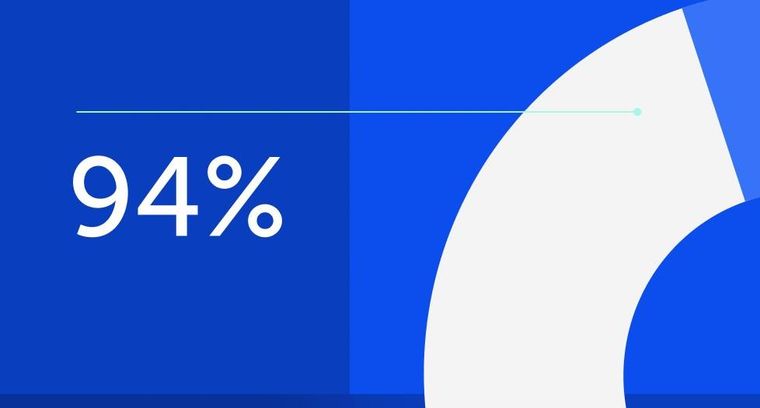
94% of researchers rate our articles as excellent or good
Learn more about the work of our research integrity team to safeguard the quality of each article we publish.
Find out more
MINI REVIEW article
Front. Sustain. Food Syst., 26 February 2020
Sec. Waste Management in Agroecosystems
Volume 4 - 2020 | https://doi.org/10.3389/fsufs.2020.00018
This article is part of the Research TopicInnovative Biorefinery Processes for Added Value Products from Organic ResiduesView all 7 articles
With the growing consumer demand for natural food products, including food flavors, efforts toward identifying biotechnological interventions for their production have increased tremendously. The present paper reviews the production of flavor compounds by microbial biosynthesis or biotransformation, and their enzymes involved in synthesis and conversion from progenitor substrates. More importantly, the use of abundant, inexpensive, and nutrient-rich lignocellulosic waste generated by the processing set-ups of agro-industries as a potential substrate for the microbial production of flavor compounds under solid-state fermentation is reviewed. The article provides insights regarding the development of an efficient and cost-effective process to produce important flavors from agroresidues. The biotechnological route will also help in safe and eco-friendly management of wastes generated from agriculture.
The sensation of flavor is stimulated by a complex matrix of volatile as well as non-volatile compounds with varying physicochemical properties. Throughout history, simple to complex flavor compounds have been extracted from plants. The subsequent elucidation of molecular structure of these compounds, has led to their synthetic production and, now almost all flavoring components are produced in this manner. However, with the growing shift of consumer preferences toward natural food products, flavors produced by chemical synthesis cannot be labeled as “natural.” Furthermore, chemical production is not environment-friendly and lacks substrate sensitivity, resulting in the formation of undesirable racemic mixtures (Longo and Sanromán, 2006). Conversely, the process of extracting natural flavors from plants also has limitations. These include the high cost of enriching flavor compounds that are present at very low concentrations, the dependence on seasonal and climatic conditions, and ecological problems associated with extraction.
Alternatively, microbial transformation or bioconversion of suitable substrates is an environment-friendly and cost-effective alternative for the production of “natural flavors.” This involves the use of (a) microbial cultures as an integral part of the food product and (b) microbes or their enzymes for bioconversion of appropriate substrates to the desired flavor compounds, followed by their extraction from the reaction medium/fermentate (Figure 1). In the latter case, solid-state fermentation (SSF), a process in which microbes are grown on a solid support in the absence of free-flowing water (Singhania et al., 2009), shows promise. Recently, SSF of food- and agroresidues has gained attention as a means for producing enzymes, flavors, colorants, and other compounds relevant to the food industry. Compared with submerged fermentation, SSF offers numerous advantages, such as higher yields, lower energy requirements, and the efficient utilization and addition of value to waste (Cerda et al., 2019).
Food and agricultural wastes, rich in carbohydrates and other nutrients, are produced in large quantities and may serve as SSF substrates for the production of bulk chemicals and other compounds. These substrates also contain various bioactive metabolites, including flavor compounds, in different concentrations, and such compounds may be available by microbial fermentation or enzymatic catalysis. This review discusses the potential of microbial cultures and their enzymes to generate aroma and flavor compounds from agroresidues for use in the food industry.
The organoleptic characteristics of a food product include its taste, flavor, and aroma. Among all these parameters, aroma is one of the most powerful characteristics that determine its acceptance and market success (Berger, 2009). Aroma and flavor compounds can be classified as acids, hydrocarbons, aldehydes, alcohols, ketones, esters, or lactones (Bicas et al., 2010). The classification of aroma compounds according to their chemical structures is depicted in Figure 2. Since time immemorial, microorganisms are employed for the production of flavors in many foods. Products like wine, beer, fermented vegetables and milk, soya, meat vinegar, and pickles are preserved, modified and flavored by means of microbes. As previously indicated, microbial strains can be used for production of flavor compounds, either as in-situ flavor production in the fermented food product or in appropriate substrates from which flavors can be extracted and subsequently used in different food items (Longo and Sanromán, 2006). The most important group of flavors used in the food industry, as well as their production from microorganisms is discussed below:
Lactones are cyclic esters of gamma- and delta-hydroxy acids, which contribute a coconut-like, creamy, creamy, sweet, fruity, or nutty flavor. The biotechnological route for production of lactones during fermentation by various microorganisms is investigated by many researchers. For instance, Collin and Halim (1972) reported 6-pentyl-2-pyrone, which has a coconut aroma, to be the main volatile flavor component in cultures of Trichoderma viride. Some other fungi such as Tyromyces sambuceus and Cladosporium suaveolens also produce coconut flavor emanating lactones, namely, γ-decalactone and δ-dodecalactone from ricinoleic and linoleic acid, respectively (Kapfer et al., 1989; Allegrone et al., 1991). Yeasts such as Candida tropicalis and Yarrownia lipolytica can degrade ricinoleic acid to C16, C14, and C12 acids and also accumulate δ-decalactone, which exhibits fruity and oily aroma (Gatfield, 1999). While, Fadel et al. (2015) identified 6-pentyl-alpha-pyrone as responsible for the strong coconut aroma produced during solid-state fermentation of sugarcane bagasse by T. viride EMCC-107. Additionally, accumulation of delta-decalactone was obtained when the yeasts Lindnera saturnus CCMA 0243 and Yarrownia lipolytica CCMA 0242 were grown in residual castor oil (De Andrade et al., 2017). Recently, Marella et al. (2019) engineered oleaginous yeast Yarrowia lipolytica for hydroxylation of fatty acids and chain-shortening via β-oxidation to preferentially 12 or 10 carbons. The engineered strains were found to produce 4-fold higher concentrations of γ-dodecalactone from oleic acid and δ-decalactone from linoleic acid than the wild strain, thereby paving the way for the higher production of lactones by fermentation of available fatty feedstocks. The milky, buttery, and coconut-like flavors of these lactones produced by microbiological route is highly desirable in dairy products.
Triacylglycerides store fatty acids, which are released from acylglycerols by enzymatic oxidative degradation. The majority of the plant volatiles, including carboxylic acids, methylketones, esters, alcohols, and aldehydes, are derived from saturated and unsaturated fatty acids by alpha and beta-oxidation (Schwab et al., 2008). Dairy flavor fatty acids such as butyric acid and lactic acid can be produced by different lactic acid bacteria (Marshall, 1987). Madhavan Nampoothiri et al. (2010) have reviewed lactic acid production by various lactic acid bacterial (LAB) strains. Apart from LAB, lactic acid can also be produced by filamentous fungi, particularly Rhizopus sp. (Meussen et al., 2012). In a study by Meussen et al. (2012), R. oryzae was found to produce L-lactic acid, fumaric acid, and ethanol on different carbon sources with lactic acid being produced as the major product (Juturu and Wu, 2016). The dairy flavor of these fatty acids is desirable in many food and beverages.
Terpenes are volatile unsaturated hydrocarbons that impart characteristic odors to essential oils of plants (Janssens et al., 1992). Among all microorganisms, fungi are mainly responsible for the production of terpenes, a typical example being the genus Ceratocystis (Collin and Halim, 1972). The amount of terpenes formed by Ceratocystis moniliformis was found to be similar to that formed in plants (Lanza and Palmer, 1977). Zhao et al. (2010) observed a significant increase in terpene production in Norway spruce (Picea abies) after inoculation with Ceratocystis polonica. While, Trametes odorata and various species of the genus Phellinus also produce monoterpenes (Welsh et al., 1989). In a study by King and Dickinson (2000), three yeast species—Saccharomyces cerevisiae, Torulospora delbrueckii, and Kluveromyces lactis—were found to biotransformation monoterpene alcohols to terpenes, namely, citronellol, linalool, and nerol. Similarly, linalool and citranool produced by Saccharomyces cerevisiae (Carrau et al., 2005) were found to accumulate in wines. Chen et al. (2014) obtained terpenes and terpenoids from lychee wines fermented by Kluvermyces lactis strain KL71, with geraniol and citronellol having higher odor activity values (OAVs). In a recent study, the genome functional annotation for two Ceratocystis species identified pathways associated with the biosynthesis of various terpenes and volatile compounds (Molano et al., 2018). These terprenes are of considerable interest for application in the food flavor industry, especially linalool, nerol, geraniol, and citronellol due to their low sensory threshold.
Menthol is a terpene alcohol with a strong cooling, minty odor, and taste. It is extracted naturally from peppermint oil and produced synthetically by the hydrogenation of thymol. The compound is used in a variety of confectionaries and cough syrups. Bacillus subtilis, Absidiahyalospora, Geotrichumcandidum, and two species of Trichoderma can catalyze the conversion of menthyl acetate, propionate, formate, and myristate to menthol and isomenthol (Moroe et al., 1970). Species from the genera Rhodotorula, Trichoderma, Nocardia, Mycobacterium, Bacillus, Rhodococcus, Rhizopus, Candida, Hansenula, Streptomyces, Aerobacter, Arthrobacter, Pseudomonas, Gibberella, and Torulopsis are also known to bioconvert appropriate substrates to menthol (Armstrong et al., 1989; Chatterjee, 2004; Chandran et al., 2011). Toogood et al. (2015) used an engineered E. coli strain expressing genes for “ene”-reductase and menthone dehydrogenases from Mentha piperita for one-pot enzymatic production of menthol and neomenthol from pulegone.
Esters are one of the most significant chemical groups that impart a fruity, candy flavor and are used in fruit-flavored dairy products (Longo and Sanromán, 2006). Volatile esters are responsible for fruity, candy, and perfume-like aroma character of the beer, wine, and sake. Among these volatile esters, isoamyl acetate, with its peculiar banana-like flavor, is the most significant acetate ester present in alcoholic beverages. The second important group comprises medium-chain fatty acid ethyl esters, which include ethyl hexanoate (aniseed aroma) and ethyl octanoate (sour apple aroma). Lactic acid bacteria and several yeasts are known to produce these fruity esters (Longo and Sanromán, 2006). Liu et al. (2004) reviewed the biosynthesis of fruity flavor esters in dairy products by lactic acid bacteria, yeasts, molds, propionibacteria, and Pseudomonads. Saerens et al. (2010) reviewed the production of volatile esters by Saccharomyces cerevisiae. Layton and Trinh (2016) reported that the fungi Saccharomyces sp., Candida utilis, and Hansenula sp. produce these esters in a glucose-based fermentation medium. While, Walsh et al. (2017) performed metagenomic sequencing of kefir milk over the course of 24 h fermentation and revealed that Lactobacillus kefiranofaciens was the dominant bacterial species during the early stages of fermentation, but Leuconostoc mesentroides became prevalent in later stages. The results thus illustrate that microbial succession pattern can be applied to optimize fermentation process and enhance particular flavor of fermented foods. On the other hand, van Mastrigt et al. (2018) demonstrated that retentostat cultivation is the preferred method to produce cheese flavors from Lactococcus lactis outside the cheese matrix by mimicking the slow growth of bacteria during cheese ripening. Ruiz Rodriguez et al. (2019) studied the diversity and functional properties of lactic acid bacteria isolated from wild fruits and flowers. The strains were found to produce fruity flavor esters and thus can be used in the manufacture of fermented fruit-based products.
Unsaturated alcohols give a characteristic aroma to food products. Many yeasts produce long-chain complex alcohols with distinctive organoleptic properties (Longo and Sanromán, 2006). Mallouchos et al. (2003) conducted batch fermentation of wine using Saccharomyces cerevisiae immobilized on delignified cellulosic material, resulting in the production of 2-phenylethanol, which produces a rose-like aroma, in the fermented wine. Thereby, providing an alternative microbial route for the production of this alcohol, which is usually extracted from rose petals or synthesized chemically. Stark et al. (2002) reported production of 2-phenylethanol from 2-phenylalanine by yeasts such as Kluyveromyces marxianus, Saccharomyces cerevisiae, Hansenula anomala. Interestingly, Kim et al. (2014) engineered Kluyveromyces marxianus to overexpress genes encoding phenylpyruvate decarboxylase (ARO10) and alcohol dehydrogenase (ADH2) from Saccharomyces cerevisiae, leading to the overproduction of 2-phenylethanol from glucose.
The methyl ketones 2-heptaneone, 2-nonanone, and 2-undecanone are used to add fruity flavors to products. However, they are also produced during ripening of blue cheese by the action of microbial lipases (Engels et al., 1997). These lipases are mainly produced by Agaricus bisporus, Aspergillus niger, Penicillium roqueforti, and Trichoderma viride (Janssens et al., 1992).
Diacetyl is a vicinal diketone that imparts butter flavor and is thus used for simulating butter-like and other dairy flavors. It is produced by lactic acid bacteria and several other microorganisms in dairy foods (Longo and Sanromán, 2006). Interestingly, a high concentration of diacetyl (1.45 g/L) was produced in metabolically engineered Enterobacter clocae, whereas acetaldehyde and diacetyl were synthesized by the yeast Candida tropicalis strain D15 on a whey-based medium (Rosca et al., 2016).
Vanillin (4-hydroxy-3-methoxybenzaladehyde) is an important flavor in the food industry. The extraction of vanillin from vanilla pods is labor-intensive and expensive. The chemical route is not preferred due to increasing consumer demand for natural vanillin (Kumar and Pruthi, 2014). As a result, many research groups have produced vanillin by microbial bioconversion of eugenol and isoeugenol, which are obtained from essential oils (Ashengroph et al., 2012; Tan et al., 2015). Interestingly, lignocellulosic agroresidue is a rich source of ferulic acid that acts as a precursor for vanillin production through microbial or enzymatic transformations (Kumar and Pruthi, 2014). However, the production of vanillin by this method requires the release of ferulic acid from the lignocellulosic waste by enzymatic or chemical treatment (Zamzuri and Abd-Aziz, 2013). The released ferulic acid can be further biotransformed to vanillin, vanillic acid, and protocatechuic acid by some bacteria and fungi. Consequently, the importance of ferulic acid as a precursor for vanillin production has been realized in the area of bioflavor production (Kumar and Pruthi, 2014). Biotransformation of ferulic acid to vanillin has been assessed using various microorganisms, including Rhodococcus ssp., Actinomycetes spp., Corynebacterium glutamicum, Saccharomyces cerevisiae, Rhodotorula rubra, Debaryomyces hanseni, Halomonas elongata, Schizophyllum commune, Bacillus licheniformis, Bacillus coagulans, Bacillus subtilis, Pycnoporus cinnabarinus CGMCC1115, Pseudomonas sp. EF3, Pseudomonas fluorescens, Pseudomonas putida, Escherichia coli JM109/pBB1, Aspergillus niger CGMCC0774, Lactic acid bacteria, Amycolatopsis sp. HR167, Streptomyces sp. V-1, Streptomyces setonii ATCC39116, Streptomyces sannanensis, Streptomyces halstedii GE107678, and genetically engineered microbes (Chen et al., 2016). Ferulic acid esterase has been identified as one of the major microbial enzymes involved in the release of ferulic acid from natural substrates, which can be subsequently biotransformed into vanillin. A two-step bioconversion process has been implemented, wherein ferulic acid from sugarbeet pulp was converted to vanillic acid by A. niger, and then vanillic acid was reduced to vanillin by Pycnoporous cinnabarinus (Lesage-Meessen et al., 1996). Interestingly, high concentrations of vanillin were obtained from ferulic acid using lactic acid bacteria (Kaur et al., 2013) and Streptomyces setonii (Gunnarsson and Almqvist, 2006). However, since the process optimization is very difficult, the development of new recombinant strains able to produce vanillin is quite attractive. Narbad and Gasson (1997) demonstrated the transformation of ferulic acid to vanillin by Pseudomonas fluorescens involving a CoA ligase and side chain cleavage. In another work by Vaithanomsat and Apiwatanapiwat (2009), ferulic acid present in steam exploded hydrolysate of Jatropha curcas stem was successfully used as the substrate for one-step vanillin production by Aspergillus niger and Pycnoporus cinnabarinus. Torre et al. (2008) optimized the release of ferulic acid (1.17 g/L) from corn cobs using alkaline hydrolysis. In an interesting study by Tilay et al. (2008), various agricultural wastes, namely, maize bran, rice bran, wheat bran, wheat straw, sugar cane bagasse, pineapple peels, orange peels, and pomegranate peels were screened for the presence of esterified FA (EFA). Among the sources screened, maize bran was found to contain the highest amount of EFA. Pineapple peels, orange peels, and pomegranate peels were also found to contain traces of EFA. Several bacteria belonging to different genera are also able to use ferulic acid as their sole carbon source, thus producing vanillin, vanillic acid, and protocatechuic acid (Converti et al., 2010). Chen et al. (2016) also showed the potential of Bacillus subtilis B7-S to produce vanillin from ferulic acid.
Benzaldehyde is the second most important aldehyde after vanillin for producing cherry and fruity flavors. It can be extracted from apricots, but the process leads to the formation of undesirable hydroxycinnamic acid. Alternatively, microbial production of benzaldehyde from phenylalanine can be labeled as “natural,” without the production of unwanted by-products. In this regard, the production of benzaldehyde in a medium supplemented with phenylalanine has been reported for cultures of Pseudomonas putida (Tsou et al., 1990), Phanerochaete chrysosporium (Jensen et al., 1994), Polyporus tuberaster (Kawabe et al., 1994), Lactobacillus plantarum (Groot, 1998), Pycnoporus cinnabarinus (Lomascolo et al., 1999), Trametes suaveolens (Lomascolo et al., 2001), and Rhizopus oligosporus (Norliza and Ibrahim, 2005).
Pyrazines are nitrogen-containing heterocyclic compounds that impart roasted and nutty flavors. Bacillus subtilis was the first microorganism reported to produce pyrazines (Kosuge and Kamiya, 1962). B. subtilis IFO 3010 grown on soybeans supplemented with threonine and acetoin produced 2,5-dimethylpyrazine (2,5-DMP) and tetramethylpyrazine (TMP) (Besson et al., 1997). Corynebacterium glutamicum is also known to produce pyrazines from amino acids (Dickschat et al., 2010). Recently, Fadel and colleagues (Fadel et al., 2018) produced pyrazines with a nutty chocolate-like flavor by C. glutamicum grown on soyabean enriched with threonine and lysine. Pyrazines can also be produced in conventional cooking and roasting by Maillard reaction. However, due to change in the cooking techniques, for e.g., use of microwave ovens, pyrazines are not produced and thus causing need to supply natural pyrazines with roasty flavor as food additives.
Agroresidues refers to lignocellulosic waste (LCW), comprising of lignin, cellulose and hemicellulose. LCW is the most abundant, renewable, and inexpensive source of organic compounds available. Furthermore, the bioconversion of LCW into useful products will help to safely dispose of this solid waste. As reviewed by Bicas et al. (2010) and Zamzuri and Abd-Aziz (2013), the high costs involved in microbial production of flavor compounds can potentially be overcome by using agri-waste as a substrate. Studies on the generation of flavor compounds from agro-waste are well-supported. In this regard, Christen et al. (1997) observed that the fungus Ceratocystis frimbriata produced a fruity aroma when grown on sugarcane bagasse supplemented with a synthetic medium containing glucose, and when the medium was supplemented with leucine or valine, banana flavor was produced. Thus, alteration of culture conditions also help in manipulating the aroma obtained from microbial fermentations of suitable substrates. Bramorski et al. (1998b) produced fruity aroma by growing a C. fimbriata strain on four agro-industrial wastes: cassava bagasse, apple pomace, amaranth, and soybean. The authors identified esters and alcohols as the major flavor components, while small amounts of acid, aldehyde, and ketones were also detected. The authors also found a clear correlation between the growth and production of volatile compounds, based on the observation that the maximum production of volatiles always occurred a few hours before or after the maximum respirometric activity (Bramorski et al., 1998b).
Food and agricultural wastes are produced in large amounts and are rich in carbohydrates as well as other nutrients and thus can be used efficiently as substrate for the production of bulk chemicals, flavors per se under solid-state fermentation conditions. In addition to the supply of nutrients to the microbial cells, solid substrate also provides an anchorage site for the cells (Yan et al., 2016). The work of various researchers that have used food and agroresidues for microbial production of flavor compounds is summarized in Table 1.
Microorganisms can be directly used for the production of flavor compounds by solid-state fermentation of suitable substrates followed by extraction of the desired flavors, as discussed above. On the other hand, microbial enzymes can also be used for the release of flavors or appropriate precursors from agroresidues, which are finally biotransformed into the desired flavor compounds. Many researchers have used various microbial enzymes for the production of flavor compounds or their precursors by fermentation of appropriate substrates as summarized in Table 2. The most commonly used enzymes related to the production of aroma compounds or their precursors are described below:
Lipases are prominent biocatalysts that catalyze the esterification of alcohols and carboxylic acids. They are some of the most important enzymes that produce flavoring esters (Rajendran et al., 2009). A number of lipases produced from microorganisms have been tested for their ability to promote ester synthesis in aqueous media, such as those from Candida cylideracea, Pseudomonas fluorescens, Rhizomucor meihei, Mucor meihei, Aspergillus sp., Candia rugosa (Longo and Sanromán, 2006). Lipases can be successfully utilized for modification of skim milk (0.5% fat) into full-fat milk flavor by producing volatile flavor components (Zhang et al., 2016). In a study by Omar et al. (2016), lipases were used to hydrolyze anhydrous milk fat for the development of milk fat flavors due to the synthesis of volatile flavoring esters, acids, and ketones.
Interestingly, lipases can also resolve D, L-methylmenthol esters into pure L-menthol (Schreier, 2006). In this context, lipase from the fungus Candida rugosa exhibited high potential to resolve D,L–menthol in an organic solvent (Wang et al., 2002). Lipase from Thermomyces lanuginosus can perform high enantioselectivity for the kinetic resolution of (±)-menthol in organic solvent (De Yan et al., 2017).
Proteases hydrolyze protein into peptides, which have significant organoleptic as well as biological activity (Longo and Sanromán, 2006). The enzymatic treatment favors pyrazine production through Maillard reaction as well as co-production of alcohols. Many Lactobacillus species are used as starter culture in the dairy industry for the degradation of milk casein into small peptides and free amino acids through the action of various proteolytic enzymes (Razzaq et al., 2019). Proteases also contribute significantly to the flavor characteristics of cheese by accelerating cheese ripening, modifying properties, and reducing the allergenicity of milk products (Damhus et al., 2013).
Esterases are hydrolases that split esters into alcohols and acid in a chemical reaction with water. Free fatty acids liberated during the process of cheese ripening by the action of microbial esterases and lipases are responsible for the development of its characteristic flavor (Esteban-Torres et al., 2014). One of the most important examples of the application of esterases to flavor production is the use of carboxylesterases in the release of ferulic acid from plant cell walls. Ferulic acid serves as a precursor for the production of vanillin, avital flavor compound (Faulds et al., 1997). Feruloyl esterases have been isolated from a wide range of microorganisms, including Aspergillus, Streptomyces, Lactobacillus, and Pseudomonas (Topakas et al., 2007). Mathew and Abraham (2004) have reviewed the release of ferulic acid from different plant materials by the action of ferulic acid esterases. Recently, Uraji et al. (2018) elucidated the relationship between the structure and activity of ferulic acid esterase from Streptomyces sp. and observed that a catalytic triad (consisting of Ser-191, Asp-214, and His-268), typical for the serine esterase family, forms a loop-like structure (the R18 loop) that is responsible for the release of ferulic acid from biomass.
Glucosidases are the enzymes involved in breaking down complex carbohydrates into their monomers. They catalyze the cleavage of individual glucosyl residues from various glycoconjugates, including alpha- or beta-linked polymers of glucose. These enzymes enhance the aroma of wines by liberating glycoside-linked terpenes and other flavor precursors (Longo and Sanromán, 2006). Beta-glucosidases are produced by many microorganisms, including species of Trichoderma, Aspergillus, Bacillus, and Candida (Kuhad et al., 1997). A very efficient beta-glucosidase from Candida molischiana releases a number of flavor compounds, namely, nerol, geraniol, linalool, 2-phenylethanol, and benzyl alcohol in Muscat wine and linalool, alpha- and gamma-terpinene, alpha-terpineol, 2-phenylethanol, and alpha-pinene from apricot fruit juice (Gueguen et al., 1996). The role of yeast beta-glucosidase in improving the aroma of wines is due to the release of glycoside terpenes, as confirmed by Villena et al. (2007).
The processing of various agricultural commodities produces a huge amount of waste biomass that needs to be recycled. Microbial fermentation of this lignocellulosic biomass not only contributes to its eco-friendly disposal but also its valorization in the form of flavor products, which can find immense applications in the food industry. This review has illustrated the various facets of microbial synthesis of aroma compounds, with a focus on the use of lignocellulosic agro-waste as a substrate in SSF. Substrates from the agro-industry often have low costs, and closeness to the food industry, in general, leads to more easily obtained consumer acceptance of bioflavors as compared to synthetic flavors of petrochemical origin. The survey of literature highlights that the production of flavor compounds through a microbial route offers several advantages when compared with chemical synthesis or extraction from plant sources. Furthermore, the use of lignocellulosic agro-waste through SSF for the production of aroma compounds by microorganisms makes the process cost-effective as well as environment-friendly.
In any fermentation process, product recovery is often a crucial step. This is especially true for aroma compounds because their volatility and low solubility often make recovery challenging during downstream processing, Presently, several methods for flavor extraction from the fermentate have been used viz. absorption on resins, extraction in two-phase systems, membrane permeation, and pervaporation (a combination of membrane permeation and evaporation). However, all of these methods make the process cost-intensive and also result in low productivities. Therefore, cost-effective technologies for the extraction of flavor compounds are the subject of growing research interest to make the process of microbial production of flavor compounds feasible at a larger scale. Another challenge is the fact that many flavor compounds or their added precursors are inhibitory or toxic to the producer strains. In this respect, slow continuous feeding of low precursor levels (fed-batch fermentation), cell protection via immobilization or in-situ flavor extraction membranes and solvents are the main fermentation technologies, which could help to circumvent these limitations. Alternatively, a careful choice of production strains is also essential, since tolerance to the product may vary widely between same species. Similarly, process conditions must be chosen to maintain high physiological as well as the catalytic activity of the microbe. Furthermore, strain adaptation—or genetic engineering—may be used to increase the tolerance to the product. The continuous development of genetic engineering and systems biology tools, in combination with improved process design, will enable more bioflavors to be produced in this manner in the future.
Besides, the ultimate purpose of an industrially produced flavor compound is to induce a sensory effect, most often in humans. However, when volatile compounds are analyzed using GC coupled to any headspace-sampling or other sample preparation techniques, it is not sure whether detected compounds are contributing to odor or flavor. Moreover, the quantity of volatiles in the original sample is not directly related to the intensity of the smell. One solution is to divide the GC eluent before the instrumental detector and steer it partly to the human nose (GC–olfactometry) or using specific methods to identify the key odorants is aroma extract dilution analysis (AEDA).
It is thus clear that interdisciplinary cooperation between microbiologists, mycologists, biochemists, organic chemists, and bioprocess engineers is a prerequisite to develop interesting flavors in laboratory leading to an industrial process for bioflavor production.
AS written the main text of the mini review by searching and analyzing the literature. PS has searched the literature and helped in making table and figure. JS has written a part of the manuscript. SS has guided the authors in writing the review and also written a part of it. LN has given guidance as well as motivation in review writing along with her knowledge and expertise in the field.
The authors declare that the research was conducted in the absence of any commercial or financial relationships that could be construed as a potential conflict of interest.
AS acknowledges fellowship received from DST (File No. LS/700/2016) under Wos-A scheme. All the authors thank ICAR-IARI, Pusa, New Delhi for providing essential facilities for the research work. Authors are also thankful to Prof. Radha Prasanna, Division of Microbiology, ICAR-IARI, New Delhi for editing the manuscript.
Ai, B., Chi, X., Meng, J., Sheng, Z., Zheng, L., Zheng, X., et al. (2016). Consolidated bioprocessing for butyric acid production from rice straw with undefined mixed culture. Front. Microbiol. 7:1648. doi: 10.3389/fmicb.2016.01648
Allegrone, G., Barbeni, M., Cardillo, R., Fuganti, C., Grasselli, P., Miele, A., et al. (1991). On the steric course of the microbial generation of (Z6)-gamma-dodecenolactone from (10R, S) 10-hydroxy-octadeca-(E8, Z12)-dienoic acid. Biotechnol. Lett. 13, 765–768. doi: 10.1007/BF01026755
Armstrong, D. W., Gillies, B., and Yamazaki, H. (1989). “Natural flavors produced by biotechnological processing,” in Flavor Chemistry, eds R. Teranishi, R. G. Buttery, and F. Shahidi (Washington, DC: American Chemical Society), 105–120. doi: 10.1021/bk-1989-0388.ch009
Ashengroph, M., Nahvi, I., Zarkesh-Esfahani, H., and Momenbeik, F. (2012). Conversion of isoeugenol to vanillin by Psychrobacter sp. strain CSW4. Appl. Biochem. Biotechnol. 166, 1–12. doi: 10.1007/s12010-011-9397-6
Baffi, M. A., Tobal, T., Lago, J. H. G., Boscolo, M., Gomes, E., and Da-Silva, R. (2013). Wine aroma improvement using a β-Glucosidase preparation from Aureobasidium pullulans. Appl. Biochem. Biotechnol. 169, 493–501. doi: 10.1007/s12010-012-9991-2
Barghini, P., Di Gioia, D., Fava, F., and Ruzzi, M. (2007). Vanillin production using metabolically engineered Escherichia coli under non-growing conditions. Microb. Cell Fact. 6:13. doi: 10.1186/1475-2859-6-13
Baroi, G. N., Baumann, I., Westermann, P., and Gavala, H. N. (2015). Butyric acid fermentation from pretreated and hydrolysed wheat straw by an adapted Clostridium tyrobutyricum strain. Microb. Biotechnol. 8, 874–882. doi: 10.1111/1751-7915.12304
Berger, R. G. (2009). Biotechnology of flavours—the next generation. Biotechnol. Lett. 31, 1651–1659. doi: 10.1007/s10529-009-0083-5
Besson, I., Creuly, C., Gros, J. B., and Larroche, C. (1997). Pyrazine production by Bacillus subtilis in solid-state fermentation on soybeans. Appl. Microbiol. Biotechnol. 47, 489–495. doi: 10.1007/s002530050961
Bicas, J. L., Silva, J. C., Dionísio, A. P., and Pastore, G. M. (2010). Biotechnological production of bioflavors and functional sugars. Ciênc. Tecnol. Aliment. 30, 7–18. doi: 10.1590/S0101-20612010000100002
Bramorski, A., Christen, P., Ramirez, M., Soccol, C. R., and Revah, S. (1998a). Production of volatile compounds by the edible fungus Rhizopus oryzae during solid state cultivation on tropical agro-industrial substrates. Biotechnol. Lett. 20, 359–362. doi: 10.1023/A:1005323229125
Bramorski, A., Soccol, C. R., Christen, P., and Revah, S. (1998b). Fruity aroma production by ceratocystis fimbriata in solid cultures from agro-industrial wastes. Rev. Microbiol. 29, 208–212. doi: 10.1590/S0001-37141998000300012
Brault, G., Shareck, F., Hurtubise, Y., Lépine, F., and Doucet, N. (2014). Short-chain flavor ester synthesis in organic media by an E. coli whole-cell biocatalyst expressing a newly characterized heterologous lipase. PLoS ONE 9:e91872. doi: 10.1371/journal.pone.0091872
Carrau, F. M., Medina, K., Boido, E., Farina, L., Gaggero, C., Dellacassa, E., et al. (2005). De novo synthesis of monoterpenes by Saccharomyces cerevisiae wine yeasts. FEMS Microbiol. Lett. 243, 107–115. doi: 10.1016/j.femsle.2004.11.050
Cerda, A., Artola, A., Barrena, R., Font, X., Gea, T., and Sánchez, A. (2019). Innovative production of bioproducts from organic waste through solid-state fermentation. Front. Sustain. Food Syst. 3:63. doi: 10.3389/fsufs.2019.00063
Chandran, S. S., Kealey, J. T., and Reeves, C. D. (2011). Microbial production of isoprenoids. Process Biochem. 46, 1703–1710. doi: 10.1016/j.procbio.2011.05.012
Chatterjee, T. (2004). Biotransformation of geraniol by Rhodococcus sp. strain GR3. Biotechnol. Appl. Biochem. 39, 303–306. doi: 10.1042/BA20030136
Chattopadhyay, P., Banerjee, G., and Sen, S. K. (2018). Cleaner production of vanillin through biotransformation of ferulic acid esters from agroresidue by Streptomyces sannanensis. J. Clean. Prod. 182, 272–279. doi: 10.1016/j.jclepro.2018.02.043
Chen, P., Yan, L., Wu, Z., Li, S., Bai, Z., Yan, X., et al. (2016). A microbial transformation using Bacillus subtilis B7-S to produce natural vanillin from ferulic acid. Sci. Rep. 6:20400. doi: 10.1038/srep20400
Chen, Y., Bao, J., Kim, I. K., Siewers, V., and Nielsen, J. (2014). Coupled incremental precursor and co-factor supply improves 3-hydroxypropionic acid production in Saccharomyces cerevisiae. Metab. Eng. 22, 104–109. doi: 10.1016/j.ymben.2014.01.005
Christen, P., Bramorski, A., Revah, S., and Soccol, C. R. (2000). Characterization of volatile compounds produced by Rhizopus strains grown on agro-industrial solid wastes. Bioresour. Technol. 71, 211–215. doi: 10.1016/S0960-8524(99)00084-X
Christen, P., Meza, J. C., and Revah, S. (1997). Fruity aroma production in solid state fermentation by Ceratocystis fimbriata: influence of the substrate type and the presence of precursors. Mycol. Res. 101, 911–919. doi: 10.1017/S0953756297003535
Collin, R. P., and Halim, A. F. (1972). Characterization of the major aroma constituent of the fungus Trichoderma viride. J. Agric. Food Chem. 20, 47–48. doi: 10.1021/jf60180a010
Converti, A., Aliakbarian, B., Domínguez, J. M., Vázquez, G. B., and Perego, P. (2010). Microbial production of biovanillin. Braz. J. Microbiol. 41, 519–530. doi: 10.1590/S1517-83822010000300001
Damasceno, S., Cereda, M. P., Pastore, G. M., and Oliveira, J. G. (2003). Production of volatile compounds by Geotrichum fragrans using cassava wastewater as substrate. Process Biochem. 39, 411–414. doi: 10.1016/S0032-9592(03)00097-9
Damhus, T., Kaasgaard, S., and Olen, H. S., (eds.). (2013). Novozymes A/S Enzymes at Work. Bagsvaerd.
De Andrade, D. P., Carvalho, B. F., Schwan, R. F., and Dias, D. R. (2017). Production of γ-decalactone by yeast strains under different conditions. Food Technol. Biotechnol. 55, 225–230. doi: 10.17113/ftb.55.02.17.5009
De Yan, H., Li, Q., and Wang, Z. (2017). Efficient kinetic resolution of (±)-menthol by a lipase from Thermomyces lanuginosus: resolution of (±)-menthol by lipozyme TL IM. Biotechnol. Appl. Biochem. 64, 87–91. doi: 10.1002/bab.1457
Di Gioia, D., Sciubba, L., Setti, L., Luziatelli, F., Ruzzi, M., Zanichelli, D., et al. (2007). Production of biovanillin from wheat bran. Enzyme Microb. Technol. 41, 498–505. doi: 10.1016/j.enzmictec.2007.04.003
Dickschat, J. S., Wickel, S., Bolten, C. J., Nawrath, T., Schulz, S., and Wittmann, C. (2010). Pyrazine biosynthesis in Corynebacterium glutamicum. Eur. J. Org. Chem. 2010, 2687–2695. doi: 10.1002/ejoc.201000155
Engels, W. J. M., Dekker, R., de Jong, C., Neeter, R., and Visser, S. (1997). A comparative study of volatile compounds in the water-soluble fraction of various types of ripened cheese. Int. Dairy J. 7, 255–263. doi: 10.1016/S0958-6946(97)00003-4
Escamilla-Hurtado, M. L., Valdés-Martínez, S. E., Soriano-Santos, J., Gómez-Pliego, R., Verde-Calvo, J. R., Reyes-Dorantes, A., et al. (2005). Effect of culture conditions on production of butter flavor compounds by Pediococcus pentosaceus and Lactobacillus acidophilus in semisolid maize-based cultures. Int. J. Food Microbiol. 105, 305–316. doi: 10.1016/j.ijfoodmicro.2005.04.014
Esteban-Torres, M., Mancheño, J. M., de las Rivas, B., and Muñoz, R. (2014). Production and characterization of a tributyrin esterase from Lactobacillus plantarum suitable for cheese lipolysis. J. Dairy Sci. 97, 6737–6744. doi: 10.3168/jds.2014-8234
Fadel, H. H. M., Lotfy, S. N., Asker, M. M. S., Mahmoud, M. G., and Al-Okbi, S. Y. (2018). Nutty-like flavor production by Corynbacterium glutamicum 1220T from enzymatic soybean hydrolysate. effect of encapsulation and storage on the nutty flavoring quality. J. Adv. Res. 10, 31–38. doi: 10.1016/j.jare.2018.01.003
Fadel, H. H. M., Mahmoud, M. G., Asker, M. M. S., and Lotfy, S. N. (2015). Characterization and evaluation of coconut aroma produced by Trichoderma viride EMCC-107 in solid state fermentation on sugarcane bagasse. Electron. J. Biotechnol. 18, 5–9. doi: 10.1016/j.ejbt.2014.10.006
Faulds, C. B., Bartolomé, B., and Williamson, G. (1997). Novel biotransformations of agro-industrial cereal waste by ferulic acid esterases. Ind. Crops Prod. 6, 367–374. doi: 10.1016/S0926-6690(97)00027-7
Garlapati, V. K., and Banerjee, R. (2013). Solvent-free synthesis of flavour esters through immobilized lipase mediated transesterification. Enzyme Res. 2013:367410. doi: 10.1155/2013/367410
Gatfield, I. L. (1999). “Biotechnological production of natural flavor materials,” in Flavor Chemistry, Thirty Years of Progress, eds R. Teranishi, E. L. Wick, and I. Hornstein (New York, NY: Plenum Press), 211–227.
Groot, M. N. N. (1998). Conversion of phenylalanine to benzaldehyde initiated by an aminotransferase in Lactobacillus plantarum. Appl. Environ. Microbiol. 64, 3009–3013. doi: 10.1128/AEM.64.8.3009-3013.
Gueguen, Y., Chemardin, P., Janbon, G., Arnaud, A., and Galzy, P. (1996). A very efficient β-glucosidase catalyst for the hydrolysis of flavor precursors of wines and fruit juices. J. Agric. Food Chem. 44, 2336–2340. doi: 10.1021/jf950360j
Gunnarsson, N., and Almqvist, E. A. (2006). Influence of pH and carbon source on the production of vanillin from ferulic acid by Streptomyces setonii ATCC 39116. Dev. Food Sci. 43, 73–76. doi: 10.1016/S0167-4501(06)80018-X
Holck, J., Fredslund, F., Moller, M. S., Brask, J., Krogh, K. B. R. M., Lange, L., et al. (2019). A carbohydrate-binding family 48 module enables feruloyl esterase action on polymeric arabinoxylan. J. Biol. Chem. 294, 17339–17353. doi: 10.1074/jbc.RA119.009523
Ito, K., Yoshida, K., Ishikawa, T., and Kobayashi, S. (1990). Volatile compounds produced by the fungus Aspergillus oryzae in rice koji and their changes during cultivation. J. Ferment. Bioeng. 70, 169–172. doi: 10.1016/0922-338X(90)90178-Y
Janssens, L., pooter, H. L., Schamp, N. M., and Vandamme, E.J. (1992). Production of flavors by microorganisms. Process Biochem. 27, 195–215. doi: 10.1016/0032-9592(92)80020-4
Jensen, K. A., Evans, K. M. C., Kirk, T. K., and Hammel, K. E. (1994). Biosynthetic pathway for veratryl alcohol in the ligninolytic fungus Phanerochaete chrysosporium. Appl. Ennviron. Microbiol. 60, 709–714. doi: 10.1128/AEM.60.2.709-714.1994
Juturu, V., and Wu, J. C. (2016). Microbial production of lactic acid: the latest development. Crit. Rev. Biotechnol. 36, 967–977. doi: 10.3109/07388551.2015.1066305
Kapfer, G.-F., Berger, R. G., and Drawert, F. (1989). Production of 4-decanolide by semicontinuous fermentation of Tyromyces sambuceus. Biotechnol. Lett. 11, 561–566. doi: 10.1007/BF01040036
Karode, B., Patil, U., and Jobanputra, A. (2013). Biotransformation of low cost lignocellulosic substrates into vanillin by white rot fungus, Phanerochaete chrysosporium NCIM 1197. Ind. J. biotechnol. 12, 281–283.
Kaur, B., and Chakraborty, D. (2013). Statistical media and process optimization for biotransformation of rice bran to vanillin using Pediococcus acidilactici. Ind. J. Exp. Biol. 51, 935–943.
Kaur, B., Chakraborty, D., and Kumar, B. (2013). Phenolic biotransformations during conversion of ferulic acid to vanillin by lactic acid bacteria. BioMed Res Int. 2013:590359. doi: 10.1155/2013/590359
Kawabe, T., Takara, S. C., and Morita, H. (1994). Production of benzaldehyde and benzyl alcohol by the mushroom polyporus tuberaster K2606. J. Agric. Food Chem. 42, 2556–2560. doi: 10.1021/jf00047a034
Kim, T.-Y., Lee, S.-W., and Oh, M.-K. (2014). Biosynthesis of 2-phenylethanol from glucose with genetically engineered kluyveromyces marxianus. Enzyme Microb. Technol. 61–62, 44–47. doi: 10.1016/j.enzmictec.2014.04.011
King, A., and Dickinson, J. (2000). Biotransformation of monoterpene alcohols by Saccharomyces cerevisiae, Torulaspora delbrueckii and Kluyveromyces lactis. Yeast 16, 499–506. doi: 10.1002/(SICI)1097-0061(200004)16:6<499::AID-YEA548>3.0.CO;2-E
Kosuge, T., and Kamiya, H. (1962). Discovery of a pyrazine in a natural product : tetramethylpyrazine from cultures of a strain of bacillus subtilis. Nature 193, 776–776. doi: 10.1038/193776a0
Kuhad, R. C., Singh, A., and Eriksson, K.-E. L. (1997). “Microorganisms and enzymes involved in the degradation of plant fiber cell walls,” in Biotechnology in the Pulp and Paper Industry Advances in Biochemical Engineering/Biotechnology, eds K.-E. L. Eriksson, W. Babel, H. W. Blanch, C. L. Cooney, S.-O. Enfors, K.-E. L. Eriksson, A. Fiechter, A. M. Klibanov, B. Mattiasson, S. B. Primrose, H. J. Rehm, P. L. Rogers, H. Sahm, K. Schügerl, G. T. Tsao, K. Venkat, J. Villadsen, U. von Stockar, and C. Wandrey (Berlin; Heidelberg: Springer), 45–125. doi: 10.1007/BFb0102072
Kumar, N., and Pruthi, V. (2014). Potential applications of ferulic acid from natural sources. Biotechnol. Rep. 4, 86–93. doi: 10.1016/j.btre.2014.09.002
Lanza, E., and Palmer, J. K. (1977). Biosynthesis of monoterpenes by Ceratocystis moniliformis. Phytochem. 16, 1555–1560. doi: 10.1016/0031-9422(77)84022-3
Layton, D. S., and Trinh, C. T. (2016). Microbial synthesis of a branched-chain ester platform from organic waste carboxylates. Metab. Eng. Commun. 3, 245–251. doi: 10.1016/j.meteno.2016.08.001
Lesage-Meessen, L., Delattre, M., Haon, M., Thibault, J. F., Ceccaldi, B. C., Brunerie, P., et al. (1996). A two-step bioconversion process for vanillin production from ferulic acid combining Aspergillus niger and Pycnoporus cinnabarinus. J. Biotechnol. 50, 107–113. doi: 10.1016/0168-1656(96)01552-0
Liu, S.-Q., Holland, R., and Crow, V. L. (2004). Esters and their biosynthesis in fermented dairy products: a review. Int. Dairy J. 14, 923–945 doi: 10.1016/j.idairyj.2004.02.010
Lomascolo, A., Asther, M., Navarro, D., Antona, C., Delattre, M., and Lesage-Meessen, L. (2001). Shifting the biotransformation pathways of L-phenylalanine into benzaldehyde by Trametes suaveolens CBS 334.85 using HP20 resin. Lett. Appl. Microbiol. 32, 262–267. doi: 10.1046/j.1472-765X.2001.0873a.x
Lomascolo, A., Lesage-Meessen, L., Labat, M., Navarro, D., Delattre, M., and Asther, M. (1999). Enhanced benzaldehyde formation by a monokaryotic strain of pycnoporus cinnabarinus using a selective solid adsorbent in the culture medium. Can. J. Microbiol. 45, 653–657. doi: 10.1139/w99-056
Longo, M. A., and Sanromán, M. A. (2006). Production of food aroma compounds: microbial and enzymatic methodologies. Food Technol. Biotechnol. 44, 335–353.
Madhavan Nampoothiri, K., Nair, N. R., and John, R. P. (2010). An overview of the recent developments in polylactide (PLA) research. Bioresour. Technol. 101, 8493–8501. doi: 10.1016/j.biortech.2010.05.092
Mallouchos, A., Komaitis, M., Koutinas, A., and Kanellaki, M. (2003). Wine fermentations by immobilized and free cells at different temperatures. effect of immobilization and temperature on volatile by-products. Food Chem. 80, 109–113. doi: 10.1016/S0308-8146(02)00247-9
Mantzouridou, F. T., Paraskevopoulou, A., and Lalou, S. (2015). Yeast flavour production by solid state fermentation of orange peel waste. Biochem. Eng. J. 101, 1–8. doi: 10.1016/j.bej.2015.04.013
Marella, E. R., Dahlin, J., Dam, M. I., Ter Horst, J., Christensen, H. B., Sudarsan, S., et al. (2019). A single-host fermentation process for the production of flavor lactones from non-hydroxylated fatty acids. Metab. Eng. 9, S1096–S7176. doi: 10.1016/j.ymben.2019.08.009
Marshall, V. M. (1987). Lactic acid bacteria: starters for flavor. FEMS Microbiol. Rev. 46, 327–336. doi: 10.1111/j.1574-6968.1987.tb02469.x
Mathew, S., and Abraham, T. E. (2004). Ferulic acid: an antioxidant found naturally in plant cell walls and feruloyl esterases involved in its release and their applications. Crit. Rev. Biotechnol. 24, 59–83. doi: 10.1080/07388550490491467
Medeiros, A. B. P., Pandey, A., Christen, P., Fontoura, P. S. G., and Soccol, C. R. (2001). Aroma compounds produced by Kluyveromyces marxianus in solid state fermentation on a packed bed column bioreactor. World J. Microbiol. Biotechnol. 17, 767–771. doi: 10.1023/A:1013596330389
Meussen, B., Graaff, L., Sanders, J. M., and Weusthuis, R. (2012). Metabolic engineering of Rhizopus oryzae for the production of platformchemicals. Appl. Microbiol. Biotechnol. 94, 875–886. doi: 10.1007/s00253-012-4033-0
Molano, E. P. L., Cabrera, O. G., Jose, J., do Nascimento, L. C., Carazzolle, M. F., Teixeira, P. J. P. L., et al. (2018). Ceratocystis cacaofunesta genome analysis reveals a large expansion of extracellular phosphatidylinositol-specific phospholipase-C genes (PI-PLC). BMC Genomics 19:58. doi: 10.1186/s12864-018-4440-4
Moroe, T., Hattori, S., Komatsu, A., and Yamaguchi, Y. (1970). Method for the Biochemical Isolation of L-menthol. French patent 1, 598,150.
Motedayen, N., Ismail, M. B. T., and Nazarpour, F. (2013). Bioconversion of ferulic acid to vanillin by combined action of Aspergillus niger K8 and Phanerochaete crysosporium ATCC 24725. Afr. J. Biotechnol. 12, 6618–6624. doi: 10.5897/AJB2013.12416
Narbad, A., and Gasson, M. J. (1997). Metabolism of ferulic acid via vanillin using a novel CoA-dependent pathway in a newly- isolated strain of Pseudornonas fluorescens. Microbiology 144, 1397–1405. doi: 10.1099/00221287-144-5-1397
Norliza, A. W., and Ibrahim, C. O. (2005). The production of benzaldehyde by rhizopus oligosporus USM R1 in a solid state fermentation (SSF) system of soy bean meal: rice husks. Malaysian J. Microbiol. 1, 17–24. doi: 10.21161/mjm.120503
Omar, K. A., Gounga, M. E., Liu, R., Mlyuka, E., and Wang, X. (2016). Effects of microbial lipases on hydrolyzed milk fat at different time intervals in flavour development and oxidative stability. J. Food Sci. Technol. 53, 1035–1046. doi: 10.1007/s13197-015-2158-8
Pastore, G. M., Park, Y. K., and Min, D. B. (1994). Production of fruity aroma by neurospora from beiju. Mycol. Res. 98, 1300–1302. doi: 10.1016/S0953-7562(09)80302-X
Rajendran, A., Palanisamy, A., and Thangavelu, V. (2009). Lipase catalyzed ester synthesis for food processing industries. Braz. Arch. Biol. Technol. 52, 207–219. doi: 10.1590/S1516-89132009000100026
Razzaq, A., Shamsi, S., Ali, A., Ali, Q., Sajjad, M., Malik, A., et al. (2019). Microbial proteases applications. Front. Bioeng. Biotechnol. 7:110. doi: 10.3389/fbioe.2019.00110
Rosca, I., Petrovici, A. R., Brebu, M., Stoica, I., Minea, B., and Marangoci, N. (2016). An original method for producing acetaldehyde and diacetyl by yeast fermentation. Braz. J. Microbiol. 47, 949–954. doi: 10.1016/j.bjm.2016.07.005
Rossi, S. C., Vandenberghe, L. P. S., Pereira, B. M. P., Gago, F. D., Rizzolo, J. A., Pandey, A., et al. (2009). Improving fruity aroma production by fungi in SSF using citric pulp. Food Res. Int. 42, 484–486. doi: 10.1016/j.foodres.2009.01.016
Ruiz Rodriguez, L. G., Mohamed, F., Bleckwedel, J., Medina, R., Vuyst, L. D., Hebert, E. M., et al. (2019). Diversity and functional properties of lactic acid bacteria isolated from wild fruits and flowers present in Northern Argentina. Front. Microbiol. 10:1091. doi: 10.3389/fmicb.2019.01091
Saerens, S. M. G., Delvaux, F., Verstrepen, K. J., van Dijck, P., Thevelein, J. M., and Delvaux, F. R. (2008). Parameters affecting ethyl ester production by Saccharomyces cerevisiae during fermentation. Appl. Environ. Microbiol. 74, 454–461. doi: 10.1128/AEM.01616-07
Saerens, S. M. G., Delvaux, F. R., Verstrepen, K. J., and Thevelein, J. M. (2010). Production and biological function of volatile esters in Saccharomyces cerevisiae. Microb. Biotechnol. 3, 165–177. doi: 10.1111/j.1751-7915.2009.00106.x
Schreier, P. (2006). “Enzymes and flavour biotechnology,” in Biotechnology of Aroma Compounds, eds R. G. Berger, W. Babel, H. W. Blanch, Ch. L. Cooney, S.-O. Enfors, K.-E. L. Eriksson, A. Fiechter, A. M. Klibanov, B. Mattiasson, S. B. Primrose, H. J. Rehm, P. L. Rogers, H. Sahm, K. Schügerl, G. T. Tsao, K. Venkat, J. Villadsen, U. von Stockar, and C. Wandrey (Berlin; Heidelberg: Springer-Veflag), 51–72. doi: 10.1007/BFb0102062
Schwab, W., Davidovich-Rikanati, R., and Lewinsohn, E. (2008). Biosynthesis of plant-derived flavor compounds. Plant J. 54, 712–732. doi: 10.1111/j.1365-313X.2008.03446.x
Singhania, R. R., Patel, A. K., Soccol, C. R., and Pandey, A. (2009). Recent advances in solid-state fermentation. Biochem. Eng. J. 44, 13–18. doi: 10.1016/j.bej.2008.10.019
Soares, M., Christen, P., Pandey, A., and Soccol, C. R. (2000). Fruity flavour production by ceratocystis fimbriata grown on coffee husk in solid-state fermentation. Process Biochem. 35, 857–861. doi: 10.1016/S0032-9592(99)00144-2
Stark, D., Munch, T., Sonnleitner, B., Marison, I. W., and von Stockar, U. (2002). Extractive bioconversion of 2-phenylethanol from L-phenylalanine by Saccharomyces cerevisiae. Biotechnol. Prog. 18, 514–523. doi: 10.1021/bp020006n
Sugawara, E., Hashimoto, S., Sakurai, Y., and Kobayashi, A. (1994). Formation by yeast of the HEMF (4-hydroxy-2(or 5)-ethyl-5 (or 2) –methyl-3(2H)-furanone) aroma component in miso with aging. Biosci. Biotech. Biochem. 58, 1134–1135 doi: 10.1271/bbb.58.1134
Tan, M. C., Liew, S. L., Maskat, M. Y., Wan Aida, W. M., and Osman, H. (2015). Optimization of vanillin production using isoeugenol as substrate by Aspergillus niger I-1472. Int. Food Res. J. 22, 1651–1656.
Tilay, A., Bule, M., Kishenkumar, J., and Annapure, U. (2008). Preparation of ferulic acid from agricultural wastes: its improved extraction and purification. J. Agric. Food Chem. 56, 7644–7648. doi: 10.1021/jf801536t
Toogood, H. S., Cheallaigh, A. N., Tait, S., Mansell, D. J., Jervis, A., Lygidakis, A., et al. (2015). Enzymatic menthol production: one-pot approach using engineered Escherichia coli. ACS Synth. Biol. 4, 1112–1123. doi: 10.1021/acssynbio.5b00092
Topakas, E., Vafiadi, C., and Christakopoulos, P. (2007). Microbial production, characterization and applications of feruloyl esterases. Process Biochem. 42, 497–509. doi: 10.1016/j.procbio.2007.01.007
Torre, P., Aliakbarian, B., Rivas, B., Domínguez, J. M., and Converti, A. (2008). Release of ferulic acid from corn cobs by alkaline hydrolysis. Biochem. Engg. J. 40, 500–506. doi: 10.1016/j.bej.2008.02.005
Tsou, A. Y., Ransom, S. C., Gerlt, J. A., Buechter, D. D., Babbitt, P. C., and Kenyon, G. L. (1990). Mandelate pathway of Pseudomonas putida: sequence relationships involving mandelate racemase, (S)-mandelate dehydrogenase, and benzoylformate decarboxylase and expression of benzoylformate decarboxylase in Escherichia coli. Biochemistry 29, 9856–9862. doi: 10.1021/bi00494a015
Uppada, S. R., Akula, M., Bhattacharya, A., and Dutta, J. R. (2017). Immobilized lipase from Lactobacillus plantarum in meat degradation and synthesis of flavor esters. J. Genet. Eng. Biotechnol. 15, 331–334. doi: 10.1016/j.jgeb.2017.07.008
Uraji, M., Tamura, H., Mizohata, E., Arima, J., Wan, K., Ogawa, K., et al. (2018). Loop of streptomyces feruloyl esterase plays an important role in the enzyme's catalyzing the release of ferulic acid from biomass. Appl. Environ. Microbiol. 84:e02300–17. doi: 10.1128/AEM.02300-17
Vaithanomsat, P., and Apiwatanapiwat, W. (2009). Feasibility study on vanillin production from Jatropha curcas stem using steam explosion as a pretreatment. Int. J. Chem. Biol. Eng. 3, 258–261.
van Mastrigt, O., Tejeda, D. G., Kristensen, M. N., Abee, T., and Smid, E. D. (2018). Aroma formation during cheese ripening is best resembled by Lactococcus lactis retenostat cultures. Microb. Cell Fact. 17:104. doi: 10.1186/s12934-018-0950-7
Villena, M. A., Iranzo, J. F. Ú., and Pérez, A. I. B. (2007). β-Glucosidase activity in wine yeasts: application in enology. Enzyme Microb. Technol. 40, 420–425. doi: 10.1016/j.enzmictec.2006.07.013
Walsh, A. M., Crispie, F., Kilcawley, K., O'Sullivan, O., O'Sullivan, M. G., and Claesson, M. J. (2017), Microbial succession flavor production in the fermented dairy beverage kefir. Appl. Env. Sci. 1, e00052–e00016. doi: 10.1128/mSystems.00003-17.
Wang, D.-L., Nag, A., Lee, G.-C., and Shaw, J.-F. (2002). Factors affecting the resolution of dl-menthol by immobilized lipase-catalyzed esterification in organic solvent. J. Agric. Food Chem. 50, 262–265. doi: 10.1021/jf010657j
Welsh, F. W., Murray, W. D., Williams, R. E., and Katz, I. (1989). Microbiological and enzymatic production of flavor and fragrance chemicals. Crit. Rev. Biotechnol. 9, 105–169. doi: 10.3109/07388558909040617
Yan, L., Chen, P., Zhang, S., Li, S., Yan, X., Wang, N., et al. (2016). Biotransformation of ferulic acid to vanillin in packed bed-stirred fermentors. Sci. Rep. 6:34644. doi: 10.1038/srep34644
Yu, P., Maenz, D. D., McKinnon, J. J., Racz, V. J., and Christensen, D. A. (2002). Release of ferulic acid from oat hulls by Aspergillus ferulic acid esterase and Trichoderma xylanase. J. Agric. Food Chem. 50, 1625–1630. doi: 10.1021/jf010984r
Zamzuri, N. A., and Abd-Aziz, S. (2013). Biovanillin from agro wastes as an alternative food flavour: biovanillin from agro wastes as an alternative food flavour. J. Sci. Food Agric. 93, 429–438. doi: 10.1002/jsfa.5962
Zhang, X. M., Ai, N. S., Wang, J., Tong, L. J., Zheng, F. P., and Sun, B. G. (2016). Lipase-catalyzed modification of the flavor profiles in recombined skim milk products by enriching the volatile components. J. Dairy Sci. 99, 8665–8679. doi: 10.3168/jds.2015-10773
Zhao, T., Krokene, P., Björklund, N., Långström, B., Solheim, H., Christiansen, E., et al. (2010). The influence of Ceratocystis polonica inoculation and methyl jasmonate application on terpene chemistry of Norway spruce, Picea abies. Phytochemistry 71, 1332–1341. doi: 10.1016/j.phytochem.2010.05.017
Keywords: flavors, microbial biotransformation, biosynthesis lignocellulose, solid-state fermentation, waste valorization
Citation: Sharma A, Sharma P, Singh J, Singh S and Nain L (2020) Prospecting the Potential of Agroresidues as Substrate for Microbial Flavor Production. Front. Sustain. Food Syst. 4:18. doi: 10.3389/fsufs.2020.00018
Received: 03 November 2019; Accepted: 07 February 2020;
Published: 26 February 2020.
Edited by:
Zeynep Cetecioglu, Royal Institute of Technology, SwedenReviewed by:
Helen Treichel, Universidade Federal da Fronteira Sul, BrazilCopyright © 2020 Sharma, Sharma, Singh, Singh and Nain. This is an open-access article distributed under the terms of the Creative Commons Attribution License (CC BY). The use, distribution or reproduction in other forums is permitted, provided the original author(s) and the copyright owner(s) are credited and that the original publication in this journal is cited, in accordance with accepted academic practice. No use, distribution or reproduction is permitted which does not comply with these terms.
*Correspondence: Lata Nain, bGF0YXJhamF0QHlhaG9vLmNvLmlu
Disclaimer: All claims expressed in this article are solely those of the authors and do not necessarily represent those of their affiliated organizations, or those of the publisher, the editors and the reviewers. Any product that may be evaluated in this article or claim that may be made by its manufacturer is not guaranteed or endorsed by the publisher.
Research integrity at Frontiers
Learn more about the work of our research integrity team to safeguard the quality of each article we publish.