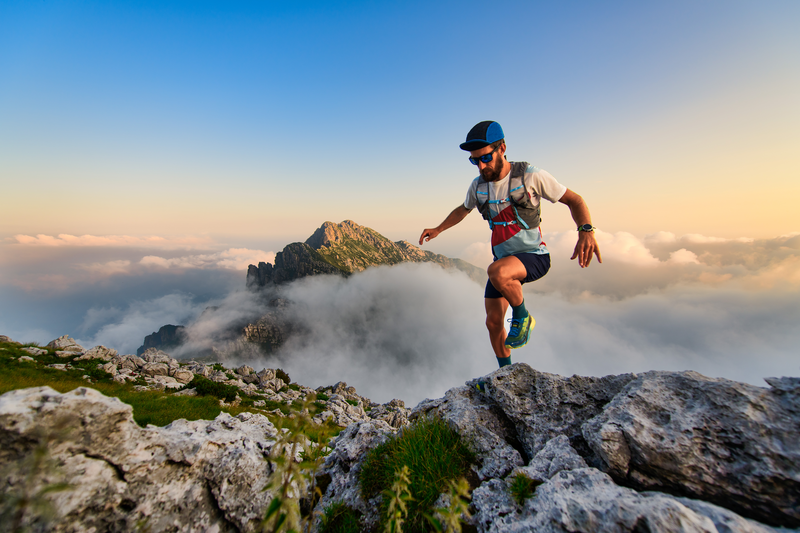
94% of researchers rate our articles as excellent or good
Learn more about the work of our research integrity team to safeguard the quality of each article we publish.
Find out more
ORIGINAL RESEARCH article
Front. Sustain. Food Syst. , 19 August 2020
Sec. Crop Biology and Sustainability
Volume 4 - 2020 | https://doi.org/10.3389/fsufs.2020.00094
This article is part of the Research Topic Intercropping Systems in Sustainable Agriculture View all 17 articles
We investigated Bambara groundnut, groundnut, mung bean, cowpea, and black gram for use as biofertilizers in cropping systems. The 15N natural abundance technique was used to measure N2 fixation in this study. The percent of N derived from fixation by mung bean (Vigna radiata L. Wilczek), Bambara groundnut (Vigna subterranea L. Verdc.), cowpea (Vigna unguiculata L. Walp.), black gram (Vigna mungo L.), and groundnut (Arachis hypogaea L) was 98, 83, 79, 66, and 45% respectively. Nitrogen contribution from these legumes was 83, 67, 39, 36, and 32 kg.ha−1 respectively for Bambara groundnut, groundnut, mung bean, black gram, and cowpea. Maize grain yield without N fertilizer was 2,449, 2,291, 2,204, 2,046, and 1,671 kg.ha−1, respectively, for maize following groundnut, Bambara groundnut, cowpea, mung bean, black gram, and maize. Grain yield increase of maize after legumes without N fertilizer was 47, 46, 37, 32, and 22%, respectively, for groundnut, Bambara groundnut, cowpea, mung bean, and black gram. Supplying 0 to 60 kg N ha−1 to maize plants increased shoot DM from 3,264 to 4,279 kg.ha−1, grain yield from 2,184 to 3,586 kg.ha−1, and whole-plant DM from 5,448 to 7,865 kg.ha−1, which represented a 31, 64, and 44% increase with N fertilizer supply from 0 to 60 kg N ha−1. Symbiotic N benefit of preceding legumes to maize without N fertilizer was 20–40 kg N. ha−1 in fertilizer equivalents. The preceding legumes increased maize grain concentrations of P, Ca, S, Fe, Mn, and Zn in zero-N plots relative to maize after maize. There was a 225, 222, 154, 149, and 108% increase in marginal returns of maize after groundnut, Bambara groundnut, cowpea, mung bean, and black gram, respectively, without N fertilizer.
Maize is a major staple food crop in Africa, especially in Southern Africa, and is the most important component of smallholder cropping systems in the continent. In Africa, maize is either grown as a monoculture or intercropped with cowpea, groundnut, or Bambara groundnut without any specific planting pattern or rotational system (Mathews and Beck, 1994). Though a staple, maize production in Africa is often constrained by drought and low rainfall, as observed during the 2018 drought in Southern Africa. But maize production can also be low on farmers' fields due to other abiotic factors.
Although commercial farmers in countries like South Africa can obtain maize yields of 4,210–6,470 kg ha−1, grain yield is <2,000 kg ha−1 on smallholder fields due to inherently low soil fertility, severe soil degradation, intensive cereal monoculture, low inputs, and inappropriate land use (Von Loeper et al., 2016; DAFF, 2018). While the use of mineral fertilizers could improve maize yields in Africa, they are inaccessible to resource-poor farmers due to their high cost. The low or lack of fertilizer use is currently the major factor limiting increased crop yields (Sinclair and Vadez, 2012) as, on average, only about 8.8 kg NPK fertilizer is applied per hectare by smallholder farmers in Africa (Henao and Baanante, 2006). The inclusion of N2-fixing legumes in traditional cropping systems can improve soil N fertility and increase crop yields for enhanced food/nutritional security (Walley et al., 2007; Lithourgidis et al., 2011; Ngwira et al., 2012).
The main food legumes cultivated in Africa include cowpea, groundnut, Bambara groundnut, pigeon pea, common bean, and in recent times, soybean. Whether intercropped or cultivated as a monoculture, these legumes are often rotated with cereal crops. While crop rotation is an age-old practice that is not novel, the science behind it is still not properly understood. The work presented here is only a small part of a wider study to understand the changes in soil fertility, soil health, and soil microbial populations using metagenomics. Although all these factors influence yields of cereal crops rotated with legumes, the soil nutrient enrichment, increase in the population of beneficial microbes, and reduction in pathogenic microbes have not been quantified.
About 32 years ago, Dakora et al. (1987) showed that monocultured groundnut and cowpea, respectively, derived 79 and 89% of their N nutrition from symbiotic N2 fixation, contributed 101 and 210 kg N ha−1, and increased grain yield by 89 and 95% in zero-N plots when maize was rotated with groundnut and cowpea as preceding crops in Northern Ghana. The net N returns to soil in leguminous residues were 68 kg ha−1 for groundnut and 150 kg ha−1 for cowpea, while the N benefit of each legume to maize in the rotation was equivalent to 60 kg ha−1 of N fertilizer based on grain and dry matter yields (Dakora et al., 1987). Clearly, those findings have shown that crop rotation has the potential to improve soil health and increase plant productivity.
In Africa, the N2-fixing ability and diversity of native soil rhizobia nodulating cowpea, groundnut, Bambara groundnut, common bean, soybean, and Kersting's bean have been established (Chibeba et al., 2017; Puozaa et al., 2017, 2019; Zinga et al., 2017; Chidebe et al., 2018; Gyogluu et al., 2018; Mohammed et al., 2018, 2019). However, their N contribution in cropping systems is still not properly understood. We also do not know the rotation effects of these legumes on the growth, grain yield, and quality of following cereal crops. The aim of this study was to evaluate N contribution by groundnut (Arachis hypogaea L.), Bambara groundnut (Vigna subterranea L. Verdc.), cowpea (Vigna unguiculata L.), black gram (Vigna mungo L.), and mung bean (Vigna radiata L. Wilczek), and to assess their rotation effect on grain yield, quality, and economics of a following maize crop.
Field experiments were conducted during 2011//2012 and 2012/2013 cropping seasons at Nelspruit (25°26′25″ S, 30°58′57″ E and 640 m above sea level), in the Mpumalanga Province of South Africa. The field trial in 2011/2012 evaluated five grain legumes for plant growth and N2 contribution, while the field experiment in 2012/2013 measured the rotation effect of each legume on the grain yield and quality of a following maize crop. The rainfall received was 288 mm during the 2011/2012 planting season and 465 mm in the 2012/2013 cropping season. Classified as Avalon series, the soil at the study site is a deep and well-drained sandy-loam (8% clay) with pH 5.95, soil organic carbon (SOC) 0.18%, total nitrogen (N) 7.6 mg.kg−1, plant-available phosphorus (P) 19.91 mg.kg−1, and potassium (K) 38.86 mg.kg−1 (SCWG, 1991).
The field experiment in 2011/2012 was laid using a randomized complete block design with four replications. A maize plot was included in each replication for assessing the residual effect of these legumes to a following maize crop planted in the 2012/2013 cropping season. Each plot measured 14.4 × 6.3 m in 2011/2012 and the legume species were planted to achieve the plant population shown in Table 1. Before planting, 10 soil samples were randomly cored at a depth of 0–30 cm across the experimental site, pooled, sieved, and analyzed for total N, extractable P, K, calcium (Ca), magnesium (Mg) copper, (Cu), zinc (Zn), manganese (Mn), and iron (Fe).
Soil pH was determined in 1 M KCl solution (Black et al., 1965) using a pH meter. The percent soil organic carbon (SOC) was determined as described by Walkley and Black (1934). Extractable P, K, Ca, Zn, and Mg in the soil were determined using the Ambic-1 method developed by Van der Merwe et al. (1984). Extractable P and K were measured on a continuous flow analyzer, and Ca, Mg, and Zn on an atomic-absorption spectrophotometer using an air-acetylene flame. Total N was determined by Kjeldahl digestion (AOAC, 1990).
At flowering to early pod-filling stage, 10 plants were dug out from each plot, and separated into shoots, roots, and nodules. The shoot samples were oven-dried at 70°C for 48 h, weighed, and ground to a fine powder (0.85 mm) for analysis of 15N and 13C. The number of nodules per plant was recorded before oven-drying (70°C) to determine dry weight. Non-legume plants growing inside the plots were concurrently sampled and processed as was done for the legumes. At physiological maturity, 10 plants from the inner plots were harvested for yield determination.
To determine the 15N/14N ratios of plant samples, about 2.0–2.5 mg of plant material was weighed in tin capsules, loaded onto the mass spectrometer, and analyzed using a Carlo Erba NA1500 elemental analyzer (Fisons Instruments SpA, Strada, Rivoltana, Italy) coupled to a Finan MAT252 mass spectrometer (Finnigan, MAT CombH, Bremen, Germany) via a Conflo II open-split device. An internal standard (Nasturtium spp.) was included in every five runs to correct for machine error during isotopic fractionation. The isotopic analysis was done for both legumes and reference plants. The combined average δ15N signature of the non-legume reference plants (+2.01‰) was used to determine the %Ndfa of the test legumes (Table 2). The isotopic composition of 15N was measured as (Junk and Svec, 1958; Mariotti, 1983):
where 15N/14Nsample was the abundance ratio of 15N and 14N in the plant sample and 15N/14Natm was the abundance ratio of 15N and 14N in the atmosphere.
The percent N derived from N2 fixation (%Ndfa) by the selected legume species was determined as (Shearer and Kohl, 1986; Unkovich et al., 2008):
where δ15Nref is the combined mean 15N natural abundance of the non-legume plant species sampled from the experimental plots and used as reference plants (Table 2), δ15Nleg is the 15N natural abundance of the legumes tested, and the B value is the 15N natural abundance of the test legumes (Bambara groundnut, groundnut, cowpea, mung bean, and black gram) solely dependent on N2 fixation for their N nutrition. The B values of the test legumes used in this study are shown in Table 1. The B value incorporates the isotopic fractionation associated with N2 fixation and replaces the value of atmospheric N2 (Shearer and Kohl, 1986). The amount of N-fixed was calculated as (Maskey et al., 2001):
The N content of plants was estimated as the product of %N and shoot biomass as (Pausch et al., 1996):
The soil N uptake was calculated as the difference between total N in the shoots and N-fixed.
The 13C/12C isotopic ratios in shoots of the test legumes were similarly analyzed as described for 15N/14N and reported in the standard notation relative to Pee Dee Belemnite standard as (Farquhar et al., 1989):
where δ13C is the 13C natural abundance of the plant shoot sample expressed in parts per million (‰), and Rsample and Rstandard the 13C/12C abundance ratios of the shoot sample (Bambara groundnut, groundnut, cowpea, mung bean, and black gram) and standard, respectively. The 13C/12Cstandard used was the isotopic ratio of Belemnite Pee Dee limestone formation (Craig, 1957), a universally accepted standard. Shoot C content per plant was calculated as the product of %C and shoot dry matter weight.
After harvesting the grain, the biomass of each legume was incorporated back into its plots and planted with maize (cv ZM521) as a following crop in the next cropping season. Maize planted after maize was included as control. A spacing of 90 × 30 cm (plant population = 37 037 plants.ha−1) was used. The plots (14.4 × 6.3 m) of preceding legume and maize crops planted in 2011/2012 were each divided into four sub-plots measuring 6.3 × 3.6 m with four rows per plot, and four N levels (N0, N20, N40, and N60 kg.ha−1) super-imposed as sub-treatments using a factorial design with four replications. The five preceding grain legumes and maize planted in the year-1 trial represented the main treatment, and N levels (N0, N20, N40, and N60), the sub-treatments. The trial was planted on 05/10/2012; N was applied (N0, N20, N40, and N60 kg.ha−1) as limestone ammonium nitrate (LAN 28% N) at planting and 9 weeks after planting prior to tasseling. At physiological maturity, shoot dry matter (DM) yield, grain yield, and harvest index were determined from 10 plants per plot. The harvest index was calculated as grain DM divided by the whole-plant oven-dried (60°C) weight times 100 as (Donald and Hamblin, 1976).
The mineral nutrients in maize grain were analyzed at the Soil, Water, and Plant Laboratory, Western Cape Department of Agriculture, Elsenburg, South Africa. Briefly, to measure Fe, Zn, Cu, Mn, and B in cowpea leaves and grain, 1.0 g of ground plant sample was ashed in a porcelain crucible at 500°C overnight, followed by dissolving the ash in 5 ml of 6 M HCl (analytical grade) and placing it in an oven at 50°C for 30 min, after which 35 ml of de-ionized water was added. The mixture was filtered through Whatman No. 1 filter paper. Trace element concentration in plant extracts was determined from four replicate samples using inductively coupled plasma mass spectrometry (IRIS/AP HR DUO Themo Electron Corporation, Franklin, Massachusetts, USA) (Ataro et al., 2008).
Economic analyses involving monetary value, total variable costs, gross margin, percentage marginal returns, and cost/benefit ratio were done on maize grain yield produced by the subsequent crop treated to four N levels (i.e., N0, N20, N40, and N60). The economic parameters were estimated as (Gomez and Gomez, 1984):
The maize market price (in South African Rands) of R2000 per ton (DAFF, 2018) and N fertilizer (LAN) cost of R4500 per ton (GRAIN, 2013) were used to estimate the total variable cost. The gross margin per hectare was based on the estimate of total variable costs per hectare for seed, land preparation, agro-chemicals, labor, and N fertilizer, which amounted to R2650, R3238, R3575, and R3913 (in South African Rand currency), for the N0, N20, N40, and N60 treatments, respectively (i.e., the total variable cost was TVCN0 = R2650; TVCN20 = R3238; TVCN40 = R3575; TVCN60 = R3913).
Correlation analysis was performed to assess if there was any relationship between fixed-N in the shoots of the preceding legume species and plant growth and/or grain yield of the following maize crop.
The data were tested for normal distribution before being subjected to a 1-way or 2-way analysis of variance (ANOVA) using Statistica version 10.1 (Statsoft Inc., 2011). Where there were significant differences, the Dancan's multiple range test was used to separate the means at p ≤ 0.05. Pearson's correlation was performed to determine the relationships between yield and symbiotic indices.
The soil used for planting the five legumes in 2011/2012 had pH (KCl) 5.95 and contained 7.65 mg.kg−1 N, 19.91 mg.kg−1 P, 38.86 mg.kg−1 K, 143.50 mg.kg−1 Ca, 37.66 mg.kg−1 Mg, 3.19 mg.kg−1 Zn, 0.18% soil organic matter, and 8.0% clay.
The δ15N of non-legume plant species used as reference plants are shown in Table 2. Their values ranged from +0.27 to 7.79‰, and it is the combined mean value (+2.01‰) of the 15 plant species analyzed that was used to estimate the percent N derived from fixation by test legumes.
There were significant differences in the shoot N concentration of the legume species studied, and these ranged from 2.19% in mung bean to 3.12% in groundnut (Table 3). Groundnut and Bambara groundnut showed significantly (p ≤ 0.05) greater shoot N concentrations than the other legumes. But the shoot N concentration of black gram and cowpea were similar. Nitrogen content was significantly greater in Bambara groundnut than the other legumes (Table 3).
Table 3. Plant growth, root nodulation, symbiotic performance, and soil N uptake of five grain legumes planted in the field at Nelspruit, South Africa, in the 2011/2012 cropping season.
The results showed that shoot δ15N was significantly greater (p ≤ 0.05) in groundnut, followed by Bambara groundnut, black gram, cowpea, and mung bean (Table 3). The δ15N in the shoots of the five test legumes ranged from −0.97‰ in mung bean to −0.10‰ in groundnut. As a result, percent N derived from atmospheric fixation (%Ndfa) was also in the range of 45% in groundnut to 93% for mung bean, which reflected the shoot δ15N values of the legumes studied (Table 3). The amount of N-fixed was, however, significantly (p ≤ 0.05) greater in groundnut and Bambara groundnut than the other legumes due to larger shoot biomass (Table 3). Legume N contribution by shoots ranged from 32 kg. ha−1 in cowpea to 83 kg. ha−1 in Bambara groundnut. Nitrogen uptake from the soil was significantly greater in groundnut (83 kg. ha−1) and <20 kg. ha−1 in the other legumes which derived more N from fixation (Table 3).
A one-way ANOVA of shoot δ13C values showed significant variation between the test legumes (Table 3). The δ13C discrimination was markedly greater (less negative) in groundnut (−26.1‰) and Bambara groundnut (−26.4‰) compared to the other legumes, which recorded −27.3‰. The shoot C/N ratios of the test legume species also revealed substantial differences, which ranged from 14.1 g.g−1 in groundnut to 19.3 g.g−1 for mung bean (Table 3). These differences in shoot C/N could imply potential variation in the decomposition of plant biomass when incorporated into soil.
Shoot biomass of maize after groundnut was much greater, followed by maize after Bambara groundnut as the preceding crop (Table 4). In contrast, the shoot dry matter of maize after black gram was the lowest and similar to maize after maize. In the rotation, whole-plant maize DM (shoot + grain DM) was similar in trend to maize shoot DM, with whole-plant maize DM after groundnut being much higher than the others, followed by Bambara groundnut and cowpea (Table 4).
Table 4. Two-way ANOVA of the effect of a preceding legume crop on growth, grain yield, and harvest index of maize planted after legumes in the field and super-imposed with four N levels at Nelspruit in the Mpumalanga Province during the 2012/2013 cropping season.
Grain yield also differed significantly between and among the rotation systems. Substantial differences were found in the grain yield of maize following food legumes and maize. This was evidenced by the greater grain yield of maize after legumes (except for black gram) than maize after maize (Table 4). More specifically, the grain yield of maize was 3,244 kg.ha−1 after groundnut, 3,086 kg.ha−1 after Bambara groundnut, 3,032 kg.ha−1 after cowpea, 2,909 kg.ha−1 after mung bean, 2,582 kg.ha−1 after black gram, and 2,682 kg.ha−1 after maize as preceding crops.
The supply of mineral N to maize plants in the rotation experiment resulted in significantly increased plant accumulation of shoot biomass and whole-plant dry matter (Table 4). Increasing N supply to maize grown after legumes markedly increased shoot biomass, total plant DM, and grain yield relative to the zero-N control (Table 4). In all instances, grain yield was much greater at N60 (3,586 kg.ha−1), followed by N40 (3,197 kg.ha−1) and N20 (2,722 kg.ha−1), and lowest in control plants receiving N0 (2,184 kg.ha−1). Shoot biomass and whole-plant DM followed the same pattern, and were similarly much higher at N60, followed by N40 and N20, and lowest in the control N0 plots (Table 4). In fact, supplying N0 up to N60 to maize plants increased shoot DM from 3,264 to 4,279 kg.ha−1, yield grain from 2,184 to 3,586 kg.ha−1, and whole-plant DM from 5,448 to 7,865 kg.ha−1, which respectively represented a 31, 64, and 44% increase with N fertilizer supply at 0 compared with 60 kg N ha−1.
Symbiotic N benefit in the rotation was assessed by measuring and comparing maize plant growth and yield from the N0 plots of each preceding legume. As shown in Figure 1, shoot biomass, grain yield, and whole-plant DM, respectively, recorded 2,905, 1,671, and 4,576 kg. ha−1 for maize after maize; 3,440, 2,440, and 5,879 kg. ha−1 for maize after Bambara groundnut; 3,558, 2,449, and 6,008 kg. ha−1 for maize after groundnut; 3,229, 2,046, and 5,275 kg. ha−1 for maize after black gram; 3,328, 2,294, and 5,622 kg. ha−1 for maize after cowpea; and 3,214, 2,204, and 5,328 kg. ha−1 for maize after mung bean. The increase in rotation benefit of maize after legume crops in the N0 plots ranged from 8 to 22% for shoot biomass, and 22–47% for grain yield (Figures 1, 2).
Figure 1. A comparison of (A) grain yield, (B) whole-plant DM, and (C) shoot biomass of maize planted after maize with maize after legumes from zero-N plots at Nelspruit, Mpumalanga Province, in the 2012/2013 cropping season. Values in brackets represent % increases in yield of maize after legume relative to maize after maize as the preceding crop in zero-N plots. The horizontal line delineates increases in yield from zero-N plots caused by a preceding legume crop relative to a maize after maize monoculture. (Mz/Mz, maize after maize; Bg/Mz, maize after black gram; Mb/Mz, maize after mung bean; Cp/Mz, maize after cowpea; Bb/Mz, maize after Bambara bean; and Gn/Mz, maize after groundnut).
Figure 2. Interactive effect of preceding crop and N fertilizer level on (A) grain yield, (B) total biomass, and (C) shoot biomass of maize planted in rotation at Nelspruit, Mpumalanga Province during the 2012/2013 cropping season. The horizontal line delineates increases in yield caused by N20, N40, and N60 relative to N0.
The fixed-N benefit of the five preceding grain legumes to the following maize crop was estimated using maize grain yield, shoot biomass, and shoot + grain dry matter yield from zero-N plots (Figures 1, 2). Comparing the grain yield from zero-N plots of maize planted after legumes with grain yield of maize after maize receiving N fertilizer showed that the symbiotic N benefit of preceding legumes to the following maize crop was about 20 kg.ha−1 in fertilizer-N equivalents for each of the test legumes (Figure 2). A similar comparison using whole-plant dry matter yield also showed that the fixed-N benefit was about 20 kg N.ha−1 for the five test legumes, except groundnut, which was 40 kg.ha−1 (Figure 2). Black gram as a preceding legume had little effect on shoot biomass when compared to maize after maize.
Plant harvest index (calculated as grain DM/whole-plant biomass × 100) was much higher for maize planted after mung bean as the preceding crop, followed by cowpea and Bambara groundnut, and lowest for maize after maize, maize after black gram, and maize after groundnut (Table 4). With N supply, however, the plant harvest index was significantly increased, and was greater at N60 and N40, followed by N20, and then N0 (Table 4).
Planting maize after legumes significantly increased maize grain quality relative to maize after maize. As shown in Figure 3, the grain of maize planted after groundnut recorded higher percentage increases in the concentrations of S, Mn, Fe, and Ca over maize after maize. The percentage increase in the grain levels of P, Zn, Mn, Fe, Cu, and Ca were also significant for Bambara groundnut as the preceding crop. Similar % increases in the concentrations of P, Ca, Zn, Mn, and Fe were found in maize grain after black gram as a preceding crop, just as maize after mung bean recorded a marked increase in the levels of P, Ca, Zn, S, Mn, Fe, and Cu in maize grain. Here, Fe and Cu were the only mineral nutrients whose concentrations were increased in the grain of maize grown after cowpea relative to maize after maize (Figure 3).
Figure 3. Percentage increases in mineral nutrient composition of maize grain from zero-N plots of (A) mung bean, (B) Bambara groundnut, (C) black gram, (D) groundnut, and (E) cowpea over maize grain from zero-N plots of maize planted after maize. % mineral A = [(mineral A content of grain from zero-N plots of maize after legume–mineral A content of grain from maize planted after maize from zero-N plots)/(mineral A content of grain from maize grown after maize from zero-N plots)] × 100. Minerals not shown in Figure 3 did not change significantly relative to maize after maize from zero-N plots.
A two-Way ANOVA of selected economic indicators revealed marked differences in profit margins and financial returns on maize grain yield from the positive effect of preceding legume crops. The significantly high maize grain yield after groundnut cultivation led to much greater monetary returns measured in South African currency (Rand) when compared to the other preceding legumes (Table 5). The grain yield was next highest in plots with Bambara groundnut and cowpea as preceding crops, and this also led to higher cash income from grain sale (Table 5). The gross margin, marginal returns, and the cost/benefit ratio of maize grain sale were similar in trend to the monetary value for each preceding crop (Table 5).
Table 5. Two-Way ANOVA of the effect of the preceding crop on economic parameters of maize planted in rotation with four N-levels at Nelspruit in the Mpumalanga Province during the 2012/2013 cropping season.
In this study, all the four economic parameters (i.e., monetary value, gross margin, marginal returns, and cost/benefit analysis) were expectedly and consistently higher with increasing N fertilizer application from N0 to N60 (Table 5). The monetary value of maize grain was thus generally greater with exogenous N supply due to the higher grain yields at increased N levels (Table 5). In contrast, the gross margin (GM) and percentage marginal returns (%MR) on the sale of maize grain was lower at the higher levels of N application due to the high cost of N fertilizers and the greater labor cost of N application when compared to N0 treatment (Figure 4). In essence, the monetary gain as cash income from N contribution by legumes in the cropping system was much higher after cost/benefit analysis than a cereal/cereal system. The increase in financial benefit with regards to percent marginal returns from these legumes over maize after maize at zero-N application was 225, 222, 154, 149, and 108% for groundnut, Bambara groundnut, cowpea, mung bean, and black gram, respectively (Figure 5).
Figure 4. Interaction effect of preceding crop and N fertilizer rate on the (A) monetary value, (B) gross margin, and (C) marginal returns of maize planted in rotation at Nelspruit, Mpumalanga Province during the 2012/2013 cropping season. The horizontal line delineates increases in yield caused by N20, N40, and N60 relative to N0.
Figure 5. A comparison of (A) gross margin, (B) marginal returns, and (C) cost/benefit ratio of maize after legumes with maize after maize at zero-N application at Nelspruit, Mpumalanga Province, in the 2012/2013 cropping season. Values in brackets represent % increases in yield of maize after legume over maize after maize in zero-N plots of the preceding crop. The horizontal line delineates increases in parameter caused by N20, N40, and N60 relative to N0. (Mz/Mz, maize after maize; Bg/Mz, maize after black gram; Mb/Mz, maize after mung bean; Cp/Mz, maize after cowpea; Bb/Mz, maize after Bambara bean; and Gn/Mz, maize after groundnut).
There was a strong and significant correlation between the amount of N-fixed by the preceding legumes and grain yield of the following crop, as well as N-fixed by preceding legumes and plant growth (shoot DM) of the following maize crop (Figure 6). Thus, symbiotic N in legume residues significantly promoted plant growth and grain yield of the subsequent maize crop in rotation.
Figure 6. Relationship between (A) average amount of N-fixed by all preceding legumes vs. maize grain yield from zero-N plots of the five grain legumes, and (B) average amount of N-fixed by all preceding legume and maize shoot DM from zero-N plots of the five grain legumes at Nelspruit, Mpumalanga Province, in the 2012/2013 season.
Smallholder farmers account for over 70% of the crops produced in Africa and their grain yield is generally low due to both biotic and abiotic factors. Inherently high climate variability (Lal, 2004), low soil fertility (Dakora and Keya, 1997), high incidence of diseases, insect pests, and parasitic weeds are a major challenge to food production in Africa (Giles, 2007; Chianu et al., 2012). Nutrient depletion in soil is also a major problem affecting crop production by smallholder and resource-poor farmers in developing countries, where most of the grain legumes are produced. Although fertilizer use could increase crop yields in Africa, only a low 8.8 kg NPK fertilizer is applied per hectare per year due to the high cost and inaccessibility to resource-poor farmers (Henao and Baanante, 2006). Therefore, strategies are needed to develop sustainably green and affordable technologies for use by smallholder and resource-poor farmers in Africa in order to enhance soil productivity and ensure food/nutritional security.
In this study, the symbiotic performance of the five grain legumes studied (namely, Bambara groundnut, black gram, cowpea, groundnut, and mung bean) were evaluated in a field trial at Nelspruit in the Mpumalanga Lowveld of South Africa during the 2011/2012 cropping season as a first step in identifying effective technologies for small-scale farmers. The non-N2-fixing reference plant species used to estimate soil N uptake by the test legumes showed greater δ15N values (Table 2) than the grain legumes, which indicated that the %Ndfa of the legumes studied were reliably estimated using the 15N natural abundance technique (Unkovich et al., 2008).
An assessment of legume performance in the field showed significant differences in plant growth of all the test species, with Bambara groundnut and groundnut exhibiting markedly greater shoot biomass production than the other legumes due to species differences and/or greater shoot N concentration and content. These results are consistent with those of Nyambati et al. (2011), who found a greater increased shoot biomass in Jack bean than hyacinth bean due to high N accumulation in the former.
Shoot δ15N is a measure of symbiotic functioning in nodulated legumes, with low δ15N values indicating high N2 fixation, and greater δ15N depicting low N2 fixation. In this study, groundnut showed significantly higher δ15N values, which resulted in less N derived from atmospheric N2 fixation relative to the other legumes (Table 3). In fact, the %Ndfa was >66% in all the grain legumes, except groundnut which was 45%. As to be expected, this low %Ndfa of groundnut could not meet all of its N requirements. As a result, there was an increased soil N uptake by groundnut, up to 83 kg. ha−1 compared with 3–18 kg.ha−1 for the other legumes that obtained over 65% of their N nutrition from symbiosis. But the 45% N derived from fixation by groundnut was nevertheless within the range reported for this legume in Ghana (32–57%), Zambia (27–70%), and South Africa (23–67%) (Nyemba and Dakora, 2010; Mokgehle et al., 2014; Oteng-frimpong and Dakora, 2018). Based on this study, the inclusion of food legumes in cropping systems of smallholder farmers can be a cost-effective and environmentally safe way of enhancing the N nutrition of both the legume and succeeding crops. The net result is sustainably increased yields with reduced soil N uptake, thus eliminating fertilizer N use, which can increase N2O emission, and hence, global warming.
With climate change, there is a need to select crop species that are drought-tolerant for use in environments with low soil moisture, typically found under rain-fed, dry land conditions in Africa. In C3 plant species, which include members of the Leguminosae, long-term water-use efficiency (or drought tolerance) is commonly measured from analysis of tissue composition of 13C and 12C, the natural isotopes of carbon (Farquhar et al., 1989). In this study, shoot δ13C, which represents a measure of water-use efficiency, ranged from −27.31‰ in mung bean to −26.08‰ in groundnut, a clear indication of significant variations in the water relations of the five legumes tested. The δ13C values obtained here were similar to those reported elsewhere for cowpea (Makoi et al., 2010) and Bambara groundnut (Mohale et al., 2014). Furthermore, Bambara groundnut and groundnut, which showed much greater δ13C values (−26.44‰ and −26.08‰, respectively), contributed the highest N to the cropping system, while legume species with the least δ13C (low water-use efficiency), made much smaller N contributions (Table 3). These findings suggest a functional relationship between symbiotic N nutrition and water-use efficiency in nodulated legumes. The significantly greater δ13C values of Bambara groundnut and groundnut (i.e., better water-use efficiency) was strongly linked to the markedly high shoot N concentration and N content, as well as the increased amount of N-fixed by these legumes, which together supported greater plant growth and biomass accumulation (Table 3). These findings could prove useful when selecting legume species for enhanced N2 fixation and tolerance to drought for use in a changing climate.
Crop rotation is an ancient practice used for sustaining soil productivity, and involves planned successive cultivation of different crops in a specific order on the same field (Karlen et al., 1994). Legumes generally meet their N requirements from symbiotic N2 fixation, while improving the N nutrition of associated non-legume crops either through N transfer (Eaglesham et al., 1981), enhancing the N-sparing effect of soil N by the legume, and/or increasing the release of symbiotic N by residues to following crops (Angus et al., 2006; Lithourgidis et al., 2011; Espinoza et al., 2012). Several studies have found substantial N contribution by legumes to subsequent crops, which led to increased plant growth and grain yield of the following cereal crops (Dakora et al., 1987; Angus et al., 2014; Kirkegaard and Ryan, 2014; Abdel-Galil et al., 2016). The inclusion of symbiotic legumes in cropping systems can therefore help to reduce increased use of N fertilizers in global agriculture, and thus decrease N2O gas emissions and global warming, thereby mitigating climate change (Dudeja and Duhan, 2005; Sengupta et al., 2015; Stewart, 2015).
A comparison of the effect of symbiotic N contribution by the five food legumes to the following maize crop in this study revealed a significant increase in plant growth, shoot biomass, and grain yield of maize planted after legumes relative to maize after maize. The increase in grain yield of maize after legumes was 769, 777, 623, 533, and 375 kg.ha−1, respectively, for Bambara groundnut, groundnut, cowpea, mung bean, and black gram from zero-N plots. The positive effect of legumes was also evident from the shoot biomass of maize planted after legumes. The increase in shoot dry matter yield was 535, 653, 423, 324, and 219 kg.ha−1, respectively, for Bambara groundnut, groundnut, cowpea, mung bean, and black gram when the zero-N treatments were compared for all five legumes. These increases in maize biomass and grain yield could be attributed to the quantum of symbiotic N in legume residues. This was evidenced by the significant correlations found between the mean amount of N-fixed by all preceding legume vs. plant growth (shoot biomass) or grain yield of the following maize crop (Figure 6). Although the soil samples from the maize rotation were mixed up and therefore not analyzed in this study, reports from the same environment revealed soil N was 3.2–4.2% after pigeon pea and 1.0% after sole maize in 2009 (Mathew and Dakora, unpubl. data). These increases could, however, also be attributed to the inclusion of legumes in the cropping system which improved soil fertility and chemical characteristics (McCallum et al., 2004), and probably altered the populations of specific microbes in the rhizosphere to the benefit of the following crops (Osborne et al., 2000; Kirkegaard et al., 2008). As a result, cereal yields after legumes are often 40–80% greater than cereal after cereal without N fertilizer, and these increases can be as high as 450–1,000 kg of additional grain yield per hectare (Seymour et al., 2012). In fact, Dakora et al. (1987) found 89 and 95% increases in grain yield from the zero-N plots of maize after cowpea and groundnut as preceding crops when compared to a maize after maize monoculture. In this study, the increase in grain yield ranged from 375 to 777 kg.ha−1, a finding consistent with the results of maize grain yield after groundnut and cowpea in Ghana (Dakora et al., 1987), or after mung bean, black gram, and soybean in Pakistan (Malik et al., 2006; Naveed et al., 2017).
Furthermore, this study revealed marked differences between and among the test legumes in their ability to promote yield increases of maize planted after legumes. As preceding crops, Bambara groundnut and groundnut, for example, caused greater increase in maize yield than the other legumes (Malik et al., 2006; Naveed et al., 2017). In contrast, there was a small increase in grain yield where maize was planted after black gram, a response similar to maize after maize. These differences in the effect of preceding legumes on maize plant growth and grain yield were likely due to the C/N ratios of the test legume species (Table 3). For example, even though Bambara groundnut and groundnut showed much greater shoot biomass, the two species also recorded significantly higher shoot N concentration, N content, and amounts of N-fixed (Table 3). As a result, Bambara groundnut and groundnut revealed the lowest C/N ratios among the five legumes tested (Table 3). It has, however, been known for a long time that plant residues with low C/N ratios tend to decompose faster under warm tropical conditions (Marschner, 1995; Nicolardot et al., 2001), leading to increased release of N and other mineral nutrients for uptake by subsequent crops. In this study, the low C/N ratio in shoots of Bambara groundnut and groundnut probably led to their increased decomposition and greater release of N and other nutrient elements for uptake by the maize crop following Bambara groundnut and groundnut. This would explain the better growth and increased grain yield of maize after Bambara groundnut and groundnut as preceding crops (Table 4). This argument is re-enforced by the results of mineral analysis of maize grain, which showed that maize after Bambara groundnut accumulated more mineral nutrients in grain (P, Ca, Fe, Mn, Zn, and Cu), in the same way that maize after groundnut showed greater mineral concentration (Ca, S, Fe, and Mn) in its grain (Figure 3).
The increase in maize grain yield caused by exogenous N supply relative to the zero-N treatment in this study was 355, 711, and 1,101 kg.ha−1 for 20, 40, and 60 kg N.ha−1. Nitrogen fertilization thus resulted in 11, 22, and 34% increase in grain yield from supplying 20, 40, and 60 kg N ha−1, respectively, when compared to the zero-N plots, which contained only symbiotic and endogenous soil N. The consistent increase in grain yield with increasing supplemental N suggests that N from the preceding legumes' residues was alone inadequate at meeting the N demand of the following maize crop to produce economic yields (Supplementary Table 1). The same could be said of the shoot + grain dry matter yield (Supplementary Table 1). The increase in plant growth with exogenous N supply was similar in pattern to grain yield, which confirmed the relationship between dry matter accumulation and grain production (Zhaosu, 1993; Zhang et al., 2008). Based on the grain yield, shoot biomass, and whole-plant dry matter, the symbiotic N benefit of legumes to the succeeding maize crop in rotation was estimated to be between 20 and 40 kg N.ha−1 fertilizer equivalents (Supplementary Table 1 and Figure 2). These results are consistent with the findings of previous studies, which also found 20–60 kg N.ha−1 fertilizer equivalent when maize was planted as a following crop after grain legumes (Dakora et al., 1987; Myaka et al., 2006).
For resource-poor farmers in Africa, 375–777 kg extra grain yield per hectare from the inclusion of grain legumes in the cropping system represents a substantial increase in household food security from BNF technology, which should be tapped for farmers' use, especially with the current high cost of N fertilizers. The monetary value of including legumes in the cropping system was also estimated, and found to be substantial, especially when measured against the commonly practiced cereal-after-cereal rotation of smallholder farmers in Africa. The increase in marginal returns recorded in this study was 222, 225, 154, 149, and 108% for Bambara groundnut, groundnut, cowpea, mung bean, and black gram, respectively, over a monoculture of maize after maize without N fertilizer (Figure 5). The monetary value of maize after legumes was significantly greater because of the higher maize grain yield produced in the zero-N plots of maize after legumes when compared to the zero-N treatment of maize after maize (Table 5). Although the maize grain yield was significantly higher with increased N supply, which resulted in a greater cash income for farmers, the percentage marginal returns from grain yield were markedly lower when the variable costs of fertilizer and labor were included (Table 5 and Figure 4).
The legume/cereal rotation in this study also significantly improved the nutritional quality of maize planted after legumes. Whether produced as food for human consumption or feed for livestock, the grain from maize grown after legumes showed significant percentage increases in the concentrations of dietarily-important mineral nutrients relative to maize after maize (Figure 3). A number of studies have similarly found an increase in the levels of protein and nutritionally-important mineral nutrients in the grain of cereal crops planted after legumes (Hauggaard-Nielsen et al., 2006; Lithourgidis and Dordas, 2010). Taken together, this study has demonstrated that the inclusion of nodulated grain legumes as biofertilizers in cropping systems in Africa can significantly increase the grain yield of a following cereal crop, enhance the nutritional quality of maize grain, and overcome food insecurity of small-scale farmers, in addition to raising their household cash-income levels in a sustainable and environmentally-friendly manner.
Taken together, this study has demonstrated that the inclusion of nodulated grain legumes as biofertilizers in cropping systems in Africa can significantly increase the grain yield of a following cereal crop, enhance the nutritional quality of maize grain, and overcome food insecurity of small-scale farmers, in addition to raising the levels of their household cash-income in a sustainable and environmentally-friendly manner. The results of this study have provided impetus for conducting similar studies on traditionally neglected and under-researched African food legumes such as the Kersting's groundnut, African yam bean, and mucuna (velvet bean).
All datasets generated for this study are included in the article/Supplementary Material.
DL conducted the field experiments, collected and prepared plant samples for isotopes and nutrient analyses, and took part in drafting the manuscript. CM assisted in plant sampling, data analysis, and took part in drafting the manuscript. FD was the supervisor of DL, conceptualized the work, edited the final manuscript, and provided funding for the work. All authors contributed to the article and approved the submitted version.
We are grateful to the South African Research Chair in Agrochemurgy and Plant Symbioses, the National Research Foundation, and the Tshwane University of Technology for financial support to FDD's research, and for a SARCHI Chair support to DML's master's studies.
The authors declare that the research was conducted in the absence of any commercial or financial relationships that could be construed as a potential conflict of interest.
The Supplementary Material for this article can be found online at: https://www.frontiersin.org/articles/10.3389/fsufs.2020.00094/full#supplementary-material
Abdel-Galil, E., Rizk, H., and Mostafa, A. (2016). Production and characterization of activated carbon from leucaena plant wastes for removal of some toxic metal ions from waste solutions. Desalin. Water Treat. 57, 17880–17891. doi: 10.1080/19443994.2015.1102768
Angus, A. A., Agapakis, C. M., Fong, S., Yerrapragada, S., Estrada-de Los Santos, P., Yang, P., et al. (2014). Plant-associated symbiotic Burkholderia species lack hallmark strategies required in mammalian pathogenesis. PLoS ONE 9:e83779. doi: 10.1371/journal.pone.0083779
Angus, J., Kirkegaard, J., and Proples, M. (2006). Nitrogen mineralization in relation to previous crops and pastures. Aust. J. Soil Res. 44, 355–365. doi: 10.1071/SR05138
AOAC (1990). Association of Official Analytical Chemists. Official Methods of Analysis 15th Edn. Washington, DC.
Ataro, A., McCrindle, R. I., Botha, B. M., McCrindle, C. M. E., and Ndibewu, P. P. (2008). Quantification of trace elements in raw cow's milk by inductively coupled plasma mass spectrometry (ICP-MS). Food Chem. 111, 243–248. doi: 10.1016/j.foodchem.2008.03.056
Belane, A. K., and Dakora, F. D. (2010). Symbiotic N2 fixation in 30 field-grown cowpea (Vigna unguiculata L. Walp.) genotypes in the Upper West Region of Ghana measured using 15N natural abundance. Biol. Fertil. Soils 46, 191–198. doi: 10.1007/s00374-009-0415-6
Black, C., Evans, D., and Dinauer, R. (1965). Methods of Soil Analysis, 9th Edn. Madison, WI: American Society of Agronomy. doi: 10.2134/agronmonogr9.1
Chianu, J., Chianu, J., and Mairura, F. (2012). Mineral fertilizers in the farming systems of Sub-Saharan Africa. Agron. Sustain. Dev. 32, 545–566. doi: 10.1007/s13593-011-0050-0
Chibeba, M. A., Kyei-boahen, S., Guimarães, M. D. F., Nogueira, A. M., and Hungria, M. (2017). Isolation, characterization and selection of indigenous Bradyrhizobium strains with outstanding symbiotic performance to increase soybean yields in Mozambique. Agric. Ecosyst. Environ. 246, 291–305. doi: 10.1016/j.agee.2017.06.017
Chidebe, I. N., Jaiswal, S. K., and Dakora, F. D. (2018). Distribution and phylogeny of microsymbionts associated with cowpea (Vigna unguiculata) nodulation in three agroecological regions of mozambique. Appl. Environ. Microbiol. 84, e01712–e01717. doi: 10.1128/AEM.01712-17
Craig, H. (1957). Isotopic standards for carbon and oxygen and correction factors for mass-spectrometric analysis of carbon dioxide. Geochim. Cosmochim. Acta 12, 133–149. doi: 10.1016/0016-7037(57)90024-8
DAFF (2018). Trends in the Agricultural Sector 2017, Directorate Communication Services. Pretoria: South Africa. Available online at: https://www.daff.gov.za/Daffweb3/Portals/0/Statistics%20and%20Economic%20Analysis/Statistical%20Information/Trends%20in%20the%20Agricultural%20Sector%202017.pdf (accessed July 28, 2020).
Dakora, F. D., Aboyinga, R. A., Mahama, Y., and Apaseku, J. (1987). Assessment of N2 fixation in groundnut (Arachis hypogaea L.) and cowpea (Vigna unguiculata L. Walp) and their relative N contribution to a succeeding maize crop in Northern Ghana. MIRCEN J. Appl. Microbiol. Biotechnol. 3, 389–399. doi: 10.1007/BF00935697
Dakora, F. D., and Keya, S. O. (1997). Contribution of legume nitrogen fixation to sustainable agriculture in Sub-Saharan Africa. Soil Biol. Biochem. 29, 809–817. doi: 10.1016/S0038-0717(96)00225-8
Donald, C., and Hamblin, J. (1976). The biological yield and harvest index of cereals as agronomic and plant breeding criteria. Adv. Agron. 28, 361–405. doi: 10.1016/S0065-2113(08)60559-3
Dudeja, S., and Duhan, J. (2005). Biological nitrogen fixation research in pulses with special reference to mung bean and urd bean. Indian J. pulses Res. 18:107.
Eaglesham, A., Ayanaba, A., Rao, V., and Eskew, D. (1981). Improving the nitrogen nutrition of maize by intercropping with cowpea. Soil Biol. Biochem. 13, 169–171.
Espinoza, S., Ovalle, C., Zagal, E., Matus, I., Tay, J., Peoples, M., et al. (2012). Contribution of legumes to wheat productivity in mediterranean environments of central Chile. Field Crop. Res. 133, 150–159. doi: 10.1016/j.fcr.2012.03.006
Farquhar, G. D., Ehleringer, J. R., and Hubick, K. T. (1989). Carbon isotope discrimination and photosynthesis. Annu. Rev. Plant Physiol. Plant Mol. Biol. 40, 503–537. doi: 10.1146/annurev.pp.40.060189.002443
Giles, J. (2007). Climate change 2007: how to survive a warming world. Nature 446, 716–717. doi: 10.1038/446716a
Gomez, K. A., and Gomez, A. A. (1984). Statistical Procedures for Agricultural Research. New York, NY: John Wiley and Sons.
GRAIN, S. (2013). Internal Statistic Database. Available online at: https://www.grainsa.co.za/sa-grain?i=90 (accessed July 28, 2020).
Gyogluu, C., Jaiswal, S. K., Kyei-boahen, S., and Dakora, F. D. (2018). Identification and distribution of microsymbionts associated with soybean nodulation in mozambican soils. Syst. Appl. Microbiol. 41, 506–515. doi: 10.1016/j.syapm.2018.05.003
Hauggaard-Nielsen, H., Trydeman Knudsen, M., Ravn Jørgensen, J., and Steen Jensen, E. (2006). “Intercropping wheat with pea for improved wheat baking quality,” in Joint Organic Congress (Denmark: Odense). Available online at: https://orgprints.org/7471/ (accessed July 28, 2020).
Henao, J., and Baanante, C. (2006). Agricultural Production and Soil Nutrient Mining in Africa: Implications for Resource Conservation and Policy Development. Muscle Shoals, AL: An International Center for Soil Fertility and Agricultural Development.
Junk, G., and Svec, H. (1958). The absolute abundance of the nitrogen isotopes in the atmosphere and compressed gas from various sources. Geochim. Cosmochim. Acta 14, 234–243. doi: 10.1016/0016-7037(58)90082-6
Karlen, D., Varvel, G., Bullock, D., and Cruis, R. (1994). Crop rotations for the 21st century. Adv. Agron. 53, 1–45. doi: 10.1016/S0065-2113(08)60611-2
Kirkegaard, J., Christen, O., Krupinsky, J., and Layzell, D. B. (2008). Break crop benefits in temperate wheat production. Field Crop. Res. 107, 185–195. doi: 10.1016/j.fcr.2008.02.010
Kirkegaard, J., and Ryan, M. (2014). Magnitude and mechanisms of persistent crop sequence effects on wheat. Field Crop. Res. 164, 154–165. doi: 10.1016/j.fcr.2014.05.005
Lal, R. (2004). Soil carbon sequestration impacts on global climate change and food security. Science 304, 1623–1627. doi: 10.1126/science.1097396
Lithourgidis, A., and Dordas, C. (2010). Forage yield, growth rate, and nitrogen uptake of faba bean intercrops with wheat, barley, and rye in three seeding ratios. Crop Sci. 50, 2148–2158. doi: 10.2135/cropsci2009.12.0735
Lithourgidis, A. S., Dordas, C. A., Damalas, C. A., and Vlachostergios, D. N. (2011). Annual intercrops: an alternative pathway for sustainable agriculture. Aust. J. Crop Sci. 5, 396–410.
Makoi, J. H. J. R., Bambara, S., Ndakidemi, P. A., Box, P. O., Town, C., and Africa, S. (2010). Rhizosphere phosphatase enzyme activities and secondary metabolites in plants as affected by the supply of rhizobium, lime and molybdenum in Phaseolus vulgaris L. Aust. J. Crop Sci. 4, 590–597.
Malik, R., Charles, C., Elise, P., and David, M. (2006). A global experimental dataset for assessing grain legume production. Sci. Data 3, 1–20. doi: 10.1038/sdata.2016.84
Mariotti, A. (1983). Atmospheric nitrogen is a reliable standard for natural 15N abundance measurements. Nature 303, 685–687. doi: 10.1038/303685a0
Maskey, S. L., Bhattarai, S., Peoples, M. B., and Herridge, D. F. (2001). On-farm measurements of nitrogen fixation by winter and summer legumes in the hill and terai regions of nepal. Field Crop. Res. 70, 209–221. doi: 10.1016/S0378-4290(01)00140-X
Mathews, C., and Beck, B. D. A. (1994). “Evaluation of foliar diseases resistant ICRISAT groundnut varieties in KaNgwane, South Africa,” in Sustainable groundnut production in Southern and Eastern Africa: Proceedings of a Workshop, 5–7 Jul 1994, Mbabane, Swaziland: ICRISAT, Patancheru 502 324, Andhra Pradesh, India, eds B. J. Ndunguru, G. L. Hilderbrand, and P. Subrahmanyam, 73–78. Available online at: http://oar.icrisat.org/1014/1/RA_00250.pdf (accessed July 28, 2020).
McCallum, M., Kirkegaard, J., Green, T., Cresswell, H., Davies, S., and Angus, J. (2004). Improved subsoil macro-porosity following perennial pastures. Aust. J. Exp. Agric. 44, 299–307. doi: 10.1071/EA03076
Mohale, K. C., Belane, A. K., and Dakora, F. D. (2014). Symbiotic N nutrition, C assimilation, and plant water use efficiency in Bambara groundnut (Vigna subterranea L. Verdc) grown in farmers' fields in South Africa, measured using 15N and 13C natural abundance. Biol. Fertil. Soils 50, 307–319. doi: 10.1007/s00374-013-0841-3
Mohammed, M., Jaiswal, S. K., and Dakora, F. D. (2018). Distribution and correlation between phylogeny and functional traits of cowpea (Vigna unguiculata L. Walp.)-nodulating microsymbionts from Ghana and South Africa. Sci. Rep. 8:18006. doi: 10.1038/s41598-018-36324-0
Mohammed, M., Jaiswal, S. K., and Dakora, F. D. (2019). Insights into the phylogeny, nodule function and biogeographic distribution of microsymbionts nodulating the orphan Kersting's groundnut [Macrotyloma geocarpum (Harms) Marechal and Baudet] in African soils. Appl. Environ. Microbiol. 85, 1–21. doi: 10.1128/AEM.00342-19
Mokgehle, S. N., Dakora, F. D., and Mathews, C. (2014). Variation in N2 fixation and N contribution by 25 groundnut (Arachis hypogaea L.) varieties grown in different agro-ecologies, measured using15N natural abundance. Agric. Ecosyst. Environ. 195, 161–172. doi: 10.1016/j.agee.2014.05.014
Myaka, F., Sakala, W., Adu-Gyamfi, J., Kamalongo, D., Ngwira, A., Odgaard, R., et al. (2006). Yields and accumulations of N and P in farmer-managed intercrops of maize–pigeonpea in semi-arid Africa. Plant Soil 285, 207–220. doi: 10.1007/s11104-006-9006-6
Naveed, M., Aziz, M., and Yaseen, M. (2017). “Perspectives of using endophytic microbes for legume improvement,” in Microbes for Legume Improvement (Switzerland: Springer, Cham), 277–299. doi: 10.1007/978-3-319-59174-2_12
Ngwira, A., Aune, J., and Mkwinda, S. (2012). On-farm evaluation of yield and economic benefit of short term maize legume intercropping systems under conservation agriculture in Malawi. Field Crop. Res. 132, 149–157. doi: 10.1016/j.fcr.2011.12.014
Nicolardot, B., Recous, S., and Mary, B. (2001). Simulation of C and N mineralisation during crop residue decomposition: a simple dynamic model based on the C:N ratio of the residues. Plant Soil 228, 83–103. doi: 10.1023/A:1004813801728
Nyambati, E., Lusweti, C., Muyekho, F., and Mureithi, J. (2011). Up-scaling napier grass (Pennisetum purpureum Schum.) production using tumbukiza method in smallholder farming systems in north western Kenya. J. Agric. Ext. Rural Dev. 3, 1–7.
Nyemba, R. C., and Dakora, F. D. (2010). Evaluating N2 fixation by food grain legumes in farmers' fields in three agro-ecological zones of Zambia, using 15N natural abundance. Biol. Fertil. Soils 46, 461–470. doi: 10.1007/s00374-010-0451-2
Osborne, E., Gioria, M., and Pysek, P. (2000). The legacy of plant invasions: changes in the soil seed bank of invaded plant communities. Bioscience 66, 40–53. doi: 10.1093/biosci/biv165
Oteng-frimpong, R., and Dakora, F. D. (2018). Selecting elite groundnut (Arachis hypogaea L) genotypes for symbiotic N nutrition, water-use efficiency and pod yield at three field sites, using 15N and 13C natural abundance. Symbiosis 75, 229–243. doi: 10.1007/s13199-017-0524-1
Pausch, R. C., Mulchi, C. L., Lee, E. H., and Meisinger, J. J. (1996). Use of 13C and 15N isotopes to investigate O3 effects on C and N metabolism in soybeans. Part II. Nitrogen uptake, fixation, and partitioning. Agric. Ecosyst. Environ. 60, 61–69. doi: 10.1016/S0167-8809(96)01062-6
Puozaa, D. K., Jaiswal, S. K., and Dakora, F. D. (2017). African origin of Bradyrhizobium populations nodulating bambara groundnut (Vigna subterranea L. Verdc) in Ghanaian and South African soils. PLoS ONE 12:e0184943. doi: 10.1371/journal.pone.0184943
Puozaa, D. K., Jaiswal, S. K., and Dakora, F. D. (2019). Phylogeny and distribution of Bradyrhizobium symbionts nodulating cowpea (Vigna unguiculata L. Walp) and their association with the physicochemical properties of acidic African soils. Syst. Appl. Microbiol. 42, 403–414. doi: 10.1016/j.syapm.2019.02.004
SCWG (1991). Soil Classification: A Taxonomic System for South Africa. Pretoria: Dept. of Agricultural Development.
Sengupta, A., Mallick, A., Gunri, S., Puste, A., and Roy, S. (2015). Performance of summer groundnut (Arachis hypogaea L.) as influenced by irrigation: a climatological approach of water engineering towards improved water use efficiency under new alluvial sub-tropics,” in International Conference on Recent Innovation in Civil Engineering for Sustainable Development (IICSD-2015) (Gazipur, Bangladesh: Department of Civil Engineering DUET).
Seymour, M., Kirkegaard, J., Peoples, M., White, P., and French, R. (2012). Break-crop benefits to wheat in Western Australia–insights from over three decades of research. Crop Pasture Sci. 63, 1–16. doi: 10.1071/CP11320
Shearer, G., and Kohl, D. H. (1986). N2-fixation in field settings: estimations based on natural 15N abundance. Aust. J. Plant Physiol. 13, 699–756. doi: 10.1071/PP9860699c
Sinclair, T. R., and Vadez, V. (2012). The future of grain legumes in cropping systems. Crop Pasture Sci. 63, 501–512. doi: 10.1071/CP12128
Statsoft Inc. (2011). Statistica (Data Analysis Software System). Version 10. Available online at: www.statsoft.com
Stewart, J. R. (2015). Agave as a model CAM crop system for a warming and drying world. Front. Plant Sci. 6:684. doi: 10.3389/fpls.2015.00684
Unkovich, M., Herridge, D., Peoples, M., Cadisch, G., Boddey, B., Giller, K., et al. (2008). Measuring Plant-Associated Nitrogen Fixation in Agricultural Systems. Canberra, ACT: Australian Centre for International Agricultural Research.
Van der Merwe, A., Johnson, J., and Ras, L. (1984). “A NH4HCO3-NH4F-(NH4) 2-EDTA-method for the determination of extractable P, K, Ca, Mg, Cu, Fe, Mn and Zn in soils,” in SIR Information Bulletin B2/2, Soil Science Society of South Africa (Pretoria, South Africa).
Von Loeper, W., Musango, J., Brent, A., and Drimie, S. (2016). Analysing challenges facing smallholder farmers and conservation agriculture in South Africa: a system dynamics approach. South Afr. J. Econ. Manag. Sci. 19, 747–773. doi: 10.4102/sajems.v19i5.1588
Walkley, A., and Black, I. A. (1934). An examination of the degtjareff method for determining soil organic matter, and a proposed modification of the chromic acid titration method. Soil Sci. 37, 29–38. doi: 10.1097/00010694-193401000-00003
Walley, F., Clayton, G., Miller, P., Carr, P., and Lafond, G. (2007). Nitrogen economy of pulse crop production in the northern great plains. Agron. J. 99, 1710–1718. doi: 10.2134/agronj2006.0314s
Zhang, X., Chen, S., Sun, H., Pei, D., and Wang, Y. (2008). Dry matter, harvest index, grain yield and water use efficiency as affected by water supply in winter wheat. Irrig. Sci. 27, 1–10. doi: 10.1007/s00271-008-0131-2
Zhaosu, C. (1993). The relations of dry matter accumulation of grain growth stages to grain weight in wheat. J. Nanjing Agric. Univ. 16: 27–32.
Keywords: grain legumes, N2 fixation, biofertilizers, crop rotation, grain nutritional quality, mineral nutrients, household cash income, marginal returns
Citation: Lengwati DM, Mathews C and Dakora FD (2020) Rotation Benefits From N2-Fixing Grain Legumes to Cereals: From Increases in Seed Yield and Quality to Greater Household Cash-Income by a Following Maize Crop. Front. Sustain. Food Syst. 4:94. doi: 10.3389/fsufs.2020.00094
Received: 13 November 2019; Accepted: 26 May 2020;
Published: 19 August 2020.
Edited by:
José Laércio Favarin, University of São Paulo, BrazilReviewed by:
Tahira Fatima, Purdue University, United StatesCopyright © 2020 Lengwati, Mathews and Dakora. This is an open-access article distributed under the terms of the Creative Commons Attribution License (CC BY). The use, distribution or reproduction in other forums is permitted, provided the original author(s) and the copyright owner(s) are credited and that the original publication in this journal is cited, in accordance with accepted academic practice. No use, distribution or reproduction is permitted which does not comply with these terms.
*Correspondence: Felix D. Dakora, RGFrb3JhRkRAdHV0LmFjLnph
Disclaimer: All claims expressed in this article are solely those of the authors and do not necessarily represent those of their affiliated organizations, or those of the publisher, the editors and the reviewers. Any product that may be evaluated in this article or claim that may be made by its manufacturer is not guaranteed or endorsed by the publisher.
Research integrity at Frontiers
Learn more about the work of our research integrity team to safeguard the quality of each article we publish.