- Research Group on Quality and Safety of Plant Foods, CEBAS-CSIC, Murcia, Spain
Antimicrobial treatment of fresh produce wash water can enable water reuse by avoiding microbial cross-contamination. In this study, the efficacy of chlorine dioxide (ClO2; 2–3 mg/L) to maintain the microbiological quality of process wash water in a tomato packinghouse was assessed. Different parameters were measured, including the physicochemical characteristics of the wash water [pH, oxidation-reduction potential (ORP), chemical oxygen demand (COD), absorbance at 254 nm (UVA254), temperature, electrical conductivity (EC)], the levels of different microorganisms present in water and in the product [aerobic mesophilic bacteria (AMB), total coliforms, yeasts and molds, E. coli], and the presence of chlorate as disinfection by-product. The treatment with ClO2 had a significant effect on the microbial populations present in the recirculated wash water and the tomatoes compared with the use of untreated recirculated water. The treated water showed levels of AMB, total coliforms and E. coli more than 1 logarithmic unit lower than the untreated water. The microbial load in tomatoes washed with tap water, and ClO2-treated recirculated water was similar. The transfer of chlorate from wash water to the fruits was very low under the conditions tested, with levels of chlorate in the washed product always lower than 0.05 mg/kg. The results obtained indicate that ClO2 could be a suitable option for the reuse of wash water in commercial packinghouses, as it reduces the microbial populations in wash water while not leading to the presence of disinfection by-products, such as chlorate, in the final product. This study shows that the monitorization and control of the microbiological quality of process wash water should be managed together with good practices able to guarantee hygienic conditions of the equipment by implementing efficient cleaning and disinfection systems.
Introduction
One of the primary needs of the fresh produce industry is to reduce water and energy consumption, as well as wastewater disposal (Manzocco et al., 2015). Some processors use drinking-quality water with or without sanitizers for washing produce by overhead spray and the effluent from this wash process is disposed without further use (“single-pass washing;” Pao et al., 2009). However, due to economic and environmental reasons, this is not a sustainable practice, and most produce processors recirculate and reuse process wash water (Casani et al., 2005; Manzocco et al., 2015). However, wash water reuse involves potential microbiological risks associated with the accumulation of microorganisms from the surface of the washed products in the water. This can cause cross-contamination with harmful microorganisms between lots of product through the wash water (Gombas et al., 2017). The existing literature indicates that the disinfection of the wash water is a necessary intervention strategy to reduce microbiological risk in the washing stage (Danyluk and Schaffner, 2011; Gombas et al., 2017; Maffei et al., 2017). The use of disinfectants in process wash water is also useful to reduce contamination of the equipment. Wang and Ryser (2014) showed that the use of disinfectant reduced microbial cross-contamination coming from the roller brushes used during the washing of tomatoes.
Different disinfectants are used by the fresh produce companies for the treatment of wash water, being chlorine the most widely applied (Fu et al., 2018). However, chlorine drawbacks [mainly the occurrence of harmful disinfection by-products (DBPs)], stimulate the use of alternative treatments (Meireles et al., 2016). One of the potential substitute treatments is chlorine dioxide (ClO2) that has some advantages compared with chlorine, including higher stability in the presence of organic matter (van Haute et al., 2017) and the lack of formation of organohalogen DBPs (Gómez-López et al., 2009). However, process water treatment with ClO2 leads to the presence of DBPs, such as chlorate and chlorite (Gil et al., 2016; van Haute et al., 2017). Aqueous ClO2 is used by processors in the USA for the washing of tomatoes (Tomás-Callejas et al., 2012; De et al., 2018).
This study aimed to determine the potential for reuse of ClO2-treated wash water in a tomato packinghouse that used a single-pass overhead spray washing with potable water. The ultimate goal was to reduce drinking water consumption for the industrial washing of tomatoes. The effect of ClO2 treatment on the microbial populations and the chlorate present in the wash water and the product was assessed.
Materials and Methods
Washing Line
The washing line used for the tests was located in the manufacturing center of a large Spanish company devoted to the production and distribution of tomato. In this commercial packinghouse, the tomato washing process was carried out at room temperature, and there was no pre-cooling of the tomato or wash water. The washing process performed by the company consisted of overhead spraying with tap water combined with brushing (roller brushes) to remove the surface dirt from tomatoes. The washing machine that was used for the test (Figure 1) had three overhead spray bar lines with a total of thirteen nozzles. The tomatoes moved on rollers with brushes and rollers with sponge for brushing and dewatering the tomatoes, respectively. The water used to wash the product was collected in plates located under the brushes. In the regular procedure of the company, the water was disposed without re-use. To adapt the washing machine to the alternative treatments that were carried out, a recirculation tank with a capacity of 400 L and a pump were coupled to the system. At the end of the washing machine, the water present on the surface of tomatoes was removed by forced air. The dwell time of the tomatoes in the washing machine, from the entry in the washing area to the exit of the dewatering area, was about 2 min.
Experimental Design
For the trial, 156 boxes of tomato (≈12 kg each) were used. In order to simulate a worst-case scenario, the water used for the water recirculation treatments was water obtained from the box washer of the packinghouse. This type of water was used to mimic the physicochemical and microbiological quality of recirculated process water at the end of a workday. At each sampling time, water samples (500 mL) were taken from the effluent of the washing machine for microbiological and physicochemical analyses. The samples taken for microbiological analyses were treated with sodium thiosulphate (Scharlau, Barcelona, Spain) to quench ClO2 residuals. The tomato samples (three and five tomatoes per sample per microbiological and chlorate analysis, respectively) were aseptically taken randomly from the boxes before washing (unwashed tomato), and after leaving the dewatering area (washed tomato). Three types of tests were carried out as described below. Each type of test was performed only once.
First Test: Overhead Spray With Tap Water Without Water Reuse
This treatment represents the regular washing procedure applied by the processor. For this test, 10 boxes of tomato (≈120 kg) were used. The recirculation tank was filled with tap water that was pumped to the overhead spray bars, and the effluent from the washing machine was led to a tank for wastewater. The global flow rate of the overhead spray bars was ~250 L/h. Water samples were taken from the effluent of the washing machine during the washing of tomatoes. Two water samples for microbiological analysis and three samples for physicochemical analysis were taken. Four samples of plant material (one of unwashed tomato and three of washed tomato at different times) were taken for microbiological analysis.
Second Test: Untreated Recirculated Wash Water
A volume of 200 L of water from the box washer was placed in the recirculation tank. The water was pumped to the overhead spray bars and, in this case, the wash water returned to the recirculation tank to be pumped back to the spray bars. During this test, 50 boxes of tomato (≈600 kg) were washed in about 10 min. A total of 10 water samples (two samples in five sampling times) were taken throughout the trial for microbiological analysis and another 10 water samples for physicochemical analysis. Fifteen tomato samples were taken for microbiological analysis. The samples of plant material corresponded to three samples of unwashed tomato and 12 samples of washed tomato taken throughout the test (three samples in four sampling times).
Third Test: ClO2-Treated Recirculated Wash Water
The configuration was the same as in the previous test, but in this case, ClO2 (AGRI DIS®, STC S.L.U., Las Palmas de Gran Canaria, Spain) was added in the recirculation tank to disinfect the water. A volume of 200 L of water from the box washer was placed in the recirculation tank. Before washing the tomatoes, the concentration of ClO2 in the wash water was adjusted by adding disinfectant to the recirculation tank and measuring the concentration in the sprayed water until a concentration of ≈3 mg/L was achieved. During this test, 50 boxes of tomato (≈600 kg) were washed in about 7 min. Ten water samples (two samples in five sampling times) were taken for microbiological analysis, and other 10 water samples were taken for physicochemical analysis. Three samples of unwashed tomato and 12 samples of washed tomato (three samples in four sampling times) were taken for microbiological analysis. Besides, at the same sampling times, three samples of unwashed tomato, and 12 samples of washed tomato were taken for analysis of the presence of chlorate.
Physicochemical Analysis of Water
The ClO2 concentration in water was measured using the chronoamperometric method ChlordioXense (Palintest, Gateshead, United Kingdom). The oxidation-reduction potential (ORP), temperature, pH, and electrical conductivity were measured using a multimeter (Hach, Loveland, USA). The chemical oxygen demand (COD) was measured by the standard photometric method using the photometer Spectroquant Nova60 (Merck). The absorbance at 254 nm (UVA254) was determined in water filtered through 0.45 μm nylon syringe filters (Fisherbrand-Fisher Scientific, Waltham, USA) using a UV-VIS spectrophotometer (Jasco V-630, Tokyo, Japan) and quartz cuvettes with a length of 1 cm (Hellma, Müllheim, Germany). The chlorate presence was analyzed by UPLC-MS as in Gil et al. (2016), expressing the results in mg/L.
Microbiological Analysis of Water
Water samples were analyzed by filtration and by surface plating. The filtration of the samples was carried out using 0.45 μm membrane filters (Sartorius, Göttingen, Germany) which were incubated in a suitable culture medium. For aerobic mesophilic bacteria (AMB), the medium used was Plate Count Agar (PCA, Scharlab, Barcelona), and incubation conditions were 30°C for 36–48 h. For total coliforms and Escherichia coli, Chromocult coliform agar (Merck, Darmstadt, Germany) and incubation at 37°C for 24 h were used. For yeasts and molds, Rose Bengal Agar (Scharlab) and incubation at 30°C for 72 h were used.
Microbiological Analysis of Tomato
For the microbiological analysis of the tomatoes, the three fruits from each sample were serially rubbed for 1 min with 100 mL of peptone water (2 g/L) supplemented with sodium thiosulphate. The same techniques and the same culture media used for water samples were utilized to study the populations of the different groups of microorganisms in the rinsate (AMB, yeasts and molds, total coliforms and E. coli). Additionally, an enrichment stage was utilized to evaluate the presence of E. coli. For this, 50 mL of rinsate was mixed with 50 mL of BPW (40 g/L) and incubated at 37°C for 24 h. The presence of E. coli in the enriched samples was analyzed by streak plating in Chromocult plates incubated at 37°C for 24 h before the reading of the results.
Presence of Chlorate in Tomato
Peel samples were obtained from the five tomatoes from each sample by using kitchen produce peelers. Samples were prepared and analyzed by UPLC-MS as in Tudela et al. (2019). The results were stated in mg/kg fresh weight (FW).
Results
Physicochemical Characteristics of Wash Water
Table 1 shows the average values of the different physicochemical parameters analyzed in recirculated water from the 2nd test (untreated recirculated water; n = 10) and the 3rd test (ClO2-treated recirculated water; n=10). The changes in disinfectant concentration throughout the 3rd test are shown in Figure 2A. ClO2 levels in treated water decreased from 2.9 to 2.3 mg/L during the test, with an average of 2.7 ± 0.3 mg/L. No more ClO2 was added during the test to compensate for this decrease since the concentration remained within the desired range (2–3 mg/L). The pH of the untreated (2nd test) and the treated recirculated water (3rd test) was very similar, in the range 7–7.5, and there were no variations caused by washing the tomatoes. The untreated recirculated water showed ORP values, in general, between 200 and 300 mV, with little variation throughout the test. In the ClO2-treated water, the values of ORP were significantly higher (p < 0.05; 600–700 mV; Table 1) and constant throughout the trial. COD and UVA254 values in treated and untreated recirculated water are shown in Figures 2B,C. In both untreated and treated recirculated water, it was observed a trend of increase in the value of these two parameters throughout the test. The increase in UVA254 was higher in the case of water treated with ClO2 while this difference was not observed in the case of COD (Figure 2). The water's temperature was close to 20°C in both tests. The conductivity was significantly higher in the treated water (p < 0.05; Table 1), and it was not affected by tomato washing.
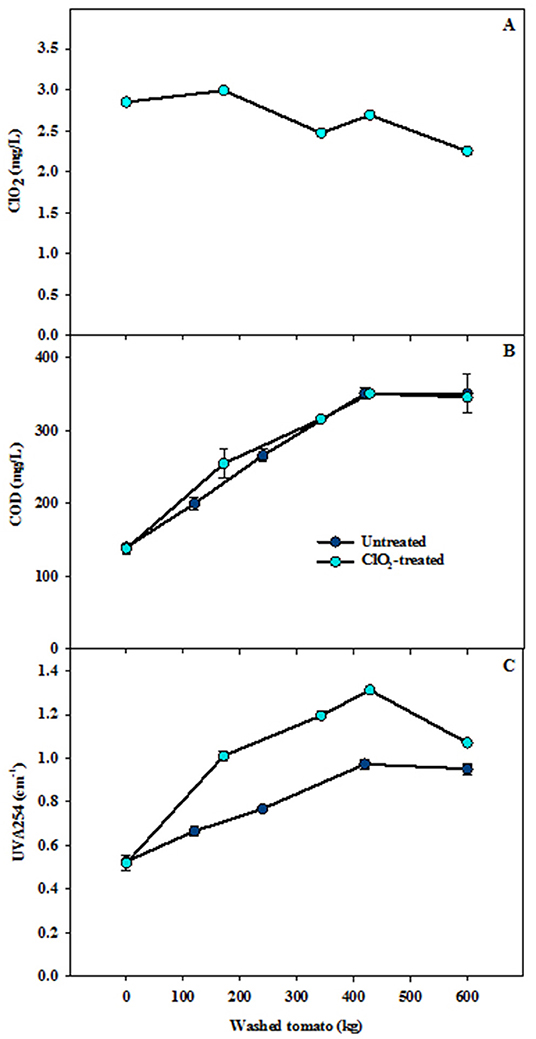
Figure 2. (A) Concentration of ClO2 in the test performed with treated recirculated water (3rd test). (B) Chemical oxygen demand (COD) in the tests performed with untreated (2nd test) and ClO2−treated recirculated water (3rd test). (C) Absorbance at 254 nm (UVA254) in the tests performed with untreated (2nd test) and ClO2−treated recirculated water (3rd test).
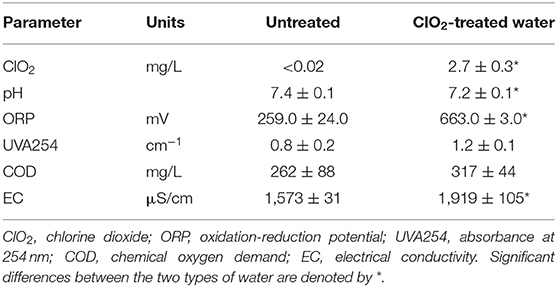
Table 1. Mean ± standard deviation of the different water physicochemical parameters measured in untreated (2nd test of recirculated water without ClO2 treatment; n = 10) and ClO2-treated water (3rd test of recirculated water with ClO2 treatment; n = 10).
Microbiological Characteristics of Wash Water
Table 2 shows the average values of AMB, total coliforms, yeasts and molds, and Escherichia coli, in the recirculated water from the 2nd and 3rd tests. Throughout the tests, the concentrations of the different microbial groups remained more or less constant in untreated water (2nd test) and water treated with ClO2 (3rd test). The microbial load present in untreated recirculated water (2nd test) was very high (>9 log cfu/100 mL AMB), and all the samples were positive for the presence of E. coli (n = 10) with an average concentration between 2 and 3 log cfu/100 mL. The recirculated wash water treated with ClO2 (3rd test) showed significantly lower AMB and total coliform counts than the untreated water (p < 0.05). Furthermore, there was an absence of E. coli in most ClO2-treated water samples (six out of 10), with populations always ≤1 log cfu/100 mL in the positive samples. It is remarkable that in the 1st test, where tap water without recirculation, the microbiological load of the water after the one-single pass washing was also high, showing levels of AMB of 7.5 ± 0.4 log cfu/100 mL, although in this case, there was an absence of E. coli (<1 cfu/100 mL).
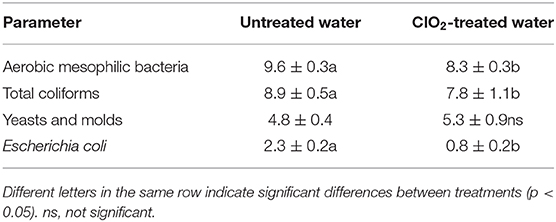
Table 2. Microbiological populations in recirculated wash waters (log cfu/100 mL; mean ± standard deviation; n = 10).
Microbial Populations in the Tomato
The graphs in Figure 3 show the populations of AMB, total coliforms, and yeasts and molds in tomatoes before and after washing. Counts of AMB, total coliforms, and yeasts and molds in the unwashed product were not significantly different (p > 0.05) from those in product washed with ClO2-treated water (3rd test). However, the populations of AMB, total coliforms, and yeasts and molds were significantly higher (p < 0.05) in tomatoes washed in untreated recirculated water (2nd test) than in tomatoes washed in ClO2-treated water (3rd test). Regarding the presence of E. coli as an indicator of fecal contamination, E. coli was not detected in any of the tomato samples analyzed, by direct plating or by plating after enrichment.
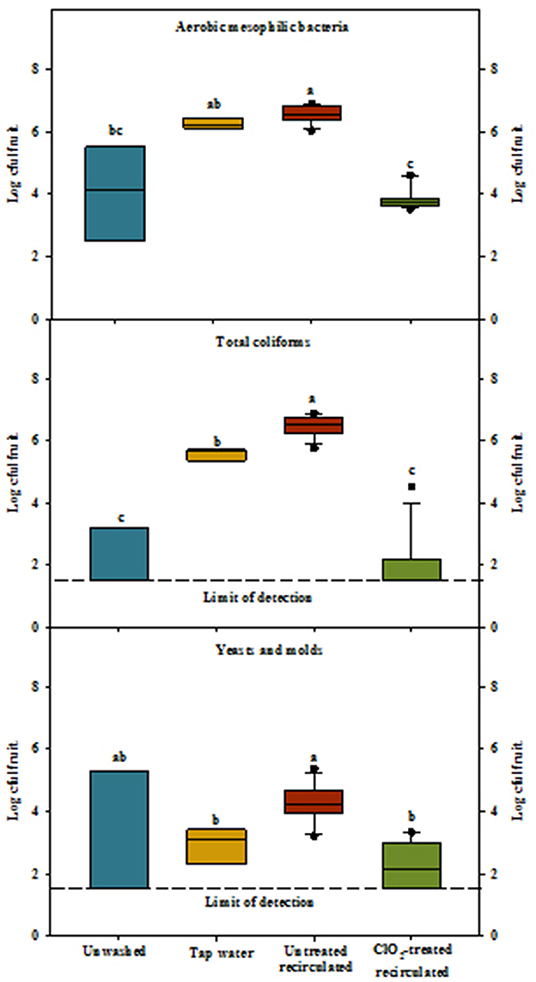
Figure 3. Boxplots of different microbial groups (log cfu/fruit) in unwashed tomatoes, tomatoes washed with tap water (1st test), washed with untreated recirculated water (2nd test), and washed with ClO2-treated recirculated water (3rd test). Different letters above the boxplots indicate significant differences in the microbial population between different tomato states.
Presence of Chlorate in Water and Tomato
Figure 4 shows the concentration of chlorate in the 3rd test (ClO2 treatment) in the water (Figure 4A) and the washed tomatoes (Figure 4B). The average concentration detected in the water treated with ClO2 was 45.9 ± 0.5 mg/L. Chlorate levels were much lower in tap water (1st test; 0.2 ± 0.0 mg/L) and in the untreated recirculated water (2nd test; 1.5 ± 0.1 mg/L). In all the washed tomato samples analyzed for chlorate (3rd test) the levels were above 0.01 mg/kg, the maximum concentration detected being 0.04 mg/kg. Unwashed tomatoes also showed detectable levels of chlorate (0.03 ± 0.01 mg/kg). The difference in chlorate concentration between unwashed tomato and the product washed in ClO2-treated water was not significant (p > 0.05).
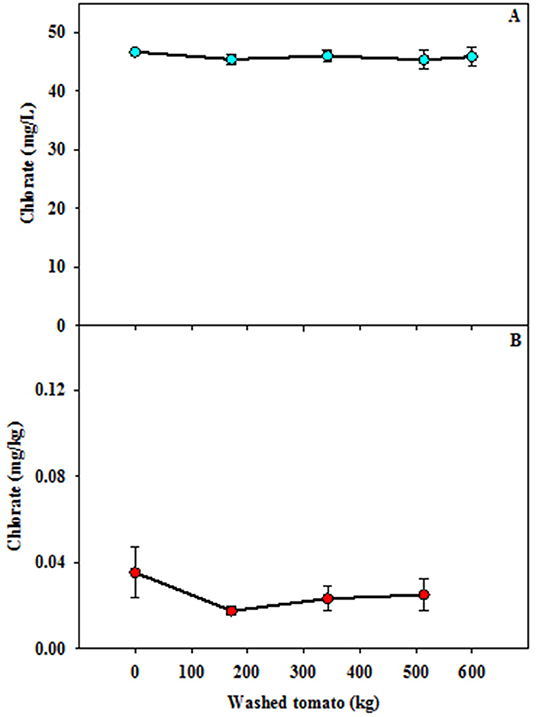
Figure 4. Concentration of chlorate in (A) ClO2-treated recirculated water (3rd test) and (B) tomato washed in ClO2-treated water (3rd test).
Discussion
The target ClO2 concentration used in the present study was selected based on the maximum concentration allowed for washing fruits and vegetables that are not raw agricultural commodities in the USA (3 mg/L; FDA). The decrease in ClO2 concentration during washing in the 3rd test had different causes, such as the volatilization of ClO2 due to the spraying of water in the overhead spray bars, and the consumption of the disinfectant by reaction with oxidizable substances present in the washing machine or fruit surfaces, as well as in the process water. ClO2 is more stable in fresh-cut produce wash water than chlorine, but less stable than peroxyacetic acid (van Haute et al., 2017). The neutral pH of the ClO2-treated water was adequate for effective antimicrobial action, as this disinfectant is efficient in a wide pH range between 3 and 9 (Junli et al., 1997). ClO2 has a higher solubility in aqueous solutions at lower temperatures than the room temperatures used in the assessed facility for tomato washing (Gevantman, 2004). However, it has been proven that the antimicrobial efficacy is higher at room temperature compared with lower temperatures (Junli et al., 1997; López-Velasco et al., 2012).
Tomás-Callejas et al. (2012), analyzing industrial tomato wash water treated with ClO2, observed ORP values similar to those detected in the present study in the ClO2-treated water. In the treated water from our study, ORP values were always above 650 mV, which indicates the presence of oxidizing species for effective disinfection (American Public Health Association (APHA)., 2012). UVA254 and COD are parameters that are linked to the content of organic matter in the fresh produce wash water (Luo et al., 2012; Chen and Hung, 2017). The increase in these two parameters when water was recirculated was caused by the accumulation in the water of substances present on the surface of the tomatoes. In the case of UVA254, the increase was higher in the water treated with ClO2, probably due to the presence of oxidants in the treated water (American Public Health Association (APHA)., 2012).
López-Gálvez et al. (2020), observed populations of AMB similar to those observed in the present study (8–10 log cfu/100 mL) in untreated bell pepper wash water. In contrast, Holvoet et al. (2012) observed lower total microbial counts (≈6 log cfu/100 mL) in untreated wash water from fresh-cut produce processing companies. The E. coli counts detected in untreated recirculated water (2nd test) were higher than those recommended for fruit and vegetable process water (EC European Commission, 2017), highlighting the need for the implementation of a disinfection treatment to maintain the microbiological quality of the process water. On the other hand, the reason for the high microbial levels in the water from the 1st test (single-pass tap water washing) could be the presence of microbial contamination in the washing machine (in pipes, rollers, and plates) that rapidly contaminated the tap water.
Although the use of ClO2 as water disinfectant reduced the microbial load in the treated water, high concentrations of AMB, coliforms, and yeasts and molds were still detected in the process water. Tomás-Callejas et al. (2012) observed much lower microbial populations in tomato wash water treated with ClO2 than those reported in the present study. This difference could be caused by the different experimental design used in the two studies. In our case, trying to mimic the situation at the end of a working day where wash water is recirculated for ~8–12 h, we started our test with highly contaminated water that was treated with ClO2 until reaching the desired residual concentration of disinfectant. In contrast, in the study by Tomás-Callejas et al. (2012), the addition of ClO2 to the process water started before the entry of microbial contamination in the water, and the disinfectant was able to keep low microbial levels during the test. Although the experimental design of Tomás-Callejas et al. (2012) was more similar to the washing dynamic in a commercial washing line, we designed our tests to evaluate a worst-case scenario.
It should be taken into account that, as established by European legislation Regulation (EC) No 852/2004 (EC European Commission, 2004) concerning the hygiene of food products, the recycled water that is used in the production process must be of drinking water quality, unless the competent authority has determined that the quality of water cannot affect the healthiness of food products in their final form. On the other hand, in a guide published by the European Commission on the good practices for the handling of vegetable products (EC European Commission, 2017), it is indicated that the pre-wash water may contain maximum levels of E. coli of 100 CFU/100 mL. However, final wash water must be of potable quality, which entails the absence of E. coli (≤1 CFU/100 mL). The washing system assessed in the tests had only one washing step, and the most restrictive limit (≤1 CFU / 100 mL) should apply. Based on the guideline recommendations, 100% of untreated recirculated water samples and 40% of the water samples treated with ClO2 had levels of E. coli higher than indicated for washing water in direct contact with the vegetable product.
The levels of microorganisms detected on the surface of the tomato before washing were similar to those observed in the study by Tomás-Callejas et al. (2012). De et al. (2018) observed higher aerobic plate counts of ≈6.5 log cfu/g in unprocessed tomatoes from packinghouses located in Florida (USA). The higher counts in the product washed in the untreated recirculated water (2nd test) could be explained by the high microbial load present in the water. Tomás-Callejas et al. (2012), observed significantly higher counts of AMB and coliforms in washed tomatoes compared with unwashed fruits in packinghouses using ClO2-treated water. In contrast, De et al. (2018) detected lower aerobic plate counts in processed tomatoes than in unprocessed tomatoes. Balaguero et al. (2015) found the combination of spray (with sanitizer) with roller brushes to be useful for the removal of microbial contamination while not affecting the quality of tomatoes.
De et al. (2018), detected a prevalence of generic E. coli < 5.5% in tomatoes from packinghouses located in Florida (USA) (n = 1678). In our study, none of the tomato samples analyzed was positive for E. coli (prevalence 0%), but it should be taken into account that the sampling size in our study was much smaller (n = 34). Pao et al. (2009) and Chang and Schneider (2012) observed significant reductions of Salmonella spp. populations in tomatoes treated with a combination of chlorine ClO2 and roller brushes. In the study by Pao et al. (2009), it was also observed that spraying with ClO2 was useful for avoiding cross-contamination between contaminated roller brushes, product, and water effluent.
The chlorate concentrations detected in ClO2-treated water (3rd test) could be representative of the levels that could be reached at the end of a workday if the water is reused and no water replenishment is made. López-Gálvez et al. (2019) detected chlorate concentrations as high as 47.4 mg/L in an industrial shredded produce washing line that used chlorine as a disinfectant. All the tomato samples showed chlorate levels above the current maximum residue level (MRL) (0.01 mg/kg; EFSA, 2015). However, a modification of MRLs is under study (EFSA, 2019), and the new MRL suggested for tomato (0.1 mg/kg) is higher than the concentration detected in the present study (≤0.04 mg/kg). Despite the high chlorate concentration in the wash water treated with ClO2, there was no significant increase in chlorate concentration in tomatoes. One of the reasons for this low transfer of chlorate from water to the product would be the absence of irregularities in the surface of the fruit, the reduced number of stomata, and the hydrophobic nature of the cuticle. Garrido et al. (2019) observed chlorate transfer rates from wash water to product ranging from 1 to 9%, in different types of fresh-cut products. In the present study, assessing an uncut product with smooth surface, the transfer was <0.1%.
Regarding the chemical safety of fresh produce, the effect of sanitizers on the presence of pesticide residues in the final product should also be taken into account (López-Fernández et al., 2013), although this aspect was not examined in the present study.
Conclusions
ClO2 treatment was able to reduce the microbial populations (including the prevalence of E. coli) in wash water with a very poor microbiological and physicochemical quality. This antimicrobial treatment would help to reduce microbial safety risks linked to wash water reuse. The microbial load was similar in tomato washed with the current method (single-pass tap water wash) vs. tomato washed with recirculated ClO2-treated water. Although ClO2 leads to the presence of chlorate in wash water, it would not be a problem for products such as uncut tomato that show minimal uptake potential. The results obtained suggest that the ClO2 treatment could be applied to enable water reuse, reducing water consumption and wastewater generation without increasing the safety risk for consumers.
Data Availability Statement
The datasets generated for this study are available on request to the corresponding author.
Author Contributions
FL-G and JT performed the experiment and analyzed the data. AA, FL-G, and MG designed the experiment and discussed the results. AA and FL-G wrote up the manuscript.
Funding
Authors are thankful for the financial support from the Ministerio de Ciencia e Innovación (Project AGL2016-75878-R), the Center for Produce Safety Grant Agreement (Project 2017-01), and CSIC (Intramural 201670E056). Support provided by the Fundación Séneca (19900/GERM/15) is also appreciated.
Conflict of Interest
The authors declare that the research was conducted in the absence of any commercial or financial relationships that could be construed as a potential conflict of interest.
References
American Public Health Association (APHA). (2012). Standard Methods for the Examination of Water and Wastewater, 22nd Edn. Washington, DC: American Public Health Association.
Balaguero, A. N., Sreedharan, A., and Schneider, K. R. (2015). Effect of overhead spray and brush roller treatment on the survival of Pectobacterium and Salmonella on tomato surfaces. J. Food Protect. 78, 51–56. doi: 10.4315/0362-028X.JFP-14-254
Casani, S., Rouhany, M., and Knøchel, S. (2005). A discussion paper on challenges and limitations to water reuse and hygiene in the food industry. Water Res. 39, 1134–1146. doi: 10.1016/j.watres.2004.12.015
Chang, A. S., and Schneider, K. R. (2012). Evaluation of overhead spray-applied sanitizers for the reduction of Salmonella on tomato surfaces. J. Food Sci. 77, M65–M69. doi: 10.1111/j.1750-3841.2011.02486.x
Chen, X., and Hung, Y.-C. (2017). Effects of organic load, sanitizer pH and initial chlorine concentration of chlorine-based sanitizers on chlorine demand of fresh produce wash waters. Food Control 77, 96–101. doi: 10.1016/j.foodcont.2017.01.026
Danyluk, M. D., and Schaffner, D. W. (2011). Quantitative assessment of the microbial risk of leafy greens from farm to consumption: preliminary framework, data, and risk estimates. J. Food Protect. 74, 700–708. doi: 10.4315/0362-028X.JFP-10-373
De, J., Li, Y., Sreedharan, A., Goodrich Schneider, R., Gutierrez, A., Jubair, M., et al. (2018). A three-year survey of Florida packinghouses to determine microbial loads on pre- and post-processed tomatoes. Food Control 86, 383–388. doi: 10.1016/j.foodcont.2017.11.036
EC European Commission. (2004). Regulation (EC) No 852/2004 of the European Parliament and of the Council of 29 April 2004 on the hygiene of foodstuffs. Off. J. Eur. Union 47, L139/1.
EC European Commission. (2017). Commission notice on guidance document on addressing microbiological risks in fresh fruits and vegetables at primary production through good hygiene. Off. J. Eur. Union C 163, 1–40.
EFSA (2015). CONTAM Panel (EFSA Panel on Contaminants in the Food Chain). Risks for public health related to the presence of chlorate in food. EFSAJ. 13, 1830–5458. doi: 10.2903/j.efsa.2015.4135
EFSA (2019). European Commission Regulation, Amending Annex III to Regulation (EC) No 396/2005 of the European Parliament and of the Council as Regards Maximum Residue Levels for Chlorate in or on Certain Products. Regulation - Ares(2019)334046 - 21/01/ 2019.FDA Food and Drug Administration. Code of Federal Regulations, Title 21, Chapter I, Subchapter B, Part 173, Subpart D—Specific Usage Additives, 173.300 Chlorine dioxide. Parma.
FDA (2019). Code of Federal Regulations, Title 21, Chapter I, Subchapter B, Part 173, Subpart D—Specific Usage Additives, 173.300 Chlorine dioxide. Silver Spring, MD: Food and Drug Administration.
Fu, T.-J., Li, Y., Awad, D., Zhou, T.-Y., and Liu, L. (2018). Factors affecting the performance and monitoring of a chlorine wash in preventing Escherichia coli O157:H7 cross-contamination during postharvest washing of cut lettuce. Food Control 94, 212–221. doi: 10.1016/j.foodcont.2018.06.035
Garrido, Y., Marín, A., Tudela, J. A., Allende, A., and Gil, M. I. (2019). Chlorate uptake during washing is influenced by product type and cut piece size, as well as washing time and wash water content. Postharvest Biol. Tec. 151, 45–52. doi: 10.1016/j.postharvbio.2019.01.014
Gevantman, L. H. (2004). “Solubility of selected gases in water”, in Handbook of Chemistry and Physics, eds D.R. Lide (Boca Raton, Fl: CRC Press, 8–87.
Gil, M. I., Marín, A., Andujar, S., and Allende, A. (2016). Should chlorate residues be of concern in fresh-cut salads? Food Control 60, 416–421. doi: 10.1016/j.foodcont.2015.08.023
Gombas, D., Luo, Y., Brennan, J., Shergill, G., Petran, R., Walsh, R., et al. (2017). Guidelines to validate control of cross-contamination during washing of fresh-cut leafy vegetables. J. Food Protect. 80, 312–330. doi: 10.4315/0362-028X.JFP-16-258
Gómez-López, V. M., Rajkovic, A., Ragaert, P., Smigic, N., and Devlieghere, F. (2009). Chlorine dioxide for minimally processed produce preservation: a review. Trends Food Sci. Tech. 20, 17–26. doi: 10.1016/j.tifs.2008.09.005
Holvoet, K., Jacxsens, L., Sampers, I., and Uyttendaele, M. (2012). Insight into the prevalence and distribution of microbial contamination to evaluate water management in the fresh produce processing industry. J. Food Protect. 75, 671–681. doi: 10.4315/0362-028X.JFP-11-175
Junli, H., Li, W., Nanqi, R., and Fang, M. Juli (1997). Disinfection effect of chlorine dioxide on bacteria in water. Water Res. 31, 607–613. doi: 10.1016/S0043-1354(96)00275-8
López-Fernández, O., Rial-Otero, R., and Simal-Gándara, J. (2013). Factors governing the removal of mancozeb residues from lettuces with washing solutions. Food Control 34, 530–538. doi: 10.1016/j.foodcont.2013.05.022
López-Gálvez, F., Truchado, P., Tudela, J. A., Gil, M. I., and Allende, A. (2020). Critical points affecting the microbiological safety of bell peppers washed with peroxyacetic acid in a commercial packinghouse. Food Microbiol. 88:103409. doi: 10.1016/j.fm.2019.103409
López-Gálvez, F., Tudela, J. A., Allende, A., and Gil, M. I. (2019). Microbial and chemical characterization of commercial washing lines of fresh produce highlights the need for process water control. Innov. Food Sci. Emerg. 51, 211–219. doi: 10.1016/j.ifset.2018.05.002
López-Velasco, G., Tomás-Callejas, A., Sbodio, A., Artés-Hernández, F., and Suslow, T. V. (2012). Chlorine dioxide dose, water quality and temperature affect the oxidative status of tomato processing water and its ability to inactivate Salmonella. Food Control 26, 28–35. doi: 10.1016/j.foodcont.2011.12.016
Luo, Y., Nou, X., Millner, P., Zhou, B., Shen, C., Yang, Y., et al. (2012). A pilot plant scale evaluation of a new process aid for enhancing chlorine efficacy against pathogen survival and cross-contamination during produce wash. Int. J. Food Microbiol. 158, 133–139. doi: 10.1016/j.ijfoodmicro.2012.07.008
Maffei, D. F., Sant'Ana, A. S., Franco, B. D. G. M., and Schaffner, D. W. (2017). Quantitative assessment of the impact of cross-contamination during the washing step of ready-to eat leafy greens on the risk of illness caused by Salmonella. Food Res. Int. 92, 106–112. doi: 10.1016/j.foodres.2016.12.014
Manzocco, L., Ignat, A., Anese, M., Bot, F., Calligaris, S., Valoppi, F., et al. (2015). Efficient management of the water resource in the fresh-cut industry: current status and perspectives. Trends Food Sci. Tech. 46, 286–294. doi: 10.1016/j.tifs.2015.09.003
Meireles, A., Giaouris, E., and Simões, M. (2016). Alternative disinfection methods to chlorine for use in the fresh-cut industry. Food Res. Int. 82, 71–85. doi: 10.1016/j.foodres.2016.01.021
Pao, S., Kelsey, D. F., and Long, 3rd W. (2009). Spray washing of tomatoes with chlorine dioxide to minimize Salmonella on inoculated fruit surfaces and cross-contamination from revolving brushes. J. Food Protect. 72, 2448–2452. doi: 10.4315/0362-028X-72.12.2448
Tomás-Callejas, A., López-Velasco, G., Valadez, A. M., Sbodio, A., Artés-Hernández, F., Danyluk, M. D., et al. (2012). Evaluation of current operating standards for chlorine dioxide in disinfection of dump tank and flume for fresh tomatoes. J. Food Protect. 75, 304–313. doi: 10.4315/0362-028X.JFP-11-347
Tudela, J. A., López-Gálvez, F., Allende, A., and Gil, M. I. (2019). Chlorination management in commercial fresh produce processing lines. Food Control 106:106760. doi: 10.1016/j.foodcont.2019.106760
van Haute, S., Tryland, I., Escudero, C., Vanneste, M., and Sampers, I. (2017). Chlorine dioxide as water disinfectant during fresh-cut iceberg lettuce washing: disinfectant demand, disinfection efficiency, and chlorite formation. LWT Food Sci. Technol. 75, 301–304. doi: 10.1016/j.lwt.2016.09.002
Keywords: water treatment, microbiological hazard, cross-contamination, fresh produce, food safety, sustainability
Citation: López-Gálvez F, Tudela JA, Gil MI and Allende A (2020) Use of Chlorine Dioxide to Treat Recirculated Process Water in a Commercial Tomato Packinghouse: Microbiological and Chemical Risks. Front. Sustain. Food Syst. 4:42. doi: 10.3389/fsufs.2020.00042
Received: 29 November 2019; Accepted: 27 March 2020;
Published: 22 April 2020.
Edited by:
Paula Teixeira, Catholic University of Portugal, PortugalReviewed by:
Guadalupe Virginia Nevárez-Moorillón, Autonomous University of Chihuahua, MexicoJesus Simal-Gandara, University of Vigo, Spain
Copyright © 2020 López-Gálvez, Tudela, Gil and Allende. This is an open-access article distributed under the terms of the Creative Commons Attribution License (CC BY). The use, distribution or reproduction in other forums is permitted, provided the original author(s) and the copyright owner(s) are credited and that the original publication in this journal is cited, in accordance with accepted academic practice. No use, distribution or reproduction is permitted which does not comply with these terms.
*Correspondence: Francisco López-Gálvez, flopez@cebas.csic.es