- 1Department of Ecology and Evolutionary Biology, Cornell University, Ithaca, NY, United States
- 2Charles H. Dyson School of Applied Economics and Management, Cornell University, Ithaca, NY, United States
Most on-farm diversification strategies to enhance ecosystem services, such as insect pest control and yield, have focused on expanding crop species diversity. While polycultures often provide valuable services, logistical constraints with planting and harvesting can hamper implementation on large scales. An alternative diversification strategy is to increase within-field intraspecific crop diversity through the use of crop varietal mixtures. Here, we evaluate an interdisciplinary body of research to determine the potential for crop varietal mixtures to support food security by providing ecological, economic, and nutritional services. Previous literature has synthesized the link between varietal mixtures and yield and insect pest suppression services. We expand on prior analyses by considering hypotheses generated from species-level research and assessing whether they also provide a useful framework for predicting how varietal mixtures affect crop productivity and insect pest suppression. In addition, we evaluate the potential for varietal mixtures to increase farm resilience and growers' profits. While there is a growing effort to quantify the economic value of ecosystem services provided by agrobiodiversity in terms of enhanced yield or revenue, much less attention has been given to quantifying the production costs associated with diversification schemes. Consequently, we know little about the effect of diversification practices on farm profitability, the metric of ultimate importance to farmers. We address this issue by evaluating the ability of varietal mixtures to reduce production costs associated with other types of agrobiodiversity and outline areas for future research to better understand the profit implications of varietal mixtures. Further, we review evidence that varieties of some crop species differ in phytochemical content—a functional trait important for insect pest suppression and human dietary diversity—suggesting that varietal mixtures could be designed to simultaneously support insect pest control and human nutrition services. Given that little research has explicitly addressed the capacity for varietal mixtures to support human nutrition, we outline predictions for where we would expect to see the greatest nutritional impact of mixtures, providing a foundation for future human nutrition research. Taken together, our review suggests that varietal mixtures are a promising and logistically feasible strategy that could simultaneously support multiple services.
Introduction
A large body of literature indicates agrobiodiversity (Jackson et al., 2007) can improve food security by sustaining a broad range of ecosystem services, such as insect pest suppression and crop productivity, which in turn provide economic and nutritional benefits to humans (Bianchi et al., 2006; Power, 2010; Letourneau et al., 2011; Tscharntke et al., 2012a; Figure 1). While agrobiodiversity encompasses multiple levels of diversity, ranging from landscape diversity to intraspecific crop diversity (diversity within a crop species), most efforts to capitalize on the ecological, economic, and nutritional aspects of agricultural systems through diversification have focused on enhancing crop species diversity. For example, push-pull agroecosystems in Sub-Saharan Africa manipulate crop species diversity by intercropping maize, an important staple food crop, with desmodium (Desmodium uncinatum) and Napier grass (Pennisetum purpureum) to enhance insect pest control services and crop productivity, resulting in improved human nutrition and economic returns (Khan et al., 2008). While in many instances polycultures—defined here as intermixing multiple crop species together in a field—is a successful practice (Poveda et al., 2008; Letourneau et al., 2011), they can pose logistical challenges for growers depending on the scale of mixing (Figure 1). Although strip cropping can be mechanized, mixing crop species within rows or in alternating rows is typically not compatible with mechanized agricultural equipment (Tooker and Frank, 2012; Reiss and Drinkwater, 2018). Polycultures are also likely to require more agronomic knowledge than monocultures because crop species differ in their planting times, management, equipment needs, and marketability (Gliessman, 1985). Therefore, alternative approaches to agricultural diversification could offer benefits to growers.
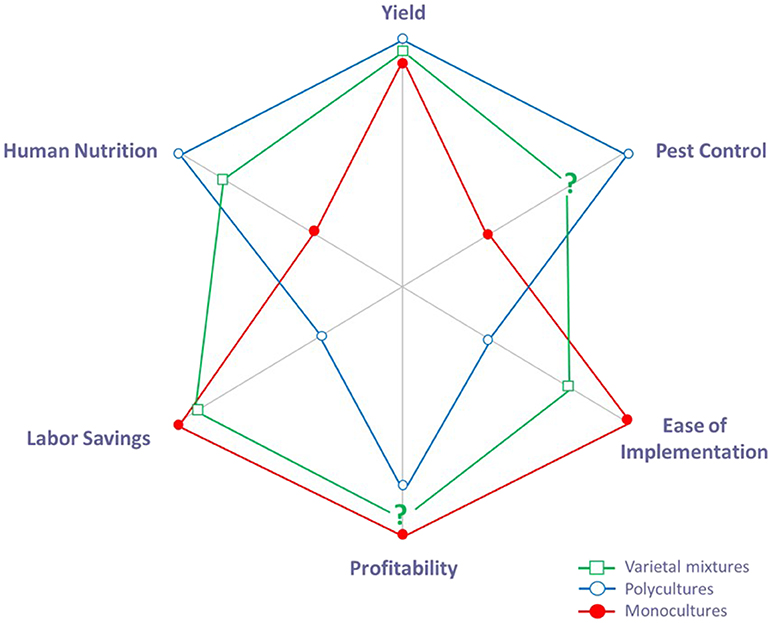
Figure 1. Conceptual framework for comparing the services (human nutrition, yield stability, insect pest control) and economic implications (labor, implementation effort, profits) associated with agricultural management practices. In this qualitative diagram, the level of service or economic implication is indicated along each axis; achieving greater distance along each axis indicates a stronger benefit. To illustrate predictions for how services and economic implications will vary with the level of diversification, we compare three hypothetical agriculture systems: a monoculture, growing a single crop variety in a field (closed circle); a polyculture, intermixing multiple crop species together in a field (open circle); and a varietal mixture, planting multiple varieties of the same crop species together in a field (open square). In general, polycultures enhance many services (Poveda et al., 2008; Letourneau et al., 2011), but production costs can be high (Gliessman, 1985; Tooker and Frank, 2012). In contrast, monocultures minimize costs, but are poor producers of some services (Altieri, 1999; Karp et al., 2012). We propose varietal mixtures could serve as an intermediate strategy that addresses some of the limitations associated with monocultures and polycultures. The symbol “?” represents predictions with the least amount of supporting evidence.
An alternative diversification strategy that could be employed when polycultures are impractical is to increase intraspecific crop diversity within a farm field by planting multiple varieties of the same crop species. Varietal mixtures have been used quite extensively in disease control programs (Mundt, 2002), yet less consideration has been given to their ability to suppress insect pests (Tooker and Frank, 2012), and to provide the economic and nutritional benefits that can be associated with polycultures. In this review, we evaluate the potential of varietal mixtures to serve as a practical, intermediate diversification strategy to create multifunctional agroecosystems that simultaneously support the ecological, economic, and nutritional components of agriculture (Figure 1). While we recognize the potential for varietal mixtures to improve ecosystem services in many cropping systems, including perennial cropping systems and agroforestry, we focus this discussion on annual crops grown for human consumption, as these are the systems in which most of the research on varietal mixtures has been conducted.
Although much remains to be explored, there are several lines of evidence to suggest varietal mixtures could be a viable diversification tactic for growers. For instance, a growing number of studies indicate that varietal mixtures can support insect pest suppression (Tooker and Frank, 2012; Koricheva and Hayes, 2018) and even more studies have demonstrated the beneficial impact of varietal mixtures on crop productivity and yield stability (Smithson and Lenné, 1996; Finckh et al., 2000; Kiær et al., 2009; Borg et al., 2018; Reiss and Drinkwater, 2018). In addition, varietal mixtures may improve agroecosystem resilience by helping to buffer crop production from external shocks and by reducing some of the management complications and labor requirements associated with polycultures (Wilhoit, 1992; Lin, 2011). Many crop species encompass a broad range of trait variation and preserving these sources of genetic variation could be important for the development of future crop varieties. By maintaining desirable crop traits such as drought tolerance or disease resistance, farmers would have the tools to adapt to a range of environmental constraints associated with variable climatic or biotic stresses (Belem et al., 2018). Furthermore, varieties of many crop species differ in their phytochemical content (Grusak and DellaPenna, 1999; Toledo and Burlingame, 2006), compounds important for plant defense as well as for human nutrition, which suggests that varietal mixtures could be designed to simultaneously support insect pest suppression and enhance human dietary diversity.
Our objective is to link typically disparate topics in the same review to promote interdisciplinary analysis that can lead to the design of multifunctional agroecosystems. To do so, we integrate perspectives from ecology, economics, and nutrition to evaluate the potential for varietal mixtures to simultaneously support several services that are important to food security. We build on previous research exploring impacts of intraspecific crop diversity on yield and insect pest suppression services (Tooker and Frank, 2012; Koricheva and Hayes, 2018; Reiss and Drinkwater, 2018), and expand to include economic (e.g., profitability and yield stability) and human nutrition services. Although many ecological services contribute to food security, such as soil health, pollination (e.g., Klein et al., 2007; Gallai et al., 2009; Garibaldi et al., 2011), and disease control (e.g., Power, 1991; Zhu et al., 2000; Mundt, 2002), in this discussion we focus on insect pest suppression and yield services. We map out areas for future research by highlighting knowledge gaps in our understanding of how varietal mixtures influence agroecosystem services that generate economic and nutritional benefits vital to food security.
Consequences of Conventional Agricultural Intensification
Planting fields with one high-yielding crop variety has become the prevailing solution for providing food to a growing human population (Pingali, 2012). Yet, this practice has eroded valuable genetic resources that are foundational to creating resilient agroecosystems, has resulted in large areas of land dedicated to a relatively small number of crop species, and has threatened pollination services and biodiversity (Altieri, 1999; Karp et al., 2012). The lack of genetic diversity in monocultures often translates into enhanced vulnerability to abiotic and biotic stressors. Consequently, important ecosystem processes, such as nutrient cycling or insect pest regulation, are not self-sustaining (Altieri, 1999; Thrupp, 2000; Figure 1). For example, there are multiple examples from natural and agricultural systems demonstrating that reduced plant genetic diversity can aggravate insect pest problems over time (Gallun, 1977; Pring and Lonsdale, 1989; Peacock and Herrick, 2000; Belloti et al., 2012). To overcome these production challenges, conventional agriculture systems rely on repeated applications of external inputs, such as synthetic pesticides and fertilizers (Altieri, 1999). Although input-intensive agriculture can substantially increase yields in the short term, it also results in increased production costs and negative environmental externalities that are often under-valued (Tilman et al., 2002; Tscharntke et al., 2012a). For instance, widespread reliance on pesticides in industrial practices has resulted in an unsustainable cycle of pesticide-resistance in insects and increased pesticide application, with environmental and human health consequences such as water pollution, habitat degradation, reduction in natural enemy populations, and chronic human health problems (Altieri, 1999; Tilman et al., 2002; Dutcher, 2007; Gibbs et al., 2009; Geiger et al., 2010; Meehan et al., 2011). For smallholder farmers in the developing world, reliance on synthetic fertilizers and pesticides is often impractical due to a lack of financial resources (Gurr et al., 2004). Therefore, exploring alternative agricultural management strategies that support multiple services is critical to creating self-sustaining, resilient food systems.
Moreover, the robustness of agricultural systems has historically been assessed based on crop yield, economic output, and cost-benefit ratios (McIntyre et al., 2009). However, it is increasingly recognized that these metrics fail to consider the diversity of nutrients provided by the agricultural system (DeFries et al., 2015), which is problematic as humans must consistently consume a wide range of nutrients (Graham et al., 2007). Today, a large percentage of the human population receives more than half of their calories and plant-based protein from just three crops—rice, wheat, and maize (Thrupp, 2000; De Shutter, 2014). Although yields have increased significantly (FAO, 2015), the associated reduction in crop diversity has resulted in human diet simplification, which is correlated with negative nutritional outcomes, such as micronutrient deficiencies, malnutrition, and obesity (Frison et al., 2006; Johns and Eyzaguirre, 2006; Figure 1). Given the importance of micronutrients in supporting human health, it is vital that agriculture and nutrition interventions evaluate not only the yield capacity of agroecosystems, but their nutrient diversity as well (Frison et al., 2006; Burlingame et al., 2009a; Remans et al., 2011; De Shutter, 2014; DeFries et al., 2015).
Evaluating the Capacity of Varietal Mixtures to Support Ecosystem Services
While much of the empirical and theoretical work on how biodiversity modulates agroecosystem functioning has targeted crop species diversity (e.g., Poveda et al., 2008; Letourneau et al., 2011), much less attention has been given to the role of varietal mixtures in agroecosystem processes, especially in terms of insect pest control (Tooker and Frank, 2012). Here, we use ecological theory on species mixtures as a framework to consider the potential yield and insect pest suppression benefits provided by varietal mixtures.
Varietal Mixtures and Productivity
Increasing plant species diversity can enhance plant productivity through several mechanisms, including the selection effect, niche complementarity, and microbial-mediated resources (Loreau and Hector, 2001; Reynolds et al., 2003; Hooper et al., 2005; Letourneau et al., 2011). For instance, increasing the number of plant species in a field translates into a higher probability of incorporating a very productive species (i.e., the sampling or selection effect) (Huston, 1997; Loreau and Hector, 2001). It is well-established that cultivars of the same crop species vary in terms of productivity. For example, seed yield of quinoa varieties can vary by an order of magnitude (Bhargava et al., 2007; Miranda et al., 2012; FAO CIRAD, 2015; Bazile et al., 2016). Therefore, the selection effect could be highly applicable to the relationship between varietal mixtures and productivity (Barot et al., 2017).
Polycultures are also expected to achieve high productivity through niche complementarity, where the species mixture is better able to exploit limited resources via resource partitioning or facilitation (Tilman et al., 1997; Loreau, 2000; Loreau and Hector, 2001) (Table 1). For example, different plant species may access limiting nutrients such as nitrogen and phosphorus at different times during the growing season or from different regions of the soil, thereby reducing competitive interactions (Harrison et al., 2008). Although the variation in resource utilization among varieties of the same species would not be expected to be as pronounced as it is between species, cultivars of the same species can vary in their nutrient requirements or adaptations for accessing resources (Sarandon and Sarandon, 1995; Mundt, 2002; Ninkovic, 2003; Cowger and Weisz, 2008). For example, there is evidence that root depth and nutrient absorption efficiency differ among wheat cultivars (Lupton et al., 1974). Groundnuts also show significant intraspecific variation in tap root length, secondary root number, and root volume. Varieties with a stronger root system perform better in phosphorus-limited conditions than cultivars with a less developed root system (Kumar et al., 2009). A meta-analysis evaluating the effect of cultivar mixtures on crop yield identified facilitation as one possible mechanism underlying the increased yield stability observed in mixtures compared to monocultures (Reiss and Drinkwater, 2018). In addition, recent work has demonstrated that increased resource complementarity in plants can be selected for over time by growing plants in high diversity conditions, promoting niche differentiation that can enhance productivity (Zuppinger-Dingley et al., 2014). Thus, crop productivity in mixtures could be strengthened by incorporating varieties that have been intentionally selected in high diversity plantings (Zuppinger-Dingley et al., 2014). Moreover, the microbial-mediated resources hypothesis proposes that species-specific soil microbes can further facilitate a plant's ability to differentially access limited resources (Reynolds et al., 2003) (Table 1). Research on microbial interactions across three cultivars of potatoes revealed cultivar-specific endophytic bacteria, soil microbes known to promote plant growth and health through beneficial metabolic interactions (Sessitsch et al., 2002). These findings suggest that niche complementarity and the microbial-mediated resources hypothesis could be relevant to varietal mixtures, at least in some systems.
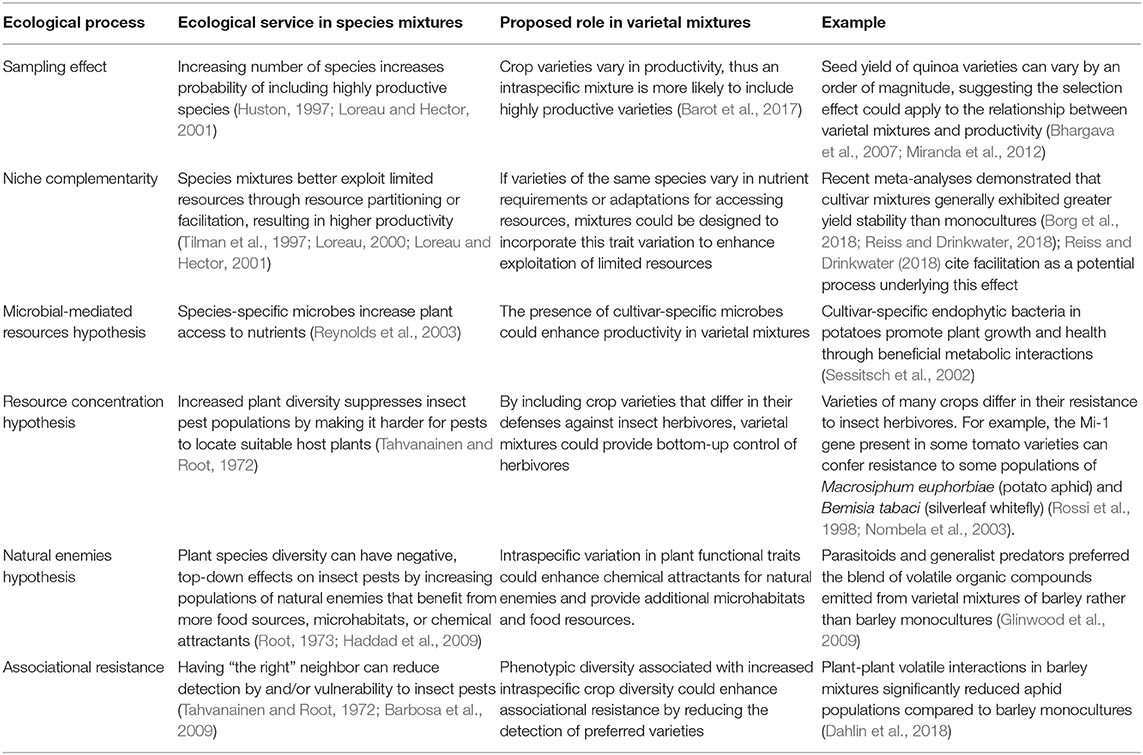
Table 1. Summary of the services provided by ecological processes in species mixtures, the proposed role of these processes in varietal mixtures, and examples from the literature on varietal crop mixtures.
However, several studies indicate that to achieve yield benefits mixtures must be designed thoughtfully; it is not varietal diversity per se that supports enhanced yields, but rather the functional components that are included in the mixtures, and the ratio at which they are combined. For example, wheat mixtures have been shown to produce significantly higher grain protein content without sacrificing yield under low input conditions (Sarandon and Sarandon, 1995). However, this effect was dependent on nitrogen availability and the proportion of the mixture components. Other research has found grain yield and protein content in wheat mixtures to be highly correlated with the average of the cultivar components, which suggests mixture performance depends on the selection of cultivars (Gallandt et al., 2001). In addition, there is evidence that mixture efficiency is enhanced by mixture complexity; fields trials show that mixtures with three or more components tend to produce higher yields than mixtures with only two (Newton et al., 1997; Mille et al., 2006).
As we continue to explore the potential for varietal mixtures to support yield services, it is important to evaluate this service across a breadth of cropping systems as the majority of studies to date have been conducted in cereal crops. In contrast, less research has evaluated the effect of varietal mixtures on crop productivity in other systems, such as annual vegetable crops and underutilized crop species.
Varietal Mixtures and Insect Pest Suppression
In addition to enhancing crop productivity, there are a number of hypotheses derived from polyculture research predicting that increased plant diversity will support insect pest control services (Tahvanainen and Root, 1972; Root, 1973; Andow, 1991; Thies et al., 2003; Poveda et al., 2008; Barbosa et al., 2009; Letourneau et al., 2011). The resource concentration hypothesis posits that increased plant species diversity can directly suppress herbivore populations, particularly those that specialize on one plant species, by making it harder for herbivores to locate the appropriate host plant (Tahvanainen and Root, 1972) (Table 1). In contrast, monocultures provide homogenous, concentrated resources that make it easier for specialist herbivores to locate a suitable host plant. For varietal mixtures to provide bottom-up control of herbivores they must be designed intentionally, using varieties that differ meaningfully in their defenses against herbivores. There are multiple examples of intraspecific variation in herbivore defenses that could be leveraged in the design of varietal mixtures, which we discuss in more detail below.
The natural enemies hypothesis suggests that increasing plant species diversity can also have negative, top-down effects on insect herbivores by increasing populations of natural enemies that benefit from an increase in different food sources, microhabitats, or chemical attractants (Root, 1973; Haddad et al., 2009) (Table 1). For example, parasitoids have been shown to prefer the blend of volatile organic compounds associated with varietal mixtures of barley rather than barley monocultures (Glinwood et al., 2009). In addition, having “the right neighbor” can enhance both bottom-up and top-down control of herbivores, referred to as associational resistance (Tahvanainen and Root, 1972; Barbosa et al., 2009) (Table 1). The phenotypic diversity associated with increased intraspecific crop diversity in some crop species could enhance associational resistance by reducing herbivore detection of preferred varieties and by providing alternative resources or attractants for natural enemies. A recent study documented significantly lower aphid populations in barley mixtures compared to barley monocultures mediated by plant-plant volatile interactions (Dahlin et al., 2018). On the other hand, there is evidence that enhanced morphological diversity can inhibit top-down insect pest control by affecting the search pattern of natural enemies or by providing shelter to insect pests from predators (Peterson et al., 2016). Therefore, we may expect varietal mixtures characterized by high variation in morphological traits to receive less top-down insect pest control than mixtures with varieties that are relatively similar in structure.
Varietal mixtures have been used successfully in agricultural disease management programs for decades, often by including varieties that vary in their resistance to a pathogen. Varieties of the same crop species can also differ in their resistance to insect pests, which suggests a similar method could be used to provide insect pest control services. For instance, in response to herbivory by the Western corn rootworm (Diabrotica virgifera) most European lines of maize release a sesquiterpene, (E)-β-caryophyllene, which attracts an entomopathogenic nematode that acts as a biological control agent of corn rootworm (Rasmann et al., 2005). However, most North American lines of maize are unable to induce this chemical distress signal in response to herbivory (Rasmann et al., 2005). Some plant-insect interactions even occur on a gene-for-gene basis, similar to many plant-pathogen systems. For instance, the Mi-1 gene in tomatoes has been found to confer resistance to some populations of Macrosiphum euphorbiae (potato aphid) and Bemisia tabaci (silverleaf whitefly) (Rossi et al., 1998; Nombela et al., 2003). Similarly, the Vat gene in melons provides increased resistance to Aphis gossypii (cotton aphid) and the transmission of viruses vectored by this aphid (Dogimont et al., 2009). The gene-for-gene interactions that occur between some insect species and crop varieties, as well as the diversity of plant defenses that can be found within a single crop species, indicate that varietal mixtures could be designed to modulate insect populations.
Previous work in natural and agricultural systems has demonstrated that increasing variation in plant traits and quality—at the individual, population, or community level—can either decrease or increase herbivore population size, and may affect generalist and specialist insect pests differently (Andow, 1991; Underwood, 2004). Many crop species exhibit intraspecific variation in traits related to insect pest resistance, which suggests that crop varietal mixtures would likely affect insect pest populations. Indeed, a recent meta-analysis demonstrated that, on average, herbivore abundance is significantly reduced in varietal mixtures compared to monocultures (Koricheva and Hayes, 2018). We refer readers to Tooker and Frank (2012) for an exhaustive review of the literature on the insect pest control potential of varietal mixtures.
In spite of the potential for varietal mixtures to support insect pest control services, additional empirical research is needed to better understand when we should expect varietal mixtures to suppress or enhance insect pest populations as well as how these effects will influence herbivory. We also need more research across cropping systems, as varietal mixtures of certain crop species may be more effective than others depending on the level of intraspecific trait variation present. Moreover, crops are often attacked by a complex of insect pest species; therefore, we need research across insect taxa as well as studies that consider the effect of mixtures on multiple insect pest species simultaneously. Studies testing the effects of varietal mixtures on different trophic levels would provide valuable information on whether the suppressive effects of mixtures are a function of bottom-up or top-down control of insect pests (Tooker and Frank, 2012). There is strong evidence that the success of on-farm management practices is often affected by the composition of the surrounding landscape (Tscharntke et al., 2012b). Therefore, we need landscape-scale studies that examine the ability of varietal mixtures to support insect pest control services across different landscape contexts.
Assessing Economic Services of Varietal Mixtures
Agricultural producers are already experiencing negative effects of global climate change, making it increasingly important to design resilient agricultural systems that can withstand greater climate variability while continuing to promote agricultural and food system health (Lin et al., 2008; Döring et al., 2015). We use the term resilience to refer to an agroecosystem that continues to supply services, such as food production and insect pest control, when challenged with abiotic and biotic stressors. Biodiversity supports ecosystem function by enhancing complementary resource use and functional redundancy, which are important in the face of environmental change. The concept of functional redundancy is linked to the insurance hypothesis (Yachi and Loreau, 1999), the idea that biodiversity acts as a buffer against environmental variability because species differ in their response to change, helping to ensure the maintenance of an agroecosystem's functional capacity even in the face of external shocks.
Research has demonstrated that biodiversity, across time and spatial scales, can promote economically valuable ecosystem services that enhance agroecosystem functioning and stability. For example, preserving forest habitats at the landscape-scale can enhance pollination services resulting in higher coffee yields, an ecosystem service with an estimated value of $60,000 annually for a single large farm (Ricketts et al., 2004). Similarly, polycultures can reduce insect pest and disease pressure, and enhance yields (Power, 2010; Letourneau et al., 2011; Iverson et al., 2014), and establishing diversified crop rotations can increase crop yield stability and resilience to environmental stressors (Gaudin et al., 2015). The value of these ecosystem services is determined based on their ability to either reduce production costs or increase revenue. For example, enhancing pest control and soil fertility services can reduce the use of pesticide and fertilizer inputs. Ecosystem services can also increase the value of agricultural products, thereby enhancing revenue generation. For example, diversified agroecosystems may produce higher yields than simplified systems, or producers may be able to obtain a price premium from products grown in diversified systems because they have characteristics desired by consumers (e.g., shade-grown coffee).
However, the value of ecosystem services is only part of the story; costs associated with production (e.g., labor and inputs) must also be taken into account if we wish to assess the capacity of these diversification strategies to provide farmers with secure livelihoods. For instance, manipulating landscape-level diversity would require coordinated efforts among multiple stakeholders, which can be difficult to achieve (but see Murray et al., 2005; Brier et al., 2008; Schellhorn et al., 2015), and would likely entail higher costs that could dampen profits. Polycultures can be incompatible with mechanized agricultural equipment, and can increase cost due to higher labor requirements (Gliessman, 1985; Tooker and Frank, 2012). Consequently, assessing the economic net benefits of varietal mixtures would require a comparison of their costs and revenues to conventional monoculture production systems, likely using a net-present value framework to capture short-and long-term differences.
Developing alternative diversification strategies that mitigate production costs would increase the options available to growers, allowing them to implement a form of diversification that meets their particular needs. Crop varietal mixtures are one alternative form of diversification that could promote resilient agroecosystems by providing valuable ecosystem services while also reducing logistical constraints associated with other scales of diversification. Unlike landscape-scale diversification, which growers have little control over, varietal mixtures can be easily implemented at the field scale. Varieties of a single species are more likely to have similar harvest schedules compared to crops of different species (Wolfe, 1985), and are often similar enough to be planted, harvested, and marketed together (Wilhoit, 1992). Although development times can vary across varieties, if mixtures are designed to incorporate varieties with similar agronomic characteristics, this problem can be avoided. Alternatively, many small-scale, tropical farmers intentionally plant fields to varieties that vary in maturation time to extend the growing or harvest period, spread out labor demands over a longer time period, and increase harvest security (Clawson, 1985). Management practices for different varieties of the same species are also likely to overlap, which means farmers can maximize their existing agronomic knowledge by expanding varietal diversity in a crop they already grow. Therefore, implementing varietal mixtures may not require significant changes to existing management practices or farmer knowledge (Tooker and Frank, 2012), or investment in new infrastructure. While varietal mixtures are not a panacea for food security, they are a powerful strategy that could complement other forms of diversification by improving the availability of and access to food.
For instance, varietal mixtures could be particularly useful in situations where growers face labor constraints and have little control over their surrounding landscape—making it difficult to manipulate landscape or crop species diversity. Small-scale farmers in developing countries face poorly functioning markets and are often resource limited (Chavas and Di Falco, 2012); a management strategy that averts risk by increasing harvest security without incurring additional labor costs would be extremely valuable. By planting multiple varieties in a mixture, farmers mitigate risk by increasing the odds that at least some varieties will produce well, resulting in an overall harvest that might be adequate to sustain a livelihood and that could improve the availability and access to food. For example, in the highlands of Mexico, farmers routinely plant multiple varieties of potatoes together to protect potato yields by reducing the spread of fungal pathogens (Ugent, 1968).
Planting varietal mixtures can further protect food availability by increasing yield stability relative to monocultures. In particular, varietal mixtures have been shown to promote yield stability under conditions of environmental stress, which has important implications for growers who benefit from risk reduction and the ability to predict their annual production (Smithson and Lenné, 1996; Mundt, 2002; Kaut et al., 2009; Bullock et al., 2017). For example, under drought and low fertility conditions in Senegal, mixtures of early and intermediate maturity cowpea varieties produce more stable yields in comparison to monocultures (Thiaw et al., 1993). Early maturing varieties of cowpea are important to food security as they can provide food and income during times when food is scarce (Hall and Patel, 1985; Thiaw et al., 1993). However, medium maturity varieties tend to produce more grain and forage than the early maturity varieties (Thiaw et al., 1993). Planting a mixture of both varieties increases food security by prolonging the period of food availability without sacrificing productivity. In Canada, wheat mixtures outperform wheat monocultures under nutrient-poor conditions and generate higher yields (Kaut et al., 2009). Similarly, oat varietal mixtures are more productive than monocultures under drought stress (Peltonen-Sainio and Karjalainen, 1991). The ability of varietal mixtures to outperform monocultures in the face of abiotic stresses indicates varietal mixtures have the potential to enhance the resilience of agroecosystems to climate change (Borg et al., 2018; Reiss and Drinkwater, 2018).
Many crop species encompass a broad range of intraspecific trait variation and plasticity that could be useful for adapting to an array of abiotic and biotic stresses, which are increasingly unpredictable due to climate change (Sthapit et al., 2010). In particular, landraces and currently underutilized crop species have been selected over generations by local growers to withstand a range of difficult growing conditions and are likely to be important for developing future crop varieties (Padulosi et al., 2014). For example, certain ecotypes of Andean quinoa are extremely drought tolerant and well-adapted to saline and sandy soils, making them well-suited to high-altitude desert environments. In contrast, coastal ecotypes of quinoa are adapted to high annual precipitation and are resistant to pre-harvest sprouting (Murphy et al., 2016). Maintaining this kind of intraspecific trait variation is useful for preserving sources of genetic diversity that may be important in the development of future crop varieties. By having access to locally desirable traits such as drought tolerance or insect pest resistance, growers could more easily adapt to changing climatic or biotic stresses (Sthapit et al., 2010).
However, due to a focus on measuring the value of services and a lack of data on costs, it remains unclear under what conditions varietal mixtures are likely to be a profitable diversification option. Future studies examining the economic impact of varietal mixtures should adopt a holistic approach that quantifies the costs of production as well as the value of ecosystem services generated by this scale of diversity. This data will allow us to measure the effect of varietal mixtures on profitability, arguably the most important economic endpoint for most farmers. Such studies should be conducted over multiple years to assess the capacity of varietal mixtures to support sustainable livelihoods.
Varietal Mixtures as a Nutrition Intervention
Given that over a quarter of the human population does not receive adequate nutrition, it is critical that nutritional benefits contributing to food security be recognized as an ecosystem service and integral goal of agroecosystems (Burlingame et al., 2009a; DeFries et al., 2015). However, the trend toward more simplified food systems has had negative impacts on human health and nutrition, such as low diet diversity, micronutrient deficiencies, and malnutrition in the developed as well as developing world (Frison et al., 2006; Graham et al., 2007; Negin et al., 2009; De Shutter, 2014). Despite global increases in agricultural productivity, more than 900 million people are still undernourished (Food and agriculture Organization of the United Nations, 2010) and over 2 billion people exhibit at least one micronutrient deficiency (IFPRI, 2014). To address these issues, we cannot focus our attention solely on ramping up agricultural production, as this alone will not guarantee food security, or improved nutrition (Herforth et al., 2012). Rather, we must pay attention to the diversity of food produced to increase food security and combat diet-related health issues (Esquinas-Alcazar, 2005; Toledo and Burlingame, 2006; Herforth et al., 2012). While we acknowledge that dietary diversity is likely most easily achieved by consuming different crop species, we highlight the meaningful nutritional diversity that exists within many annual food crop species.
Varietal mixtures may play an important role in diet diversification and human health as different crop cultivars vary in their nutrient compositions (Toledo and Burlingame, 2006; Burlingame et al., 2009a). This could be particularly true for traditional local varieties and underutilized crop species that tend to exhibit high intraspecific variation in nutritional profiles (Padulosi et al., 2014). For example, a study examining the nutritional characteristics of six cultivated quinoa genotypes found significant differences in seed protein content and antioxidant activity across the genotypes (Miranda et al., 2012) (Table 2). The Villarrica genotype had the highest protein content, while the Faro genotype exhibited the highest antioxidant activity. Jute is another example of an underutilized species that exhibits significant variation in nutrient content across varieties (Table 2). Traditionally grown for fiber production, the young leaves of jute plants are consumed as a leafy green vegetable in rural parts of Asia and Africa. Interestingly, some of the varieties that provide high levels of beta-carotene are less nutritious in terms of iron content (Choudhary et al., 2013). Landraces of Andean potatoes are an example of a staple food crop that encompasses broad variation in nutrient content, including fiber, ascorbic acid (vitamin C), potassium, and carotenoids (Andre et al., 2007) (Table 2). The nutritional diversity found in potato varieties is particularly evident in the Andes where varietal mixtures are suggested to play an important role in dietary diversity (Picón-Reátegui, 1976). In this region, varieties can vary in concentrations of a given micronutrient by an order of magnitude and small portions of certain varieties can provide up to half of the daily required intake of a micronutrient, such as vitamin C (Andre et al., 2007). Given the tradeoffs in nutrient content across varieties in each of these crop species, planting a varietal mixture of quinoa, jute, or potatoes would increase the nutritional profile of a field relative to a monoculture of a variety that has a high level of only one nutrient (Figure 2).
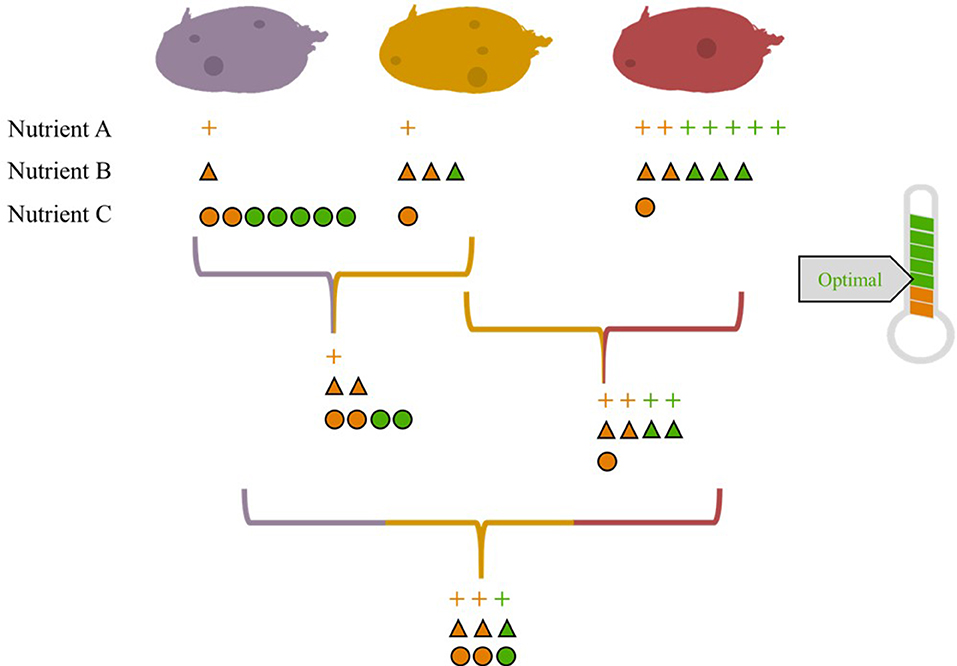
Figure 2. In this illustration, we show three hypothetical varieties of potato (purple, yellow, and red) that have varying levels of nutrients A, B, and C. A field planted to two or three of these varieties provides a more balanced nutrient profile relative to a field planted with one variety. Based on the assumption that individuals would consume the same quantity of potatoes regardless of variety, we show the average level of each nutrient provided by two- and three-variety mixtures. In this hypothetical example, three units of each nutrient are needed to achieve the daily recommended intake. Green indicates optimal nutrient levels that meet this requirement, while orange indicates sub-optimal nutrient levels.
Varieties of more common staple food crops, such as wheat can also differ significantly in their concentrations of micronutrients such as magnesium and zinc (Oury et al., 2006) (Table 2). In some instances, the highest concentrations of these nutrients are found in rare, underutilized varieties. Zinc, which can only be acquired through diet, is an essential trace element that is vital to a range of human functions due to its role as a cofactor of many enzymes (Prasad, 1998). However, there is evidence that high magnesium and zinc content in grains comes at a cost to yield (Oury et al., 2006). Therefore, it would be antagonistic to breed simultaneously for high nutrient content and yield (Oury et al., 2006) and to plant a field to just one cultivar would result in a tradeoff either in terms of yield or nutritional content. However, planting a mixture of wheat that includes both high yielding and nutrient-rich cultivars would allow yield and nutrition metrics to be met simultaneously.
Interestingly, intraspecific variation in phytochemicals that are important to human nutrition also serve as plant defense compounds against insect herbivory. Recent research suggests that increasing nutrient heterogeneity within agroecosystems via intraspecific crop diversity could enhance insect pest control services (Wetzel et al., 2016). Therefore, increasing phytochemical diversity via varietal mixtures provides the opportunity to simultaneously enhance nutritional and ecological benefits. For example, ascorbic acid, a vitamin critical to human nutrition, contributes to defending plants against photo-oxidative stress (Smirnoff, 1996). Carotenoids are not only important antioxidants for humans, they are also secondary metabolites key to plant defense (Hahlbrock and Scheel, 1989). Significant variation in both compounds has been found among tomato varieties (George et al., 2004), suggesting that consuming a variety of cultivars could provide a more complete nutritional profile. Varieties of Brassica oleracea are also known to vary widely in their mineral nutrient composition and glucosinolate profiles—phytochemicals that offer protection from insect herbivores (Broadley et al., 2007). In the human gut, glucosinolates are hydrolyzed into isothiocyanates (Johnson, 2002) and there is strong evidence that these compounds play a major role in protecting against cancer (Talalay and Fahey, 2001). Indeed, research suggests that the variation in mineral composition found within B. oleracea crops is substantial enough to warrant use in genetic biofortification programs aimed at alleviating human dietary deficiencies, as varieties of this crop species can vary more than 20-fold in their concentrations of zinc (Broadley et al., 2007).
Given the wide range of nutrients found within a single crop species, the capacity to measure crop nutritional diversity has important implications for human health and nutrition, and deserves further attention (DeFries et al., 2015). To this end, we must transition from traditional yield- or calorie-based metrics to new metrics, such as nutritional functional diversity (FD), that consider the nutrient diversity of agroecosystems (Remans et al., 2011). Nutritional functional diversity (FD) was originally developed to describe the crop species composition in an agroecosystem as well as the nutritional composition of crops in terms of important nutrients, which are categorized as functional traits. In this context, functional diversity measures the variation in nutrient composition and content in a crop community (Remans et al., 2011). For example, incorporating a crop with a distinct nutrient profile would increase the nutritional functional diversity of a field. While the nutritional FD metric was originally developed to assess nutrient diversity at the species level, it could be extended to varietal mixtures as there is meaningful nutritional diversity to be leveraged within crop species (George et al., 2004; Oury et al., 2006; Andre et al., 2007; Broadley et al., 2007; Burlingame et al., 2009b; Remans et al., 2011). The key difference would be that most varieties within a single species are likely to contain the same nutrients, whereas different species are more likely to have different nutrients from one another. Therefore, in the case of varietal mixtures, the purpose of the nutritional FD metric would be to evaluate the relative amount of key nutrients provided by each variety (Figure 2), rather than measuring whether a variety contributes a new nutrient. This would be more akin to measuring nutrient evenness than nutrient diversity. Applying this metric to varietal mixtures could promote their use as a method for increasing dietary diversity, at little cost to growers, and would provide a more effective strategy for diversifying diets than randomly increasing the number of varieties in a mixture.
We predict the greatest nutritional impact of varietal mixtures would be seen in subsistence or semi-subsistence agriculture systems where households predominantly consume crops they have grown or participate in local food supply chains. We might expect to see similar outcomes in local-scale food supply chains, such as those still common throughout Europe. In these food systems, households could increase dietary diversity by expanding varietal diversity in their own fields or by purchasing food directly from local growers with diversified fields. Indeed, varietal mixtures are quite common in primarily subsistence agriculture systems where they are typically used to extend the harvest period and income generation, and curb crop disease (Smithson and Lenné, 1996). Whether these mixtures are currently planted with the explicit intent of increasing dietary diversity or the nutritional benefits are a “side-effect” of a strategy aimed primarily at providing other services, increasing awareness of the nutritional benefits associated with varietal mixtures could be particularly impactful for communities suffering from micronutrient deficiencies and would allow multiple services to be achieved simultaneously.
In contrast, we would expect fewer direct nutritional impacts of varietal mixtures in commercial food systems where the majority of households purchase food from retailers. Mainstream food supply chains provide a wide range of annual crop species and varieties, regardless of whether the crops were grown in a monotypic or diversified field, as produce is pooled from multiple growers. However, a meaningful and growing number of households in the United States—where mainstream food supply chains are ubiquitous—are increasingly procuring produce from emerging food channels, such as community-supported agriculture (CSA), farmers markets, and farm stands (King et al., 2010). The nutritional benefits of varietal mixtures are more likely to emerge in these local food supply chains where households are purchasing directly from growers.
The extent of nutritional services provided by varietal mixtures will also vary depending on the crop species in question. For instance, we might expect varieties of vegetable and fruit crops that are directly consumed by humans to provide a greater nutritional impact than annual grain crops grown for human consumption, which often require processing. However, depending on the particular grain and type of processing, micronutrients can remain stable and, in some cases, become more concentrated during food processing (Slavin et al., 2000).
Conclusions
There is clear evidence that varietal mixtures are a feasible agricultural manipulation with the potential to support agroecosystem services that provide economic and nutritional benefits to humans (Figure 1). Implementation of varietal mixtures seems quite viable in small markets dominated by farmers who are growing primarily for subsistence purposes, where changes to existing infrastructure and practices would be small in comparison to large-scale, conventional systems. However, with appropriate policy incentives, there is also potential for this practice to be adopted more widely in conventional agricultural systems, as has already been done for pathogen management in small grains (Finckh et al., 2000; Mundt, 2002; Tooker and Frank, 2012). Where marketing or processing requires the separation of varieties, varietal mixtures could be designed to accommodate mechanized equipment in the form of strip mixtures where the varieties are mixed in alternating strips of rows.
To expand the adoption of varietal mixtures, a number of knowledge gaps require attention. We lack a clear understanding of when and where the services provided by varietal mixtures are likely to be strongest in agroecosystems. For instance, most research evaluating the effect of varietal mixtures on yield have been conducted in cereal crops (Reiss and Drinkwater, 2018) and little is known about the role of varietal mixtures in supporting crop productivity in other cropping systems, such as annual vegetable crops. We also have yet to understand how a range of insect herbivores and natural enemies respond to this level of diversity (Tooker and Frank, 2012). Further research is also needed to determine how genetically diverse a varietal mixture should be to simultaneously support the ecological, nutritional, and economic components of agroecosystems, and whether or not this level of diversity is consistent across agricultural systems (e.g., primarily subsistence vs. small scale market oriented vs. large scale commercial).
It is also imperative that we explore the mechanisms underlying the ecological impacts associated with varietal mixtures (Hughes et al., 2008). Understanding these mechanisms will allow us to enhance agroecosystem services that support food security. Research in natural systems has highlighted the need to comprehensively compare the relative importance of interspecific and intraspecific plant diversity for ecological processes (Cook-Patton et al., 2011; Tooker and Frank, 2012); this knowledge would be particularly valuable in an agricultural context as it would expand management options for growers.
Understanding the effects of varietal mixtures on farm profitability under different management regimes is another key area of research that will help farmers design agroecosystems that capitalize on returns from ecosystem services. Implementing varietal mixtures may not require significant changes to existing management practices, but to understand whether or not this diversification strategy can benefit farmers, we need to accurately assess its effects on profitability through empirical economic analyses that measure both production costs and revenue generation, ideally over multiple years.
Given the importance of micronutrients in supporting human health and their interlinked roles in physiological functions (Frison et al., 2006), it is vital that agricultural interventions measure not only the yield capabilities of agroecosystems, but nutrient diversity as well. To address nutritional services, further research and outreach underscoring the capacity of varietal mixtures to support dietary diversity and human well-being is needed, as its value is often underappreciated. For instance, in some regions where nutrient-related diseases remain common, intraspecific variation in nutrient content is not routinely considered to be an important characteristic when extension agents recommend cultivars to farmers (Huang et al., 1999; Toledo and Burlingame, 2006). The nutritional functional diversity metric is one tool that can address this issue by providing another dimension by which we can measure cultivar characteristics (Remans et al., 2011). By moving beyond the ecological aspects of functional diversity, this metric allows us to broaden our perspective on the functional capacity of agroecosystems.
As we seek to fill these knowledge gaps, we cannot focus our attention solely on varietal diversity per se, as the composition, and functional diversity of varietal mixtures are likely to be significant drivers of agroecosystem processes (Newton et al., 1997; Gallandt et al., 2001; Mille et al., 2006). To better understand the linkages between agrobiodiversity, resilient agroecosystems, and human nutrition, we need to simultaneously explore impacts on multiple outcomes, such as ecosystem services, dietary diversity, labor productivity, and livelihood status. Studies integrating agroecology, socioeconomics, and nutrition will guide us toward multi-functional, sustainable food systems.
Author Contributions
LS generated the idea for the manuscript and wrote the initial draft. AP and MG contributed to the development of the manuscript. MG contributed substantially to the economic components of the manuscript. All authors contributed to manuscript revision, read, and approved the submitted version.
Funding
LS was supported by the National Science Foundation (NSF) through a Graduate Research Fellowship and the Food Systems and Poverty Reduction Integrative Graduate Education and Research Traineeship at Cornell University (#DGE-0903371). AP received support from Cornell University, USDA-NIFA AFRI Grant #2013-67013-21235, and the Collaborative Crop Research Program of the McKnight Foundation.
Conflict of Interest
The authors declare that the research was conducted in the absence of any commercial or financial relationships that could be construed as a potential conflict of interest.
Acknowledgments
The authors are grateful to L. Figueroa, A. Iverson, E. Lombardi, R. Nelson, R. Perez-Alvarez, J. Peters, K. Poveda, and J. Thaler for discussion and helpful comments. The authors also thank R. Perez-Alvarez for designing Figure 2.
References
Altieri, M. A. (1999). The ecological role of biodiversity in agroecosystems. Agric. Ecosyst. Environ. 74, 19–31. doi: 10.1016/S0167-8809(99)00028-6
Andow, D. A. (1991). Vegetational diversity and arthropod population response. Rev. Entomol. 36, 561–566. doi: 10.1146/annurev.en.36.010191.003021
Andre, C. M., Ghislain, M., Bertin, P., Oufir, M., del Rosario Herrera, M., Hoffman, L., et al. (2007). Andean potato cultivars (Solanum tuberosum L.) as a source of antioxidant and mineral micronutrients. J. Agric. Food Chem. 55, 366–378. doi: 10.1021/jf062740i
Barbosa, P., Hines, J., Kaplan, I., Martinson, H., Szczepaniec, A., and Szendrei, Z. (2009). Associational resistance and associational susceptibility: having right or wrong neighbors. Annu. Rev. Ecol. Evol. Syst. 40, 1–20. doi: 10.1146/annurev.ecolsys.110308.120242
Barot, S., Allard, V., Cantarel, A., Enjalbert, J., Gauffreteau, A., Goldringer, I., et al. (2017). Designing mixtures of varieties for multifunctional agriculture with the help of ecology. a review. Agron. Sustain. Dev. 37:13. doi: 10.1007/s13593-017-0418-x
Bazile, D., Pulvento, C., Verniau, A., Al-Nusairi, M. S., Ba, D., Breidy, J., et al. (2016). Worldwide evaluations of quinoa: preliminary results from post international year of quinoa FAO projects in nine countries. Front. Plant Sci. 7:850. doi: 10.3389/fpls.2016.00850
Belem, M., Bazile, D., and Coulibaly, H. (2018). Simulating the impacts of climate variability and change on crop varietal diversity in Mali (West-Africa) using agent-based modeling approach. Jasss 21:8. doi: 10.18564/jasss.3690
Belloti, A., Herrera Campo, B. V., and Hyman, G. (2012). Cassava production and pest management: present and potential threats in a changing environment. Trop. Plant Biol. 5, 39–72. doi: 10.1007/s12042-011-9091-4
Bhargava, A., Shukla, S., and Ohri, D. (2007). Genetic variability and interrelationship among various morphological and quality traits in quinoa (chenopodium quinoa willd.). Field Crop. Res. 101, 104–116. doi: 10.1016/j.fcr.2006.10.001
Bianchi, F. J. J. A., Booij, C. J. H., and Tscharntke, T. (2006). Sustainable pest regulation in agricultural landscapes: a review on landscape composition, biodiversity and natural pest control. Proc. Biol. Sci. 273, 1715–1727. doi: 10.1098/rspb.2006.3530
Borg, J., Kiær, L. P., Lecarpentier, C., Goldringer, I., Gauffreteau, A., Saint-Jean, S., et al. (2018). Unfolding the potential of wheat cultivar mixtures: a meta-analysis perspective and identification of knowledge gaps. Field Crop Res. 221, 298–313. doi: 10.1016/j.fcr.2017.09.006
Brier, H. B., Murray, D. A. H., Wilson, L. J., Nicholas, A. H., Miles, M. M., Grundy, P. R., et al. (2008). An overview of integrated pest management (IPM) in north-eastern Australian grain farming systems: past, present and future prospects. Aust. J. Exp. Agric. 48, 1574–1593. doi: 10.1071/EA08166
Broadley, M., Meacham, M., Bowen, H., Hammond, J., Hyden, R., Mead, A., et al. (2007). Natural genetic variation in the mineral nutrient composition of Brassica oleracea. Comp. Biochem. Physiol A Mol. Integr. Physiol. 146, S246–47. doi: 10.1016/j.cbpa.2007.01.573
Bullock, J. M., Dhanjal-Adams, K. L., Milne, A., Oliver, T. H., Todman, L. C., Whitmore, A. P., et al. (2017). Resilience and food security: rethinking an ecological concept. J. Ecol. 105, 880–884. doi: 10.1111/1365-2745.12791
Burlingame, B., Charrondiere, R., and Mouille, B. (2009a). Food composition is fundamental to the cross-cutting initiative on biodiversity for food and nutrition. J. Food Compos. Anal. 22, 361–365. doi: 10.1016/j.jfca.2009.05.003
Burlingame, B., Mouille, B., and Charrondiere, R. (2009b). Nutrients, bioactive non-nutrients and anti-nutrients in potatoes. J. Food Compos. Anal. 22, 494–502. doi: 10.1016/j.jfca.2009.09.001
Chavas, J. P., and Di Falco, S. (2012). On the role of risk versus economies of scope in farm diversification with an application to ethiopian farms. J. Agric. Econ. 63, 25–55. doi: 10.1111/j.1477-9552.2011.00319.x
Choudhary, S. B., Sharma, H. K., Karmakar, P. G., Anil Kumar, A., Saha, A. R., Hazra, P., et al. (2013). Nutritional profile of cultivated and wild jute (corchorus) species. Aust. J. Crop Sci. 7, 1973–1982.
Clawson, D. L. (1985). Harvest security and intraspecific diversity in traditional tropical agriculture. Econ. Bot. 39, 56–67. doi: 10.1007/BF02861175
Cook-Patton, S. C., McArt, S. H., Parachnowitsch, A. L., Thaler, J. S., and Agrawal, A. A. (2011). A direct comparison of the consequences of plant genotypic and species diversity on communities and ecosystem function. Ecology 92, 915–923. doi: 10.1890/10-0999.1
Cowger, C., and Weisz, R. (2008). Winter wheat blends (mixtures) produce a yield advantage in North Carolina. Agron. J. 100, 169–177. doi: 10.2134/agronj2007.0128
Dahlin, I., Rubene, D., Glinwood, R., and Ninkovic, V. (2018). Pest suppression in cultivar mixtures is influenced by neighbor-specific plant – plant communication. Ecol. Appl. 28, 2187–2196. doi: 10.1002/eap.1807
De Schutter, O. (2014). Report of the Special Rapporteur on the Right to Food, Olivier De Schutter. Report A/HRC/25/57 to the Twenty-Fifth Session of the Human Rights Council.
DeFries, R., Fanzo, J., Remans, R., Palm, C., Wood, S., and Aderman, T. L. (2015). Metrics for land-scarce agriculture. Science. 349, 238–240. doi: 10.1126/science.aaa5766
Dogimont, C., Bendahmane, A., Pitrat, M., Burget-Bigeard, E., Hagen, L., Le Menn, A., et al. (2009). Gene resistant to Aphis gossypii. U.S. Patent 7576264.
Döring, T. F., Vieweger, A., Pautasso, M., Vaarst, M., Finckh, M. R., and Wolfe, M. S. (2015). Resilience as a universal criterion of health. J. Sci. Food Agric. 95, 455–465. doi: 10.1002/jsfa.6539
Dutcher, J. D. (2007). “A review of resurgence and replacement causing pest outbreaks in IPM,” in General Concepts in Integrated Pest and Disease Management. Integrated Management of Plants Pests and Diseases, Vol. 1, eds A. Ciancio, and K. G. Mukerji (Dordrecht: Springer), 27–43. doi: 10.1007/978-1-4020-6061-8_2
Esquinas-Alcazar, J. (2005). Protecting crop genetic diversity for food security: political, ethical and technical challenges. Nat. Rev. Genet. 6, 946–953. doi: 10.1038/nrg1729
Finckh, M. R., Gacek, E. S., Goyeau, H., Lannou, C., Merz, U., Mundt, C. C., et al. (2000). Cereal variety and species mixtures in practice, with emphasis on disease resistance. Agronomie 20, 813–837. doi: 10.1051/agro:2000177
Food and agriculture Organization of the United Nations, R. (2010). The State of Food Insecurity in the World Addressing Food Insecurity in Protracted Crises 2010. Key Messages, Notes.
Frison, E., Smith, I. F., Johns, T., Cherfas, J., and Eyzaguirre, P. B. (2006). Agricultural diversity, nutrition, and health : making a difference to hunger and nutrition in the developing world. Food Nutr. Bull. 27, 167–179. doi: 10.1177/156482650602700208
Gallai, N., Salles, J. M., Settele, J., and Vaissière, B. E. (2009). Economic valuation of the vulnerability of world agriculture confronted with pollinator decline. Ecol. Econ. 68, 810–821. doi: 10.1016/j.ecolecon.2008.06.014
Gallandt, E. R., Dofing, S. M., Reisenauer, P. E., and Donaldson, E. (2001). Diallel analysis of cultivar mixtures in winter wheat. Crop Sci. 41, 792–796. doi: 10.2135/cropsci2001.413792x
Gallun, R. L. (1977). Genetic basis of Hessian fly epidemics. Ann. N. Y. Acad. Sci. 287, 223–229. doi: 10.1111/j.1749-6632.1977.tb34241.x
Garibaldi, L. A., Aizen, M. A., Klein, A. M., Cunningham, S. A., and Harder, L. D. (2011). Global growth and stability of agricultural yield decrease with pollinator dependence. Proc. Natl. Acad. Sci. U.S.A. 108, 5909–5914. doi: 10.1073/pnas.1012431108
Gaudin, A. C. M., Tolhurst, T. N., Ker, A. P., Janovicek, K., Tortora, C., Martin, R. C., et al. (2015). Increasing crop diversity mitigates weather variations and improves yield stability. PLoS ONE 10:e0113261. doi: 10.1371/journal.pone.0113261
Geiger, F., Bengtsson, J., Berendse, F., Weisser, W. W., Emmerson, M., Morales, M. B., et al. (2010). Persistent negative effects of pesticides on biodiversity and biological control potential on European farmland. Basic Appl. Ecol. 11, 97–105. doi: 10.1016/j.baae.2009.12.001
George, B., Kaur, C., Khurdiya, D. S., and Kapoor, H. C. (2004). Antioxidants in tomato (lycopersium esculentum) as a function of genotype. Food Chem. 84, 45–51. doi: 10.1016/S0308-8146(03)00165-1
Gibbs, K. E., MacKey, R. L., and Currie, D. J. (2009). Human land use, agriculture, pesticides and losses of imperiled species. Divers. Distrib. 15, 242–253. doi: 10.1111/j.1472-4642.2008.00543.x
Gliessman, S. R. (1985). “Multiple cropping systems: a basis for developing an alternative agriculture,” in Innovative Biological Technologies for Lesser Developed Countries—Workshop Proceedings (Washington, DC: OTA), 69–83.
Glinwood, R., Ahmed, E., Qvarfordt, E., Ninkovic, V., and Pettersson, J. (2009). Airborne interactions between undamaged plants of different cultivars affect insect herbivores and natural enemies. Arthropod. Plant Interact. 3, 215–224. doi: 10.1007/s11829-009-9072-9
Graham, R. D., Welch, R. M., Saunders, D. A., Ortiz-Monasterio, I., Bouis, H. E., Bonierbale, M., et al. (2007). Nutritious subsistence food systems. Adv. Agron. 92, 1–74. doi: 10.1016/S0065-2113(04)92001-9
Grusak, M. A., and DellaPenna, D. (1999). Improving the nutrient composition of plants to enhance human nutrition and health1. Annu. Rev. Plant Physiol. Plant Mol. Biol. 50, 133–161. doi: 10.1146/annurev.arplant.50.1.133
Gurr, G. M., Wratten, S. D., and Altieri, M. A. (2004). Ecological engineering for pest management. Plant Prot. Q. 30:244. doi: 10.1071/9780643098411
Haddad, N. M., Crutsinger, G. M., Gross, K., Haarstad, J., Knops, J. M. H., and Tilman, D. (2009). Plant species loss decreases arthropod diversity and shifts trophic structure. Ecol. Lett. 12, 1029–1039. doi: 10.1111/j.1461-0248.2009.01356.x
Hahlbrock, K., and Scheel, D. (1989). Physiology and molecular biology of phenylpropanoid metabolism. Annu. Rev. Plant Physiol. 40, 347–369. doi: 10.1146/annurev.pp.40.060189.002023
Hall, A. E., and Patel, P. N. (1985). “Cowpea breeding for resistance to drought and heat,” in: Cowpea Research, Production, and Utilization. eds S. R. Singh, and K. O. Rachie (New York, NY: John Wiley & Sons), 137–151.
Harrison, K. A., Bol, R., and Bardgett, R. D. (2008). Do plant species with different growth strategies vary in their ability to compete with soil microbes for chemical forms of nitrogen? Soil Biol. Biochem. 40, 228–237. doi: 10.1016/j.soilbio.2007.08.004
Herforth, A., Jones, A., and Pinstrup-Andersen, P. (2012). “Prioritizing nutrition in agriculture and rural development,” HNP Discussion Papers Series (Washington, DC).
Hooper, D. U., Chapin, F. S., Ewel, J. J., Hector, A., Inchausti, P., Lavorel, S., et al. (2005). Effects of biodiversity on ecosystem functioning: a consensus of current knowledge. Ecol. Monogr. 75, 3–35. doi: 10.1890/04-0922
Huang, A. S., Tanudjaja, L., and Lum, D. (1999). Content of alpha-, beta-carotene, and dietary fiber in 18 sweetpotato varieties grown in Hawaii. J. Food Compos. Anal. 151, 147–151. doi: 10.1006/jfca.1999.0819
Hughes, A. R., Inouye, B. D., Johnson, M. T. J., Underwood, N., and Vellend, M. (2008). Ecological consequences of genetic diversity. Ecol. Lett. 11, 609–623. doi: 10.1111/j.1461-0248.2008.01179.x
Huston, M. A. (1997). Hidden treatments in ecological experiments : re-evaluating the ecosystem function of biodiversity. Oecologia 110, 449–460. doi: 10.1007/s004420050180
IFPRI (2014). Global Nutrition Report 2014: Actions and Accountability to Accelerate the World's Progress on Nutrition. Washington, DC.
Iverson, A. L., Marin, L. E., Ennis, K. K., Gonthier, D. J., Connor-Barrie, B. T., Remfert, J. L., et al. (2014). Do polycultures promote win-wins or trade-offs in agricultural ecosystem services? A meta-analysis. J. Appl. Ecol. 51, 1593–1602. doi: 10.1111/1365-2664.12334
Jackson, L. E., Pascual, U., and Hodgkin, T. (2007). Utilizing and conserving agrobiodiversity in agricultural landscapes. Agric. Ecosyst. Environ. 121, 196–210. doi: 10.1016/j.agee.2006.12.017
Johns, T., and Eyzaguirre, P. B. (2006). Linking biodiversity, diet and health in policy and practice. Proc. Nutr. Soc. 65, 182–189. doi: 10.1079/PNS2006494
Johnson, I. T. (2002). Glucosinolates in the human diet. Bioavailability and implications for health. Phytochem. Rev. 1, 183–188. doi: 10.1023/A:1022507300374
Karp, D. S., Rominger, A. J., Zook, J., Ranganathan, J., Ehrlich, P. R., and Daily, G. C. (2012). Intensive agriculture erodes β-diversity at large scales. Ecol. Lett. 15, 963–970. doi: 10.1111/j.1461-0248.2012.01815.x
Kaut, A. H. E. E., Mason, H. E., Navabi, A., O'Donovan, J. T., and Spaner, D. (2009). Performance and stability of performance of spring wheat variety mixtures in organic and conventional management systems in western Canada. J. Agric. Sci. 147, 141–153. doi: 10.1017/S0021859608008319
Khan, Z. R., Midega, C. A. O., Amudavi, D. M., Hassanali, A., and Pickett, J. A. (2008). On-farm evaluation of the “push-pull” technology for the control of stemborers and striga weed on maize in western Kenya. Field Crop Res. 106, 224–233. doi: 10.1016/j.fcr.2007.12.002
Kiær, L. P., Skovgaard, I. M., and Østergård, H. (2009). Grain yield increase in cereal variety mixtures: a meta-analysis of field trials. Field Crop Res. 114, 361–373. doi: 10.1016/j.fcr.2009.09.006
King, R. P., Hand, M. S., DiGiacomo, G., Clancy, K., Gomez, M. I., Hardesty, S. D., et al. (2010). Comparing the Structure, Size, and Performance of Local and Mainstream Food Supply Chains, ERR-99, U.S. Dept. of Agr., Econ. Res. Serv.
Klein, A. M., Vaissière, B. E., Cane, J. H., Steffan-Dewenter, I., Cunningham, S. A., Kremen, C., et al. (2007). Importance of pollinators in changing landscapes for world crops. Proc. R. Soc. 274, 303–313. doi: 10.1098/rspb.2006.3721
Koricheva, J., and Hayes, D. (2018). The relative importance of plant intraspecific diversity in structuring arthropod communities: a meta-analysis. Funct. Ecol. 32, 1704–1717. doi: 10.1111/1365-2435.13062
Kumar, A., Kusuma, P, and Gowda, M. V. C. (2009). Genetic variation for root traits in groundnut germplasm under phosphorus stress conditions. J. Sat. Agric. Res. 7, 1–4.
Letourneau, D. K., Armbrecht, I., Rivera, B. S., Lerma, J. M., Carmona, E. J., Daza, M. C., et al. (2011). Does plant diversity benefit agroecosystems? A synthetic review. Ecol. Appl. 21, 9–21. doi: 10.1890/09-2026.1
Lin, B. B. (2011). Resilience in agriculture through crop diversification: adaptive management for environmental change. Bioscience 61, 183–193. doi: 10.1525/bio.2011.61.3.4
Lin, B. B., Perfecto, I., and Vandermeer, J. (2008). Synergies between agricultural intensification and climate change could create surprising vulnerabilities for crops. Bioscience 58, 847–854. doi: 10.1641/B580911
Loreau, M. (2000). Biodiversity and ecosystem functioning: recent theoretical advances. Oikos 91, 3–17. doi: 10.1034/j.1600-0706.2000.910101.x
Loreau, M., and Hector, A. (2001). Partitioning selection and complementarity in biodiversity experiments. Nature 412, 72–76. doi: 10.1038/35083573
Lupton, F. G. H., Olover, R. H., Ellis, F. B., Barnes, B. T., Howse, K. R., Welbank, P. J., et al. (1974). Root and shoot growth of semi-dwarf and taller winter wheats. Ann. Appl. Biol. 77, 129–144. doi: 10.1111/j.1744-7348.1974.tb06881.x
McIntyre, B., Herre, H. R., Wakhungu, J., and Watson, R. T., (eds.). (2009). International Assessment of Agricultural Knowledge, Science and Technology for Development. Washington, DC: Island Press.
Meehan, T. D., Werling, B. P., Landis, D. A., and Gratton, C. (2011). Agricultural landscape simplification and insecticide use in the Midwestern United States. Proc. Natl. Acad. Sci. U.S.A. 108, 11500–11505. doi: 10.1073/pnas.1100751108
Mille, B., Belhaj Fraj, M., Monod, H., and de Vallavieille-Pope, C. (2006). Assessing four-way mixtures of winter wheat cultivars from the performances of their two-way and individual components. Eur. J. Plant Pathol. 114, 163–173. doi: 10.1007/s10658-005-4036-0
Miranda, M., Vega-Gálvez, A., Martinez, E., López, J., Rodríguez, M. J., Henríquez, K., et al. (2012). Genetic diversity and comparison of physicochemical and nutritional characteristics of six quinoa (Chenopodium quinoa willd.) genotypes cultivated in Chile. Food Sci. Technol. 32, 835–843. doi: 10.1590/S0101-20612012005000114
Mundt, C. C. (2002). Use of multiline cultivars and cultivar mixtures for disease management. Annu. Rev. Phytopathol. 40, 381–410. doi: 10.1146/annurev.phyto.40.011402.113723
Murphy, K. M., Bazile, D., Kellogg, J., and Rahmanian, M. (2016). Development of a worldwide consortium on evolutionary participatory breeding in Quinoa. Front. Plant Sci. 7:608. doi: 10.3389/fpls.2016.00608
Murray, D. A. H., Miles, M. M., Mclennan, A. J., Lloyd, R. J., and Hopkinson, J. E. (2005). “Area-wide management of Helicoverpa spp. in an Australian mixed cropping agroecosystem,” in Proceedings of the 2005 Beltwide Cotton Conference, (New Orleans).
National Academy of Sciences (2009). Dietary Reference Intakes: Recommended Intakes for Individuals. Washington, DC.
Negin, J., Remans, R., Karuti, S., and Fanzo, J. C. (2009). Integrating a broader notion of food security and gender empowerment into the African green revolution. Food Secur. 1, 351–360. doi: 10.1007/s12571-009-0025-z
Newton, A. C., Ellis, R. P., Hackett, C. A., and Guy, D. C. (1997). The effect of component number on rhynchosporium secalis infection and yield in mixtures of winter barley cultivars. Plant Pathol. 46, 930–938. doi: 10.1046/j.1365-3059.1997.d01-83.x
Ninkovic, V. (2003). Volatile communication between barley plants affects biomass allocation. J. Exp. Bot. 54, 1931–1939. doi: 10.1093/jxb/erg192
Nombela, G., Williamson, V. M., and Muñiz, M. (2003). The root-knot nematode resistance gene Mi-1.2 of tomato is responsible for resistance against the whitefly bemisia tabaci. Mol. Plant Microbe. Interact. 16, 645–649. doi: 10.1094/MPMI.2003.16.7.645
Oury, F. X., Leenhardt, F., Rémésy, C., Chanliaud, E., Duperrier, B., Balfourier, F., et al. (2006). Genetic variability and stability of grain magnesium, zinc and iron concentrations in bread wheat. Eur. J. Agron. 25, 177–185. doi: 10.1016/j.eja.2006.04.011
Padulosi, S., Amaya, K., Jäger, M., Gotor, E., Rojas, W., and Valdivia, R. (2014). A holistic approach to enhance the use of neglected and underutilized species: The case of andean grains in Bolivia and Peru. Sustainability 6, 1283–1312. doi: 10.3390/su6031283
Peacock, L., and Herrick, S. (2000). Responses of the willow beetle Phratora vulgatissima to genetically and spatially diverse salix spp. plantations. J. Appl. Ecol. 37, 821–831. doi: 10.1046/j.1365-2664.2000.00528.x
Peltonen-Sainio, P., and Karjalainen, R. (1991). Agronomic evaluation of growing oat cultivar mixtures under various stress conditions in Finland. Acta Agric. Scand. 41, 47–53. doi: 10.1080/00015129109438582
Peterson, J. A., Ode, P. J., Oliveira-hofman, C., Harwood, J. D., Brennan, R., and Birch, A. N. (2016). Integration of plant defense traits with biological control of arthropod pests : challenges and opportunities. Front. Plant Sci. 7:1794. doi: 10.3389/fpls.2016.01794
Picón-Reátegui, E. (1976). “Nutrition,” in Man in the Andes, eds P. T. Baker and M. A. Little (Stroudsburg, PA: Dowden, Hutchinson and Ross), 208–236.
Pingali, P. L. (2012). Green revolution: impacts, limits, and the path ahead. PNAS 109, 12302–12308. doi: 10.1073/pnas.0912953109
Poveda, K., Gomez, M., and Martinez, E. (2008). Diversification practices: their effect on pest regulation and production. Rev. Colomb. Entomol. 34, 131–144.
Power, A. G. (1991). Virus spread and vector dynamics in genetically diverse plant populations. Ecology 72, 232–241. doi: 10.2307/1938917
Power, A. G. (2010). Ecosystem services and agriculture: tradeoffs and synergies. Philos. Trans. R. Soc. Lond. B. Biol. Sci. 365, 2959–2971. doi: 10.1098/rstb.2010.0143
Prasad, A. S. (1998). Zinc in human health: an update. J. Trace Elem. Exp. Med. 11, 63–87. doi: 10.1002/(SICI)1520-670X(1998)11:2/3<
Pring, D. R., and Lonsdale, D. M. (1989). Cytoplasmic male sterility and maternal inheritance of disease susceptibility in maize. Annu. Rev. Phytopathol. 27, 483–502. doi: 10.1146/annurev.py.27.090189.002411
Rasmann, S., Kollner, T. G., Degenhardt, J., Hiltpold, I., Toepfer, S., Kuhlmann, U., et al. (2005). Recruitment of entomopathogenic nematodes by insect-damaged maize roots. Nature 434, 732–737. doi: 10.1038/nature03451
Reiss, E. R., and Drinkwater, L. E. (2018). Cultivar mixtures: a meta-analysis of the effect of intraspecific diversity on crop yield. Ecol. Appl. 28, 62–77. doi: 10.1002/eap.1629
Remans, R., Flynn, D. F. B., DeClerck, F., Diru, W., Fanzo, J., Gaynor, K., et al. (2011). Assessing nutritional diversity of cropping systems in African villages. PLoS ONE 6:e21235. doi: 10.1371/journal.pone.0021235
Reynolds, H. L., Packer, A., Bever, J. D., and Clay, K. (2003). Grassroots ecology : plant – microbe – soil interactions as drivers of plant community structure and dynamics. Ecology 84, 2281–2291. doi: 10.1890/02-0298
Ricketts, T. H., Daily, G. C., Ehrlich, P. R., and Michener, C. D. (2004). Economic value of tropical forest to coffee production. Proc. Natl. Acad. Sci. U.S. A. 101, 12579–12582. doi: 10.1073/pnas.0405147101
Root, R. B. (1973). Organization of a plant-arthropod association in simple and diverse habitats : the fauna of collards (Brassica Oleracea). Ecol. Monogr. 43, 95–124. doi: 10.2307/1942161
Rossi, M., Goggin, F. L., Milligan, S. B., Kaloshian, I., Ullman, D. E., and Williamson, V. M. (1998). The nematode resistance gene Mi of tomato confers resistance against the potato aphid. PNAS 95, 9750–9754. doi: 10.1073/pnas.95.17.9750
Sarandon, S. J., and Sarandon, R. (1995). Mixture of cultivars - pilot field trial of an ecological alternative to improve production or quality of wheat (Triticum Aestivum). J. Appl. Ecol. 32, 288–294. doi: 10.2307/2405096
Schellhorn, N. A., Parry, H. R., Macfadyen, S., Wang, Y., and Zalucki, M. P. (2015). Connecting scales : achieving in-field pest control from areawide and landscape ecology studies. Insect Sci. 22, 35–51. doi: 10.1111/1744-7917.12161
Sessitsch, A., Reiter, B., Pfeifer, U., and Wilhelm, E. (2002). Cultivation-independent population analysis of bacterial endophytes in three potato varieties based on eubacterial and actinomycetes -specific PCR of 16S rRNA genes. FEMS Microbiol. Ecol. 39, 23–32. doi: 10.1111/j.1574-6941.2002.tb00903.x
Slavin, J. L., Jacobs, D., and Marquart, L. (2000). Grain processing and nutrition. Crit. Rev. Food Sci. Nutr. 40, 309–326. doi: 10.1080/10408690091189176
Smirnoff, N. (1996). The function and metabolism of ascorbic acid in plants. Ann. Bot. 78, 661–669. doi: 10.1006/anbo.1996.0175
Smithson, J. B., and Lenné, J. M. (1996). Varietal mixtures: a viable strategy for sustainable productivity in subsistence agriculture. Ann. Appl. Biol. 128, 127–158. doi: 10.1111/j.1744-7348.1996.tb07096.x
Sthapit, B., Padulosi, S., and Mal, B. (2010). Role of on-farm/in situ conservation and underutilized crops in the wake of climate change*. Indian J. Plant Genet. Resour 23, 145–156.
Tahvanainen, J. O., and Root, R. B. (1972). The influence of vegetational diversity on the population ecology of a specialized herbivore, Phyllotreta cruciferae (coleoptera: chrysomelidae). Oecologia 10, 321–346. doi: 10.1007/BF00345736
Talalay, P., and Fahey, J. W. (2001). Phytochemicals from cruciferous plants protect against cancer by modulating carcinogen metabolism. J. Nutr. 131, 3027S−33S. doi: 10.1093/jn/131.11.3027S
Thiaw, S., Hall, A. E., and Parker, D. R. (1993). Varietal intercropping and the yields and stability of cowpea production in semiarid Senegal. Field Crop Res. 33, 217–233. doi: 10.1016/0378-4290(93)90081-W
Thies, C., Steffan-Dewenter, I., and Tscharntke, T. (2003). Effects of landscape context on herbivory and parasitism at different spatial scales. Oikos 101, 18–25. doi: 10.1034/j.1600-0706.2003.12567.x
Thrupp, L. A. (2000). Linking agricultural biodiversity and food security : the valuable role of sustainable agriculture. Int. Aff. 76, 265–281. doi: 10.1111/1468-2346.00133
Tilman, D., Cassman, K. G., Matson, P. A., Naylor, R., Polasky, S., Chikowo, R., et al. (2002). Agriculture sustainability and intensive production practices. Nature 418, 671–677. doi: 10.1038/nature01014
Tilman, D., Tilman, D., Lehman, C. L., Lehman, C. L., Thomson, K. T., and Thomson, K. T. (1997). Plant diversity and ecosystem productivity: theoretical considerations. Proc. Natl. Acad. Sci. U.S.A. 94, 1857–1861. doi: 10.1073/pnas.94.5.1857
Toledo, Á., and Burlingame, B. (2006). Biodiversity and nutrition: a common path toward global food security and sustainable development. J. Food Compos. Anal. 19, 477–483. doi: 10.1016/j.jfca.2006.05.001
Tooker, J. F., and Frank, S. D. (2012). Genotypically diverse cultivar mixtures for insect pest management and increased crop yields. J. Appl. Ecol. 49, 974–985. doi: 10.1111/j.1365-2664.2012.02173.x
Tscharntke, T., Clough, Y., Wanger, T. C., Jackson, L., Motzke, I., Perfecto, I., et al. (2012a). Global food security, biodiversity conservation and the future of agricultural intensification. Biol. Conserv. 151, 53–59. doi: 10.1016/j.biocon.2012.01.068
Tscharntke, T., Tylianakis, J. M., Rand, T. a., Didham, R. K., Fahrig, L., et al. (2012b). Landscape moderation of biodiversity patterns and processes - eight hypotheses. Biol. Rev. 87, 661–685. doi: 10.1111/j.1469-185X.2011.00216.x
Ugent, D. (1968). The potato in Mexico : geography and primitive culture. Econ. Bot. 22, 108–123. doi: 10.1007/BF02860554
Underwood, N. (2004). Variance and skew of the distribution of plant quality influence herbivore population dynamics. Ecology 85, 686–693. doi: 10.1890/03-0030
Wetzel, W. C., Kharouba, H. M., Robinson, M., Holyoak, M., and Karban, R. (2016). Variability in plant nutrients reduces insect herbivore performance. Nature 539, 425–427. doi: 10.1038/nature20140
Wilhoit, L. R. (1992). “Evolution of herbivore virulence to plant resistance: influence of variety mixtures,” in Plant Resistance to Herbivores and Pathogens: Ecology, Evolution and Genetics, eds R. S. Fritz, E. L. Simms (Chicago: University of Chicago Press), 91–119.
Wolfe, M. S. (1985). Prospects of multiline mixtures for disease. Annu. Rev. Phytopathol. 23, 251–273. doi: 10.1146/annurev.py.23.090185.001343
Yachi, S., and Loreau, M. (1999). Biodiversity and ecosystem productivity in a fluctuating environment: the insurance hypothesis. Proc. Natl. Acad. Sci. U.S.A. 96, 1463–1468. doi: 10.1073/pnas.96.4.1463
Zhu, Y., Chen, H., Fan, J., Wang, Y., Li, Y., Chen, J., et al. (2000). Genetic diversity and disease control in rice. Nature 406, 718–722. doi: 10.1038/35021046
Keywords: varietal mixtures, insect pest control, crop yield, resilience, human nutrition, food security
Citation: Snyder LD, Gómez MI and Power AG (2020) Crop Varietal Mixtures as a Strategy to Support Insect Pest Control, Yield, Economic, and Nutritional Services. Front. Sustain. Food Syst. 4:60. doi: 10.3389/fsufs.2020.00060
Received: 22 June 2019; Accepted: 14 April 2020;
Published: 06 May 2020.
Edited by:
Rebecca Chaplin-Kramer, Stanford University, United StatesReviewed by:
Liming Ye, Ghent University, BelgiumDidier Bazile, Centre de Coopération Internationale en Recherche Agronomique pour le Développement (CIRAD), France
Copyright © 2020 Snyder, Gómez and Power. This is an open-access article distributed under the terms of the Creative Commons Attribution License (CC BY). The use, distribution or reproduction in other forums is permitted, provided the original author(s) and the copyright owner(s) are credited and that the original publication in this journal is cited, in accordance with accepted academic practice. No use, distribution or reproduction is permitted which does not comply with these terms.
*Correspondence: Lauren D. Snyder, bGRzOTdAY29ybmVsbC5lZHU=