- 1Department of Ecology, Brandenburg University of Technology, Cottbus, Germany
- 2Department of Conservation Ecology and Entomology, Faculty of AgriSciences, Stellenbosch University, Stellenbosch, South Africa
- 3Department of Ecology, Swedish University of Agricultural Sciences, Uppsala, Sweden
- 4Department of Zoology and Entomology, University of the Free State, Bloemfontein, South Africa
- 5South African Sugarcane Research Institute, Durban, South Africa
- 6Iziko Museums of South Africa, Cape Town, South Africa
- 7Department of Physical Geography, Stockholm University, Stockholm, Sweden
- 8Nemlab, Klapmuts, South Africa
- 9Department of Plant Sciences, University of the Free State, Bloemfontein, South Africa
Organic orchards may have higher biodiversity and levels of ecosystem services compared to conventionally managed orchards. However, it is not well-understood how management decisions within organic orchards alter biotic communities and ecosystem services. The simultaneous provision of individual ecosystem services and mitigation of disservices is crucial for organic growers who cannot replace natural regulatory processes by artificial inputs. This study addresses one of the major constraints for organic fruit production in South Africa, namely the availability of strategies for pest control and nutrient management in soils. Partly due to these constraints, organic certification of deciduous fruits is very uncommon in South Africa and limited our selection of study plots. A field experiment on a single farm was established to study the impact of a treatment with dead organic mulch compared to controls on the composition of biotic communities, the simultaneous provision of ecosystem services, and the mitigation of disservices in five organic deciduous fruit orchards in the Western Cape province. Mulching did not significantly reduce weed cover or alter the taxonomic composition of weed communities, but affected soil organisms. Mulched subplots had significantly higher densities of Collembola and phytophagous nematodes and lower microbial activity and woodlice numbers. Independent of mulch treatment, both orchard type (conventional: apricot and organic: apricot, peach, plum, and quince) and weed cover had pronounced effects on the composition of biotic communities and ecosystem service and disservice potentials. The community composition of plants, microbes and web-building spiders differed significantly between organically and conventionally managed plots. The composition of communities and levels of ecosystem service and disservice potentials also differed significantly between organic orchards of different fruit type. Two potential pest groups (phytophagous nematodes and arthropods) were most abundant in peach subplots with high weed cover and tree age and least abundant in conventionally managed apricot plots. These results emphasize the crucial importance to consider weed-microbe-animal interactions when developing management practices in organic orchards. Management decisions in organic orchards hold the potential to affect biotic communities to the benefit of pest control and soil nutrient services, but can also result in unexpected detrimental effects on ecosystem services.
Introduction
The global demand for organically farmed products is continuously increasing (Willer and Lernoud, 2019) and organic farming practices may benefit several key ecosystem services (Birkhofer et al., 2016). Organically certified orchards may have higher microbial activity (Pokharel and Zimmerman, 2016), soil quality (Vogeler et al., 2006), pest predator (natural enemy) numbers (Happe et al., 2019), levels of biological control of pests (Porcel et al., 2018), and often overall higher biodiversity (Simon et al., 2011, but see Tuck et al., 2014) compared to conventional orchards (see also Samnegård et al., 2019). Pest infestation and damage, as ecosystem disservices, may also have higher levels under organic farming (Muneret et al., 2018).
However, it is less well-understood how management differences within organic orchards alter the composition of biotic communities and levels of associated ecosystem services. This limitation is particularly evident for the simultaneous management of biodiversity and multiple services and disservices (Birkhofer et al., 2015; Demestihas et al., 2017). Organic growers have to rely on the provision of multiple ecosystem services, as they cannot replace natural regulatory processes by artificial inputs (e.g., replacing pest control by natural enemies by insecticide application, Zehnder et al., 2007). The provision of ecosystem services and mitigation of disservices in organic production systems can be actively supported by management practices (Marliac et al., 2015, 2016), but it remains largely unknown to what extent individual orchard management practices affect the relationships between community composition and ecosystem services (Birkhofer et al., 2018).
South Africa is the third most productive fruit cultivating country in Africa (6.9 Mt per annum) with temperate fruit orchards covering an area of 297,636 ha (2017 data: FAOSTAT 2019). The country is among Africa's largest organic producers (41,377 ha organically managed land in 2017: Willer and Lernoud, 2019). However, organically certified temperate fruits are currently only cultivated in a small area of ~77 ha, representing <0.2% of all organically farmed land in South Africa (Willer and Lernoud, 2019). Naturally, the rarity of organic temperate fruit cultivation also limited our selection of field sites to five different fruit orchards on a single farm.
This study addresses one of the major constraints for organic fruit production, namely the availability of strategies for pest control and nutrient management in soils (Wyss et al., 2005; Peck et al., 2006; Wooldridge et al., 2013; Hammermeister, 2016). Several above- (e.g., biological control) and belowground (e.g., nutrient mineralization, soil structure) services and disservices (e.g., pest infestation) affect fruit production (Clothier et al., 2013). Fruit trees, for example, suffer from competition with weeds for nutrients and water. This issue is particularly relevant in regions such as the Western Cape Province in South Africa, which recently experienced severe drought periods (e.g., Baudoin et al., 2017). Ground cover management aims to reduce competition for soil nutrients and water between weeds and fruit trees (Atucha et al., 2011; Andersen et al., 2013), and alters the composition of weed communities in South African orchards (Fourie et al., 2011). These practices, may thereby further affect natural enemy and pest populations (Mathews et al., 2002; Bostanian et al., 2004; Markó et al., 2013). A range of natural enemy groups contribute to below- and aboveground biological control in fruit orchards (Blommers, 1994; Mody et al., 2011). Habitat management to benefit natural enemies (“conservation biological control,” Barbosa, 1998) is therefore a key natural regulatory process in organic farming systems (Diekötter et al., 2010, 2016).
Soil communities and soil ecosystem services are also affected by organic farming practices and the resulting weed communities (Yao et al., 2005; St. Laurent et al., 2008; Andersen et al., 2013; Williams and Hedlund, 2013; Pokharel et al., 2015). Organic growers often manage nutrient levels by promoting nutrient cycles and mineralization processes that are affected by the composition of soil biota and weed cover (de Vries et al., 2013). Soil biota also responds to the composition of weed plant communities, with distinct communities and levels of belowground ecosystem services observed in association with different plant species (Bezemer et al., 2010; Simon et al., 2011; Latz et al., 2015). Effects of ground cover management on the taxonomic composition of weed communities may therefore simultaneously alter the relationships between other taxonomic groups and multiple ecosystem services and disservices (Lavorel and Grigulis, 2012).
Here we aim to understand the impact of two ground cover management practices on the composition of several taxonomic groups, the simultaneous provision of ecosystem services, and the mitigation of disservices in organic fruit orchards in South Africa. We further analyse how weeds can be managed to mitigate trade-offs and to maximize synergies between services (“multifunctionality of production systems”; Hector and Bagchi, 2007; Birkhofer et al., 2018). We hypothesize that a treatment with dead organic mulch compared to unmulched control plots: (1) reduces weed cover and simplifies weed communities significantly, (2) promotes densities of beneficial soil biota and levels of soil ecosystem services, and (3) reduces densities of below- and aboveground pests. Conversely and trading off with these benefits, mulch treatment may (4) reduce the density of natural enemies, as well as aboveground biological control services. Ultimately, an improved understanding of the practices that affect relationships between community composition and multiple ecosystem services and disservices will help to improve multifunctionality in organic fruit production systems in South Africa.
Materials and Methods
Study Sites and Treatments
At Tierhoek Organic Farm, in the Breede river Valley, Robertson, South Africa (−33.729, 19.793) ~24–30 ha land is under cultivation and certified as organic since 2005 (total farm size = 180 ha). The grower cultivates apricot (6 ha), plum (2 ha), quince (1.5 ha), and peach (1.4 ha). Most fruits are used for drying or canning, but apricots and plums are also packed and sold as fresh fruits. All orchard plots are irrigated throughout summer on demand with drippers (apricot, plum, and quince) or micro-jets (peach). Ground cover is managed in all orchards by mechanically cutting weeds under tree canopies 4–5 times a year, while working rows are only cut 1–2 times a year. The grower applies compost and certified organic fertilizer (chicken manure and liquid guano). Eight organic orchard plots with each of the four cultivated temperate fruits were selected on the farm (two plots of each: apricot, peach, plum, and quince, Table 1). These fruits share several economically important pests (e.g., armored scale insect, fruit fly, and leaf roller species, Prinsloo and Uys, 2015) and suffer from the same competition for nutrients and water with weeds in organic orchards. As being part of the temperate fruit group (family Rosaceae) they all share important traits (general growth form as shrubs or small trees). In addition to the eight selected organic plots, we included two conventional orchard plots that were treated with pesticides and synthetic fertilizers on a neighboring farm (−33.758, 19.777). In each organic orchard plot we established one control and one mulched 20 × 20 m subplot, resulting in 18 study subplots [8 organic orchard plots × 2 subplots plus 2 conventional subplots (only control)]. The very limited availability of organically certified orchards in the Western Cape did not allow for a selection of one temperate fruit type in a well replicated organic vs. conventional design. The two conventional apricot plots were selected as the only temperate fruit types cultivated in a conventional orchard nearby Tierhoek Organic Farm.
The ground cover treatments that were established in the eight organic orchard plots were (a) “business as usual,” with 4–5 cuts under the canopy and 1–2 cuts in the working row and no removal of cut material (control) vs. (b) “mow and blow,” with 4–5 cuts under the canopy and 1–2 cuts in the working row with the cut material added under tree canopies as dead organic mulch (Figure 1; Hammermeister, 2016). All mowing was performed mechanically; placement of mulch was performed manually. The treatments were established in October 2016. On average, mulch covered an area of ~1.2 m2 below the canopy at a height of 24 cm after treatment establishment and an area of 0.7 m2 with an average height of 9 cm at the time of the second sampling in June 2017. Generally, dead organic mulches should be at least 10 cm thick to supress weeds (Lanini et al., 2011), so that a replacement or addition would be necessary after 8 months in the studied orchards.
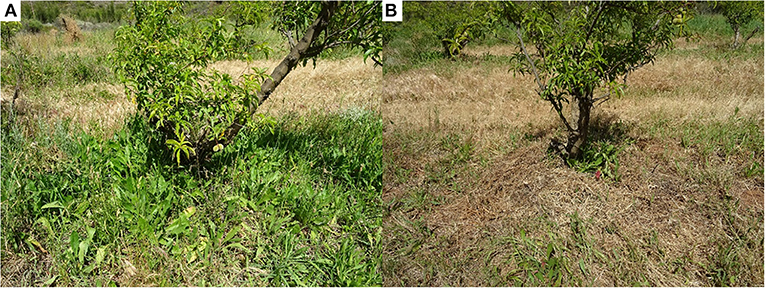
Figure 1. Ground cover treatments with (A) control without added mulch under the canopy and (B) treatment with mulch from cutting the working rows added under the canopy to supress weeds.
Sampling
The 10 orchard plots were sampled in November/December 2016 (before harvest) and in June/July 2017 (after harvest) for taxonomic composition (Table 2A) and ecosystem service (Table 2B) or disservice (Table 2C) potentials. Sampling focused on the impact area of mulch treatments under the tree canopy and the fruit tree rhizosphere, if not stated differently. Samples for microbial measures, soil nutrients and nematodes were derived from joint soil sampling and identical bulk soil samples. Soil nutrients (total available nitrogen and phosphorous in ppm) were analyzed from samples taken under the canopy of fruit trees in all mulched and control study plots in November/December 2016 and were analyzed by Ward Laboratories (Kearney, Nebraska, USA) in association with Soil Health Solutions (Bellville, South Africa).
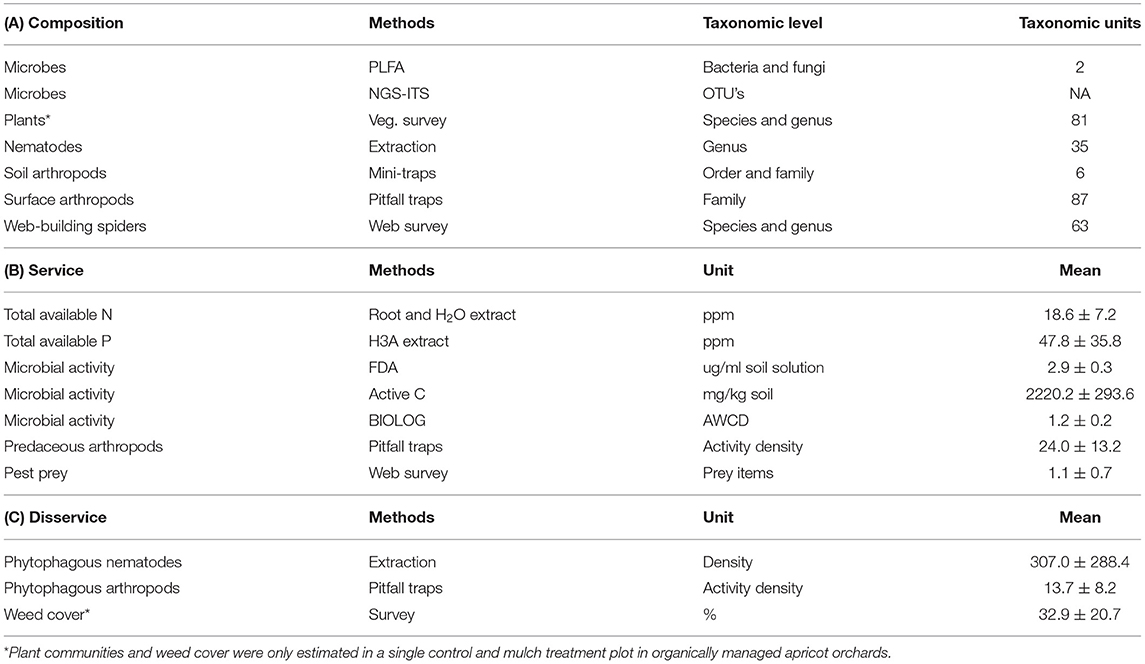
Table 2. Summary of (A) community composition, (B) ecosystem service, and (C) disservice potentials quantified in organically (apricot, peach, plum, and quince) and conventionally (apricot) managed orchards with the respective methods, taxonomic level (community composition) or unit of measure (service and disservice) and number of taxa or mean and standard deviation.
Microbial composition was analyzed based on Phospholipid fatty acid (PLFA) and Next Generation Sequencing of the Internal Transcribed Spacer region (NGS-ITS) analyses. Phospholipid fatty acids are components of the membranes of all organisms, and different organism groups have characteristic fatty acid patterns. To obtain fatty acid profiles, fatty acids were extracted from soil and the biomass of groups such as bacteria and fungi, and could be estimated by determining the concentration of biomarker fatty acids. For high-throughput deep amplicon sequencing of the Internal Transcribed Spacer (NGS-ITS) region, DNA was extracted in duplicate from soil samples using a NucleoSpin® Soil kit (Macherey-Nagel, Separations, Randburg, South Africa). The PCR products of each soil sample were combined for downstream analyses at the Next Generation Sequencing Facility at the University of the Free State, following the Illumina MiSeq ITS metagenomics sequencing library preparation guide (Illumina MiSeq, http://support.illumina.com). Quality of sequences were assessed using FastQC (Andrews, 2010) and sequences were trimmed and filtered using PrinSeq-lite v0.20.4 (Schweitzer et al., 2011) to have a mean quality score (QC) > 20 and sequence length > 200 bp. Paired end reads were merged using PEAR 0.9.6 (Zhang, 2014). QIIME 1.9.1 framework was used for subsequent sequence data analyses (Caporaso et al., 2010). A Closed-reference OTU's picking workflow was followed. Operational Taxonomic Units were picked against the Greengenes database (version gg_13_8_otus) at 97% sequence identity. Chimeric sequences were identified, using usearch 6.1.544 (Edgar, 2010) against the RDP “Gold” database (Edgar, 2010). Operational Taxonomic Units tables were normalized and cluster analyses was performed using XLSTAT (Addinsoft). For the analysis of microbial composition, PLFA concentrations of biomarker fatty acids for bacteria and fungi and the Shannon diversity from NGS-ITS data were jointly analyzed based on Gower distances (internally standardized for different measurement scales in the three variables).
Microbial activity was quantified based on the analysis of fluorescein-diacetate (FDA), active C and Biolog EcoPlate® assays. Fluorescein diacetate (FDA) hydrolysis was determined according to the method of Zabaloy et al. (2008), with minor modifications. The rate of FDA hydrolysis in soil is a suitable index of overall soil enzyme activity. Bacteria and fungi both produce extra-cellular enzymes to decompose organic matter, and the amount of enzymes in a sample is indicative of the presence, and viability, of the microbial biomass. The active C procedure measures the fraction of organic matter that is readily utilizable as an energy source by microorganisms. It measures the fraction of C and nutrients in total % organic matter (%OM) that is biologically available for soil organisms and plants. Community-level physiological profiles (CLPP), based on carbon source utilization, was determined using Biolog EcoPlates® (Biolog, Hayward, CA, United States of America). The Biolog EcoPlate® assay allows testing of a number of ecologically relevant C substrates in soil in order to differentiate between microbial communities present that are able to utilize these substrates. Communities of organisms will give a characteristic reaction pattern called a metabolic fingerprint. The overall color development was expressed as the average well color development (AWCD) for all C sources (Zak et al., 1994) in order to provide a single value of the substrate utilization activity by the microbial community. For the analysis of microbial activity, FDA, active C, and Biolog EcoPlates® assay data were jointly analyzed based on Gower distances (internally standardized for different measurement scales in the three variables).
For all analyses of biodiversity responses, multivariate taxonomic composition data was used. Taxonomic composition is an important dimension of diversity and its analysis allows to identify taxa that are sensitive to the factors in our study design. Vegetation was sampled and identified between 4 and 5 December 2017 in one 1 m2 quadrat adjacent to the tree trunk (always southwards) in each subplot and in one 1 m2 quadrat 1 m away from the tree trunk. All species were recorded from these quadrats based on estimates of their coverage along a percentage scale and total weed cover was also estimated. However, in organically managed apricot plots, only one treatment and one control subplot were sampled for plants due to logistical problems with one apricot plot. For the analysis of plant community composition, frequencies of all individual species and genera were analyzed as compositional data based on Bray-Curtis similarities. The weed coverage in each subplot was analyzed separately based on Euclidean distances.
In each subplot, 300 g rhizosphere soil was collected for microbial analyses from each of five trees per subplot and placed in plastic bags on ice packs to transfer it back to the laboratory. For the analytical procedures to study microbial communities, sub-samples were made from the original samples by combining the five samples in each subplot. Soil samples for nematode extraction were collected from the root zone of five randomly selected trees in each subplot to a depth of 30 cm close to the trunk of the tree. These subsamples were transported back to the laboratory where they were thoroughly mixed to comprise one sample per subplot. Nematodes were extracted from the soil samples by means of the Cobb's decanting and sieving method, in combination with a modified Baermann funnel. The extracted nematodes were counted and identified to genus level. The nematodes were then categorized into feeding groups.
Soil arthropod communities were sampled using small (diameter 15 mm, containing 10 ml ethyl glycol) and large pitfall traps (diameter 55 mm, containing 100 ml ethyl glycol), placed no further than 30 cm from the tree trunks, so that they were placed below the mulch, where applicable. Three small pitfalls and three large pitfalls were placed into each subplot, resulting in a total of 54 × 2 pitfalls altogether. Sampling took place on 13 December 2016 and on 17 June 2017, with pitfalls left in the field for a period of 14 days. Samples were brought back to the laboratory and sorted into 70% ethanol and identified to family level.
Web-building spiders and their prey were hand collected from all subplots for 90 min in the morning and for a second period of 90 min in the afternoon between 01 and 28 November 2016 and on a second date between 12 June and 08 July 2017. All spiders and prey items were transported to the University of the Free State in Bloemfontein for identification.
Statistical Analyses
All data were averaged over the two sampling dates and analyzed for the factors orchard type (levels: conventional apricot and organically managed apricot, peach, plum, and quince plots) and treatment (levels: control and mulched subplots) in two-factorial permutational analyses of variance (PERMANOVA: providing F- and P-values; Anderson, 2001). The conventional apricot plots lacked the mulch treatment level. The two-way interaction between both factors was included in all models. After obtaining a significant model term for the factor orchard plot or the interaction term, post-hoc tests (pairwise PERMANOVA: providing t- and P-values) were used to identify significant differences between different levels of the term. All PERMANOVA analyses were performed based on sums of squares type 3, permutation of residuals under a reduced model and 9999 permutations. In pairwise PERMANOVA the number of unique permutations was considerably lower than 9999, and therefore P-values were derived from Monte-Carlo permutations (Anderson, 2014). Pairwise relationships between taxonomic compositions and ecosystem service or disservice potentials were calculated based on rank-based Mantel tests relating pairs of resemblance matrices and deriving Spearman (rs) correlation coefficients for these matrix correlations.
Results
Community Composition
In general, mulching had small effects on community composition of the studied groups (Table 3), while orchard type and farming system (conventional vs. organic) affected the community composition in several organism groups (Table 3; Figure 2).
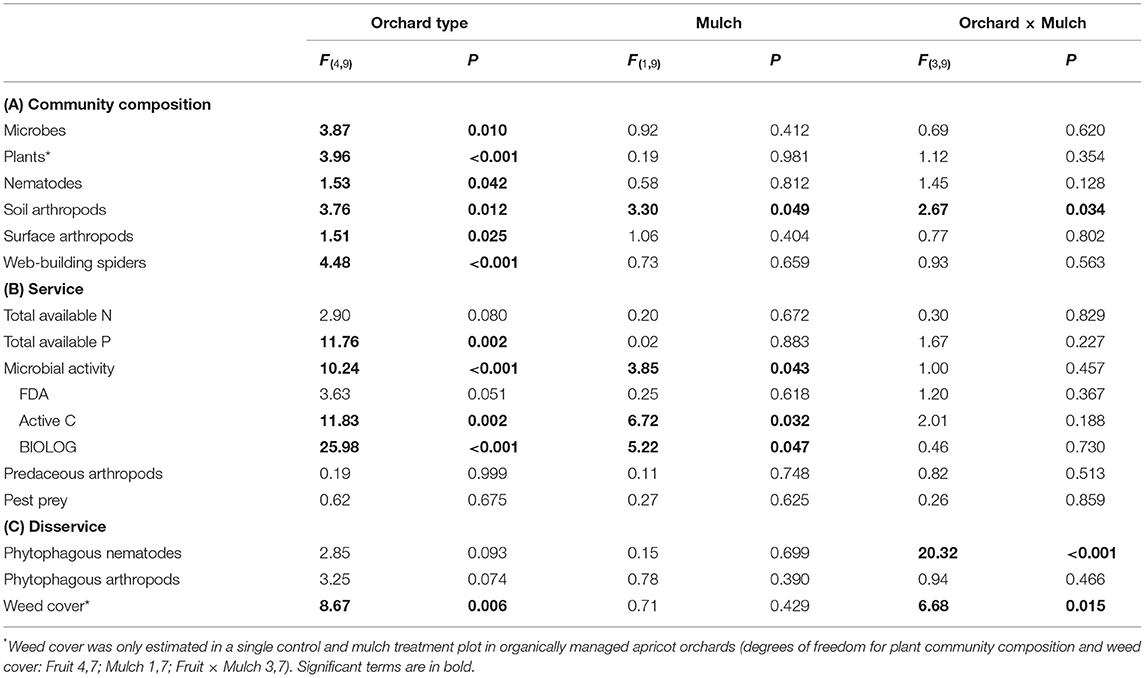
Table 3. Effects of Orchard type and mulch treatment on (A) community composition (taxonomic composition), (B) ecosystem service, and (C) ecosystem disservice potentials in organically (apricot, peach, plum, and quince) and conventionally (apricot) managed orchards.
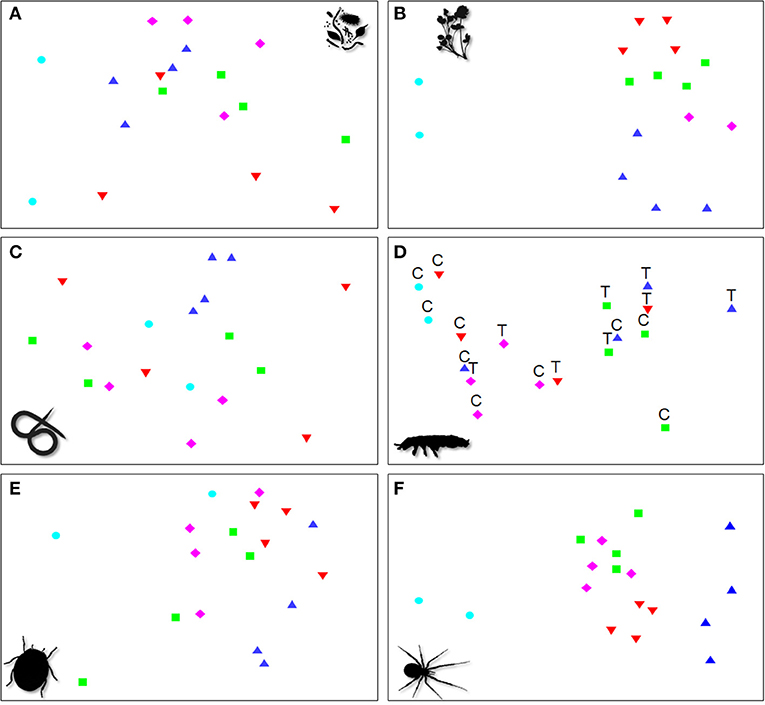
Figure 2. Non-metric multidimensional scaling ordinations based on the multivariate community composition of (A) microbial (2-d stress: 0.05), (B) plant (0.12), (C) nematode (0.18), (D) soil arthropod (0.07), (E) surface-active arthropod (0.19), and (F) web-building spider (0.13) communities in conventional apricot () and organically managed apricot (
), peach (
), plum (
), and quince (
) plots. Symbols and labels are given for significant factors as shown in Table 3, with treatment (T) and control (C) shown in (D) due to the significant interaction term.
The composition of microbial, plant, nematode, surface-active arthropod and web-building spider communities differed significantly between orchard types (Table 3A). Microbial communities in plum differed significantly from quince (t = 2.33, P = 0.049) and conventionally managed apricot (t = 3.62, P = 0.018; Figure 2A) plots due to 1.3 and 8.2 times higher concentration of bacterial PLFAs in plum plots.
The composition of plant communities differed significantly between conventionally managed apricot and peach (t = 2.43, P = 0.023) or plum (t = 2.66, P = 0.022) plots and between quince and peach (t = 2.16, P = 0.027) or plum (t = 1.97, P = 0.038; Figure 2B) plots. The invasive weed species Plantago lanceolata was absent from conventionally managed apricot, had low frequencies in quince and was abundant in peach and plum plots. In general, orchard plots showed a high susceptibility to invasive weed species, as more than 50% of all recorded weed species were invasive.
Nematode communities differed significantly between organically managed quince and apricot (t = 1.84, P = 0.049) or plum (t = 1.93, P = 0.042; Figure 2C) plots. Nematodes from the genus Scutellonema were absent from plum, but abundant in quince plots and nematodes from the genus Coslenchus were 5.5 times more abundant in quince than in organically managed apricot plots.
The community composition of surface-active arthropods differed significantly between peach and quince plots (t = 1.57, P = 0.038; Figure 2E) due to Collembola of the family Brachystomellidae and ants (Formicidae) being 1.8 and 1.4 times more abundant in quince plots.
The composition of web-building spider communities differed significantly between conventionally managed apricot and organically managed apricot (t = 2.52, P = 0.025), peach (t = 3.33, P = 0.013), plum (t = 2.52, P = 0.027), and quince (t = 2.42, P = 0.031) plots. The composition also differed significantly between organically managed apricot and quince (t = 1.94, P = 0.040) and between peach and plum (t = 2.04, P = 0.033; Figure 2F) plots. The conventionally managed apricot plots were characterized by the absence of spiders from the genera Tidarren and Gandanameno, as well as the species Larinia bifida and Crozetulus scutatus, which were always present in organically managed plots (with the exception of Tidarren not being present in plum).
Mulching had no significant effects on the studied groups, with the exception of the composition of soil arthropods which differed significantly between mulch treatments. Woodlice (Fam. Clyisticidae) were more abundant (3.1 times) in control subplots and Collembola (Fam. Poduridae) were more abundant (2.7 times) in mulched subplots. The effect of mulching on soil arthropods further depended on orchard type (Table 3A interaction term “orchard type × mulch”). Furthermore, microbial activity was slightly larger in control than in mulched plots (Table 3).
In addition, the composition of soil arthropod communities differed significantly between conventionally managed apricot and organically managed control apricot plots (t = 3.50, P = 0.043) or plum plots (t = 3.48, P = 0.037; Figure 2D). Collembola of the family Entomobryidae were more abundant in the organically managed apricot (1.8 times) and plum (5.7 times) than in the conventionally managed apricot plots. The composition of soil arthropod communities did not differ significantly between different orchard types in mulched plots (all P > 0.05).
Ecosystem Service Potentials
Several soil ecosystem services differed both between orchard types, while we found no significant differences in neither the abundance of predaceous arthropods nor in pest prey abundances (Tables 3B, 4). Total available P and microbial activity differed significantly between orchard types (Table 3B). Total available P differed significantly between conventionally managed apricot and organically managed peach (t = 4.02, P = 0.027) and plum (t = 3.99, P = 0.028; Table 4A). Total available P also differed significantly between organically managed quince and peach (t = 5.58, P = 0.005), plum (t = 6.68, P = 0.003), and apricot (t = 4.69, P = 0.009). Active C [F(4, 17) = 11.83; P = 0.002] and community–level physiological profiles (CLPP) [F(4, 17) = 25.98; P = < 0.001] both differed significantly between orchards (Table 4A). Active C differed significantly between organically managed apricot and peach (t = 6.68, P = 0.003) or plum (t = 6.68, P = 0.003) plots. Active C also differed significantly between organically managed quince and peach (t = 5.63, P = 0.005) or plum (t = 6.34, P = 0.004) plots. CLPP's differed significantly between quince and conventionally managed apricot (t = 4.42, P = 0.023) or organically managed apricot (t = 4.19, P = 0.015), peach (t = 6.51, P = 0.003) or plum (t = 7.67, P = 0.001) plots. CLPP's also differed significantly between plum and peach (t = 3.51, P = 0.024) or apricot (t = 3.72, P = 0.020) plots. Active C (t = 2.59, P = 0.033) and physiological profiles (t = 2.29, P = 0.046) were significantly higher in control compared to mulched plots, independent of orchard type (Tables 3B, 4B).
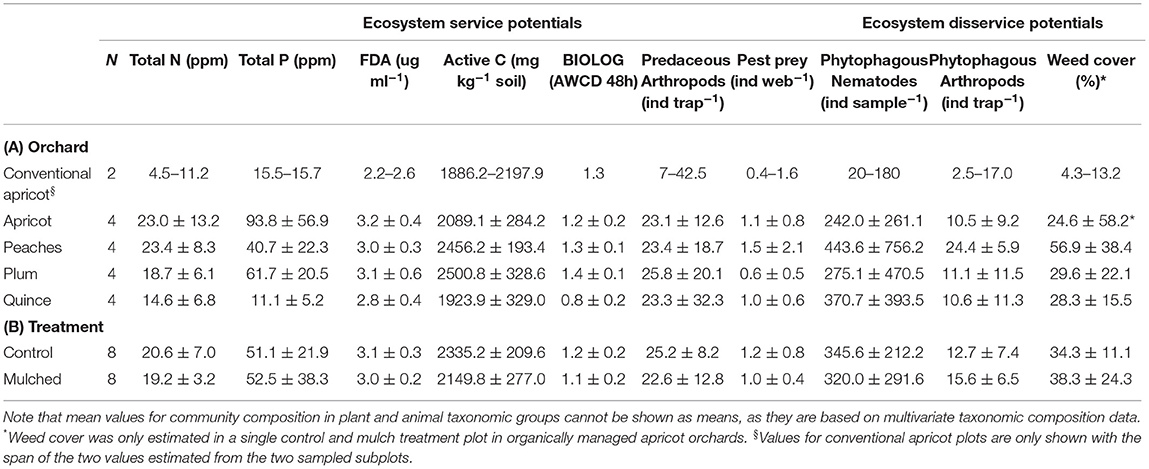
Table 4. Mean values of ecosystem service [Total N, Total P, microbial activity (FDA, Active C and BIOLOG), predaceous arthropods, and pest prey] and disservice (phytophagous Nematodes, phytophagous Arthropods, and weed cover) potentials ±95% confidence intervals of the mean in (A) different orchards and (B) control or mulched subplots.
Ecosystem Disservice Potentials
The density of plant feeding nematodes differed significantly between control and mulched plots, but depended on orchard type (Table 3C). Their abundance was clearly lower in the conventional plots than in the organic ones (Table 4). Mulched plots in peach had higher densities of plant feeding nematodes than control plots (t = 7.10, P = 0.020; Table 4B). In plum (t = 4.67, P = 0.040) and quince (t = 4.77, P = 0.043), control plots had higher densities of plant feeding nematodes than mulched plots.
The density of phytophagous arthropods was not significantly affected by orchard type or treatment (Table 3C). Weed cover differed significantly between orchard types, depending on mulch treatment (Tables 3C, 4), while neither orchard type nor treatments affect the number of pest prey in spider webs (Tables 3B, 4), except that it tended to be lower in plum plots.
Weed cover did not differ significantly between control plots in the different orchards. Weed cover in mulched plots differed significantly between peach and apricot (t = 43.14, P = 0.014) or plum (t = 89.63, P < 0.001), between apricot and plum (t = 46.35, P = 0.014), and between peach and quince (t = 6.84, P = 0.019) plots (Table 4B).
Relationships
Among all analyzed taxonomic groups, plant (8 out of 13 relationships with P < 0.1) and microbial (5 out of 13) composition showed the strongest relationship to the composition of other taxonomic groups or ecosystem service and disservice potentials (Figure 3). Orchard plots that resembled each other in plant community composition had similar web-building spider communities, comparable levels of microbial activity and total N and P contents in soils. Orchard plots resembling each other in microbial communities resembled each other in the number of pest prey items caught by web-building spiders, infestation with potential pest arthropods and weed cover. Weed cover was significantly related to the number of phytophagous nematodes (Figure 4A) and arthropods (Figure 4B). The composition of web-building spider communities was significantly related to the composition of four out of the five other taxonomic groups (excluding nematodes, Figure 3). Levels of microbial activity were significantly related to the composition of plant, soil arthropod and web-building spider communities and levels of total P (Figure 3).
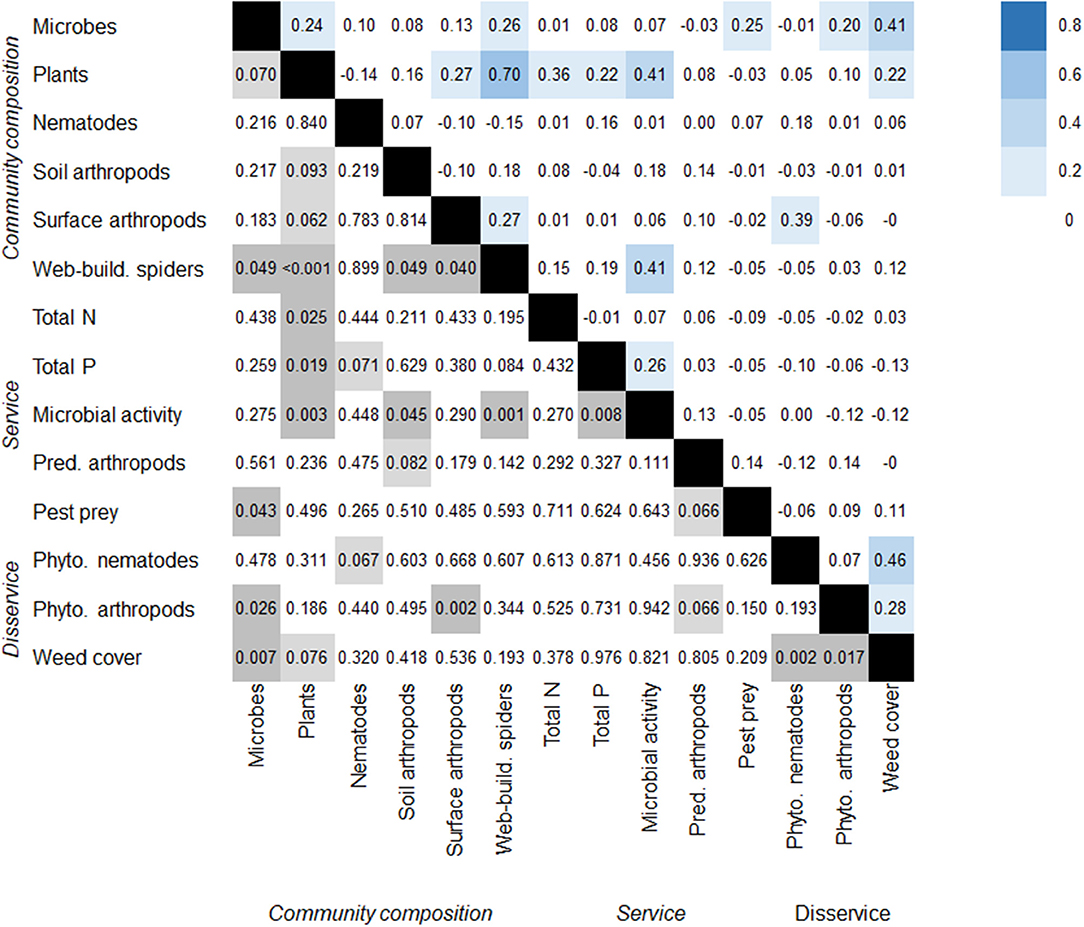
Figure 3. Correlation matrix between community composition and potentials for ecosystem services and disservices with the upper triangle giving Spearman correlation coefficients and the lower triangle providing the respective P-values from matrix correlations (Mantel tests based on Spearman correlation coefficients). Individual Spearman rs are significant at the p < 0.05 if rs > |0.40|, n = 18. Note that for plant community composition and weed cover N = 16 and rs are significant at the p < 0.05 if rs > |0.43|.
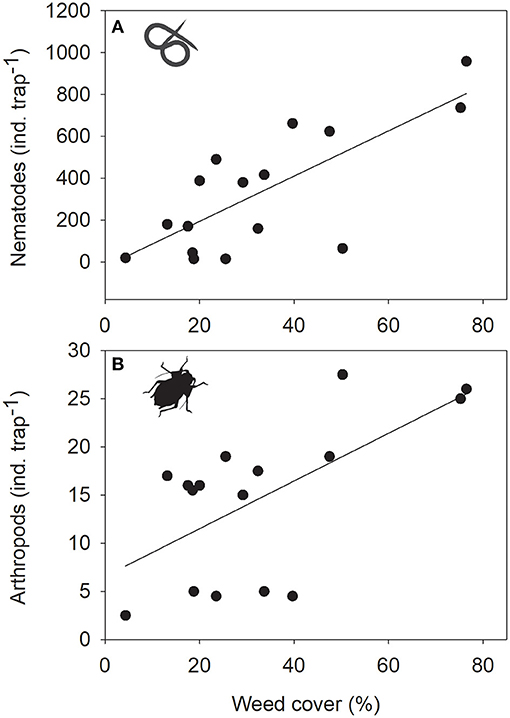
Figure 4. Relationship between weed cover and densities of phytophagous (A) nematodes and (B) arthropods across orchard plots. Note that N = 16 for weed cover.
Discussion
Mulching did not significantly reduce weed cover or alter the taxonomic composition of weed communities, but affected soil organisms by increasing densities of Collembola and phytophagous nematodes and by reducing microbial activity and woodlice numbers significantly even over the relatively short study period. These effects were observed within 8 months after subplot treatment with dead organic mulch and therefore document short-term responses. Independent of the mulch treatment, both orchard type and weed cover had pronounced effects on the composition of biotic communities and associated ecosystem service and disservice potentials.
Mulching
Mulching is considered an important management practice to conserve moisture in orchard soils, to buffer against climatic extremes and to reduce weed cover (for review see Bakshi et al., 2015). However, this beneficial effect on water conservation may be more pronounced in rain-fed orchards, as soil moisture can be, at an economic cost, controlled in irrigated orchards like in our study. A range of mulch materials can be used in organic agriculture to supress weeds, with dead organic mulches recommended for farms with fertile soils and for control of annual weeds (for review Hammermeister, 2016). Steinmaus et al. (2008) studied effects of dead organic mulch that was produced from cover crops in work rows and was then applied to plant rows. Their results indicate that if supplied in sufficient quantity, dead organic mulch may provide satisfactory levels of weed control in vineyards. In our study, weeds were not suppressed significantly by dead organic mulch and two differences between studies may explain the observed discrepancies. First, Steinmaus et al. (2008) studied effects over longer time periods, and repeated application of dead organic mulch in the South African orchards may have resulted in higher levels of weed control. However, mulch material from the work roads is scarce due to the harsh climatic conditions and additional application would result in higher costs. Second, our study was performed in times of a major drought period in the Western Cape Province. The extreme climatic conditions may have been the dominating constraint for weed growth in control and treatment plots independent of mulch application.
Ground cover management with mulch or compost affects detritivorous organisms in organically managed orchard systems if compared to herbicide-treated plots. Mathews et al. (2002) showed that orchard plots with compost had higher abundances of detritivores compared to conventionally-treated plots. A high percentage of bare ground was detrimental to ground-active detritivores in New Zealand orchards (Todd et al., 2016). This pattern may be even more pronounced in regions with a dryer climate like South Africa. The contrasting response of different detritivorous arthropods (Collembola and Isopoda) to mulching in the studied subplots may reflect both, differences in dietary and microclimatic preferences between taxonomic groups. Isopods can be a dominant soil macrofauna group in irrigated fruit orchards (Walmsley and Cerdà, 2017) and the negative numeric response to mulching may lower their contribution to decomposition processes in these subplots.
The abundance of predaceous arthropods can be negatively affected by mulching with straw compared to tillage or herbicide-treated orchard plots (Miñarro et al., 2009). In contrast to these previous studies, the studied organic orchard plots in South Africa always showed relatively high ground cover, either due to mulch or weed cover and due to the absence of herbicide applications. Plant-parasitic nematodes (Pratylenchus penetrans) in Canadian apple orchards were negatively affected by mulching of work rows with alfalfa hay compared to control plots that were treated with herbicides (Forge et al., 2003). In our study, phytophageous nematodes benefitted from the addition of dead organic mulch, but our control plots were not treated with herbicides. Studies with more appropriate replication and paired organic and conventional orchards of the same fruit type would address mulching effects in more detail, but are currently not possible in the Western Cape province.
Microbial activity in our study probably benefitted from the altered microclimate, as mulching increases the soil water content (Granatstein and Mullinix, 2008) and the water holding capacity of soils (Bakshi et al., 2015). Higher microbial activity in our study may result in higher levels of organic matter decomposition, as microbes are major contributors to these processes in orchards (Bubán et al., 2000). Neither N nor P levels differed between mulched and control plots, but the short-term nature of this study may have contributed to this limited build-up of nutrient levels in mulched plots. This may equally hold true for additional soil properties that were not considered in this study, like soil organic C levels, which may need up to 8 years to change significantly after conversion from conventional to alternative orchard management practices (Montanaro et al., 2017).
Orchard Type
Weed cover in conventionally managed orange and persimmon orchards with drip irrigation differed markedly in previous studies (75 vs. 93%; Walmsley and Cerdà, 2017). The authors explained the observed differences by tree age (15 vs. 40+ years). In our study, organic orchards differed in tree age between 10 and 26 years. Similar to Walmsley and Cerdà (2017), the oldest (peach) orchard had by far the highest weed coverage. Horak et al. (2013) compared the diversity of biotic communities between apple, cherry, pear, and plum orchards and deciduous forests and grasslands in Czech Republic, but the authors did not provide individual results of differences between orchard types. Differences in community composition, soil ecosystem services and weed cover between organically managed orchard types were among the most pronounced effects in this study. These results suggest that organic fruit growers need to be aware of the advantages and disadvantages between fruit types to maximize benefits from biodiversity and ecosystem services and mitigate ecosystem disservices.
Comparisons between conventional and organic plots in our study are hampered by the low number of conventional plots, and the fact that the comparison is between two different fruit farms, although fairly close to each other (3.5 km). Nevertheless, species composition of microbes, plants and web-building spiders were significantly different between the two conventional plots and the organic ones (Figure 2; the two left-hand blue dots in the NMDS analysis vs. the symbols to the right). Diversity, measured as species richness, is not generally higher in organic orchards compared to conventionally managed farms (see Figure 1 in Tuck et al., 2014). Our results suggest, that community composition may be more sensitive to differences between major farming systems, than more simple richness metrics.
Trade-Offs and Synergies
Ecosystem services can be positively (synergies) or negatively (trade-offs) related to each other (Birkhofer et al., 2015). Pest control services and soil fertility in orchards, for example, may be positively related due to simultaneously, but independently responding to ground cover management (Demestihas et al., 2017). Ecosystem services and biodiversity may show similar relationships, as for example Todd et al. (2016) suggested that management practices in orchards could increase invertebrate biodiversity and at the same time may improve ecosystem services. However, it is important to consider potential trade-offs, as pest populations may also benefit from these practices (Todd et al., 2016). Amongst the ecosystem services and disservices studied here, only 3 out of 28 potential pairwise relationships were significant (11% of all pairwise relationships). The only synergy was observed between total P content of soils and microbial activity. Regarding relationships between disservices, plots with higher weed cover had higher densities of phytophagous nematodes and arthropods. This result is partly driven by the observed low herbivore densities in the conventional orchard subplots with low weed cover and high herbivore numbers in peach orchards with very high weed cover. Our results highlight the importance to consider individual management practices and orchard types in studies that aim to generalize about trade-offs and synergies between ecosystem services.
For the relationships between community composition and ecosystem services or disservices, 9 out of 48 pairwise relationships were significant (19% of all pairwise relationships). Microbial and plant community composition were most frequently related to services amongst all studied taxonomic groups (both 3 out of 8 service and disservice potentials). Considering the significant relationships highlights issues in the interpretation of these statistical relationships. While weed community composition may reflect local nutrient levels and can be affected by microbial activity (Haynes, 1980; Yang et al., 2007), plant species also hold the potential to alter soil nutrient levels and the community composition of soil biota (Bezemer et al., 2010). The composition of microbial communities was significantly related to weed cover and the number of phytophagous arthropods in subplots. While it is probable that the first relationship (weed cover vs. microbial community composition) is based on functional links between weed and microbial taxa, the second relationship (phytophagous arthropods vs. microbial community composition) more likely results from simultaneous responses of both taxa to weed cover.
The only significant relationships between the composition of different taxonomic groups were present between web-building spider communities and four of the five other analyzed taxonomic groups. Web-building spiders strongly rely on structural complexity in their habitats (Diehl et al., 2013) and weeds alter the habitat to the advantage or disadvantage of individual species (Costello and Daane, 1998). Spiders have been previously reported to be valid indicators of biodiversity properties in other taxonomic groups in alpine habitats (Finch and Löffler, 2010), Brazilian Atlantic forests (Leal et al., 2010), or across terrestrial habitats in general (Gerlach et al., 2013). The observed significant relationships between the composition of spider and soil or surface-active arthropod communities may reflect specific habitat selection preferences of spider species due to the availability of preferred prey (Birkhofer et al., 2010; Jurczyk et al., 2012).
Conclusions
The composition of biotic communities and levels of associated ecosystem services and disservices are known to differ between conventionally and organically managed orchards or between young and old orchards. Our study highlights pronounced differences between organically managed orchards with comparable age and close proximity, but different temperate fruit species (e.g., Nematodes between quince and apricot, Figure 2C) and to a lesser extent effects of management decisions within organic orchard systems. Based on our results, we would not recommend mulching with dead organic matter in South African organic orchards as weed control was not effective in the short term and as higher weed cover may come at the risk of more serious pest infestation. However, it is important to consider that soil nutrients and certain ecosystem services like pest control by natural enemies probably require more time and repeated mulch application to build-up. In addition to the rather short term nature of our study over 8 months, the very limited availability of organic temperate fruit orchards naturally constrained the number of orchards and level of replication in this study.
Data Availability Statement
The raw data supporting the conclusions of this manuscript will be made available by the authors, without undue reservation, to any qualified researcher.
Author Contributions
KB, MA, CB, JB, DC, CJ-S, RL, SL, SS, WS, and PA conceived and designed the experiments. MA, FA, DC, CJ-S, CK, SL, AM, WS, and PA performed the experiments. KB, FA, CB, RB, CH, CJ-S, CK, SL, AM, SS, WS, and PA analyzed the data or samples. KB, JB, CH, SS, WS, and PA wrote the manuscript.
Funding
We thank the Ekhaga Foundation (Ansökan 2015-11) and Volkswagen Foundation (Az.: 94646) for the financial support that allowed us to perform this study and publish the results.
Conflict of Interest
SS is the founder of Nemlab.
The remaining authors declare that the research was conducted in the absence of any commercial or financial relationships that could be construed as a potential conflict of interest.
Acknowledgments
This publication is dedicated to the memory of the late SL at the University of the Free State. SL contributed invaluable expertise and knowledge to the project and was a wonderful colleague and friend. The authors are very grateful to the farm owners for their support, in particular the owner of Tierhoek organic Farm. Vegetation was sampled and identified by Suzaan Kritzinger-Klopper. We thank two referees for valuable comments that helped to improve this manuscript.
References
Andersen, L., Kühn, B. F., Bertelsen, M., Bruus, M., Larsen, S. E., and Strandberg, M. (2013). Alternatives to herbicides in an apple orchard, effects on yield, earthworms and plant diversity. Agric. Ecosyst. Environ. 172, 1–5. doi: 10.1016/j.agee.2013.04.004
Anderson, M. J. (2001). A new method for non-parametric multivariate analysis of variance. Austral. Ecol. 26, 32–46. doi: 10.1111/j.1442-9993.2001.01070.pp.x
Anderson, M. J. (2014). Permutational Multivariate Analysis of Variance (PERMANOVA). Wiley StatsRef: statistics reference online, 1–15. doi: 10.1002/9781118445112.stat07841
Andrews, S. (2010). FastQC: A Quality Control Tool for High Throughput Sequence Data. Available online at: http://www/bioinformatics.babraham.ac.uk/projects/fastqc
Atucha, A., Merwin, I. A., and Brown, M. G. (2011). Long-term effects of four groundcover management systems in an apple orchard. HortScience 46, 1176–1183. doi: 10.21273/HORTSCI.46.8.1176
Bakshi, P., Wali, V. K., Iqbal, M., Jasrotia, A., Kour, K., Ahmed, R., et al. (2015). Sustainable fruit production by soil moisture conservation with different mulches: a review. Afr. J. Agric. Res. 10, 4718–4729. doi: 10.5897/AJAR2014.9149
Baudoin, M. A., Vogel, C., Nortje, K., and Naik, M. (2017). Living with drought in South Africa: lessons learnt from the recent El Niño drought period. Int. J. Disast. Risk Reduct. 23, 128–137. doi: 10.1016/j.ijdrr.2017.05.005
Bezemer, T. M., Fountain, M. T., Barea, J. M., Christensen, S., Dekker, S. C., Duyts, H., et al. (2010). Divergent composition but similar function of soil food webs of individual plants: plant species and community effects. Ecology 91, 3027–3036. doi: 10.1890/09-2198.1
Birkhofer, K., Andersson, G. K., Bengtsson, J., Bommarco, R., Dänhardt, J., Ekbom, B., et al. (2018). Relationships between multiple biodiversity components and ecosystem services along a landscape complexity gradient. Biol. Conserv. 218, 247–253. doi: 10.1016/j.biocon.2017.12.027
Birkhofer, K., Diehl, E., Andersson, J., Ekroos, J., Früh-Müller, A., Machnikowski, F., et al. (2015). Ecosystem services—current challenges and opportunities for ecological research. Front. Ecol. Evol. 2:87. doi: 10.3389/fevo.2014.00087
Birkhofer, K., Scheu, S., and Wiegand, T. (2010). Assessing spatiotemporal predator–prey patterns in heterogeneous habitats. Basic Appl. Ecol. 11, 486–494. doi: 10.1016/j.baae.2010.06.010
Birkhofer, K., Smith, H. G., and Rundlöf, M. (2016). Environmental Impacts of Organic Farming. Wiley eLS, 1–7. doi: 10.1002/9780470015902.a0026341
Blommers, L. H. (1994). Integrated pest management in European apple orchards. Annu. Rev. Entomol. 39, 213–241. doi: 10.1146/annurev.en.39.010194.001241
Bostanian, N. J., Goulet, H., O'hara, J., Masner, L., and Racette, G. (2004). Towards insecticide free apple orchards: flowering plants to attract beneficial arthropods. Biocontrol Sci. Technol. 14, 25–37. doi: 10.1080/09583150310001606570
Bubán, T., Helmeczi, B., and Lakatos, T. (2000). “Effects on the number of soil microorganisms and tree nutrition of groundcover management systems,” in IV International Symposium on Mineral Nutrition of Deciduous Fruit Crops, 201–207. doi: 10.17660/ActaHortic.2001.564.22
Caporaso, J. G., Kuczynski, J., Stombaugh, J., Bittinger, K., Bushman, F. D., Costello, E. K., et al. (2010). QIIME allows analysis of high-throughput community sequencing data. Nat. Methods 7:335. doi: 10.1038/nmeth.f.303
Clothier, B. E., Green, S. R., Müller, K., Gentile, R., Herath, I. K., Mason, K. M., et al. (2013). “Orchard ecosystem services: bounty from the fruit bowl,” in Ecosystem Services in New Zealand–Conditions and Trends, ed J. R. Dymond (Lincoln: Manaaki Whenua Press, 94–101.
Costello, M. J., and Daane, K. M. (1998). Influence of ground cover on spider populations in a table grape vineyard. Ecol. Entomol. 23, 33–40. doi: 10.1046/j.1365-2311.1998.00108.x
de Vries, F. T., Thébault, E., Liiri, M., Birkhofer, K., Tsiafouli, M. A., Bjørnlund, L., et al. (2013). Soil food web properties explain ecosystem services across European land use systems. Proc. Natl. Acad. Sci. U.S.A. 110, 14296–14301. doi: 10.1073/pnas.1305198110
Demestihas, C., Plénet, D., Génard, M., Raynal, C., and Lescourret, F. (2017). Ecosystem services in orchards. A review. Agron. Sustain. Dev. 37:12. doi: 10.1007/s13593-017-0422-1
Diehl, E., Mader, V. L., Wolters, V., and Birkhofer, K. (2013). Management intensity and vegetation complexity affect web-building spiders and their prey. Oecologia 173, 579–589. doi: 10.1007/s00442-013-2634-7
Diekötter, T., Wamser, S., Dörner, T., Wolters, V., and Birkhofer, K. (2016). Organic farming affects the potential of a granivorous carabid beetle to control arable weeds at local and landscape scales. Agric. For. Entomol. 18, 167–173. doi: 10.1111/afe.12150
Diekötter, T., Wamser, S., Wolters, V., and Birkhofer, K. (2010). Landscape and management effects on structure and function of soil arthropod communities in winter wheat. Agric. Ecosyst. Environ. 137, 108–112. doi: 10.1016/j.agee.2010.01.008
Edgar, R. C. (2010). Search and clustering orders of magnitude faster than BLAST. Bioinformatics 26, 2460–2461. doi: 10.1093/bioinformatics/btq461
Finch, O. D., and Löffler, J. (2010). Indicators of species richness at the local scale in an alpine region: a comparative approach between plant and invertebrate taxa. Biodivers. Conserv. 19, 1341–1352. doi: 10.1007/s10531-009-9765-5
Forge, T. A., Hogue, E., Neilsen, G., and Neilsen, D. (2003). Effects of organic mulches on soil microfauna in the root zone of apple: implications for nutrient fluxes and functional diversity of the soil food web. Appl. Soil Ecol. 22, 39–54. doi: 10.1016/S0929-1393(02)00111-7
Fourie, J., Joubert, M., and Freitag, K. (2011). Effect of organic and integrated soil management practices on the weed population in a'Pink Lady'apple orchard in the Elgin region. SA Fruit J. 10, 41–45.
Gerlach, J., Samways, M., and Pryke, J. (2013). Terrestrial invertebrates as bioindicators: an overview of available taxonomic groups. J. Insect Conserv. 17, 831–850. doi: 10.1007/s10841-013-9565-9
Granatstein, D., and Mullinix, K. (2008). Mulching options for Northwest organic and conventional orchards. HortScience 43, 45–50. doi: 10.21273/HORTSCI.43.1.45
Hammermeister, A. M. (2016). Organic weed management in perennial fruits. Sci. Hortic. 208, 28–42. doi: 10.1016/j.scienta.2016.02.004
Happe, A. K., Alins, G., Blüthgen, N., Boreux, V., Bosch, J., García, D., et al. (2019). Predatory arthropods in apple orchards across Europe: responses to agricultural management, adjacent habitat, landscape composition and country. Agric. Ecosyst. Environ. 273, 141–150. doi: 10.1016/j.agee.2018.12.012
Haynes, R. J. (1980). Influence of soil management practice on the orchard agro-ecosystem. Agro Ecosyst. 6, 3–32. doi: 10.1016/0304-3746(80)90003-7
Hector, A., and Bagchi, R. (2007). Biodiversity and ecosystem multifunctionality. Nature 448:188. doi: 10.1038/nature05947
Horak, J., Peltanova, A., Podavkova, A., Safarova, L., Bogusch, P., Romportl, D., et al. (2013). Biodiversity responses to land use in traditional fruit orchards of a rural agricultural landscape. Agric. Ecosyst. Environ. 178, 71–77. doi: 10.1016/j.agee.2013.06.020
Jurczyk, M., Wolters, V., and Birkhofer, K. (2012). Utilization of prey-rich patches leads to reproductive advantages for clustered individuals of a web-building spider. Ecoscience 19, 170–176. doi: 10.2980/19-2-3517
Lanini, W. T., McGourty, G. T., and Thrupp, L. A. (2011). “Weed management for organicvineyards,” in Organic Winegrowing Manual, ed G. McGourty (Richmond, VA: University ofCalifornia, Agriculture and Natural Resources, 69–82.
Latz, E., Eisenhauer, N., Scheu, S., and Jousset, A. (2015). Plant identity drives the expression of biocontrol factors in a rhizosphere bacterium across a plant diversity gradient. Funct. Ecol. 29, 1225–1234. doi: 10.1111/1365-2435.12417
Lavorel, S., and Grigulis, K. (2012). How fundamental plant functional trait relationships scale-up to trade-offs and synergies in ecosystem services. J. Ecol. 100, 128–140. doi: 10.1111/j.1365-2745.2011.01914.x
Leal, I. R., Bieber, A. G. D., Tabarelli, M., and Andersen, A. N. (2010). Biodiversity surrogacy: indicator taxa as predictors of total species richness in Brazilian Atlantic forest and Caatinga. Biodivers. Conserv. 19, 3347–3360. doi: 10.1007/s10531-010-9896-8
Markó, V., Jenser, G., Kondorosy, E., Ábrahám, L., and Balázs, K. (2013). Flowers for better pest control? The effects of apple orchard ground cover management on green apple aphids (Aphis spp.) (Hemiptera: Aphididae), their predators and the canopy insect community. Biocontrol Sci. Technol. 23, 126–145. doi: 10.1080/09583157.2012.743972
Marliac, G., Mazzia, C., Pasquet, A., Cornic, J. F., Hedde, M., and Capowiez, Y. (2016). Management diversity within organic production influences epigeal spider communities in apple orchards. Agric. Ecosyst. Environ. 216, 73–81. doi: 10.1016/j.agee.2015.09.026
Marliac, G., Penvern, S., Barbier, J. M., Lescourret, F., and Capowiez, Y. (2015). Impact of crop protection strategies on natural enemies in organic apple production. Agron. Sustain. Dev. 35, 803–813. doi: 10.1007/s13593-015-0282-5
Mathews, C. R., Bottrell, D. G., and Brown, M. W. (2002). A comparison of conventional and alternative understory management practices for apple production: multi-trophic effects. Appl. Soil Ecol. 21, 221–231. doi: 10.1016/S0929-1393(02)00105-1
Miñarro, M., Espadaler, X., Melero, V. X., and Suárez-Álvarez, V. (2009). Organic versus conventional management in an apple orchard: effects of fertilization and tree-row management on ground-dwelling predaceous arthropods. Agricult. Forest Entomol. 11, 133–142. doi: 10.1111/j.1461-9563.2008.00403.x
Mody, K., Spoerndli, C., and Dorn, S. (2011). Within-orchard variability of the ecosystem service ‘parasitism': effects of cultivars, ants and tree location. Basic Appl. Ecol. 12, 456–465. doi: 10.1016/j.baae.2011.05.005
Montanaro, G., Xiloyannis, C., Nuzzo, V., and Dichio, B. (2017). Orchard management, soil organic carbon and ecosystem services in Mediterranean fruit tree crops. Sci. Hortic. 217, 92–101. doi: 10.1016/j.scienta.2017.01.012
Muneret, L., Mitchell, M., Seufert, V., Aviron, S., Pétillon, J., Plantegenest, M., et al. (2018). Evidence that organic farming promotes pest control. Nat. Sustain. 1:361. doi: 10.1038/s41893-018-0102-4
Peck, G. M., Andrews, P. K., Reganold, J. P., and Fellman, J. K. (2006). Apple orchard productivity and fruit quality under organic, conventional, and integrated management. HortScience 41, 99–107. doi: 10.21273/HORTSCI.41.1.99
Pokharel, R., Marahatta, S. P., Handoo, Z. A., and Chitwood, D. J. (2015). Nematode community structures in different deciduous tree fruits and grape in Colorado, USA and impact of organic peach and apple production practices. Eur. J. Soil Biol. 67, 59–68. doi: 10.1016/j.ejsobi.2015.02.003
Pokharel, R. R., and Zimmerman, R. (2016). Impact of organic and conventional peach and apple production practices on soil microbial populations and plant nutrients. Organ. Agric. 6, 19–30. doi: 10.1007/s13165-015-0106-6
Porcel, M., Andersson, G. K., Pålsson, J., and Tasin, M. (2018). Organic management in apple orchards: higher impacts on biological control than on pollination. J. Appl. Ecol. 55, 2779–2789. doi: 10.1111/1365-2664.13247
Prinsloo, G. L., and Uys, V. M. (eds.). (2015). Insects of Cultivated Plants and Natural Pastures in Southern Africa. Entomological Society of Southern Africa.
Samnegård, U., Alins, G., Boreux, V., Bosch, J., García, D., Happe, A. K., et al. (2019). Management trade-offs on ecosystem services in apple orchards across Europe: direct and indirect effects of organic production. J. Appl. Ecol. 56, 802–811. doi: 10.1111/1365-2664.13292
Schweitzer, J. A., Fischer, D. G., Rehill, B. J., Wooley, S. C., Woolbright, S. A., Lindroth, R. L., et al. (2011). Forest gene diversity is correlated with the composition and function of soil microbial communities. Popul. Ecol. 53, 35–46. doi: 10.1007/s10144-010-0252-3
Simon, S., Bouvier, J. C., Debras, J. F., and Sauphanor, B. (2011). Biodiversity and pest management in orchard systems. Sustain. Agricult. 2, 693–709. doi: 10.1007/978-94-007-0394-0_30
Steinmaus, S., Elmore, C. L., Smith, R. J., Donaldson, D., Weber, E. A., Roncoroni, J. A., et al. (2008). Mulched cover crops as an alternative to conventional weed management systems in vineyards. Weed Res. 48, 273–281. doi: 10.1111/j.1365-3180.2008.00626.x
St. Laurent, A., Merwin, I. A., and Thies, J. E. (2008). Long-term orchard groundcover management systems affect soil microbial communities and apple replant disease severity. Plant Soil 304, 209–225. doi: 10.1007/s11104-008-9541-4
Todd, J. H., Malone, L. A., Benge, J., Poulton, J., Barraclough, E. I., and Wohlers, M. W. (2016). Relationships between management practices and ground-active invertebrate biodiversity in New Zealand kiwifruit orchards. Agric. For. Entomol. 18, 11–21. doi: 10.1111/afe.12121
Tuck, S. L., Winqvist, C., Mota, F., Ahnström, J., Turnbull, L. A., and Bengtsson, J. (2014). Land-use intensity and the effects of organic farming on biodiversity: a hierarchical meta-analysis. J. Appl. Ecol. 51, 746–755. doi: 10.1111/1365-2664.12219
Vogeler, I., Cichota, R., Sivakumaran, S., Deurer, M., and McIvor, I. (2006). Soil assessment of apple orchards under conventional and organic management. Soil Res. 44, 745–752. doi: 10.1071/SR06096
Walmsley, A., and Cerdà, A. (2017). Soil macrofauna and organic matter in irrigated orchards under Mediterranean climate. Biol. Agric. Hortic. 33, 247–257. doi: 10.1080/01448765.2017.1336486
Willer, H., and Lernoud, J. (2019). The World of Organic Agriculture. Statistics and Emerging Trends 2019. Bonn: Research Institute of Organic Agriculture (FiBL); Frick; IFOAM – Organics International.
Williams, A., and Hedlund, K. (2013). Indicators of soil ecosystem services in conventional and organic arable fields along a gradient of landscape heterogeneity in southern Sweden. Appl. Soil Ecol. 65, 1–7. doi: 10.1016/j.apsoil.2012.12.019
Wooldridge, J., Fourie, J., and Joubert, M. E. (2013). Effects of soil surface management practices on soil and tree parameters in a ‘Cripps Pink'/M7 apple orchard 1. Mineral nutrition. South Afr. J. Plant Soil 30, 163–170. doi: 10.1080/02571862.2013.848948
Wyss, E., Luka, H., Pfiffner, L., Schlatter, C., Gabriela, U., and Daniel, C. (2005). Approaches to Pest Management in Organic Agriculture: A Case Study in European Apple Orchards. Wallingford: Cab International.
Yang, Y., Wang, H., Tang, J., and Chen, X. (2007). Effects of weed management practices on orchard soil biological and fertility properties in southeastern China. Soil Till. Res. 93, 179–185. doi: 10.1016/j.still.2006.04.001
Yao, S., Merwin, I. A., Bird, G. W., Abawi, G. S., and Thies, J. E. (2005). Orchard floor management practices that maintain vegetative or biomass groundcover stimulate soil microbial activity and alter soil microbial community composition. Plant Soil 271, 377–389. doi: 10.1007/s11104-004-3610-0
Zabaloy, M. C., Garland, J. L., and Gomez, M. A. (2008). An integrated approach to evaluate the impacts of the herbicides glyphosate, 2, 4-D and metsulfuron-methyl on soil microbial communities in the Pampas region, Argentina. Appl. Soil Ecol. 40, 1–12. doi: 10.1016/j.apsoil.2008.02.004
Zak, J. C., Willig, M. R., Moorhead, D. L., and Wildman, H. G. (1994). Functional diversity of microbial communities: a quantitative approach. Soil Biol. Biochem. 26, 1101–1108. doi: 10.1016/0038-0717(94)90131-7
Zehnder, G., Gurr, G. M., Kühne, S., Wade, M. R., Wratten, S. D., and Wyss, E. (2007). Arthropod pest management in organic crops. Annu. Rev. Entomol. 52, 57–80. doi: 10.1146/annurev.ento.52.110405.091337
Keywords: biodiversity, community composition, ecosystem disservices, farm management, multiple ecosystem services, organic farming, synergies, trade-offs
Citation: Birkhofer K, Addison MF, Arvidsson F, Bazelet C, Bengtsson J, Booysen R, Conlong D, Haddad C, Janion-Scheepers C, Kapp C, Lindborg R, Louw S, Malan AP, Storey SG, Swart WJ and Addison P (2019) Effects of Ground Cover Management on Biotic Communities, Ecosystem Services and Disservices in Organic Deciduous Fruit Orchards in South Africa. Front. Sustain. Food Syst. 3:107. doi: 10.3389/fsufs.2019.00107
Received: 17 June 2019; Accepted: 04 November 2019;
Published: 19 November 2019.
Edited by:
Rebecca Chaplin-Kramer, Stanford University, United StatesReviewed by:
Nobuhiro Kaneko, Fukushima University, JapanGiuseppe Montanaro, University of Basilicata, Italy
Copyright © 2019 Birkhofer, Addison, Arvidsson, Bazelet, Bengtsson, Booysen, Conlong, Haddad, Janion-Scheepers, Kapp, Lindborg, Louw, Malan, Storey, Swart and Addison. This is an open-access article distributed under the terms of the Creative Commons Attribution License (CC BY). The use, distribution or reproduction in other forums is permitted, provided the original author(s) and the copyright owner(s) are credited and that the original publication in this journal is cited, in accordance with accepted academic practice. No use, distribution or reproduction is permitted which does not comply with these terms.
*Correspondence: Klaus Birkhofer, a2xhdXMuYmlya2hvZmVyJiN4MDAwNDA7Yi10dS5kZQ==
†Deceased