- 1Tecnologico de Monterrey, Centro de Biotecnología-FEMSA, Monterrey, Mexico
- 2Universidad Iberoamericana Puebla-IDIT, Puebla, Mexico
- 3Tecnologico de Monterrey, Departamento de Bioingeniería, Puebla, Mexico
The hot chili pepper industry represents one of the most important staple foods in Mexico and many Asian countries. Nowadays, large amounts of waste materials are produced from the pepper supply chain that could be used as a source to obtain nutraceuticals. Among the most common and important bioactive compounds contained in pepper residues are the capsaicinoids, which are the responsible of the pungency of the pepper. Capsaicinoids, mainly capsaicin, may ameliorate obesity, gastric disorder, diabetes, cardiovascular diseases, cancer, rhinitis, asthma, immune system diseases, and important viral diseases as the recent COVID-19. The aim of this review is to review the industrial process for the extraction of capsaicinoids ingredients from pepper residues and to examine the relation of the capsaicin and other chili pepper phytochemicals to prevent and treat chronic diseases explained through the key role of the TRPV1 receptor. The extraction and incorporation of these compounds into nutraceutical formulations depend mainly on the development of new methods to improve not only the yield of a particular compound but the validation of the bioactivity and phytochemical characterization.
Introduction
The annual production of chili peppers is about 3.2 millions of ton that correspond to the 3.5% of the Mexican agricultural Gross Domestic Product (GDP) (SAGARPA, 2017). Globally, the industry related with chili pepper production increased in other countries where this condiment is relevant for their gastronomy and other cultural purposes. The exportations of dry crushed chili pepper of countries like India, China, or Spain represent 6 billion USD of the global production and correspond to 65% of the chili pepper exportations (Carmona, 2013). Currently in Mexico, chili peppers are the most important cultivars for exportation, where 29.71% of the total production go to international market, mainly USA, Canada, and Guatemala (SAGARPA, 2017). Nevertheless, in order to guarantee this exportation, these products must meet rigorous quality criteria and a big amount of material is discarded. These crop waste materials correspond to 18.4% of the total national crops, which mainly includes seeds, and incomplete and defective fruits. These pepper wastes could be used as byproducts due to their content of compounds with antioxidant activity, which could be used to produce innovative ingredients (Sandoval-Castro et al., 2017).
In many of fruits and vegetables, only the epithelium or pulp is consumed but substantial amounts of nutraceutical compounds are present in the seeds, peels, and other components as peduncle, loculum or base that are not commonly consumed (Rudra et al., 2015). Extraction processes are the main critical phase to obtain bioactive compounds from waste material. Sample preparation is one of the essential factors to regulate the type and amount of bioactive compounds extracted. Extraction methods can be categorized into two main categories: conventional and innovative techniques (Sagar et al., 2018).
Enzymatic pre-treatment is as a novel and useful way to recover bound compounds from the cell wall and increase their yield of extraction (Sagar et al., 2018). The use of enzymatic treatments coupled to the oil extraction processes such as organic solvent extraction, supercritical fluids extraction, and others improve the yield of extraction resulting into promising high percentages of recovery (Stoica et al., 2016). Enzymatic techniques have been established fundamentally for the extraction of bioactive compounds from different seeds. Different elements including catalyst type, molecular size of plant materials, and the hydrolysis time are observed as main factors for extraction. Enzymatic treatment for extraction is contemplated as an eco-friendly method to extract oils and bioactive compounds because water is used as the solvent instead of organic solvents (Sagar et al., 2018). Cellulases, pectinases, beta-glucosidases, or enzymes cocktails are used to hydrolyze and degrade cell wall components and in consequence release the nutraceutical compounds that are linked to cell wall such as carotenoids, phenolic compounds, capsaicinoids, or other compounds of interest (Walczak et al., 2018).
Pepper seeds contain sterols, saponins and phenolic compounds responsible for the biological activities (Sung and Lee, 2016). Red pepper seed oils are abundant in polyunsaturated fatty acids, bioactive compounds such as polyphenols, tocopherols, and phytosterols (Chouaibi et al., 2019) that have been related with activities described previously and result an interesting field of study. Around 90% of capsaicinoids are located in the pericarp (fruit), which comprises 40% of the pepper, while the other 10% are found in the seeds and then their concentration in the waste parts is 102–154 mg/g while in non-waste material it is 250–450 mg/g of dry material (Oguzkan, 2019). Capsaicinoids have been used in traditional medicine for the treatment of cough, toothache, sore throat, rheumatism, antiseptic, antioxidant and immunomodulator (Sanati et al., 2018). They possess anti-inflammatory effects, antioxidant activity and anti-obesity properties, and have been related to anti-carcinogenic and anti-mutagenic properties that could be exploited as a new nutraceutical approach to the extracts related with chili peppers (Friedman et al., 2019).
Recently, the potential effects of the capsaicinoids under the immune responses have been studied. Grüter et al. (2020) reported that capsaicin exerts an immunomodulatory response on neurilemma cells due to the anti-inflammatory effect provoked by numerous biochemical neural pathways. Nowadays, the worldwide attention is focused in the global pandemic of COVID-19 and the scientific efforts are concentrated on finding alternative treatments to the viral disease, one of the research route is the evaluation of phytochemical compounds that could relieve the symptoms of the SARS-Cov-2 infection.
Chemistry and Biosynthesis of Capsaicinoids
Capsaicinoids (Figure 1A) are alkaloid compounds responsible for the pungency associated with consumption of Capsicum fruit. Capsaicinoids are highly volatile, pungent, hydrophobic, colorless, and odorless white crystalline powder, with a melting point of 60–65°C and a molecular weight of 305.4 Da (Sharma et al., 2013). The basic chemical structure of these compounds is an acid amide of vanillyl amine combined with branched-chain fatty acids from 7 to 13 carbons in length (Aza-González et al., 2011). Their pungency is mainly related to the benzene ring but also to the acyl chain length (Fattori et al., 2016). Although more than 10 structures exist, the most prominent forms are capsaicin and dihydrocapsaicin, accounting for almost 90% of capsaicinoids (Meghvansi et al., 2010). Nordihydrocapsaicin, homocapsaicin and homodihydrocapsaicin are present in lesser amounts (Tewksbury et al., 2006).
A similar group of compounds named capsinoids (Figure 1B) also have the beneficial effects of capsaicinoids but without the pungency (Hursel and Westerterp-Plantenga, 2010). Due to their slightly different structure, they stimulate receptors in the intestine but not in the mouth. This is a particularly valuable attribute, as many people struggle to consume capsaicinoids due to their “spiciness” (Luo et al., 2011). In contrast to capsaicinoids, capsinoids are esters of vanillyl alcohol with fatty acids similar to those of capsaicinoids (Fayos et al., 2019). This structural difference could also be responsible for the lower stability of capsinoids and a pungency 1,000 times lower than that of capsaicinoids (Tanaka et al., 2015).
Capsaicinoids are natural compounds related to defense mechanisms of plants against mammalian herbivores and fungi (Tewksbury et al., 2006). Their content in Capsicum fruit varieties is largely influenced by genetics, in which the total capsaicinoid content ranges from 720 to 4,360 ppm for cayenne (Capsicum annuum) cultivars, 1,110–7,260 ppm for jalapeno (Capsicum annuum jalapeño) cultivars, and 5,200–14,000 ppm for habanero (Capsicum chinense) cultivars. In addition to genetics, capsaicinoid content is influenced by the fertilization practices, environmental growing conditions, application of bioregulators, and maturity of the fruit (Sharma et al., 2013).
Capsaicinoids are synthetized primarily in the epidermal cells of placental tissue, with low levels found in the pericarp and seeds. L-phenylalanine and L-valine or L-leucine through vanillylamine are capsaicinoids precursors. The proposed pathway for synthesis of the vanillylamine moiety of capsaicinoids from L-phenylalanine is as follows: L-phenylalanine to trans-cinnamic acid by Phenyl Alanine Ammonialyase (PAL), trans-cinnamic acid to trans-p-coumaric acid by Cinnamic acid-4-hydroxylase (Ca4H), trans-p-coumaric acid to trans-caffeic acid by Coumaric acid-3-hydroxylase (Ca3H), trans-caffeic to trans-ferulic acid by Caffeic acid O-methyl transferase (CoMT), and vanillin to vanillylamine by Amino transferase (AMT) (Kehie et al., 2013). Furthermore, the proposed pathway for the biosynthesis of valine to 8-methyl-6-nonenoic acid is as follows: valine to alpha keto isovalerate by Branched Chain Amino acid Transferase (BCAT), alpha keto isovalerate to isobutyryl CoA, isobutyryl CoA to Malonyl CoA using the enzyme complex of Fatty Acid Thioesterase- Acyl Carrier Protein- Keto Acyl Synthase (FAT-Acl-KAS), Malonyl CoA to 8-methyl-6-nonenoic acid. Finally, vanillylamine and 8-methyl-6-nonenoic acid are condensed by the action of Capsaicin Synthase (CS) to obtain one molecule of capsaicin (Kehie et al., 2013). Commercially, the capsaicinoids are synthetized by the reaction of vanillylamine with 7-methyloct-5-ene1-carboxylic acid chloride.
Technological Advances for the Extraction of Capsaicinoids
Among the different upstream processes, the extraction process is the main critical phase to obtain bioactive compounds from agricultural residues. The sample preparation is critical for the appropriate release of the phytochemicals form the residue matrix, which confers the essential factors to regulate the type and amount of bioactive compounds to extract. The industrial methods (pretreatment and extraction) to obtain capsaicinoids can be categorized into two main categories: conventional and innovative techniques (Sagar et al., 2018).
The conventional methods focus in using organic solvents as the extraction vehicle of capsaicinoids, by the implementation of different techniques such as solvent, hydro-distillation, Soxhlet, and maceration extraction (Table 1). Depending on the extraction conditions, the extraction yield, quality, and bioactivity of the extracted compounds is affected. The most extensively used method to recover capsaicinoids is based on the extraction with hexane, which is very toxic and produces residues that could compromise the quality of the compounds (Martins et al., 2017). However, various extraction methods for capsaicinoids have been developed expanding the extraction solvents alternatives to methanol, ethanol, acetonitrile, and water (Lu et al., 2017). The conventional methods, involving organic solvents reach an extraction yield between 70 and 80% of total capsaicinoids and the time of extraction is inversely proportional to the polarity of the solvent (Abdurahman and Olalere, 2016). Another extraction method is the hydro-distillation, which is the simplest technique to obtain capsaicinoids from residues materials but with low yield. Jiang et al. (2013) developed the hydro-distillation with an extraction yield of only 3.1% after 2 h of process. Furthermore, the Soxhlet method is another conventional method broadly used with higher extraction yields. Boonkird et al. (2008) extracted capsaicinoids using ethanol as solvent during 8 h at 78°C to obtain up to 92% of global yield. In another study, Santos et al. (2015) extracted the capsaicinoids from Malagueta pepper (Capsicum frutescens L.) using Soxhlet extraction with dichloromethane as a solvent to shift the mixture polarity to 3.1. This solvent modification allowed a recovery of 92.1% of the capsaicinoids. Despite these techniques provide higher extract yield and total content of capsaicinoids, they required high amount of solvents and longtime extractions that increases the cost and limit its applications due to the environmental and health regulations.
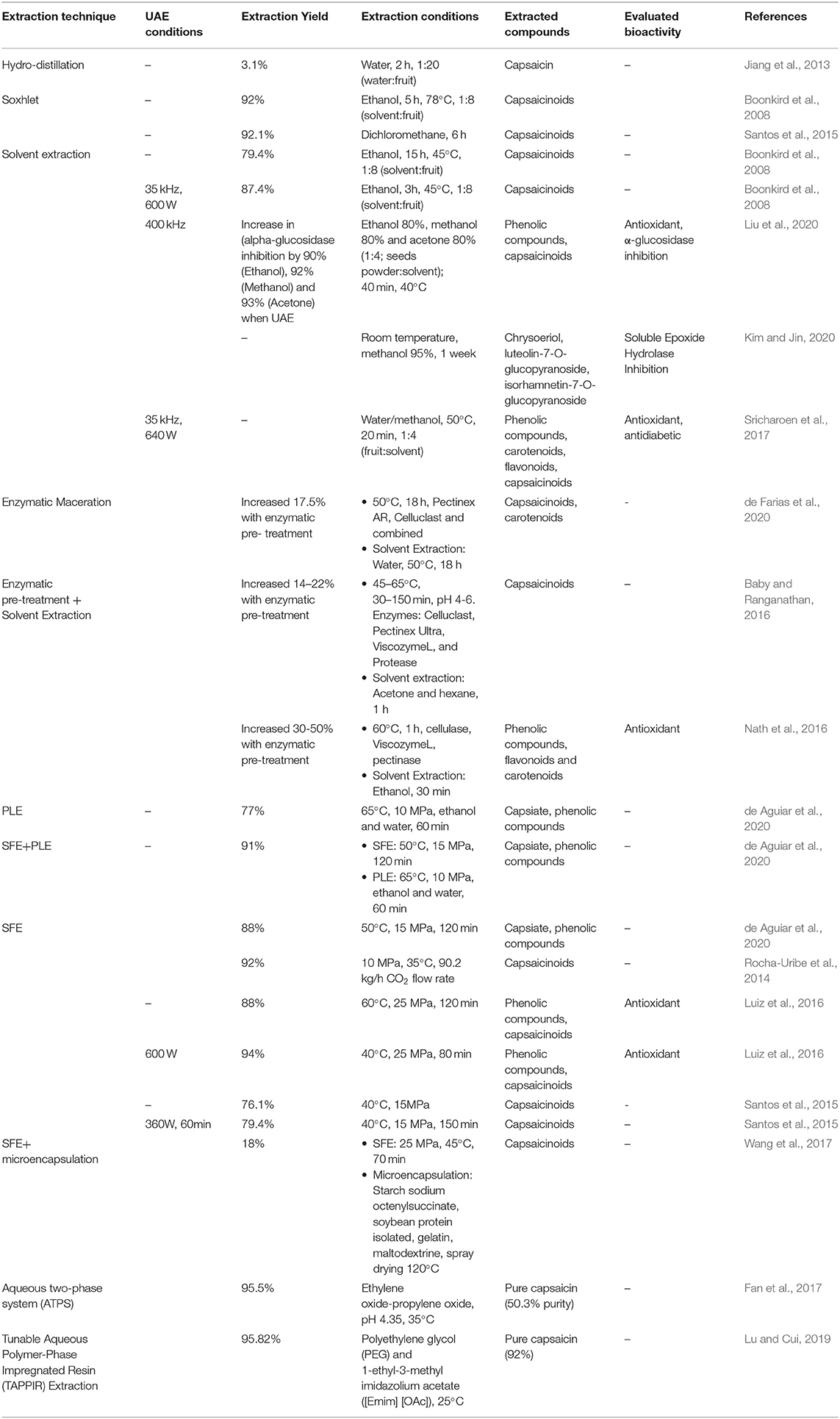
Table 1. Comparison of different extraction techniques used to recover capsaicinoids and other phytochemicals with potential beneficial effects on health.
Nowadays, due to sustainability consciousness of consumers, industry and governments, modern methods require the minimal use of solvents that could negatively affect the environment. Additionally, there is a growing requirement for new extraction methods with shortened extraction time and reduced organic solvents utilization that not only prevent pollution but also decrease extraction costs. Among innovative technologies for the extraction of bioactive compounds from food by-products are the pressurized liquid extraction, microwave assisted extraction, ultrasound assisted extraction, enzyme-assisted extraction, and supercritical fluid extraction. The selection of extraction method must be fast, low-cost, adaptable, and effective.
The pressurized liquid extraction (PLE) is a technology that required small amounts of solvents, in which depending on the polarity of the target bioactive compounds a variety of green solvents may be used. This process involves the use of elevated temperatures and pressure with the liquid solvent to increase the mass transfer of the solvents within the solid matrix. Barbero et al. (2006), evaluated the use of water, ethanol and methanol as solvents to extract capsaicinoids from different varieties of pepper from Spain using pressurized liquid technologies at 200°C, 100 bar 30 min preheating sample and extraction cycles of 5 min. The methanol extracted the highest content of capsaicinoids (450 μmol/kg), followed by ethanol (400 μmol/kg) and water (250 μmol/kg). In another study, Liu et al. (2013), determined that the optimal conditions of capsaicin (97.1%), dihydrocapsaicin (97.9%), and nordihydrocapsaicin (97.6%) extraction from commercial Capsicum annuum were 100°C and 1 cycle of 30 min. Finally, Bajer et al. (2015), used water as solvent for the total recovery of capsaicinoids (28.9 μg/g) at 20 MPa, 200°C, 20 min of static and 10 min continuous extraction. Despite its short time of process, the cartridges used limits the load of residues to process, require the pretreatment of the materials to increase the yields of extraction and further downstream process (evaporation and drying) are required to eliminate the solvents.
Another innovative technology is the microwave assisted extraction (MAE), which is a technology that uses the microwave energy to heat solvents and its increase the diffusivity within the matrix. Similarly, to the PLE, the advantages of this process is to reduce the solvents amounts and time, in which the extraction efficiency depends on medium used to match the low polarity of capsaicinoids (Nazari et al., 2007).
In terms of adaptability at an industrial scale, the ultrasound-assisted extraction (UAE) has been widely studied for the recovery of bioactive compounds from natural products (Esclapez et al., 2011). This technology focus on the cavitation principle, which consist in forming small cavities to release energy into the matrix/solvent mixture. Sricharoen et al. (2017) found that the UAE produces an oleoresin rich in capsaicinoids, phenolics, carotenoids, flavonoids, and reducing sugars that could have a synergism to induce antioxidant and antidiabetic activity (Table 1).The scale-up process is feasible due to the low cost of equipment required and that could be easily integrated into the industry. Furthermore, water could be used as medium of reaction, thus avoiding the use of organic solvents. However, downstream process is required due to the poor selectivity of the extraction process.
Another innovative technology is the enzyme assisted extraction (EAE), which is as a novel and useful way to recover bound compounds from the cell wall and increase their yield of extraction (Sagar et al., 2018). The use of enzymatic treatments coupled to the oil extraction processes such as organic solvent extraction, an novel extraction technologies improves the yield of extraction resulting into promising high percentages of recovery (Stoica et al., 2016). Enzymatic techniques have been established fundamentally for the extraction of bioactive compounds from different seeds. Different elements including catalyst type, molecular size of plant materials, and the hydrolysis time are observed as main factors for extraction. Enzymatic treatment for extraction is contemplated as an eco-friendly method to extract oils and bioactive compounds because water is used as the solvent instead of organic solvents (Sagar et al., 2018). Cellulases, pectinases, beta-glucosidases, or enzymes cocktails are used to hydrolyze and degrade cell wall components and in consequence release the nutraceutical compounds that are linked to cell wall such as carotenoids, phenolic compounds, capsaicinoids, or other compounds of interest (Walczak et al., 2018). The utilization of enzymatic processes (Table 2) as a pre-treatment to increase the extraction yield, selectivity, quality, and purity of the capsaicinoids is a novel approach of the green extraction techniques. Baby and Ranganathan (2016) demonstrated that the use of commercial enzymes like Vizcozyme L for the extraction of capsaicinoids from entire fresh green chili peppers (Capsicum annum L.) increased by 22% the extraction yield compared with the conventional solvent extraction with methanol (Table 2). Nath et al. (2016) use an enzymatic pre-treatment to extract phenolic compounds, flavonoids, and carotenoids from mature fruits of Capsicum annum L., the enzymatic activity of pectinase from Aspergillus niger spores, Viscozyme L (Beta-glucanase), and cellulase from Trichoderma reesei was evaluated through the extraction yield shown that the use of an enzymatic treatment increased 30–50% the yield compared with the control. Enzymatic maceration of fruit pulp has positive results in color and flavor (de Farias et al., 2020). Commercial enzymes such as Pectinex AR (Pectinase), Celluclast 1.5 L (Cellulase) alone or in mixture increased 17.5% the extraction yield and changed the volatile composition of the extract improving the bioactive profile (Table 2). Lu et al. (2017) described that the use of an accurate temperature with a proper agitation speed could improve significantly the yield of extraction; using commercial pectinases and carbohydrases increased the extraction yield above 88% compared with conventional techniques from dehydrated chili pepper fruits. Gamarra Mendoza et al. (2020) demonstrated that cellulolytic enzymes obtained from Aspergillus niger spores increased 5 times the extraction yield of carotenoids and capsaicinoids from Capsicum baccatum fruits in contrast with solvent extraction (hexane:methanol) (Table 2). The hydrolytic action of the cellulases on the molecular structures of the cellulose in the cell wall improve the extraction of the bioactive compounds compared with the conventional methods. Baby and Ranganathan (2016) and Gamarra Mendoza et al. (2020), proposed that the increase in the capsaicin extraction due the enzymatic treatment is also related to a higher antioxidant activity. However, the EAE ha certain limitations in terms of industrial scale-up processes. Depending on the type of enzyme to use and environmental conditions that affects the enzyme efficiency, may require a high cost of investment.
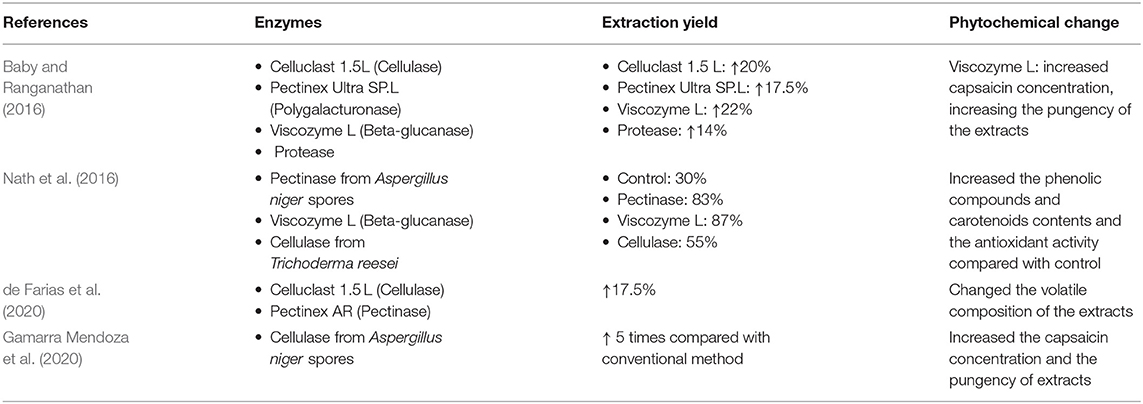
Table 2. Increased yields and phytochemical changes obtained with different enzymes used to release capsaicinoids from chili pepper.
Finally, the supercritical fluid extraction (SFE) is a green technology that uses elevated pressure and temperatures to reach a critical point, in which the solvent (carbon dioxide CO2) possess the diffusivity properties of a gas and the solvation power of a liquid. Nowadays, there a number of companies dedicated to install tailor-made industrial equipment's of SFE. For several decades the evaluation of how the different conditions of the SFE influenced into the capsaicinoids extraction yield and the quality of the extracts have generated important data to optimize and standardize these conditions. An interesting consideration in the use of the SFE is the cost of this green technology. de Aguiar et al. (2018) found that the optimal process that assured the minimal cost of manufacturing was reached at 240 min, 50°C and 15 MPa with a cost of 125.41 US$/kg of extract. However, to achieve an optimal process a profound knowledge of the mass transfer mechanism must be carried out and specific technology parts are required, which make this technology more expensive compared to UAS and EAE.
In order to enhance the yield of extraction with SFE, the use of coupled assisted technologies improve the mass transfer through convection and diffusion. Stoica et al. (2016) suggested that SFE might significantly increase the extraction yield of capsaicinoids using the most accurate combination of extraction conditions and the use of assisted technologies. Some of these assisted technologies are the use of ultrasound waves, microwaves, co-solvent extraction, enzymatic pre/during treatment. de Aguiar et al. (2020) showed that the use of SFE and PLE in the same process reduced 1.39 times the cost of manufacturing compared with the methods by separate. This suggests that the use simultaneous techniques could be economically affordable. Santos et al. (2015) improved the yield of extraction up to 77% compared to regular SFE (Table 1). The application of ultrasonic waves (US) in SFE processes as an alternative strategy to increase the extraction yield also enhance the quality of the bioactive compounds. Depending on the material matrix, US assisted SFE improved the extraction yield from the regular SFE by 6% (Luiz et al., 2016). In the case of the extraction of capsaicin using US assisted technology, Santos et al. (2015) found that the use of a US frequency of 360 W during 60 min enhanced the extraction yield up to 77% compared to regular SFE. Luiz et al. (2016) suggested that the use of 600 W frequency during 40 minutes improved the total capsaicinoids yield extraction into 45%.
Combining technologies is also relevant to produce stable ingredients with high contents of capsaicin. De Aguiar et al. (2016) applied ultrasound emulsification coupled with SFE to produce high stable emulsions rich in capsaicinoids using the modified food starch Hi-Cap100. Other interesting approach for the use of combined technologies is the use of microencapsulation by high speed homogenization and spray drying assembled with SFE to obtain highly stable capsaicinoids extracts. The process proposed by Wang et al. (2017) consisted on the homogenization of an extract obtained by SFE with starch sodium octenylsuccinate, soybean protein isolate, gelatin, and maltodextrin using high speed stirring and spray drying, giving a highly stable powder with high solubility properties that also provides additional protection for the lipophilic compounds of the capsicum oleoresins. In order to improve the bioavailability of lipophilic compounds in Capsicum oleoresins obtained by SFE, nanoemulsification with Tween 80 by coupled high pressure homogenization and ultrasonication produced highly stable nanoemulsions with good antimicrobial activity (Akbas et al., 2018).
The ultimate stage of the extraction process is the purification of the bioactive compounds. To obtain high purity capsaicin the methods currently used are the high-speed counter-current chromatography (HSCCC) and reversed-phase preparative chromatography but due to the elevated costs of the materials and equipment they are not suitable for the industrial production of pure capsaicin (Goll et al., 2013). Fan et al. (2017), propose the use of the macroporous adsorption resin (MAR) as an inexpensive alternative to obtain pure capsaicin after its extraction with an aqueous two-phase system; recovering 83.7% of the capsaicin with 50.3% of purity in the final product. Lu and Cui (2019) achieved a purity of capsaicin of 92% after Tunable Aqueous Polymer-Phase Impregnated Resin (TAPPIR) Extraction with an extraction yield of 95.82% and suggested that the combination of TAPPIR technology and reverse chromatography as a downstream processing technique is a practical approach for extracting and purifying capsaicin from the capsicum oleoresin.
Beyond extraction yield, the bioactivity of the extracts must be considered since capsaicinoids are extracted along with other phytochemicals such as carotenoids and phenolics compounds. The bioactivity of compounds like capsaicinoids, carotenoids, and phenolic acids from waste materials of chili pepper includes antioxidant activity, α-glucosidase inhibitory activity or anti-inflammatory, and immune regulatory activity (Liu et al., 2020). Sricharoen et al. (2017) found that the UAE produces an oleoresin rich in capsaicinoids, phenolics, carotenoids, flavonoids, and reducing sugars that could have a synergism to induce antioxidant and antidiabetic activity (Table 1).
Capsaicinoids in Human Health via TRPV-1 Receptor
Capsaicin selectively binds to the vanilloid receptor subtype 1 (VR1), now on referred to as TRPV-1, a member of the superfamily of transient receptor potential ion channels. Friedman et al. (2017) suggested that the synthetic capsaicin analogs are a promising class of non-pungent compounds that mimetic with TRPV-1 receptor and enhance the pro-health properties of capsaicin without the side-effects of the natural compound. TRPV-1 is expressed peripherally in primary afferent nociceptors, and it is physiologically stimulated and sensitized by external factors like heat, protons, and various inflammatory mediators such as prostaglandins, metabolites, and others that make up an “inflammatory broth” (Gerner et al., 2008). New technologies related with painkillers could be either TRPV-1 agonists or antagonists. This breaking opportunity toward the intelligent drug design of TRPV-1 modulators requires a basic understanding of how known ligands interact with TRPV-1 and which are the possible reactions that these drugs could trigger.
TRPV-1 permits ions such as calcium and sodium to pass freely through the membrane of the primary sensory/nociceptive neurons, causing depolarization and excitation and leading to nociceptive responses that are related with analgesic and other kind of reactions (Figure 2). Capsaicin exhibits high selectivity for TRPV-1 and, in a very interesting way, it does not activate the other homologous channels related with the TRPV family. In several studies, two open states of TRPV-1 were determined at atomic resolution with either capsaicin or resiniferatoxin/double knot toxin (Yang and Zheng, 2017). A small electron density was observed inside the capsaicin-binding pocket in the capsaicin-bound structure. This provided so far, the most direct evidence of the location of bound capsaicin and could indicate how this cascade of electrons intervenes into the nociceptive responses (Yang et al., 2015). In the closed (apo)state of TRPV-1, an electron density was also observed, indicating that this pocket may be occupied by a lipid molecule in the absence of capsaicin (Yang and Zheng, 2017). Consequently, capsaicin may have to compete with a lipid molecule within a similar structure in order to bind and activate TRPV-1.
Grüter et al. (2020) reported that TRPV-1 and its downstream molecules calcitonin gene-related peptide (CGRP) and Substance P are important for recognition of nociception and thermal inflammatory pain. Additionally, these authors have shown that an augmented expression of TRPV-1 in peripheral nerve terminals mediate thermal nociception in the Fabry disease mouse model. Moreover, previous studies demonstrated the key relation between TRPV-1 and P2Y receptors that are involved in the vasodilation and immune responses. The interaction of TRPV-1 and capsaicin could downregulate the receptor expression and reduce nociceptions that are proper to inhibition of pro inflammatory cytokines and oxidative stress in neurons (Khalid et al., 2019).
Anti-obesity and Gastric Disorders
Capsaicin may induce satiety by increasing the release of hormones from the intestine. In in vitro studies about stimulation of TRPV-1 with capsaicin in intestinal enteroendocrine cells, it was found a calcium-dependent release of glucagon like peptide-1 (GLP-1), which is directly related with the mediation of satiety (Szolcsányi and Pintér, 2013). GLP-1 concentration increased after a lunch that contained capsaicin (24 μg/mL of infusion in the lunch) in comparison with a placebo lunch (Van Avesaat et al., 2016). In some cases, the anti-obesity effects of capsaicinoids may be disguised by other factors related to age, sex, ethnic origin, and body composition related with a “desensitization” and a fragile response to capsaicin/TRPV-1 (Van Avesaat et al., 2016). Capsaicin induces satiety in a short amount of time (25 min) and, in consequence, decreases caloric intake, which, in turn, could lead to weight loss.
As a thermogenic compound, capsaicin in a concentration of 6 mg/kg of body weight increases catecholamine secretion from the adrenal medulla. Specific capsaicin-sensitive neurons are similarly affected by the administration of β-adrenergic blockers such the propranolol and metoprolol, drugs used in the treatment of hearth diseases and hypertension at a concentration less than a half that the chemical drugs (Figure 3) (Diepvens et al., 2007). In small rodents, after the direct administration (intraperitoneal and intragastric) of capsaicin and other capsaicinoids (135 mg/day), the whole-body energy spending significantly augmented, the adrenosympathetic nervous system was activated and by consequence increased the core temperature in 1%. These effects openly impact into the brown adipose tissue (BAT) thermogenesis, producing an anti-obesity property (Saito and Yoneshiro, 2013). Baskaran et al. (2017) demonstrated that feeding capsaicin (1 μM) boosted adipogenic and thermogenic proteins in BAT; these effects were primarily mediated by the activation of SIRT-1/PRDM-16-dependent mechanism and finally produced an anti-obesity effect. N-vanillil oleamide has been proved to increase lipolysis, decrease or inhibit adipogenesis, decrease weight of adipose tissue, modify the size and/or number of adipocytes, increase satiety and inhibit appetite, demonstrating an outstanding affinity toward the vanilloid receptor of subtype 1 (VR1/TRPV-1) (Leung, 2014).
Another potential mechanism related with the anti-obesity effects of the capsaicin is the gut microbiota. Wang et al. (2020), demonstrated that intergastrical administration of capsaicin at concentration of 2 mg/kg of body mass, suppressed obesity due to the increase of the relative abundance of some gut microorganisms such as Bacteroides, Coprococcus, Prevotella, Akkermansia, Odoribacter, and Allobaculum in obese mice. Additionally, the change in the relative abundances of SCFA-producing bacterial species enhanced acetate and propionate production by 5% compared with control which is beneficial for energy balance. Capsaicin also reduced Proteobacteria spp. such as Desulfovibrio, Escherichia, Sutterella, and Helicobacter, which can produce a chronic, low-grade inflammatory response related with higher risks of obesity (Wang et al., 2020). Previous studies demonstrated that capsaicin at concentrations greater that 10 μg/mL had bactericidal activity and inhibited the growth of certain bacteria among is H. pylori in vitro (Jones et al., 2006). Capsaicin administrated in a vehicle at concentration of 4 mM decreased H. pylori-induced gastric ulcer in human via topically or g.i., the reduction was related to the diminished IL-8 production and reduced NF-κB activity (Satyanarayana, 2006).
The gastroprotective effects of capsaicin recline in the modulation of the sensory neurons. Co-treatment of capsaicin (10 mg/kg of body mass) and L-nitro-arginine methyl ester (L-NAME, a NOS inhibitor) activated TRPV-1 located at gastric sensory neurons that stimulated the release of CGRP and NO in mice (Fattori et al., 2016). Treatment with capsaicin in mice at a concentration of 1–8 μg/mL of gastric acid level also decreased indomethacin-induced microbleeding (Mózsik et al., 2005).
Diabetes
Capsaicin consumption has a positive impact on the reduction of glucose and insulin levels in humans. Regular daily consumption of 5 mg of capsaicin diminished postprandial hyperinsulinemia in healthy adults (Yuan et al., 2016). Capsaicin also inhibits adipose tissue inflammatory responses in obesity (Kang et al., 2007). In vitro studies reported that capsaicin at a dose of 35 mg/kg of body mass overturns IL-6 and MCP-1 gene expression and protein discharge from adipose tissue and adipocytes; furthermore, dietary capsaicin significantly reduces seven times the adipose tissue macrophages and levels of inflammatory adipocytokines such as TNF-α, MCP-1, IL-6, and leptin and regularizes fasting glucose levels in obese mice (Xu et al., 2017). Obesity-related inflammatory proteins can block insulin signaling.
Recent advances in research have discovered that TRPV-1 receptors play a central role in the development and progression of diabetes type 1 and 2 (Xu et al., 2017). The ablation of TRPV-1 expressing sensory nerves by capsaicin modulates disease development and/or progression. The specific sensory nerves innervating the pancreas are major players in the progress of pancreatitis and islet inflammation and destruction. Capsaicin-induced permanent exclusion of TRPV1-expressing pancreatic sensory neurons decreases islet infiltration, insulin resistance, and β-cell stress in neonatal diabetes-prone non-obese diabetic (NOD) mice (Fattori et al., 2016). Therefore, capsaicin-induced depletion of TRPV1-expressing neurons prevents the development of diabetes in mice that are genetically predisposed to type 1 diabetes. Similarly, in Zucker diabetic fat (ZDF) rats, which are used to study various aspects of human type 2 diabetes, the capsaicin selective eradication of TRPV-1 expressing sensory fibers in the Langerhans islets prevented high plasma glucose levels and improved glucose tolerance and insulin secretion (Fattori et al., 2016).
Some studies demonstrate that resident macrophages use ATP receptors and more probably TRPV-1 receptors to, respectively, monitor beta cell activity and the acidity of the interstitial milieu in the islet. Since capsaicin activates the TRPV-1 activity, it is an important regulator of the islet activities. Identifying the microenvironment helps the macrophage gauge its production of factors that promote islet tissue stability (Yin et al., 2012). Islet macrophages are key actors in autoimmune destruction of the islet caused by type 1 diabetes and have a role in generating inflammation during type 2 diabetes (Weitz et al., 2018). The effects in diabetes are directly connected to the capsaicin-sensitive nerves in blood glucose control and mainly in the type 2 diabetes pathogenesis, since this specific diabetes type show progressive scarring of the pancreas and degenerating of the islet (Gram et al., 2017).
Interestingly, capsaicin also shields mice from the development of type 1 diabetes via TRPV-1 by a possible mechanism related to gut-mediated immune tolerance. Oral administration of 10 μg of capsaicin per kg of body mass could mitigate the proliferation and activation of autoreactive T cells in pancreatic lymph nodes (PLNs), protecting mice from diabetes development (Fattori et al., 2016).
Cardiovascular Disease
Capsaicinoids also have potential beneficial effects on the cardiovascular system. It is well-known that the cardiovascular system is plenty of capsaicin-sensitive sensory nerves, which play an extensive role in controlling cardiovascular function across the release of numerous neurotransmitters such as CGRP (Calcitonin gene-related peptide), substance P, and others. Capsaicin is able to stimulate the release of CGRP through activating TRPV-1 and consequently it has potential benefits on cardiovascular function (Peng and Li, 2010).
Capsaicin and dihydrocapsaicin inhibit platelet aggregation and the activity of clotting factors VIII and IX (Adams et al., 2009). This specific property intervenes directly to the benefits on the prevention of the onset and the lower incidence of cardiovascular diseases. Capsaicin is capable to insert into the plasma membrane of platelets and to alter membrane fluidity and/or ionic permeability (Panchal et al., 2018). Conversely, it has been discovered that TRPV-1 was indeed present in the human platelets and capsaicin was capable to induce the Calcium cation release from intracellular platelet supplies and successively contributed to ADP and thrombin induced platelet triggering (Harper et al., 2009).
The antioxidant property of capsaicinoids also contributes to their beneficial effects on cardiovascular system. The oxidation of low-density lipoprotein (LDL) is the inducting factor for the development and succession of atherosclerosis (Fattori et al., 2016). Capsaicin at concentration of 15 μg/kg of body mass reduced serum total cholesterol and lipid peroxide level in high-fat-fed rats (Manjunatha and Srinivasan, 2007). Regular ingest of chili fruits for 4 weeks (≈ 3 μg per day) or more augmented the resistance of serum lipoproteins to oxidation in adult men and women (Ahuja et al., 2006). These statements support the antioxidant property of capsaicinoids described above and their potential clinical value on the prevention of cardiovascular diseases, such as atherosclerosis, coronary heart disease, and others.
Anti-cancerogenic
Capsaicin also induces apoptosis in cancer cells and suppress carcinogenesis in some tissues like prostate, colon, skin, breast, lung, and bladder (Wang et al., 2016). In the specific case of prostate cancer, capsaicin may act by two different pathways: primarily, a direct pathway in which capsaicin acts like antagonist of coenzyme Q controlling electron transport. Increase in reactive oxygen species (ROS) induces cell damage and finally apoptosis. Secondly, in the indirect pathway, its interaction with the TRPV-1 receptor leads into the accumulation of Ca2+ cations into the cancer cells and consequently produces apoptosis (Wang et al., 2016). It is acknowledged that intracellular Ca2+ levels have a substantial effect on cancer cells and TRP channels, and they have imperative roles in intracellular oxidative stress and apoptosis. The upturn of intracellular calcium level leads to an intensification of ROS amount, mitochondrial membrane depolarization and apoptosis in cancer cells (Övey and Güler, 2020).
Furthermore, capsaicin activates the phosphorylation of the STAT3 transcriptors and also activates the pro-survival transcription factor Tyr705 p-STAT3 related to antitumoral processes such as apoptosis, mutagenesis, and tumor suppression (Bhatta et al., 2019). Moreover, these studies exposed that the use of capsaicin as complementary treatment could improve the cisplatin ototoxicity activating TRPV-1 and STAT1 with contrasting downstream signaling pathways (Bhatta et al., 2019). Chu et al. (2019) found out that capsaicin exerts a negative effect on cancer cell viability, and induced apoptosis of human melanoma via the activation of cleaved caspase-3 and PARP.
In specific cases such as prostate cancer, capsaicin results in a synergistic effect with Docetaxel activating in a more effective way the AMP-activated kinase that is related with energy homeostasis, blocks cell cycle, induces apoptosis, regulates autophagy and suppresses the anabolic processes required for rapid cell growth (Sánchez et al., 2019). Another line of evidence was presented by Mori et al. (2006) who showed that capsaicin inhibits the activation of the nuclear factor-kappa (NF-k) and tumor necrosis factor-alpha (TNF-α) in prostatic cancer cell lines. Similar evidence of anti-cancer effect has been shown in numerous conditions like gastric cancer (Huh et al., 2011; Wang et al., 2011), colon cancer (Lu et al., 2010), breast cancer (De-Sá-Júnior et al., 2013), lung cancer (Anandakumar et al., 2012), leukemia (Tsou et al., 2006), and hepatocellular carcinoma (Moon et al., 2012). Capsaicin treatment reduced significantly the metastatic load in transgenic adenocarcinoma of the mouse prostate (TRAMP) mice (Venier et al., 2015).
Zheng et al. (2016) demonstrated the activation of TRPV-1 by capsaicin blocked nuclear translocation of proliferating cell nuclear antigen and prove that TRPV-1 and capsaicin could be a target relation for the bladder cancer treatment. Likewise it was demonstrated that capsaicin drastically decreased the cell migration and invasion of cholangiocarcinoma HuCCT1 cells via the inactivation of the NF-κB/p65 signaling pathway and subsequently suppressed the expression of MMP-9. Xu et al. (2018), through the shRNA mediated knockdown of TRPV-1. Besides the role of TRPV-1 to promote the cancer cell apoptosis and avoid the damaged cell proliferation, the vanilloid receptor also possess anti-metastatic effects when it binds with capsaicin.
Anesthetic
Owing to their ability to relieve a variety of human pain disorders, the capsaicinoids (capsaicin mainly) have been used to prevent several clinical conditions related with pain at different scales including postmastectomy syndrome, urticaria, psoriasis, diabetic neuropathy, arthritis, pruritis, contact allergy, postsurgical neuromas, shingles (Herpes zoster), cluster headaches, urological disorders and many others (Howard and Wildman, 2006). Tumors progression occurs in an acidotic environment and this increases the interference of TRPV-1 antagonists that may relieve pain symptoms (Friedman et al., 2019).
Capsaicin interacts with transgerminal nerve endings, which release a neurotransmitter called substance P (neuropeptide with amino acid sequence as follows Arg-Pro-Lys-Pro-Gln-Gln-Phe-Phe-Gly-Leu-Met [RPKPQQFFGLM]). Specifically in arthritis, capsaicin stimulates the release of substance P, after recurrent application, capsaicin drains the neuron of substance P and prevents its reaccumulation (Yang and Du, 2018). In rheumatoid arthritis (RA), capsaicin-sensitive sensory afferents densely innervate the articular capsule and the synovium. A distinctive characteristic of these nerve terminals is their dual nature: as regular afferents they participate in pain signaling on the central nervous system and they also control the inflammatory reaction by performing as afferents by the liberation of sensory neuropeptides (Borbély et al., 2018).
Capsaicin triggers non-neuronal TRPV-1, which provoke the liberation of interleukin (IL-)8, IL-6, and prostaglandin E2 (PGE2) (Salari et al., 2019). Additionally, inactivation of voltage-gated Na+ channels and direct pharmacological desensitization of plasma membrane TRPV-1 receptors may contribute to an instantaneous reduction on neuronal excitability and responsiveness. Microtubule depolymerization may interject fast axonal transport. In excessive concentrations of capsaicin than those required to TRPV-1, this antagonist can also reduce mitochondria dysfunction by openly inhibiting electron chain transport (Anand and Bley, 2011).
Capsaicin activates TRPV-1, which also obstructs Piezo proteins, a family of mammalian cation-selective ion channels that react to mechanical stretch (Borbiro et al., 2015). Obstruction of Piezo proteins occurs due to calcium-dependent stimulation of phospholipase Cδ (PLCδ), which depletes phosphoinositides. In fact, injection of phosphoinositides in the cytosol by expurgated inside-out patch clamp decreases rundown inner current of Piezo channels and reverts inactivation (Della Pietra et al., 2020). Therefore, the depletion of these phosphoinositides relates with inhibition of mechanical-stimulation of Piezo channels across inhibition of inward current.
Rhinitis and Asthma
Non-allergic rhinitis (NAR) or idiopathic rhinitis (IR) may be defined as chronic nasal symptoms, such as impediment and rhinorrhea that occur in relation to non-allergic, non-infectious triggers such as change in the weather, exposure to caustic odors or cigarette smoke, and barometric pressure differences (Van Gerven et al., 2014). Intranasal application of capsaicin has potential beneficial effects in this type of diseases, while this application is firstly irritating to the applied area, it can progressively desensitize the sensory neural fibers and decrease nasal hyper-responsiveness (Van Rijswijk et al., 2003). Studies report that the desensitization of sensory nerves with capsaicin relieves the NAR and IR symptoms for up to 9 months (Fattori et al., 2016). A recent study has shown that NAR/IR is related with an amplified expression of TRPV-1 in the nasal mucosa and substance P levels in nasal secretions. Systematic studies discovered that capsaicin employs its therapeutic action by ablating TRPV1-substance P nociceptive signaling pathway in the nasal mucosa (Van Gerven et al., 2014).
Capsaicin Effects as Immuno-Stimulant and COVID-19 Treatment
Capsaicin is directly related with other immune related syndromes. TRPV-1 was described as potential goal in autoimmune diseases as well as a modulator of neuroinflammation. The TRPV-1 receptor is a target for direct immuno-modulatory pathways in immune cells, especially on regulatory macrophages (Pedreiturria et al., 2018; Weitz et al., 2018). Nevius et al. (2012) showed that a capsaicin concentration of 10 μg by oral administration producing IL-10 instead of inflammatory cytokines in an autoimmune diabetes mouse model. This receptor is also known as part of indirectly immunomodulatory pathways when expressed in unmyelinated nerve fibers (Pedreiturria et al., 2018).
Nowadays, the importance of the enhancement of the immune system becomes an important public health care issue due to the SARS-Cov-2 pandemic outbreak. From the immunity point of view, COVID-19 patients have a lower level of lymphocytes, especially Natural Killer (NK) cells. Additionally, a mined immune system revealed the atrophy of spleen and lymph nodes along with the minimal lymphocytes infiltration into the lung lesion that leads the majority infiltration of monocytes and macrophages that conducts to the early endotracheal intubation. Finally, all these processes conclude into a mimicry of hypercoagulability, vasculitis, and multiple organs damage (Zhang et al., 2020).
The newest studies shown that the SARS-Cov-2 virus enters the cell via the angiotensin-converting enzyme-2 (ACE-2) (Prompetchara et al., 2020) and it is identified mostly by Toll-like receptor 7 (TLR7). Activation leads to the production of alpha interferon and the secretion of IL-12 and IL-6 and the production of CD8+ specific cytotoxic T cells that through the CD4+ T cell form the antigen-specific B cells and antibody production (Ahmadpoor and Rostaing, 2020). Giamarellos-Bourboulis et al. (2020), demonstrated that more than one fourth of SARS-Cov-2 patients shown Severe Respiratory Failure (SRF) and later can develop Macrophage Activation Syndrome (MAS), that finally leads into a general immune dysregulation subjugated by low expression of HLA-DR on CD14 monocytes, which is triggered by monocyte hyperactivation, disproportionate liberation of IL-6, and intense lymphopenia. All these combined pathogeneses provoked by the virus proliferation produce, in the majority of the cases, a cytokine storm also called Cytokine Release Syndrome (CRS) and symptoms of sepsis that are the cause of death in 28% of fatal COVID-19 cases. In all these cases the uncontrolled inflammation perpetrates multi-organ damage leading to organ failure, especially of the cardiac, hepatic, and renal systems, that eventually conducts to the death in the great majority of the clinical cases (Shi et al., 2020; Zirui Tay et al., 2020).
An interesting approach by Janda and Iadarola (2020) suggest that TRPV-1 receptor plays an important role in the prognosis of a very specific viral infections such as COVID-19. Some preclinical studies propose that since SARS-Cov-2 is linked to a strong immune response and an immense chain of reactions related with the immune system, the possible inhibition of afferent activity TRPV-1 fibers from the lung and airways could have a beneficial consequence on the compromised lung function. The scoop that could connect the TRPV-1 receptor to the COVID-19 is primarily based on finding a potent TRPV-1 agonist that can down regulate the inflammatory response. According with Nahama et al. (2020), the connection of TRPV-1 expressing innervation combined with the virally focused hyperinflammation in COVID-19 cases may be the root cause of the lethal characteristic of the disease particularly for the mature patients. They propose that interfering TRPV-1 signaling might decline the severity of the acute respiratory distress syndrome (ARDS) present in COVID-19 patients. Blocking lung sensory neurons signaling might result in inactivation of efferent system decreasing associated inflammation and cytokine storm adverse effects. Suppressing TRPV-1 afferent pathways as part of the treatment for COVID19-related pneumonia/ARDS will not only tackle the inherent pathophysiology of the viral infection and systemic inflammation but would also help to decrease the complications connected with the current gold-standard therapy (mechanical ventilation/oxygen therapy) in severely compromised patients. Silencing TRPV-1 positive nerve fibers could ultimately be additionally of interest to limit the progression of the disease from mild stages to acute respiratory distress.
Since numerous studies demonstrated that the protein responsible of the replication of the virus is the viral protease 3CL-protease and that the inhibition of this enzyme can stop the virus replication in the human body (Chen et al., 2005). This enzyme owns three functional domains where different compounds can bind and inhibit it, stopping the virus replication (Kumar et al., 2020). The major international research about possible treatments against the COVID-19 goes focused to develop or elucidate possible inhibition routes of the 3CL-Protease activity, exploring the use of a numerous group of chemical compounds like azithromycin, oseltamivir, ritonavir, indinavir, remdesivir, and other drugs used in diverse viral diseases as influenza A and B, and HIV (Kadil et al., 2020). Also based on molecular docking analysis, Das et al. (2020) reported a total of 33 compounds including natural products such as capsaicin, to investigate their blocking effects toward 3CL-protease. The capsaicin formed hydrogen bonds with 4 different sites of 3CL-protease (THR190, CYS145, HIS163, and PRO168) with an estimated free energy of binding of −8.15 kcal/mol. In another preliminary study, capsaicin was found to be in the active site and interacted with GLU166 hydrogen bonds but could not exhibit good binding affinity toward target enzyme (Barros et al., 2020).
Furthermore, RNA-dependent RNA polymerase (RdRp), is a key enzyme in virus life cycle for replication and a potential target to find therapeutic agents for COVID-19 (Elfiky, 2020). The molecular docking results of capsaicin with RNA dependent RNA polymerase (RdRp) showed a free energy of binding of −7.3 kcal/mol, similar to remdessivir drug with a value of −9.0 Kcal/mol (Elfiky, 2020). These results suggest that capsaicin may be used as COVID-19 antiprotease drug to prevent the viral replication of SARS-CoV-2. Efficient therapeutic strategies are important to identify drugs for novel viruses that influence the life cycle of viruses by inhibiting the entry and stopping the viral replication or targeting intracellular signal transduction pathways in order to treat the disease or control the further spread of the virus.
The actual fact that capsaicin acts like a TRPV-1 agonist, an anti-inflammatory compound and that could be used as a 3CL-protease and/or RNA-dependent RNA polymerase (RdRp) inhibitor, suggest that the study and subsequent development of a clinical product containing this chili pepper main compound or its derivatives could be promising to the COVID-19 alternative clinical treatment.
Conclusions
Chili pepper waste product are a potential source of capsaicin and other possible analogs that may interact with TRPV-1 receptor. The industrial and technological approach to the extraction, purification and possible use of these bioactive compounds from waste materials is an important field to focus the scientific efforts. Based on the identification of molecular targets, capsaicin along with other phytochemicals that may be recovered from waste could be incorporated into a new generation of nutraceuticals to motivate a greener production in Mexico and other countries. From the commercial point of view, capsaicin is considered an active ingredient to prevent or treat obesity, gastric disorders, arthritis, asthma, diabetes, and immune system diseases and combinations with other phytochemicals may improve its potential benefits for health.
Author Contributions
HC-F, DG-F, and GR-D: compiled, reviewed, and wrote this paper. JG-U: conceived and supervised this work. All authors: have read and approved the final manuscript.
Funding
This work was financially supported by the Consejo Nacional de Ciencia y Tecnología (CONACyT) for the scholarship 2018-000068-02NACF-07952 and by the Instituto Tecnológico de Estudios Superiores de Monterrey and CSIC i-Link project LINKB20023.
Conflict of Interest
The authors declare that the research was conducted in the absence of any commercial or financial relationships that could be construed as a potential conflict of interest.
References
Abdurahman, N. H., and Olalere, O. A. (2016). A comparative review of conventional and microwave assisted extraction in capsaicin isolation from chili pepper. Aust. J. Basic Appl. Sci. 10, 263–275.
Adams, M. J., Ahuja, K. D. K., and Geraghty, D. P. (2009). Effect of capsaicin and dihydrocapsaicin on in vitro blood coagulation and platelet aggregation. Thromb. Res. 124, 721–723. doi: 10.1016/j.thromres.2009.05.001
Ahmadpoor, P., and Rostaing, L. (2020). Why the immune system fails to mount an adaptive immune response to a COVID-19 infection. Transpl. Int. 33, 824–825. doi: 10.1111/tri.13611
Ahuja, K. D. K., Kunde, D. A., Ball, M. J., and Geraghty, D. P. (2006). Effects of capsaicin, dihydrocapsaicin, and curcumin on copper-induced oxidation of human serum lipids. J. Agric. Food Chem. 54, 6436–6439. doi: 10.1021/jf061331j
Akbas, E., Soyler, B., Oztop, M. H., Uarrota, V. G., Maraschin, M., de Bairros, Â., et al. (2018). Formation of capsaicin loaded nanoemulsions with high pressure homogenization and ultrasonication. LWT 96, 266–273. doi: 10.1016/j.lwt.2018.05.043
Anand, P., and Bley, K. (2011). Topical capsaicin for pain management: therapeutic potential and mechanisms of action of the new high-concentration capsaicin 8 patch. Br. J. Anaesth. 107, 490–502. doi: 10.1093/bja/aer260
Anandakumar, P., Kamaraj, S., Jagan, S., Ramakrishnan, G., Asokkumar, S., Naveenkumar, C., et al. (2012). Capsaicin inhibits benzo(a)pyrene-induced lung carcinogenesis in an in vivo mouse model. Inflamm. Res. 61, 1169–1175. doi: 10.1007/s00011-012-0511-1
Aza-González, C., Núñez-Palenius, H. G., and Ochoa-Alejo, N. (2011). Molecular biology of capsaicinoid biosynthesis in chili pepper (Capsicum spp.). Plant Cell Rep. 30, 695–706. doi: 10.1007/s00299-010-0968-8
Baby, K. C., and Ranganathan, T. V. (2016). Effect of enzyme pretreatment on yield and quality of fresh green chilli (Capsicum annuum L) oleoresin and its major capsaicinoids. Biocatal. Agric. Biotechnol. 7, 95–101. doi: 10.1016/j.bcab.2016.05.010
Bajer, T., Bajerová, P., Kremr, D., Eisner, A., and Ventura, K. (2015). Central composite design of pressurised hot water extraction process for extracting capsaicinoids from chili peppers. J. Food Compos. Anal. 40, 32–38. doi: 10.1016/j.jfca.2014.12.008
Barbero, G. F., Palma, M., and Barroso, C. G. (2006). Pressurized liquid extraction of capsaicinoids from peppers. J. Agric. Food Chem. 54, 3231–3236. doi: 10.1021/jf060021y
Barros, R. O., Junior, F. L. C. C., Pereira, W. S., Oliveira, N. M. N., and Ramos, R. M. (2020). Interaction of drugs candidates with various SARS-CoV-2 receptors: an in silico study to combat COVID-19. J. Proteome Res. 11, 4567–4575. doi: 10.26434/CHEMRXIV.12100968.V4
Baskaran, P., Krishnan, V., Fettel, K., Gao, P., Zhu, Z., Ren, J., et al. (2017). TRPV1 activation counters diet-induced obesity through sirtuin-1 activation and PRDM-16 deacetylation in brown adipose tissue. Int. J. Obes. 41, 739–749. doi: 10.1038/ijo.2017.16
Bhatta, P., Dhukhwa, A., Sheehan, K., Al Aameri, R. F. H., Borse, V., Ghosh, S., et al. (2019). Capsaicin protects against Cisplatin ototoxicity by changing the STAT3/STAT1 ratio and activating cannabinoid (CB2) receptors in the cochlea. Sci. Rep. 9:4131. doi: 10.1038/s41598-019-40425-9
Boonkird, S., Phisalaphong, C., and Phisalaphong, M. (2008). Ultrasound-assisted extraction of capsaicinoids from Capsicum frutescens on a lab-and pilot-plant scale. Ultrason. Sonochem. 15, 1075–1079. doi: 10.1016/j.ultsonch.2008.04.010
Borbély, É., Kiss, T., Szabadfi, K., Pintér, E., Szolcsányi, J., Helyes, Z., et al. (2018). Complex role of capsaicin-sensitive afferents in the collagen antibody-induced autoimmune arthritis of the mouse. Sci. Rep. 8:15916. doi: 10.1038/s41598-018-34005-6
Borbiro, I., Badheka, D., and Rohacs, T. (2015). Activation of TRPV1 channels inhibits mechanosensitive piezo channel activity by depleting membrane phosphoinositides. Sci. Signal. 8:ra15. doi: 10.1126/scisignal.2005667
Carmona, I. (2013). Situación global de especias y condimentos : una oportunidad para el ají procesado picante. Agrimundo Inteligencia Competitiva para el Sector Agroalimentario. 1–5. Available online at: https://chilealimentos.com/situacion-global-de-especias-y-condimentos-una-oportunidad-para-el-aji-procesado-picante/
Chen, L. R., Wang, Y. C., Yi, W. L., Chou, S. Y., Chen, S. F., Lee, T. L., et al. (2005). Synthesis and evaluation of isatin derivatives as effective SARS coronavirus 3CL protease inhibitors. Bioorganic Med. Chem. Lett. 15, 3058–3062. doi: 10.1016/j.bmcl.2005.04.027
Chouaibi, M., Rezig, L., Hamdi, S., and Ferrari, G. (2019). Chemical characteristics and compositions of red pepper seed oils extracted by different methods. Ind. Crops Prod. 128, 363–370. doi: 10.1016/j.indcrop.2018.11.030
Chu, H., Li, M., and Wang, X. (2019). Capsaicin induces apoptosis and autophagy in human melanoma cells. Oncol. Lett. 17, 4827–4834. doi: 10.3892/ol.2019.10206
Das, S., Sarmah, S., Lyndem, S., and Singha Roy, A. (2020). An investigation into the identification of potential inhibitors of SARS-CoV-2 main protease using molecular docking study. J. Biomol. Struct. Dyn. doi: 10.1080/07391102.2020.1763201. [Epub ahead of print].
de Aguiar, A. C., Osorio-Tobón, J. F., Silva, L. P. S., Barbero, G. F., and Martínez, J. (2018). Economic analysis of oleoresin production from malagueta peppers (Capsicum frutescens) by supercritical fluid extraction. J. Supercrit. Fluids 133, 86–93. doi: 10.1016/j.supflu.2017.09.031
de Aguiar, A. C., Osorio-Tobón, J. F., Viganó, J., and Martínez, J. (2020). Economic evaluation of supercritical fluid and pressurized liquid extraction to obtain phytonutrients from biquinho pepper: analysis of single and sequential-stage processes. J. Supercrit. Fluids 165:104935. doi: 10.1016/j.supflu.2020.104935
De Aguiar, A. C., Silva, L. P. S., Alves De Rezende, C., Barbero, G. F., and Martínez, J. (2016). Encapsulation of pepper oleoresin by supercritical fluid extraction of emulsions. J. Supercrit. Fluids 112, 37–43. doi: 10.1016/j.supflu.2016.02.009
de Farias, V. L., da Araújo, Í. M. S., da Rocha, R. F. J., dos Garruti, D. S., and Pinto, G. A. S. (2020). Enzymatic maceration of Tabasco pepper: effect on the yield, chemical and sensory aspects of the sauce. Lwt 127:109311. doi: 10.1016/j.lwt.2020.109311
Della Pietra, A., Mikhailov, N., and Giniatullin, R. (2020). The emerging role of mechanosensitive Piezo channels in migraine pain. Int. J. Mol. Sci. 21:696. doi: 10.3390/ijms21030696
De-Sá-Júnior, P. L., Pasqualoto, K. F. M., Ferreira, A. K., Tavares, M. T., Damião, M. C. F. C. B., De Azevedo, R. A., et al. (2013). RPF101, A new capsaicin-like analogue, disrupts the microtubule network accompanied by arrest in the G2/M phase, inducing apoptosis and mitotic catastrophe in the MCF-7 breast cancer cells. Toxicol. Appl. Pharmacol. 266, 385–398. doi: 10.1016/j.taap.2012.11.029
Diepvens, K., Westerterp, K. R., and Westerterp-Plantenga, M. S. (2007). Obesity and thermogenesis related to the consumption of caffeine, ephedrine, capsaicin, and green tea. Am. J. Physiol. Regul. Integr. Comp. Physiol. 292, R77–R85. doi: 10.1152/ajpregu.00832.2005
Elfiky, A. A. (2020). Ribavirin, remdesivir, sofosbuvir, galidesivir, and tenofovir against SARS-CoV-2 RNA dependent RNA polymerase (RdRp): a molecular docking study. Life Sci. 253:117592. doi: 10.1016/j.lfs.2020.117592
Esclapez, M. D., García-Pérez, J. V., Mulet, A., and Cárcel, J. A. (2011). Ultrasound-assisted extraction of natural products. Food Eng. Rev. 3, 108–120. doi: 10.1007/s12393-011-9036-6
Fan, Y., Lu, Y., min Yu, B., Tan, C., and ping Cui, B. (2017). Extraction and purification of capsaicin from capsicum oleoresin using an aqueous two-phase system combined with chromatography. J. Chromatogr. B Anal. Technol. Biomed. Life Sci. 1063, 11–17. doi: 10.1016/j.jchromb.2017.07.006
Fattori, V., Hohmann, M. S. N., Rossaneis, A. C., Pinho-Ribeiro, F. A., and Verri, W. A. (2016). Capsaicin: current understanding of its mechanisms and therapy of pain and other pre-clinical and clinical uses. Molecules 21:844. doi: 10.3390/molecules21070844
Fayos, O., Savirón, M., Orduna, J., Barbero, G. F., Mallor, C., and Garcés-Claver, A. (2019). Quantitation of capsiate and dihydrocapsiate and tentative identification of minor capsinoids in pepper fruits (Capsicum spp.) by HPLC-ESI-MS/MS(QTOF). Food Chem. 270, 264–272. doi: 10.1016/j.foodchem.2018.07.112
Friedman, J. R., Perry, H. E., Brown, K. C., Gao, Y., Lin, J., Stevenson, C. D., et al. (2017). Capsaicin synergizes with camptothecin to induce increased apoptosis in human small cell lung cancers via the calpain pathway. Biochem. Pharmacol. 129, 54–66. doi: 10.1016/j.bcp.2017.01.004
Friedman, J. R., Richbart, S. D., Merritt, J. C., Brown, K. C., Denning, K. L., Tirona, M. T., et al. (2019). Capsaicinoids: multiple effects on angiogenesis, invasion and metastasis in human cancers. Biomed. Pharmacother. 118:109317. doi: 10.1016/j.biopha.2019.109317
Gamarra Mendoza, N., Velasquez Rodriguez, S., and Roque Lima, B. (2020). Improvement of the extraction of carotenoids and capsaicinoids of chili pepper native (Capsicum baccatum), assisted with cellulolytic enzymes. Rev. Peru. Biol. 27, 55–060. doi: 10.15381/rpb.v27i1.17588
Gerner, P., Binshtok, A. M., Wang, C. F., Hevelone, N. D., Bean, B. P., Woolf, C. J., et al. (2008). Capsaicin combined with local anesthetics preferentially prolongs sensory/nociceptive block in rat sciatic nerve. Anesthesiology 109, 872–878. doi: 10.1097/ALN.0b013e31818958f7
Giamarellos-Bourboulis, E. J., Netea, M. G., Rovina, N., Akinosoglou, K., Antoniadou, A., Antonakos, N., et al. (2020). Complex immune dysregulation in COVID-19 patients with severe respiratory failure. Cell Host Microbe 27, 992–1000.e3. doi: 10.1016/j.chom.2020.04.009
Goll, J., Frey, A., and Minceva, M. (2013). Study of the separation limits of continuous solid support free liquid-liquid chromatography: separation of capsaicin and dihydrocapsaicin by centrifugal partition chromatography. J. Chromatogr. A 1284, 59–68. doi: 10.1016/j.chroma.2013.01.116
Gram, D. X., Holst, J. J., and Szallasi, A. (2017). TRPV1: a potential therapeutic target in type 2 diabetes and comorbidities? the human and monetary cost of type 2 diabetes: a need for novel therapeutic interventions. Trends Mol. Med. 23, 1002–1013. doi: 10.1016/j.molmed.2017.09.005
Grüter, T., Blusch, A., Motte, J., Sgodzai, M., Bachir, H., Klimas, R., et al. (2020). Immunomodulatory and anti-oxidative effect of the direct TRPV1 receptor agonist capsaicin on Schwann cells. J. Neuroinflammation 17:145. doi: 10.1186/s12974-020-01821-5
Harper, A. G. S., Brownlow, S. L., and Sage, S. O. (2009). A role for TRPV1 in agonist-evoked activation of human platelets. J. Thromb. Haemost. 7, 330–338. doi: 10.1111/j.1538-7836.2008.03231.x
Howard, L. R., and Wildman, R. E. (2006). “Antioxidant vitamin and phytochemical content of fresh and processed pepper fruit (Capsicum annuum),” in Handbook of Nutraceuticals and Functional Foods, 2nd Edn, eds R. E. C. Wildman, R. Wildman, and T. C. Wallace (Boca Raton, FL: CRC Press), 165–191.
Huh, H. C., Lee, S. Y., Lee, S. K., Park, N. H., and Han, I. S. (2011). Capsaicin induces apoptosis of cisplatin-resistant stomach cancer cells by causing degradation of cisplatin-inducible aurora-A protein. Nutr. Cancer 63, 1095–1103. doi: 10.1080/01635581.2011.607548
Hursel, R., and Westerterp-Plantenga, M. S. (2010). Thermogenic ingredients and body weight regulation. Int. J. Obes. 34, 659–669. doi: 10.1038/ijo.2009.299
Janda, K. D., and Iadarola, M. J. (2020). Potential TRPV1 blockade to treat severe lung dysfunction in COVID-19 infection. Med. Drug Discov. 6:100034. doi: 10.1016/j.medidd.2020.100034
Jiang, Z.-T., Feng, X., Li, R., and Wang, Y. (2013). Composition comparison of essential oils extracted by classical hydro distillation and microwave-assisted hydrodistillation from Pimenta dioica. J. Essent. Oil Bear. Plants 16, 45–50. doi: 10.1080/0972060X.2013.764178
Jones, N. L., Shabib, S., and Sherman, P. M. (2006). Capsaicin as an inhibitor of the growth of the gastric pathogen Helicobacter pylori. FEMS Microbiol. Lett. 146, 223–227. doi: 10.1111/j.1574-6968.1997.tb10197.x
Kadil, Y., Mouhcine, M., and Filali, H. (2020). In silico study of pharmacological treatments against SARS-CoV2 main protease. J. Pure Appl. Microbiol. 14, 1065–1071. doi: 10.22207/JPAM.14.SPL1.45
Kang, J. H., Kim, C. S., Han, I. S., Kawada, T., and Yu, R. (2007). Capsaicin, a spicy component of hot peppers, modulates adipokine gene expression and protein release from obese-mouse adipose tissues and isolated adipocytes, and suppresses the inflammatory responses of adipose tissue macrophages. FEBS Lett. 581, 4389–4396. doi: 10.1016/j.febslet.2007.07.082
Kehie, M., Kumaria, S., and Tandon, P. (2013). In vitro plantlet regeneration from cotyledon segments of Capsicum chinense Jacq. cv. Naga King Chili, and determination of capsaicin content in fruits of in vitro propagated plants by high performance liquid chromatography. Sci. Hortic. 164, 1–8. doi: 10.1016/j.scienta.2013.08.018
Khalid, S., Khan, A., Shal, B., Ali, H., Kim, Y. S., and Khan, S. (2019). Suppression of TRPV1 and P2Y nociceptors by honokiol isolated from Magnolia officinalis in 3 rd degree burn mice by inhibiting inflammatory mediators. Biomed. Pharmacother. 114:108777. doi: 10.1016/j.biopha.2019.108777
Kim, J. H., and Jin, C. H. (2020). Inhibitory activity of flavonoids, chrysoeriol and luteolin-7-O-glucopyranoside, on soluble epoxide hydrolase from Capsicum chinense. Biomolecules, 10:180. doi: 10.3390/biom10020180
Kumar, A., Choudhir, G., Shukla, S. K., Sharma, M., Tyagi, P., Bhushan, A., et al. (2020). Identification of phytochemical inhibitors against main protease of COVID-19 using molecular modeling approaches. J. Biomol. Struct. Dyn. doi: 10.1080/07391102.2020.1772112. [Epub ahead of print].
Leung, F. W. (2014). Capsaicin as an anti-obesity drug. Prog. Drug Res. 68, 171–179. doi: 10.1007/978-3-0348-0828-6_7
Liu, A., Han, C., Zhou, X., Zhu, Z., Huang, F., and Shen, Y. (2013). Determination of three capsaicinoids in Capsicum annuum by pressurized liquid extraction combined with LC-MS/MS. J. Sep. Sci. 36, 857–862. doi: 10.1002/jssc.201200942
Liu, Y., Chen, Y., Wang, Y., Chen, J., Huang, Y., Yan, Y., et al. (2020). Total phenolics, capsaicinoids, antioxidant activity, and α-glucosidase inhibitory activity of three varieties of pepper seeds. Int. J. Food Prop. 23, 1016–1035. doi: 10.1080/10942912.2020.1775646
Lu, H. F., Chen, Y. L., Yang, J. S., Yang, Y. Y., Liu, J. Y., Hsu, S. C., et al. (2010). Antitumor activity of capsaicin on human colon cancer cells in vitro and colo 205 tumor xenografts in vivo. J. Agric. Food Chem. 58, 12999–13005. doi: 10.1021/jf103335w
Lu, M., Ho, C. T., and Huang, Q. (2017). Extraction, bioavailability, and bioefficacy of capsaicinoids. J. Food Drug Anal. 25, 27–36. doi: 10.1016/j.jfda.2016.10.023
Lu, Y., and Cui, B. (2019). Extraction and purification of capsaicin from Capsicum oleoresin using a combination of Tunable Aqueous Polymer-Phase Impregnated Resin (TAPPIR) extraction and chromatography technology. Molecules 24:3956. doi: 10.3390/molecules24213956
Luiz, A., Dias, B., Scarelli, C., Sergio, A., Santos, P., Fernandéz Barbero, G., et al. (2016). Effect of ultrasound on the supercritical CO 2 extraction of bioactive compounds from dedo de moça pepper (Capsicum baccatum L. var. pendulum). Ultrason. Sonochem. 31, 284–294. doi: 10.1016/j.ultsonch.2016.01.013
Luo, X. J., Peng, J., and Li, Y. J. (2011). Recent advances in the study on capsaicinoids and capsinoids. Eur. J. Pharmacol. 650, 1–7. doi: 10.1016/j.ejphar.2010.09.074
Manjunatha, H., and Srinivasan, K. (2007). Hypolipidemic and antioxidant effects of curcumin and capsaicin in high-fat-fed rats. Can. J. Physiol. Pharmacol. 85, 588–596. doi: 10.1139/Y07-044
Martins, F. S., Borges, L. L., Ribeiro, C. S. C., Reifschneider, F. J. B., and Conceição, E. C. (2017). Novel approaches to extraction methods in recovery of capsaicin from habanero pepper (CNPH 15.192). Pharmacogn. Mag. 13, S375–S379. doi: 10.4103/0973-1296.210127
Meghvansi, M. K., Siddiqui, S., Khan, M. H., Gupta, V. K., Vairale, M. G., Gogoi, H. K., et al. (2010). Naga chilli: a potential source of capsaicinoids with broad-spectrum ethnopharmacological applications. J. Ethnopharmacol. 132, 1–14. doi: 10.1016/j.jep.2010.08.034
Moon, D. O., Kang, C. H., Kang, S. H., Choi, Y. H., Hyun, J. W., Chang, W. Y., et al. (2012). Capsaicin sensitizes TRAIL-induced apoptosis through Sp1-mediated DR5 up-regulation: involvement of Ca 2+ influx. Toxicol. Appl. Pharmacol. 259, 87–95. doi: 10.1016/j.taap.2011.12.010
Mori, A., Lehmann, S., O'Kelly, J., Kumagai, T., Desmond, J. C., Pervan, M., et al. (2006). Capsaicin, a component of red peppers, inhibits the growth of androgen-independent, p53 mutant prostate cancer cells. Cancer Res. 66, 3222–3229. doi: 10.1158/0008-5472.CAN-05-0087
Mózsik, G., Szolcsányi, J., and Rácz, I. (2005). Gastroprotection induced by capsaicin in healthy human subjects. World J. Gastroenterol. 11, 5180–5184. doi: 10.3748/wjg.v11.i33.5180
Nahama, A., Ramachandran, R., Cisternas, A. F., and Ji, H. (2020). The role of afferent pulmonary innervation in ARDS associated with COVID-19 and potential use of resiniferatoxin to improve prognosis: a review. Med. Drug Discov. 5:100033. doi: 10.1016/j.medidd.2020.100033
Nath, P., Kaur, C., Rudra, S. G., and Varghese, E. (2016). Enzyme-assisted extraction of carotenoid-rich extract from red capsicum (Capsicum annuum). Agric. Res. 5, 193–204. doi: 10.1007/s40003-015-0201-7
Nazari, F., Ebrahimi, S. N., Talebi, M., Rassouli, A., and Bijanzadeh, H. R. (2007). Multivariate optimisation of microwave-assisted extraction of capsaicin from Capsicum frutescens L. and quantitative analysis by1H-NMR. Phytochem. Anal. 18, 333–340. doi: 10.1002/pca.987
Nevius, E., Srivastava, P. K., and Basu, S. (2012). Oral ingestion of capsaicin, the pungent component of chili pepper, enhances a discreet population of macrophages and confers protection from autoimmune diabetes. Mucosal Immunol. 5, 76–86. doi: 10.1038/mi.2011.50
Oguzkan, S. B. (2019). Extraction of capsinoid and its analogs from pepper waste of different genotypes. Nat. Prod. Commun. 14:1934578X19865673. doi: 10.1177/1934578X19865673
Övey, I. S., and Güler, Y. (2020). Apoptotic efficiency of capecitabine and 5-fluorouracil on human cancer cells through TRPV1 channels. Indian J. Biochem. Biophys. 57, 64–72.
Panchal, S. K., Bliss, E., and Brown, L. (2018). Capsaicin in metabolic syndrome. Nutrients 10:630. doi: 10.3390/nu10050630
Pedreiturria, X., Motte, J., Bachir, H., Ambrosius, B., Gold, R., Sgodzai, M., et al. (2018). Capsaicin-enriched diet ameliorates autoimmune neuritis in rats. J. Neuroinflammation 15:122. doi: 10.1186/s12974-018-1165-x
Peng, J., and Li, Y. J. (2010). The vanilloid receptor TRPV1: role in cardiovascular and gastrointestinal protection. Eur. J. Pharmacol. 627, 1–7. doi: 10.1016/j.ejphar.2009.10.053
Prompetchara, E., Ketloy, C., and Palaga, T. (2020). Immune responses in COVID-19 and potential vaccines: Lessons learned from SARS and MERS epidemic. Asian Pac. J. Allergy Immunol. 38, 1–9. doi: 10.12932/AP-200220-0772
Rocha-Uribe, J. A., Novelo-P?rez, J. I., and Ruiz-Mercado, C. A. (2014). Cost estimation for CO2 supercritical extraction systems and manufacturing cost for habanero Chili. J. Supercr. Fluids 93, 38–41. doi: 10.1016/j.supflu.2014.03.014
Rudra, S. G., Nishad, J., Jakhar, N., and Kaur, C. (2015). Food industry waste: mine of nutraceuticals. Int. J. Sci. Environ. Technol. 4, 205–229. doi=10.1.1.680.1213&rep=rep1&type=pdf
Sagar, N. A., Pareek, S., Sharma, S., Yahia, E. M., and Lobo, M. G. (2018). Fruit and vegetable waste: Bioactive compounds, their extraction, and possible utilization. Compr. Rev. Food Sci. Food Saf. 17, 512–531. doi: 10.1111/1541-4337.12330
SAGARPA (2017). Agrícola Nacional. Planeación agrícola Nac. 2017-2030 I, 1–14. Available online at: https://www.gob.mx/cms/uploads/attachment/file/255627/Planeaci_n_Agr_cola_Nacional_2017-2030-_parte_uno.pdf
Saito, M., and Yoneshiro, T. (2013). Capsinoids and related food ingredients activating brown fat thermogenesis and reducing body fat in humans. Curr. Opin. Lipidol. 24, 71–77. doi: 10.1097/MOL.0b013e32835a4f40
Salari, M., Salari, R., Rafatpanah, H., Ravanshad, Y., Zirachi, D., and Sahebari, M. (2019). Skin inflammatory reactions to capsaicin in rheumatoid arthritis patients compared to healthy controls. Avicenna J. Phytomed. 9, 54–61.
Sanati, S., Razavi, B. M., and Hosseinzadeh, H. (2018). A review of the effects of Capsicum annuum L. and its constituent, capsaicin, in metabolic syndrome. Iran. J. Basic Med. Sci. 21, 439–448. doi: 10.22038/IJBMS.2018.25200.6238
Sánchez, B. G., Bort, A., Mateos-Gómez, P. A., Rodríguez-Henche, N., and Díaz-Laviada, I. (2019). Combination of the natural product capsaicin and docetaxel synergistically kills human prostate cancer cells through the metabolic regulator AMP-activated kinase. Cancer Cell Int. 19, 1–14. doi: 10.1186/s12935-019-0769-2
Sandoval-Castro, C. J., Valdez-Morales, M., Oomah, B. D., Gutiérrez-Dorado, R., Medina-Godoy, S., and Espinosa-Alonso, L. G. (2017). Bioactive compounds and antioxidant activity in scalded Jalapeño pepper industrial byproduct (Capsicum annuum). J. Food Sci. Technol. 54, 1999–2010. doi: 10.1007/s13197-017-2636-2
Santos, P., Aguiar, A. C., Barbero, G. F., Rezende, C. A., and Martínez, J. (2015). Supercritical carbon dioxide extraction of capsaicinoids from malagueta pepper (Capsicum frutescens L.) assisted by ultrasound. Ultrason. Sonochem. 22, 78–88. doi: 10.1016/j.ultsonch.2014.05.001
Satyanarayana, M. N. (2006). Capsaicin and gastric ulcers. Crit. Rev. Food Sci. Nutr. 46, 275–328. doi: 10.1080/1040-830491379236
Sharma, S. K., Vij, A. S., and Sharma, M. (2013). Mechanisms and clinical uses of capsaicin. Eur. J. Pharmacol. 720, 55–62. doi: 10.1016/j.ejphar.2013.10.053
Shi, Y., Wang, Y., Shao, C., Huang, J., Gan, J., Huang, X., et al. (2020). COVID-19 infection: the perspectives on immune responses. Cell Death Differ. 27, 1451–1454. doi: 10.1038/s41418-020-0530-3
Sricharoen, P., Lamaiphan, N., Patthawaro, P., Limchoowong, N., Techawongstien, S., and Chanthai, S. (2017). Phytochemicals in Capsicum oleoresin from different varieties of hot chilli peppers with their antidiabetic and antioxidant activities due to some phenolic compounds. Ultrason. Sonochem. 38, 629–639. doi: 10.1016/j.ultsonch.2016.08.018
Stoica, R., Moscovici, M., Tomulescu, C., and Băbeanu, N. (2016). Extraction and analytical methods of capsaicinoids - a review. Sci. Bull. Ser. F. Biotechnol. 20, 93–98.
Sung, J., and Lee, J. (2016). Capsicoside G, a furostanol saponin from pepper (Capsicum annuum L.) seeds, suppresses adipogenesis through activation of AMP-activated protein kinase in 3T3-L1 cells. J. Funct. Foods 20, 148–158. doi: 10.1016/j.jff.2015.10.024
Szolcsányi, J., and Pintér, E. (2013). Transient receptor potential vanilloid 1 as a therapeutic target in analgesia. Expert Opin. Ther. Targets 17, 641–657. doi: 10.1517/14728222.2013.772580
Tanaka, Y., Sonoyama, T., Muraga, Y., Koeda, S., Goto, T., Yoshida, Y., et al. (2015). Multiple loss-of-function putative aminotransferase alleles contribute to low pungency and capsinoid biosynthesis in Capsicum chinense. Mol. Breed. 35:142. doi: 10.1007/s11032-015-0339-9
Tewksbury, J. J., Manchego, C., Haak, D. C., and Levey, D. J. (2006). Where did the chili get its spice? biogeography of capsaicinoid production in ancestral wild chili species. J. Chem. Ecol. 32, 547–564. doi: 10.1007/s10886-005-9017-4
Tsou, M.-F., Lu, H.-F., Chen, S.-C., Wu, L.-T., Chen, Y.-S., Kuo, H.-M., et al. (2006). Involvement of Bax, Bcl-2, Ca2+ and caspase-3 in capsaicin-induced apoptosis of human leukemia HL-60 cells. Anticancer Res. 26, 1965–1971.
Van Avesaat, M., Troost, F. J., Westerterp-Plantenga, M. S., Helyes, Z., Le Roux, C. W., Dekker, J., et al. (2016). Capsaicin-induced satiety is associated with gastrointestinal distress but not with the release of satiety hormones. Am. J. Clin. Nutr. 103, 305–313. doi: 10.3945/ajcn.115.123414
Van Gerven, L., Alpizar, Y. A., Wouters, M. M., Hox, V., Hauben, E., Jorissen, M., et al. (2014). Capsaicin treatment reduces nasal hyperreactivity and transient receptor potential cation channel subfamily V, receptor 1 (TRPV1) overexpression in patients with idiopathic rhinitis. J. Allergy Clin. Immunol. 133, 1332–1339. doi: 10.1016/j.jaci.2013.08.026
Van Rijswijk, J. B., Boeke, E. L., Keizer, J. M., Mulder, P. G. H., Blom, H. M., and Fokkens, W. J. (2003). Intranasal capsaicin reduces nasal hyperreactivity in idiopathic rhinitis: a double-blind randomized application regimen study. Allergy Eur. J. Allergy Clin. Immunol. 58, 754–761. doi: 10.1034/j.1398-9995.2003.00203.x
Venier, N. A., Yamamoto, T., Sugar, L. M., Adomat, H., Fleshner, N. E., Klotz, L. H., et al. (2015). Capsaicin reduces the metastatic burden in the transgenic adenocarcinoma of the mouse prostate model. Prostate 75, 1300–1311. doi: 10.1002/pros.23013
Walczak, J., Buszewski, B., Krakowska, A., and Rafińska, K. (2018). Enzyme-assisted optimized supercritical fluid extraction to improve Medicago sativa polyphenolics isolation. Ind. Crops Prod. 124, 931–940. doi: 10.1016/j.indcrop.2018.08.004
Wang, F., Zhao, J., Liu, D., Zhao, T., Lu, Z., Zhu, L., et al. (2016). Capsaicin reactivates hMOF in gastric cancer cells and induces cell growth inhibition. Cancer Biol. Ther. 17, 1117–1125. doi: 10.1080/15384047.2016.1235654
Wang, H. M., Chuang, S. M., Su, Y. C., Li, Y. H., and Chueh, P. J. (2011). Down-regulation of tumor-associated NADH oxidase, tNOX (ENOX2), enhances capsaicin-induced inhibition of gastric cancer cell growth. Cell Biochem. Biophys. 61, 355–366. doi: 10.1007/s12013-011-9218-0
Wang, Y., Liu, B., Wen, X., Li, M., Wang, K., and Ni, Y. (2017). Quality analysis and microencapsulation of chili seed oil by spray drying with starch sodium octenylsuccinate and maltodextrin. Powder Technol. 312, 294–298. doi: 10.1016/j.powtec.2017.02.060
Wang, Y., Tang, C., Tang, Y., Yin, H., and Liu, X. (2020). Capsaicin has an anti-obesity effect through alterations in gut microbiota populations and short-chain fatty acid concentrations. Food Nutr. Res. 64, 3525–3539. doi: 10.29219/fnr.v64.3525
Weitz, J. R., Makhmutova, M., Almaça, J., Stertmann, J., Aamodt, K., Brissova, M., et al. (2018). Mouse pancreatic islet macrophages use locally released ATP to monitor beta cell activity. Diabetologia 61, 182–192. doi: 10.1007/s00125-017-4416-y
Xu, S., Zhang, L., Cheng, X., Yu, H., Bao, J., and Lu, R. (2018). Capsaicin inhibits the metastasis of human papillary thyroid carcinoma BCPAP cells through the modulation of the TRPV1 channel. Food Func. 9, 344–354. doi: 10.1039/c7fo01295k
Xu, W., Liu, J., Ma, D., Yuan, G., Lu, Y., and Yang, Y. (2017). Capsaicin reduces Alzheimer-associated tau changes in the hippocampus of type 2 diabetes rats. PLoS ONE 12:e0172477. doi: 10.1371/journal.pone.0172477
Yang, F., Xiao, X., Cheng, W., Yang, W., Yu, P., Song, Z., et al. (2015). Structural mechanism underlying capsaicin binding and activation of the TRPV1 ion channel. Nat. Chem. Biol. 11, 518–524. doi: 10.1038/nchembio.1835
Yang, F., and Zheng, J. (2017). Understand spiciness: mechanism of TRPV1 channel activation by capsaicin. Protein Cell 8, 169–177. doi: 10.1007/s13238-016-0353-7
Yang, X. Y., and Du, G. H. (2018). “Capsaicin,” in Natural Small Molecule Drugs from Plants, ed G. H. Du (Singapore: Springer), 397–402.
Yin, N., Xu, J., Ginhoux, F., Randolph, G. J., Merad, M., Ding, Y., et al. (2012). Functional specialization of islet dendritic cell subsets. J. Immunol. 188, 4921–4930. doi: 10.4049/jimmunol.1103725
Yuan, L. J., Qin, Y., Wang, L., Zeng, Y., Chang, H., Wang, J., et al. (2016). Capsaicin-containing chili improved postprandial hyperglycemia, hyperinsulinemia, and fasting lipid disorders in women with gestational diabetes mellitus and lowered the incidence of large-for-gestational-age newborns. Clin. Nutr. 35, 388–393. doi: 10.1016/j.clnu.2015.02.011
Zhang, W., Zhao, Y., Zhang, F., Wang, Q., Li, T., Liu, Z., et al. (2020). The use of anti-inflammatory drugs in the treatment of people with severe coronavirus disease 2019 (COVID-19): the experience of clinical immunologists from China. Clin. Immunol. 214:108393. doi: 10.1016/j.clim.2020.108393
Zheng, L., Chen, J., Ma, Z., Liu, W., Yang, F., Yang, Z., et al. (2016). Capsaicin enhances anti-proliferation efficacy of pirarubicin via activating TRPV1 and inhibiting PCNA nuclear translocation in 5637 cells. Mol. Med. Rep. 13, 881–887. doi: 10.3892/mmr.2015.4623
Keywords: capsaicinoids, capsaicin, TRPV1 receptor, immune system diseases, waste
Citation: Cortés-Ferré HE, Guajardo-Flores D, Romero-De La Vega G and Gutierrez-Uribe JA (2021) Recovery of Capsaicinoids and Other Phytochemicals Involved With TRPV-1 Receptor to Re-valorize Chili Pepper Waste and Produce Nutraceuticals. Front. Sustain. Food Syst. 4:588534. doi: 10.3389/fsufs.2020.588534
Received: 29 July 2020; Accepted: 15 December 2020;
Published: 18 January 2021.
Edited by:
Andrea Gomez-Zavaglia, National University of La Plata, ArgentinaReviewed by:
Guadalupe Virginia Nevárez-Moorillón, Autonomous University of Chihuahua, MexicoAzila Abdul-Aziz, University of Technology Malaysia, Malaysia
Copyright © 2021 Cortés-Ferré, Guajardo-Flores, Romero-De La Vega and Gutierrez-Uribe. This is an open-access article distributed under the terms of the Creative Commons Attribution License (CC BY). The use, distribution or reproduction in other forums is permitted, provided the original author(s) and the copyright owner(s) are credited and that the original publication in this journal is cited, in accordance with accepted academic practice. No use, distribution or reproduction is permitted which does not comply with these terms.
*Correspondence: Janet Alejandra Gutierrez-Uribe, amFndSYjeDAwMDQwO3RlYy5teA==