffee (Coffea arabica) leaves from Brazil and Costa Rica after solvent extraction and infusion. Food Anal. Methods 9, 2649–2655. doi: 10.1007/s12161-016-0457-y
USDA (2018). Coffee: World Markets and Trade. United States Department of Agriculture. Available online at: https://apps.fas.usda.gov/psdonline/circulars/coffee.pdf (accessed July 16, 2020).
Vaast, P., Angrand, J., Franck, N., Dauzat, J., and Génard, M. (2005). Fruit load and branch ring-barking affect carbon allocation and photosynthesis of leaf and fruit of Coffea arabica in the field. Tree Physiol. 25, 753–760. doi: 10.1093/treephys/25.6.753
Vieira, G. S., Marques, A. S. F., Machado, M. T. C., Silva, V. M., and Hubinger, M. D. (2017). Determination of anthocyanins and non-anthocyanin polyphenols by ultra performance liquid chromatography/electrospray ionization mass spectrometry (UPLC/ESI-MS) in jussara (Euterpe edulis) extracts. J. Food Sci. Technol. 54, 2135–2144. doi: 10.1007/s13197-017-2653-1
Viñas, M., Gruschwitz, M., Schweiggert, R. M., Guevara, E., Carle, R., Esquivel, P., et al. (2012). Identification of Phenolic and Carotenoid Compounds in Coffee (Coffea Arabica) Pulp, Peels and Mucilage by HPLC Electrospray Ionization Mass Spectrometry. San José: Association Scientifique Internationale du Café (ASIC), 127–135.
Weisz, G. M., Kammerer, D. R., and Carle, R. (2009). Identification and quantification of phenolic compounds from sunflower (Helianthus annuus L.) kernels and shells by HPLC-DAD/ESI-MSn. Food Chem. 115, 758–765. doi: 10.1016/j.foodchem.2008.12.074
Keywords: anthocyanins, carotenoids, (epi)catechin hexoside, chlorogenic acids, Coffea arabica, coffee, cyanidin, lutein
Citation: Esquivel P, Viñas M, Steingass CB, Gruschwitz M, Guevara E, Carle R, Schweiggert RM and Jiménez VM (2020) Coffee (Coffea arabica L.) by-Products as a Source of Carotenoids and Phenolic Compounds—Evaluation of Varieties With Different Peel Color. Front. Sustain. Food Syst. 4:590597. doi: 10.3389/fsufs.2020.590597
Received: 02 August 2020; Accepted: 14 September 2020;
Published: 21 October 2020.
Edited by:
Laurent Dufossé, Université de la Réunion, FranceReviewed by:
Dalene De Beer, Agricultural Research Council of South Africa (ARC-SA), South AfricaCopyright © 2020 Esquivel, Viñas, Steingass, Gruschwitz, Guevara, Carle, Schweiggert and Jiménez. This is an open-access article distributed under the terms of the Creative Commons Attribution License (CC BY). The use, distribution or reproduction in other forums is permitted, provided the original author(s) and the copyright owner(s) are credited and that the original publication in this journal is cited, in accordance with accepted academic practice. No use, distribution or reproduction is permitted which does not comply with these terms.
*Correspondence: Patricia Esquivel, cGF0cmljaWEuZXNxdWl2ZWxAdWNyLmFjLmNy
†ORCID: Patricia Esquivel orcid.org/0000-0002-2555-9535
María Viñas orcid.org/0000-0003-0644-0579
Christof B. Steingass orcid.org/0000-0001-8269-4525
Ralf M. Schweiggert orcid.org/0000-0003-0546-1335
Víctor M. Jiménez orcid.org/0000-0003-3771-6072
Disclaimer: All claims expressed in this article are solely those of the authors and do not necessarily represent those of their affiliated organizations, or those of the publisher, the editors and the reviewers. Any product that may be evaluated in this article or claim that may be made by its manufacturer is not guaranteed or endorsed by the publisher.
Research integrity at Frontiers
Learn more about the work of our research integrity team to safeguard the quality of each article we publish.