- 1Department of Soil Science, Punjab Agricultural University, Ludhiana, India
- 2Department of Agronomy, Horticulture and Plant Science, South Dakota State University, Brookings, SD, United States
Fertilizer-N application and straw incorporation impacts crop productivity due to changes in total organic carbon (TOC), its labile pools and the soil enzymatic activity. A field experiment was established (in 2010) to study the effect of fertilizer-N application (0, 90, 120, and 150 kg N ha−1) and rice straw (RS) incorporation (0, 5.0, 7.5, and 10 Mg ha−1) on crop yield, C input, TOC and its labile pools and soil enzymatic activity under rice (Oryza sativa L.)—wheat (Triticum aestivum L.) cropping system (RWCS) in north-western India. Data showed that fertilizer-N application and RS incorporation significantly (p < 0.05) increased the rice and wheat grain yield, compared with control (CK). However, the RS incorporation alone did not impact crop yields. The sustainable yield index (SYI) for wheat was significantly lower with RS incorporation alone as compared with the other treatments. For rice, SYI was significantly higher for RS7.5N120, and was non-significant compared with the RS10.0N120. Annual total C input in soil plow layer (0–15 cm) under RWCS varied between 3.34 and 9.78 Mg C ha−1, which was higher by 4.4 Mg C ha−1 yr−1 (~2.3-times) in RS10.0, compared with CK. The conjoint application of fertilizer-N and RS incorporation (RS10.0N150) significantly increased the TOC, water extractable organic C (WEOC), hot water C (HWC), microbial biomass C (MBC) and basal soil respiration (BSR) due to increased soil enzymatic activity viz. dehydrogenase activity (DHA), fluorescein diacetate (FDA) and alkaline phosphatise (Alk-P). A significant increase in potassium permanganate oxidizable C (KMnO4-C) with RS incorporation under RS10.0N150 increased the C management index (CMI), and hence the soil quality. The inter-correlations between highly weighted soil variables among different principle components (PCs) revealed that KMnO4-C in PC1, FDA activity in PC2, and BSR in PC3 with the highest correlation were the sensitive indicators for assessing soil quality in a RWCS.
Introduction
Rice-wheat cropping system (RWCS) occupies ~10 million ha (M ha) in Indian Indo-Gangetic Plains (IGPs) (Saharawat et al., 2012) that has been a lifeline for millions of food producers and consumers contributing ~85% toward country's cereal production (Timsina and Connor, 2001). Since green revolution, the RWCS has successfully maintained the balance between food grain supply and human population growth. This has become possible with the development of high yielding varieties of rice and wheat, availability of chemical fertilizers at the cheap prices, extension in irrigation network and farm mechanization, besides expansion of arable area. Nonetheless, the resource and energy intensive tillage practices under RWCS (Singh et al., 2019a,b) have caused negative environmental externalities, groundwater depletion (Hira et al., 2004), soil health degradation (Ladha et al., 2009) and loss of nutrients through emissions and leaching, declining farm productivity and shrinking farm profits (Chauhan et al., 2012; Srinivasan et al., 2012). A limited or no use of organic manures and crop residues removal (Ghosh et al., 2016), wide-spread adoption of mono-cropping (Hazra et al., 2014), imbalanced use of chemical fertilizers (Brar et al., 2013; Singh and Benbi, 2018a) have aggravated problems related to deterioration in soil quality.
Extensive tillage and removal of crop residue either as feedstock or as most prevalent practice of in-situ burning after mechanical harvesting of crops in the Indian IGPs (Beri et al., 2003), has raised soil health and environment threatening concerns due to large emersions of greenhouse gases (GHGs) (Singh et al., 2020). The TOC is the most important component in maintaining soil quality due to its role in improving physical, chemical and biological properties of the soil (Singh and Benbi, 2016, 2018b; Sharma et al., 2019a) and crop productivity (Yang et al., 2012; Thind et al., 2019). Because of the historic losses of organic C from the croplands (Singh and Benbi, 2018b), most of the cultivated soils are exhausted of TOC and are far away from saturation (Vaccari et al., 2011). But the capacity of soils to sequester C depends on amount of C input into the soil from plant productivity, relative to its export that is controlled by microbial decomposition (Benbi et al., 2016; Singh and Benbi, 2020a). The management practices that ensure greater amounts of C returned to the soil are expected to cause a net build-up of TOC stock (Singh and Benbi, 2020b). Maintaining TOC stocks is important for sustainable agricultural development and the mitigation of global warming by reducing C emissions (Lal, 2004; Li et al., 2005; Singh and Benbi, 2020a).
The crop residue return and incorporation into the soil has been widely accepted approach for maintaining TOC stocks in croplands (Hadas et al., 2004; Lian et al., 2016). Understanding the dynamics and fate of crop residues' C, which are decomposed by series of extracellular enzymes in soil help to clarify the underlying mechanisms of C sequestration in soils (Saha et al., 2005; Cotrufo et al., 2015). A significantly improved soil enzymatic activity following residue incorporation (Garg and Bahl, 2008) had shown positive impact on crop productivity under contrasting tillage systems (Jin et al., 2009).
Soil microbial biomass and enzymatic activity are the potential indicators of soil quality that rapidly responds to management and environmental induced changes (Mohammadi, 2011). All crop residues' C passes through soil microbial biomass at least once, and thereby transferred from one C pool to another and intern lost as carbon dioxide (CO2) (Ryan and Aravena, 1994). The un-decomposed crop residue remains in soil contributes toward C sequestration (Lorenz and Lal, 2005; Majumder and Kuzyakov, 2010). In fact decomposition pattern of rice residues is related to its quality, with non-cellulosic polysaccharides and hemi-celluloses being fast degradable rates are decomposed during the initial phase (Thevenot et al., 2010), while the stable/resistant C in lignin and cellulose are decomposed later in second phase (Majumder and Kuzyakov, 2010). Although, degradation and transformation of cellulose and lignin are highly complex, extra-cellular enzymatic activity of β-glucosidases, cellobiohydrolases and endo-glucanases enzymes had the high cellulose degradation abilities (Haichar et al., 2007; Baldrian and Valášková, 2008), while lignin degradation is caused by laccases, lignin peroxidases and Mn-peroxidases enzymes (Higuchi, 2004).
Soil organic C is a heterogeneous mixture of organic materials comprising labile and stabilized (i.e., recalcitrant) organic C pools based on variable turnover times (Singh and Benbi, 2018a). The dynamics of TOC is described by partitioning the soil organic matter (SOM) into physical, chemical and biological pools (Banger et al., 2010). The change in C input to soil can rapidly persuade TOC pools viz. potassium permanganate oxidizable C (Blair et al., 1995), water extractable organic C (Benbi et al., 2015), hot water C (Li et al., 2012; Singh and Benbi 2018b), and microbial biomass C (Chan et al., 2001). Labile C fractions act as sensitive indicators to soil management induced changes in TOC pool under short to medium term effects (Benbi et al., 2015). These pools have shorter turnover times and are more sensitive indicators to soil management practices compared with TOC (Yan et al., 2007; Chen et al., 2016). Conversely, the recalcitrant pools are difficult for soil microorganisms to decompose and have relatively long turnover times (Yan et al., 2007).
A large amount of RS is produced under RWCS and has been the major C input (Singh et al., 2020). Soil incorporation of rice residue has beneficial impact on the succeeding wheat crop (Mandal et al., 2004; NAAS, 2017). On the other hand, soil degradation has been the serious problem aroused due to in-situ open field burning of above-ground crop residues under conventional tillage system (Beri et al., 1995). Rice straw is an important organic C source which contains nutrients for the optimum crop growth (Beri et al., 1995; Yadvinder-Singh, 2014) and has been the most viable management option for improved soil fertility and crop productivity (Huang et al., 2013; Wang et al., 2015a). The RS incorporation increased TOC pool by 13% and its labile fractions by 42%, compared with the application of chemical fertilizers alone (Liu et al., 2014). Several studies showed that crop yield and TOC increased by straw incorporation under different cropping systems (Sidhu and Beri, 1989; Singh et al., 2005; Wang et al., 2015a; Zhu et al., 2015). However, the C:N ratio gets widened after RS incorporation, because straw temporarily lock-up N and influence crop growth and yield of succeeding wheat crop (Malhi et al., 2012; Huang et al., 2013). The RS incorporation intern impacts the C dynamics in the soil due to changed enzymatic activity in the soil (Bera et al., 2018; Sharma et al., 2019a). Soil enzymatic activity had a key role to play in SOM decomposition and mineralization via several metabolic processes (Denef et al., 2009). Although, decomposition of SOM is decreased under anaerobic conditions simulated during rice (Kögel-Knabner et al., 2010), yet alternate wet and dry spells characterized by rice-wheat rotation stimulate microbial activity and fasten SOM degradation and mineralization due to increased enzymatic activity (Denef et al., 2001). Soil enzymatic activity of arylsulfatase, urease, β-glucosidase and acid phosphatase has dynamically altered the TOC and its labile pools (Green et al., 2007; Medeiros et al., 2017; Hok et al., 2018). The soil enzymatic activity is considered as sensitive indicator to reflect management and cropping system induced change in soil C dynamics (Green et al., 2007; Rabary et al., 2008).
Surface retention of crop residues under no-tillage system has been reported to greatly enhance the soil enzymatic activity, which was related to increased microbial biomass in the soil (Hok et al., 2018; Saikia et al., 2019). This has significance in RWCS, where alteration in moisture regimes during two seasons changed the pattern of microbial decomposition of C input into the soil triggered by enzymatic activity (Choudhary et al., 2018; Parihar et al., 2018). The TOC pools and enzymatic activity under different straw management regimes vary substantially depending on climate factors, soil types, and experimental conditions (Wang et al., 2015a; Zhao et al., 2015). Surface retention of crop residues under no-tillage system has been reported to increase the enzymatic activity of alkaline phosphatase (Alk-P), cellulose and acid phosphatase by ~61, 90, and 46%, respectively, compared with conventionally tilled soils (Balota et al., 2004). The studies on how fertilizer-N application and in-situ RS incorporation impacts the TOC pool and its fractions due to changed enzymatic activity under RWCS are lacking. Based on the review of aforesaid studies, we hypothesized that fertilizer-N application conjointly with in-situ RS incorporation would increase crop productivity, C input and enzymatic activity to accelerate the SOM decomposition process. The present study was therefore, conducted to investigate the effect of fertilizer-N application and RS incorporation on (1) crop productivity and C input through above-and below-ground plant biomass (2) change in soil enzymatic activity and TOC pools, and (3) C sequestration potential of crop residue management under RWCS. We aimed at identifying organic C pools influenced due to changed soil enzymatic activities to assess ability of rice residue management to sequester C and suggest long-term best agricultural sustainability options.
Materials and Methods
Experimental Site and Climate
A field experiment on rice (Oryza sativa L.)-wheat (Triticum aestivum L.) cropping system was established during 2010 at the research farm of Department of Soil Science at Punjab Agricultural University (P.A.U.), Ludhiana, Punjab (30°56′N and 75°52′E) in the IGPs of north-western India. The surface (0–15 cm) soil was sandy loam (128 g claykg−1, 155 g silt kg−1, and 715 g sand kg−1) and classified as Typic Ustochrept (USDA classification). The climate of the region is typically a sub-tropical, semi-arid with mean annual rainfall of ~700 mm, of which ~75–80% is received during summer (July-August), while remaining ~20–25% during winter season (December-January). Mean monthly temperature varied between 12.8 and 26.7°C. At the start of experimentation, the surface soil was non-saline (electrical conductivity, E.C.1:2 = 0.34 dSm−1) with pH1:2 of 6.6 and had 3.91 g TOC kg−1, 29.4 mg P kg−1 and 78.4 mg K kg−1. Total organic C was determined using wet digestion method (Snyder and Trofymow, 1984; Singh and Benbi, 2018a), available-P was determined using ascorbic acid method, and available-K by extracting the soil with neutral (pH = 7.0) normal ammonium acetate followed by K determination on flame photometer.
Experimental Layout and Treatments and Crop Management Practices
The experiment consisted of four fertilizer-N levels viz. 0, 90, 120, and 150 kg N ha−1, and four RS incorporation rates viz. 0, 5.0, 7.5, and 10.0 Mg ha−1 with four replications, thus constituting a total of 16 treatments under RWCS. In rice, fertilizer-N (as per treatments) in the form of urea (46% N) was applied in three equal splits viz. 1/3rd at transplanting, 1/3rd 21 days after transplanting, and the remaining 1/3rd 42 days after transplanting every year. In rice, 10 kg Zn ha−1 as zinc sulfate heptahydrate (ZnSO47H2O, 21% Zn) was applied at transplanting in all treatment plots every year. Rice was established after wet-tillage (tillage in standing water) for making an impervious soil layer to facilitate seedling growth and establishment, and management of weeds at initial stage. After wet-tillage (i.e., puddling), thirty daysold rice seedlings (var. PR-118) were transplanted manually at 15 × 20 cm spacing in the second week of June every year. All plots were kept flooded (50 mm) for initial 15 days after seedling transplanting, followed by irrigation (50 mm) 2 days after the disappearance of standing water from the previous irrigation till physiological maturity. Rice was harvested (near ground surface) manually in the 3rd week of October, and whole of the RS was removed from the plots. It was chopped with RS chopper and incorporated in soil plow layer with a rotavator as per treatment. Following pre-sowing irrigation, wheat was sown in rows 20 cm apart with a zero-till drill at field capacity moisture content in the second week of November. In wheat, a basal dose of 60 kg P2O5 ha−1 and 30 kg K2O ha−1 was applied every year. Fertilizer-N (as per treatments) was applied in three equal splits, 1/3rd basal (at sowing), and remaining 2/3rd in equal splits i.e., immediately before 1st irrigation (crown root initiation) and at 2nd irrigation (maximum tillering) every year. Irrigations of (75 mm each) were applied at critical growth stages viz. crown root initiation, maximum tillering, panicle initiation and at dough stage. Crop was harvested manually at physiological maturity in the 1st fortnight of April every year. For yield measurement, a net plot area of 10 m2 (2.0 × 5.0 m) was harvested manually, and grains from the above-ground biomass were removed using plot-thresher. Grain yield of rice and wheat were reported at 14 and 10% moisture content, respectively. Straw yields (rice and wheat) were reported on oven-dry weight basis. The SYI was estimated based on yield data of 4 years (2012–2016) when the field treatments get stabilized using equation (Equation 1).
where, “Y” refers to actual yield (Mg ha−1), “σ” refers to standard deviation in yield (Mg ha−1) and “YMax” refers to the maximum yield (Mg ha−1) obtained. The harvest index (HI) was estimated as a ratio of grain yield to that of total above-ground (grain + straw) biomass.
Estimation of Carbon Input
The C input through above-and below-ground vegetative biomass viz. roots and shoot biomass were estimated considering C concentration in roots of rice and wheat of 41.2 and 39.1%, respectively. The C concentration of 31.8% in rice and 35.2% in wheat shoot was considered (Majumder et al., 2007) for estimating shoots derived C. The C contribution of rhizodeposition of 15 and 12.6%, respectively, of above-ground biomass at harvest for rice and wheat was considered in the calculations (Bronson et al., 1998).
Collection and Preparation of Soil Samples
Soil samples were collected in the last week of April 2016 after six cycles of rice-wheat cropping system from surface (0–7.5 cm) and sub-surface (7.5–15 cm) soil depths with core sampler (inner diameter 7.2 cm). After removing visible root debris, the soil samples from each plot were sieved through 2 mm sieve and divided into two halves. While, one-half of sample was immediately stored at 4°C to assay microbial activities viz. DHA, FDA, Alk-P, MBC, and C mineralization studies, the remaining half was air-dried and stored for the estimation of C pools. The methods used for assaying enzymatic activity and C pools are detailed (see Appendix-I).
Carbon Management Index
Based on changes in TOC between the reference (CK, RS0N0) and a treatment, C management index (CMI) (Equation 2) was calculated using a mathematical procedure of Blair et al. (1995).
Where, “CPI” is the C pool index and “LI” is the lability index which were estimated using following equations (Equations 3–5).
The CPI and the LI were calculated as follows:
where, “L” refers to the C lability, calculated as
The content of non-labile C was estimated from the difference between TOC pool and labile C (KMnO4-C).
Statistical Analysis
The data on crop yield, C pools and soil enzyme activity were statistically analyzed using analysis of variance (ANOVA) as per completely randomized block design (RBD). The differences between the treatment means were separated by the Duncan's Multiple Range Test (DMRT) at p < 0.05 using IRRISTAT package data analysis (IRRI, 2000). The principal component analysis (PCA) capable of avoiding biasness in data was used to study the effect of treatments on different variables using biplots. The principal components (PCs) with high “eigen values” and higher “factor loading” were considered best predictors of system attributes. In PCs, the highly weighted factors were retained, while others were discarded for obtaining minimum data set (MDS). Only the highly correlated variables were considered redundant and considered for MDS, and were arranged in an order depending on bases of “good” or “bad” in terms of soil functions. To test for statistical significance, Monte Carlo Analysis (MCA) was performed using MC-PCA software, and sphericity of Bertlette test was performed (at p < 0.05). The correlation matrix depicting relationship between soil organic C pools and enzymatic activity were developed using SPSS for Windows (SPSS, Inc, Chicago). The relationships significant at p < 0.05 and p < 0.01 were marked as * and **, respectively while the non-significant relationships were marked as NS.
The soil quality index (SQI) was calculated using integrated score and weight factor of each indicator using Equation 6.
where, “S” = indicator score, W= PCs weight factor.
Results
Rice and Wheat Yields, Harvest Index, and Sustainable Yield Index
The periodic differences in grain and straw yield of rice and wheat under the influence of fertilizer-N application and RS incorporation are presented in Table 1. From 2012 to 2015, the average rice and wheat grain yields varied from 4.87 to 6.41 Mg ha−1 and 2.01–5.76 Mg ha−1, respectively. Fertilizer-N application and RS incorporation significantly (p < 0.05) increased the rice and wheat yield, compared with CK (Table 1). Significantly higher rice and wheat yields were obtained under RS7.5N120, than the CK. Grain yield did not differ significantly among RS7.5N120 and RS7.5N150 treatments. Alone RS incorporation resulted in a non-significant change in rice and wheat grain yields during different years. As compared with RS7.5N0, RS7.5N90, RS7.5N120, and RS7.5N150 treatments increased the rice grain yield by 7.2–13.8% and wheat grain yield by 24.3–63.7%. Rice straw yield was significantly higher in RS7.5 N120, compared with other treatments. Rice and wheat yields differ significantly among years and treatments (Table 2). Results revealed that rice straw yield did not differ significantly among years, but wheat straw yield was statistically significant among years. The interaction effect of years and treatments was significant on straw yields of rice and wheat.
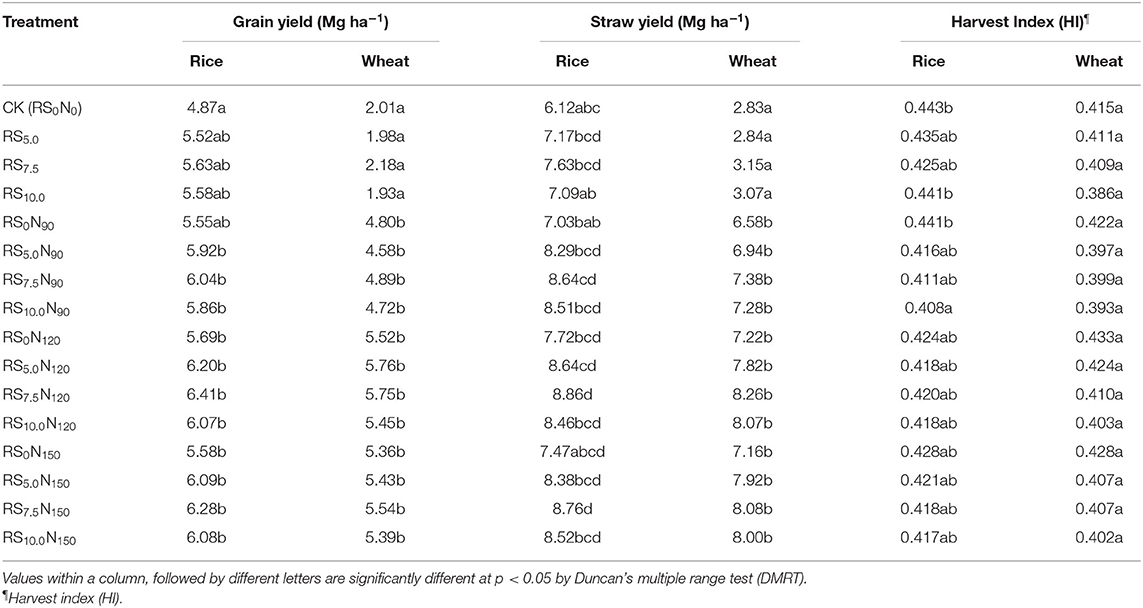
Table 1. Effect of fertilizer-N application and rice straw (RS) incorporation on grain and straw yield and harvest index (HI) of rice and wheat in a sandy loam soil under rice-wheat cropping system (data pooled for 4 years) in north-western India.
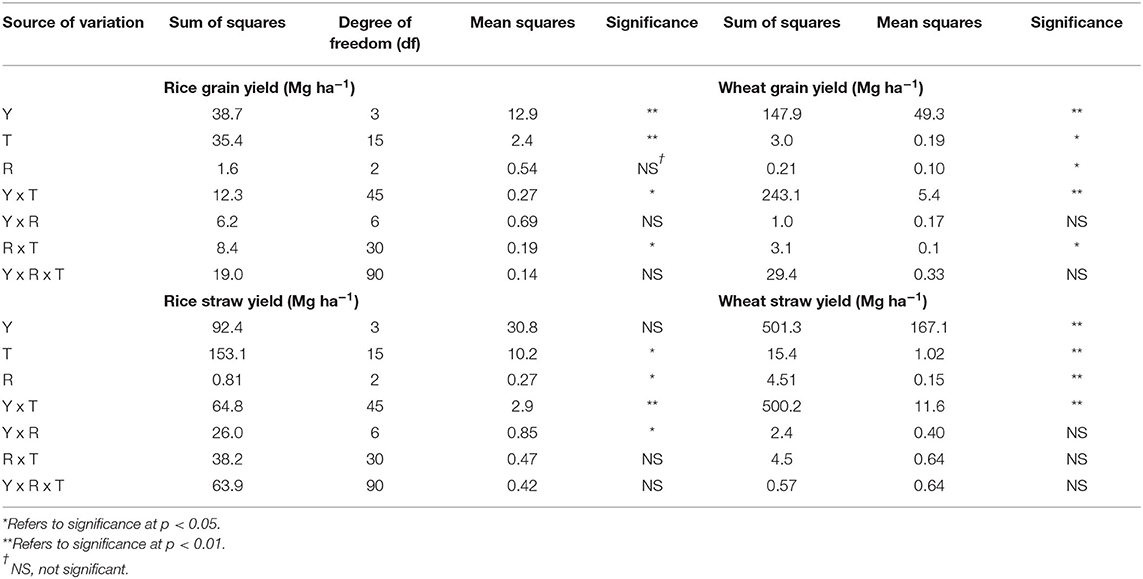
Table 2. Results of two-way analysis of variance (ANOVA) on the effects of years (Y), treatments (T), replications (R), and their interaction effect on rice and wheat yields (grain and straw).
The HI of rice was significantly lower in RS10.0N90, compared with other treatments (Table 1). However, HI of wheat did not differ significantly among treatments. The SYI for wheat was significantly lower for alone RS incorporation treatments (viz. RS5.0, RS7.5, and RS10.0), compared with other treatments (Figure 1). These results revealed that increased RS incorporation rates even at higher fertilizer-N application rates leads to reduced SYI. It also did not differ significantly among different RS incorporation and fertilizer-N application rates. The SYI for wheat was significantly higher for RS0N120, although it was at par with RS5.0N150. Conversely, SYI for rice was significantly higher for RS7.5N120, and was statistically non-significant compared with RS7.5N90 and RS10.0N120.
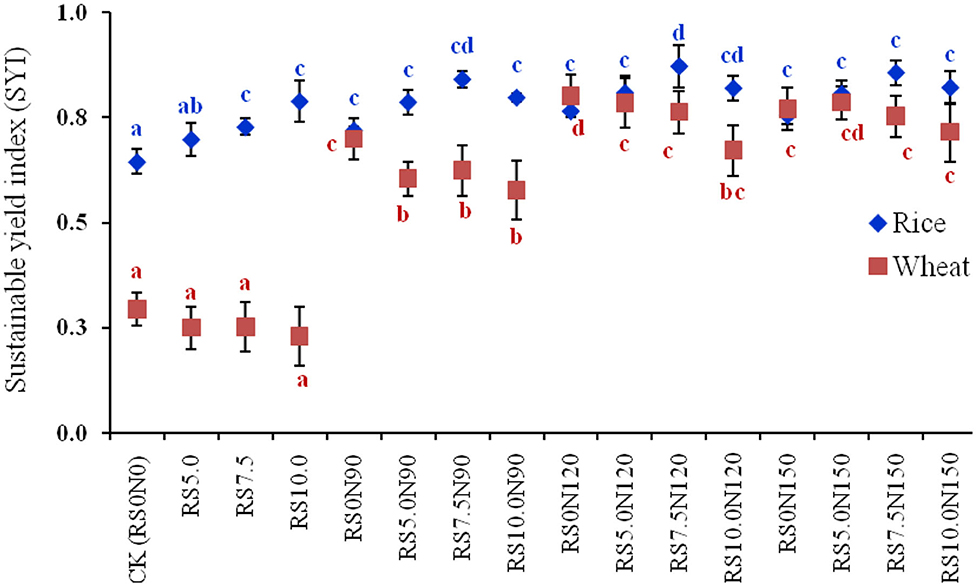
Figure 1. Sustainable yield index (SYI) of rice and wheat grown under rice-wheat cropping system in north-western India. Line bars indicate standard error from mean. Values for a crop followed by different letters are significantly different at p < 0.05 by Duncan's multiple range test (DMRT).
Carbon Input Into Soil Under Rice-Wheat Cropping System
In an system annual RWCS, root C input varied between 0.87 and 1.49 Mg C ha−1 in the surface (0–15 cm) soil depth (Table 3). Root C input in soil did not differ significantly with fertilizer-N application rates (90 and 150 kg N ha−1). The highest root and stubble biomass C was obtained in RS7.5N120. The total C input in the surface soil layer under RWCS varied between 3.34 and 9.78 Mg C ha−1 yr−1. The total C input increased by 4.4 Mg C ha−1 yr−1 (~2.3-times) in RS10.0, compared with CK. The C input through root, stubble and rhizodeposition respond significantly to fertilizer-N application. In RS0N90 treatment, total C input increased by ~47.3% as compared to CK. With further increase in fertilizer-N application rate (RS0N120), total C input increased by ~9% than in RS0N90. The fertilizer-N application at higher rate (RS0N150) did not significantly increase the total C input, compared with RS0N120.
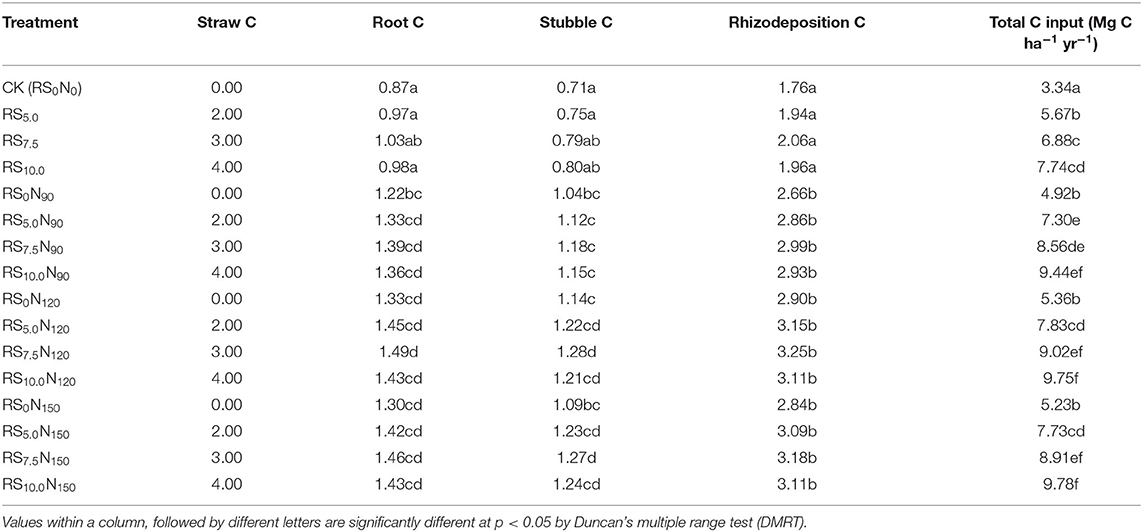
Table 3. Mean annual carbon input (Mg C ha−1) through roots, stubble and rhizodeposition, and addition of rice straw in soil plow (0–15 cm) depth under rice-wheat cropping system in north-western India.
Total Organic Carbon and Its Labile Pools
The TOC concentration varied between 4.37 and 6.44 g C kg−1 in the surface (0–7.5 cm) and 3.50 and 5.98 g C kg−1 in the sub-surface (7.5–15 cm) soil layers (Table 4). Alone fertilizer-N application (RS0N90, RS0N120, and RS0N150) did not significantly increase the TOC concentration in the surface soil layer, as compared with CK at both soil layers. Average across the treatments, TOC in the surface soil layer was ~18.4% higher, compared with sub-surface soil layer. The WEOC was the smallest fraction constituted only ~0.6% of TOC in both the layers, although the concentration of WEOC was ~20.4% higher in the surface than the sub-surface soil layer. The WEOC concentration was significantly lower in CK, and increased by 14.9 and 14.6 mg C kg−1 in the surface and sub-surface soil layers, respectively in RS10.0N150 treatment. The HWC constituting 4.9–5.2% of TOC pool was ~21% higher in the surface than the sub-surface soil layer. The concentration of HWC was significantly increased by ~2/3rd in RS10.0N150, compared with CK. Fertilizer-N application and RS incorporation significantly increased MBC, BSR and mineralization quotients, compared with CK (Table 5). The highest MBC was observed under RS10.0N150 (408.1 μg g−1 soil), and the lowest under CK (243.9 μg g−1 soil). The BSR was lowest (0.169 μg g−1 soil h−1) in CK, and was the highest under RS10.0N120 (0.214 μg g−1 soil h−1) treatment. A significant increase in microbial quotients (qCO2 and qmic) values were observed under fertilizer-N application and RS incorporation treatment. The MBC, BSR, microbial quotient were ~20.3, 30.2, and 56.4% higher in surface, compared with sub-surface soil layer.
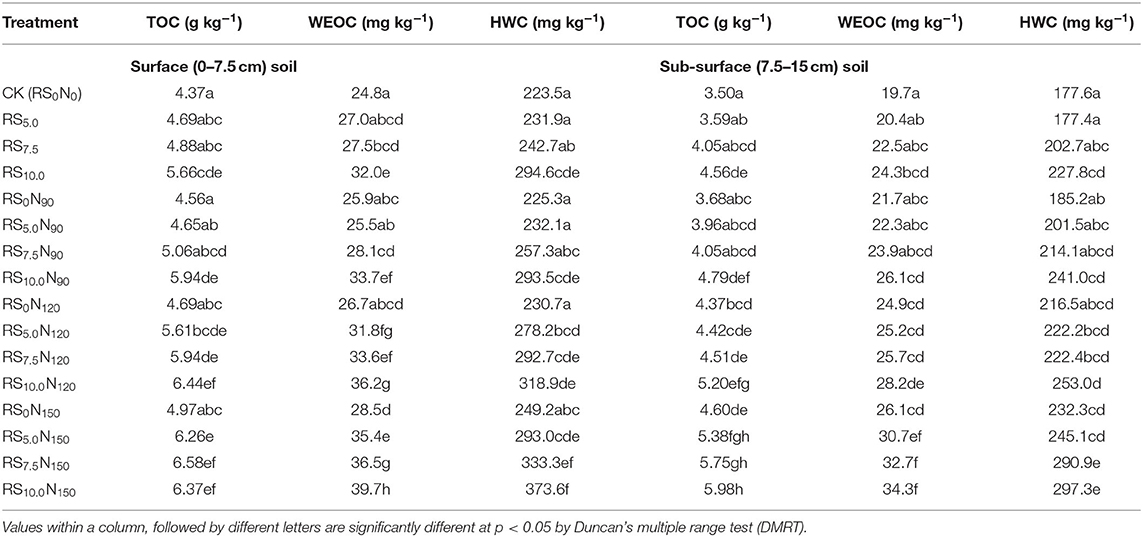
Table 4. Effect of fertilizer-N application and rice straw (RS) incorporation on total organic carbon (TOC), water extractable organic carbon (WEOC) and hot water carbon (HWC) in surface (0–7.5 cm) and sub-surface (7.5–15 cm) soil depth under rice-wheat cropping system in north-western India.
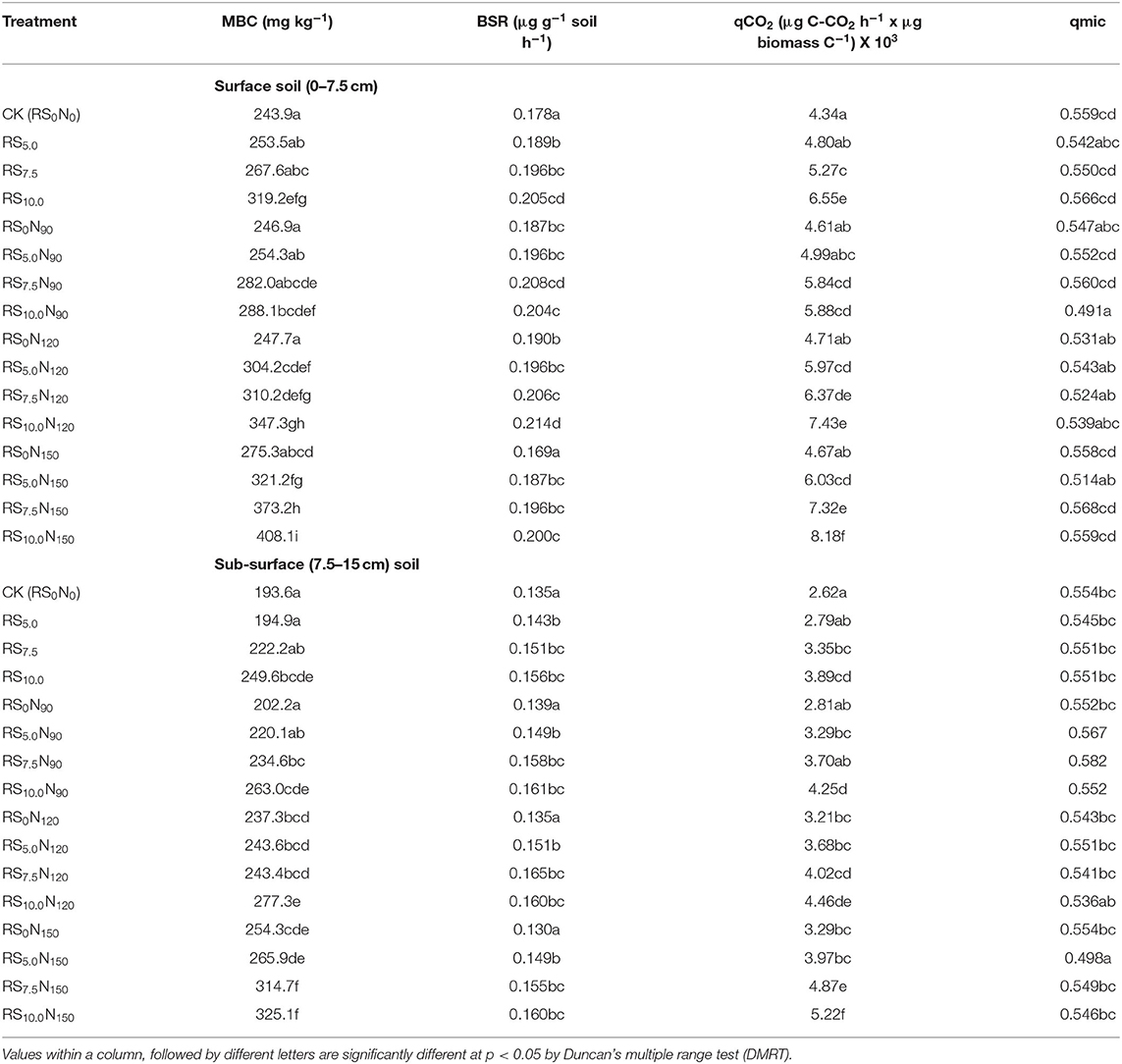
Table 5. Effect of fertilizer-N application and rice straw (RS) incorporation on microbial biomass carbon (MBC), basal soil respiration (BSR), respiratory (qCO2), and microbial (qmic) quotients of surface (0–7.5 cm) and sub-surface (7.5–15 cm) soil depth under rice-wheat cropping system in north-western India.
Potassium Permanganate Oxidizable Carbon and Carbon Rehabilitation
The concentration of KMnO4-C was significantly lower in CK and was the highest in RS10.0N150 (Table 6). KMnO4-C did not increase significantly in RS10.0N90 and RS10.0N120 treatments, but increased significantly with higher fertilizer-N application rate (RS10.0N150) as compared to RS alone (RS10.0). KMnO4-C concentration in the surface soil layer was ~34.9% higher than in sub-surface soil layer. The KMnO4-C concentration in the surface and sub-surface soil layers comprised ~10.1 and 8.9% of TOC, respectively. Our results showed that non-labile C comprised the major proportion of TOC in soil under RWCS. The non-labile C pool, was however ~10% higher in the surface, compared with the sub-surface soil layer. The CMI exhibited significantly higher values of 167 and 172, respectively for surface and sub-surface soil layers in RS10.0N150 treatment, as compared with their respective lower RS application rate treatments (RS7.5N150).
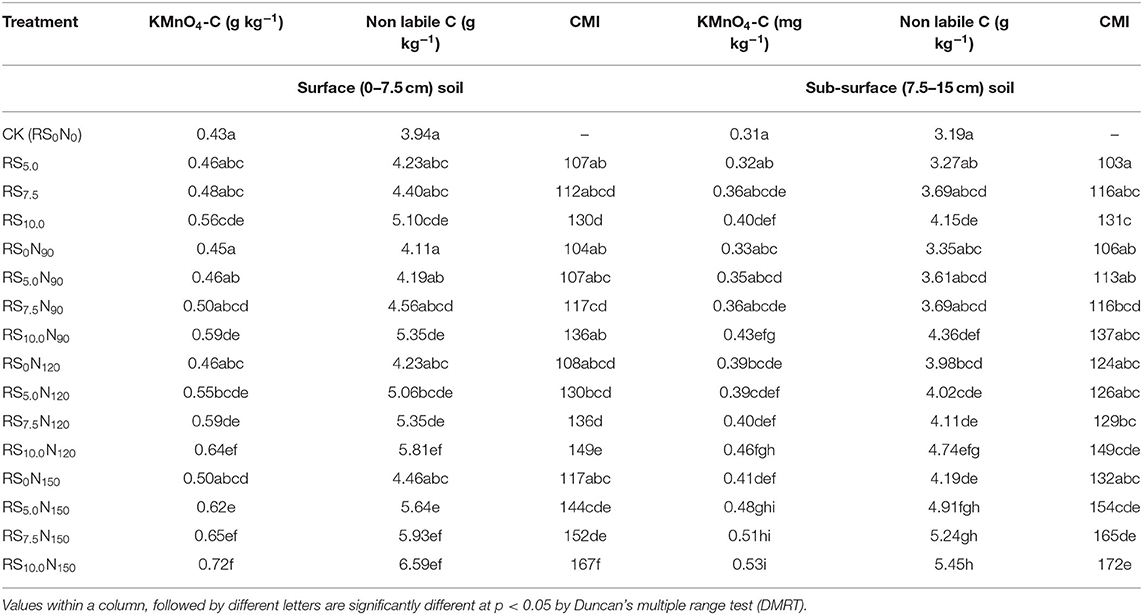
Table 6. Effect of fertilizer-N application and rice straw (RS) incorporation on potassium permanganate oxidizable carbon (KMnO4-C), non-labile carbon and carbon management index (CMI) of surface (0–7.5 cm) and sub-surface (7.5–15 cm) soil depth under rice-wheat cropping system in north-western India.
Soil Bulk Density and Carbon Stocks
Soil BD was significantly higher in CK, and was lowest for RS10.0N150 treatment (Figure 2). The alone RS10.0 application resulted in a significant decrease in soil BD, as compared with lower RS application rates. Fertilizer-N application at 90 and 120 kg N ha−1 did not significantly decrease the soil BD. However, TOC stocks were significantly lower in CK and were the highest in RS10.0N150 treatment (Figure 3). TOC stocks increased significantly by 2.3 Mg C ha−1 (~42.4%) in the surface, and 2.0 Mg C ha−1 (~49.3%) in the sub-surface soil layer in RS10.0N150 treatment, compared with their CK treatments. Data pooled for two soil depths revealed that TOC stocks increased by ~12.2, 14.4, and 21.8%, respectively in RS10N90, RS10N120, and RS10N150 treatments as compared with RS0 N90. Average across the treatments, TOC stocks were ~27.8% higher in the surface, compared with the sub-surface soil layer. Figure 4 illustrates the relationship between total annual C input (through above-and below-ground biomass) into the soil plow layer (0–15 cm) and TOC after 6 cycles of RWCS. The relationship could best be described by Equation 7.
Our results revealed that fertilize-N application at 90 kg N ha−1 maintained higher TOC level in soil at all RS incorporation rates under rice-wheat cropping system.
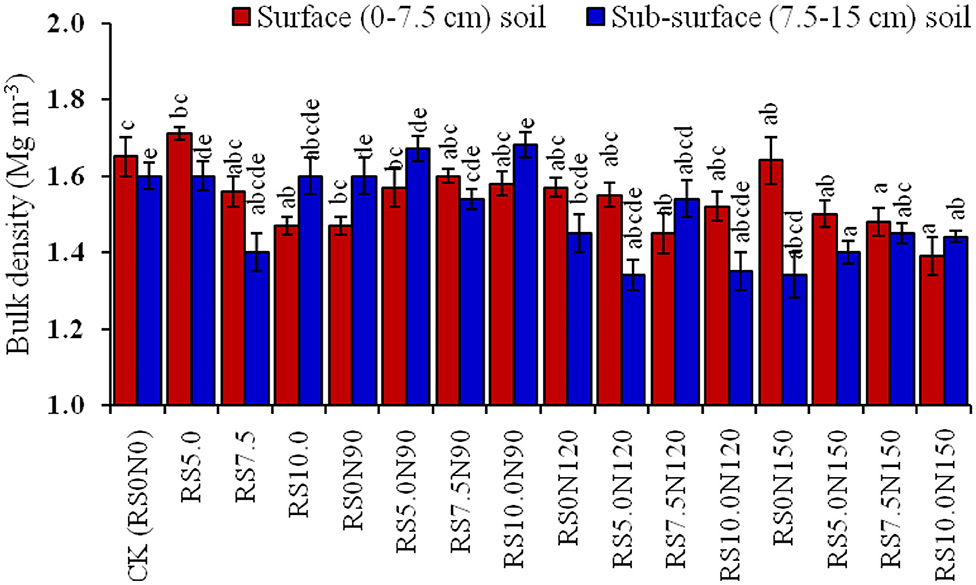
Figure 2. Effect of fertilizer-N application and rice straw (RS) incorporation on bulk density (BD, Mg m−3) in the surface (0–7.5 cm) and sub-surface (7.5–15 cm) soil depth under rice-wheat cropping system in north-western India. Line bars indicate standard error frommean. The values for a soil depth, followed by different letters are significantly different at p < 0.05 by Duncan's multiple range test (DMRT).
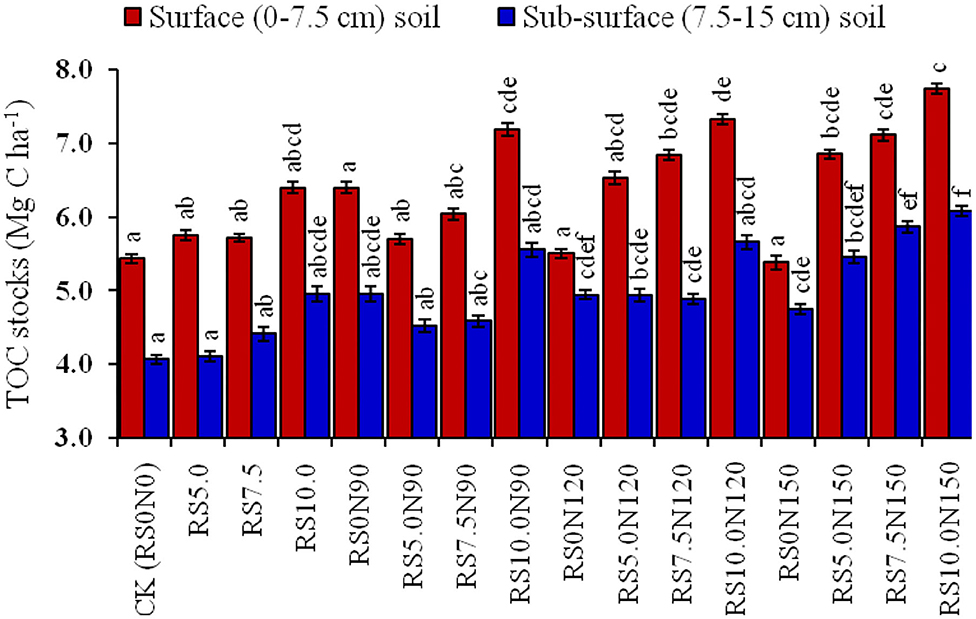
Figure 3. Effect of fertilizer-N application and rice straw (RS) incorporation TOC stock (Mg m−3) in the surface (0–7.5 cm) and sub-surface (7.5–15 cm) soil depth under rice-wheat cropping system in north-western India. Line bars indicate standard error from mean. The values for a soil depth, followed by different letters are significantly different at p < 0.05 by Duncan's multiple range test (DMRT).
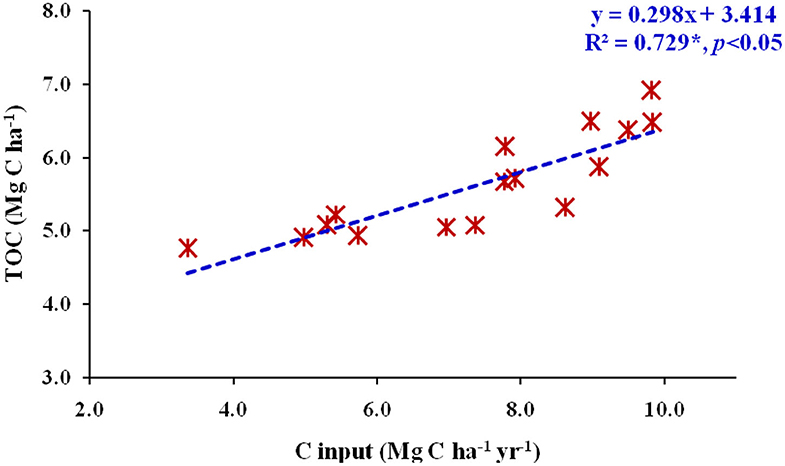
Figure 4. Relationship between total annual C input (Mg C ha−1 yr−1) and total organic C (TOC) (Mg C ha−1) in soil plow layer (0–15 cm) after six cycles of rice-wheat cropping system in north-western India.
Soil Enzymatic Activity and Microbial Quotients
Soil enzymatic activity (DHA, FDA and Alk-P) was significantly lower in CK and highest under RS10.0N150 treatment (Table 7). DHA activity did not increase significantly in RS10.0N90 and RS10.0N120 treatments, but increased significantly with increased fertilizer-N application rate (RS10.0N150), compared with RS10.0. Average across treatments, DHA in the surface soil layer was ~68.4% higher, than the sub-surface soil layer. The corresponding increase in FDA and Alk-P activity was ~10.5 and ~62.4%, respectively. The DHA, FDA, and Alk-P increased by ~49.4, 57.8, and 99.9%, ~45.1, 47.6, and 34.1% and ~65.2, 71.9, and 27.5%, respectively in RS10N90, RS10N120, and RS10N150 treatments as compared to CK.
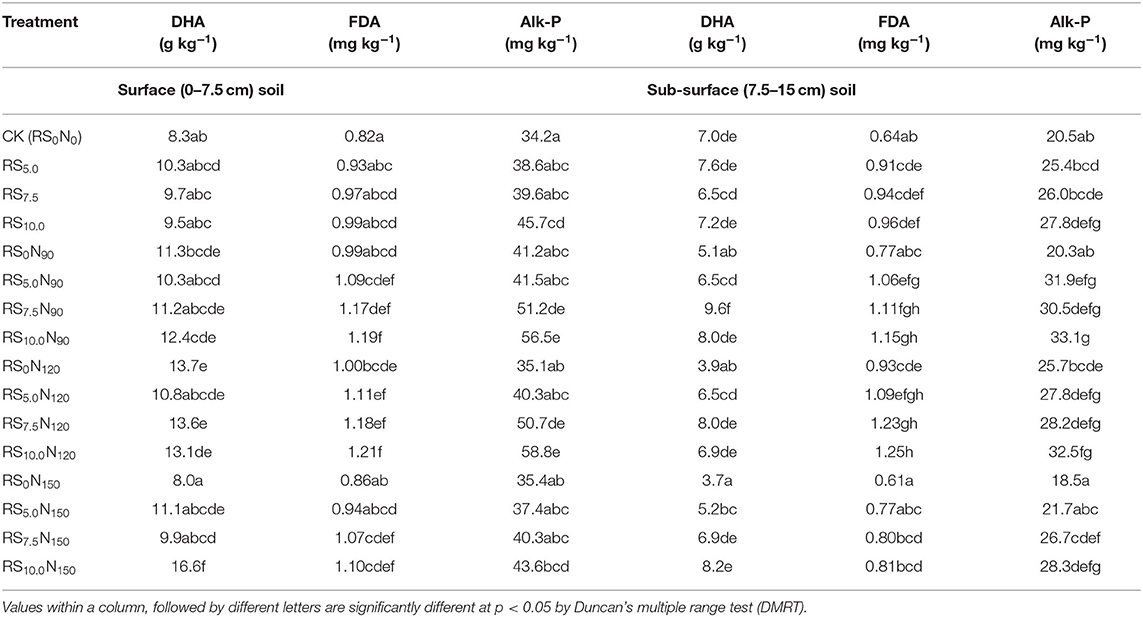
Table 7. Effect of fertilizer-N application and rice straw (RS) incorporation on dehydrogenase activity (DHA), fluorescein diacetate (FDA) and alkaline phosphatise (Alk-P) in surface (0–7.5 cm) and sub-surface (7.5–15 cm) soil depth under rice-wheat cropping system in north-western India.
Principal Component Analysis
The PCA analysis of 14 variables showed that first 3PCs had the Eigen value >1.0, and explained 87.8 % of the total variance in the data-set (see Appendix-II). The PC1 had 4 high weighted loading variables viz. KMnO4-C, non-labile-C, WEOC and TOC having loading values of 0.959, 0.958, 0.951, and 0.944, respectively. The cumulative variability explained by PC1was 66.8% with eigen value of 10.7. The PC2 explained ~12.8% of data-set variance, and had eigen value of 2.05. The PC2 illustrates the highest loading value of 0.621 for FDA activity, followed by −0.611 for qmic and −0.575 for Alk-P activity. The PC3 revealed the highly weighted variables of BSR, BD and qCO2 with corresponding loading values of −0.524, −0.204, and −0.192, respectively. The inter-correlations between highly weighted soil variables among different PCs (see Appendix-III) reveal that KMnO4-C in PC1, FDA activity in PC2, and BSR in PC3 with highest correlation could be chosen for MDS. The percent of total variance estimated based on weight of each PC ranged between 0.09 and 0.76. The weighted factor for three distinct MDS followed PC1 (0.76) > PC2 (0.15) > PC3 (0.09). Figure 5 illustrates the position of different variables and field treatments in the orthogonal space. The PC1 clearly separated N120 from N0, and RS10.0 from RS0 treatments in the factorial space. The KMnO4-C, FDA and BSR were located on the right end of the scoring plot indicating positive score for PC.
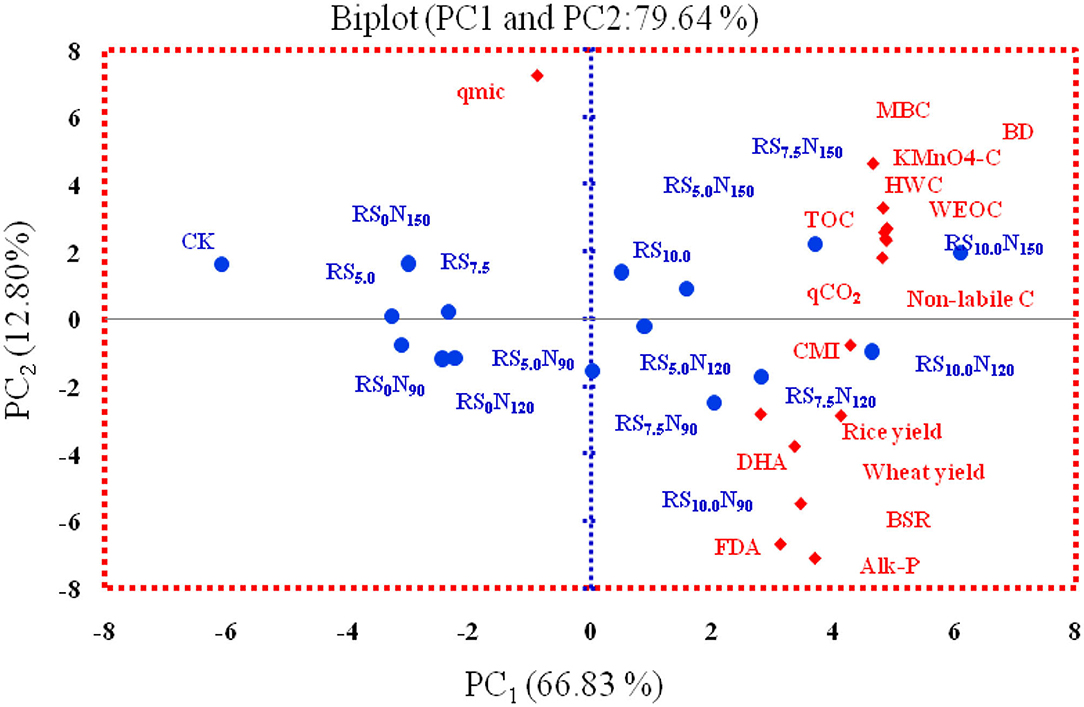
Figure 5. Biplots of Principal Component Analysis (PCA) using variables and field treatments for explaining variability in the data-set using principle components (PCs) (DHA, Dehydrogenase activity; FDA, Fluorescein diacetate activity; Alk-P, Alkaline phosphatase activity; TOC, Total organic carbon; KMnO4-C, Potassium permanganate oxidizable carbon; WEOC, Water extractable organic carbon; HWC, Hot water carbon; MBC, Microbial biomass carbon; BSR, Basal soil respiration; qCO2, Microbial quotient; Qmic, Metaboloic quotient; BD, Bulk density; N, Fertilizer-N and RS, Rice straw.
Among the treatments combination, the SQI ranged from 0.831 to 0.844 (Figure 6). The highest SQI value was obtained in RS10.0N120 (0.844), followed by RS10.0N90 (0.843). The lowest SQI value was obtained for the CK (0.831), followed by RS0N90 (0.897) suggesting relatively less aggregative effect of field treatments. The specific contribution of MDS toward SQI (see Appendix-IV), showed the highest contribution of KMnO4-C, followed by FDA and the lowest by BSR.
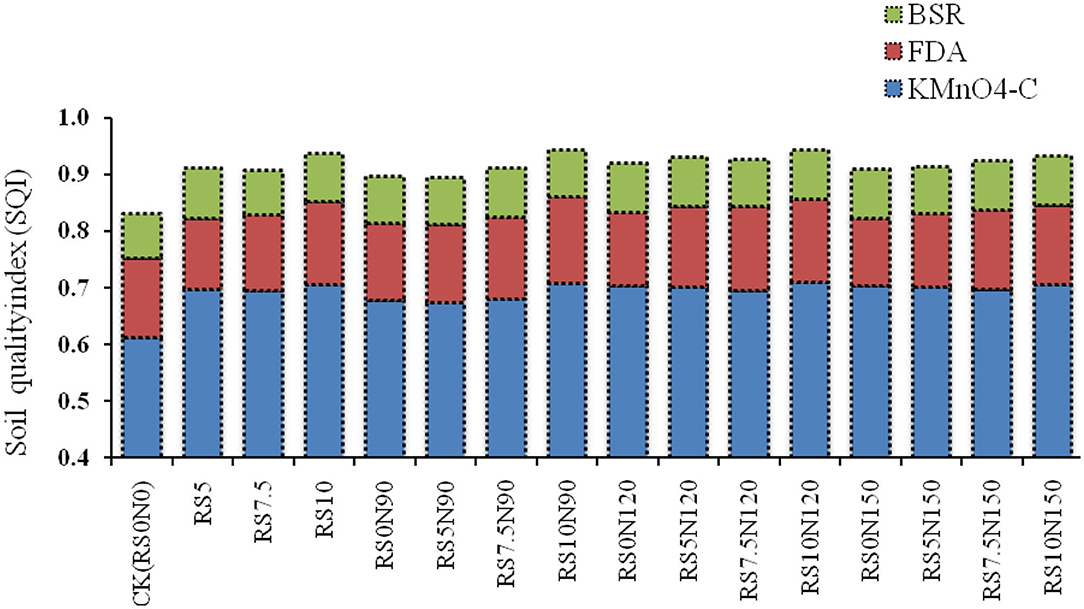
Figure 6. Average effect of fertilizer-N application and rice straw (RS) incorporation on soil quality index (SQI) and the individual contribution of key indicators (KMnO4-C, Potassium permanganate oxidizable carbon; FDA, Fluorescein diacetate activity; and BSR, Basal soil respiration).
Relationship Between Different Soil Organic Carbon Pools and Enzymatic Activity
A correlation matrix developed among different organic C pools showed that across different treatments, TOC and different C pools viz. HWC, WEOC, MBC, and KMnO4-C were significantly (p < 0.01) related to each other (see Appendix-V). Therefore, an enrichment and/or depletion in one would shift the equilibrium and change in the size of other pools. The FDA and DHA showed a highly significant relationship (r = 0.685**, p < 0.01). DHA showed a significant relationship with HWC (0.529*, p < 0.05) and non-labile C (r = 0.504*, p < 0.05).
Discussion
Effects of Fertilizer-N Application and Straw Incorporation on Crop Productivity
Previous studies showed inconsistent effect of RS incorporation on rice and wheat grain yields, those were attributed to straw quantity, time and method of straw incorporation, soil characteristics and the amount of fertilizer-N applied (Singh et al., 2005; Wang et al., 2010). In rice based cropping system, non-significant change in grain yield has been reported due to RS incorporation (Singh et al., 2005). In a long-term fertilizer experiment, continuous incorporation of residue significantly increased the rice grain yield by ~16% (Shang et al., 2011). In another study, Thomsen and Christensen (2004) reported a significant increase in wheat grain yield with RS incorporation over a period of 18 years. Our results showed that RS incorporation resulted in a significant increase in rice grain yield by 18.8% and wheat grain yield by ~3.8%, compared with no-straw incorporation. It suggests the positive response that occurred in subsequent years contributing toward a consistent supply of recycled P and K to the wheat crop in substantial amounts. Further, RS incorporation improved the soil physical and biological properties (Singh et al., 2005; Bera et al., 2018; Saikia et al., 2019). A significant increase in rice grain yield was observed because the decomposition of RS supplements an additional N and P to the soil (Yadvinder-Singh, 2014). The positive effect of RS incorporation on crop grain yield was attributed to improvement in soil fertility and soil properties (Huang et al., 2013; Zhang et al., 2015; Zhu et al., 2015). In contrast, a negative impact on wheat yield has been observed due to microbial N immobilization following RS incorporation (Huang et al., 2013). However, such effects on crop yield were not observed in this study. It could be ascribed to the fact that wheat sowing commence after about 3 weeks of RS incorporation, and therefore escapes the chances of the negative impacts on crop yield caused by N immobilization.
Effects of Fertilizer-N Application and Straw Incorporation on SOC Pools
This study revealed that variable rates of fertilizer-N application and RS incorporation significantly increased C pools viz. TOC, WEOC, HWC, KMnO4-C, compared with the CK. The magnitude of this increase varied with the fertilizer-N application and RS incorporation rates. A significant increase in TOC concentrations with RS incorporation could be ascribed to shoot C (4.92–9.75 Mg C ha−1 year−1), root C (0.97–1.46 Mg C ha−1 yr−1), rhizodeposition (1.94–3.18 Mg C ha−1 yr−1) input into the soil. An increase in TOC concentration due to above-and below-ground C input has been reported in several other studies (e.g., Wang et al., 2015b; Singh and Benbi, 2018a). Nonetheless, increased crop yield under RS incorporation treatments could enhance soil C inputs due to rhizodeposition and root C (Xu et al., 2011). Addition of straw together with fertilizer-N resulted in higher increase in TOC, compared with addition of straw alone (Kirkby et al., 2013). This is due to the fact that C use efficiency of soil microbes increased under optimal N condition with balanced use of other nutrients and mining of labile C pool by microbes with increased residues C in in-situ straw retained in the field (Fontaine et al., 2004; Murphy et al., 2015; Poeplau et al., 2015). It has been observed that crop residue derived C retention in soil slightly decreased with increased fertilizer-N application rates (Kirkby et al., 2014), which accelerates C sequestration upon fertilizer-N application.
Increase in TOC concentrations was higher in the surface than the sub-surface layer. It might be due to the fact that depth of rotary tillage or rotavator was confined to top 10–15 cm, resulting in the accumulation and distribution of straw C in the surface soil layers. Besides, the root biomass density values of rice and wheat were significantly higher in the upper 0–15 cm soil depth, compared with lower soil depth (Singh, 2016). The C sequestration in the lower layers in soil profile has been related to higher C rhizodeposition through deep rooting system of crops such as sorghum and Congo grass (Séguy et al., 2006). Since in the present study, the tillage depth was confined to the upper 15 cm soil depth, higher TOC pool was concentrated in the surface soil layer due to RS incorporation. In addition, in the present study assured irrigation was ensured, therefore roots tend to proliferate in the upper soil layers (Singh, 2016). Due to decreased nutrients' concentration and low microbial activity reduces C turnover and rooting depth in the lower soil layers (Ingram and Fernandes, 2001).
The labile C pools are sensitive indicators for assessing management induced changes in TOC pool, because they respond promptly in short-term dynamics (Benbi et al., 2015; Singh and Benbi, 2018a). It is widely accepted that RS could increase the labile C pools in the surface soil (Yan et al., 2007; Wang et al., 2015a). Accumulation of rhizodeposition by root biomass enhances net accumulation of HWC in soil (Ghani et al., 2003). The higher concentration of HWC than WEOC in the present study was due to its higher biodegradable composition (Gregorich et al., 2003). The KMnO4-C, WEOC, and HWC are considered important C and energy sources for soil microorganisms (Benbi et al., 2015), and are mainly composed of un-decomposed plant, animal residue and microbial debris. Much soluble organic matter, such as starch, protein, and mono-saccharides are released into the soil during decomposition of crop straw (Chantigny, 2003), which increased the labile C pool under the RS incorporation treatments.
Effects of Fertilizer-N Application and Straw Incorporation on Microbial Quotients
In our study, microbial quotients viz. BSR, qCO2 and qmic were higher under RS10.0N120, compared with CK. The increased qCO2 values indicate decreased transformation efficiency due to low energy of microbial population (Dilly and Munch, 1996). Increased qCO2 value reflects the nutritional constraint because C substrates become less degradable (Trumbore, 2000). This increase in qCO2 values under RS incorporation treatments showed the change in structure of microbial community (Grayston et al., 2001), because of bacterial population had the lower C biomass than the fungi, but had much higher respiration (Anderson and Domsch, 1975). The higher qCO2 values indicate the younger age of existing microbial community (Anderson and Domsch, 1990). Crop residue incorporation has been the essential component with key multi-functional roles in soil biological properties of soil (Smith et al., 2000; Bhaduri et al., 2017). Crop residues and rhizodeposition, root and shoot C are nutrient and energy sources for microbial activity and thus straw incorporation improves soil physical environment for microbial growth (Wang et al., 2015b). Therefore, soil MBC concentration was increased under RS incorporation treatment. The RS10.0N120 treatment showed the highest improvement in TOC concentration, which indicated availability of additional substrate resulted in enhanced organic C accumulation and microbial C use efficiency (Ye et al., 2014). Increased MBC due to residue incorporation has been related to the addition of hydrolysable and decomposable C substrate stimulating microbial activity in the soil (Kaur et al., 2008). The respiration quotient has valuable application as a relative measure of how efficiently the soil microbial biomass utilizes C resources and the degree of substrate limitation for soil microbes (Dilly and Munch, 1998). As a result, efficient microbes had lower metabolic quotient under RS10.0. Thus, RS served as an important C source for microorganisms especially in low fertility soils. An increase of microbial C per unit soil C has been due to RS incorporation (Powlson et al., 1987). The decomposition of plant residues to the field is therefore, important to maintain and improve soil fertility and microbial activity. In both the layers, MBC, BSR and mineralization quotients were lowest in CK, compared with RS incorporation treatments because of decreased availability of substrate C across time and poor root exudation, root water uptake and respiration in no-straw treatments (Li et al., 2012).
Effects of Fertilizer-N Application and Straw Incorporation on Carbon Management Index
The CMI is derived from the TOC pool and KMnO4-C, and is useful index for quantitative evaluation of the capacity of management systems to promote soil quality (Blair et al., 1995). The CMI was developed to determine the rates of soil TOC change in response to management practices (Blair et al., 1995), and is also a useful criteria to assess soil fertility (Xu et al., 2011). It compares the changes that occur in TOC and KMnO4-C in response to varying agricultural management practices (Singh and Benbi, 2018a). Higher CMI indicates that the soil can supply more nutrients for crop growth (Wang et al., 2015b). A management system is considered sustainable, if the value of CMI is >100. Thus, the higher is the CMI value, the higher is the soil quality. The CMI was well above this value, and was improved with RS incorporation indicating C rehabilitation in surface and sub-surface soil layers. An improvement in CMI values for the treatments involving integration of RS incorporation and fertilizer-N could be ascribed to the supplementation of above-and below-ground biomass C. Higher CMI values for surface than the sub-surface soil layer, regardless of the differential rate of fertilizer-N application has been associated to the higher concentration of TOC and KMnO4-C in 0–7.5 cm soil layer. An increased CMI values were associated to a significant modification in labile C pool and the substrate (residues) quality (Tirol-Padre and Ladha, 2004; Singh and Benbi, 2018a).
Effects of Fertilizer-N Application and Straw Incorporation on Soil Enzyme Activity
Crop residue incorporation into soil rapidly stimulates labile C and MBC for micro-organism turnover and nutrient supply (De Gryze et al., 2005). Immediately after adding C substrate to soil, microbial population starts utilizing freshly added organic matter and tends intensifying SOM decomposition (Na et al., 2014). Our results showed that RS incorporation (RS10.0) resulted in increased enzyme activities (DHA, FDA, and Alk-P), which was linked to the increased C input, root exudates containing C rich substrate such as organic acids and carbohydrates (Sharma et al., 2019a,b). It could be ascribed to the fact that immediately after addition of fresh organic C substrate to soil, microbes came out of starvation state, became active and start multiplying at much faster rate (Blagodatskaya et al., 2009).
Soil organic C not only represents a source of enzyme production but also a substrate for enzyme degradation, and thus impacts the enzyme activities in soil (Hok et al., 2018). Enzyme activities of soils are generally correlated with the TOC (Taylor et al., 2002). After 5 years of crop residue incorporation in soil, Wei et al. (2015) reported increased phosphatase, urease and invertase activity in soil, which directly related to SOM content. Extracellular enzymes are unevenly distributed in the soil and are sensitive to environmental changes (Nannipieri et al., 2002). The use of manures and/or crop residues enhances soil microbial biomass, microbial activity and their diversity (Bera et al., 2017). Crop residue incorporation increased microbial population and MBC and provide energy and conducive environment for accumulation of soil enzymes (Jiao et al., 2011). Change in enzyme activities influenced by RS incorporation could be ascribed to C input and nutrient availability in soil (Saikia et al., 2019). Due to crop residue retention under no-tillage system in corn-soybean cropping system, Green et al. (2007) reported 82% higher β-glucosidase, compared with under disk plow tillage system.
Principal Component Analysis
In this study, the weights of selected soil properties derived after dividing the percent variation in each PC to that of percent cumulative variation for all the selected PCs. The most influential variables for were KMnO4-C for PC1, FDA for PC2, and BSR for PC3 based on eigen vector weight value or factor loading. These three variables could therefore, be considered as potential indicators of soil quality under RS incorporation and fertilizer-N application treatments. TheKMnO4-C was the most important and pragmatic soil quality indicator appeared as important energy source for microorganisms and promotes biological activity in the soil. Therefore, it could be used as sensitive indicator for detecting soil management induced changes in C cycling (Dhaliwal et al., 2017). Among three variables viz. FDA, Alk-P, qmic qualified in PC2, FDA has been the indicator of soil's overall biological activity which was correlated with microbial biomass, adenosine triphosphate (ATP) content, and cell density (Federle et al., 1990). In PC2, BSR was the potential indicator for soil quality because it has been strongly influenced by management induced changes and system perturbations (Smith and Paul, 1990). It provides an indication of soil's ability to store and recycle nutrients and energy (Smith and Paul, 1990). It serves as a sensitive indicator of change and in SOM and equilibrium (Gregorich et al., 1994).
Relationship Among Different Soil Quality Attributes
Highly significant linear relationship among TOC, WEOC, HWC, KMnO4-C and soil enzymes (DHA and FDA) has been reported in several other studies (Lou et al., 2011; Li et al., 2012; Yang et al., 2012; Wang et al., 2015a). There are reports that KMnO4 stimulates rapid oxidation of microbial metabolites and reflects the soil enzymatic activity for the decomposition of labile organic C (Loginow et al., 1987; Weil et al., 2003). Evidences had shown that initial stage degradation products of cellulose comprised between 11 and 19% of dissolved organic matter (Steffen et al., 2000), which during the later stages gets assimilated in microbial biomass that eventually gets transformed to SOM (Cotrufo et al., 2015). Therefore, the strong relationship between the labile C pool and soil enzymatic activity was observed in the present study. The MBC exhibited significantly higher linear relationship with WEOC, HWC, and KMnO4-C pools. A linear relationship between MBC and HWC was due to the fact that hot water also extracts a massive microbial metabolites and related decomposable products (Landgraf et al., 2006). Other researchers have suggested that higher sensitivity of C fractions of varying oxidizability (Chan et al., 2001), WEOC (Bolinder et al., 1999) and MBC (Tian et al., 2015) were directly related to net primary production through different soil management practices. Relative to other pools (KMnO4-C, WEOC, and HWC), MBC had the lowest sensitivity, because of its more labile nature and smaller size (Janzen et al., 1992).
Conclusions
Unlike RS incorporation alone, crop yields were highly responsive to N application, showing ~10.8% higher yields in rice and ~2.6 times higher yields for wheat compared to the control. After 6-cycle of RWCS, fertilizer-N application and RS incorporation significantly increased TOC, its labile C pools and soil enzymes compared with the CK. Among the labile pools; KMnO4-C, WEOC, and BRS were the most sensitive indicators for assessing changes in TOC in the surface soil. The change in labile C pool in soil was related to change in soil enzymatic activity. The CMI was suitable for use as a sensitive indicator for assessing TOC changes in a RWCS. The TOC exhibited a linear increase with above-and below-ground plant biomass C. These results indicate that straw incorporation is a suitable management practice or improving surface soil TOC sequestration, although its effect on crop yield need to be verified under long-term evaluation in a RWCS.
Data Availability Statement
The original contributions presented in the study are included in the article/Supplementary Material, further inquiries can be directed to the corresponding author/s.
Author Contributions
SS was involved in conducted field experiment and soil analysis. PS was involved in data analysis. SK was involved in editing of research paper. All authors contributed to the article and approved the submitted version.
Conflict of Interest
The authors declare that the research was conducted in the absence of any commercial or financial relationships that could be construed as a potential conflict of interest.
Acknowledgments
We thank the Head, Department of Soil Science, Punjab Agricultural University, Ludhiana (India) for providing the financial assistance for necessary laboratory and field facilities. This research did not receive any specific funding.
Supplementary Material
The Supplementary Material for this article can be found online at: https://www.frontiersin.org/articles/10.3389/fsufs.2020.532704/full#supplementary-material
References
Anderson, J. P. E., and Domsch, K. H. (1975). Measurement of bacterial and fungal contributions to respirations of selected agricultural and forest soils. Can. J. Microbiol. 31, 314–321. doi: 10.1139/m75-045
Anderson, T. H., and Domsch, K. H. (1990). Application of eco-physiological quotients (qCO2 and qD) on microbial biomass from soils of different cropping histories. Soil Bio. Biochem. 22, 251–255. doi: 10.1016/0038-0717(90)90094-G
Baldrian, P., and Valášková, V. (2008). Degradation of cellulose by basidiomycetous fungi. FEMS Microbiol. Rev. 32, 501–521. doi: 10.1111/j.1574-6976.2008.00106.x
Balota, E. L., Kanashiro, M., Colozzi-Filho, A., Andrade, D. S., and Dick, R. P. (2004). Soil enzymeactivities under long-term tillage and crop rotation systems in subtropical agro-ecosystems. Braz. J. Microbio. 35, 300–306. doi: 10.1590/S1517-83822004000300006
Banger, K., Toor, G. S., Biswas, A., Sidhu, S. S., and Sudhir, K. (2010). Soil organic carbon fractions after 16-years of applications of fertilizers and organic manure in a typic rhodalfs in semi-arid tropics. Nutrient Cycly. Agroecosys. 86, 391–399. doi: 10.1007/s10705-009-9301-8
Benbi, D. K., Brar, K., Toor, A. S., and Sharma, S. (2015). Sensitivity of labile soil organic carbon pools to long-term fertilizer, straw and manure management in rice-wheat system. Pedosphere 25, 534–545. doi: 10.1016/S1002-0160(15)30034-5
Benbi, D. K., Singh, P., Toor, A. S., and Verma, G. (2016). Manure and fertilizer application effects on aggregate and mineral-associated organic carbon in a loamy soil under rice-wheat system. Comm. Soil Sci. Plant Anal. 47, 1828–1844. doi: 10.1080/00103624.2016.1208757
Bera, T., Sharma, S., Thind, H. S., Singh, Y., Sidhu, H. S., and Jat, M. L. (2017). Soil biochemical changes at different wheat growth stages in response to conservation agriculture practices in rice-wheat system of north-western India. Soil Res. 56, 91–104. doi: 10.1071/SR16357
Bera, T., Sharma, S., Thind, H. S., Singh, Y., Sidhu, H. S., and Jat, M. L. (2018). Changes in soil biochemical indicators at different wheat growth stages under conservation based sustainable intensification agriculture practices of rice-wheat system. J. Integ. Agricul. 17, 1871–1880. doi: 10.1016/S2095-3119(17)61835-5
Beri, V., Sidhu, B. S., Bahl, G. S., and Bhat, A. K. (1995). Nitrogen and phosphorus transformations as affected by crop residue and their influence on crop yield. Soil Use Manage. 11, 51–54. doi: 10.1111/j.1475-2743.1995.tb00496.x
Beri, V., Sidhu, B. S., Gupta, A. P., Tiwari, R. C., Pareek, R. P., Rupela, O. P., et al. (2003). Organic Resources of a Part of Indo-Gangetic Plain and their Utilization: Department of Soils. Punjab Agricultural University: Ludhiana, India. 93p.
Bhaduri, D., Purakayastha, T. J., Patra, A. K., Singh, M., and Wilson, B. R. (2017). Biological indicators of soil quality in a long-term rice–wheat system on the indo-gangetic plain: combined effect of tillage–water–nutrient management. Envir. Earth Sci. 76:202. doi: 10.1007/s12665-017-6513-0
Blagodatskaya, E. V., Blagodatsky, S. A., Anderson, T. H., and Kuzyakov, Y. (2009). Contrasting effects of glucose, living roots and maize straw on microbial growth kinetics and substrate availability in soil. Euro. J. Soil Sci. 60, 186–197. doi: 10.1111/j.1365-2389.2008.01103.x
Blair, G., Lefroy, R. B. D., and Lisle, L. (1995). Soil carbon fractions based on their degree of oxidation, and the development of a carbon management index for agricultural systems. Aus. J. Agri. Res. 46, 1459–1466. doi: 10.1071/AR9951459
Bolinder, M. A., Angers, D. A., Gregorich, E. G., and Carter, M. R. (1999). The response of soil quality indicators to conservation management. Can. J. Soil Sci.79, 37–45. doi: 10.4141/S97-099
Brar, B. S., Singh, K., and Dheri, G. S. (2013). Carbon sequestration and soil carbon pools in a rice–wheat cropping system: effect of long–term use of inorganic fertilizers and organic manure. Soil Till. Res. 128, 30–36. doi: 10.1016/j.still.2012.10.001
Bronson, K. F., Cassman, K. G., Wassman, R., Olk, D. C., Noordwijk, M., and van Garrity, D. P. (1998). “Soil carbon dynamics in different cropping systems in principal eco-regions of Asia,” in Management of Carbon Sequestration in Soil, eds. R. Lal, J. M. Kimble, R. F. Follett, B. A. Stewart (CRC, Boca Raton: NY), 35–57.
Chan, K. Y., Bowman, A., and Oates, A. (2001). Oxidizable organic carbon fractions and soil quality changes in an OxicPaleustalf under different pasture clays. Soil Sci. 166, 61–67. doi: 10.1097/00010694-200101000-00009
Chantigny, M. H. (2003). Dissolved and water-extractable organic matter in soils: a review on the influence of land use and management practices. Geoderma 113, 357–380. doi: 10.1016/S0016-7061(02)00370-1
Chauhan, B. S., Mahajan, G., Sardana, V., Timsina, J., and Jat, M. L. (2012). Productivity and sustainability of the rice-wheat cropping system in the indo-gangetic plains of the Indian subcontinent: problems, opportunities, and strategies. Adv. Agron. 117, 316–355. doi: 10.1016/B978-0-12-394278-4.00006-4
Chen, S., Xu, C. M., Yan, J. X., Zhang, X. G., Zhang, X. F., and Wang, D. Y. (2016). The influence of the type of crop residue on soilorganic carbon fractions: an 11-year field study of ricebasedcropping systems in southeast China. Agri. Ecosys. Envir. 223, 261–269. doi: 10.1016/j.agee.2016.03.009
Choudhary, M., Datta, A., Jat, H. S., Yadav, A. K., Gathala, M. K., Sapkota, T. B., et al. (2018). Changes in soil biology under conservation agriculture based sustainable intensification of cereal systems in indo-gangetic Plains. Geoderma 313, 193–204. doi: 10.1016/j.geoderma.2017.10.041
Cotrufo, M. F., Soong, J. L., Horton, A. J., Campbell, E. E., Haddix, M. L., Wall, D. H., et al. (2015). Formation of soil organic matter via biochemical and physical pathways of litter mass loss. Nati. Geosci. 8, 776–779. doi: 10.1038/ngeo2520
De Gryze, S., Six, J., Brits, C., and Merckx, R. (2005). A quantification of short-term macro-aggregate dynamics: influences of wheat residue input and texture. Soil Bio. Biochem. 37, 55–66. doi: 10.1016/j.soilbio.2004.07.024
Denef, K., Plante, A. F., and Six, J. (2009). “Characterization of soil organic matter,” in Soil Carbon Dynamics: An Integrated Methodology, eds W. L. Kutsch, M. Bahn, A. Heinemeyer (Cambridge University Press: New York), 91–126. doi: 10.1017/CBO9780511711794.007
Denef, K., Six, J., Paustian, K., and Merckx, R. (2001). Importance of macro-aggregate dynamics in controlling soil carbon stabilization: short term effects of physical disturbance induced by dry–wet cycles. Soil Bio. Biochem. 33, 2145–2153. doi: 10.1016/S0038-0717(01)00153-5
Dhaliwal, J., Kukal, S. S., and Sharma, S. (2017). Soil organic carbon stock in relation to aggregate size and stability under tree-based cropping systems in typic ustochrepts. Agrofor. Syst. 92, 275–284. doi: 10.1007/s10457-017-0103-8
Dilly, O., and Munch, J. C. (1996). Microbial biomass content, basal respiration and enzyme activities during the course of decomposition of leaf litter in a black alder (Alnusglutinosa(L.) Gaertn.) forest. Soil Bio. Biochem. 28, 1073–1081. doi: 10.1016/0038-0717(96)00075-2
Dilly, O., and Munch, J. C. (1998). Ratios between estimates of microbial biomass content and microbial activity in soils. Biol. Fertil. Soils 27, 374–379. doi: 10.1007/s003740050446
Federle, M. L., Ventullo, R. M., and White, D. C. (1990). Spatial distribution of microbial biomass, activity, community structure, and the biodegradation of linear alkyl benzene sulfonate (LAS) and linear alcohol ethoxylate (LAE) in the subsurface. Micro. Ecol. 20, 297–313. doi: 10.1007/BF02543885
Fontaine, S., Bardoux, G., Abbadie, L., and Mariotti, A. (2004). Carbon input to soil may decrease soil carbon content. Ecol. Lett. 7, 314–320. doi: 10.1111/j.1461-0248.2004.00579.x
Garg, S., and Bahl, G. (2008). Phosphorus availability to maize as influenced by organic manures and fertilizer P associated phosphatase activity in soils. Biores. Tech. 99, 5773–5777. doi: 10.1016/j.biortech.2007.10.063
Ghani, A., Dexter, M., and Perrott, K. W. (2003). Hot-water extractable carbon in soils: a sensitive measurement for determining impacts of fertilisation, grazing and cultivation. Soil Bio. Biochem. 35, 1231–1243. doi: 10.1016/S0038-0717(03)00186-X
Ghosh, P. K., Hazra, K. K., Venkatesh, M. S., Singh, K. K., Kumar, N., and Mathur, R. S. (2016). Potential of crop residue and fertilizer on enrichment of carbon pools in upland soils of subtropical India. Agriculture 5, 261–268. doi: 10.1007/s40003-016-0215-9
Grayston, S. J., Griffith, G. S., Mawdsley, J. L., Campbell, C. D., and Bardgett, R. D. (2001). Accounting for variability in soil microbial communities of temperate upland grassland ecosystems. Soil Bio. Biochem. 33, 533–551. doi: 10.1016/S0038-0717(00)00194-2
Green, V. S., Stott, D. E., Cruz, J. C., and Curi, N. (2007). Tillage impacts on soil biological activity and aggregation in a Brazilian Cerrado Oxisol. Soil Till. Res. 92, 114–121. doi: 10.1016/j.still.2006.01.004
Gregorich, E. G., Beare, M. H., Stoklas, U., and St Georges, P. (2003). Biodegradability of soluble organic matter in maize-cropped soils. Geoderma 113, 237–252. doi: 10.1016/S0016-7061(02)00363-4
Gregorich, E. G., Monreal, C. M., Carter, M. R., Angers, D. A., and Ellert, B. H. (1994). Towards a minimum data set to assess soil organic matter quality in agricultural soils. Can. J. Soil Sci. 74, 367–385. doi: 10.4141/cjss94-051
Hadas, A., Kautsky, L., Goek, M., and Kara, E. E. (2004). Rates of decomposition of plant residues and available nitrogen in soil, related to residue composition through simulation of carbon and nitrogen turnover. Soil Bio. Biochem. 36, 255–266. doi: 10.1016/j.soilbio.2003.09.012
Haichar, F. E. Z., Achouak, W., Christen, R., Heulin, T., Marol, C., Marais, M. -F., et al. (2007). Identification of cellulolytic bacteria in soil by stable isotope probing. Environ. Microbiol. 9, 625–634. doi: 10.1111/j.1462-2920.2006.01182.x
Hazra, K. K., Venkatesh, M. S., Ghosh, P. K., Ganeshamurthy, A. N., Kumar, N., Nadarajan, N., et al. (2014). Long–term effect of pulse crops inclusion on soil–plant nutrient dynamics in puddled rice (Oryza sativa L.)–wheat (Triticum aestivum L.) cropping system on an Inceptisol of indo–gangetic plain zone of India. Nut. Cycl. Agroecosys. 100, 95–110. doi: 10.1007/s10705-014-9629-6
Higuchi, T. (2004). Microbial degradation of lignin: role of lignin peroxidase, manganese peroxidase, and laccase. Pro. Jpn Aca. Ser. B Phy. Bio. Sci. 80, 204–214. doi: 10.2183/pjab.80.204
Hira, G. S., Jalota, S. K., and Arora, V. K. (2004). Efficient Management of Water Resources for Sustainable Cropping in Punjab. Department of Soils. Punjab Agricultural University: Ludhiana.
Hok, L., de MoraesSá, J. C., Reyes, M., Boulakia, S., Tivet, F., Leng, V., et al. (2018). Enzymes and C pools as indicators of C build up in short-term conservation agriculture in a savanna ecosystem in Cambodia. Soil Till. Res.177, 125–133. doi: 10.1016/j.still.2017.11.015
Huang, S., Zeng, Y. J., Wu, J. F., Shi, Q. H., and Pan, X. H. (2013). Effect of crop residue retention on rice yield in China: a meta-analysis. Field Crops Res. 154, 188–194. doi: 10.1016/j.fcr.2013.08.013
Ingram, J. S. I., and Fernandes, E. C. M. (2001). Managing carbon sequestration in soils: concept and terminology. Agri. Ecosy. Envir. 87, 111–117. doi: 10.1016/S0167-8809(01)00145-1
IRRI (International Rice Research Institute). (2000). IRRISTAT for Window (CD-ROM). ver. 4.02b. Los Banos: International Rice Research Institute.
Janzen, H. H., Campbell, C. A., Brandt, S. A., Lafond, G. P., and Townley-Smith, L. (1992). Light-fraction organic matter in soils from long-term crop rotations. Soil Sci. Soc. Am. J. 56, 1799–1806. doi: 10.2136/sssaj1992.03615995005600060025x
Jiao, X. G., Gao, C. S., Lü, G. H., and Sui, Y. Y. (2011). Effect of long-term fertilization on soil enzyme activities under different hydrothermal conditions in Northeast China. Agri. Sci. China 10, 412–422. doi: 10.1016/S1671-2927(11)60020-5
Jin, K., Sleutel, S., Buchan, D., De Neve, S., Cai, D. X., Gabriels, D., et al. (2009). Changes of soil enzyme activities under different tillage practices in the Chinese Loess Plateau. Soil Till. Res. 104, 115–120. doi: 10.1016/j.still.2009.02.004
Kaur, T., Brar, B. S., and Dhillon, N. S. (2008). Soil organic matter dynamics as affected by long-term use of organic and inorganic fertilizers under maize–wheat cropping system. Nut. Cycl. Agroecosys. 81, 59–69. doi: 10.1007/s10705-007-9152-0
Kirkby, C. A., Richardson, A. E., Wade, L. J., Batten, G. D., Blanchard, C., and Kirkegaard, J. A. (2013). Carbon-nutrient stoichiometry to increase soil carbon sequestration. Soil Bio. Biochem. 60, 77–86. doi: 10.1016/j.soilbio.2013.01.011
Kirkby, C. A., Richardson, A. E., Wade, L. J., Passioura, J. B., Batten, G. D., Blanchard, C., et al. (2014). Nutrient availability limits carbon sequestration in arable soils. Soil Bio. Biochem. 68, 402–409. doi: 10.1016/j.soilbio.2013.09.032
Kögel-Knabner, I., Amelung, W., Cao, Z., Fiedler S,Frenzel, P., Jahn R,Kalbitz, K., Kölbl, A., et al. (2010). Biogeochemistry of paddy soils. Geoderma 157, 1–14. doi: 10.1016/j.geoderma.2010.03.009
Ladha, J. K., Singh, Y., Erenstein, O., and Hardy, B. (2009). Integrated Crop and Resource Management in the Rice–Wheat System of South Asia. International Rice Research Institute: Los Banos, Philippines. 395p.
Lal, R. (2004). Agricultural activities and the global carbon cycle. Nut. Cycl. Agroecosy. 70, 103–116. doi: 10.1023/B:FRES.0000048480.24274.0f
Landgraf, D. P., Leinweber, F., and Makeschin, F. (2006). Cold and hot waterextractable organic matter as indicators of litter decomposition in forest soils. J. Plant Nut. Soil Sci. 169, 76–82. doi: 10.1002/jpln.200521711
Li, D., Niu, S., and Luo, Y. (2012). Global patterns of the dynamics of soil carbon and nitrogen stocks following afforestation: a meta analysis. New Phyt. 19, 172–181. doi: 10.1111/j.1469-8137.2012.04150.x
Li, J. M., Sun, Y. L., and Zhuang, M. F. (2005). Taiwan Carbon Dioxide Emissions Decoupling Indicators Construction and Evaluation. China Development Fund Management Committee: The Paper Collection of Cross-Strait Environmental Protection and Sustainable Development Seminar.
Lian, T., Wang, G., Yu, Z., Li, Y., Liu, X., and Jin, J. (2016). Carbon input from 13C-labelled soybean residues in particulate organic carbon fractions in a Mollisol. Bio. Fert. Soils 52, 331–339. doi: 10.1007/s00374-015-1080-6
Liu, C., Lu, M., Cui, J., Li, B., and Fang, C. (2014). Effects of straw carbon input on carbon dynamics in agricultural soils: a meta-analysis. Glob. Change Bio. 20, 1366–1381. doi: 10.1111/gcb.12517
Loginow, W., Wisniewski, W., Gonet, S. S., and Ciescinska, B. L. (1987). Fractionation of organic carbon based on susceptibility to oxidation. Polish J. Soil Sci. 20, 47–52.
Lorenz, K., and Lal, R. (2005). The depth distribution of soil organic carbon in relation to land use and management and the potential of carbon sequestration in subsoil horizons. Adv. Agron. 88, 35–66. doi: 10.1016/S0065-2113(05)88002-2
Lou, Y., Wang, J., and Liang, W. (2011). Impacts of 22-year organic and inorganic N managements on soil organic C fractions in a maize field, northeast China. Catena 87, 386–390. doi: 10.1016/j.catena.2011.07.006
Majumder, B., and Kuzyakov, Y. (2010). Effect of fertilization on decomposition of 14C labeled plant residues and their incorporation into soil aggregates. Soil Till. Res. 109, 94–102. doi: 10.1016/j.still.2010.05.003
Majumder, B., Mandal, B., Bandyopadhyay, P. K., and Chaudhury, J. (2007). Soil organic carbon pools and productivity relationships for a 34 year old rice-wheat-jute agroecosystem under different fertilizer treatments. Plant Soil 297, 53–67. doi: 10.1007/s11104-007-9319-0
Malhi, S. S., Nyborg, M., Goddard, T., and Puurveen, D. (2012). Long-term tillage, straw management, and nitrogen fertilization effects on organic matter and mineralizable carbon and nitrogen in a black chernozem soil. Comm. Soil Sci. Pl. Anal. 43, 2679–2690. doi: 10.1080/00103624.2012.711880
Mandal, K. G., Misra, A. K., Hati, K. M., Bandyopadhyay, K. K., Ghosh, P. K., and Mohanty, M (2004). Rice residue management options and effects on soil properties and crop productivity. Food Agri. Envir. 2, 224–231.
Medeiros, E. V., Duda, G. P., Rodrigues dos Santos, L. A., de Sousa Lima, J. R., Almeida-Cortêz, J., Sd Hammecker, C., et al. (2017). Soil organic carbon, microbial biomass and enzyme activities responses to natural regeneration in a tropical dry region in Northeast Brazil. Catena 151, 137–146. doi: 10.1016/j.catena.2016.12.012
Mohammadi, K. (2011). Soil microbial activity and biomass as influenced by tillage and fertilization in wheat production. Am. Eur. J. Agri. Envir. Sci. 10, 330–337.
Murphy, C. J., Baggs, E. M., Morley, N., Wall, D. P., and Paterson, E. (2015). Rhizosphere priming can promote mobilisation of N-rich compounds from soil organic matter. Soil Bio. Biochem. 81, 236–243. doi: 10.1016/j.soilbio.2014.11.027
Na, Q., Schaefer, D., Blagodatskaya, E., Zou, X. M., Xu, X. L., and Kuzyakov, Y. (2014). Labile carbon retention compensates for CO2 released by priming in forest soils. Glob. Change Biol. 20, 1943–1954. doi: 10.1111/gcb.12458
NAAS (2017). Innovative Viable Solution to Rice Residue Burning in Rice-Wheat Cropping System Through Concurrent Use of Super Straw Management System-Fitted Combines and Turbo Happy Seeder. Policy Brief No. 2. New Delhi: National Academy of Agricultural Sciences. 1–16.
Nannipieri, P., Kandeler, E., and Ruggiero, P. (2002). “Enzyme activities and microbiological and biochemical processes in soil,” in Enzymes in the Environment: Activity, Ecology and Applications, eds R. G. Burns and R. P. Dick (New York: Marcel Dekker), 1–33. doi: 10.1201/9780203904039.ch1
Parihar, C. M., Jat, S. L., Singh, A. K., Datta, A., Parihar, M. D., Varghese, E., et al. (2018). Changes in carbon pools and biological activities of a sandy loam soil under medium-term conservation agriculture and diversified cropping systems. Euro. J. Soil Sci. 69, 902–912. doi: 10.1111/ejss.12680
Poeplau, C., Kätterer, T., Bolinder, M. A., Börjesson, G., Berti, A., and Lugato, E. (2015). Low stabilization of aboveground crop residue carbon in sandy soils of Swedish long-term experiments. Geoderma 237–238, 246–255. doi: 10.1016/j.geoderma.2014.09.010
Powlson, D. S., Brookes, P. C., and Christensen, B. T. (1987). Measurement of soil microbial biomass provides an early indication of changes in total soil organic matter due to straw incorporation. Soil Bio. Biochem. 19, 159–164. doi: 10.1016/0038-0717(87)90076-9
Rabary, B., Sall, S., Letourmy, P., Husson, O., Ralambofetra, E., Moussa, N., et al. (2008). Effects of living mulches or residue amendments on soil microbial properties in direct seeded cropping systems of Madagascar. App. Soil Ecol. 39, 236–243. doi: 10.1016/j.apsoil.2007.12.012
Ryan, M. C., and Aravena, R. (1994). Combining 13C natural abundance and fumigation-extraction methods to investigate soil microbial biomass turnover. Soil Bio. Biochem. 26, 1583–1585. doi: 10.1016/0038-0717(94)90101-5
Saha, B. C., Iten, L. B., Cotta, M. A., and Wu, Y. V. (2005). Dilute acid pre-treatment, enzymatic saccharification and fermentation of wheat straw to ethanol. Pro. Biochem. 40, 3693–3700. doi: 10.1016/j.procbio.2005.04.006
Saharawat, Y. S., Ladha, J. K., Pathak, H., Gathala, M., Chaudhary, N., and Jat, M. L. (2012). Simulation of resource-conserving technologies on productivity, income and greenhouse gas GHG emission in rice-wheat system. J. Soil Sci. Envir. Manage. 3, 9–22. doi: 10.5897/JSSEM11.108
Saikia, R., Sharma, S., Thind, H. S., Sidhu, H. S., and Singh, Y. (2019). Temporal changes in biochemical indicators of soil quality in response to tillage, crop residue and green manure management in a rice-wheat system. Ecol. Ind. 103, 383–394. doi: 10.1016/j.ecolind.2019.04.035
Séguy, L., Bouzinac, S., and Husson, O. (2006). “Direct-seeded tropical soil systems with permanent soil cover: learning from Brazilian experience,” in Biologicalapproach to Sustainable Soil Systems, eds. N. Uphoff, A. S. Ball, E. Fernandes, H. Herren, O. Husson, M. Laing, et al. (CRC Press, Taylor and Francis), 326–346. doi: 10.1201/9781420017113.ch22
Shang, Q. Y., Yang, X. X., Gao, C. M., Wu, P. P., Liu, J. J., Xu, Y. C., et al. (2011). Net annual global warming potential and greenhouse gas intensity in Chinese double rice-cropping systems: a 3-year field measurement in long-term fertilizer experiments. Glob. Change Bio. 17, 2196–2210. doi: 10.1111/j.1365-2486.2010.02374.x
Sharma, S., Monika, V., and Yadvinder-Singh Thind, H. S. (2019b). Soil carbon pools and enzyme activities in aggregate size fractions after seven years of conservation agriculture in a rice–wheat system. Crop Pas. Sci. 70, 473–485. doi: 10.1071/CP19013
Sharma, S., Thind, H. S., Yadvinder-Singh Sidhu, H. S., Jat, M. L., and Parihar, C. M. (2019a). Effects of crop residue retention on soil carbon pools after 6 years of rice–wheat cropping system. Envir. Earth Sci. 78:296. doi: 10.1007/s12665-019-8305-1
Sidhu, B. S., and Beri, V. (1989). Effect of crop residue management on the yields of different crops and on soil properties. Bio. Wastes 27, 15–27. doi: 10.1016/0269-7483(89)90027-X
Singh, P. (2016). Organic carbon dynamics in soils under different cropping sequences and nutrient management practices. (Ph.D. Dissertation). Department of Soil Science, Punjab Agricultural University: Ludhiana, Punjab, India. 1–220.
Singh, P., and Benbi, D. K. (2016). Effect of inorganic fertilizers and farm yard manure on physical properties of soil under rice-wheat cropping. Agri. Res. J. 53, 328–333. doi: 10.5958/2395-146X.2016.00064.8
Singh, P., and Benbi, D. K. (2018a). Nutrient management effects on organic carbon pools in a sandy loam soil under rice0wheat cropping. Arc. Agron. Soil Sci. 64, 1879–1891. doi: 10.1080/03650340.2018.1465564
Singh, P., and Benbi, D. K. (2018b). Soil organic pool changes in relation to slope position and land-use in Indian lower Himalayas. Catena 166, 171–180. doi: 10.1016/j.catena.2018.04.006
Singh, P., and Benbi, D. K. (2020a). Modeling soil organic carbon with DNDC and RothC models in different wheat-based cropping systems in north-western India. Comm. Soil Sci. Pl. Anal. 51, 1184–1203. doi: 10.1080/00103624.2020.1751850
Singh, P., and Benbi, D. K. (2020b). Nutrient management impacts on net ecosystem carbon budget and energy flow nexus in intensively cultivated cropland ecosystems of north-western India. Paddy Water Environ. 18, 697–715. doi: 10.1007/s10333-020-00812-9
Singh, P., Singh, G., and Sodhi, G. P. S. (2019a). Energy auditing and optimization approach for improving energy efficiency of rice cultivation in south-western Punjab. Energy 174, 269–279. doi: 10.1016/j.energy.2019.02.169
Singh, P., Singh, G., and Sodhi, G. P. S. (2019b). Applying DEA optimization approach for energy auditing in wheat cultivation under rice-wheat and cotton-wheat cropping systems in north-western India. Energy 181, 18–28. doi: 10.1016/j.energy.2019.05.147
Singh, P., Singh, G., and Sodhi, G. P. S. (2020). Energy and carbon footprints of wheat establishment following different rice residue management strategies vis-à-vis conventional tillage coupled with rice residue burning in north-western India. Energy 200:117554. doi: 10.1016/j.energy.2020.117554
Singh, Y., Singh, B., and Timsina, J. (2005). Crop residue management for nutrient cycling and improveing soil productivity in rice-based cropping systems in the tropics. Adv. Agron. 85, 269–407. doi: 10.1016/S0065-2113(04)85006-5
Smith, J. L., and Paul, E. A. (1990). “The significance of soil microbial biomass estimations,” in Soil Biochemistry Vol. 6, eds. J. M. Bollag and G. Stotzky (Marcel Dekker: New York), 357–396.
Smith, K. A., Dobbie, K. E., Ball, B. C., Bakken, L. R., Sitaula, B. K., Hansen, S. R., et al. (2000). Oxidation of atmospheric methane in Northern European soils, comparison with other ecosystems, and uncertainties in the global terrestrial sink. Glob. Change Bio. 6, 791–803. doi: 10.1046/j.1365-2486.2000.00356.x
Snyder, J. D., and Trofymow, J. A. (1984). A rapid accurate wet oxidation diffusion procedure for determining organic and inorganic carbon in pot and soil samples. Comm. Soil Sci. Pl. Anal. 15, 587–597. doi: 10.1080/00103628409367499
Srinivasan, V., Maheswarappa, H. P., and Lal, R. (2012). Long term effects of topsoil depth and amendments on particulate and non-particulate carbon fractions in a miamian soil of central ohio. Soil Till. Res. 121, 10–17. doi: 10.1016/j.still.2012.01.014
Steffen, K. T., Hofrichter, M., and Hatakka, A. (2000). Mineralisation of 14C-labelled synthetic lignin andligninolytic enzyme activities of litter-decomposing basidiomycetous fungi. App. Microbio. Biotech. 54, 819–825. doi: 10.1007/s002530000473
Taylor, J. P., Wilson, B., Mills, M. S., and Burns, R. G. (2002). Comparison of microbial numbers and enzymatic activities in surface soils and sub soils using various techniques. Soil Bio. Biochem. 34, 387–401. doi: 10.1016/S0038-0717(01)00199-7
Thevenot, M., Dignac, M. F., and Rumpel, C. (2010). Fate of lignins in soils: a review. Soil Bio. Biochem. 42, 1200–1211. doi: 10.1016/j.soilbio.2010.03.017
Thind, H. S., Sharma, S., and Yadvinder-Singh Sidhu, H. S. (2019). Rice–wheat productivity and profitability with residue, tillage and green manure management. Nut. Cycl. Agroecosys. 113, 113–125. doi: 10.1007/s10705-018-09967-8
Thomsen, I. K., and Christensen, B. T. (2004). Yields of wheat and soil carbon and nitrogen contents following long-term incorporation of barley straw and ryegrass catch crops. Soil Use Manage. 20, 432–438. doi: 10.1079/SUM2004281
Tian, G. L., Bi, Y. M., Sun, Z. J., and Zhang, L. S. (2015). Phenolic acids in the plow layer soil of strawberry fields and their effects on the occurrence of strawberry anthracnose. Euro. J. Pl. Path. 143, 581–594. doi: 10.1007/s10658-015-0711-y
Timsina, J., and Connor, D. J. (2001). The productivity and management of rice–wheat cropping systems: issues and challenges. Field Crops Res. 69, 93–132. doi: 10.1016/S0378-4290(00)00143-X
Tirol-Padre, A., and Ladha, J. K. (2004). Assessing the reliability of permanganate-oxidizable carbon as an index of soil labile carbon. Soil Sci. Soc. Am. J. 68, 969–978. doi: 10.2136/sssaj2004.9690
Trumbore, S. (2000). Age of soil organic matter and soil respiration: radiocarbon constraints on belowground C dynamics. Ecol. Appl. 10, 399–411. doi: 10.1890/1051-0761(2000)0100399:AOSOMA2.0.CO
Vaccari, F. P., Baronti, S., Lugato, E., Genesio, L., Castaldi, S., Fornasier, F., et al. (2011). Biochar as a strategy to sequester carbon and increase yield in durum wheat. Euro. J. Agron. 58, 478–486. doi: 10.1016/j.eja.2011.01.006
Wang, D., Li, H. X., Qin, J. T., Li, D. M., and Hu, F. (2010). Growth characteristics and yield of late-season rice under no-tillage and non-flooded cultivation with straw mulching. Rice Sci. 17, 141–148. doi: 10.1016/S1672-6308(08)60117-1
Wang, R., Dorodnikov, M., Yang, S., Zhang, Y., Filley, T. R., Turco, R. F., et al. (2015a). Responses of enzymatic activities within soil aggregates to 9-year nitrogen and water addition in a semi-arid grassland. Soil Bio. Biochem. 81, 159–167. doi: 10.1016/j.soilbio.2014.11.015
Wang, Y., Hu, N., Xu, M., Li, Z., Lou, Y., Chen, Y., et al. (2015b). 23-year manure and fertilizer application increases soil organic carbon sequestration of a rice–barley cropping system. Bio. Fert. Soils 51, 583–591. doi: 10.1007/s00374-015-1007-2
Wei, T., Zhang, P., Wang, K., Ding, R., Yang, B., Nie, J., et al. (2015). Effects of wheat straw incorporation on the availability of soil nutrients and enzyme activities in semiarid areas. PLoS ONE 10:e0120994. doi: 10.1371/journal.pone.0120994
Weil, R. R., Islam, K. R., Stine, M. A., Gruver, J. B., and Samson-Liebig, S. E. (2003). Estimating active carbon for soil quality assessment: a simplified method for laboratory and field use. Ame. J. Altern. Agri. 18, 3–17. doi: 10.1079/AJAA2003003
Xu, M., Lou, Y, Sun, X., Wang, W., Baniyamuddin, M., and Zhao, K. (2011). Soil organic carbon active fractions as early indicators for total carbon change under straw incorporation. Bio. Fert. Soils 47, 745–752. doi: 10.1007/s00374-011-0579-8
Yadvinder-Singh, and Sidhu, H. S. (2014). Management of cereal crop residues for sustainable rice-wheat production system in the indo-gangetic plains of India. Pro. Ind. Nat. Sci. Acad. 80, 95–114. doi: 10.16943/ptinsa/2014/v80i1/55089
Yan, D., Wang, D., and Yang, L. (2007). Long-term effect of chemical fertilizer, straw, and manure on labile organic matter fractions in a paddy soil. Bio. Fert. Soils 44, 93–101. doi: 10.1007/s00374-007-0183-0
Yang, X., Ren, W., Sun, B., and Zhang, S. (2012). Effect of contrasting soil management regimes on total and labile soil organic carbon fractions in a loess soil in China. Geoderma 177–178, 49–56. doi: 10.1016/j.geoderma.2012.01.033
Ye, X. F., Liu, H. G., Li, Z., Wang, Y., Wang, Y. Y., Wang, H. F., et al. (2014). Effects of green manure continuous application on soil microbial biomass and enzyme activity. J. Pl. Nut. 37, 498–508. doi: 10.1080/01904167.2013.867978
Zhang, X., Dong, W., Dai, X., Schaeffer, S., Yang, F., Radosevich, M., et al. (2015). Responses of absolute and specific soil enzyme activities to long term additions of organic and mineral fertilizer. Sci. Total Enviro. 536, 59–67. doi: 10.1016/j.scitotenv.2015.07.043
Zhao, H., Sun, B. F., Lu, F., Zhang, G., Wang, X. K., and Ouyang, Z. Y. (2015). Straw incorporation strategy on cereal crop yield in China. Crop Sci. 55, 1773–1781. doi: 10.2135/cropsci2014.09.0599
Keywords: total organic carbon, carbon input, enzymatic activity, straw incorporation, rice-wheat
Citation: Sharma S, Singh P and Kumar S (2020) Responses of Soil Carbon Pools, Enzymatic Activity, and Crop Yields to Nitrogen and Straw Incorporation in a Rice-Wheat Cropping System in North-Western India. Front. Sustain. Food Syst. 4:532704. doi: 10.3389/fsufs.2020.532704
Received: 19 March 2020; Accepted: 29 September 2020;
Published: 04 November 2020.
Edited by:
Elisabeth Simelton, World Agroforestry Centre, VietnamReviewed by:
Jacobo Arango, International Center for Tropical Agriculture (CIAT), ColombiaFrancisco Javier Solorio, Universidad Autónoma de Yucatán, Mexico
Copyright © 2020 Sharma, Singh and Kumar. This is an open-access article distributed under the terms of the Creative Commons Attribution License (CC BY). The use, distribution or reproduction in other forums is permitted, provided the original author(s) and the copyright owner(s) are credited and that the original publication in this journal is cited, in accordance with accepted academic practice. No use, distribution or reproduction is permitted which does not comply with these terms.
*Correspondence: Sandeep Sharma, sandyagro@pau.edu