- 1International Potato Center (CIP), Sub-Saharan Africa Regional Office, Nairobi, Kenya
- 2Department of Plant Breeding, Swedish University of Agricultural Sciences, Alnarp, Sweden
- 3International Potato Center (CIP), Kigali, Rwanda
- 4International Potato Center (CIP), Maputo, Mozambique
- 5International Crops Research Institute for the Semi-arid Tropics, Addis Ababa, Ethiopia
- 6International Potato Center (CIP), Headquarters, Lima, Peru
Almost half of children <5 years old living in sub-Saharan Africa (SSA) suffer from vitamin A deficiency and 60% suffer from iron deficiency. Thus, there has been a strong commitment to breeding for, promoting awareness of, and delivering adapted pro-vitamin A rich orange-fleshed sweetpotato (OFSP) in SSA during the past two decades and for enhanced iron content since 2014. This review article focuses on major breeding efforts in SSA to enhance the drought tolerance of OFSP and reviews integrated crop management practices for improved and sustained sweetpotato production in SSA farming systems. Under climate change, the frequency and severity of droughts is expected to increase. Technical issues are presented in the context of addressing challenges along the entire value chain to ensure adoption. First, the use of an accelerated breeding scheme reduced the breeding cycle from 8–10 to 4–5 years. Since 2010, 19 drought-tolerant OFSP cultivars have been released in Mozambique, 7 in Malawi, and 2 in South Africa. Moreover, research in four breeding populations using the heterosis exploiting breeding scheme (HEBS) demonstrated that within one breeding cycle of 5 years, clones with significantly higher root yield, abiotic tolerance, host plant resistance to pests and diseases, and early maturity can be produced. In the future, HEBS will be combined with greater use of modern genomic tools, new phenotyping tools, and CRISPR/Cas9-mediated gene editing. Second, beyond genetic enhancements, evidence is presented that using improved crop management systems, existing sweetpotato yields can be increased 2–4 times. Current knowledge is reviewed concerning sweetpotato's role in diverse farming systems, but integrated crop management is clearly under researched. Third, the outlook for drought tolerance breeding indicates that two distinct classes of nutrient-rich cultivars are emerging: (1) Early maturing cultivars (<4 month growing period) that escape drought but also serve humid environments with small landholding size per capita; and (2) Medium maturing (4–6 month growing period) cultivars that avoid drought, are drought tolerant and exhibit continuous root formation. Increasing commercialization of the crop and climate change will drive demand, and the willingness of farmers to invest in improved sweetpotato crop management.
Introduction
Africa is the continent most affected by the triple burden of malnutrition. Thirty African countries suffer undernutrition, micronutrient malnutrition, and the increasing problem of overweight (Development Initiatives, 2018). The quantity of undernourished people in sub-Saharan Africa (SSA) rose 23%, from 181 million in 2010 to almost 222 million in 2016 (FAO et al., 2018). Moreover, micronutrient malnutrition remains a major public health concern in SSA and, when experienced early in life, can lead to irreversible negative health consequences for physical work and cognitive capacity. As of 2011, among the major regions of the world, SSA had the highest prevalence of inadequate intake of vitamin A and second highest for iron, zinc, niacin, vitamin B12, and calcium (Beal et al., 2017). Furthermore, in many SSA countries the problem of overnutrition is increasing at a significant rate, particularly as urban families are relying more on convenient, less micronutrient-dense, processed foods (Barrett and Bevis, 2015). The economic cost of undernutrition is staggering—estimated to cost up to 11% of the Gross Domestic Product (GDP) in Africa (FAO et al., 2019).
Almost half of the children <5 years old living in SSA suffer from vitamin A deficiency and 60% suffer from iron deficiency, which is the major cause of anemia and the most common micronutrient deficiency in the world. Iron deficiency impairs learning capacity and mental development during childhood and adolescence (Barrett and Bevis, 2015). Biofortification is the process of increasing the pro-vitamin A and trace mineral contents of a crop through plant breeding or agronomic practice (Bouis, 2002). Poor households get more than half of their calories from staple foods, and rural households most often consume what they produce. Hence, biofortification of staple foods self-targets those households most at risk of undernutrition and micronutrient malnutrition (Bouis et al., 2019). Emphasis during the past two decades has been on enhancing three major micronutrients [vitamin A, iron (Fe), and zinc (Zn)] in staple food crops. Just 125 g of biofortified orange-fleshed sweetpotato (OFSP) meets a young child's daily vitamin A needs (Low et al., 2009). All flesh-types are good sources of vitamins C, K, E, and several B vitamins, and the mineral magnesium. Sweetpotato roots are also a good source of dietary fiber, stimulating feelings of fullness, and cooked sweetpotatoes have a medium glycemic index (Allen et al., 2012). Given the high levels of vitamin A deficiency in SSA, there has been a strong commitment to breeding for, promoting awareness of, and delivering adapted OFSP cultivars in SSA during the past two decades (Low J. W. et al., 2017). Breeding to enhance iron content in OFSP began in 2014. Strong evidence supports that OFSP introduced in combination with a community-level nutrition education campaign can significantly contribute to reducing vitamin A deficiency among children under 5 years of age (Low et al., 2007; Hotz et al., 2012b).
Sweetpotato grows from sea level to an altitude of 2,400 meters in SSA and it is able to fit into diverse farming systems. Therefore, it is not surprising that the production of sweetpotato has been increasing over the past 20 years (1997–2016) in SSA, with a compound annual growth rate of 3.3% in area and 5.1% in production (FAOSTAT, 2019), indicative of yield improvement. Production per capita figures shown in Table 1 for the top 15 sweetpotato producing countries in SSA in 2018 indicate that sweetpotato is a primary staple food (>50 kg/capita) in 4 countries (Malawi, Tanzania, Rwanda, Burundi) and a secondary staple in an additional 10 countries (10–50 kg/capita). However, average sweetpotato yields in SSA are still remarkably low, with an average of 6 t ha−1 compared to 13 t ha−1 in the South America, 22 t ha−1 in China and 25 t ha−1 in Northern America (FAOSTAT, 2019). The yield gap between existing average yields in SSA on smallholder farms (6–10 t ha−1) and the potential yields like those seen on commercial farms in South Africa (60–80 t ha−1) is huge (Niederwieser, 2004; Low et al., 2009). For sweetpotato to reach its full potential of contributing to improved nutrition in SSA, the causes of these yield gaps need to be addressed in conjunction with biofortification. This can be achieved by breeding for abiotic and biotic stress tolerances, combined with utilization of appropriate agronomic practices on sweetpotato cultivars that are fully integrated into diverse cropping systems.
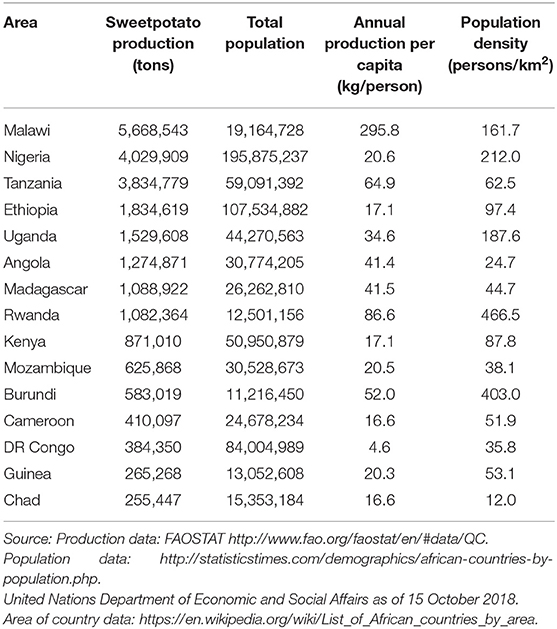
Table 1. Total and Per Capita production of sweetpotato, estimated total population, and population density in 15 top sweetpotato producing countries in SSA in 2018.
A major abiotic stress prevalent in sweetpotato producing areas is drought, which globally affects 52 million people annually (Belesova et al., 2019). Under climate change the frequency and severity of droughts is expected to increase, particularly in West Africa (Intergovernmental Panel on Climate Change (IPCC), 2012). Much of Southern Africa is characterized by unimodal production systems, with dry seasons lasting 5 months or longer, but with several countries vulnerable to flooding due to cyclones. For example, severe flood and extreme drought occurred twice or more during the 2000s in central and southern Mozambique. The International Potato Center (CIP) established a breeding program in disaster-prone Mozambique in 2006, specifically focused on breeding for drought-tolerant OFSP. Scientists in South Africa and Kenya have also specifically engaged in drought research. With regards to integrated crop management (ICM) practices, only improved cultivars and improving access to quality planting materials have received substantial support since 2010, as smallholders are viewed as unwilling to invest in intensifying soil and water management for sweetpotato cultivation until the crop has higher market value. In fact, sweetpotato is known for giving reliable, if less than optimal, yields under sub-optimal growth conditions (Oswald et al., 2009).
This article will discuss major breeding efforts to enhance the tolerance of sweetpotato, especially orange-fleshed types, to drought and will subsequently review integrated crop and farm management practices for improved and sustained sweetpotato production in various farming systems and agro-ecologies in SSA. More specifically, this article provides an overview on (i) research advances made during the past 15 years for breeding drought-tolerant OFSP, (ii) new methods to accelerate breeding for drought tolerance, (iii) methods for managing sweetpotato on-farm and sustaining productivity, and (iv) prospects for breeding sweetpotato for existing and emergent cropping systems over the next two decades.
Breeding for Drought-Tolerance in Sub-Saharan Africa
Sweetpotato is a hexaploid (2n = 6x = 90 chromosomes) outcrossing species with a high degree of genomic duplication. It originated in Latin America but is grown worldwide. The main aim in the genetic enhancement of sweetpotato is to raise the frequency of favorable alleles for desired trait(s) in the breeding population (e.g., population development), and thereafter to select therein outstanding clones for further cultivar development. Each cycle of mass or recurrent selection begins with the botanical seeds of the selected clones. The most important breeding targets are increasing storage root yields, improving flesh quality, enhancing host plant resistance to pathogens (especially viruses) and pests, bettering tolerance to drought, and improving both pro-vitamin A (beta-carotene) and mineral content, particularly Fe and Zn.
To date, drought tolerance has been the key abiotic constraint addressed by breeding in Southern Africa, with 19 drought-tolerant orange-fleshed sweetpotato (OFSP) cultivars released in Mozambique (Andrade et al., 2016a, 2017b), several of which have been further released in other African countries. In addition, considerable research has been undertaken to understand physiological characteristics related to drought in neighboring South Africa (Van Heerden and Laurie, 2008; Omotobora et al., 2014; Laurie R. N. et al., 2015) and some cultivars in Malawi and Kenya were selected for drought tolerance. This is especially relevant in the context of climate change, as the intensity and frequency of drought spells are expected to increase in many parts of Africa over the next decades (Niang et al., 2014). Moreover, since 2014, considerable progress has been made toward the next goal of an iron biofortified, drought tolerant OFSP type.
Sweetpotato and the Effects of Drought and Related Stresses
Large areas in the world (up to two-thirds) are prone to drought and desertification: The west and mid-west of north America, central America, southern and eastern parts of South America, southern Africa, West- and North-Africa, the Near East, Central Asia, three-quarters of India, western and northern China, Western Europe and Australia. These areas have in common that months of humidity are followed by months of dryness. Often the drought stress is coupled with heat and salinity stress. Crop evolution of sweetpotato has taken place in Central America and the western part of South America, including the Pacific coast of Peru, which is one of the driest places in the world. Sweetpotato has been found in Caral (Peru), which is the oldest urban settlement on the American continent (tracing back 2800 BC) and close to the equator. This region of South America is also one of the hottest regions in the world, often facing salinity stress in cropping systems. The Spaniards brought sweetpotato to the Philippines during the sixteenth century. By 1594, the crop was established in China, where the Qing Dynasty heavily promoted it to mitigate droughts and increase food security (Jia, 2013). Currently, for drought prone areas, the crop offers a variety of ways to be consumed as food, including processed items such as, inter alia, baked products, ready-to-eat breakfast foods, french-fries, syrup, starch and beverages (Woolfe, 1992; Bovell-Benjamin, 2007; Padmaja, 2009) as well as animal feed, such as fresh chopped vines for dairy feed (Kinyua, 2013), roots for pigs and poultry (Murugan et al., 2012) or vines and roots as components of silage for pigs and cattle (Kiragu, 2015; Gakige et al., 2020). Cultivars used both as food and feed are known as “dual-purpose” sweetpotatoes, possessing high foliage yields for their use as fresh fodder, in silage or as high-protein feed supplements plus adequate root output (Zhang et al., 1993; León-Velarde and De Mendiburu, 2007). Supplementary fodder availability is key for livestock grazing management and assists in stopping and reversing desertification processes. Research in Rwanda has demonstrated that vines can be cut after 80 days without significant root yield loss (Niyireeba et al., 2013). Climate change is expected to have a negative effect on the quantity of animal feed available and the quality of that feed (Hidosa and Guyo, 2017). Thus, drought tolerant sweetpotato cultivars might become even more significant as animal feed compared to less resilient grasses and cereal crops.
Sweetpotato is commonly grown in rainfed regions. In the context of climate change, the intensity and frequency of drought spells are expected to increase and their predictability decrease in many parts of Africa over the next decades, coupled with heat waves, or floods, especially in Southern Africa (Boko et al., 2007; Niang et al., 2014). Sweetpotato is susceptible to drought, particularly at the root initiation and bulking stages. Cross breeding for tolerance to drought, as well as the identification of main traits to be targeted and ideotypes remain a challenge. Due to differences in time of occurrence and intensity during the period of cultivation, several drought “types” exist—drought escape (earliness); drought avoidance (root depth), and drought tolerance (maintaining assimilation under drought conditions), each having physiological mechanisms underpinning tolerance (Blum, 1988). Moreover, these mechanisms may lead to differing responses according to phenological stages.
Sweetpotato shows a great genetic variability for earliness (Yanfu et al., 1989). In this regard, CIP developed an entire OFSP hybrid population with crop duration requirements of only 90 days (Grüneberg et al., 2015). Some sweetpotato cultivars and breeding clones can grow about 1.5 m deep into the soil, thus absorbing water from deep soil layers (Weaver and Bruner, 1927; Yoshida et al., 1970; Khan et al., 2016). Cultivar and breeding clones differ significantly in their rooting depth (Yen et al., 1964; Grüneberg et al., 2015). These associations between canopy characteristics, water-use efficiency and storage root yields in plants under water deficit deserve further investigation. Drought-tolerant genotypes often exhibit narrow leaves, erect to semi-erect growth types, and their canopy temperature has medium to high location specific heritability when drought occurs late in the cropping season. Sweetpotato can recover from wilting, another trait which differs among genotypes (Laurie R. N. et al., 2015). The tolerance to drought seems to correlate with the relative content of free amino acids, soluble sugars, ATP and the chlorophyll a/b ratio (Zhang et al., 2004, 2005).
Sweetpotato is adaptable to dry areas but severe drought leads to significant storage root yield loss. Drought reduces establishment, stunts growth, seriously damages the photosynthetic apparatus, decreases net photosynthesis, and lessens nutrient uptake (Villordon et al., 2012; Kivuva, 2013; Laurie R. N. et al., 2015). Drought intensity and duration, plant developmental stages and genotypic variability will determine the extent of decrease in storage root yield. There is increasing interest and research on root structure architecture (RSA) in sweetpotato, particularly in breeding for abiotic stress. Khan et al. (2016) review the RSA literature for major root and tuber crops. In sweetpotato, final storage root yield depends on how many lateral roots are formed; their presence is heavily influenced by nutrient and moisture distribution in the soil. Compared to cereal crops, RSA in roots and tubers is very under-researched, in spite of the edible component of the crops being underground (Khan et al., 2016).
Sweetpotato is spreading more and more into the semi-arid tropics and subtropics (Hijmans et al., 2002; Low et al., 2009; Thiele et al., 2017). Breeders for such areas consider drought tolerance to be a critical target for realizing the yield potential of new sweetpotato cultivars and better assure their adoption. A significant percentage of the sweetpotato germplasm appears to be tolerant to drought, heat, and salinity, and they provide storage root harvests in critical drought years (Ding et al., 1997; Anselmo et al., 1998; Xie et al., 1998; Hou et al., 1999; Chávez et al., 2000; Wang et al., 2003; Agili et al., 2012; Kivuva, 2013; Laurie et al., 2013; Yooyongwech et al., 2013; Omotobora et al., 2014; Grüneberg et al., 2015). Sweetpotato needs adequate water supply at planting and for several weeks thereafter (Indira and Kabeerathumma, 1988; Nair et al., 1996; Ravi and Indira, 1996). The crop tolerates, however, moderate drought stress in the second and third months of growth, and severe drought in the fourth or fifth months (Grüneberg et al., 2015).
Van Heerden and Laurie (2008) found two different drought responses in sweetpotato. Limited water supply brought down the leaf relative water content in the breeding clones “A15” and “Resisto.” The negative effects on stomatal conductance disappeared with time in “A15,” suggesting high drought acclimation in “A15.” During restricted water supply, “A15” showed lower suppression of above ground biomass accumulation than “Resisto.” Furthermore, “A15” did not inhibit its photosynthesis on a leaf area basis, whereas CO2 assimilation in “Resisto” was inhibited. As a result, “A15” had higher storage root yield than “Resisto” under restricted water supply (Van Heerden and Laurie, 2008). Other breeding clones and bred-cultivars with similar responses to restricted water supply are “Chissicuana-2,” “Nhacutse-5,” “ADMARC.” “Xiadlaxakau,” “Nwanaqtsjo,” “199062.1,” and “TIS-2534.”
Chávez et al. (2000) selected cultivars showing tolerance to drought, salinity, and boron in the arid Pacific coast of southern Peru and northern Chile. Bernstein (1974) noticed that sweetpotato is semi-tolerant to salinity when electrical conductivity (EC) was 4 dS m−1 in irrigation water or when the EC of soil saturated extract ranged from 6 to 11 dS m−1, with yield reductions of 50%. Mukherjee et al. (2015) found 11 salt tolerant genotypes with adequate yields (>15 t ha−1) after in vitro and in vivo screening of 171 genotypes and further evaluation under salt stress in situ (6.0–8.0 dS m−1) in Odisha and West Bengal.
Sweetpotato Breeding for Drought During the Past 15 Years
For high genetic gains across traits, plant breeding needs to be divided into two components: cultivar development and population improvement (Gallais, 2003). The goal of cultivar development is the selection of the best or very few best clones. In other words, maximizing the response to selection and completing or nearly completing the exploitation of the genetic variation for target breeding traits. The purpose of population improvement, on the other hand, is to select the “best” parents for crossing with the aim of generating genetic variation around a superior population mean. Appropriate multi-trait selection procedures must be used in cultivar development (Elston, 1963), e.g., a base index (Wricke and Weber, 1986). Moreover, a population improvement approach must ensure that undesired genetic gains do not occur in one or more traits (Pesek and Baker, 1969). The cycles of population improvement from a given set of parents to the next set of improved parents should be short (i.e., 2–3 years in sweetpotato with one population without offspring parent analysis and 4–5 years with two populations for a hybrid breeding scheme); while cultivar development has to be rapid with one selection step for early breeding stages (in at least 2 sites) and two selection steps for later breeding stages (1st step in 3–4 sites, and 2nd step in 6–9 locations) (Mwanga et al., 2017). Clearly, continued investment from one breeding stage to the next is driven by the frequency of new clones outperforming the cultivars they aim to replace.
In 2009, sweetpotato breeders in Africa had the opportunity through the Sweetpotato Action for Security and Health in Africa (SASHA) project, funded by the Bill & Melinda Gates Foundation (BMGF), and projects supporting national breeding programs, funded by the Alliance for a Green Revolution in Africa (AGRA), to organize coordinated and collaborative sweetpotato breeding programs based on improved methods and protocols (Grüneberg W. J. et al., 2009). Three sub-regional sweetpotato support platforms (SSPs) were established in SSA with emphasis on population improvement providing 14 national breeding programs with improved true-seed populations and better parental material for key traits, whereas national agricultural research institute (NARI) breeding programs emphasized cultivar development. As a result, decentralized breeding was achieved along with allowing each national partner to keep independence and autonomy. The breeding program in Mozambique became the lead population and cultivar development program for drought tolerance, with 234,962 seeds from this program shared with 18 NARIs partners in Southern Africa, East and Central Africa, West Africa, Brazil, and South East Asia from 2010 through 2019. This joint effort had as desired strategic objectives improved efficiency of breeding methods, increased recombination and number of parents, accelerated breeding and better allocation of resources, more controlled cross breeding, and concomitant stepwise reduction of polycross breeding. Heterosis-exploiting schemes and genomic-led breeding are likely to be pursued further.
In addition to developing a higher number of quality crosses, the time from crossing to releasing a cultivar for one recurrent selection cycle in population improvement1 remains a key factor for improving sweetpotato breeding. In 2006, CIP-Mozambique began implementing the accelerated breeding scheme [ABS (Andrade et al., 2017b)], which reduced the time needed to select a potential cultivar from 8 to 10 years to 4 or 5 years (Figure 1). The ABS is used in the early stages of breeding clonally propagated crops for augmenting breeding efficiency (Grüneberg et al., 2015). Spatial variation of testing environments replaces temporal variation of testing environments when the genotype-by-year variance () is not very important. Testing genotypes, which derive directly from true-seed, are grown in small 1-m row plots of three plants in either 2 or 3 environments without using plot replications. More sites are used in the early stages of breeding, one of which is the stress environment. This accelerates the elimination of clones, speeding up the selection process. Different traits measured simultaneously may be aggregated into an index or selected sequentially across environments (i.e., independent culling). Such an approach may allow selecting 200–500 clones, which subsequently enter into later breeding stages. Grüneberg W. et al. (2009) proposed ABS, which Lebot (2010) discussed further; Andrade et al. (2017b) initiated ABS in Mozambique and Grüneberg in Peru. In April 2010, CIP and INIA in Peru released four cultivars developed following the ABS approach: “Adriano” (CIP 105228.1), “Alexander” (CIP 105240.1), “Arne” (CIP-105086.1) and “Benjamin” (CIP 105085.2). “Benjamin” can yield up to 80 t ha−1 and shows a high number of large roots suitable for markets in drought prone areas with irrigation facilities such as those found on the coasts of Perú and Tajikistan. The sweetpotato breeding network adopted the ABS approach, with 13 African NARIs breeding in 2013, compared to 2 in 2005 (Low J. W. et al., 2017).
“Vine survival” is an underestimated key trait for tolerance in drought-prone areas (Yanggen and Nagujja, 2006; Lebot, 2010). For example, a popular introduced cultivar “Resisto” disappeared in farming systems after drought years in Mozambique due to its inadequate “vine survival.” Desired features include strong and thick vines (often lignified) and medium to high upper biomass production that can provide enough planting material after the dry season and is capable of withstanding short dry spells after planting. Vine survival became an easy-to-assess attribute for selecting cultivars in Mozambique (Andrade et al., 2017a).
Clearly, when breeding for drought-tolerance, one of the ABS sites must be chronically drought-prone. However, drought-tolerant cultivars must also be able to respond positively to water supply. Hence, one of the other ABS sites must be known for having consistently good growing conditions. Geometric mean, percent yield reduction, drought sensitivity index and harvest index (the ratio of storage root weight/storage root plus foliage weight) are useful for selecting breeding clones and cultivars when comparing them under irrigation vs. not having irrigation in the middle of the root initiation growth stage. Harvest index stability and the geometric mean are key for identifying clones with both high storage root yield and stability. Promising clones showed biomass production positively correlated with harvest index stability (Andrade et al., 2016b).
Available Sweetpotato Cultivars for Drought Prone Areas in SSA
The joint CIP-IIAM2 sweetpotato drought tolerance breeding program in Southern Africa based in Maputo, Mozambique has released 22 sweetpotato cultivars that are tolerant to drought since 2010, of which 19 are OFSP. The target population of environments are regions which face a shortened growing season and where drought starts toward the end of the growing season. In February 2011, 15 drought-tolerant OFSP cultivars (“Amelia,” “Bela,” “Delvia,” “Erica,” “Esther,” “Gloria,” “Ininda,” “Irene,” “Ivone,” “Jane,” “Lourdes,” “Melinda,” “Namanga,” “Sumaia,” and “Tio Joe”), bred using ABS, were released in Mozambique (Andrade et al., 2017b). Seven additional drought-tolerant cultivars were released in 2016 (four are orange-fleshed [“Ivone,” “Alisha,” “Victoria,” and “Lawrence”], while “Bie,” “Bita,” and “Caelan” are purple-fleshed cultivars) (Andrade et al., 2016a). Under unimodal rainfed conditions, with no fertilizers, the yield potential of these improved cultivars ranges from 18–25 t ha−1. One of the 2011 releases, Irene, has shown wide adaptability, and has been released in four other SSA countries and has shown good producing ability under irrigation in saline soils in Abu Dhabi. The mechanisms for drought tolerance vary in each cultivar. “Alisha,” “Irene” and “Sumaia” show early maturity. In addition, thick vines provide a morphological adaption to drought in “Alisha,” “Bie,” “Bita,” “Caelan,” and “Irene.” Vine survival was a feature in “Alisha,” “Bita,” “Caelan,” and “Irene.” The best three cultivars combining more than three drought tolerant traits are “Alisha,” “Irene,” and “Sumaia.” As of 2015, drought-tolerant OFSP represents 32% of total sweetpotato production in Mozambique (Ministério da Agricultura e Segurança Alimentar, 2015).
Since 2010, the Malawi national program also bred and released nine cultivars they considered to be drought tolerant, of which seven are orange-fleshed (Chipungu et al., 2011). Three of them were from botanical seed obtained from the population development program in Uganda. In addition, in 2015 South Africa released two OFSP cultivars considered to be widely adapted, including adequate performance in lower rainfall sites (Laurie S. et al., 2015).
On-going research in sweetpotato breeding includes assessing the usefulness of remote sensing via thermal infrared imaging through unmanned aerial vehicles. Higher yield under drought seems to be related to both higher leaf area index and high stomatal conductance (Laurie R. N. et al., 2015). These traits may therefore be used as indirect selection criteria. Additionally, key physiological characteristics involving carbon isotope discrimination (associated with water use efficiency) and leaf temperature are under assessment for their utility in screening sweetpotato genotypes for drought tolerance.
Future Sweetpotato Breeding for Drought Tolerance
In each breeding cycle, new breeding populations should increase the frequency of key traits for drought tolerance. They include strong vine survival attributes, deep rooting measured indirectly via canopy temperature at critical growing stages, storage root yield with low risk to fail under drought in combination with adequate root yield in response to rains (i.e., no inhibition of photosynthesis under conditions of restricted water supply), and harvest index stability in combination with attractive upper biomass production to supply the fodder needs in semi-arid farming systems. Another key trait for smallholder systems is the ability of the root to produce prolific and hardy sprouts when the rains arrive after the dry season. This relates to the dominant traditional practice for how most farmers get their planting material in drought-prone areas. Drought tolerance indices are often used for selecting among sweetpotato breeding clones in stress-prone areas of SSA. Such indices are based on measuring root yields under normal deficit and water deficit. In this regard, Agili et al. (2012) were able to identify breeding clones with a high score on a stress tolerance index and yield potential. They noted that yield potential and yield under stress correlated positively with mean productivity, geometric mean productivity, and stress tolerance, thus suggesting their use for drought tolerance screening in this crop.
In the drought-tolerant breeding programs, more nutritious sweetpotato cultivars are under development. The orange-fleshed cultivars are rich in vitamin A and increasingly in iron and also show high root yields under drought, short crop duration, and tolerance to heat and salinity. The purple-fleshed cultivars are rich in anthocyanins, which are anti-oxidants. The sweetpotato genome is large and heterogeneous, which makes its breeding a challenging task. Controlled cross breeding requires the hand crossing of two breeding clones or cultivars to obtain true seed, which is thereafter planted. The crossing involves several parents and their resulting offspring are grown and evaluated over several generations, with the aim of selecting the best breeding clones. However, the results may be disappointing when using genetically similar parental plants. Sweetpotato breeders should start with crossing cultivars or breeding clones that are genetically different to allow their offspring to exhibit heterosis, thus increasing storage root yield. The genetically different sweetpotato parents exhibiting hybrid vigor in offspring are called “mutually heterotic parental groups.”
CIP recently used 31 DNA markers to assess the relatedness of 141 sweetpotato parents included in polycross seed nurseries in the three population development programs in Africa, and another 80 parents used in Peru. Parents have been clustered into genetically distinct groups (David et al., 2018). Future plant breeding can maximize genetic distances between parental groups to obtain hybrids quickly by crossing an A group parent with a B group parent. This hybrid breeding method is a new approach for population improvement in sweetpotato (Figure 2). The use of mutually heterotic groups leads to a “heterosis exploiting breeding scheme” (HEBS) (Figure 3). In this manner, the offspring show much increased hybrid vigor, and so the improvement in important key traits such as root yield is dramatic. Research using CIP's four breeding populations demonstrated that this scheme will lead rapidly, within one breeding cycle of 5 years, to nutritious improved breeding clones with significant increases in root yield, abiotic tolerance, early maturing, and host plant resistance to pests and diseases. The breeders estimate that for storage root yield and earliness this method accomplished as much as would have been achieved in 36 years of using the older method of polycross breeding in which each mother plant is pollinated randomly by the entire set of parents in the polycross seed nursery.
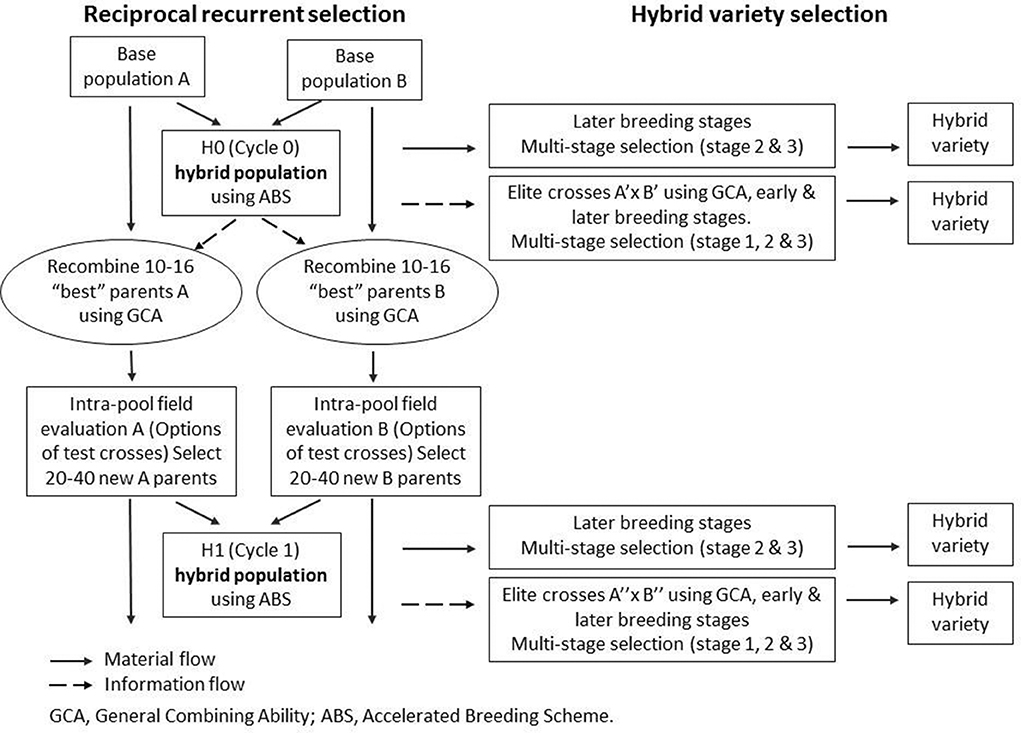
Figure 2. Population hybrid breeding – a heterosis exploiting breeding scheme for clonally propagated crops.
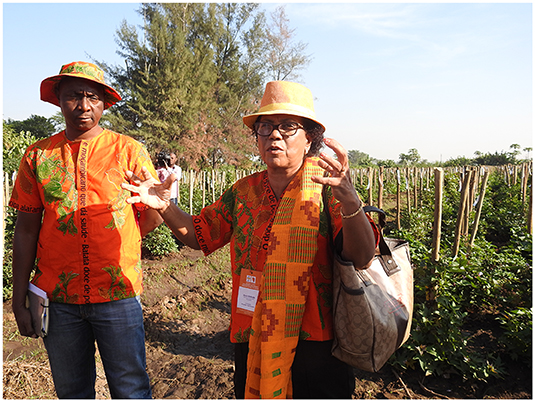
Figure 3. Breeders José Ricardo (IIAM) and Maria Andrade (CIP) in front of 100 parent diallel crossing block in Mozambique (Credit J. Low).
For theoretical reasons, controlled cross breeding is significantly better than polycross breeding (Ssali et al., 2019). However, polycross breeding will continue to be important as bi-parental isolation crosses produce large amounts of true seeds in open pollination, enabling the full exploitation of the large segregation potential within the best sweetpotato parental combinations (Elite-crosses).
Breeding research has observed large yield gains due to hybrid vigor in sweetpotato. Combining ability tests using half-diallel or factorial mating designs are used to assess heterosis increments. Genetic gains for root yield in OFSP hybrid population 1 (H1) vis-à-vis its original baseline after one 5-year reciprocal recurrent selection cycle in Peru (Grüneberg et al., 2019) were 69.7% when harvesting after 90 days (the H1 population storage root mean was 18.5 t ha−1), 117.6% in the high iron population at 120 days harvest (the H1 population storage root mean was 44.9 t ha−1), and 96.2% for the low sweetness population when harvesting after 120 days (the H1 population storage root mean was 30.8 t ha−1). Exploiting the heterosis concept in Kenya, Kivuva et al. (2015) found three high root yielding hybrid progeny with mid- and best parent heterosis ranging from 117.8 to 269.6% under drought conditions.
Population hybrid breeding is already intensifying using DNA markers for genepool separation, because performance, morphological attributes, and origin provide only very limited information on genetic distances among parental material. However, once population hybrid breeding is established, there are tremendous opportunities for selection based on genomic-estimated breeding values (GEBV) or “genomic selection.” First, bi-parental isolation crosses or elite crosses, respectively, can be used to train models on the basis of on-going breeding evaluations with closely related validation populations. The advantages of applying genomic tools for breeding in a comprehensive breeding scheme are huge, because these are actual breeding populations and not research populations unlikely to be used. Moreover, these populations can be generated from in vitro germination, thus avoiding the time-consuming cleanup (i.e., virus removal) of breeding clones to be shared across borders for further evaluation. The vision of sweetpotato breeders is to conduct the entire first breeding stage of elite crosses using in vitro germination and GEBV-led selection. Second, offspring-parent analysis using a chain of datasets from 2 or 3 environments offers an opportunity to delink offspring-parent associations when assessing agronomic performance. This approach is unlikely to replace field testing but will provide additional information on the breeding value of each parent, which is very hard to forecast.
Breeding for tolerance to abiotic stresses is more complex than improving many other traits. One reason is the challenge of defining what are the characteristics that tolerant genotypes should show. For example, leaf area index and stomatal conductance influence storage root yield in sweetpotato as noted by Laurie R. N. et al. (2015), who advocate their use for screening sweetpotato germplasm under drought stress. Furthermore, as indicated by Reynolds et al. (2015), there are also different levels and mechanisms of tolerance for each stress, which lead to different responses according to the phenological stage. Likewise, these traits are multigenic, thus generating a continuous variation, which means dealing with quantitative trait loci or QTL (Collins et al., 2008). Likewise, both additive and non-additive gene actions control leaf-related traits, while additive genes affect SPAD chlorophyll meter readings and root-pull resistance, respectively, as revealed by diallel analysis (Mwije, 2014). Success in cross breeding depends, therefore, on finding an appropriate donor of tolerance genes. Research to identify and use QTL and related mechanisms involved in cellular and whole-plant responses related to drought acclimation will also contribute toward breeding sweetpotato cultivars for drought-prone areas worldwide. Research should also focus on studying the interactions between roots, shoots, the rhizosphere and reproductive traits, as well as their collective effects on storage root yields under various climate scenarios with limited water availability (Tardieu et al., 2018). Fitting plant phenology and other related traits to the probable drought scenario in a defined region is key (Khan et al., 2016; Chenu et al., 2018). Drought, heat, and salinity stress are often synchronous, so combined tolerance to them is very important, although the genetic architectures of their tolerance may differ (Tricker et al., 2018). Some traits to consider for further selecting sweetpotato under drought include early vigor, root architecture, carbon isotope discrimination, stomatal conductance, canopy temperature depression, osmotic adjustment, chlorophyll concentration, stay green and delayed leaf senescence and remobilization of water-soluble carbohydrates (Turner, 1997; Tuberosa, 2012; Chenu et al., 2018; Tardieu et al., 2018).
To succeed on marker-assisted selection (MAS) for both drought and salt tolerance in sweetpotato, the QTLs responsible for the respective stress tolerance must be identified and thereafter linked to diagnostic DNA markers. The discovery of DNA markers, which may be further used to develop ultra-high-density genetic maps, may be facilitated by next generation sequencing (NGS) technology. These maps are very useful for precisely locating QTL and paving the way for their cloning. Likewise, MAS with DNA markers near the target QTL reduces linkage drag during trait introgression. Moreover, microsatellites (SSR) and single nucleotide polymorphisms (SNPs) obtained from NGS enable the production of high-throughput genotyping platforms, which are essential for the simultaneous analysis of many DNA markers and many individuals from segregating populations. Genomic research coupled with transcriptomic analysis provides means for gene discovery and encountering regulatory systems and their positions (Roorkiwal et al., 2014). Bararyenya et al. (2020) were able to find 12 genetic markers for continuous root formation, and another seven for discontinuous root formation, along with their putative functional genes. Omics-based technology may be also useful for unearthing the genes and their specific function of target trait(s). Transcriptome, genome, microme, proteome, and metabolome data allow locating candidate genes when following such an approach. However, suitable phenotyping protocols to assess large populations are essential for identifying tolerance sources and in selection procedures. Various phenotyping platforms are becoming available based on non-invasive or minimally invasive techniques that yield big data (Fiorani and Schurr, 2013).
Remote sensing of growth-related parameters based on spectral reflectance and infrared thermometry are now available to measure plant water status (Masuka et al., 2012). Cool canopy and high stomatal conductance appear to be associated with high yield in drought-prone sites. These traits may be useful as indirect selection criteria. Likewise, canopy spectral reflectance seems to be an effective non-invasive high-throughput phenotyping technique that enables quick and easy measurements of various dynamic and complex traits such as carbon assimilation, biomass accumulation, and plant canopy size. Hence, canopy spectral reflectance may facilitate mass screening of sweetpotato genotypes for tolerance to drought. Thermal infrared imaging or infrared thermography, which estimates leaf or canopy temperature, may be used for drought tolerance screening in sweetpotato. Plant canopy temperature is closely related to canopy conductance at the vegetative stage, providing insights on plant water status. Other key physiological characteristics such as water use efficiency, root growth, carbon isotope discrimination (Δ13C) and leaf temperature provide means for screening sweetpotato genotypes for drought tolerance. Furthermore, a sound characterization of the target population of environments is mandatory to increase the effectiveness of deploying drought tolerance-related characteristics, focusing on those that highly correlate with storage root yield. Currently, image-based systems have been developed, but are in limited use to study roots in their natural environment. Eventually, a major goal would be to develop models capturing the responses of root traits to relevant stresses combined with high-throughput root phenotyping techniques (Khan et al., 2016).
Modern biometrics permit calibrating and validating data that are used for building robust prediction models (Cabrera-Bosquet et al., 2012; Römer et al., 2012). Very recently, CRISPR/Cas9-mediated gene editing was used for mutagenesis of two starch biosynthesis genes (IbGBSSI encoding granule-bound starch synthase I and IbSBEII controlling starch branching enzyme II) in sweetpotato (Wang et al., 2019). Most mutations are related to nucleotide substitutions, thus leading to amino-acid changes and rarely to stop codons. Clasen et al. (2016) used transcription activator-like effective nucleases to silence a gene in potato that breaks down sucrose to glucose and fructose during cold storage, thus improving processing quality of stored tubers. These results show the potential value of this gene editing approach for changing storage root traits in sweetpotato.
Beyond Genetic Enhancement to Ensure OFSP Farming Sustainability
Sweetpotato in Smallholder Cropping and Farming Systems in SSA
To plan for next-generation cropping systems, it is crucial to understand how sweetpotato fits into current farming systems—looking beyond breeding. Hence, a review of research efforts based on field experimentations and scenario modeling efforts has been undertaken.
Sweetpotato is widely grown in different cropping and farming systems in SSA, from the humid tropics of Central and East Africa with its bimodal rainfall pattern, to drought-prone regions in Southern and East Africa with unimodal rainfall pattern and extended dry seasons, and the forest margins and savanna regions of West Africa (Andrade et al., 2009). Two types of food systems with sweetpotato can roughly be distinguished: root crop-based systems where sweetpotato is a staple, and cereal-based systems where sweetpotato is a secondary crop, but with an important role in food security (Ewell and Mutuura, 1994). Root-dominant systems are found in the highland perennial farming system, the root crop farming system and the tree crop farming system, which together cover 15% of the SSA's land area with 32% of her people (Table 2). The major cereal-based systems are the maize-mixed farming system and the cereal-root crop mixed system, which cover 24% of SSA's land area and are home to 34% of her population (Table 2). Among all the area dedicated to sweetpotato in SSA, 89% of it is concentrated in the above five farming systems where 66% of population resides.
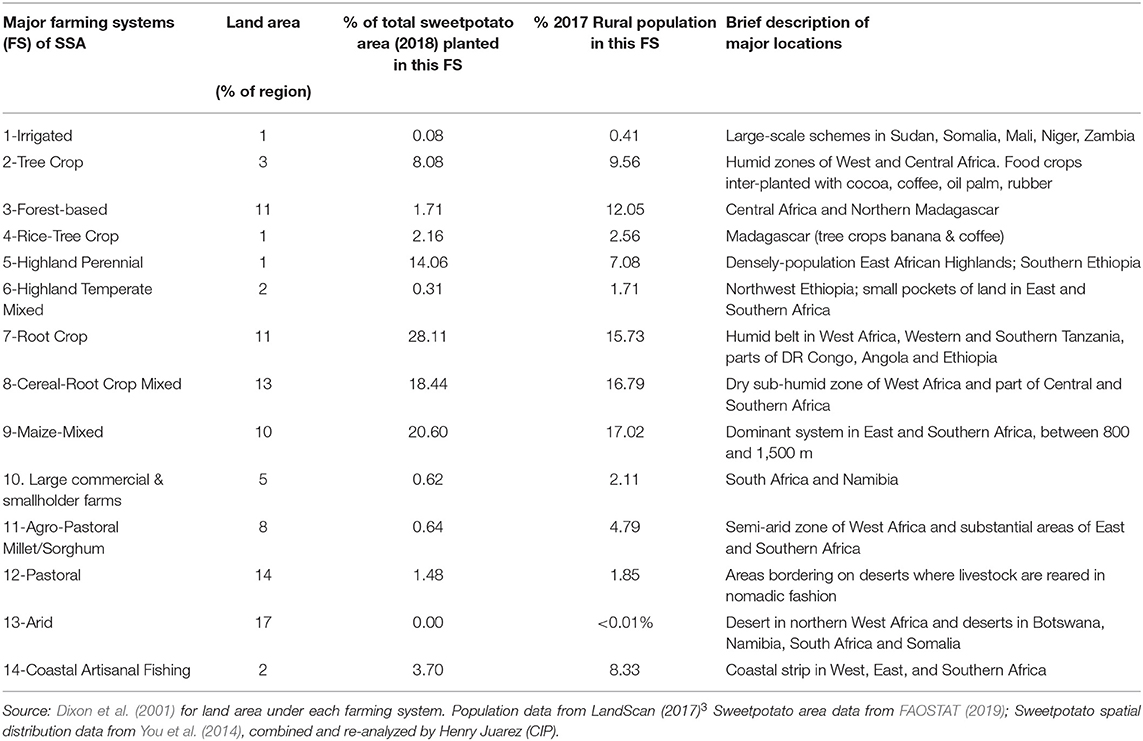
Table 2. Major farming systems in SSA, the percent of land area occupied and where located, and the percent of population and sweetpotato area in each system.
The largest producers of sweetpotato in Africa are Malawi, Nigeria, and Tanzania, with the area under cultivation approximately equal in East and West Africa (FAOSTAT, 2019). Levels of sweetpotato root supply per capita per year are much higher in East, Central and Southern Africa compared to West Africa, being the highest in Malawi, Rwanda, Burundi, and Tanzania (FAOSTAT, 2019). In the humid tropics of Central Africa, sweetpotato is grown on nearly every farm at mid-elevation (Ewell and Mutuura, 1994). Breeding for short maturing (3–4 months) cultivars (drought escape) has been a feature of many sweetpotato breeding programs. Because of such cultivars, in combination with sweetpotato's capacity to tolerate marginal soil and moisture conditions, considerable flexibility exists for incorporating sweetpotato into diverse cropping systems (Andrade et al., 2009). The dominant cropping systems with sweetpotato in SSA encompass (1) monocropping, (2) rotation or intercropping with cereals or legumes, and (3) intercropping with perennials such as cassava, enset, coffee, and fruit trees. Sweetpotato is grown as a monocrop during the main rainy season commonly in root crop-based systems where it is a staple food and a major source of income. In Ethiopia, sweetpotato is grown as a relay crop with maize planted once the maize has tasseled to ensure establishment of sweetpotato before the main rainy season ends (Mudege et al., 2019). Systems with sweetpotato grown on residual moisture in inland valleys or floodplains following a rice crop during the main rainy season can be found in West Africa, Tanzania and Madagascar (Andrade et al., 2009; Ngailo et al., 2015). Intercropping with legumes such as pigeon pea and cowpea are common across the continent4 (Kapinga et al., 1995; Ngailo et al., 2015). Sweetpotato has also a role in crop-livestock systems where vines are fed to livestock, especially dairy cattle (Ewell and Mutuura, 1994; Larbi et al., 2007; Claessens et al., 2008; Mudege et al., 2019).
Factors Which Drive Adoption of OFSP
The most important criteria of smallholder farmers in SSA to adopt OFSP include yield, drought tolerance, maturity period, resistance to pathogens and pests, taste, nutritive value, multi-purpose use (human and animal feeds), market demand, and external appearance of leaves and roots (Ssebuliba et al., 2006; Tomlins et al., 2007; Low J. et al., 2017). Another important factor which has not received enough attention by breeders until recently, is the trait of continuous root formation. This trait is associated with the practice of “piecemeal” harvesting, that is removing mature roots as needed; then others continue to mature over time. Discontinuous root formation, associated with many cultivars bred for early maturity, means that mature root formation occurs at the same time (Bararyenya et al., 2019). For such cultivars, most roots need to be harvested within a few weeks, and must be eaten, stored post-harvest or sold. On the other hand, the selection of newly introduced crops and cultivars by urban consumers depends on sensory profiles, level of promotion of the crop, knowledge, and previous experiences of consumers on similar crops among others (Birol et al., 2015). Hence, research for development organizations and their key investors have recognized the need for integrated agriculture, nutrition and market development approaches for effective introduction of new biofortified crops such as OFSP for sustained improvements of food and nutrition security in the developing world (Low, 2013; Jenkins et al., 2015; Low J. W. et al., 2017).
Effective adoption of OFSP depends on proper participation of the various actors and service providers in the introduction and dissemination processes. Farmers who participated in plant breeding and cultivar selection efforts in Uganda were, respectively, 37 and 7 times more likely to adopt improved sweetpotato cultivars than those who had not (Kiiza et al., 2012). CIP's Sweetpotato for Profit and Health Initiative (SPHI), a coalition of national governments and non-governmental research and development organizations, followed standardized training and participatory variety selection processes across SSA. Farmers trained specifically in sweetpotato production were 9 times more likely to adopt bred-cultivars than those who had not received this type of training (Kiiza et al., 2012).
The Critical Need for a Value Chain Approach
The introduction and wider dissemination of nutritious, drought tolerant OFSP in SSA requires improved understanding of the commodity value chain challenges, constraints, and opportunities (Mmasa and Msuya, 2012; Parmar et al., 2017). Value chain challenges in the predominantly smallholder-based sweetpotato production and marketing systems include: (1) seasonality (in terms of availability of the product in the market); (2) lack of access to ample and quality planting material at the beginning of the rains; (3) post-harvest losses associated with the bulky nature and improper handling of storage roots; (4) low farm gate price during the peak harvest seasons; (5) poorly developed marketing systems; and (6) the image of the crop as a poor person's crop. For example, the retail price of sweetpotato roots in southern Ethiopia was 225% higher in March compared to December, the latter being a peak harvest season and the former a low supply month (Biazin et al., 2019). In SSA, post-harvest losses associated with improper handling along a chain from production sites to retail locations can vary between 40 and 80%, depending on the distance to market locations (Tomlins et al., 2007; Parmar et al., 2017).
One of the major value chain challenges in SSA is that sweetpotato in cereal-based systems is consumed mostly as a breakfast food or snack being boiled, steamed, or fried (Low et al., 2009). This is in contrast with the untapped potential of sweetpotato to be a primary staple food and become a key ingredient in the food processing industry. Given that it has the highest edible energy per unit of area and time among food crops and is one of the cheapest sources of vitamin A in the densely populated areas of Africa, its expanded use makes economic and nutritional sense (Woolfe, 1992; Hotz et al., 2012a). Awareness creation on sustainable production and healthy diets to address both micronutrient deficiencies and obesity (over nutrition) should be considered in value chain developments (Low et al., 2015; Laurie et al., 2018).
Sweetpotato Yield Gaps in SSA
Limited data are available in the scientific literature concerning on-farm root yield levels of sweetpotato in SSA. When evaluating OFSP cultivars in 221 on-farm sites in Malawi, van Vugt and Franke (2018) found that 60–90% of the on-farm root yields were below 10 t ha−1. Mean on-farm root yields varied between 4 and 9 t ha−1 among cultivars in Malawi (van Vugt and Franke, 2018) and between 1 and 9 t ha−1 in Uganda (Omiat et al., 2005). Average sweetpotato root yields are 8, 7.5, 3, and 3 t ha−1 for East, Central, Southern and West Africa, respectively (FAOSTAT, 2019), which are far below attainable yield levels. van Vugt and Franke (2018) defined attainable yield as the mean on-farm yield plus three times the standard deviation and found that attainable root yields varied from 18 to 32 t ha−1 among districts in Malawi. Attainable root yields in experimental farms under rainfed conditions were 25 t ha−1 in Cameroon (Yengoh and Ardo, 2014), 25–29 t ha−1 in South Africa (Laurie et al., 2017), 27 t ha−1 in Mozambique (Andrade et al., 2017b), 27–32 t ha−1 in Rwanda (Rukundo, 2015) and 39 t ha−1 in Nigeria (Uwah et al., 2013). Despite the reputation of sweetpotato as a robust crop tolerant to low inputs and minimal crop management, based on these numbers it appears that a 100–200% yield increase can realistically be obtained with improved crop management (Oswald et al., 2009). This means that the potential impact of introducing OFSP in farming and food systems on food security, nutrition, and income can be largely increased by investments in improved crop management.
The most frequently mentioned constraints for sweetpotato production, contributing to yield gaps, are drought, poor access to quality planting material, pests and pathogens, and poor soil fertility. In an expert survey, control of viruses and availability, and quality of planting material were identified as priority research needs for sweetpotato in the developing world, with control of weevils an additional priority for SSA (Fuglie, 2007). In participatory rural appraisals in Rwanda and Tanzania, farmers identified pest and diseases, drought stress, and availability of bred-cultivars and planting material as the main constraints limiting sweetpotato production (Ngailo et al., 2015; Rukundo, 2015).
van Vugt and Franke (2018) systematically analyzed factors contributing to sweetpotato yield gaps in SSA and found that the most yield limiting factor was total rainfall during the growing period, reported for 30% of the 221 on-farm plots included in their study. Second was elevation (23%), followed by planting date (21%), percentage crop establishment (18%) and rainfall exposure period (8%). Although sweetpotato is considered a drought tolerant crop, late planting does result in yield losses, which is related to water stress during the root initiation and main bulking periods. Nevertheless, these factors together could explain only 31% of the yield gap. There were only weak relationships between root yields and soil characteristics, and soil fertility constraints were, therefore, not captured in their model. Data on soil fertility limitations to sweetpotato yields in SSA are scarce. Niederwieser (2004) provides details on the amount of different nutrients removed by one ton of sweetpotato planting material and guidance on nutrient application rates when soil analysis is not feasible.
Potential Returns to Investing in Improved Crop and Cropping System Management for Enhancing Sweetpotato Productivity
Considerations on Crop Management Choices for Sweetpotato
Suitable best practices for sweetpotato (land preparation, planting methods, nutrient and pest and disease management, etc.) are available (Niederwieser, 2004; Mukhopadhyay et al., 2011; Stathers et al., 2013; Abidin and Carey, 2017), but need to be adapted to local contexts. Given that sweetpotato in SSA is often a secondary crop grown for food reserves rather than high yields, there has been limited interest by farmers to invest in improved crop management (Ewell and Mutuura, 1994; Andrade et al., 2009). This may explain why research on investments in improved crop management of sweetpotato in SSA has remained behind. In this context, when promoting improved crop management for sweetpotato, understanding trade-offs and synergies at farm level, farmer behavior and constraints for adoption, especially the need for market access and demand, is critical.
Addressing the Seed System Bottleneck
Sweetpotato is propagated using both roots and vines, the latter being widely important in Africa (McEwan, 2016). Unlike major cereal crops whose seeds can be safely stored, sweetpotato vine conservation during the dry season is a prevailing challenge causing a low seed security status and possible shift to other crops (Mudege et al., 2019). Sweetpotato vine conservation and multiplication practices are largely influenced by rainfall patterns. Planting materials are more available in bimodal rainfall areas than in unimodal rainfall areas affected by long dry spells (Barron et al., 2003; Biamah et al., 2005; Biazin and Sterk, 2013). The other challenge is lack of quality planting material mainly associated with contamination by various sweetpotato virus diseases, weevils (Cylas formicarius), sweetpotato butterfly (Acraea acerata), Alternaria blight (Alternaria spp.) and erinose mites (Aceria spp.) (Clark et al., 2012; McEwan, 2016). Generally, the lack of access to and availability of quality planting material at the beginning of the rainy season is a prevailing challenge against wider dissemination of improved sweetpotato cultivars across SSA (Gurmu et al., 2015; Mudege et al., 2019).
Addressing the seed system challenges requires a closer understanding of and building upon indigenous practices, innovative technologies and working with multi-stakeholder and cross-sectoral approaches (Gurmu et al., 2015; McEwan, 2016; Rajendran et al., 2017). The most widely practiced traditional seed conservation and multiplication practices by smallholder farmers in SSA include growing vines using residual moisture and in flood plains, growing vines under perennial crops such as fruits and enset, taking advantage of the shade to prevent vine desiccation (Gurmu et al., 2015; Mudege et al., 2019), and using volunteer roots that remained in the soil during previous harvests and start sprouting at the onset of the rainy season (McEwan, 2016). Recent efforts by CIP in collaboration with African national agricultural research institutes (NARIs) have focused on developing innovative sweetpotato seed system technologies such as Triple S (storage, sand, and sprouting) for root-based vine multiplication, the use of net tunnels to protect foundation material from insect vectors that spread viruses (Figure 4), sandponics for vine multiplication from pathogen-tested plantlets and irrigated vine multiplication linked to established decentralized vine multipliers.
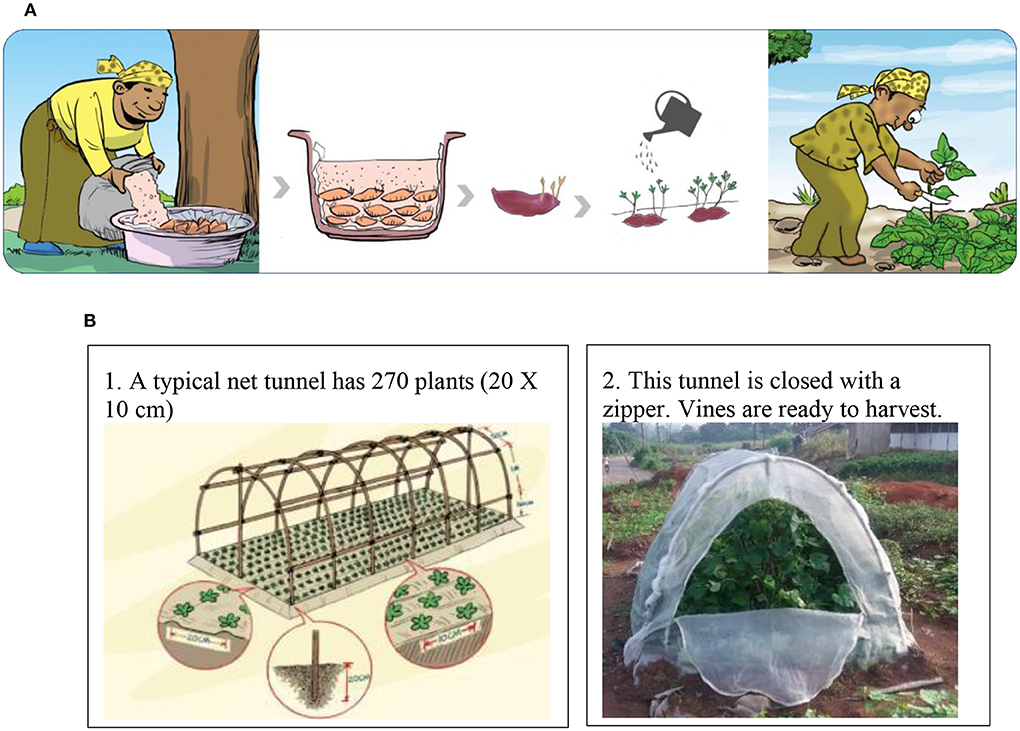
Figure 4. Technologies to enhance access to seed in drought prone areas (A. Triple S) and to protect a stock of high quality planting material from insect vectors (B. Net tunnels) developed under the SASHA research program. (A) Triple S is a root based method where small and medium roots are stored in layers of sand in the home during the dry season, then planted out in a nursery bed 6–8 weeks prior to the start of the rain and watered twice a week. On average one root gives 40 cuttings (each 30 cm long). (B) Net tunnels protect hardened disease-free cuttings obtained usually from national research programs from insect vectors. They are appropriate for use by vine multipliers in high virus pressure areas, enabling them to retain their own stock of starter material. Under good management, a net tunnel lasts 3 years. (Credit on photo: K. Ogero).
Pest and Disease Management
Sweet potato virus disease (SPVD) is the most serious and widely spread disease affecting sweetpotato in SSA (Gibson et al., 2004; Tairo et al., 2004; Valkonen et al., 2015). It is a result of a combined infection by Sweet potato chlorotic stunt virus (whitefly-transmitted crinivirus) and Sweet potato feathery mottle virus (aphid-transmitted potyvirus) (Gibson et al., 2004; Clark et al., 2012). In field trials in Uganda, SPVD resulted in 65 to 90% root yield losses (Adikini et al., 2016). Sweetpotato weevils (Cylas spp.) are the most important pest of sweetpotato in SSA with yield losses up to 100% (Okonya et al., 2016). van Vugt and Franke (2018) encountered weevil damage in 74–85% of on-farm sites in Malawi. In addition to viruses and weevils, other important sweetpotato pests and diseases are the sweetpotato butterfly (Acraea acerata), millipedes and rats (Ebregt et al., 2004a,b), and the fungal stem blight disease (Alternaria bataticola) (Osiru et al., 2007) and Fusarium wilt (Fusarium oxysporum f. sp. batatas) (Thompson et al., 2011). For a comprehensive review of major and minor pests and diseases of sweetpotato and their occurrence in SSA, refer to Ames et al. (1996).
As sweetpotato is a vegetatively propagated crop, pests and diseases easily spread through contaminated planting material (Reynolds et al., 2015). The use of disease-free or “clean” planting material is, therefore, paramount for good yields. Nevertheless, only a very small portion of sweetpotato farmers in SSA use quality-declared planting material. There is a need to intensify awareness raising efforts among farmers concerning the benefits of clean planting material, while strengthening the seed production and supply system. Other management strategies include field sanitation through removing and destroying diseased plants (rogueing), crop rotation and plot separation (for both virus and weevil control), and hilling up, mulching, and flooding (for weevil control) (Stathers et al., 2013). Farmers often lack knowledge of these practices or do not adopt them (Osiru et al., 2007). For instance, in a farmer survey by Ebregt et al. (2004a) in Uganda, most respondents did not see any problem in establishing new sweetpotato plots next to existing ones. van Vugt and Franke (2018) indicated that farmers can reduce weevil infestation by earlier harvesting, prior to soils drying and cracking5. The use of pesticides on sweetpotato is very low in SSA, except in Nigeria and Uganda, where use is increasing (Reynolds et al., 2015). Okonya et al. (2019) reported that 14% of farmers surveyed in Rwanda and Burundi used insecticides on sweetpotato, while none used herbicides or fungicides.
Low-cost options to control damage by pests and diseases may reside in cropping system innovations. Abidin et al. (2015) reported that strip intercropping sweetpotato with maize or onions reduced weevil damage in Malawi. In India, rotation with rice and cowpea, as well as intercropping with rice, cowpea, and maize reduced weevil infestation (Nedunchezhiyan et al., 2012). Rice-sweetpotato rotation in China reduced damage by weevils and other pests and diseases of sweetpotato due to the wet-dry cycle (Gitomer, 1996). Talekar (1987) showed, however, that rotations are not effective in reducing weevil damage when there is still a weevil source close to the field, indicating the importance of plot separation. In Indonesia, sweetpotato is strip intercropped with yam bean (Pachyrhizus spp.)—a storage root forming legume—to avoid or significantly reduce weevil damage (Karuniawan, 2004). Ichinose et al. (2019) indicated that intra-planting a sweetpotato cultivar preferred by weevils with a less preferred cultivar in the same field may reduce total weevil damage.
Soil Fertility Management
There is a general perception that sweetpotato can produce yields on marginal soils with very poor soil fertility. A modest sweetpotato root yield of 10 t ha−1 removes ~80 kg of potassium (K) ha−1, 50 kg of nitrogen (N), and 10 kg of phosphorus (P) with the harvest of the roots6, not including nutrients removed with the vines. This is approximately the same amount of N and P removed with a moderate to high yielding maize crop, and a much higher quantity of K is removed by sweetpotato than by maize6. This can explain why sweetpotato is considered by many farmers to be nutrient depleting, making some hesitant to intercrop sweetpotato with cash crops. Smallholder farmers in SSA rarely use inorganic or organic inputs on sweetpotato, in part because of the perceived tolerance to low soil fertility, but also because of lack of awareness and in some areas low prices of the roots relative to the cost of fertilization (Ngailo et al., 2015). This combined with the relatively high nutrient extraction rates implies that sweetpotato production may contribute significantly to soil nutrient depletion in SSA.
Despite its tolerance to poor soil fertility, sweetpotato responds to improved nutrient management. In Nigeria, sweetpotato yields were nearly doubled (from 7–10 to 14–17 t ha−1) by applying moderate rates of NPK and poultry manure, while yields increased by only 50% with application of high rates of either NPK or manure separately (Agbede, 2010); thus showing the benefit of integrated soil fertility management. van Vugt (2018) found that mean on-farm root yields increased from 11.8 to 14.5 t ha−1 with application of NPKS in combination with lime in Malawi. Uwah et al. (2013) found that mean root yields increased from 4 t ha−1 with no K application to 39 t ha−1 with 160 kg K ha−1. Nitrogen application rates should be moderated as too much N will lead to vigorous vine growth at the expense of root yield (Niederwieser, 2004; Kirchhof, 2009). For optimal response, appropriate fertilizer recommendations tailored to the nutrient requirements of sweetpotato and adapted to local soil conditions are needed. For maximal effectiveness, this should be coupled with breeding for improved nutrient efficiency (Villordon et al., 2014). This will require considerably more research investments on nutrient management and efficiency of sweetpotato, which to date have remained extremely low compared to crops such as maize (Villordon et al., 2014; Reynolds et al., 2015). Rationally, investments in improved nutrient management for sweetpotato should be made only in combination with improved cultivars and clean planting material.
Farmers need greater awareness of the benefits of improved nutrient management on sweetpotato, possibly through demonstrations. However, where sweetpotato is a secondary crop, farmers may just prioritize investments in fertilizers on other crops in their farming system. In this context, opportunities for improving nutrient supply to sweetpotato may reside in other cropping system options rather than directly applying fertilizers. For instance, intercropping sweetpotato with legumes such as pigeon pea or the yam bean (Karuniawan, 2004) could improve nitrogen supply to sweetpotato. Intercropping with a fertilized maize crop has been reported to benefit sweetpotato yields in Malawi (Abidin et al., 2015). Alternatively, sweetpotato may be rotated with fertilized cash crops such as maize, potato or rice (Kyalo and Lamo, 2017), and benefit from residual fertilizer effects (Ewell and Mutuura, 1994; Andrade et al., 2009). Depending on the crop preceding sweetpotato, the effectiveness of such residual effects could be increased by applying additional K to the sweetpotato crop on soils deficient in K, while relying on residual fertilizers for N and P.
Water Management
There is a wide acceptance in SSA of sweetpotato's ability to tolerate drought. Hence, smallholder farmers in SSA cultivate sweetpotato in drought-prone areas both during the wet and dry seasons (Lebot, 2010; Motsa et al., 2015; Markos, 2016). Farmers tend to grow sweetpotato either when the wet season rainfall is too low for cereals such as maize (Motsa et al., 2015) or during the dry season by establishing sweetpotato in the field before the end of the wet season. Daryanto et al. (2016) argued that the reputation of sweetpotato as a drought tolerant crop relates to its survival and recovery after dry periods rather than its yield potential under drought conditions. Unlike many cereals, sweetpotato can quickly recover following the onset of rains. This is a key characteristic for dryland areas, as survival of vines and immediate sprouting enables farmers to get vines on-time for main season planting, while also harvesting roots during the hunger period. However, water stress during establishment can substantially reduce root yield due to diminished adventitious root development and storage root formation (Belehu et al., 2004; Villordon et al., 2012; Solis et al., 2014). Root formation on freshly planted cuttings is optimal at a soil water content of 80% of field capacity. Nonetheless, considerable root formation still occurs even at 40% of field capacity (Belehu, 2003).
There has been meager research regarding the critical understanding of the water requirement and use efficiency of sweetpotato (Gomes and Carr, 2003; Gomes et al., 2005; Laurie et al., 2017). An important proxy for the water requirements of a crop is the crop coefficient (Kc), which relates crop water requirement to the reference evapotranspiration figure of a given area7. Based on an experimental study in a dry area of Mozambique, Gomes and Carr (2003) concluded that the Kc for each stage of sweetpotato growth was consistent across wet and dry seasons: at crop establishment: 0.55–0.7; at mid-season: 1.1–1.2; at end of the season: 0.80, with peak rates of crop evapotranspiration around 6 mm day−1. The maximum effective rooting depth of sweetpotato ranges from 0.6 to 1.6 meters (Allen et al., 1998; Rankine et al., 2015) depending on cultivar, soil type and crop management. However, much of the soil water is taken up from the top 25% of soil depth. Sweetpotato vines grow laterally and most extend adventitious roots that contribute to the total water absorption, thus resulting in drought tolerance. The crop requires 500 mm of water for a 4-month period under typical semi-arid growing conditions (King, 1985; Onyekwere and Nweinyi, 1989; Chukwu, 1995).
Although sweetpotato has been predominantly grown in rainfed systems in SSA, there are some efforts in Southern Africa and elsewhere to grow irrigated sweetpotato for improved yields and staggered production of vines and roots. Research in SSA and the USA revealed that sweetpotato root yields can be significantly reduced when irrigation levels drop below 30% of the crop evapotranspiration (ETc) (Ekanayake and Collins, 2004; Laurie et al., 2009; Kivuva et al., 2014; Solis et al., 2014). The total root yields of sweetpotato in South Africa and Ethiopia were not significantly different between irrigation levels at 60 and 100% ETc, thereby implying that deficit irrigation at 40% of the crop water requirement can be recommended under water scarce conditions (Laurie R. N. et al., 2015; Alemayehu, 2019). The water use efficiency of sweetpotato varies between 11 and 90 kg ha−1 mm−1 depending on cultivar, agro-climate, soil types, and management (Gomes and Carr, 2003; Laurie et al., 2012; Masango, 2015; Ntsieni, 2017), with an average of 65 kg ha−1 mm−1. The beta-carotene concentration of OFSP (per unit of weight) was 15–34% higher at a low irrigation level (30% ETc) compared to optimal irrigation (100% ETc), although the total beta-carotene yield per hectare was higher for the latter due to associated yield increments (Laurie et al., 2012; Nyathi et al., 2019). Ntsieni (2017) quantified ETc dynamics under a range of growing conditions and determined average crop coefficient (Kc) values to be 0.46, 0.92, and 0.57 during initial, middle, and late growing stages. Growing OFSP using an infield water harvesting system showed significantly higher yields than that with conventional tillage; thus enabling farmers in semi-arid areas to grow small plots of OFSP and add vitamin A to their diets (Laurie et al., 2017). Yooyongwech et al. (2016) reported that inoculation of arbuscular mycorrhizal fungi8 in sweetpotato plants improves plant growth characteristics and enhances tolerance to water deficit via soluble sugars and free proline accumulation.
Potential Contributions of Sweetpotato to Cropping and Farming Systems Sustainability
Contributions of Sweetpotato to Cropping and Farming Systems Productivity and Resource Use Efficiency
Although intercropping sweetpotato with other crops is common in SSA, few research articles have addressed its impact on systems' productivity, soil conservation, and resource use efficiency. Land equivalent ratios above one have been found for strip intercropping OFSP with maize, onion, and soybean in Malawi (Abidin et al., 2015) and maize-sweetpotato intercropping systems in Uganda (Asiimwe et al., 2016). Maize yields were not affected by simultaneously interplanting sweetpotato in Ethiopia (Amede and Nigatu, 2001). Intercropping sweetpotato with crops such as maize may help to reduce soil erosion, improve soil moisture conservation, and reduce weed pressure, as sweetpotato rapidly develops a dense foliage (Liebman and Dyck, 1993; Mukhopadhyay et al., 2011; Nedunchezhiyan et al., 2012; Weerarathne et al., 2017).
Amede and Nigatu (2001) suggested that a maize-sweetpotato intercrop is beneficial by providing vines as feed in addition to the maize grain yield. This could support more sustainable crop-livestock systems and improve the availability of manure for soil fertility management at the farm level. In an ex-ante assessment, Claessens et al. (2008) demonstrated that integrating dual-purpose sweetpotato in crop-livestock systems could economically benefit farmers, but optimal strategies of defoliation are needed to minimize penalties on root yields. Trade-offs between root and vine yield were found in Kenya by Lukuyu et al. (2014), who suggested selecting cultivars based on feed needs in farms.
Rotating rice with sweetpotato in Uganda led to higher rice and sweetpotato yields and revenue to cost ratios compared to monocropping (Kyalo and Lamo, 2017). The increased rice yields were hypothesized to involve improved water percolation in the rotation plots compared to the control. Rotating rice with sweetpotato in lowlands could also break pest and disease cycles in otherwise continuously monocropped rice.
Sweetpotato as a Food Security Crop
The contribution of sweetpotato to smallholders' food security results from several characteristics including: (1) its capacity to produce under marginal conditions (drought and low soil fertility) and with lower labor inputs compared to other crops; (2) its capacity to produce a high amount of energy per unit of land and time; and (3) the large harvest window that allows piecemeal harvesting of both roots and leaves throughout the year, in the absence of high weevil populations (Low et al., 2009; Mudege et al., 2019). Because of the first two characteristics, sweetpotato vines are often distributed to mitigate disasters (Kapinga et al., 1995; Motsa et al., 2015). For the same reasons, sweetpotato can grow in very short rainy seasons not suitable for most other crops, such as “Season C” in Rwanda (NISR, 2018). An important food security aspect of drought tolerant sweetpotato is that it can mature and be harvested during the hunger period (Ewell and Mutuura, 1994; Motsa et al., 2015; Mudege et al., 2019). Staggered harvesting and selling on local markets also helps smallholder farmers meet timely cash needs (Ewell and Mutuura, 1994), thereby enhancing income stability. Alvaro et al. (2017) reported that for some non-early maturing cultivars, harvesting can commence from 90 days after planting with root yields increasing up to 180 days after planting. Delayed harvesting may lead, however, to increased weevil damage (Smit, 1997; Ebregt et al., 2004b; Alvaro et al., 2017) and cause a decline in the root quality due to cracking. The benefits of piecemeal harvesting can be maximized by growing cultivars with varying maturity periods in the same farm (Ewell and Mutuura, 1994). Decision-support systems based on knowing the yield impacts of staggered planting for specific locations or agroecological zones could help farmers optimize returns and household food security.
Outlook
Sub-Saharan Africa needs to produce more food to feed its rapidly growing population but given the high rates of micronutrient malnutrition among young children and women of childbearing age, this food must have higher micronutrient density. Sweetpotato roots and leaves9 are both highly nutritious and breeders are committed to increasing the iron content in OFSP to reach biofortified levels (Low J. W. et al., 2017). Sweetpotato is a complex hexaploid, whose genetic diversity makes it one of the few crops that grows from sea level to 2,400 meters on the continent. In many parts of SSA, prolonged drought periods are a major constraint for sweetpotato farming, especially in Southern Africa. Areas facing water scarcity are likely to increase substantially by 2030, and soil degradation is already widespread.
From 2009 through 2019, the sweetpotato breeding network of 14 SSA countries has bred 98 new cultivars in Africa, of which 60 (61%) were orange-fleshed and 5 (5%) were purple-fleshed (Okello et al., 2019). Africa still needs more high yielding sweetpotato cultivars with host plant resistance plus high dry matter content in the storage roots under drought. At the moment, only 3 countries (Mozambique, Malawi, Kenya) report drought tolerance as being a key selection trait; but an additional 7 countries (Ethiopia, Mozambique, Madagascar, Zambia, South Africa, Burkina Faso, and Ghana) breed for earliness, which is much less costly to select for. The continued sharing of true (botanical) hybrid seeds and breeding methods among crossing programs worldwide may further accelerate breeding gains and exploit heterosis for storage root yields. In fact, from 2014 through 2019, 11 of the new cultivars released were selected from hybrid seed from CIP population development platforms (Mwanga et al., 2019). The success of any breeding program should be measured as the genetic improvement in target traits over time, plus the adoption and impact of released cultivars. Key traits for sweetpotato breeding in SSA include, as noted before, high storage root yield, high dry matter, and enhanced beta-carotene content but the population development programs have focused on different traits, e.g., virus resistance in Uganda; drought tolerance plus increased iron and zinc contents in Mozambique; and reduced sweetness and perishability in Ghana (Mwanga et al., 2017).
The call for sustainable intensification in the context of climate change calls for faster and smarter breeding, but also gives significant attention to improved crop management practices, to maximize efficient utilization of water and added nutrients. The length of the growing period (LGP) reflects key conditions for crop growth, including soil, and temperature conditions and the adequacy of moisture availability. For most of SSA, the LGP is projected to decrease by up to 20% by 2050 (Thornton et al., 2011). An examination of various climate trend scenarios through 2050 and associated predictions of nutrition outcomes, found that even under the most optimistic scenario, micronutrient deficiencies are expected to remain widespread in most of the world through at least mid-century. Moreover, recent studies show micronutrient malnutrition will intensify under climate change. First, due to rising CO2 levels driven by climate change, plants will probably lose nutritional value. However, overall yield in a crop like sweetpotato is actually expected to rise, even though nutrient density is anticipated to decline (Myers et al., 2014). Hence, breeding concurrently for increased micronutrient content and improved crop resilience is requisite. In this context, sweetpotato is a key candidate for increased research investments, as it is both a nutrient-dense crop and a potential climate winner, but nevertheless highly under researched as noted by Manners and van Etten (2018).
In terms of breeding for drought-prone conditions, two distinct classes of cultivars are emerging:
1. Early maturing cultivars (<4 month LGP) that escape drought. Short duration, nutrient-rich sweetpotato cultivars will enable sweetpotato use to expand in the dry sub-humid zone of West Africa and other parts of the cereal-root crop mixed farming system. In addition, these cultivars can also serve the humid, highland perennial farming system, where small landholding size is driving demand for earlier maturing cultivars. Early maturing cultivars could easily be integrated as rotation crops in rice irrigation schemes (the irrigated and rice-tree farming systems) and vegetable production efforts (in peri-urban zones). With irrigation, these cultivars will also be able to expand into agro-pastoral millet/sorghum zones, where feed use is expected to be as important as food use. The seed systems, however, would differ. In the highland perennial farming systems, vine conservation would be the norm; in dry sub-humid zones, the root-based Triple S method would be preferred. The latter would require breeders to ensure that selected cultivars perform well in the Triple S system and that storage roots exhibit reasonable shelf-life under ambient conditions once harvested.
2. Medium maturing and nutrient-rich cultivars (4–6 months LGP) that avoid drought (deep roots), are drought tolerant (assimilation continues under drought stress), and exhibit continuous root formation. These sweetpotato cultivars will be particularly important for assuring food security in farming systems with substantial maize production, which is expected to experience significant yield declines under climate change (Thornton et al., 2011). Use of these cultivars would be appropriate in intercropping and strip cropping situations in combination with cereal and legume crops.
Ideally in maize-mixed, cereal-root crop, and root crop farming systems, both early and medium maturity cultivars would be introduced into the same household, which would assure root availability for 6–7 months in unimodal growing areas. The dominant smallholder practice for obtaining seed under these conditions is for roots to be left in the ground, that sprout with the next rains or plots of vines are sustained in valley bottoms with residual moisture. In this case, selecting for vine vigor, in-ground storability, and ability to sprout would be key. Deep rooting cultivars would be included to avoid weevil infestation during the dry season. Again, given the importance of livestock in these systems, emphasis on dual purpose cultivars is warranted.
Beyond genetic enhancements, the sustainability of sweetpotato farming depends on the sweetpotato production systems in different dominant farming systems. With improved crop management systems, current sweetpotato yields can be increased 2–4 times. Overall, a lack of data on the magnitude of yield gaps and the factors contributing to yield gaps in different agro-ecological zones limits our understanding of constraints to sweetpotato production across SSA. Large-scale mapping of yield gaps and factors contributing to determining yield gaps could assist in prioritizing target research-for-development areas (van Oort et al., 2017) for closing yield gaps.
Dissemination and wider adoption of OFSP can be achieved through proper tackling of the value chain challenges from accessing planting materials of adapted cultivars to processing and consumption barriers. Thus, future efforts to promote OFSP in SSA should increase awareness among rural and urban consumers, develop recipes to integrate OFSP into local culinary systems, and support processors to aptly develop and scale up OFSP-based products (e.g., bread, juices, biscuits, chips). This would make OFSP more convenient and diverse for urban consumers and increase market demand, thus encouraging producers to engage in staggered production using supplementary irrigation systems to supply markets year-round. Ideally, gender-transformative value chain development approaches should be used to improve women's access to training, information, financial services, and market opportunities (Mudege et al., 2017). Analyzing each stage along the value chain (inputs, production, postharvest handling, processing, distribution, marketing, and consumption) will enable the identification of appropriate actions to be pursued by private and public sector actors. Such analysis will reveal constraints to be addressed through research as well as opportunities favoring production, postharvest handling investments, and markets for sweetpotato vine multipliers, growers and processors in SSA. Increasing diversified use will also increase the number of traits which must be integrated alongside drought tolerance in breeding programs, including shape, more refined organoleptic parameters, ability to withstand damage during transport and post-harvest storability.
Despite known potential positive benefits of sweetpotato in cropping systems, the quantified contributions of sweetpotato to productivity increases, soil conservation and resource use efficiency remain poorly described. A much deeper understanding of root system architecture and how to exploit it is needed (Khan et al., 2016). As sweetpotato is highly competitive for soil nutrients, the success of intercrops with sweetpotato also depends on the correct spacings, crop combinations, and planting times (Oswald et al., 2009), but little information on such optimized intercrops is available. New research efforts should fill those gaps. As the crop increasingly commercializes, willingness to invest in improved agronomic practice and increased input use to improve soil and water management will grow.
Combined improved conventional methods and gene editing techniques will be needed to develop drought-tolerant sweetpotato cultivars more quickly, and this should be accompanied by site-specific agronomic packages to increase productivity. Our vision is to reposition sweetpotato as a key crop to combat desertification and drought. Among major food crops, it is perhaps the crop with the most pronounced adaptation to semi-arid areas and drought. It provides food for growing populations and feed for animals vital for sustained livelihoods in dry lands. However, in a semi-arid modern world, it is critical that sweetpotato becomes also an ingredient for the food industry. The availability of low sugar cultivars is likely to enhance the use of the crop by the food industry. Widespread integration of sweetpotato as an ingredient in processed food products may be a key driver to unfold the crop's potential in the dry land world.
Consent for Publication
Written informed consent was obtained from the individual for the publication of Image 3.
Author Contributions
JL, RO, EV, MA, BB, and WG all contributed to the writing of this article. RO, EV, and JL contributed to the editing of the article. JL developed the outline and assured compliance with author guidelines.
Conflict of Interest
The authors declare that the research was conducted in the absence of any commercial or financial relationships that could be construed as a potential conflict of interest.
Acknowledgments
Time of all authors from the International Potato Center was covered by the Sweetpotato Action for Security and Health in Africa project, funded by the Bill & Melinda Gates Foundation [OPP1019987]. This research undertaken is also part of, and benefited from funding by, the CGIAR Research Program on Roots, Tubers and Bananas and supported by CGIAR Trust Fund contributors (https://www.cgiar.org/research/).
Footnotes
1. ^That is, selecting a new set of parents for further crossing within the breeding population for a target population of environments.
2. ^The Institute of Agrarian Research of Mozambique (IIAM) is the national research program in Mozambique. The CIP Southern Africa breeding program is based at their station.
3. ^Available at: https://datacatalog.worldbank.org/dataset/world-external-geospatial-platforms-population/resource/6bf27ac7-e596-4450-85cb-cf911648605c.
4. ^FAO. The progression from arable cropping to integrated crop and livestock production [Online]. Available: www.fao.org/3/X6543E/X6543E03.htm.
5. ^Weevils cannot dig, so they reach roots to lay eggs when soils crack.
6. ^IPNI Crop Nutrient Removal Calculator: http://www.ipni.net/article/IPNI-3346.
7. ^The crop's water use can be determined by multiplying the calculated evapo-transpiration (ET) by a crop coefficient, Kc, which considers the difference in ET between the crop and reference evapotranspiration.
8. ^In an arbuscular mycorrhizal fungus, the fungus penetrates the cortical cells of the roots of a vascular plant.
9. ^In some countries, sweetpotato leaves are widely consumed; in others not. These leaves contain health-promoting components such as lutein, carotenoids, anthocyanins and caffesoyquinic acids (Tanaka et al., 2017), which led to releasing some sweetpotato cultivars with such traits in Japan.
References
Abidin, P. E., and Carey, E. (2017). Sweetpotato Cropping Guide. Nairobi: Africa Soil Health Consortium.
Abidin, P. E., Chipungu, F., Nyekanyeka, T., Chilanga, T., Mwenye, O., Kazembe, J., et al. (2015). “Maize-orange-fleshed sweetpotato intercropping: potential for use to enhance food security and scaling-up the nutrition effort in Malawi,” in Potato and Sweetpotato in Africa. Transforming the Value Chains for Food and Nutrition Security, eds J. Low, M. Nyongesa, S. Quinn, and M. Parker (Oxfordshire: CABI International), 405–413.
Adikini, S., Mukasa, S. B., Mwanga, R. O. M., and Gibson, R. W. (2016). Effects of sweet potato feathery mottle virus and sweet potato chlorotic stunt viruson the yield of sweet potato in uganda. J. Phytopathol. 164, 242–254. doi: 10.1111/jph.12451
Agbede, T. M. (2010). Tillage and fertilizer effects on some soil properties, leaf nutrient concentrations, growth and sweet potato yield on an alfisol in southwestern Nigeria. Soil Tillage Res. 110, 25–32. doi: 10.1016/j.still.2010.06.003
Agili, S., International potato center, N., Kenya, B., Nyende, K., and Ngamau, P. (2012). Selection, yield evaluation, drought tolerance indices of orange-flesh sweet potato (Ipomoea batatas Lam) hybrid clone. J. Nutr. Food Sci. 2:1–8. doi: 10.4172/2155-9600.1000138
Alemayehu, A. (2019). Growth, Yield and Physiological Response of Sweetpotato (Ipomoea Batatas (L.) LAM.) Genotypes to Different Deficit Irrigation Levels at Hawassa Zuriya Woreda, Southern Ethiopia (Master's Thesis). Hawassa University, Hawassa.
Allen, J. C., Corbitt, A. D., Maloney, K. P., Butt, M. S., and Truong, V.-D. (2012). Glycemic index of sweet potato as affected by cooking methods. Open Nutr. J. 6, 1–11. doi: 10.2174/1874288201206010001
Allen, R. G., Pereira, L. S., Raes, D., and Smith, M. (1998). Crop Evapotranspiration (Guidelines for Computing Crop Water Requirements). Rome: FAO.
Alvaro, A., Andrade, M. I., Makunde, G. S., Dango, F., Idowu, O., and Grüneberg, W. (2017). Yield, nutritional quality and stability of orange-fleshed sweetpotato cultivars successively later harvesting periods in Mozambique. Open Agric. 2, 464–468. doi: 10.1515/opag-2017-0050
Amede, T., and Nigatu, Y. (2001). Interaction of components of sweetpotato-maize intercropping under the semi-arid conditions of the Rift-Valley, Ethiopia. Trop. Agric. 78, 1–7. Available online at: https://journals.sta.uwi.edu/ta/index.asp?action=viewPastAbstract&articleId=1395&issueId=195
Ames, T., Smit, N., Braun, A. R., O'Sullivan, J. N., and Skoglund, L. G. (1996). Sweetpotato: Major Pests, Diseases, and Nutritional Disorders. Lima: International Potato Center (CIP).
Andrade, M., Barker, I., Cole, D., Dapaah, H., Elliott, H., Fuentes, S., et al. (2009). Unleashing the Potential of Sweetpotato in Sub-Saharan Africa: Current Challenges and Way Forward. Lima: International Potato Center (CIP)).
Andrade, M. I., Alvaro, A., Menomussanga, J., Makunde, G. S., Ricardo, J., Grüneberg, W. G., et al. (2016a). 'Alisha', 'Anamaria', 'Bie', 'Bita', 'Caelan', 'Ivone', 'Lawrence', 'Margarete', 'Victoria', Sweetpotato. HortScience 51, 597–600. doi: 10.21273/HORTSCI.51.5.597
Andrade, M. I., Makunde, G. S, Ricardo, J., Menomussanga, J., Alvaro, A., Grunberg, et al. (2017a). Survival of sweetpotato (Ipomoea batatas [L] Lam) vines in cultivars subjected to long dry spells after the growing season in Mozambique. Open Agric. 2, 58–63. doi: 10.1515/opag-2017-0006
Andrade, M. I., Naico, A., Ricardo, J., Eyzaguirre, R., Makunde, G. S., Ortiz, R., et al. (2016b). Genotype × environment interaction and selection for drought adaptation in sweetpotato (Ipomoea batatas [L.] Lam.) in Mozambique. Euphytica 209, 261–280. doi: 10.1007/s10681-016-1684-4
Andrade, M. I., Ricardo, J., Naico, A., Alvaro, A., Makunde, G. S., Low, J. W., et al. (2017b). Release of orange-fleshed sweetpotato (Ipomoea batatas [l.] Lam.) cultivars in Mozambique through an accelerated breeding scheme. J. Agric. Sci. 155:919–29. doi: 10.1017/S002185961600099X
Anselmo, B. A., Ganga, Z. N., Badol, E. O., Heimer, Y. M., and Nejidat, A. (1998). Screening sweetpotato for drought tolerance in the Philippine highlands and genetic diversity among selected genotypes. Trop. Agric. 75, 189–196.
Asiimwe, A., Tabu, I. M., Lemaga, B., and Tumwegamire, S. (2016). Effect of maize intercrop plant densities on yield and β-carotene contents of orange-fleshed sweetpotatoes. African Crop Sci. J. 24:75–87. doi: 10.4314/acsj.v24i1.6
Bararyenya, A., Olukolu, B. A., Tukamuhabwa, P., Grüneberg, W. J., Ekaya, W., Low, J., et al. (2020). Genome-wide association study identified candidate genes controlling continuous storage root formation and bulking in hexaploid sweetpotato. BMC Plant Biol. 20:1–16. doi: 10.1186/s12870-019-2217-9
Bararyenya, A., Tukamuhabwa, P., Gibson, P., Gruneberg, W., Ssali, R., Low, J., et al. (2019). Continuous storage root formation and bulking in sweetpotato [version 2; referees: awaiting additional peer review]. Gates Open Res. 3, 1–27. doi: 10.12688/gatesopenres.12895.2
Barrett, C. B., and Bevis, L. E. M. (2015). “The micronutrient deficiencies challenge in african food systems,” in The Fight Against Hunger and Malnutrition: The Role of Food, Agriculture, and Targeted Policies, ed. D.E. Sahn (Oxford: Oxford University Press), 61–88.
Barron, J., Rockström, J., Gichuki, F., and Hatibu, N. (2003). Dry spell analysis and maize yields for two semi-arid locations in east Africa. Agric. For. Meteorol. 117, 23–37. doi: 10.1016/s0168-1923(03)00037-6
Beal, T., Massiot, E., Arsenault, J. E., Smith, M. R., and Hijmans, R. J. (2017). Global trends in dietary micronutrient supplies and estimated prevalence of inadequate intakes. PLoS ONE 12:e0175554. doi: 10.1371/journal.pone.0175554
Belehu, T. (2003). Agronomical and Physiological Factors Affecting Growth, Development and Yield of Sweet Potato in Ethiopia (Dissertation). University of Pretoria, Pretoria.
Belehu, T., Hammes, P. S., and Robbertse, P. J. (2004). The origin and structure of adventitious roots in sweet potato (Ipomoea batatas). Aust. J. Bot. 52, 551–558. doi: 10.1071/BT03152
Belesova, K., Agabiirwe, C. N., Zou, M., Phalkey, R., and Wilkinson, P. (2019). Drought exposure as a risk factor for child undernutrition in low- and middle-income countries: a systematic review and assessment of empirical evidence. Environ. Int. 131:104973. doi: 10.1016/j.envint.2019.104973
Bernstein, L. (1974). Crop growth and salinity, in Drainage for Agriculture. Agronomy Monograph 17, ed. J. van Schilfgaarde (Madison, WI: Soil Science Society of America), 39–54.
Biamah, E. K., Sterk, G., and Sharma, T. C. (2005). Analysis of agricultural drought in Iiuni, Eastern Kenya: application of a markov model. Hydrol. Process. 19, 1307–1322. doi: 10.1002/hyp.5556
Biazin, B., and Sterk, G. (2013). Drought vulnerability drives land-use and land cover changes in the rift valley dry lands of ethiopia. Agric. Ecosyst. Environ. 164, 100–113. doi: 10.1016/j.agee.2012.09.012
Biazin, B. T., Low, J., Brouwer, R., Feyissa, T. A., WoldeGiorgis, M., and Ayele (2019). “Sweetpotato marketing in southern Ethiopia: Examining market chains, seasonal availability, volume and price fluctuations at district and provincial markets,” in Ethiopian Economics Association Conference (Hawassa: Ethiopian Economics Association), 1–11.
Birol, E., Meenakshi, J. V., Oparinde, A., Perez, S., and Tomlins, K. (2015). Developing country consumers' acceptance of biofortified foods: a synthesis. Food Secur. 7, 555–568. doi: 10.1007/s12571-015-0464-7
Boko, M., Niang, I., Nyong, A., Vogel, C., Githeko, A., Medany, M., et al. (2007). “Africa,” in Climate Change 2007: Impacts, Adaptation and Vulnerability. Contribution of Working Group II to the Fourth Assessment Report of the Intergovernmental Panel on Climate Change, eds. M. L. Parry, O. F. Canziani, J. P. Palutikof, P. J. van der Linden, and C. E. Hanson (Cambridge: Cambridge University Press), 433–467.
Bouis, H. E. (2002). Plant breeding: a new tool for fighting micronutrient malnutrition. J. Nutr. 132, 491S−494S. doi: 10.1093/jn/132.3.491S
Bouis, H. E., Saltzman, A., and Birol, E. (2019). “Improving nutrition through biofortification,” in Agriculture for Improved Nutrition: Seizing the Momentum, eds S. Fan, S. Yosef and R. Pandya-Lorch (Wallingford: International Food Policy Research Institute (IFPRI), CAB International), 47–57.
Bovell-Benjamin, A. C. (2007). Sweet potato: a review of its past, present, and future role in human nutrition. Adv. Food Nutr. Res. 52, 1–59. doi: 10.1016/S1043-4526(06)52001-7
Cabrera-Bosquet, L., Crossa, J., von Zitzewitz, J., Serret, M. D., and Luis Araus, J. (2012). High-throughput phenotyping and genomic selection: the frontiers of crop breeding converge. J. Integr. Plant Biol. 54, 312–320. doi: 10.1111/j.1744-7909.2012.01116.x
Chávez, R., Mendoza, H., Mahesh, U., Espinoza, J., Cabello, R., Arévalo, N., et al. (2000). Genetic improvement and adaptation of sweet potato (Ipomoea batatas) to dry and saline conditions. IDESIA 18:97.
Chenu, K., Van Oosterom, E. J., McLean, G., Deifel, K. S., Fletcher, A., Geetika, G., et al. (2018). Integrating modelling and phenotyping approaches to identify and screen complex traits: transpiration efficiency in cereals. J. Exp. Bot. 69, 3181–3194. doi: 10.1093/jxb/ery059
Chipungu, F., Mkandawire, T., Benesi, I., Pamkomera, P., Mwenye, O., Abidin, E., et al. (2011). Proposal to Release LU06/0146, LU06/0252, LU06/0527, LU06/0428, BVU07/028, BV07/008 AND BV07/016 Sweetpotato Varieties in Malawi. Lilongwe: Department of Agricultural Research Services.
Chukwu, G. O. (1995). Crop irrigation water needs of sweet potato (Ipomoea batatas). African J. Root Tuber Crops 1, 35–38.
Claessens, L., Stoorvogel, J. J., and Antle, J. M. (2008). Ex ante assessment of dual-purpose sweet potato in the crop–livestock system of western Kenya: a minimum-data approach. Agricu. Syst. 99, 13–22. doi: 10.1016/j.agsy.2008.09.002
Clark, C., Davis, J., Abad, J., Cuellar, W., Fuentes, S., Kreuze, J., et al. (2012). Sweetpotato viruses: 15 years of progress on understanding and managing complex diseases. Plant Dis. 96, 168–185. doi: 10.1094/PDIS-07-11-0550
Clasen, B. M., Stoddard, T. J., Luo, S., Demorest, Z. L., Li, J., Cedrone, F., et al. (2016). Improving cold storage and processing traits in potato through targeted gene knockout. Plant Biotechnol. J. 14, 169–176. doi: 10.1111/pbi.12370
Collins, N. C., Tardieu, F., and Tuberosa, R. (2008). Quantitative trait loci and crop performance under abiotic stress: where do we stand? Plant Physiol. 147, 469–486. doi: 10.1104/pp.108.118117
Daryanto, S., Wang, L., and Jacinthe, P.-A. (2016). Drought effects on root and tuber production: a meta-analysis. Agric. Water Manag. 176, 122–131. doi: 10.1016/j.agwat.2016.05.019
David, M. C., Diaz, F. C., Mwanga, R. O. M., Tumwegamire, S., Mansilla, R. C., and Grüneberg, W. J. (2018). Gene pool subdivision of East African sweetpotato parental material. Crop Sci. 58, 1–13. doi: 10.2135/cropsci2017.11.0695
Development Initiatives (2018). 2018 Global Nutrition Report: Shining a Light to Spur Action on Nutrition. Development Initiatives, Bristol.
Ding, C. W., Niu, F. X., Guo, X. D., and Hua, X. X. (1997). Identification on the drought resistance in sweetpotato genetic resources. J. Henan Agric. Sci. 10, 3–5.
Dixon, J., Gulliver, A., Gibbon, D., and Hall, M. (2001). Farming Systems and Poverty: Improving Farmers' Livelihoods in a Changing World. Washington, DC: World Bank.
Ebregt, E., Struik, P. C., Abidin, P. E., and Odongo, B. (2004a). Farmers' information on sweet potato production and millipede infestation in north-eastern Uganda. I. Associations between spatial and temporal crop diversity and the level of pest infestation. NJAS - Wageningen J. Life Sci. 52, 47–68. doi: 10.1016/s1573-5214(04)80029-0
Ebregt, E., Struik, P. C., Abidin, P. E., and Odongo, B. (2004b). Farmers' information on sweet potato production and millipede infestation in north-eastern Uganda. II. Pest incidence and indigenous control strategies. Wageningen J. Life Sci. 52, 69–84. doi: 10.1016/s1573-5214(04)80030-7
Ekanayake, I. J., and Collins, W. (2004). Effect of irrigation on sweet potato root carbohydrates and nitrogenous compounds. J. Food Agric. Environ. 2, 243–248. doi: 10.1234/4.2004.130
Elston, R. C. (1963). A weight-free index for the purpose of ranking or selection with respect to several traits at a time. Biometrics 19, 669–680.
Ewell, P. T., and Mutuura, J. (1994). Sweet potato in food systems of Eastern and Southern Africa. Acta Horticu. 380, 405–412.
FAO IFAD, UNICEF, WFP, and WHO. (2019). The State of Food Security and Nutrition in the World 2019: Safeguarding Against Economic Slowdowns and Downturns. Rome: FAO.
FAO IFAD, UNICEF, World Food Program (WFP) and WHO. (2018). The State of Food Security and Nutrition in the World 2018: Building Climate Resilience for Food Security and Nutrition (Rome: FAO).
FAOSTAT (2019). Available online at: http://www.fao.org/faostat/en/ (accessed December 4, 2019).
Fiorani, F., and Schurr, U. (2013). Future scenarios for plant phenotyping. Ann. Rev. Plant Biol. 64, 267–291. doi: 10.1146/annurev-arplant-050312-120137
Fuglie, K. O. (2007). Priorities for sweetpotato research in developing countries: results of a survey. HortScience 42, 1200–1206. doi: 10.21273/HORTSCI.42.5.1200
Gakige, J. K., Gachuri, C., Butterbach-bahl, K., and Goopy, J. P. (2020). Sweet Potato (Ipomoea batatas) Vine Silage: A Cost-Effective Supplement for Milk Production in Smallholder Dairy-Farming Systems of East Africa? CSIRO Publishing: Animal Production Science. Available online at: https://www.publish.csiro.au/AN/pdf/AN18743
Gallais, A. (2003). Quantitative Genetics and Breeding Methods in Autopolyploid Plants. Paris, France: Institut National de la Recherche Agronomique.
Gibson, R. W., Aritua, V., Byamukama, E., Mpembe, I., and Kayongo, J. (2004). Control strategies for sweet potato virus disease in Africa. Virus Res. 100, 115–122. doi: 10.1016/j.virusres.2003.12.023
Gitomer, C. S. (1996). Potato and Sweetpotato in China: Systems, Constraints and Potential. Lima: International Potato Center (CIP).
Gomes, F., and Carr, M. K. V. (2003). Effects of water availability and vine harvesting frequency on the productivity of sweet potato in Southern Mozambique. II. crop water use. Exp. Agric. 39, 39–54. doi: 10.1017/s0014479702001047
Gomes, F., Carr, M. K. V., and Squire, G. R. (2005). Effects of water availability and vine harvesting frequency on the productivity of sweet potato in Southern Mozambique. IV. radiation interception, dry matter production and partitioning. Exp. Agric. 41, 93–108. doi: 10.1017/s0014479704002352
Grüneberg, W., Mwanga, R., Andrade, M., and Espinoza, J. (2009). Selection Methods. Part 5: Breeding Clonally Propagated Crops. Rome: Food and Agriculture Organization of the United Nations (FAO), 275–322.
Grüneberg, W. J., Ma, D., Mwanga, R. O. M., Carey, E. E., Huamani, K., Diaz, F., et al. (2015). “Advances in sweetpotato breeding from 1992 to 2012,” in Potato and Sweetpotato in Africa: Transforming the Value Chains for Food and Nutrition Security, eds J. Low, M. Nyongesa, S. Quinn, and M. Parker (Oxfordshire: CAB International), 3–68.
Grüneberg, W. J., Mwanga, R., Andrade, M., and Dapaah, H. (2009). “Sweetpotato breeding,” in Unleashing the Potential of Sweetpotato in Sub-Saharan Africa: Current Challenges and Way Forward. Working Paper 2009-1, eds M. Andrade, I. Barker, D. Cole, H. Dapaah, H. Elliott, S. Fuentes, W. J. Grüneberg, K. Kapinga, J. Kroschel, R. Labarta, B. Lemaga, C. Loechl, J. Low, J. Lynam, R. Mwanga, O. Ortiz, A. Oswald, and G. Thiele (Lima: International Potato Center (CIP)), 1–42.
Grüneberg, W. J., Swanckaert, J., and Low, J. W. (2019). A breakthrough: hybrid population breeding validated in Peru, Uganda, and Mozambique. SASHA Brief. No. 2 (International Potato Center (CIP)), 6. Available online at: https://hdl.handle.net/10568/105971
Gurmu, F., Hussein, S., and Laing, M. (2015). Diagnostic assessment of sweetpotato production in Ethiopia: constraints, post-harvest handling and farmers' preferences. Res. Crops 16, 104–115. doi: 10.5958/2348-7542.2015.00016.9
Hidosa, D., and Guyo, M. (2017). Climate change effects on livestock feed resources: a review. J. Fisheries Livest. Prod. 5:259. doi: 10.4172/2332-2608.1000259
Hijmans, R., Huaccho, L., and Zhang, D. (2002). “Description and analysis of a geo-referenced database of the global distribution of sweetpotato area,” in Proceedings, First International Conference on Sweet Potato. Food and Health for the Future (Lima: Acta Horticulturae) 583, 41–49.
Hotz, C., Loechl, C., de Brauw, A., Eozenou, P., Gilligan, D., Moursi, M., et al. (2012a). A large-scale intervention to introduce orange sweet potato in rural Mozambique increases vitamin A intakes among children and women. Br. J. Nutr. 108, 163–176. doi: 10.1017/S0007114511005174
Hotz, C., Loechl, C., Lubowa, A., Tumwine, J. K., Ndeezi, G., Nandutu Masawi, A., et al. (2012b). Introduction of β-carotene-rich orange sweet potato in rural Uganda resulted in increased vitamin A intakes among children and women and improved vitamin A status among children. J. Nutr. 142, 142, 1871–1880. doi: 10.3945/jn.111.151829
Hou, L. X., Xiao, L. Z., Kang, Z. H., Yang, X. P., Gu, J. H., Tang, B. J., et al. (1999). Identification on drought resistance of sweetpotato varieties. J. Henan Agric. Sci. 2, 2–5.
Ichinose, K., Reddy, G. V. P., Shrestha, G., Sharma, A., Okada, Y., Yoshida, M., et al. (2019). Interplanting different varieties of a sweet potato crop to reduce damage by oligophagous insect pests. Ann. Entomol. Soc. Am. 112, 365–371. doi: 10.1093/aesa/say058
Indira, P., and Kabeerathumma, S. (1988). Physiological response of sweetpotato under water stress. 1: effect of water stress during the different phases of tuberization. J. Root Crops 14, 37–40.
Intergovernmental Panel on Climate Change (IPCC) (2012). Managing the Risks of Extreme Events and Disasters to Advance Climate Change Adaptation. A Special Report of Working Groups I and II of the Intergovernmental Panel on Climate Change, eds C. B. Field, V. Barros, T. F. Stocker, D. Qin, D. J. Dokken, K. L. Ebi, M. D. Mastrandrea, K. J. Mach, G. K. Plattner, S. K. Allen, M. Tignor, and P. M. Midgley (Cambridge; New York, NY: Cambridge University Press), 582.
Jenkins, M., Byker Shanks, C., and Houghtaling, B. (2015). Orange-fleshed sweet potato: successes and remaining challenges of the introduction of a nutritionally superior staple crop in Mozambique. Food Nutr. Bull. 36, 36, 327–353. doi: 10.1177/0379572115597397
Jia, R. (2013). Weather shocks, sweet potatoes and peasant revolts in historical China. Econ. J. 124, 92–118. doi: 10.1111/ecoj.12037
Kapinga, R. E., Ewell, P. T., Jeremiah, S. C., and Kileo, R. (1995). Sweetpotato in Tanzanian Farming and Food Systems: Implications for Research. Nairobi: Tanzania national root and tuber crops and farming systems research program and the international potato center (CIP).
Karuniawan, A. (2004). Cultivation status and genetic diversity of yam bean (Pachyrhizus erosus (L) Urban) in Indonesia (Dissertation). University of Göttingen, Cuvillier Verlaag Göttingen, Germany.
Khan, M. A., Gemenet, D. C., and Villordon, A. (2016). Root system architecture and abiotic stress tolerance: current knowledge in root and tuber crops. Front. Plant Sci. 7:1584. doi: 10.3389/fpls.2016.01584
Kiiza, B., Kisembo, L. G., and Mwanga, R. O. M. (2012). Participatory plant breeding and selection impact on adoption of improved sweetpotato varieties in Uganda. J. Agric. Sci. Technol. A 2, 673–681. Available online at: https://hdl.handle.net/10568/66545
King, G. A. (1985). The effect of time of planting on yield of six varieties of sweet potato (Ipomoea batatas (L.) Lam.) in the southern coastal lowlands of Papua New Guinea. Trop. Agric. 62, 225–228.
Kinyua, J. M. (2013). Assessment of Sweet Potato Cultivars as Protein and Energy Feed Supplements in the Kenyan Highlands (Master's thesis). Egerton University.
Kiragu, A. K. (2015). Pig Production Systems in Central Kenya and the Effectof Feeding Sweet Potato Silage on Performance of Grower Pigs (Master's Thesis). University of Nairobi, Nairobi.
Kirchhof, G. (2009). Soil Fertility in Sweetpotato-Based Cropping Systems in the Highlands of Papua New Guinea. ACIAR Technical Reports Canberra: Australian Centre for International Agricultural Research.
Kivuva, B. (2013). Breeding Sweetpotato (Ipomoea batatas [L.] Lam.) for Drought Tolerance in Kenya (Dissertation). University of KwaZulu-Natal, Pietermaritzburg.
Kivuva, B., Githiri, S., Yencho, C., and Sibiya, J. (2015). Combining ability and heterosis for yield and drought tolerance traits under managed drought stress in sweetpotato. Euphytica 201, 423–440. doi: 10.1007/s10681-014-1230-1
Kivuva, B. E., Githiri, S. M., Yencho, G. C., and Sibiya, J. (2014). Genotype X environment interaction for storage root yield in sweetpotato under managed drought stress conditions. J. Agric. Sci. 6:1–16. doi: 10.5539/jas.v6n10pxx
Kyalo, G., and Lamo, J. (2017). Rice-Sweetpotato Rotation System: Improving Rice Yields and Profitability by Utilizing Irrigated Rice Schemes for Off-Season Production of Sweetpotato Vines and Roots. Nairobi: CIP.
Larbi, A., Etela, I., Nwokocha, H. N., Oji, U. I., Anyanwu, N. J., Gbaraneh, L. D., et al. (2007). Fodder and tuber yields, and fodder quality of sweet potato cultivars at different maturity stages in the West African humid forest and savanna zones. Anim. Feed Sci. Technol. 135, 126–138. doi: 10.1016/j.anifeedsci.2006.05.021
Laurie, R. N., Du Plooy, C. P., and Laurie, S. M. (2009). “Effect of moisture stress on growth and performance of orange fleshed sweetpotato varieties,” in 9th African Crop Science Society Conference Proceedings (Capetown: African Crop Science Society), 235–239.
Laurie, R. N., Laurie, S. M., du Plooy, C. P., Finnie, J. F., and Van Staden, J. (2015). Yield of drought-stressed sweet potato in relation to canopy cover, stem length and stomatal conductance. J. Agric. Sci. 7, 201–214. doi: 10.5539/jas.v7n1p201
Laurie, S., Booyse, M., Labuschagne, M., and Greyling, M. (2015). Multienvironment performance of new orange-fleshed sweetpotato cultivars in South Africa. Crop Sci. 55, 1585–1595. doi: 10.2135/cropsci2014.09.0664
Laurie, S., Calitz, F., Mtileni, M., Mphela, W., and Tjale, S. (2017). Performance of informal market sweet potato cultivars in on-farm trials in South Africa. Open Agric. 2, 431–441. doi: 10.1515/opag-2017-0047
Laurie, S. M., Calitz, F. J., Adebola, P. O., and Lezar, A. (2013). Characterization and evaluation of South African sweet potato (Ipomoea batatas (L.) LAM) land races. S. Afr. J. Bot. 85, 10–16. https://doi.org/10.1016/j.sajb.2012.11.004
Laurie, S. M., Faber, M., and Claasen, N. (2018). Incorporating orange-fleshed sweet potato into the food system as a strategy for improved nutrition: the context of South Africa. Food Res. Int. 104, 77–85. doi: 10.1016/j.foodres.2017.09.016
Laurie, S. M., Faber, M., van Jaarsveld, P. J., Laurie, R. N., du Plooy, C. P., and Modisane, P. C. (2012). β-carotene yield and productivity of orange-fleshed sweet potato (Ipomoea batatas L. Lam.) as influenced by irrigation and fertilizer application treatments. Sci. Hortic. 142, 180–184. doi: 10.1016/j.scienta.2012.05.017
Lebot, V. (2010). “Sweetpotato: hand book of plant breeding,” in Root and Tuber Crops, ed J. E. Bradshaw (Basel: Springer Nature), 97–125.
León-Velarde, C., and De Mendiburu, F. (2007). “Variedades de batata de doble propósito,” in Producción y Uso de la Batata (Ipomoea batatas (L.) Lam.): Estrategias de Alimentación Animal, eds C. León-Velarde and R. Amable (Santiago de los Caballeros, Dominican Republic: Universidad ISA), 36–44.
Liebman, M., and Dyck, E. (1993). Crop rotation and intercropping strategies for weed management. Ecol. App. 3, 92–122.
Low, J. (2013). “Biofortified crops with an invisible trait: the example of orange-fleshed sweetpotato in sub-Saharan Africa,” in Handbook of Food Fortification and Health: From Concepts to Public Health Applications, eds V. Preedy, R. Srirajaskanthan and V. Patel (New York, NY: Springer Science Business Media), 371–384.
Low, J., Ball, A., van Jaarsveld, P. J., Namutebi, A., Faber, M., and Grant, F. K. (2015). “Assessing nutritional value and changing behaviours regarding orange-fleshed sweetpotato use in Sub-Saharan Africa,” in Potato and Sweetpotato in Africa: Transforming the Value Chains for Food and Nutrition Security, ed. J. Low (Oxfordshire: CAB International), 551–579.
Low, J., Ball, L. A., Magezi, S., Njoku, J., Mwanga, R., Andrade, M., et al. (2017). Sweet potato development and delivery in sub-Saharan Africa. Afr. J. Food Agric. Nutr. Dev. 17, 11955–11972. doi: 10.18697/ajfand.78.HarvestPlus07
Low, J., Lynam, J., Lemaga, B., Crissman, C., Barker, I., Thiele, G., et al. (2009). “Sweetpotato in Sub-Saharan Africa,” in The Sweetpotato, eds G. Loebenstein and G. Thottappilly (Dordrecht: Springer Science+Business Media B.V), 359–390.
Low, J. W., Arimond, M., Osman, N., Cunguara, B., Zano, F., and Tschirley, D. (2007). A food-based approach introducing orange-fleshed sweet potatoes increased vitamin A intake and serum retinol concentrations in young children in rural Mozambique. J. Nutr. 137, 1320–1327. doi: 10.1093/jn/137.5.1320
Low, J. W., Mwanga, R. O. M., Andrade, M. I., Carey, E., and Ball, A.-M. (2017). Tackling vitamin A deficiency with biofortified sweetpotato in sub-Saharan Africa. Glob. Food Secur. 14, 23–30. doi: 10.1016/j.gfs.2017.01.004
Lukuyu, B. A., Kinyua, J., Agili, S., Gachuiri, C. K., and Low, J. (2014). “Evaluation of sweetpotato varieties for the potential of dual-purpose in different agroecological zones of kenya,” in Challenges and Opportunities for Agricultural Intensification of the Humid Highland Systems of Sub-Saharan Africa, ed B. E. A. Vanlauwe (Cham: Springer International Publishing), 217–231.
Manners, R., and van Etten, J. (2018). Are agricultural researchers working on the right crops to enable food and nutrition security under future climates? Glob. Environ. Change 53, 182–194. doi: 10.1016/j.gloenvcha.2018.09.010
Markos, D. (2016). Sweet potato agronomy research in ethiopia: summary of past findings and future research directions. Agric. Food Sci. Res. 3, 1–11. doi: 10.20448/journal.512/2016.3.1/512.1.1.11
Masango, S. (2015). Water Use Efficiency of Orange Fleshed Sweet Potato (Ipomoea Batatas L. Lam.) (Master's Thesis). University of Pretoria, Pretoria.
Masuka, B., Araus, J. L., Das, B., Sonder, K., and Cairns, J. E. (2012). Phenotyping for abiotic stress tolerance in maize. J. Integr. Plant Biol. 54, 238–249. doi: 10.1111/j.1744-7909.2012.01118.x
McEwan, M. (2016). Sweetpotato Seed Systems in SUB-Saharan Africa: a Literature Review to Contribute to the Preparation of Conceptual Frameworks to Guide Practical Interventions for Root, Tuber and Banana Seed Systems. RTB Working Paper No. 2016-4(International Potato Center), 45. doi: 10.4160/23096586RTBWP20164
Ministério da Agricultura e Segurança Alimentar (2015). Annuário de Estatísticas Agrárias 2015. Maputo, Mozambique.
Mmasa, J. J., and Msuya, E. E. (2012). Mapping of the sweet potato value Chain linkages between actors, processes and activities in the value Chain: a case of “Michembe” and “Matobolwa” products. Sustain. Agric. Re. 1, 130–146. doi: 10.5539/sar.v1n1p130
Motsa, N. M., Modi, A. T., and Mabhaudhi, T. (2015). Sweet potato (Ipomoea batatas L.) as a drought tolerant and food security crop. S. Afr. J. Sci. Vol. 111, 1–8. doi: 10.17159/sajs.2015/20140252
Mudege, N. N., Biazin, B. T., and Brouwer, A. R. (2019). Gender situational analysis of the sweetpotato value chain in selected districts in Sidama and Gedeo Zones, Southern Ethiopia. Social Science Working Paper No. 2019–1. doi: 10.4160/02568748CIPWP20191
Mudege, N. N., Mdege, N., Abidin, P. E., and Bhatasara, S. (2017). The role of gender norms in access to agricultural training in Chikwawa and Phalombe, Malawi. Gender Place Culture 24, 1689–1710. doi: 10.1080/0966369x.2017.1383363
Mukherjee, A., Ray, R., Pati, K., and Mukherjee, A. (2015). Sweet potato and taro resilient to stresses: sustainable livelihood in fragile zones vulnerable to climate changes. J. Environ. Soc. 12, 53–64. Available online at: http://www.informaticsjournals.com/index.php/jes/article/view/2705
Mukhopadhyay, S. K., Chattopadhyay, A., Chakraborty, I., and Bhattacharya, I. (2011). Crops that feed the world 5. Sweetpotato. Sweetpotatoes for income and food security. Food Secur. 3, 3, 283–305. doi: 10.1007/s12571-011-0134-3
Murugan, S., Paramasivam, S. K., and Nedunchezhiyan, M. (2012). Sweet potato as animal feed and fodder. Fruit Veg. Cereal Sci. Biotechnol. 6, 106–114. Available online at: http://www.globalsciencebooks.info/Online/GSBOnline/images/2012/FVCSB_6(SI1)/FVCSB_6(SI1)106-114o.pdf
Mwanga, R., Carey, E., Andrade, M., and Grüneberg, W. (2019). The sweetpotato breeding community of practice: breeding in Africa for Africa (2009-2019). SASHA Brief. No. 1(International Potato Center), 4. Available online at: https://hdl.handle.net/10568/105972.
Mwanga, R. O. M., Andrade, M. I., Carey, E. E., Low, J. W., Yencho, G. C., and Grüneberg, W. J. (2017). “Sweetpotato (Ipomoea batatas L.),” in Genetic Improvement of Tropical Crops, eds H. Campos and P. D. S. Caligari (Cham: Springer), 181–218.
Mwije, A. (2014). Heritability analysis of putative drought adaptation traits in sweet potato. Afr. Crop Sci. J. 22, 79–87. Available online at: https://www.ajol.info/index.php/acsj/article/view/101374
Myers, S. S., Zanobetti, A., Kloog, I., Huybers, P., Leakey, A. D. B., Bloom, A. J., et al. (2014). Increasing CO2 threatens human nutrition. Nature 510, 139–42. doi: 10.1038/nature13179
Nair, G. M., Nair, V. M., and Sreedharan, C. (1996). Response of sweet potato to phasic stress irrigation in summer rice fallows. J. Root Crops 22, 45–49.
Nedunchezhiyan, M., Jata, S. K., and Byju, G. (2012). Sweet potato-based cropping systems. Fruit Veg. Cereal Sci. Biotechnol. 6, 11–16. Available online at: http://www.globalsciencebooks.info/Online/GSBOnline/images/2012/FVCSB_6(SI1)/FVCSB_6(SI1)11-16o.pdf
Ngailo, S., Shimelis, H. A., Sibiya, J., and Mtunda, K. (2015). Assessment of sweetpotato farming systems, production constraints and breeding priorities in eastern Tanzania. S. Afr. J. Plant Soil 33, 105–112. doi: 10.1080/02571862.2015.1079933
Niang, I., Ruppel, O. C., Abdrabo, M. A., Essel, A., Lennard, C., Padgham, J., et al. (2014). “Africa,” in Climate Change 2014: Impacts, Adaptation, and Vulnerability. Part B: Regional Aspects. Contribution of Working Group II to the Fifth Assessment Report of the Intergovernmental Panel on Climate Change, eds V. R. Barros, C. B. Field, D. J. Dokken, M. D. Mastrandrea, K. J. Mach, T. E. Bilir, M. Chatterjee, K. L. Ebi, Y. O. Estrada, R. Genova, B. Girma, E. S. Kissel, A. N. Levy, S. MacCracken, P. R. Mastrandrea, and L. L. White (Cambridge; New York, NY, USA: Cambridge University Press), 1199–1265.
Niederwieser, J. G. (2004). Guide to Sweet Potato Production in South Africa. Pretoria (South Africa) ARC-Roodeplant Vegetable and Ornamental Plant Institute.
Niyireeba, R. T., Ebong, C., Agili, S., Low, J. W., Lukuyu, B. A., Kirui, J., et al. (2013). Evaluation of dual purpose sweet potato (Ipomea batatas (L.) Lam) cultivars for root and fodder production in Eastern Province, Rwanda. Agric. J. 8, 242–247. doi: 10.3923/aj.2013.242.247
Ntsieni, M. (2017). Quantifying Water Use and Nutritional Water Productivity of Two Sweet Potato (Ipomoea Batatas) Cultivars Grown in South Africa (Master's Thesis). University of Pretoria, Pretoria.
Nyathi, M. K., Du Plooy, C. P., Van Halsema, G. E., Stomph, T. J., Annandale, J. G., and Struik, P. C. (2019). The dual-purpose use of orange-fleshed sweet potato (Ipomoea batatas var. Bophelo) for improved nutritional food security. Agric. Water Manag. 217, 23–37. doi: 10.1016/j.agwat.2019.02.029
Okello, J., Wanjohi, L., Makohka, P., Low, J.W., and Kwikiriza, N. (2019). “Status of Sweetpotato in Sub-Saharan Africa: September 2019,” in Sweetpotato for Profit and Health Initiative (Nairobi: International Potato Center).
Okonya, J. S., Mujica, N., and Carhuapoma, P. (2016). “Sweetpotato weevil, cylas puncticollis (Boheman 1883),” in Pest Distribution and Risk Atlas for Africa. Potential Global and Regional Distribution and Abundance of Agricultural and Horticultural Pests and Associated Biocontrol Agents Under Current and Future Climates, eds J. Kroschel, N. Mujica, P. Carhuapoma and M. Sporleder (Lima: International Potato Center (CIP)), 54–63.
Okonya, J. S., Petsakos, A., Suarez, V., Nduwayezu, A., Kantungeko, D., Blomme, G., et al. (2019). Pesticide use practices in root, tuber, and banana crops by smallholder farmers in Rwanda and Burundi. Int. J. Environ. Res. Public Health 16:400. doi: 10.3390/ijerph16030400
Omiat, E. G., Kapinga, R. E., Tumwegamire, S., Odong, T. L., and Adipala, E. (2005). “On-farm evaluation of orange-fleshed sweetpotato varieties in northeastern Uganda,” in 7th African Crop Science Conference Proceedings (Entebbe: African Crop Science Society), 603–609.
Omotobora, B., Adebola, P., Modise, D., Laurie, S., and Gerrano, A. (2014). Greenhouse and field evaluation of selected sweetpotato (Ipomoea batatas (L.) LAM) accessions for drought tolerance in South Africa. Am. J. Plant Sci. 21, 3328–3339. doi: 10.4236/ajps.2014.521348
Onyekwere, P. S. N., and Nweinyi, S. C. O. (1989). Water Requirements of Sweetpotato (Ipomoea batatas). Annual Report: National Root Crops Research Institute, Umudike.
Osiru, M., Adipala, E., Olanya, O. M., Lemaga, B., and Kapinga, R. (2007). Occurence and distribution of alternaria leaf petiole and stem blight on sweetpotato in Uganda. Plant Pathol. J. 6, 112–119. doi: 10.3923/ppj.2007.112.119
Oswald, A., Kapinga, R., Lemaga, B., Ortiz, O., Kroschel, J., and Lynam, J. (2009). “Integrated crop management,” in Unleashing the Potential of Sweetpotato in Sub-Saharan Africa: Current Challenges and Way Forward, ed C. Barker (Lima: International Potato Center (CIP), 130–153.
Padmaja, G. (2009). “Uses and nutritional data of sweet potato,” in The Sweetpotato, eds G. Loebenstein and G. Thottapilly (Dordrecht: Springer Science+Business Media B.V), 189–234.
Parmar, A., Hensel, O., and Sturm, B. (2017). Post-harvest handling practices and associated food losses and limitations in the sweetpotato value chain of southern Ethiopia. NJAS Wageningen J. Life Sci. 80, 65–74. doi: 10.1016/j.njas.2016.12.002
Pesek, J., and Baker, R. J. (1969). Desired improvement in relation to selection indices. Can. J. Plant Sci. 49, 803–804.
Rajendran, S., Kimenye, L. N., and McEwan, M. (2017). Strategies for the development of the sweetpotato early generation seed sector in eastern and southern Africa. Open Agric. 2, 236–243. doi: 10.1515/opag-2017-0025
Rankine, D. R., Cohen, J. E., Taylor, M. A., Coy, A. D., Simpson, L. A., Stephenson, T., et al. (2015). Parameterizing the FAO aquacrop model for rainfed and irrigated field-grown sweet potato. Agron. J. 107, 375–387. doi: 10.2134/agronj14.0287
Ravi, V., and Indira, P. (1996). Investigation on the Physiological Factors Limiting Yield Potential in Sweetpotato Under Drought Stress. Annual Report Trivandrum, India (Central Tuber Crops Research Institute).
Reynolds, T. W., Waddington, S. R., Anderson, C. L., Chew, A., True, Z., and Cullen, A. (2015). Environmental impacts and constraints associated with the production of major food crops in Sub-Saharan Africa and South Asia. Food Secur. 7, 795–822. doi: 10.1007/s12571-015-0478-1
Römer, C., Wahabzada, M., Ballvora, A., Pinto, F., Rossini, M., Panigada, C., et al. (2012). Early drought stress detection in cereals: simplex volume maximisation for hyperspectral image analysis. Func. Plant Biol. 39, 878–890. doi: 10.1071/FP12060
Roorkiwal, M., Nayak, S. N., Thudi, M., Upadhyaya, H. D., Brunel, D., Mournet, P., et al. (2014). Allele diversity for abiotic stress responsive candidate genes in chickpea reference set using gene based SNP markers. Front. Plant Sci. 5:248. doi: 10.3389/fpls.2014.00248
Rukundo, P. (2015). Breeding of Sweetpotato (Ipomoea batatas (L.) Lam.) for Drought Tolerance and High Dry Matter Content in Rwanda (Dissertation). University of KwaZulu-Natal, Pietermaritzburg.
Smit, N. E. J. M. (1997). The effect of the indigenous cultural practices of in-ground storage and piecemeal harvesting of sweetpotato on yield and quality losses caused by sweetpotato weevil in Uganda. Agric. Ecosyst. Environ. 64, 191–200.
Solis, J., Villordon, A., Baisakh, N., LaBonte, D., and Firon, N. (2014). Effect of drought on storage root development and gene expression profile of sweetpotato under greenhouse and field conditions. J. Am. Soc. Hortic. Sci. 139, 317–324. doi: 10.21273/JASHS.139.3.317
Ssali, R. T., Sseruwu, G., Yada, B., Ssemakula, G., Wasonga, C., Grüneberg, W. J., et al. (2019). Efficiency of the polycross and controlled hybridization methods in sweetpotato breeding in Uganda. J. Agric. Sci. 11, 123–134. doi: 10.5539/jas.v11n17p123
Ssebuliba, J. M., Muyonga, J. H., and Ekere, W. (2006). Performance and acceptability of orange fleshed sweetpotato cultivars in Eastern Uganda. Afr. Crop Sci. J. 14, 231–240. Available online at: https://www.ajol.info/index.php/acsj/article/view/135856
Stathers, T., Carey, E., Mwanga, R., Njoku, J., Malinga, J., Njoku, A., et al. (2013). “Manual. 4: Sweetpotato production and management; Sweetpotato pest and disease management,” in Everything You Ever Wanted to Know about Sweetpotato: Reaching Agents of Change ToT, eds. T. Stathers, J. Low, R. Mwanga, T. Carey, S. David, R. Gibson, S. Namanda, M. McEwan, A. Bechoff, J. Malinga, M. Benjamin, H. Katcher, J. Blakenship, M. Andrade, S. Agili, J. Njoku, K. Sindi, G. Mulongo, S. Tumwegamire, A. Njoku, E. Abidin and A. Mbabu. (Nairobi: International Potato Center), 144–188.
Tairo, F., Kullaya, A., and Valkonen, J. P. T. (2004). Incidence of viruses infecting sweetpotato in Tanzania. Plant Dis. 88, 88, 916–920. doi: 10.1094/PDIS.2004.88.9.916
Talekar, N. S. (1987). Influence of cultural pest management techniques on the infestation of sweetpotato weevil. Insect Sci. App. 8, 809–814.
Tanaka, M., Ishiguro, K., Oki, T., and Okuno, S. (2017). Functional components in sweetpotato and their genetic improvement. Breed. Sci. 67:16125. doi: 10.1270/jsbbs.16125
Tardieu, F., Simonneau, T., and Muller, B. (2018). The physiological basis of drought tolerance in crop plants: a scenario-dependent probabilistic approach. Ann. Rev. Plant Biol. 69, 733–759. doi: 10.1146/annurev-arplant-042817-040218
Thiele, G., Khan, A., Heider, B., Kroschel, J., Harahagazwe, D., Andrade, M., et al. (2017). Roots, tubers and bananas: planning and research for climate resilience. Open Agric. 2, 2, 350–361. doi: 10.1515/opag-2017-0039
Thompson, A., Narayanin, C. D., Smith, M. F., and Slabbert, M. M. (2011). A disease survey of fusarium wilt and alternaria blight on sweet potato in South Africa. Crop Protect. 30, 1409–1413. doi: 10.1016/j.cropro.2011.07.017
Thornton, P. K., Jones, P. G., Ericksen, P. J., and Challinor, A. J. (2011). Agriculture and food systems in sub-Saharan Africa in a 4°C+ world. Philos. Trans. R. Soc. A Mathemat. Phys. Eng. Sci. 369, 117–136. doi: 10.1098/rsta.2010.0246
Tomlins, K., Ndunguru, G., Kimenya, F., Ngendello, T., Rwiza, E., Amour, R., et al. (2007). On-farm evaluation of methods for storing fresh sweet potato roots in East Africa. Trop. Sci. 47, 197–210. doi: 10.1002/ts.214
Tricker, P. J., ElHabti, A., Schmidt, J., and Fleury, D. (2018). The physiological and genetic basis of combined drought and heat tolerance in wheat. J. Exp. Bot. 69, 3195–3210. doi: 10.1093/jxb/ery081
Tuberosa, R. (2012). Phenotyping for drought tolerance of crops in the genomics era. Front. Physiol. 3:347. doi: 10.3389/fphys.2012.00347
Uwah, D. F., Undie, U. L., John, N. M., and Ukoha, G. O. (2013). Growth and yield response of improved sweet potato (Ipomoea batatas (L.) Lam) varieties to different rates of potassium fertilizer in Calabar, Nigeria. J. Agric. Sci. 5, 61–69. doi: 10.5539/jas.v5n7p61
Valkonen, J. P. T., Kreuze, J. F., and Ndunguru, J. (2015). “Disease management, especially viruses in potato and sweetpotato,” in Potato and Sweetpotato in Africa: Transforming the Value Chains for Food and Nutrition Security, eds J. Low, M. Nyongesa, S. Quinn, and M. Parker (Oxfordshire: CABI), 339–349.
Van Heerden, P. D. R., and Laurie, R. (2008). Effects of prolonged restriction in water supply on photosynthesis, shoot development and storage root yield in sweet potato. Physiol. Plantarum 134, 99–109. doi: 10.1111/j.1399-3054.2008.01111.x
van Oort, P. A. J., Saito, K., Dieng, I., Grassini, P., Cassman, K. G., and van Ittersum, M. K. (2017). Can yield gap analysis be used to inform R&D prioritisation? Glob. Food Secur. 12, 12, 109–118. doi: 10.1016/j.gfs.2016.09.005
van Vugt, D. (2018). Participatory Approaches to Diversification and Intensification of Crop Production on Smallholder Farms in Malawi. dissertation, Wageningen University.
van Vugt, D., and Franke, A. C. (2018). Exploring the yield gap of orange-fleshed sweet potato varieties on smallholder farmers' fields in Malawi. Field Crops Res. 221, 245–256. doi: 10.1016/j.fcr.2017.11.028
Villordon, A., LaBonte, J., Solis, J., and Firon, N. (2012). Characterization of lateral root development at the onset of storage root initiation in ‘Beauregard' sweetpotato adventitious roots. HortScience 47, 961–968. doi: 10.21273/HORTSCI.47.7.961
Villordon, A. Q., Ginzberg, I., and Firon, N. (2014). Root architecture and root and tuber crop productivity. Trends Plant Sci. 19, 419–425. doi: 10.1016/j.tplants.2014.02.002
Wang, H., Wu, Y., Zhang, Y., Yang, J., Fan, W., Zhang, H., et al. (2019). CRISPR/Cas9-based mutagenesis of starch biosynthetic genes in sweet potato (Ipomoea Batatas) for the improvement of starch quality. Int. J. Mol. Sci. 20:4702. doi: 10.3390/ijms20194702
Wang, Y. P., Liu, Q. C., Li, A. X., Zhai, H., Zhang, S. S., and Liu, B. L. (2003). In vitro selection and identification of drought-tolerant mutants in sweetpotato. Agric. Sci. China 2, 1314–1320.
Weaver, J. E., and Bruner, W. E. (1927). Root Development of Vegetable Crops. New York, NY: McGraw-Hill Book Company.
Weerarathne, L. V. Y., Marambe, B., and Chauhan, B. S. (2017). Intercropping as an effective component of integrated weed management in tropical root and tuber crops: a review. Crop Protect. 95, 89–100. doi: 10.1016/j.cropro.2016.08.010
Woolfe, J. A. (1992). Sweet Potato: An Untapped Food Resource. Cambridge; New York, NY: Cambridge University Press.
Wricke, G., and Weber, W. E. (1986). Quantitative Genetics and Selection in Plant Breeding. New York, NY: Walter de Gruyter.
Xie, S. Q., Feng, Y. W., and Xi, L. G. (1998). On the drought resistance of local sweetpotato germplasm resources from Yunnan. Crop Genet. Resour. 1, 31–32.
Yanfu, Y., Jialan, T., Yunchu, Z., and Ruilian, Q. (1989). “Breeding for early-maturing sweetpotato varieties,” in Sweetpotato Research and Development for Small Farmers, eds K. T. Mackay, M. K. Palomer, and R. T. Sanieo (Laguna: SEAMEO-SEARCA), 67–82.
Yanggen, D., and Nagujja, S. (2006). “The use of orange-fleshed sweetpotato to combat Vitamin A deficiency in Uganda: a study of varietal preferences, extension strategies and post-harvest utilization,” in Social Science Working Paper No. 2006-2. (Lima: International Potato Center (CIP)), 80 p.
Yen, C. T., Chu, C. V., and Sheng, C. L. (1964). Studies on the drought resistance of sweetpotato varieties. Crop Sci. 3, 183–190.
Yengoh, G. T., and Ardo, J. (2014). Crop yield gaps in cameroon. Ambio 43, 175–190. doi: 10.1007/s13280-013-0428-0
Yooyongwech, S., Samphumphuang, T., Tisarum, R., Theerawitaya, C., and Cha-um, S. (2016). Arbuscular mycorrhizal fungi (AMF) improved water deficit tolerance in two different sweet potato genotypes involves osmotic adjustments via soluble sugar and free proline. Sci. Hortic. 198, 107–117. doi: 10.1016/j.scienta.2015.11.002
Yooyongwech, S., Theerawitaya, C., Samphumphuang, T., and Cha-um, S. (2013). Water-deficit tolerant identification in sweet potato genotypes (Ipomoea batatas (L.) Lam.) in vegetative developmental stage using multivariate physiological indices. Sci. Hortic. 162, 242–251. doi: 10.1016/j.scienta.2013.07.041
Yoshida, T., Hozyo, Y., and Murata, T. (1970). Studies on the development of tuberous roots in sweet potato (Ipomea batatas, Lam. var edulis, Mak.) : the effect of deep placement of mineral nutrients on the tuber-yield of sweet potato. Jap. J. Crop Sci. 39, 105–110. doi: 10.1626/jcs.39.105
You, L., Wood-Sichra, U., Fritz, S., Guo, Z., See, L., and Koo, J. (2014). Spatial Production Allocation Model (SPAM) 2005 v2.0 Database. Available online at: http://mapspam.info/global-data/#sort/harvested_area/total.
Zhang, D. P., Collins, W. W., and Belding, S. (1993). Improving sweetpotato starch digestibility for animal feeding. HortScience 28, 325–326.
Zhang, J., Jia, W., Yang, J., and Ismail, A. M. (2005). Role of ABA in integrating plant responses to drought and salt stresses. Field Crops Res. 97, 111–119. doi: 10.1016/j.fcr.2005.08.018
Keywords: orange-fleshed sweetpotato, breeding, drought tolerance, heterosis, intensification, cropping system
Citation: Low JW, Ortiz R, Vandamme E, Andrade M, Biazin B and Grüneberg WJ (2020) Nutrient-Dense Orange-Fleshed Sweetpotato: Advances in Drought-Tolerance Breeding and Understanding of Management Practices for Sustainable Next-Generation Cropping Systems in Sub-Saharan Africa. Front. Sustain. Food Syst. 4:50. doi: 10.3389/fsufs.2020.00050
Received: 31 December 2019; Accepted: 07 April 2020;
Published: 12 May 2020.
Edited by:
Urs Feller, University of Bern, SwitzerlandReviewed by:
Leonard Rusinamhodzi, International Institute of Tropical Agriculture, NigeriaYanfei Mao, Shanghai Institutes for Biological Sciences (CAS), China
Sunette M. Laurie, Agricultural Research Council of South Africa (ARC-SA), South Africa
Copyright © 2020 Low, Ortiz, Vandamme, Andrade, Biazin and Grüneberg. This is an open-access article distributed under the terms of the Creative Commons Attribution License (CC BY). The use, distribution or reproduction in other forums is permitted, provided the original author(s) and the copyright owner(s) are credited and that the original publication in this journal is cited, in accordance with accepted academic practice. No use, distribution or reproduction is permitted which does not comply with these terms.
*Correspondence: Jan W. Low, ai5sb3dAY2dpYXIub3Jn