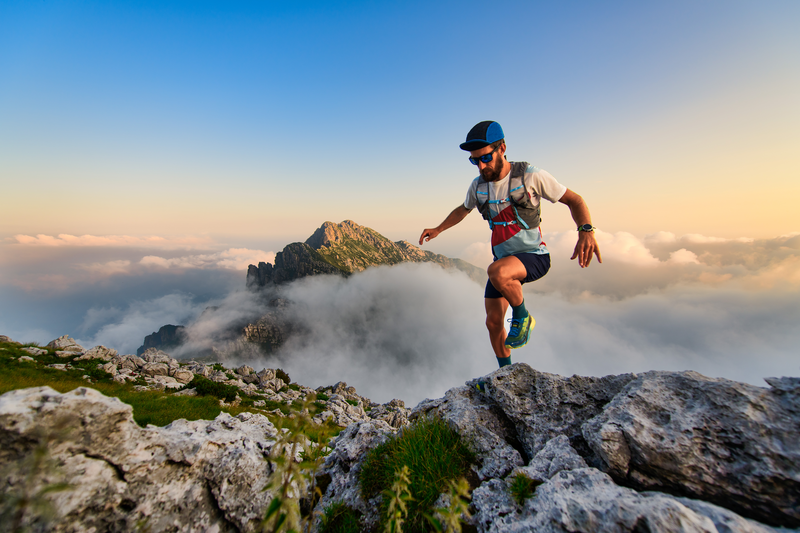
94% of researchers rate our articles as excellent or good
Learn more about the work of our research integrity team to safeguard the quality of each article we publish.
Find out more
REVIEW article
Front. Sustain. Food Syst. , 06 October 2020
Sec. Agroecology and Ecosystem Services
Volume 4 - 2020 | https://doi.org/10.3389/fsufs.2020.576606
This article is part of the Research Topic Adaptive Farming Sustainability Practices: Fundamentals to Advances View all 5 articles
Soil degradation poses a major challenge to agricultural systems in sub-Saharan Africa. Whereas there is a wealth of knowledge on the consequences of degraded soils, the evidence base for farm management practices that could prevent and mitigate soil degradation is still emerging and farmer uptake of such practices remains mostly low. We propose that low adoption rates are due, in part, to a lack of near-term, farm-level incentives, specifically immediate financial benefits accruing to farmers who implement sustainable management practices associated with enhanced soil health. To encourage adoption of soil-enhancing production practices by African smallholder farmers, a focus on farm-level return on investment (ROI) is essential. To be effective at scale, soil health initiatives must be built on realistic predictions of context-specific benefits, adoption barriers, and farm-level financial incentives. In this paper, we postulate that monetizable benefits of soil-enhancing practices accrue from increased agricultural production, reduced cost of production, greater resilience and environmental benefits. We review value chain, policy, and finance strategies that can shift the structural incentives that powerfully influence farm-level ROI and smallholder adoption of soil-enhancing practices. We conclude that agricultural development initiatives should integrate evidence-based estimation of soil-related biophysical changes and thorough analysis of monetizable benefits of promoted interventions, both on-farm and off-farm. This should be built on robust regional datasets and models, developed over several consecutive seasons and considering local specificities.
Soil health can be defined as the continued capacity of soils to function as a vital living system to sustain biological productivity and maintain the quality of air and water (Doran and Safley, 1997). As such, healthy soils are a basis for many environmental services to humanity, including agricultural production, water recharge, and climate regulation (Rojas et al., 2016). However, historical and current land use practices continue to degrade the structural integrity and fertility of soils across sub-Saharan Africa. As a consequence, most cropland is characterized by poor soil health, threatening the productivity and resilience of agricultural systems that depend on them (Vanlauwe et al., 2014). Particularly for smallholder farmers, this results in production risks which will likely aggravate in the context of climate change as poor soils tend to retain less water and are more prone to erosion (Morton, 2007; IPCC, 2019).
Soil health improvement is a pressing need for farmers, ecosystems, food systems, and humanity and it is not surprising that soil health is a policy priority in many parts of the world (Rockström et al., 2017). Yet, farm management decisions are often guided by structural incentives that encourage extractive production and reduce long-term soil health (Vermeulen et al., 2019).
Soil health can be maintained and enhanced when improved soil management practices are widely adopted by the individuals who manage agricultural land. Such practices take many forms including soil inputs, crop combinations, variety selection, reduced tillage, ridging, residue utilization, and integrated weed and pest management. In a global context, the technical potential of improved soil management appears to be significant for agricultural productivity, climate change mitigation, and other sustainability objectives (Smith et al., 2007). Improved soil management practices are commonly incorporated into holistic concepts for sustainable farming and there are numerous regional- and global-scale projections of the potential benefits accruing from large-scale adoption of various agriculture practices, technologies, or combinations (Pretty et al., 2018; Searchinger et al., 2019).
In Africa however, the adoption of improved soil management practices is mostly limited to growers that farm at a larger and more commercial scale (Mutoko et al., 2014; Vanlauwe et al., 2014; Stringer et al., 2020). Despite promising initial reports on the uptake of such practices and concepts (Pretty et al., 2006), more recent data suggests that aggregated adoption rates have been as low as 3–18% in areas and communities where they have been promoted (Nkonya et al., 2016; Stevenson and Vlek, 2018), indicating a significant gap between hopes and realities of farm-level adoption (Stevenson et al., 2019).
Beyond Africa, low adoption levels are observed in other parts of the world, in part because sustainable farming practices tend to deliver very heterogenous public and private benefits (Cui et al., 2018). Especially in the context of smallholder farming, these benefits are rarely properly quantified or, more importantly, monetized at the farm level to provide a reliable return on investment (ROI) for their adoption. Individual farmers will have distinct rationales and capacity for adopting single or multiple improved soil management practices, on some or all of their fields, and the timing and duration of adoption can be highly varied. Adopting new practices often requires farmers to outlay financial resources (e.g., to purchase improved inputs or machinery) and accept new risks (e.g., lower yield or market price than expected). For resource-constrained smallholder farmers, an immediate and reliable ROI, ideally building on a low initial investment base to reduce financial risks, is vital.
When soil-enhancing practices are promoted to them, farmers assess the potential benefits, factoring in their available resources (e.g., finances; labor; land) and competing options (e.g., current practice). Potential farm-level ROI is greatly influenced by farm-specific factors (e.g., farm size and location; soil type; household capacity and assets) as well as structural incentives (i.e., in value chains, government policies, and agricultural finance).
Large commercial farms in advanced economies have access to robust, spatially-resolved agronomic recommendations (i.e., precision agriculture) that rely on long-term public and private investment in rich datasets and regionally-calibrated models. They also benefit from reliable market information systems. Such capacity facilitates assessment of farm-level ROI for adoption of soil-enhancing practices (e.g., in the US, adoption of conservation agriculture was accelerated by quantified farm-level benefits from improved soil management). Neither long-term regional datasets and models, nor market information systems are commonly available to African smallholders, who have adopted soil-enhancing practices at quite low rates.
A large number of donor- and government-led initiatives promote adoption of a variety of practices designed to improve soil health and the overall environmental sustainability of smallholder farms. Without access to quantitative estimates of potential farm-level risks and benefits, such initiatives have not adequately integrated factors guiding smallholder adoption. We propose that agricultural development initiatives will more successfully select, promote, and scale soil-enhancing practices if they can be designed around evidence-based quantification of the biophysical benefits and financial incentives that underlie assessment of farm-level ROI.
In this paper, we propose strategies for the design and deployment of interventions that aim to sustainably scale the adoption of improved soil management practices in smallholder farming systems in sub-Saharan Africa. We review soil enhancement benefits that accrue at the farm level, in the African context, and survey barriers to smallholders' adoption of soil-enhancing practices. We propose necessary preconditions and development interventions for overcoming adoption barriers as well as metrics suitable to farm-level ROI assessment. We conclude by proposing value chain, policy, and finance strategies that can facilitate emergence of necessary preconditions that can shift farm-level ROI calculations toward adoption of soil-enhancing practices. Assessment of adoption benefits and barriers is essential to robustly quantifying potential financial incentives and to designing appropriate supportive interventions that foster immediate and sustainable value at farmer level.
The uptake of improved soil management practices depends on the benefits they deliver to individual farmers in the near-term as compared to the status quo, the barriers to adoption, and the incentives provided to overcome adoption barriers.
Resource-poor smallholder farmers require options that are relatively low-risk and provide short-term returns on investment. Environmental sustainability is often a less-immediate concern than the economic viability of adopted practices and technologies, so economic factors and monetizable benefits will be essential considerations for creating smallholder incentives to adopt soil-enhancing practices (Pannell et al., 2014).
Yet, low adoption rates indicate that such benefits are often not appropriately quantified and attributed nor are adoption barriers (e.g., cultural norms, access, logistics, labor, timing) well-characterized or understood. As a result, incentives for such practices are often inaccessible or insufficiently attractive to targeted individuals.
Globally, there is a solid evidence base for the benefits of many soil-enhancing practices. While such evidence is patchy in the African context (Stevenson and Vlek, 2018), Table 1 illustrates that there are studies demonstrating beneficial biophysical change for a range of practices.
Table 1. Selected evidence of biophysical changes and farm-level benefits from soil-enhancing practices.
Biophysical changes commonly include increased soil organic matter and biodiversity, improved plant-available water, better nutrient availability, and reduced weed, pest, and disease pressure. These biophysical changes can increase productivity (e.g., higher yield; extended growing seasons), reduce cost of production (e.g., more efficient fertilizer use; less need for crop protection products), and boost resilience (e.g., to drought) as well as delivering environmental benefits (e.g., reduced erosion; water recharge; carbon sequestration) accruing both on- and off-farm.
A number of practices to improve soil health have been developed and introduced to smallholder farming communities across sub-Saharan Africa. Amongst others, these include diversified cropping systems, crop rotations, continuous soil cover (e.g., cover crops; mulching), minimum tillage, and intercropping and agroforestry systems. Some of these practices have been integrated into holistic concepts for sustainable land management, such as conservation agriculture (Hobbs et al., 2007; Franke et al., 2018), integrated soil fertility management (ISFM) (Vanlauwe et al., 2014), and permanent agroforestry (Rahman et al., 2017; De Giusti et al., 2019).
In Africa, a history of relatively low research investment in soil health has resulted in a limited evidence base (e.g., low spatial resolution; gaps for many potentially beneficial practices) for soil biophysical changes and farm-level benefits (i.e., yield, production costs, resilience). This impedes quantification and ROI assessment of soil-enhancing practices for a specific farming context and can be improved through expanded research using a common set of metrics.
At the farm level, the potential benefits of soil-enhancing practices are weighed against the known benefits and risks of current farm practice. To abandon the low uncertainty of adhering to the status quo, the high uncertainty of new practice adoption must be accompanied by sufficiently large, near-term benefits and, ideally, some form of risk mitigation.
To adopt new practices, smallholders need low-risk, near-term ROI. However, the magnitude and timeframe for biophysical changes that arise from soil-enhancing practices is not yet well-quantified (e.g., soil organic matter accumulation). Additionally, some portion of the environmental benefits arising from farm-level biophysical changes may accrue beyond the farm (e.g., climate change mitigation; reduced erosion). Smallholders with insecure land tenure lack guarantees that they will derive soil-related benefits beyond the current growing season.
Given high variability among farming operations and contexts, site-specific factors will influence the attractiveness of new practices (Pannell et al., 2014; Vanlauwe et al., 2019) and limit the potential success of one-size-fits-all approaches (Palm et al., 2014). Yet, agronomic knowledge is commonly limited or incomplete among smallholders who require training in soil, water, and pest management, marketing and financing strategies, and more. Context specificity tends to go beyond local soil properties and predominant production systems to include potential resource use tradeoffs, socio-economic dimensions, local market linkages (both input and offtake), as well as the policy and extension environment.
Farm-level incentives are strongly affected by structural factors. National and sub-national agricultural policies such as subsidies (e.g., for commodity crops; for generic fertilizers), regulations (e.g., seed registration), tariffs, and taxes tip the scales toward certain types of crops (e.g., cereals) and farm management practices (e.g., monocropping). Similarly, local value chains fundamentally control the type and timing of farm products that receive adequate remuneration to generate farm-level profits. Taken together, the complexity of farm-level and structural factors may work against smallholders opting for new practices over the status quo.
While emerging data substantiates potential benefits from the adoption of more sustainable production practices (see Table 1), the current evidence base may not be sufficient to establish a robust business case at farmer level to justify adoption. Many promoted practices and technologies require investment, yet most smallholder farms have limited financial assets, or access to affordable credit, for purchasing improved seeds, nursery stock, equipment (e.g., irrigation; storage; machinery), and other investments necessary to implement or derive benefit from new practices.
Promoted practices also commonly involve resource use tradeoffs (Klapwijk et al., 2014; Kanter et al., 2016). Such tradeoffs are often acute for land (e.g., to produce for markets or household consumption), organic matter (e.g., to use for fodder, fuel, or SOM enrichment), labor (e.g., for crops, livestock, or off-farm employment), and inputs (e.g., which crops to receive limited irrigation water or expensive fertilizers and crop protection products). For instance, rotation crops may improve yields and reduce production costs of successive cash crops. Yet, if they have limited market value, adoption of rotation crops could actually reduce farm-level income in the season in which they are grown.
Farm-level adoption barriers are illustrated by conservation agriculture (the combination of minimum soil disturbance, crop rotations and continuous soil cover), which has seen a substantial expansion in many parts of the world yet is only sparingly practiced in sub-Saharan Africa (Giller et al., 2009). This can partially be attributed to (i) low incremental productivity of smallholder farming systems; (ii) competing interests for crop residues and organic matter (for feed and fuel); (iii) increased labor requirements (e.g., for weed control); (iv) lack of offtake markets for rotation crops; and (v) lack of immediate financial benefits, as productivity gains only materialize over years (Palm et al., 2014; Pannell et al., 2014; Rigolot et al., 2017). Similar patterns are seen for other agricultural innovations, such as Integrated Soil Fertility Management, which relies on organic inputs and synthetic fertilizer that may not always be readily available or affordable (Vanlauwe et al., 2010). There is surprisingly little information available on such context-specific factors at the local and farm level.
Monetization of benefits is a key step to assessing whether adoption of more sustainable practices will deliver an appropriate, near-term ROI at the farm level. Marketing of farm products, reduced production costs, subsidies, direct payments, and insurance can all contribute to net farm income (see Table 2). A combination of such incentives can help overcome barriers and increase farmer implementation of improved soil management practices.
Table 2. Potential interventions to shift toward necessary preconditions for adoption of soil-enhancing practices.
When soil-enhancing practices deliver significantly increased yield or reduced costs in the same growing season, there is an inherent incentive for adoption and only facilitative interventions may be needed (e.g., capacity building; access to inputs and markets). For example, where high-quality seeds and remunerative markets are made available, integration of a legume rotation might rapidly improve nitrogen availability and break pest or disease cycles resulting in higher or less variable yields.
However, accrual of agronomic benefits may occur over extended periods of time or, in the case of increased resilience, may only become apparent during an extreme event. Delayed agronomic benefits will not sufficiently incentivize smallholders to adopt new farming practices without additional interventions that offset new costs (including foregone alternative uses of land, labor, and resources) and risks during the period of delay. For example, leaving crop residue in the field, rather than using it for fuel or fodder, requires provision of an alternative (e.g., efficient cookstove; fodder trees) until increased soil organic matter delivers greater productivity (e.g., via improved water and nutrient efficiency).
When the environmental benefits of soil-enhancing practices primarily accrue beyond the farm, smallholder adoption may require monetary incentives as either a temporary or permanent subsidy (e.g., carbon offset payments; public support programs). Adherence to specified sustainable production practices and accrual of environmental benefits can lead to increased farmer income through access to premium offtake markets (e.g., via certification schemes), specialized finance, subsidies, or payments for ecosystem services (PES).
Current value chain, policy, and financial systems rarely provide sufficient incentives for smallholders to adopt improved soil management practices (Vermeulen et al., 2019), and in many cases, supportive interventions are necessary. Many agricultural development initiatives promote soil-enhancing practices among smallholders in sub-Saharan Africa, but may not take advantage of the full array of farm-level, value chain, policy, and finance interventions that can facilitate accrual of farm-level benefits and shift the farm-level ROI calculation toward adoption of soil-enhancing practices. In Table 2, we list and categorize preconditions for establishing a robust, farm-level business case for adoption improved soil management practices.
Adoption of new practices requires access to knowledge and equipment, to high-quality farm inputs, and to remunerative offtake markets that convert surplus or diversified farm production (e.g., rotation crops) into net increase in farm income (Vanlauwe et al., 2014). In addition, adoption of promoted practices is likely to be higher when these are complementary to existing farming systems and have moderate knowledge requirements and low adoption risks.
Offtake agreements can reduce farmers' risk in adopting new practices or crop types by guaranteeing access to markets or premium prices if farmers adhere to offtakers' requirements or certification standards. The absence of reliable, remunerative offtake markets in most parts of sub-Saharan Africa inhibits adoption of sustainable practices (AGRA, 2019). Yet market dimensions are often neglected in smallholder-focused initiatives intended to increase the adoption of improved soil management practices.
A key challenge will be to develop commercial models for not-yet-commercial activities, without distorting existing markets. Amongst others, the following strategies may be effective in meeting this challenge:
• Incentivize farm and market diversification: Create market demand (price signals; market infrastructure) for rotation crops or tree crops that overcome financial and other risks of diversification.
• Better value chain integration and service delivery through farmer aggregation mechanisms (e.g., “hubs” that facilitate input supply, financing, crop aggregation/marketing and provide timely, integrated support that helps farmers fully capitalize on soil health practices).
• Develop models that combine soil diagnostic services and related input supply: improving soil fertility, plant nutrition, and productivity through soil testing, agronomic advising, and improved access to soil amendments and quality seed/improved varieties.
While this paper does not review supportive policy strategies for farmer adoption of soil enhancing practices and technologies in detail, we note the tremendous influence of policy and institutional factors on farm-level options and decisions (Agrawal, 2008). Fundamental elements of an enabling policy environment include well-designed subsidy programs (Jayne et al., 2019), efficient crop variety registration, and land titling to ensure long-term interest in improved soils (WBCSD, 2018).
For instance, government- or philanthropy-supported subsidies can accelerate development of smallholder-inclusive markets such as for customized fertilizers, soil amendments, and rotation crop varieties that promote soil health. Subsidies can include government funding for market infrastructure (e.g., storage facilities; price information systems, credit registries) and technical assistance. Ideally, short-term catalytic subsidies will focus on long-term, strategic market-building activities (McCann et al., 2018).
There is an expanding set of traditional and innovative financial strategies being adapted to smallholder production systems with objectives ranging from financial inclusion to incentivizing farm-level contributions to climate change mitigation. The feasibility of some newer approaches has not yet been demonstrated for environmental objectives in smallholder production.
If appropriately structured, loans, insurance, or credit schemes that bridge the gap to immediate ROI could incentivize smallholder farmers to adopt soil-enhancing practices. Smallholders could be offered various forms of value chain finance such as pre-financing, receivables financing, and insurance (Havemann, 2016). Farm credit targeted to smallholders commonly necessitates innovative risk-screening and collateral requirements that enable favorable interest rates in the absence of credit history or assets.
Developing appropriate financing strategies, financial structures, and specific arrangements requires well-conceived soil health related interventions since all their success relies on delivery of actual agronomic (e.g., increased yield) and/or environmental (e.g., carbon, water) benefits. Debt-based financing strategies can increase farm-level risk if not paired with mechanisms for increasing farmers' ability to repay loans. Accordingly, debt instruments should be pursued after (or in parallel) with implementation of strategies that increase farmers' revenue (e.g., increased market prices; PES) or reduce their net costs (e.g., better access to affordable, high-quality seeds).
Globally, numerous carbon credit schemes and other types of PES, designed to enhance the farm-level ROI of improved practice adoption, have been tested (Henderson et al., 2018). PES is fundamentally dependent on ‘buyers' willing to pay for environmental performance (e.g., development finance institutions; GHG-emitting businesses; water supply agencies) and are reliant on development of low-cost monitoring and transaction mechanisms. Where funds are available, direct monetary support can incentivize farmers to enter contracts (usually through an aggregating entity) to adopt specified practices for a given period of time (e.g., per hectare payments) or to be paid for environmental outcomes (e.g., per ton of carbon sequestered).
The variety and complexity of methodologies applied, the high costs of farm-level verification of environmental performance (e.g., water quality; carbon stocks) have produced significant headwinds for PES approaches. With uneven evidence for actual soil carbon sequestration and accrual of benefits from new practices (Palm et al., 2014; Vermeulen et al., 2019; World Bank, 2020) and weak agreement about permanence and eligibility for compliance schemes, prices for agricultural carbon credits have been low and volatile.
Very few smallholders in sub-Saharan Africa have access to monetary incentives for improved environmental performance (e.g., carbon sequestration/GHG emission reduction; water use efficiency; watershed protection) such as lower cost of farm credit or PES schemes. Given the fragmented nature of smallholder farming systems, the long-term viability and scalability of PES initiatives will depend on reliable metrics and low verification and transaction costs (Salzman et al., 2018). More cost-efficient models for field verification of environmental performance (e.g., remote sensing, digital solutions for self-verification) are being tested (Eitzinger et al., 2019).
In the short-term, finance needed to test interventions and to establish new arrangements is likely to come from a mix of public, philanthropic, and private sources. This could take the form of blended finance in which structured vehicles combined diverse sources of finance for specific purposes under defined terms (e.g., risk allocation) or simply mobilization of different sources of finance toward different interventions, ideally in a coordinated manner. In the longer-term, finance needed for continuation of new value chain activities is likely to come primarily from private sources (e.g., commercial banks, value chain companies) or public lending programs.
Investment in improved soil health can only create value at farm level when promoted agronomic practices, either individually or combined as a package, lead to increased short-term, farm-level profitability (Henderson et al., 2018; Vermeulen et al., 2019). In some cases, benefits from the adoption of soil-enhancing practices, such as increased yields and resilience, and reduced costs of production, can translate directly into near-term ROI, if essential knowledge, equipment, and inputs are available. In most cases, changes within value chains (e.g., provision of inputs; reliable, remunerative markets), government policies (e.g., appropriate farm subsidies; legal frameworks for PES), and agricultural finance mechanisms (e.g., concessionary credit) will be needed for farm-level incentives to materialize.
Like many aspects of sustainable agriculture, there are (cost-effectiveness) tradeoffs for agricultural soil health enhancement in Africa (Westermann et al., 2018). To overcome barriers to adoption of soil-enhancing practices, agricultural development initiatives should combine and sequence appropriate value chain, policy, and finance interventions, adapted to specific local farming contexts. Design of agricultural development initiatives should also recognize that some smallholder farmers do not yet have sufficient farm-level capacity (e.g., knowledge; labor; risk tolerance) and assets (e.g., finances; land; equipment) required to adopt soil-enhancing practices. These pre-commercial smallholders will need greater public support to build the necessary assets and to endure the attendant risks.
While the evidence base for quantifying benefits, costs, and risks of adopting sustainable agronomic practices is not yet complete, it can inform sustainable agriculture interventions. To achieve broad and sustained smallholder adoption, soil-enhancing practices must be compatible with agroecological conditions, agronomic variables, regional supply chains, and policy context (Akpoti et al., 2019). To effectively promote adoption, soil health initiatives will robustly consider whether potential practices are compatible with these determinative contextual factors.
Design of agricultural development initiatives should be based on estimates of reliable benefit accrual for targeted smallholder groups, and should be organized around flexible, replicable delivery models that appropriately integrate and sequence key supportive functions including:
In-region farmer participatory testing to build an evidence base for ROI quantification, to ensure context-specificity, to validate improved agriculture practices and technologies, and to screen them for viability within existing value chains and policies (e.g., led by national and international research institutes). While it is tempting to ‘import' estimates developed in the U.S., Europe, and other developed economies with better-studied agriculture sectors, there are real risks that imported economic estimates will be over-optimistic and poorly aligned with the specific biophysical, socio-cultural, and market conditions relevant to African smallholders (Pannell et al., 2014). A rigorous, farmer-engaged “learning-while-doing” approach can reveal meaningful heterogeneity among smallholder farmers and generate a portfolio of evidence-based interventions designed to increase profitability and resilience through improved soil health.
Demand-led R&D networks. Meaningful progress toward soil health can be made within existing value chain, policy, and finance contexts. Demand-led, network-based capacity for R&D and technology transfer can support linked interventions across value chains and co-investment partnerships (e.g., with offtakers, insurance companies, input suppliers, traders, financial institutions). The CGIAR and their national partners have built an impressive body of soil-related research over recent decades. Ongoing and expanded investment is needed to develop quantification and predictive capacity for the relationship among investments in smallholder capacity building, improvements in soil management and soil quality, and changes in risk and resilience at multiple scales (e.g., farm, community, value chain). Paired R&D and scaling initiatives can test and validate farm management options in close partnership with organizations who support farmer capacity building.
Supportive interventions that build access to agronomic knowledge, farm inputs, markets, and finance. Smallholder farmers need a “toolbox” of options to navigate their specific conditions and tradeoffs, which may change dynamically (e.g., through shifts in climate, policies, or markets). Holistic approaches that recognize the effects of structural incentives on farm-level decisions can pair technical knowledge with value chain, policy, and financial interventions.
Appropriate finance strategies that offer appropriate combinations of loans, insurance, or credit that bridge the gap to immediate ROI (e.g., led by governments, DFI, and impact investors) (Viable soil health initiatives would not be dependent on long-term donor funding). “Right-sized” finance will: (i) Overcome farm-level barriers to adoption of sustainable practices (e.g., opportunity costs of alternative uses for land, organic matter, labor; new production costs); (ii) Align with farmers' needs/capacities and target net reduction of farm-level risk; (iii) Be anchored in technical feasibility and risk-adjusted financial return; (iv) Be delivered through appropriate mechanisms (e.g., farmer aggregation strategies); and (v) Be paired with necessary policy adjustments (e.g., secure land use titling) infrastructure investments (e.g., seed access, soil testing, product storage).
Essential metrics to assess the effectiveness and sustained impact of soil-related, smallholder-focused interventions. A wide array of soil-related biophysical metrics can be monitored from farm- to landscape-level and combined with socio-economic, policy, and finance metrics to understand the effectiveness and (direct or indirect) impact of smallholder-focused interventions (see Table 3). Estimation of farm-level environmental and economic benefits of soil health improvement strategies should be built on in-region data, acquired over several consecutive seasons following clear impact assessment criteria (Stevenson et al., 2019).
We encourage global donors, agricultural development program implementers, and public sector partners to focus on developing holistic models that provide long-term, sustained impact, potentially at the cost of short-term impact measures. To facilitate adoption of improved soil management practices by African smallholder farmers, such initiatives should incorporate a thorough analysis of both anticipated benefits and beneficiaries of promoted interventions. By promoting change in value chains, government policy, and agricultural finance mechanisms, structural incentives can shift encouraging smallholder farmers to adopt new practices and generate productivity, income, and resilience benefits at scale.
All authors listed have made a substantial, direct and intellectual contribution to the work, and approved it for publication.
The authors declare that the research was conducted in the absence of any commercial or financial relationships that could be construed as a potential conflict of interest.
We would like to thank Samuel Steiner for the support in literature research and Paul Castle for language edits. Furthermore, we would like to thank Cheryl Palm and Bernard Vanlauwe for providing critical inputs to this work.
AGRA (2019). Africa Agriculture Status Report: The Hidden Middle: A Quiet Revolution in the Private Sector Driving Agricultural Transformation. 7. Available online at: https://agra.org/wp-content/uploads/2019/09/AASR2019-The-Hidden-Middleweb.pdf
Agrawal, A. (2008). The Role of Local Institutions in Adaptation to Climate Change. Washington, DC: World Bank. doi: 10.1596/28274
Akpoti, K., Kabo-bah, A. T., and Zwart, S. J. (2019). Review - Agricultural land suitability analysis: State-of-the-art and outlooks for integration of climate change analysis. Agric. Syst. 173, 172–208. doi: 10.1016/j.agsy.2019.02.013
Aune, J. B., Coulibaly, A., and Giller, K. E. (2017). Precision farming for increased land and labour productivity in semi-arid West Africa. a review. Agron Sustain. Dev. 37:16. doi: 10.1007/s13593-017-0424-z
Bàrberi, P. (2019). “Chapter Four - Ecological weed management in Sub-Saharan Africa: Prospects and implications on other agroecosystem services,” in Advances in Agronomy, ed D. L. Sparks (Academic Press). 156, 219–264. doi: 10.1016/bs.agron.2019.01.009
Bayala, J. (2012). Cereal yield response to conservation agriculture practices in drylands of West Africa: a quantitative synthesis. J. Arid. Environ. v. 78: 2078. doi: 10.1016/j.jaridenv.2011.10.011
Belete, S., Bezabih, M., Abdulkadir, B., Tolera, A., Mekonnen, K., and Wolde-Meskel, E. (2019). Inoculation and phosphorus fertilizer improve food-feed traits of grain legumes in mixed crop-livestock systems of Ethiopia. Agric. Ecosyst. Environ. 279, 58–64. doi: 10.1016/j.agee.2019.04.014
Bielders, C. L., and Gérard, B. (2015). Millet response to microdose fertilization in south–western Niger: Effect of antecedent fertility management and environmental factors. Field Crops Res. 171, 165–175. doi: 10.1016/j.fcr.2014.10.008
Blanchart, E., Villenave, C., Viallatoux, A., Barthès, B., Girardin, C., Azontonde, A., et al. (2006). Long-term effect of a legume cover crop (Mucuna pruriens var. utilis) on the communities of soil macrofauna and nematofauna, under maize cultivation, in southern Benin. Eur J. Soil Biol. 42, S136–S144. doi: 10.1016/j.ejsobi.2006.07.018
Bright, M. B. H., Diedhiou, I., Bayala, R., Assigbetse, K., Chapuis-Lardy, L., Ndour, Y., et al. (2017). Long-term Piliostigma reticulatum intercropping in the Sahel: Crop productivity, carbon sequestration, nutrient cycling, and soil quality. Agric. Ecosyst. Environ. 242, 9–22. doi: 10.1016/j.agee.2017.03.007
Corbeels, M., Cardinael, R., Naudin, K., Guibert, H., and Torquebiau, E. (2019). The 4 per 1000 goal and soil carbon storage under agroforestry and conservation agriculture systems in sub-Saharan Africa. Soil Tillage Res. 188, 16–26. doi: 10.1016/j.still.2018.02.015
Cui, Z., Zhang, H., Chen, X., Zhang, C., Ma, W., Huang, C., et al. (2018). Pursuing sustainable productivity with millions of smallholder farmers. Nature 555, 363–6. doi: 10.1038/nature25785
Dahlin, A. S., and Rusinamhodzi, L. (2019). Yield and labor relations of sustainable intensification options for smallholder farmers in sub-Saharan Africa. A meta-analysis. Agron. Sustain. Develop. 39:32. doi: 10.1007/s13593-019-0575-1
De Giusti, G., Kristjanson, P., and Rufino, M. C. (2019). Agroforestry as a climate change mitigation practice in smallholder farming: evidence from Kenya. Clim. Change 153, 379–394. doi: 10.1007/s10584-019-02390-0
Doran, J. W., and Safley, M. (1997). Defining and assessing soil health and sustainabile productivity. Washington, DC: USDA.
Eitzinger, A., Cock, J., Atzmanstorfer, K., Binder, C. R., Läderach, P., Bonilla-Findji, O., et al. (2019). Geofarmer: a monitoring and feedback system for agricultural development projects. Comput. Electr. Agricult. 158, 109–121. doi: 10.1016/j.compag.2019.01.049
Franke, A. C., van den Brand, G. J., Vanlauwe, B., and Giller, K. E. (2018). Sustainable intensification through rotations with grain legumes in Sub-Saharan Africa: a review. Agric. Ecosyst. Environ. 261, 172–185. doi: 10.1016/j.agee.2017.09.029
Gbèhounou, G., and Adango, E. (2003). Trap crops of Striga hermonthica: in vitro identification and effectiveness in situ. Crop Protection 22, 395–404. doi: 10.1016/S0261-2194(02)00196-5
Giller, K. E., Witter, E., Corbeels, M., and Tittonell, P. (2009). Conservation agriculture and smallholder farming in Africa: the heretics' view. Field Crops Res. 114, 23–34. doi: 10.1016/j.fcr.2009.06.017
Hassanali, A., Herren, H., Khan, Z. R., Pickett, J. A., and Woodcock, C. M. (2008). Integrated pest management: the push-pull approach for controlling insect pests and weeds of cereals, and its potential for other agricultural systems including animal husbandry. Philos. Trans. R. Soc. Lond. B Biol. Sci. 363, 611–621. doi: 10.1098/rstb.2007.2173
Havemann, T. (2016). Value Chain Finance for Agricultural Climate Change Resilience. Wageningen: Technical Centre for Agricultural and Rural Cooperation.
Henderson, B., Cacho, O., Thornton, P., van Wijk, M., and Herrero, M. (2018). The economic potential of residue management and fertilizer use to address climate change impacts on mixed smallholder farmers in Burkina Faso. Agric. Syst. 167, 195–205. doi: 10.1016/j.agsy.2018.09.012
Hobbs, P. R., Sayre, K., and Gupta, R. (2007). The role of conservation agriculture in sustainable agriculture. Phil. Trans. R. Soc. B 363, 543–555. doi: 10.1098/rstb.2007.2169
Iorkua, S. A. (2012). The effects of mulch cover on splash erosion in makurdi town. Glob. J. Pure Appl. Sci. 18, 163–167. doi: 10.4314/gjpas.v18i3-4.7
IPCC (2019). Climate Change and Land: An IPCC Special Report on Climate Change, Desertification, Land Degradation, Sustainable Land Management, Food Security, and Greenhouse Gas Fluxes in Terrestrial Ecosystems, eds P. R. Shukla, J. Skea, E. Calvo Buendia, V. Masson-Delmotte, H.-O. Pörtner, D. C. Roberts, P. Zhai, R. Slade, S. Connors, R. van Diemen, M. Ferrat, E. Haughey, S. Luz, S. Neogi, M. Pathak, J. Petzold, J. Portugal Pereira, P. Vyas, E. Huntley, K. Kissick, M. Belkacemi, and J. Malley (In Press). Available online at: https://www.sciencedirect.com/science/article/pii/S0065211319300331
Jayne, T. S., Snapp, S., Place, F., and Sitko, N. (2019). Sustainable agricultural intensification in an era of rural transformation in Africa. Glob. Food Sec. 20, 105–113. doi: 10.1016/j.gfs.2019.01.008
Jemai, I., Ben Aissa, N., Ben Guirat, S., Ben-Hammouda, M., and Gallali, T. (2013). Impact of three and seven years of no-tillage on the soil water storage, in the plant root zone, under a dry subhumid Tunisian climate. Soil Tillage Res. 126, 26–33. doi: 10.1016/j.still.2012.07.008
Kanter, D. R., Schwoob, M.-H., Baethgen, W. E., Bervejillo, J. E., Carriquiry, M., Dobermann, A., et al. (2016). Translating the sustainable development goals into action: a participatory backcasting approach for developing national agricultural transformation pathways. Glob Food Sec. 10, 71–79. doi: 10.1016/j.gfs.2016.08.002
Kätterer, T., Roobroeck, D., Andrén, O., Kimutai, G., Karltun, E., Kirchmann, H., et al. (2019). Biochar addition persistently increased soil fertility and yields in maize-soybean rotations over 10 years in sub-humid regions of Kenya. Field Crops Res. 235, 18–26. doi: 10.1016/j.fcr.2019.02.015
Khan, Z. R., Pickett, J. A., Wadhams, L. J., Hassanali, A., and Midega, C. A. O. (2006). Combined control of striga hermonthica and stemborers by maize–desmodium spp. intercrops. Crop Protection 25, 989–995. doi: 10.1016/j.cropro.2006.01.008
Klapwijk, C. J., van Wijk, M. T., Rosenstock, T. S., van Asten, P. J. A., Thornton, P. K., and Giller, K. E. (2014). Analysis of trade-offs in agricultural systems: current status and way forward. Curr. Opin. Environ. Sustain. 6, 110–115. doi: 10.1016/j.cosust.2013.11.012
Kouyaté, Z., Franzluebbers, K., Juo, A. S. R., and Hossner, L. R. (2000). Tillage, crop residue, legume rotation, and green manure effects on sorghum and millet yields in the semiarid tropics of Mali. Plant Soil 225, 141–151. doi: 10.1023/A:1026589528352
Lal, R., Wilson, G. F., and Okigbo, B. N. (1978). No-till farming after various grasses and leguminous cover crops in tropical alfisol. I. Crop performance. Field Crops Res. 1, 71–84. doi: 10.1016/0378-4290(78)90008-4
McCann, M., Mehta, R., Shakhovskoy, M., Zook, D., and Carroll, T. (2018). Getting Smarter on Subsidy: The Role of Grant Funding in Smallholder Finance. Washington DC, ISF Advisors.
Morton, J. F. (2007). The impact of climate change on smallholder and subsistence. Agriculture 104, 19680–19685. doi: 10.1073/pnas.0701855104
Mupangwa, W., Thierfelder, C., and Ngwira, T. C. (2016). Fertilization strategies in conservation agriculture systems with maize–legume cover crop rotations in southern africa. Exp Agric. 53, 288–307. doi: 10.1017/S0014479716000387
Mutoko, M. C., Shisanya, C. A., and Hein, L. (2014). Fostering technological transition to sustainable land management through stakeholder collaboration in the western highlands of Kenya. Land Use Policy 41, 110–120. doi: 10.1016/j.landusepol.2014.05.005
Nkonya, E., Mirzabaev, A., and von Braun, J., (eds.). (2016). “Economics of land degradation and improvement: an introduction and overview,” in Economics of Land Degradation and Improvement - A Global Assessment for Sustainable Development (Cham: Springer). doi: 10.1007/978-3-319-19168-3_1
Nurudeen, A. R., Larbi, A., Kotu, B. H., Tetteh, F. M., and Hoeschle-Zeledon, I. (2018). Does nitrogen matter for legumes? Starter nitrogen effects on biological and economic benefits of cowpea (Vigna unguiculata L.) in guinea and sudan savanna of west Africa. Agronomy 8, 1–12. doi: 10.3390/agronomy8070120
Odhiambo, B. O., and Mihara, M. (2018). Effects of gypsum and rice husk biochar on surface discharge and nutrient loss from farmlands in Budalangi, Kenya. Int J. Environ. Rural Develop. 9, 94–100. Available online at: https://www.jstage.jst.go.jp/article/ijerd/9/1/9_94/_article
Palm, C., Blanco-Canqui, H., DeClerck, F., Gatere, L., and Grace, P. (2014). Conservation agriculture and ecosystem services: an overview. Agric. Ecosyst. Environ. 187, 87–105. doi: 10.1016/j.agee.2013.10.010
Pannell, D. J., Llewellyn, R. S., and Corbeels, M. (2014). The farm-level economics of conservation agriculture for resource-poor farmers. Agric. Ecosyst. Environ. 187, 52–64. doi: 10.1016/j.agee.2013.10.014
Paterson, R. T., Karanja, G. M., Nyaata, O. Z., Kariuki, I. W., and Roothaert, R. L. (1998). A review of tree fodder production and utilization within smallholder agroforestry systems in Kenya. Agrofor. Syst. 41, 181–199. doi: 10.1023/A:1006066128640
Pretty, J., Benton, T. G., Bharucha, Z. P., Dicks, L. V., Flora, C. B., Godfray, H. C. J., et al. (2018). Global assessment of agricultural system redesign for sustainable intensification. Nat. Sustain. 1, 441–446. doi: 10.1038/s41893-018-0114-0
Pretty, J. N., Noble, A. D., Bossio, D., Dixon, J., Hine, R. E., Penning de Vries, F. W. T., et al. (2006). Resource-conserving agriculture increases yields in developing countries. Environ. Sci. Technol. 40, 1114–1119. doi: 10.1021/es051670d
Rahman, S. A., Jacobsen, J. B., Healey, J. R., Roshetko, J. M., and Sunderland, T. (2017). Finding alternatives to swidden agriculture: does agroforestry improve livelihood options and reduce pressure on existing forest?. Agrof. Syst. 91, 185–199. doi: 10.1007/s10457-016-9912-4
Rigolot, C., de Voil, P., Douxchamps, S., Prestwidge, D., Van Wijk, M., Thornton, P. K., et al. (2017). Interactions between intervention packages, climatic risk, climate change and food security in mixed crop–livestock systems in Burkina Faso. Agric. Syst. 151, 217–224. doi: 10.1016/j.agsy.2015.12.017
Rockström, J., Williams, J., Daily, G., Noble, A., Matthews, N., Gordon, L., et al. (2017). Sustainable intensification of agriculture for human prosperity and global sustainability. Ambio 46, 4–17. doi: 10.1007/s13280-016-0793-6
Rojas, R. V., Achouri, M., Maroulis, J., and Caon, L. (2016). Healthy soils: a prerequisite for sustainable food security. Environ. Earth Sci. 75:180. doi: 10.1007/s12665-015-5099-7
Rosenstock, T. S., Tully, K. L., Arias-Navarro, C., Neufeldt, H., Butterbach-Bahl, K., and Verchot, L. V. (2014). Agroforestry with N2-fixing trees: sustainable development's friend or foe?” Curr. Opin. Environ. Sustain. 6, 15–21. doi: 10.1016/j.cosust.2013.09.001
Salzman, J., Bennett, G., Carroll, N., Goldstein, A., and Jenkins, M. (2018). The global status and trends of payments for ecosystem services. Nature Sustanab. 1, 136–144. doi: 10.1038/s41893-018-0033-0
Schuler, J., Voss, A. K., Ndah, H. T., Traore, K., and de Graaff, J. (2016). A socioeconomic analysis of the zaï farming practice in northern burkina faso. Agroecol. Sustain. Food Syst. 40, 988–1007. doi: 10.1080/21683565.2016.1221018
Searchinger, T., Waite, R., Hanson, C., and Ranganathan, J. (2019). Creating a Sustainable Food Future: A Menu of Solutions to Feed Nearly 10 Billion People by 2050. Washington, DC: World Resources Institute.
Shitindi, M., Kpomblekou, K.-A., McElhenney, W., Ankumah, R., Semoka, J., Bekunda, M., et al. (2019). Maize response to leguminous biomass composted with phosphate rocks in the northern zone of Tanzania. J. Exp. Agric. Int. 35, 1–15. doi: 10.9734/jeai/2019/v35i430209
Sileshi, G., Akinnifesi, F. K., Ajayi, O. C., and Place, F. (2008). Meta-analysis of maize yield response to woody and herbaceous legumes in sub-Saharan Africa. Plant Soil 307, 1–19. doi: 10.1007/s11104-008-9547-y
Smith, P., Daniel, M., Zucong, C., Daniel, G., Henry, J., Pushpam, K., et al. (2007). Greenhouse gas mitigation in agriculture. Phil. Trans. R. Soc. B 363789–363813. doi: 10.1098/rstb.2007.2184
Soma, D. M., Kiba, D. I., Ewusi-Mensah, N., Gnankambary, Z., Lompo, F., Sedogo, M. P., et al. (2018). Changes in sorghum production, soil P forms and P use efficiency following long-term application of manure, compost and straw in a Ferric Lixisol. Acta Agric. Scand. Sec. B Soil Plant Sci. 68, 401–411. doi: 10.1080/09064710.2017.1414871
Stevenson, J., Vanlauwe, K., Macours, N., Johnson, L., Krishnan, F., Place, D., Spielman, K., et al. (2019). Farmer adoption of plot- and farm-level natural resource management practices: between rhetoric and reality. Glob. Food Sec. 20, 101–104. doi: 10.1016/j.gfs.2019.01.003
Stevenson, J. R., and Vlek, P. (2018). Assessing the Adoption and Diffusion of Natural Resource Management Practices: Synthesis of a New Set of Empirical Studies. Rome: Independent Science and Partnership Council (ISPC).
Stringer, L. C., Fraser, E. D. G., Harris, D., Lyon, C., Pereira, L., Ward, C. F. M., et al. (2020). Adaptation and development pathways for different types of farmers. Environ. Sci. Policy 104, 174–189. doi: 10.1016/j.envsci.2019.10.007
Thierfelder, C., Bunderson, W. T., Jere, Z. D., Mutenje, M., and Ngwira, A. (2016). Development of conservation agriculture (CA) systems in Malawi: Lessons learned from 2005 TO 2014. Exp. Agric. 52, 579–604. Available online at: https://www.cambridge.org/core/journals/experimental-agriculture/article/development-of-conservation-agriculture-ca-systems-in-malawi-lessons-learned-from-2005-to-2014/9B734C7FB469342FC158F4B5B3F759B9s
Tian, G., Hauser, S., Koutika, L.-S., Ishida, F., and Chianu, J. N. (2001). Pueraria cover crop fallow systems: benefits and applicability. Sustaining Soil Fertility in West Africa, Soil Science Society of America Inc. doi: 10.2136/sssaspecpub58.c7
Vanlauwe, B., Chianu, J., Giller, K.E., Merckx, R., Mokwunye, U., Pypers, P. K., et al. (2010). Integrated soil fertility management: operational definition and consequences for implementation and dissemination. Outlook Agric. 39, 17–24. doi: 10.5367/000000010791169998
Vanlauwe, B., Coyne, D., Gockowski, J., Hauser, S., and Huising, J. (2014). Sustainable intensification and the African smallholder farmer. Curr. Opin. Environ. Sustain. 8, 15–22. doi: 10.1016/j.cosust.2014.06.001
Vanlauwe, B., Descheemaeker, K., Giller, K. E., Huising, J., Merckx, R., Nziguheba, G., et al. (2015). Integrated soil fertility management in sub-Saharan Africa: unravelling local adaptation. Soil 1, 491–508. doi: 10.5194/soil-1-491-2015
Vanlauwe, B., Hungria, M., Kanampiu, F., and Giller, K. E. (2019). The role of legumes in the sustainable intensification of African smallholder agriculture: lessons learnt and challenges for the future. Agric. Ecosyst. Environ. 284:106583. doi: 10.1016/j.agee.2019.106583
Vermeulen, S., Bossio, D., Lehmann, J., Luu, P., Paustian, K., Webb, C., et al. (2019). A global agenda for collective action on soil carbon. Nat. Sustain. 2, 2–4. doi: 10.1038/s41893-018-0212-z
Westermann, O., Förch, W., Thornton, P., Körner, J., Cramer, L., and Campbell, B. (2018). Scaling up agricultural interventions: case studies of climate-smart agriculture. Agric. Syst. 165, 283–293. doi: 10.1016/j.agsy.2018.07.007
World Bank (2020). Kenya Project Boosts Maize Production and Climate Change Benefits. Available online at: https://www.worldbank.org/en/news/feature/2017/07/18/kenya-project-boosts-maize-production-and-climate-change-benefits (accessed August 5, 2020).
Keywords: soil health, sustainable agriculture, smallholder farming, soil management and conservation, conservation agriculture, technology adoption
Citation: Klauser D and Negra C (2020) Getting Down to Earth (and Business): Focus on African Smallholders' Incentives for Improved Soil Management. Front. Sustain. Food Syst. 4:576606. doi: 10.3389/fsufs.2020.576606
Received: 26 June 2020; Accepted: 31 August 2020;
Published: 06 October 2020.
Edited by:
Vijay Singh Meena, Borlaug Institute for South Asia (BISA), IndiaReviewed by:
Robert J. Kremer, University of Missouri, United StatesCopyright © 2020 Klauser and Negra. This is an open-access article distributed under the terms of the Creative Commons Attribution License (CC BY). The use, distribution or reproduction in other forums is permitted, provided the original author(s) and the copyright owner(s) are credited and that the original publication in this journal is cited, in accordance with accepted academic practice. No use, distribution or reproduction is permitted which does not comply with these terms.
*Correspondence: Dominik Klauser, ZG9taW5pay5rbGF1c2VyQHN5bmdlbnRhLmNvbQ==
Disclaimer: All claims expressed in this article are solely those of the authors and do not necessarily represent those of their affiliated organizations, or those of the publisher, the editors and the reviewers. Any product that may be evaluated in this article or claim that may be made by its manufacturer is not guaranteed or endorsed by the publisher.
Research integrity at Frontiers
Learn more about the work of our research integrity team to safeguard the quality of each article we publish.