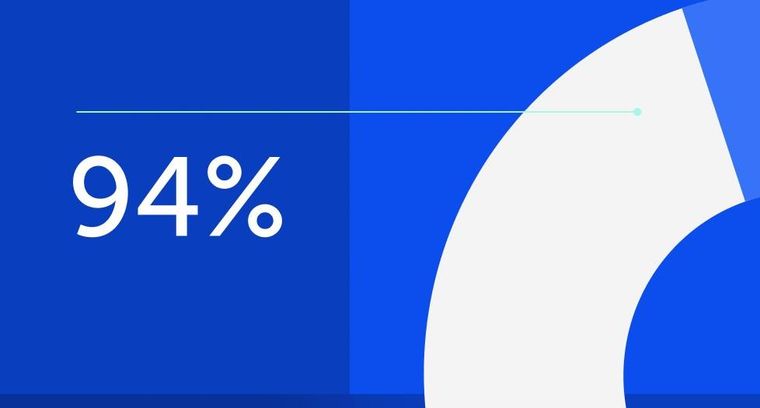
94% of researchers rate our articles as excellent or good
Learn more about the work of our research integrity team to safeguard the quality of each article we publish.
Find out more
MINI REVIEW article
Front. Sustain. Food Syst., 15 September 2020
Sec. Sustainable Food Processing
Volume 4 - 2020 | https://doi.org/10.3389/fsufs.2020.00139
This article is part of the Research TopicStructured Edible Oil: Towards a New Generation of Fat MimeticsView all 8 articles
The field of oleogel research has been active in recent decades, generating numerous oleogels with desirable properties like thermal resistance, texture, and structural stability. Moreover, several food matrices have been formulated with oleogels. In some cases, oleogels in these food products have been shown to resemble textural attributes of products made with conventional hardstock fat, to enhance dietary nutrition, to demonstrate high physical and oxidative stability, and to exhibit a high oil binding capacity. These innovations clearly illustrate the potential of the field of oleogels, but certain drawbacks and a lack of in-depth information in various aspects have delayed its commercialization in the food industry. This review aims to update the current status of the oleogel field and to cover some areas that need to be addressed to make oleogel foods a reality in the near future.
In response to nutritionists' recommendations and governmental legislations for replacing saturated and trans fats in food, the field of oil structuring has been extensively investigated in recent decades (Hughes et al., 2009; Astrup et al., 2019). In particular, oleogels have emerged as a promising potential means of replacing hardstock fats in food systems. Oleogels can be characterized as semisolid systems in which continuous liquid phases are physically immobilized by self-assembled networks of gelators (Hughes et al., 2009; Rogers et al., 2014). Under the premise of improving nutrition and the well-being of consumers, oleogels are often structured with healthy liquids oils while exhibiting acceptable solid-like behavior. Despite the recent exponential growth in the oleogel field, the use of oleogels is still in the early stages of development due to a number of challenges. These challenges include the food regulations that not only require food grade gelators, but also impose restrictions on gelator concentrations in food products. So far, various oleogels with different classes of gelators have been formulated, but not all of them are food grade. Other limitations include the lack of knowledge of and uncertainties about their interaction with other food ingredients, how they behave under different processing conditions for different food products, and the need for inexpensive and easily-accessible food grade gelators. This review will evaluate the progress in the field of oleogel and discuss other key shortcomings that need to be addressed in future work.
Due to a growing surge in enthusiasm for the development of oleogels with desirable physical characteristics, recently various forms of oleogels have been produced with different combinations of gelators and vegetable oils.
As listed in Table 1, many food products including meat products, dairy, spreads, confectionaries, and pastries have been formulated with oleogels. Detailed information about gelators utilized in edible oleogels as well as oleogelation mechanisms can be found in the references listed in Table 1 and other studies in the field. Although the substitution of saturated fat seems to be the major objective for oleogel application, these studies documented that oleogels can also offer other functionalities such as texture, thermal-reversible properties, oil binding capacity, and delivery of bioactive compounds. For instance, Patel et al. (2014a) formulated cakes with methylcellulose (MC) oleogels and documented similar hardness and chewiness to those made with shortening. In another study, emulsion-based shellac oleogels were shown to crystallize emulsion phases and water-oil interfaces, stabilizing the emulsion for 4 months (Patel et al., 2014b). In addition, cookies made with wax oleogels showed oil binding capacity above 93% with a minimum oil loss for 30 days (Fayaz et al., 2017). Stortz and Marangoni (2011) produced a heat resistant chocolate made with ethylcellulose (EC) oleogel, resisting melting up to 86°C. Lastly, O′Sullivan et al. (2017) incorporated β-carotene in EC oleogels and demonstrated that the oleogelation reduced the degradation of β-carotene compared to β-carotene in just oil, thus elevating its bioaccessibility. These examples serve as a library of possible beneficial oleogel properties suitable for food applications. However, it is important to remember that not all oleogels offer the aforementioned functionalities.
The appropriate selection of oils and oleogels is very important in providing successful products. For instance, Huang et al. (2018), who structured high oleic acid soybean oil into processed cheese products, reported that in a comparison of rice bran wax (RBW) and sunflower wax (SW), only RBW oleogels were able to mimic hardness of a milk fat control. This was shown to be related to chemical compositions of SW and RBW gelators. In particular, RBW consists of about 100% wax ester compared to sunflower (77% wax ester), which significantly affected physical properties of the cheese products (Huang et al., 2018). Yilmaz and Ögütcü (2015a) showed different hardness for SW oleogels made with hazelnut vs. virgin olive oils. Figure 1 shows network comparisons of oleogel cream cheese products developed by Bemer et al. (2016) who replaced the milk fat with EC or RBW oleogels. Although the authors demonstrated the similarity of the morphology and distribution of the fat globules in oleogel cream cheeses (either made with EC or RBW) to the control product, it seems EC and RBW had different effects on the protein networks. The EC oleogel networks contained small lipid globules dispersed throughout a continuous protein matrix, while the RBW oleogel created a denser protein network compared to the EC samples. Overall, these examples show that while the combinations of oils and gelators are important for food production, their interactions with other food ingredients need careful evaluations.
Figure 1. Microstructure of commercial cream cheese (P1) and rice bran wax (RBW 1) and ethylcellulose (EC 1) oleogel cream cheese products. Lipids and proteins shown in red and green, respectively. Adopted from Bemer et al. (2016) with permission from Elsevier.
Clearly, one major aim of oleogel development is to improve the health benefits of fatty food products. As expected, certain oleogel-based food products have been shown to contain healthier nutritional profiles compared to those made with conventional hardstock fats. For example, Bemer et al. (2016) incorporated RBW oleogels in cream cheese products and showed that replacing milkfat led to a 120% increase in unsaturated fat content and about a 90% reduction in saturated fat content. Bologna-type sausages formulated with oleogels replacing pork back fats showed about a 6% reduction in total saturated fat content and up to 21.15% increase in oleic acid levels (da Silva et al., 2019). A similar trend was observed in meat patties made with hydroxypropyl methylcellulose (HPMC) oleogels (Oh et al., 2019). These results clearly illustrate oleogels' capability for enhancing food nutrition; however, the digestibility of these products and their consumption for people with different diets is still not studied.
Some oleogels, particularly those made with EC and some waxes, have high melting points (70 ~ 135°C) that may impact the meltability of lipid networks in human body temperature. Moreover, oleogels with low meltability may retard the release of the lipid matrix, hence possibly influencing the overall metabolism of oleogel food. To better validate oleogel usage in food, clinical trials with human subjects are necessary. As a response to this need, some studies monitored the metabolism of oleogel food products. For example, Stortz et al. (2012) showed that mean serum triacylglycerol and free fatty acid levels from 12 young individuals consuming meals containing oleogels (2% 12-hydroxystearic acid and canola oil) were significantly lower compared to those who had meals with butter and margarine. Another study by Tan et al. (2017) reported higher blood triglycerides levels in 16 participants who consumed a meal with coconut oil compared to those consumed coconut oleogel gelled with 11% w/w EC. Such findings highlighted the effects of oleogelation on the digestion of fats and suggested that structural properties of oleogels may either restrict the absorption of dietary lipids or require a longer interval for lipids to be digested. Similarly, O′Sullivan et al. (2017), who studied the bioaccessibility of EC oleogels through in vitro digestion, noted that the lipid lipolysis percentage of EC oleogels was significantly lower than that of canola oil. The authors linked this finding with greater mechanical properties of the EC oleogels compared to the oil. Specifically, the former was more resistant to the mechanical breakdown, impeding the release of oil from the gel matrix and subsequently lowering the lipid lipolysis rate. These results may also be attributed to the high melting points of EC oleogels (135°C) and its meltability in the human body (Davidovich-Pinhas et al., 2015). Using human trials, Marangoni et al. (2007) noted that unlike liquid oil, a consumption of monoglyceride structured emulsion oleogel lowered serum triglyceride and insulin levels. This result suggested that oleogel matrices could be engineered in a way that they can modulate physiological responses of nutrient metabolism and provide healthier nutrition for consumers. However, Wright et al. (2014) observed that when this monoglyceride emulsion oleogel was baked in cookies and cakes, both serum triglyceride and insulin levels of human panelists were comparable to those who consumed ungelled oleogels.
All of these studies clearly document the complexity in the metabolism of oleogels and oleogel-formulated food products. It is also important to consider that not only the structure and composition of oleogels, but also the food formulations and their network formations can influence the digestion of oleogel food. Considering the high melting points of oleogels and the thermal processing required for their engineering, possible thermal degradation of final products needs investigations, as well. Oils and other micronutrients are prone to the oxidation that causes nutritional degradations and development of off-flavors. The review of studies on oil oxidation in some oleogel food products is provided in the stability section of this paper. However, no data was found on the effects of oleogelation on the nutritional properties of non-oil compounds. Robust clinical trials on oleogel food products evaluating how the structural and processing parameters required for oleogelation influence metabolism would help define values of oleogels for food applications. Considering a greater number of participants in different spectrums of age groups could also result in more conclusive findings.
In addition to nutritional improvements, one of the main objectives of oleogel use is mimicking textural functionality of trans and hardstock fats. Although some studies reported acceptable consistency and appearance for oleogel products, not all of them demonstrated satisfying textural attributes compared to saturated fat controls. Frankfurters made with EC oleogels showed similar hardness and chewiness values compared to the beef fat controls, and the authors connected these results to the samples' similar microstructure (Zetzl et al., 2012). The authors' finding regarding the role of oleogel microstructure on the texture of oleogel food may be seen better in the comparison of the effects of different gelators on vegetable oil structuring, shown in Figure 2. Oleogels made with monoglycerides (Figure 2A) show large plate-like shapes of crystals (López-Martínez et al., 2014) while fibrous oleogel microstructures are obtained from phytosterols (Figure 2B) (Sawalha et al., 2013). Figure 2C shows thin fibrillar crystals with a branching out pattern in oleogels structured with 12-hydroxystearic acid (Co and Marangoni, 2013). Interestingly, ethylcellulose gelators are shown to create polymer-like oleogel networks with numerous pockets or holes between polymers as illustrated in Figure 2D (Zetzl et al., 2014). As expected, oleogels made with different waxes exhibit considerably different matrices. For instance, Figure 2E shows small linear crystal particles of candelilla wax oleogels (Patel et al., 2015) while thin needle-like crystal morphologies are reported for sunflower wax oleogels (Figure 2F) (Yilmaz and Ögütcü, 2014). These examples clearly indicate that oleogels structured with various gelator types generate different microstructural properties that may subsequently affect physical properties of the oleogels and the food made of them. It is also important to remember that processing parameters (cooling rate, gelator concentrations, oil-gelator combinations, etc.) can influence the oleogel formations (Blake and Marangoni, 2015). Patel et al. (2014a) documented the effects of oleogelation on minimizing crosslinking of the gluten network in MC oleogel cakes. In that study, the hardness and chewiness of cakes made with just sunflower oil considerably increased (due to the crosslinking of gluten) over time while those of the MC oleogel cakes changed considerably less. This was due to the MC oleogel acting as a physical barrier that helped reduce the movement of gluten networks (Patel et al., 2014a). The successful applications of oleogels on hardness, spreadability, and stickiness in cream cheese and processed cheese products are also reported by the Maleky group (Bemer et al., 2016; Huang et al., 2018). As mentioned before, the authors linked their observation to the similarity in the solid fat network between the oleogels and controlled products.
Figure 2. Microstructures of oleogels structured with (A) monoglycerides in high oleic safflower oil (adopted from López-Martínez et al., 2014 with permission from Elsevier), (B) phytosterol tubules in sunflower oil (adopted from Sawalha et al., 2013 with permission from Wiley-VCH), (C) 12-hydroxystearic acid in canola oil (adopted from Co and Marangoni, 2013 with permission from Wiley-VCH), (D) ethylcellulose in canola oil (adopted from Zetzl et al., 2014 with permission from Elsevier), (E) candelilla wax in high oleic sunflower oil with a scale bar of 50 μm (adopted from Patel et al., 2015 with permission from ACS Publications), and (F) sunflower wax in olive oil (adopted from Yilmaz and Ögütcü, 2014 with permission from Wiley-VCH).
As anticipated, not all oleogel systems can provide mechanical properties comparable to the solid fat products, thus requiring investigations on various gelator concentrations or liquid oil compositions. For instance, Huang et al. (2018) structured processed cheese products with RBW or SW oleogels at wax concentrations of 0.5 or 1% and reported that SW oleogels were not able to mimic hardness of the control cheese product. Specifically, only 1% RBW oleogel showed comparable hardness to the control. As previously stated, this could be due to the higher wax ester content of RBW compared to SW, however further analysis is required to clarify the observed discrapany. In a comparison of oleogel cookies with those made with shortening, Yilmaz and Ögütcü (2015b) reported lower hardness and higher fracturability for cookies made of hazelnut oil and 5% beeswax or sunflower wax. Interestingly, the same group reported comparable texture and acceptability for the oleogel and control cookies based on hedonic tests performed with 200 participants (Yilmaz and Ögütcü, 2015b). The observed discrepancy in the textural assessment of those cookies between panelists and a texture analyzer instrument clearly shows challenges of accurately assessing texture of oleogels and oleogel food using just instrumental analyses. Another example of unsuccessful oleogel application in texture mimicking is shown in meat patties made with various concentrations of HPMC oleogels (Oh et al., 2019). Oh et al. reported different tenderness and juiciness in oleogel samples due to their inherently different solid fat content, hardness, and storage modulus values; 30 panelists confirmed the samples' discrepancies.
These findings highlight that although certain oleogel matrices are able to mimic textural properties of hardstock fats, predicting how consumers will perceive the final physical properties of oleogel-formulated food remains challenging. So far, there are numerous studies describing textural attributes of oleogels and/or oleogel food, but not so many sensory evaluations have been conducted (Rogers et al., 2014). This lack of sensory evaluation may be attributed to challenges of designing sensory testing, requiring safe protocols (e.g., types and concentrations of gelators), and ensuring that oleogel food products are safe for consumption. Another limitation could be possible off-flavors and odors that oleogel foods may generate. Previously, Bemer et al. (2016) showed that panelists detected off-flavor and bitterness from the RBW oleogel cream cheese products, suggesting an area of the oleogel field that needs to be investigated. Although the aforementioned challenge in oleogel cream cheese remains untouched, similar analysis and information on other products may help identify optimal oleogels for specific food applications. This type of analysis is needed to increase the consumer acceptance of oleogel food.
Oil binding capacity, which refers to how strongly oil is bound in a given network, is a challenging phenomenon for many food producers. Food products with a low oil binding capacity release oil and undergo oil migration, which negatively affects their textural and sensory attributes. Considering the relatively low solid fat content of many oleogels and their high liquid oil content, appropriate processing and storage conditions are necessary for optimal oil retention. Several studies developed oleogels with different gelators and showed a linear relationship between mechanical strength of gels and oil binding capacity, suggesting that oleogels could minimize the oil loss when designed with greater mechanical strength and tightly arranged networks (Fayaz et al., 2017; da Silva et al., 2018; Meng et al., 2018). Blake and Marangoni (2015) illustrated that the oil binding capacity of wax oleogels can be improved by increasing wax concentrations and/or fast cooling, last of which forms a homogenous distribution of small fat crystals and subsequently small areas of pore space. Currently, limited information is available on the oil binding capacity of oleogel-formulated food. Processed cheese products made with 0.5% RBW showed a marginal oil loss of 0.53% after 24 h of storage at 20°C (Huang et al., 2018). In that study, increasing RBW concentration to 1% led to a lower oil loss around 0.34%. A study by Tanti et al. (2016b) also demonstrated that no oil loss was observed for peanut butters when stabilized with 2.2% of freeze-dried HPMC and MC. These results are promising, but oil loss may be problematic for an industrial scale shearing process. It is also important to consider food regulations limiting high concentrations of gelators (e.g., FDA approves up to 3% of RBW in food). Additionally, dramatic changes in processing and crystallization temperature may influence other functional characteristics of the final products including polymorphic, microstructural, and physical properties. Clearly, more work is needed to examine how well other forms of oleogels preserve their physical entities and prevent the oil leakage and mass transfer in various food networks.
Another factor associated with oil stability is its oxidation and thermal degradation. As mentioned earlier, thermal processing required for oleogelation can induce lipid oxidation (Rogers et al., 2014). Studies reported peroxide values increased in oleogels post-processing as well as during storage (Gravelle et al., 2012; Yilmaz and Ögütcü, 2014; Park et al., 2018). Although, the reported peroxide values were under 10 mEq/kg, the value that is considered as rancid oil (Sebastian et al., 2014), further analysis on peroxide degradation and secondary oxidation during the oleogelation processing is not provided for many oleogel food products. Interestingly, some studies showed a reduction in the oil oxidation during the storage and post-processing of oleogels and oleogel food products. For example, Pandolsook and Kupongsak (2019) showed that RBW oleogels and water-in-oil emulsions made with RBW oleogels stored at 4 and 30 °C exhibited a higher oxidative stability compared to their controls (rice bran oil and emulsion, respectively) at both temperatures. Certain studies even documented further stability of the oils when oleogel networks were incorporated in food matrices. For example, Park et al. (2018) reported a lower aldehyde content in RBW oleogel cream cheeses compared to the oleogel itself while documenting no change in aldehyde content for 2 weeks. Oleogelation also significantly reduced the oxidation in bologna-type sausages and fish oil oleogels compared to control sausages made with pork back fat and fish oil, respectively (Hwang et al., 2018; da Silva et al., 2019). Low temperature storage (4°C) of frankfurters structured with sunflower oil and γ-oryzanol and phytosterol showed similar aldehyde content compared to controls for 30 days (Panagiotopoulou et al., 2016). Although these findings suggest that the oleogelations with carefully designed formulations, processing, and post-processing conditions may reduce the oxidation, not all oleogel systems exhibited good oxidative stability. Martins et al. (2017) reported a significantly greater amount of aldehyde formation over 20 days of storage in beeswax oleogels. Frankfurter-type sausages constructed with 10% RBW oleogels also generated significantly higher amounts of aldehyde compared to those made with pork fat (Wolfer et al., 2018). Meat patties structured with HPMC oleogels also showed a significantly lower oxidative stability compared to patties made with beef tallow (Oh et al., 2019). To overcome this issue, the authors suggest increasing the gelator concentrations for a greater oxidative stability. This increase may not be a good solution for many products considering the legislations on gelator concentrations and the effects of gelator concentrations on other physical properties of the product.
Hence, the assessment of oxidative stability of oleogels in food products needs further investigation. Performing multiple tests (e.g., both primary and secondary oxidation analyses) may address the lack of information regarding the oxidative stability of oleogel-formulated food. It would be also beneficial if future studies monitor the effects of the incorporation of lipid soluble antioxidants to the liquid oils on the minimization of the lipid oxidation of oleogel food.
The field of oleogels has grown extensively in the last decade, generating various food products with oleogels. However, to fulfill its potential usage in food products, there is a need for further investigations on various dimensions. In order to validate the oleogel applications in food to replace hardstock fats, the overall impact of oleogel digestion/metabolism must be clearly understood. In addition, acquiring detailed information regarding physical and oxidative stability of oleogels and their behavior in the presence of other ingredients and food matrices may advance oleogels utilization in many food products. Flavor development, textural qualities, and physical properties of oleogels and oleogel-formulated food must be also evaluated by sensory panels. Doing so may play a key role on the consumer acceptance of oleogel food products. While there are great potentials for oleogel applications in food products, more information is needed before the widespread use. Bridging the aforementioned knowledge gaps may play key roles on the future success of the oleogel field and increase the consumer acceptance of oleogel food products.
All authors listed have made a substantial, direct and intellectual contribution to the work, and approved it for publication.
The authors declare that the research was conducted in the absence of any commercial or financial relationships that could be construed as a potential conflict of interest.
Astrup, A., Bertram, H. C. S., Bonjour, J. P., de Groot, L. C. P., Otto, M. C. D. O., Feeney, E. L., et al. (2019). WHO draft guidelines on dietary saturated and trans fatty acids: time for a new approach? BMJ 366, 1–6. doi: 10.1136/bmj.l4137
Bemer, H. L., Limbaugh, M., Cramer, E. D., Harper, W. J., and Maleky, F. (2016). Vegetable organogels incorporation in cream cheese products. Food Res. Int. 85, 67–75. doi: 10.1016/j.foodres.2016.04.016
Blake, A. I., and Marangoni, A. G. (2015). The use of cooling rate to engineer the microstructure and oil binding capacity of wax crystal networks. Food Biophys. 10, 456–465. doi: 10.1007/s11483-015-9409-0
Co, E., and Marangoni, A. G. (2013). The formation of a 12-hydroxystearic acid/vegetable oil organogel under shear and thermal fields. J. Am. Oil Chem. Soc. 90, 529–544. doi: 10.1007/s11746-012-2196-6
da Silva, S. L., Amaral, J. T., Ribeiro, M., Sebastiao, E. E., Vargas, C., de Lima Franze, F., et al. (2019). Fat replacement by oleogel rich in oleic acid and its impact on the technological, nutritional, oxidative, and sensory properties of Bologna-type sausages. Meat Sci. 149, 141–148. doi: 10.1016/j.meatsci.2018.11.020
da Silva, T. L. T., Arellano, D. B., and Martini, S. (2018). Physical properties of candelilla wax, monoacylglycerols, and fully hydrogenated oil oleogels. J. Am. Oil Chem. Soc. 95, 797–811. doi: 10.1002/aocs.12096
Davidovich-Pinhas, M., Barbut, S., and Maraongoni, A. G. (2015). The gelation of oil using ethyl cellulose. Carbohydr. Polym. 117, 869–878. doi: 10.1016/j.carbpol.2014.10.035
Doan, C. D., Patel, A. R., Tavernier, I., De Clercq, N., Van Raemdonck, K., Van de Walle, D., et al. (2016). The feasibility of wax-based oleogel as a potential co-structurant with palm oil in low-saturated fat confectionery fillings. Eur. J. Lipid Sci. Tech. 118, 1903–1914. doi: 10.1002/ejlt.201500172
Fayaz, G., Goli, S. A. H., Kadivar, M., Valoppi, F., Barba, L., Calligaris, S., et al. (2017). Potential application of pomegranate seed oil oleogels based on monoglycerides, beeswax and propolis wax as partial substitutes of palm oil in functional chocolate spread. LWT 86, 523–529. doi: 10.1016/j.lwt.2017.08.036
Gravelle, A. J., Barbut, S., and Marangoni, A. G. (2012). Ethylcellulose oleogels: manufacturing considerations and effects of oil oxidation. Food Res. Int. 48, 578–583. doi: 10.1016/j.foodres.2012.05.020
Huang, H., Hallinan, R., and Maleky, F. (2018). Comparison of different olegels in processed cheese products formulation. Int. J. Food Sci. 53, 2525–2534. doi: 10.1111/ijfs.13846
Hughes, N. E., Marangoni, A. G., Wright, A. J., Rogers, M. A., and Rush, J. W. E. (2009). Potential food applications of edible oil organogels. Trends Food Sci. Technol. 20, 470–480. doi: 10.1016/j.tifs.2009.06.002
Hwang, H. S., Fhaner, M., Winkler-Moser, J. K., and Liu, S. X. (2018). Oxidation of fish oil oleogels formed by natural waxes in comparison with bulk oil. Eur. J. Lipid Sci. Tech. 120:1700378. doi: 10.1002/ejlt.201700378
Hwang, H. S., Singh, M., Winkler-Moser, J. K., Bakota, E. L., and Liu, S. X. (2013). Margarine from oranogels of plant wax and soybean oil. J. Am. Oil Chem. Soc. 90, C1926–1932. doi: 10.1007/s11746-013-2315-z
Jang, A., Bae, W., Hwang, H. S., Lee, H. G., and Lee, S. (2015). Evaluation of canola oil oleogels with candelilla wax as an alternative to shortening in baked goods. Food Chem. 187, 525–529. doi: 10.1016/j.foodchem.2015.04.110
López-Martínez, A., Morales-Rueda, J. A., Dibildox-Alvarado, E., Charó-Alonso, M. A., Marangoni, A. G., and Toro-Vazquez, J. F. (2014). Comparing the crystallization and rheological behavior of organogels developed by pure and commercial monoglycerides in vegetable oil. Food Res. Int. 64, 946–957. doi: 10.1016/j.foodres.2014.08.029
Marangoni, A. G., Idziak, S. H. J., Vega, C., Batte, H., Ollivon, M., Jantiz, P. S., et al. (2007). Encapsulation-structuring of edible oil attenuates acute elevation of blood lipids and insulin in humans. Soft Matter. 3, 183–187. doi: 10.1039/B611985A
Martins, A. J., Cerqueira, M. A., Cunha, R. L., and Vincente, A. A. (2017). Fortified beeswax oleogels: effect of β-carotene on the gel structure and oxidative stability. Food Funct. 8, 4241–4250. doi: 10.1039/C7FO00953D
Martins, A. J., Lorenzo, J. M., Franco, D., Vincente, A. A., Cunha, R. L., Pastrana, L. M., et al. (2019). Omega-3 and polyunsaturated fatty acids-enriched hamburgers using sterol-based oleogels. Eur. J. Lipid Sci. Tech. 121:1900111. doi: 10.1002/ejlt.201900111
Meng, Z., Qi, K., Guo, Y., Wang, Y., and Liu, Y. (2018). Effects of thickening agents on the formation and properties of edible oleogels based on hydroxypropyl methyl cellulose. Food Chem. 246, 137–149. doi: 10.1016/j.foodchem.2017.10.154
Moghtadaei, M., Soltanizadeh, N., and Goli, S. A. H. (2018). Production of sesame oil oleogels based on beeswax and application as partial substitutes of animal fat in beef burger. Food Res. Int. 108, 368–377. doi: 10.1016/j.foodres.2018.03.051
Moriano, M. E., and Alamprese, C. (2017). Organogels as novel ingredients for low saturated fat ice creams. LWT 86, 371–376. doi: 10.1016/j.lwt.2017.07.034
Oh, I., Lee, J., Lee, H. G., and Lee, S. (2019). Feasibility of hydroxypropyl methylcellulose oleogel as an animal fat replacer for meat patties. Food Res. Int. 122, 566–572. doi: 10.1016/j.foodres.2019.01.012
Oh, I. K., Amoah, C., Lim, J., Jeong, S., and Lee, S. (2017). Assessing the effectiveness of wax-based sunflower oil oleogels in cakes as a shortening replacer. LWT 86, 430–437. doi: 10.1016/j.lwt.2017.08.021
O′Sullivan, C. M., Davidovich-Pinhas, M., Wright, A. J., Barbut, S., and Marangoni, A. G. (2017). Ethylcellulose oleogels for lipophilic bioactive delivery – effect of oleogelation on in vitro bioaccessibility and stability of beta-carotene. Food Funct. 8, 1438–1451. doi: 10.1039/C6FO01805J
Panagiotopoulou, E., Moschakis, T., and Katsanidis, E. (2016). Sunflower oil organogels and organogel-in-water emulsions (part II): implementation in frankfurter sausages. LWT 73, 351–356. doi: 10.1016/j.lwt.2016.06.006
Pandolsook, S., and Kupongsak, S. (2017). Influence of bleached rice bran wax on the physiochemical properties of organogels and water-in-oil emulsions. J. Food Eng. 214, 182–192. doi: 10.1016/j.jfoodeng.2017.06.030
Pandolsook, S., and Kupongsak, S. (2019). Storage stability of bleached rice bran wax oranogels and water-in-oil emulsions. J. Food Meas Charact. 13, 431–443. doi: 10.1007/s11694-018-9957-3
Park, C., Bemer, H. L., and Maleky, F. (2018). Oxidative stability of rice bran wax oleogels and an oleogel cream cheese product. J. Am. Oil Chem. Soc. 95, 1267–1275. doi: 10.1002/aocs.12095
Patel, A. R., Babaahmadi, M., Lesaffer, A., and Dewettinck, K. (2015). Rheological profiling of organogels prepared at critical gelling concentrations of natural waxes in a triacylglycerol solvent. J. Agric. Food Chem. 63, 4862–4869. doi: 10.1021/acs.jafc.5b01548
Patel, A. R., Cludts, N., Sintang, M. D. B., Lesaffer, A., and Dewettinck, K. (2014a). Edible oleogels based on water soluble food polymers: preparation, characterization and potential application. Food Funct. 5, 2833–2841. doi: 10.1039/C4FO00624K
Patel, A. R., Rajarethinem, P. S., Gredowska, A., Turhan, O., Lesaffer, A., De Vos, W. H., et al. (2014b). Edible applications of shellac oleogels: spreads, chocolate paste and cakes. Food Funct. 5, 645–652. doi: 10.1039/C4FO00034J
Pehlivanoglu, H., Ozulku, G., Yildirim, R. M., Demirci, M., Toker, O. S., and Sagdic, O. (2018). Investigating the usage of unsaturated fatty acid-rich and low-calorie oleogels as a shortening mimetics in cake. J. Food Process Pres. 42:e13621. doi: 10.1111/jfpp.13621
Rogers, M. A., Strober, T., Bot, A., Toro-Vazquez, J. F., Stortz, T., and Marangoni, A. (2014). Edible olegels in molecular gastronomy. Int. J. Gastron. Food Sci. 2, 22–31. doi: 10.1016/j.ijgfs.2014.05.001
Sawalha, H., Margry, G., den Adel, R., Venema, P., Bot, A., Flöter, E., et al. (2013). The influence of the type of oil phase on the self-assembly process of γ oryzanol + β-sitosterol tubules in organogel systems. Eur. J. Lipid Sci. Tech. 115, 295–300. doi: 10.1002/ejlt.201100395
Sebastian, A., Ghazani, S. M., and Marangoni, A. G. (2014). Quality and safety of frying oils used in restaurants. Food Res. Int. 64, 420–423. doi: 10.1016/j.foodres.2014.07.033
Stortz, T. A., and Marangoni, A. G. (2011). Heat resistant chocolate. Trends Food Sci. Technol. 22, 201–214. doi: 10.1016/j.tifs.2011.02.001
Stortz, T. A., and Marangoni, A. G. (2013). Ethylcellulose solvent substitution method of preparing heat resistant chocolate. Food Res. Int. 51, 797–803. doi: 10.1016/j.foodres.2013.01.059
Stortz, T. A., Zetzl, A. K., Barbut, S., Cattaruzza, A., and Marangoni, A. G. (2012). Edible oleogels in food products to help maximize health benefits and improve nutritional profiles. Lipid Technol. 24, 151–154. doi: 10.1002/lite.201200205
Tan, S. Y., Peh, E. W. Y., Marangoni, A. G., and Henry, C. J. (2017). Effects of liquid oil vs. oleogel co-ingested with a carbohydrate-rich meal on human blood triglycerides, glucose, insulin, and appetite. Food Funct. 8, 241–249. doi: 10.1039/C6FO01274D
Tanti, R., Barbut, S., and Marangoni, A. G. (2016a). Hydroxypropyl methylcellulose and methylcellulose structured oil as a replacement for shortening in sandwich cookie creams. Food Hydrocoll. 61, 329–337. doi: 10.1016/j.foodhyd.2016.05.032
Tanti, R., Barbut, S., and Marangoni, A. G. (2016b). Oil stabilization of natural peanut butter using food grade polymers. Food Hydrocoll. 61, 399–408. doi: 10.1016/j.foodhyd.2016.05.034
Wendt, A., Abraham, K., Wernecke, C., Pfeiffer, J., and Flöter, E. (2017). Application of β-sitosterol + γ-oryzanol-structured organogel as migration barrier in filled chocolate products. J. Am. Oil Chem. Soc. 94, 1131–1140. doi: 10.1007/s11746-017-3024-9
Wolfer, T. L., Acevedo, N. C., Prusa, K. J., Sebranek, J. G., and Tart,é, R. (2018). Replacement of pork fat in frankfurter-type sausages by soybean oil oleogels structured with rice bran wax. Meat Sci. 145, 352–362. doi: 10.1016/j.meatsci.2018.07.012
Wright, A., Pinto, C., Tulk, H., McCluskey, J., Goldstein, A., Huschka, B., et al. (2014). Monoacylglycerol gel offers improved lipid profiles in high and low moisture baked products but does not influence postprandial lipid and glucose responses. Food Funct. 5, 882–893. doi: 10.1039/C3FO60596E
Yilmaz, E., and Ögütcü, M. (2014). Comparative analysis of olive oil organogels containing beeswax and sunflower wax with breakfast margarine. J. Food Sci. 79, E1732–1738. doi: 10.1111/1750-3841.12561
Yilmaz, E., and Ögütcü, M. (2015a). Oleogels as spreadable fat and butter alternatives: sensory description and consumer perception. RSC Adv. 5, 50259–50267. doi: 10.1039/C5RA06689A
Yilmaz, E., and Ögütcü, M. (2015b). The texture, sensory properties and stability of cookies prepared with wax oleogels. Food Funct. 6, 1194–1204. doi: 10.1039/C5FO00019J
Zetzl, A. K., Gravelle, A. J., Kurylowicz, M., Dutcher, J., Barbut, S., and Marangoni, A. G. (2014). Microstructure of ethylcellulose oleogels and its relationship to mechanical properties. Food Struct. 2, 27–40. doi: 10.1016/j.foostr.2014.07.002
Zetzl, A. K., Marangoni, A. G., and Barbut, S. (2012). Mechanical properties of ethylcellulose oleogels and their potential for saturated fat reduction in frankfurters. Food Funct. 3, 327–337. doi: 10.1039/c2fo10202a
Keywords: oleogel, food grade gelators, trans-fat replacer, oleogel's physical properties, oleogel's oxidation
Citation: Park C and Maleky F (2020) A Critical Review of the Last 10 Years of Oleogels in Food. Front. Sustain. Food Syst. 4:139. doi: 10.3389/fsufs.2020.00139
Received: 14 May 2020; Accepted: 03 August 2020;
Published: 15 September 2020.
Edited by:
Stephen Robert Euston, Heriot-Watt University, United KingdomReviewed by:
Khakhanang Wijarnprecha, Ryerson University, CanadaCopyright © 2020 Park and Maleky. This is an open-access article distributed under the terms of the Creative Commons Attribution License (CC BY). The use, distribution or reproduction in other forums is permitted, provided the original author(s) and the copyright owner(s) are credited and that the original publication in this journal is cited, in accordance with accepted academic practice. No use, distribution or reproduction is permitted which does not comply with these terms.
*Correspondence: Farnaz Maleky, bWFsZWt5LjFAb3N1LmVkdQ==
Disclaimer: All claims expressed in this article are solely those of the authors and do not necessarily represent those of their affiliated organizations, or those of the publisher, the editors and the reviewers. Any product that may be evaluated in this article or claim that may be made by its manufacturer is not guaranteed or endorsed by the publisher.
Research integrity at Frontiers
Learn more about the work of our research integrity team to safeguard the quality of each article we publish.