- 1Department of Wildlife, Fish, and Conservation Biology, University of California, Davis, Davis, CA, United States
- 2Department of Environmental Science, Policy, and Management, University of California, Berkeley, Berkeley, CA, United States
- 3Institute for Resources, Environment, and Sustainability, University of British Columbia, Vancouver, BC, Canada
- 4Department of Entomology, University of Kentucky, Lexington, KY, United States
- 5Department of Zoology, Biodiversity Research Centre, University of British Columbia, Vancouver, BC, Canada
- 6The Organic Center, Washington, DC, United States
California's Central Coast rose to national food safety prominence following a deadly 2006 outbreak of Escherichia coli O157:H7 that was traced to spinach grown in this intensive agricultural region. Since then, private food safety protocols and subsequent public regulations targeting farm-level practices have developed extensively, aiming to avert future foodborne illness crises. However, amidst sweeping reforms in prescribed best practices for food safety, growers were pressured to take precautionary approaches to control pathogenic contamination—suppressing wildlife near fields, removing habitat, restricting biological soil amendments (e.g., compost, manure), and most recently, chemically treating irrigation water—that may generate negative unintended consequences for environmental and social sustainability. We synthesize socio-ecological data from three qualitative, interview-based studies to examine grower perceptions and experiences of food safety reforms in California's Central Coast region and explore the effects of food safety regulations on environmental and socio-economic sustainability. We identify three disjunctures between food safety requirements and farming realities in practice: (1) Growers perceive that some food safety practices legitimately mitigate risk, while others fail to reduce or even accentuate risk; (2) Food safety requirements can create contradictions in the co-management of food safety and environmental sustainability; and (3) Food safety requirements may foster impediments to regional food systems socioeconomic sustainability. We argue that these disjunctures warrant changes in food safety policy, implementation, and/or food safety education. We provide concrete suggestions for shifting the focus of food safety reform away from the narrow surveillance of individual grower compliance and toward an integrated perspective on regional risk, vulnerability, and resilience.
Introduction
Outbreaks of foodborne illnesses pose serious public health risks worldwide and are increasingly linked to microbial contamination of fresh produce (Lynch et al., 2009; Painter et al., 2013; Li et al., 2018; Turner et al., 2019). In the US alone, more than 34,000 foodborne outbreak-related illnesses resulting in 72 deaths were reported to the US Centers for Disease Control from 1998 to 2013, and although the total number of outbreaks reported during this time decreased, the proportion attributed to fresh produce increased (Bennett et al., 2018). Over the past two decades, vegetable row crops have been implicated in 31% of multistate foodborne Shiga toxin-producing Escherichia coli outbreaks (Figure 1A) and 42% of illnesses associated with those outbreaks (Figure 1B) in the US. In California, 78% of 46 outbreaks with a link to leafy greens between 1996 and 2016 occurred after 2007 (Turner et al., 2019).
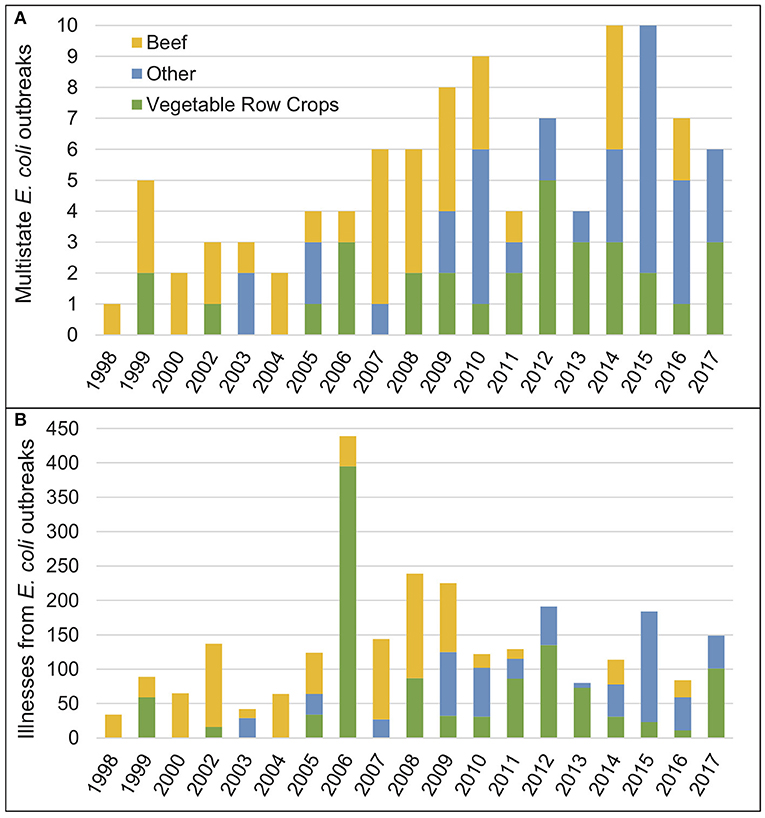
Figure 1. (A) Number of multistate E. coli outbreaks linked to a food source in the US, 1998–2017. (B) Number of illnesses resulting from multistate E. coli outbreaks linked to a food source in the US, 1998–2017. For both charts, source attribution follows Interagency Food Safety Analytics Collaboration (IFSAC) categories identified in the NORS database. For simplicity, all IFSAC categories other than beef and vegetable row crops (the two largest single categories) are combined into a single “Other” category. CDC, National Outbreak Reporting System (NORS), https://wwwn.cdc.gov/norsdashboard/, accessed July 17, 2019.
In the evolving socio-ecological system in which growers, industry buyers, government agencies, and food safety professionals work to mitigate pathogen contamination and foodborne illness outbreaks, California's Central Coast affords a unique context to examine pre-harvest food safety reform (Karp et al., 2015a)1. The region's primary crops—fruits and vegetables—comprise a large portion of the US supply [California Department of Food and Agriculture (CDFA), 2018]. Frequently consumed raw, produce often bypasses any process that would kill pathogens. Pathogens traced to California's raw produce have caused severe illnesses and several deaths (Turner et al., 2019), including the 2006 multistate outbreak of enterohemorrhagic E. coli O157:H7 (EHEC) in spinach that caused 205 illnesses and three deaths (California Food Emergency Response Team, 2007). The spinach outbreak triggered industry-wide reform for produce food safety standards [California Leaf Green Products Handler Marketing Agreement (LGMA), 2019]. As a result, growers faced pressure to implement significant changes to their farm management practices. Even though raw produce may be contaminated at any point along the supply chain, food safety reforms are increasingly directed at the pre-harvest environment on individual farms. Food safety protocols developed in the Central Coast have generated a cascade of ecological and social impacts across the region (Karp et al., 2015a) and have strongly influenced government regulation and industry practice nationwide (Gennet et al., 2013). In the context of liability and market reputation risks, food safety pressures have perpetuated an escalating cycle of crisis and reform in which risk is never zero but the consequences of failure steadily increase (Karp et al., 2015a; Baur et al., 2017). DuPuis (2015, p. 119–124) argues that this cycle traps growers on a “sanitary treadmill,” driving them to scale up their operations to keep pace with the mounting risks generated by a centralizing agrifood system.
This analysis examines how growers in California's Central Coast respond to food safety reforms and considers the implications of these responses for regional sustainability. We ask: how do growers perceive the efficacy of current food safety standards in mitigating food safety risks? Further, what are the potential effects of food safety requirements on environmental and socio-economic sustainability? Our novel approach links social and ecological outcomes in Central Coast agriculture to the overarching food safety policy landscape by analyzing farmer perceptions and experiences of food safety reform in practice. Although aspects of pre-harvest food safety reform have been conceptualized abstractly in the literature (e.g., Karp et al., 2015a; Nguyen-The et al., 2016) or studied in relative isolation [e.g., ecological impacts as in Gennet et al. (2013); Karp et al. (2016); or social impacts as in Baur et al. (2017); Adalja and Lichtenberg (2018b)], our work contributes new insights by systematically tracing social and ecological processes together across the Central Coast.
We find that although many growers in our study perceived positive benefits generated from food safety reform, many also experienced significant disjunctures between food safety requirements and farming realities. First, although growers perceive that some food safety requirements reduce risk, they question the legitimacy and validity of many other requirements that merely “check a box” or may even accentuate risk. Second, although formally many food safety standards promote co-management of food safety and environmental protection, in practice food safety requirements can conflict with growers' management philosophies and environmental sustainability goals. Third, while food safety reform arose to ensure the sustainability and economic viability of the produce industry in the face of public health crises, these reforms create conditions that impede broader socioeconomic sustainability. We argue that these disjunctures warrant a shift in food safety standards implementation, away from narrow surveillance of individual grower compliance and toward an integrated perspective on vulnerability and resilience to risks from human foodborne pathogens in the pre-harvest environment.
In this paper, we first contextualize our analysis by providing an overview of the landscape of food safety reform, including outbreak trends, regulatory changes, and shifts in practices in California's Central Coast. We next present our qualitative interview results, describing grower perceptions and experiences of food safety reform and the impact of reform on food safety risks. Subsequently, we evaluate how food safety reform and the surveillance system implemented to monitor grower compliance impact environmental and socioeconomic sustainability. We conclude with concrete suggestions to help foster food safety practices that are more effective, equitable, and sustainable.
Materials and Methods
Materials: Food Safety Landscape
Trends in Outbreaks
Foodborne illness outbreaks linked to produce are concentrated in the last decade, a trend which epidemiologists believe may be increasing at national (Bennett et al., 2018) and state levels in the US (Turner et al., 2019). Several contributing factors may explain these trends: (1) technical capacity to detect outbreaks and attribute them to produce has grown (Gottlieb, 2018); (2) per-capita consumption of fruits and vegetables has increased in the US (Pollack, 2001; Lynch et al., 2009); (3) produce is transported across long distances to meet this growing year-round demand (Olaimat and Holley, 2012); (4) consolidation in retail and shipping sectors may magnify cross-contamination risks (Beuchat, 2002; Delind and Howard, 2008); and (5) crop fields are in closer contact with known sources of foodborne pathogens, such as confined animal feeding operations [Lejeune et al., 2007; US Food and Drug Administration (FDA), 2019]. Irrigation water and manure represent the top two sources of contamination for leafy greens, including recent outbreaks [Franz and van Bruggen, 2008; Gelting et al., 2011; US Food and Drug Administration (FDA), 2019].
Study Area: California' Central Coast Agricultural Region
Farmers in California's central coast produce a wide variety of row vegetables and berries. Monterey County alone grows ~40% of the United States' lettuce and 25% of its strawberries [California Department of Food and Agriculture (CDFA), 2017-2018]. Our study focuses on farms in the river valleys that flow into Monterey Bay, which are concentrated in Monterey, San Benito, and Santa Cruz counties (Table 1). The 2017 Census of Agriculture counted 405 vegetable farms and 798 fruit and nut farms in these three counties (the Census does not quantify farms that grow both types). Among all farms in the region, 56% gross under $25,000 annually, and only 16% have annual farm sales over $500,000 (United States Department of Agriculture, National Agricultural Statistics Service, 2019).
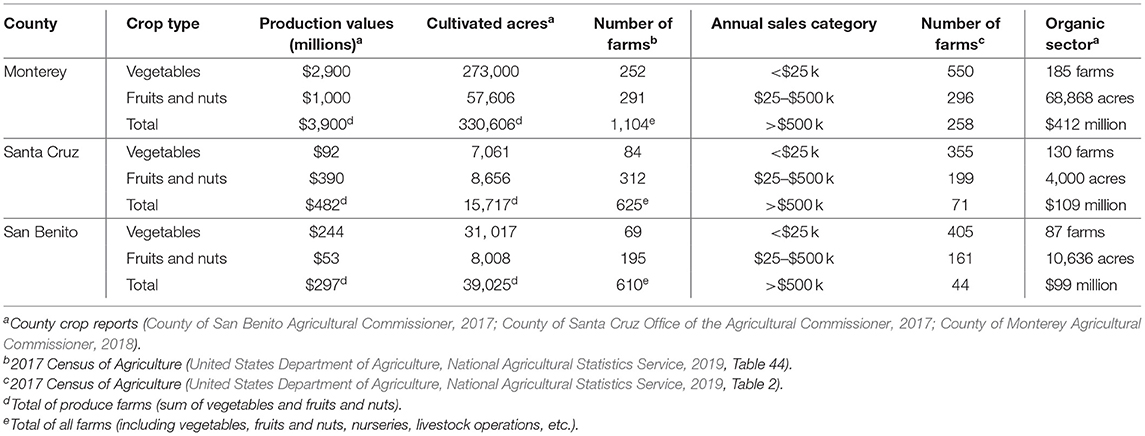
Table 1. Central coast agriculture: selected production statistics for Monterey, Santa Cruz, and San Benito Counties.
Aside from its productive farmland, California's Central Coast also comprises densely developed urban areas that drive high land rents (Guthman, 2004). To compete for land, farmers must maximize crop value per acre. As a result, the region specializes in high-value crops, including highly perishable fruits and vegetables that are usually consumed raw and which thus pose a risk of transmitting foodborne pathogens to consumers. With a mild climate, many farms extend the growing season year-round through multiple plantings or crop rotations to achieve two or more harvests per year. Annual sales tend to be high relative to national averages, even for low-acreage produce farms, which means that many are subject to food safety regulations (see section, Food Safety Regulatory Reforms Affecting California's Central Coast)2.
Agricultural and urban land use both put pressure on ecologically important habitat in the Central Coast, which is home to several federally-protected endangered species [e.g., the California tiger salamander (Ambystoma californiense) and the California red-legged frog (Rana aurora draytonii)], provides harborage for birds migrating along the Pacific Flyway, and connects fragmented wilderness in the hills and riparian zones that dot the region (Thorne et al., 2006; Gennet et al., 2013). Moreover, land use in the region directly impacts the health of the downstream Monterey Bay National Marine Sanctuary (Gennet et al., 2013).
Food Safety Regulatory Reforms Affecting California's Central Coast
Repeated large-scale outbreaks (Figure 2) and an increasing number of product recalls have placed tremendous pressure on government regulatory agencies and the produce industry to institute effective reforms (Lytton, 2019). These reforms comprise an integrated, hybrid regime of private- and public-sector initiatives to determine, disseminate, and incentivize management standards at the farm level (Baur et al., 2017). This hybrid regime is based on the Good Agricultural Practices (GAPs) framework, defined by the FDA in 1998 [US Food and Drug Administration (FDA), 1998]. GAPs, in turn, were adapted from the Hazard Analysis and Critical Control Points (HACCP) framework developed to regulate pathogenic risks in food processing facilities and factories (Baur et al., 2017). For California farmers, the first enforceable GAPs-based standard was created by the leafy greens shipping industry (the first point of aggregation for this sector) in response to the aforementioned 2006 EHEC outbreak in California Central Coast spinach. Media coverage of this deadly outbreak precipitated widespread consumer panic, which catalyzed the formation of the California Leafy Green Products Handler Agreement (LGMA). Formalized in 2007, the LGMA represented a public-private partnership: industry experts set the voluntary growing standards and government officials with the California Department of Food and Agriculture monitored growers' compliance3.
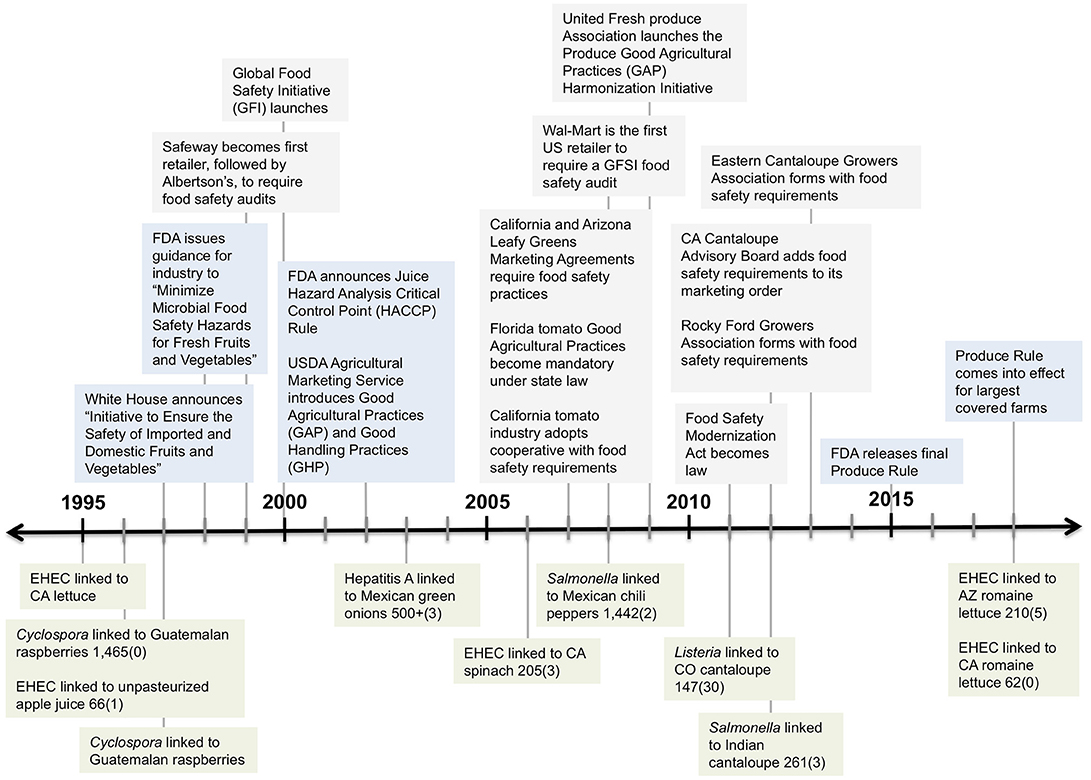
Figure 2. Timeline of selected outbreaks of foodborne illness and food safety policy responses in the US, 1995–2018. Green boxes indicate selected major outbreaks with the number of illnesses, and deaths in parentheses, for the US and Canada combined (for outbreaks with available data). Blue boxes indicate food safety actions by government. Gray boxes indicate food safety responses by industry. Adapted from Table 1 in Minor et al. (2019) with additional data from the California Food Emergency Response Team (2007) and US Centers for Disease Control (CDC) (2019).
As food safety concerns spread, new pan-commodity food safety standards gained prominence in California produce agriculture, for products ranging from avocados to strawberries to peaches (Lowell et al., 2010; Baur et al., 2017). These various standards are increasingly harmonized through the Global Food Safety Initiative, a global regime that coordinates private-sector food safety standards for multinational food corporations (Verbruggen and Havinga, 2016). However, specific food safety requirements often vary depending upon the precise demands of a given retail buyer (Minor et al., 2019). To reinforce the patchwork system of private-sector standards, the US Food Safety Modernization Act of 2011 directed the FDA to establish and enforce science-based minimum standards for growing, harvesting, and handling fruits and vegetables that are typically consumed raw. Codified in 2015 (80 FR 74353), the FDA's Produce Rule requires all farms with more than USD $500,0004 in annual sales to comply with standards related to worker health and hygiene, microbial water quality, soil amendment microbial quality, environmental monitoring (including animal intrusion), and tool and equipment sanitization. Moreover, the Produce Rule introduced minimum employee training and record-keeping requirements, although many third-party audits require more extensive records5. While some small-scale direct market farms may be exempt or partially exempt from the Rule, such farms are nevertheless often subject to third-party food safety certification standards to meet buyer demands.
Food Safety Practices in California's Central Coast Region
As food safety reforms propagated in the wake of the 2006 EHEC outbreak, Central Coast growers faced intense pressure from food safety auditors, inspectors, and other food safety professionals to control potential sources of human pathogens in the farm environment (Beretti and Stuart, 2008; Beretti, 2009; Lowell et al., 2010; Baur et al., 2016). Many growers reported yielding to this pressure, and adopted costly practices—including suppressing wildlife and removing non-crop vegetation near fields (Table 2)—that conflicted with their environmental sustainability goals (Table 3; Beretti and Stuart, 2008; Crohn and Bianchi, 2008; Beretti, 2009; Stuart, 2009; Lowell et al., 2010; Baur et al., 2016). Some of these actions have left tangible marks on the landscape. For example, from 2005 to 2009, food safety reform spurred the removal of ~13% of Salinas River riparian habitat, an important conservation corridor (Gennet et al., 2013). Growers in the region reported as early as 2007 that farm management changes were driven more by fear than by science (Beretti and Stuart, 2008); many practices commonly implemented in response to food safety concerns are neither evidence-based nor proven to be effective (e.g., Langholz and Jay-Russell, 2013; Karp et al., 2015b; Sellers et al., 2018).
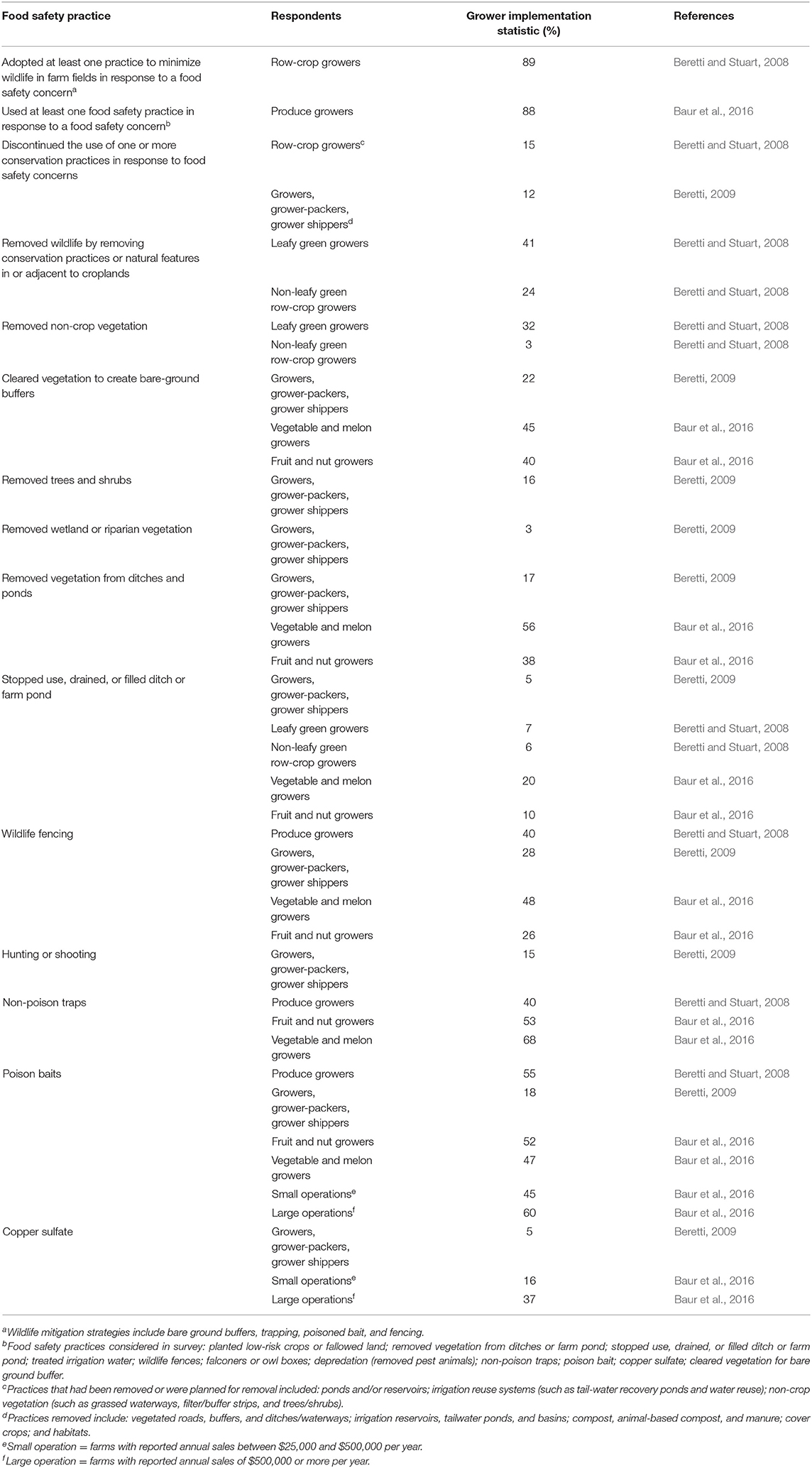
Table 2. Grower adoption of food safety practices and abandonment of conservation practices in response to food safety concerns.
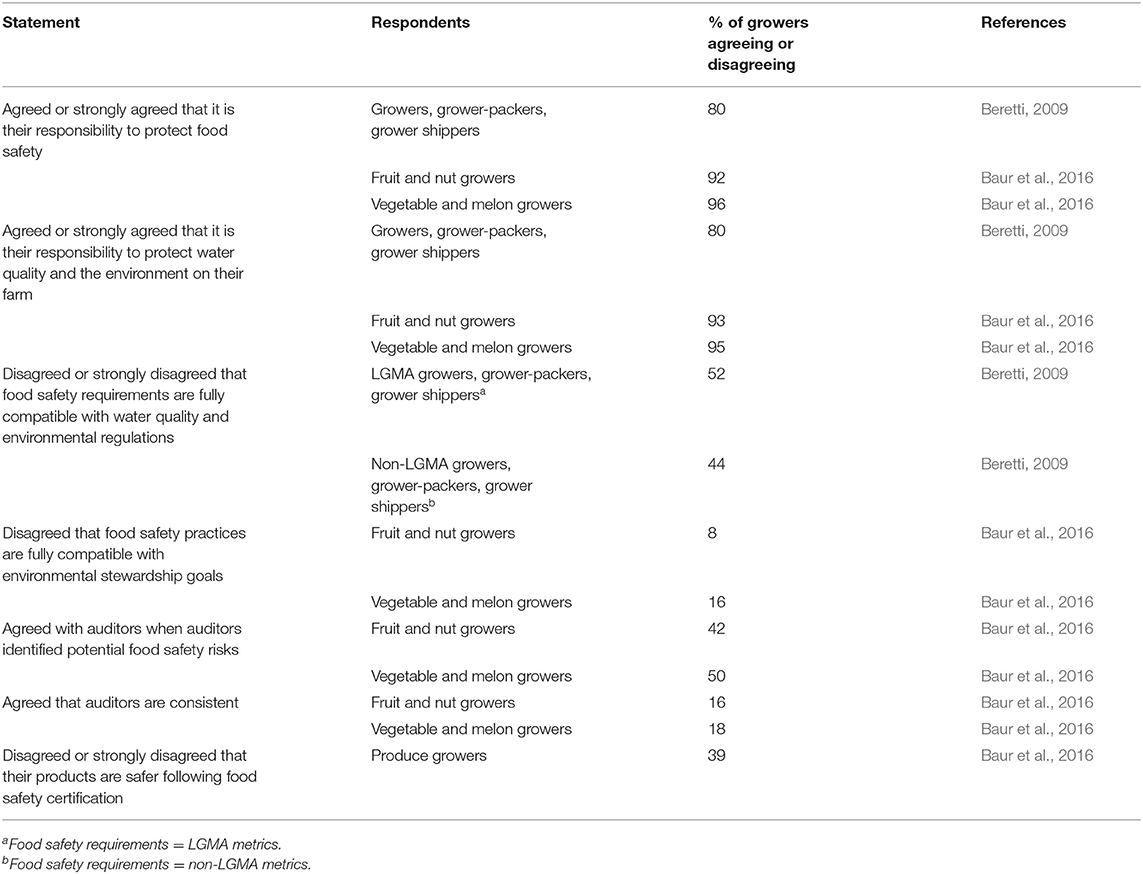
Table 3. Percent of growers agreeing or disagreeing with statements relevant to food safety and environmental stewardship.
Methods
We analyze data from three related qualitative, interview-based studies of growers in the California Central Coast region. Broadly, each of these studies examined how growers in the Central Coast perceive and respond to food safety reform via changes to their on-farm practices, as well as their operational management challenges. All studies were conducted in accordance with a human subjects research protocol approved by an institutional review board (IRB).
For each study, combined purposive sampling and network sampling approaches were used to identify and contact potential research subjects (Blaikie, 2000, p. 204–206; Bryman, 2016, p. 410–420). First, we conducted informational interviews with key informants (e.g., cooperative extension specialists, industry trade association liaisons) to define relevant problem areas, assess the kind of information that growers would be able to provide, and identify initial growers to contact. We identified further potential grower respondents through organic certification and agricultural pesticide use permit records, which are kept at the county level, for the Central Coast region. Next, we contacted potential grower respondents using standardized recruitment scripts as approved by our respective IRBs. Contact was made by phone, by email, through personal referral, and in person (e.g., at a food safety training event).
Each study utilized a standardized interview guide (Appendix I in Supplementary Material), which was developed based on past field experience of the researchers, preliminary exploratory interviews, and review of the literature; each interview guide was reviewed and approved by our respective IRBs. Using those guides, we conducted in-depth, semi-structured interviews with growers. In contrast to structured questionnaires (such as are used in surveys), semi-structured interviews follow a flexible format better suited for natural conversation and that is designed to allow respondents to direct the interview in ways that reflect their own perceptions, experiences, and priorities. Semi-structured interviews allow interviewers to adapt to conventional conversational structures; as such, questions and responses may flow in a different order than is documented in the interview guide. Interviews were audio recorded and transcribed; in the rare case (n = 5) that growers did not agree to be recorded, responses were recorded in field notes made by the interviewer in real-time.
Collectively, we conducted semi-structured interviews with 55 specialty crop growers between 2013 and 2018 (Table S-1). Growers in our sample (1) produced a diverse mix of specialty crops, including leafy greens, mixed vegetables, and berries; (2) represented a range of production approaches, including conventional (13% of respondents), organic (55% of respondents), and mixed conventional and organic (33% of respondents); and (3) represented a range of operational sizes, ranging from <10 to 10,000 cultivated acres. As a high estimate based on 2017 Census of Agriculture data (see section, Study Area: California' Central Coast Agricultural Region), we calculate that there could be as many as 530 produce farms in our study area that are subject to food safety requirements. This estimate assumes (a) no overlap between vegetable and fruit and nut farms, yielding 1,203 total produce farms, and (b) 44% of produce farms sell more than $25,000 of product annually and are at least partly subject to the Produce Rule. Conservatively, the 55 growers we interviewed likely comprise at least one-tenth of all produce farms subject to food safety requirements in the study area.
We coded interview responses for key emergent themes within eight overarching topic areas, which included pre-harvest food safety management—of animals (Appendix B in Supplementary Material), vegetation (Appendix C in Supplementary Material), soil (Appendix D in Supplementary Material), water (Appendix E in Supplementary Material), human hygiene and equipment/facilities sanitization (Appendix H in Supplementary Material)—as well as implementation costs (Appendix F in Supplementary Material), decision-making power (Appendix G in Supplementary Material), and acceptance of food safety reform in general (Appendix A in Supplementary Material). We then compared our findings across all three studies. Our findings significantly aligned in key themes related to wildlife management, the efficacy of food safety practices, and the disjuncture growers experienced between food safety requirements and farming realities. We noted minor thematic divergences in relation to race and ethnicity and food safety regulation implementation that reflected a difference in the racial composition of our interview samples. After coding, we analyzed the interviews to examine how socio-ecological changes affect sustainability and food safety risks in the Central Coast region. In the main text we provide illustrative quotes selected from the full set of responses (Appendices A–H in Supplementary Material) to represent the common or typical response on a given topic. These quotes are attributed to respondents using an anonymized code of the form Respondent X that can be cross-referenced with the respondent meta-data provided in Table S-1. Several respondents were interviewed twice; interviews from these respondents are distinguished with an a or b appended to their number. Due to variation in interview guides across the three studies and to the fluid nature of semi-structured interviews, we have chosen not to quantify responses to specific questions.
Results
We found that produce growers in California's Central Coast region perceived some food safety requirements to generate positive benefits, particularly those that improve worker hygiene (e.g., hand-washing, better portable bathrooms) or reinforce their personal values around “clean fields.” Nevertheless, the majority of growers also perceived significant disjunctures between pressures to improve food safety and their farming realities. Our results suggest that many of the tensions observed between food safety reform and agricultural sustainability (as reviewed in section, Food Safety Practices in California's Central Coast Region) originate from incompatibilities between an embedded “factory logic” of hazard analysis and control (designed for closed systems) and the porous dynamics of agroecosystems (see also Stuart, 2008; Stuart and Worosz, 2012). This incompatibility may be magnified when growers' desire for proven practices to help them grow safe food is overridden by skepticism of external oversight (if growers perceive that regulators lack legitimacy) and prescribed practices (if growers perceive that requirements lack validity)6. Here, we first examine the ways in which growers questioned the legitimacy of regulators and validity of food safety requirements, revealing a disconnect between prescribed practices growers perceived to be effective vs. those perceived to merely “check a box” (i.e., fulfill an ineffective requirement). We then explore how this disconnect contributes to the crisis-and-reform cycle described in the introduction (Disjuncture 1).
Second, we detail the ways in which growers perceived that implementing food safety requirements diverged from their own farm management philosophies and environmental sustainability goals (Disjuncture 2). We align our presentation of these results with the structure of the curriculum used to train growers in food safety, which identifies three environmental sources of pathogenic contamination: water, animals (domestic or wild), and soil7. In addition, we contextualize growers' perceptions with evidence from the ecological literature on the impact of required practices on actual food safety risks. We conclude our results with an analysis of the ways in which the current direction of food safety reforms may impede regional socioeconomic food systems sustainability (Disjuncture 3).
Disjuncture 1: Legitimacy of Regulators and Validity of Requirements
Growers overwhelmingly supported proven measures to make their produce safer for consumption. This value was frequently expressed as a version of the “golden rule”: treat the food you produce as you would like others to treat the food they produce for you. As one strawberry grower put it, “I don't want to eat the fruit if somebody doesn't take care of it or doesn't have food safety on [their] farm” (Respondent 9). Many attested, as did Respondent 54, “We're doing everything that we can to prevent anything (i.e., an outbreak) from happening again,” and believed, as Respondent 42 phrased it, “Anything that we can do to make things safer, is rockin'.”
Growers tended to accept several prescribed practices as sensible and positive. For example, growers often expressed appreciation for consistent worker hygiene and equipment sanitation standards (Appendix H in Supplementary Material). Respondent 3 captured this sentiment, saying, “The most important thing for food safety is to be careful… So for me, I understand that we have to be careful… put on gloves, wash our hands first and foremost… We ourselves set the example.” This view coincided with a desire for improved trust and transparency of purpose between farm management and farm labor, following the belief that “this whole farming thing is about people management” (Respondent 45). Respondent 54 tied these themes together:
“Training has become very critical, a big component on the farming and the harvesting side. But not only just talking to the people…More critical is educating the people, educating them… [on] the importance of why we have, [why] we follow good hygiene practices, why are we mandating, requiring everybody to wash your hands with soap and water before for work, after using the restroom, after break. I'm educating them [on] why we require that and not just telling them, ‘Just do this, do this.”'
In a similar vein of food safety lining up with other farm management goals, growers' personal preference for what they called “clean” fields—visually free of detritus, weeds, or clutter—also appeared to align well with several underlying assumptions about food safety requirements. Many growers equated “clean” fields with safer fields (Appendix C, Theme 2 in Supplementary Material):
“I think that side of food safety has been really good because we've done a lot of work to just keep the place clean, minimize the habitat for things like rats and squirrels and keeping things off the ground and disposing of your tape and all your cardboard boxes. That's really a positive thing.” (Respondent 25)
However, although growers embraced food safety requirements that aligned with their values around order and cleanliness, we also found that growers questioned the validity of requirements that claimed to improve food safety but either seemed ineffective in practice or imposed what they felt were unreasonable burdens on their time and resources. Moreover, many growers we interviewed expressed a lack of confidence in food safety professionals and questioned their legitimacy in determining how growers' farms should be managed. For example, a consistent complaint was that too many of the people who oversee food safety—government officials, buyer representatives, and third-party auditors—lack experience with and understanding of farm practices and management (Appendix A, Theme 1 in Supplementary Material). Some growers communicated their impressions that requirements were based on arbitrary hunches rather than sound evidence (Appendix A, Theme 2 in Supplementary Material). Reported inconsistency in food safety requirements likely magnified this perception (Appendix A, Theme 3 in Supplementary Material). For some growers, oversight requirements appeared not only arbitrary, but actively biased against them and designed to absolve processors, retailers, or consumers of liability in the case of foodborne illness (Appendix A, Theme 4 in Supplementary Material).
Despite their reservations, most growers felt compelled to adopt a “grin-and-bear-it” attitude toward questionable requirements: “If I want to play ball with buyers, I need to do certain things that I'm not particularly happy about, but I do it anyway” (Respondent 46). For this reason, growers viewed some requirements as a performance or “for show” (Appendix A, Theme 5 in Supplementary Material). Respondent 46 later added, “…It's show. You're not doing anything that's going to make the food any safer. It's still grown outdoors.” Growers frequently communicated an overall feeling that there are two types of food safety requirements—those that actually reduce risk and those that merely “check a box.” We next illustrate, in the context of agricultural water, how the iterative cycle of crisis-and-reform (Karp et al., 2015a; Baur et al., 2017) accentuates this disjuncture.
Agricultural Water Accentuates Disjuncture 1
To control the microbial quality of irrigation water, wash water, and water used to mix agrichemical sprays, both the Produce Rule and LGMA require strict sampling and laboratory testing regimens for all agricultural water sources. These requirements have increased in intensity following the two EHEC outbreaks in romaine lettuce in 2018 that were linked to contaminated agricultural water (Ward, 2019), and LGMA now requires “a scientifically valid antimicrobial treatment” (e.g., chlorination) for all surface water that may contact the edible portion of the crop (e.g., overhead irrigation) within 21 days of harvest (California Leaf Green Products Handler Marketing Agreement (LGMA), 2019). Although our interviews were conducted prior to this regulatory shift, we suggest complications that these requirements may pose for growers moving forward.
First, instantiating Disjuncture 1, growers widely agreed that they need clean, uncontaminated agricultural water, but some also believed that water testing requirements were prescribed in a way that did not adequately reflect actual risks. They reported that either test results consistently showed no contamination (e.g., for well-water, which most Central Coast growers rely upon) or that sampling and laboratory protocols themselves might introduce human error that skewed results (Appendix E, Theme 1 in Supplementary Material). Respondent 50a represented this position concisely, saying,
“There's certain things that really nobody should be exempt from. People should test their water source, that just makes sense. The frequency we're testing it, [however,] it's probably a bit overkill, now that we've been testing for so long. I think testing your well water every month is kind of, it's total overkill. We never—it's always the same thing. You never find anything.”
Some growers also indicated that using surface water entailed more frequent testing, more paperwork, and higher food safety risk compared to groundwater. Even prior to the new LGMA requirements, two growers felt the need to chlorinate surface water used for irrigation (Appendix E, Theme 2 in Supplementary Material). Respondent 41 also recalled, “Early on, there were farmers that were worried about the audits… and tried to kill whatever was in their ponds.”
Currently, scientific evidence on the efficacy of water testing and treatment protocols is limited. Although irrigation water may transmit pathogens to produce (Uyttendaele et al., 2015) and even cause outbreaks [e.g., US Centers for Disease Control (CDC), 2008, 2018, 2019], evidence-based strategies to manage microbial quality of agricultural water remain limited due to the complexity of this problem (Allende and Monaghan, 2015; Havelaar et al., 2017; Topalcengiz et al., 2017). The low prevalence of many pathogens in surface water, and the prohibitive cost of pathogen testing, makes the use of microbial indicators of fecal contamination, particularly generic E. coli (which is used in the Produce Rule), a common approach (Park et al., 2013). However, it remains unclear whether common fecal indicators can accurately and reliably predict pathogen loads in water: while some studies argue that generic E. coli is a conservative predictor of pathogen loads in surface water (Wilkes et al., 2009), other studies suggest that generic E. coli alone is not a suitable indicator (Benjamin et al., 2013; Pachepsky et al., 2016). In cases where water samples test positively for bacterial contamination, water treatment is recommended; yet approved water sanitization options are associated with additional costs and may not result in zero-risk irrigation water (Lewis Ivey and Miller, 2013; Allende and Monaghan, 2015). For example, minimum effective chlorination doses are influenced by various factors, including water properties and weather conditions, making it difficult to specify minimum effective standards that apply across diverse farm environments (López-Gálvez et al., 2017). Additionally, residual sanitization agents in irrigation water could potentially disrupt soil microbial communities and have adverse effects on soil pathogen suppression (Truchado et al., 2018).
The recent LGMA changes to microbial water quality standards bear the markings of the next iteration in the crisis-and-reform cycle for food safety in produce agriculture (Karp et al., 2015a,b; Baur et al., 2017). These reforms arose in response to prominent public health crises [i.e., the two outbreaks linked to romaine lettuce; US Centers for Disease Control (CDC) (2018, 2019)], and comprise rapid, sweeping rule revisions that carry real costs for farmers8, but for which the scientific evidence is highly uncertain. The rate of regulatory change combined with the fact that growers will bear the cost suggests further entrenchment of a perception among growers (Disjuncture 1) that some food safety practices are for show and designed primarily to benefit down-stream supply chain actors, rather than to protect consumers or to reduce risks for growers. We now proceed to illustrate a second disjuncture between perceived food safety requirements and practical management of animals (domestic or wild), habitat, and soil.
Disjuncture 2: Managing for Food Safety and Environmental Sustainability Goals
In an attempt to limit wildlife intrusion and pathogen introduction into farm fields, many farmers have cleared wildlife habitat in and around farms, fenced fields, used poison baits, trapped rodents, and ceased applying raw manures or composts (Baur et al., 2016). These practices may conflict with conservation practices that protect biodiversity, reduce runoff from agricultural fields, prevent soil erosion, enhance soil quality, and (paradoxically) suppress foodborne pathogens (Kilonzo et al., 2013; Staley et al., 2014; Karp et al., 2015a,b; Sellers et al., 2018; Gonthier et al., 2019). Many species promoted by agricultural conservation practices provide ecosystem services that benefit farm production, including biological control, pollination, soil and water quality, and pathogen suppression (Kremen and Miles, 2012; Karp et al., 2013; Blaauw and Isaacs, 2014; Bender et al., 2016; Schulte et al., 2017; Jones et al., 2019a). However, growers, regulators, and/or auditors may also perceive conservation practices as a risk in light of food safety requirements.
Controlling Wildlife
Early literature on tensions between safety and sustainability highlighted how controlling the risk of contamination from animals led to suppression of wildlife and natural habitat (Beretti and Stuart, 2008; Beretti, 2009; Lowell et al., 2010). Managing for animal intrusion also carries substantial costs, as growers are prohibited from harvesting products within a given radius of evidence of animal intrusion (e.g., no-harvest buffers) due to risk of pathogen transmission from feces. Large-scale leafy greens growers in the region reported that product discarded due to animal activity accounts for 11% of their total food safety compliance costs (Calvin et al., 2017).
Even though LGMA standards and the official training curriculum for FDA's Produce Rule now incorporate recommendations to co-manage for both food safety and environmental conservation goals [Produce Safety Alliance, 2017, Module 5; California Leaf Green Products Handler Marketing Agreement (LGMA), 2019], a majority of growers reported facing ongoing pressure to keep wildlife away from fields (Appendix B, Theme 1 in Supplementary Material). Although a common belief was that the 2006 EHEC outbreak provoked extreme initial reactions to wildlife and habitat that have abated over time as “people are looking more to the science” (Respondent 36), our interviews reveal that disjunctures persist between food safety requirements and on-farm realities.
After deer and pigs were implicated in outbreaks, growers erected hundreds of miles of fencing throughout the Central Coast to exclude terrestrial animals. These fences are concentrated along river corridors, and growers noted that the Salinas River is “fenced all the way to the Pacific Ocean” (Appendix B, Theme 2 in Supplementary Material). Growers generally agreed that “(large animal intrusion has) gotten a lot better in the Salinas Valley” due to fences (Respondent 49), but several raised concerns about the effect on wild animals. “There's no way for them to get out if it ever floods,” noted Respondent 27. More critically, wildlife fencing surrounding farms may impede the movement of species between natural habitat patches, disrupting typical dispersal patterns (Jakes et al., 2018). For example, extensive fencing along the Salinas River corridor in the Central Coast limits wildlife movement, as evidenced by concentrated wildlife movement where gaps in fencing occur (Atwill, 2008).
Although food safety guidelines do not recommend the active removal or poisoning of wildlife to mitigate food safety risks, some farmers report using chemical treatments, including poison baits such as rodenticides to limit small mammals (Lowell et al., 2010) and copper sulfate algaecide treatments in surface water, which also controls amphibians (Baur et al., 2016). Poison baits and traps may attract both target (e.g., pocket gophers, Thomomys spp.) and nontarget species (Elliott et al., 2014), including native, at-risk mammals (i.e., Monterey dusky-footed woodrat, Neotoma macrotis luciana) (California Department of Fish and Wildlife, 2019). Anticoagulant rodenticides may also bioaccumulate in predators such as raptors and mammalian carnivores (Hosea, 2000), causing increased mortality due to acute severe hemorrhage (Poessel et al., 2015), increased susceptibility to mange (Serieys et al., 2013), and sublethal differential expression of immune-related genes (Fraser et al., 2018). Similarly, the illicit use of copper sulfate to control amphibian and fish populations also affects aquatic invertebrates (Eisler, 1998) and could trigger cascading effects on species in higher trophic levels that depend on amphibians and fish as prey.
Increased attention to small mammals and amphibians highlights a key development in food-safety regulation: the evolution of expectations regarding animal intrusion from controlling a few species “of significant risk” (e.g., deer, pigs, cattle) to “assessment of field intrusion risks from any animal,” as occurred within the LGMA standards (Hornick, 2013). The message on animal intrusion has become more ambiguous, moving from the language of “control” to one of “assessment,” which places the onus of judgement on growers. Indeed, some growers also perceived that birds pose a more immediate food safety risk than terrestrial animals: “The thing that worries me more actually are birds… you can't control birds and they constantly like flying over your field. They come and sit in the field. They carry Salmonella” (Respondent 50a) (see also Appendix B, Theme 3 in Supplementary Material). But most growers simply contended that birds, regardless of risk, cannot be stopped and that efforts to eliminate them do not align with on-farm realities: “You can't keep them out. It's not like deer or some kind of wildlife where you can have a fence around the field and a legitimate barrier” (Respondent 49). Another grower, when a squirrel ran through a torn silt fence during an interview, commented ruefully, “So you can see how good our rodent fences do. So, what functional purpose that serves, other than to tell McDonald's you got it?” (Respondent 55).
Nonetheless, coupled with the high financial and liability stakes of a food safety incident, many growers felt they must take a proactive, precautionary stance toward both bird and animal intrusion. Not every grower was happy being put in this position. For example, Respondent 44a exclaimed, “I don't like killing all the ****ing frogs. I mean, to me, it's not right. But it's also—you've got to do it.” In the case of raptors and other predators, most growers perceived that suppressing these species would forego the food safety benefits they provide: controlling pathogen vectors such as rodents (Appendix B, Theme 4 in Supplementary Material).
The scientific literature suggests that growers' skepticism toward the focus on suppressing wildlife may be well-founded, as the role of wildlife in precipitating foodborne disease outbreaks remains unclear. For example, while wildlife has been implicated in EHEC outbreaks, it is the association between wildlife and livestock that presents the primary contamination pathway (Langholz and Jay-Russell, 2013). Cattle are the primary reservoir of EHEC and Salmonella for human infections (Wells et al., 2001; Callaway et al., 2014), and multiple studies have shown a positive association between cattle density and EHEC infections in humans (e.g., Valcour et al., 2002; Frank et al., 2008). In a review of studies that screened wildlife for EHEC, EHEC was relatively rare (Langholz and Jay-Russell, 2013); in the Central Coast, cattle are more likely than wildlife to test positive for EHEC (Cooley et al., 2013). An investigation of the 2006 EHEC outbreak linked to spinach in the Central Coast found the outbreak strain in cattle and feral pig feces, surface water samples, and soil and sediment samples. This investigation suggested that feral pigs could have transferred EHEC from cattle pastures to crop fields via shared surface water sources (Jay et al., 2007). In addition to feral pigs, birds associated with cattle may be competent pathogen vectors (Callaway et al., 2014), and birds have been linked to at least one food safety outbreak (Gardner and McLaughlin, 2008).
Habitat Removal
Habitat removal was among the most common actions that growers were pressured to take to mitigate wildlife intrusion (Appendix C, Theme 1 in Supplementary Material). Nonetheless, neither non-crop habitat at farm edges nor in the landscape surrounding farms has been correlated with greater incidence of pathogens in farm fields. At the farm scale, one study in California found that hedgerows at farm edges were not associated with greater mammalian wildlife intrusion or increased crop contamination (Sellers et al., 2018). At the landscape scale, Karp et al. (2015b) found no evidence of higher pathogen levels on farms surrounded by more semi-natural vegetation, and instead found that vegetation removal was weakly correlated with increased pathogen incidence.
Correspondingly, one group of growers—primarily those pursuing ecological farm management—tended to perceive that maintaining on-farm biodiversity leads to safer and healthier agroecosystems (Appendix A, Theme 6 in Supplementary Material). A few even reasoned that removing habitat might actually increase food safety risks:
“Science supports diversity as a control mechanism for outbreaks on all levels, be it the macro level with the hawks and birds or down to the microbial level… Intuitively I know there's truth in that…” (Respondent 12b).
Indeed, even “clean field” proponents who stripped the land down to bare dirt with herbicides or mechanical scrapers (Appendix C, Theme 2 in Supplementary Material) seemed to do so from a belief that food safety is for “show” (Appendix A, Theme 5 in Supplementary Material). As Respondent 50a explained, “If you have customers or you have auditors out, if you have things like looking neat and clean and tidy, it goes so far… I guess it means you care.” Thus, although we found significant variance among growers in their tolerance for biodiversity on their farms, many of those who felt pressured to remove wildlife habitat widely considered the practice ineffective or even counterproductive for reducing food safety risks.
Vegetation removal could indeed exacerbate food safety risks for several reasons. Vegetative buffers can reduce pathogen loads in runoff and surface water (Tate et al., 2006; Winkworth et al., 2008), attenuating pathogen prevalence on farm fields, especially during flooding (Strawn et al., 2013). Clearing vegetation may also favor wildlife that are more likely to vector pathogens, such as deer mice (Kilonzo et al., 2013; Sellers et al., 2018), if competitors or larger predatory wildlife (e.g., mountain lions, bobcats) are excluded by widespread habitat removal. Maintaining non-crop habitat in and around farms can also increase mammalian diversity (Sellers et al., 2018), which may help mitigate pathogen prevalence by constraining intraspecific pathogen transmission and limiting pathogen spreading (Kilonzo et al., 2013). Finally, habitat removal can cause declines in dung beetle (Coleoptera: Scarabaeidae) communities that suppress pathogens directly by consuming feces, and indirectly by disrupting the spread of pathogens by flies (Hutton and Giller, 2003; Jones et al., 2015, 2019a,b). Taken together, these findings suggest the available science may align with many growers' perceptions that habitat removal is not an effective risk mitigation strategy.
Beyond its questionable efficacy regarding improvements in food safety, habitat removal likely carries significant risks for biodiversity and key ecosystem services upon which growers depend. Non-crop vegetation within, adjacent to, or surrounding farms benefits bird, bat, mammal, pollinator, and beneficial arthropod biodiversity (Bengtsson et al., 2005; Batáry et al., 2011; Gonthier et al., 2014). Eliminating habitat in and around farms can also reduce habitat connectivity and interfere with the ability of species to access important resources (Penrod et al., 2001; Brady et al., 2011). Recent studies in California also show that increasing on-farm diversification and natural habitat promotes biodiversity. For example, increasing the amount of semi-natural habitat surrounding farms increased bird species richness (Gonthier et al., 2019). In two other studies in California agriculture, incorporation of hedgerows along field edges increased mammalian diversity (Sellers et al., 2018), and field margins with woody vegetation (hedgerows, treelines, or riparian vegetation) increased bird species richness and abundance compared to bare and weedy field margins (Heath et al., 2017). Hedgerows enhanced pollinator species and functional diversity at site and regional scales, as well as enhanced the persistence, colonization and occupancy of pollinator species (M'Gonigle et al., 2015; Ponisio et al., 2016, 2019).
Decades of research also suggest that biodiversity promotes ecosystem services (Cardinale et al., 2012), upon which many growers may depend. In California's Central Coast, more semi-natural habitat surrounding farms has also been correlated with increased abundance of natural enemies (predators of crop pests), decreased abundance of arthropod and bird pests (Chaplin-Kramer et al., 2013; Gonthier et al., 2019), enhanced pest control (Letourneau et al., 2012; Karp et al., 2016), lower levels of bird damage to strawberries (Gonthier et al., 2019), and enhanced pollination services to various crops (Kremen et al., 2004; Klein et al., 2013). Within farms, Morandin et al. (2016) showed that the pest control and pollination services associated with hedgerows can cover the cost of hedgerow installation and become profitable 7 years after hedgerows are established. In intensively managed agricultural landscapes, the presence of even a few trees at field edges increased bird abundance, diversity, and bird-mediated pest control (Kross et al., 2016).
Our interviews revealed that growers recognize many of the ecological benefits of retaining habitat around farm fields and are resistant to removing habitat for food-safety concerns (Appendix C, Theme 3 in Supplementary Material). “Trees protect us a lot from diseases and pests” remarked Respondent 9. Similarly, Respondent 53 stated “You can definitely see a lot of times, we'll have better biological control if we're adjacent to, say a riparian area.” Many growers believed that the negative consequences of habitat removal for food safety extended far beyond pest control. As Respondent 42 explained,
“One thing I think is kinda dumb, but any kind of waterway, or any of that kind of riparian area that we border, we keep all that clean now. Where we [would usually] have native grasses there, you know good for erosion and stuff like that, also good for containing nutrients. So we keep all of those kind of stripped right now with herbicides…”
Indeed, when food safety precautions threatened vegetation that growers believed provided benefits, frustration turned to resistance (Appendix C, Theme 3 in Supplementary Material). For example, one grower related how food safety auditors “get all up in arms” about the vegetation he used to protect his levee from floods and erosion, noting that “food-safety-wise, that doesn't look good” (Respondent 43). Another grower remarked, “I draw the line when it comes to habitat” (Respondent 21).
Grower's perceptions of the value of habitat conservation for improving water quality are well-founded. Vegetative filter strips capture nutrients (Bedard-Haughn et al., 2004), and many insecticides and herbicides (Chen et al., 2016) from agricultural runoff before they enter waterways. Vegetation can also reduce sediment loss and control erosion, thus improving water quality (Haddaway et al., 2016) and retaining soil on farm fields (Schulte et al., 2017). Removal of these vegetative filter strips could result in the loss of these ecosystem services, exacerbating regional water quality issues (Harter et al., 2012; Phillips et al., 2012), and jeopardize funding from governmental conservation organizations that provide financial assistance to implement vegetative filter strips (e.g., Conservation Stewardship Program, Environmental Quality Incentives Program).
Organic Soil Amendments
Previous work in the Central Coast has documented aversion to using organic soil amendments due to concern over the potential for cross-contamination of human pathogens from soils to crops, in particular composted animal manure (Baur et al., 2016; Karp et al., 2016). Replacing manure and compost with more highly-processed or synthetic fertilizers can affect soil health. For example, use of such fertilizers can reduce soil organic matter and disrupt a variety of key soil processes, including nutrient cycling and retention, water infiltration and retention, water filtration, erosion control, and carbon sequestration (Drinkwater et al., 1998; Weil and Magdoff, 2004).
Our analysis reveals that some buyers discourage or prohibit growers from using compost due to food safety concerns (Appendix D, Theme 1 in Supplementary Material). This poses a dilemma for growers who believe that compost is essential for soil health, especially in organic production (Appendix D, Theme 3 in Supplementary Material). “It's really getting tough,” said Respondent 43, “I hate using soil amendments. I mean, I love using them, but I hate using them ever since all these protocols came out… This was the first year (2017) I switched over in a big way and stopped using compost completely.”
Food safety regulations make it onerous and costly for growers to produce their own compost on site. Also, some growers contend that using compost is risky. As one grower explained, “you're actually bringing in foreign material to your ranch” and risking introducing disease, “So we stick with the [physically heat-treated] pellets…” (Respondent 44a), which add organic matter and nutrients but are intended to be functionally sterile (Chen and Jiang, 2014; Jung et al., 2014). Our interviews tentatively suggest that growers without dedicated food safety staff, like Respondent 44, or with particularly strict buyers, seem to be shifting toward the physically heat-treated pellets or opting to build soil fertility through cover-cropping alone. Food safety reform has also virtually ended the practice of purchasing and applying raw manure to fields (Baur et al., 2016), even during fallow periods, despite its easy availability from cattle ranches in the region and one grower's reminiscence that manure “was just good, cheap stuff” (Respondent 54).
Nonetheless, some growers continue to maintain strong relationships with their compost suppliers, who supply them with excellent documentation that eases the bureaucratic burden (Appendix D, Theme 2 in Supplementary Material). At least one grower in this position believed that applying compost might convey protective benefits: “You want to build your soil up—your organic matter. You'll have healthier crops, and not just quality-wise. A healthy crop means it's not going to have bacteria and things like that” (Respondent 47).
Scientific research on the impacts of compost and manure is mixed and appears to support both growers who view these soil amendments as risky, as well as those who perceive that they boost comprehensive soil health and enhance pathogen suppression. For example, on the one hand, biological soil amendments can present food safety risks if not properly managed (Ferens and Hovde, 2011; Oliveira et al., 2011). Composting via thermal treatment aims to reduce pathogen levels without eliminating beneficial microorganisms (Fuchs, 2010), but low levels of pathogens may survive in the soil for prolonged periods following incorporation (Jiang et al., 2003; Islam et al., 2004). Determining appropriate time intervals between compost application and harvest is challenging, since pathogen persistence depends on field conditions (Suslow et al., 2003; Millner and Reynolds, 2009).
On the other hand, if growers substitute synthetic fertilizers for compost and manures (Lowell et al., 2010), changes in soil fertility and organic matter will likely result in soil microbial communities that are less diverse and less effective at suppressing pathogens (Jones et al., 2019a). This undermines the ability of natural processes to reduce food safety risks. For example, while the direct effects of synthetic fertilizers on pathogen persistence are unknown, other agrochemicals have been shown to increase EHEC survival by reducing the capacity of soil microbiota to naturally suppress pathogens (Staley et al., 2014).
Disjuncture 3: Food Safety Reform Impeding Regional Food Systems Socioeconomic Sustainability
In response to prominent public health crises that threatened the socioeconomic viability of Central Coast produce agriculture, food safety standards were reformed in an effort to restore consumer confidence and ensure the sustainability of the produce industry. However, food safety reforms created conditions that impede socioecological sustainability of regional agriculture. Sustainable agricultural systems not only maintain environmental integrity over time (e.g., biodiversity, ecosystem services) but also human well-being and economic viability (e.g., rural livelihoods, human health) over time (Loos et al., 2014).
There is long-standing concern that the formalization of food safety management and oversight in the fresh produce sector may pose higher compliance costs for small-scale farms that could drive them out of business, potentially intensifying existing trends toward socioeconomic consolidation and concentration (Hassanein, 2011; Karp et al., 2015a). Many food safety compliance costs are fixed relative to farm size, meaning that smaller farms pay relatively more for food safety than larger farms due to economies of scale (Bovay et al., 2018). This cost differential may be partially offset as small farms may (1) qualify for full or partial exemption from FSMA regulations, and (2) sell through direct market channels (e.g., farmer's markets, CSAs, restaurant contracts) that may impose less food safety pressure than do wholesale channels. In this section, we first review the state of knowledge on food safety compliance costs, and then examine recent evidence and findings specific to the effects of differential compliance costs on socioeconomic sustainability in the Central Coast.
Differential Food Safety Compliance Costs
Recent food safety compliance cost estimates produced by USDA's Economic Research Service (ERS) demonstrate, at the national scale, a consistent reduction in cost as a share of farm revenue from 6.8% for the smallest regulated farms to 0.3% for the largest regulated farms (Table 4; Bovay et al., 2018). Ribera et al. (2016) modeled FSMA compliance costs specifically for California farms growing cabbage, melons, spinach, and tomatoes; across the models, FSMA's impact on farm profitability correlated inversely with farm size.
Modeled compliance costs match results from a nationwide ERS farmer survey (Astill et al., 2018; Table 5). According to the survey, larger farms are not only more prepared to comply with the rule, but are also proportionally paying up to seven times less to remain in compliance than their smaller counterparts. In a different national survey of produce farmers (N = 394), Adalja and Lichtenberg (2018b) found that a large farm will proportionally spend 45–90% less on food safety practices than will a farm 10 times smaller (in acreage). They also found that small-scale, self-identified sustainable, and beginning farmers are more likely to be further from compliance with the Produce Rule with respect to water testing, record-keeping, and animal intrusion monitoring than their larger, conventional, and well-established counterparts (Adalja and Lichtenberg, 2018a). Taken together, these findings underscore the scale-based structural bias imposed by food safety standards.
In considering the systemic effect of differential food safety compliance costs, Ribera et al. (2016) warn that the FSMA roll-out could lead to “adverse structural impacts” including “considerably reducing the number of small farms and/or diversity of farms.” In other words, differential compliance costs imply a competitive disadvantage for small-scale farms (which likely also correlate with diversified farms and beginning farmers; see Adalja and Lichtenberg, 2018a). Even though they may not directly compete in the same markets (e.g., wholesale vs. farmer's markets), both small and large-scale farms compete for land and labor, and if food safety impairs the economic viability of small-scale farms, these growers may exit the industry, freeing their land for consolidation by larger farm enterprises.
Growers in the Central Coast also reported that meeting the technical complexity of food safety standards now requires full-time attention and specialized expertise. Whether a given operation can afford to hire dedicated food safety staff has become a dividing line among growers (Appendix F, Theme 1 in Supplementary Material). The largest leafy greens growers in the region spend 60% of their food safety budget on personnel with dedicated food safety responsibilities (Calvin et al., 2017). Many smaller operations cannot hire specialized staff, and in our interviews many growers reported trying to cover the difference by recruiting family members—sons, daughters, and spouses—to take on food safety oversight responsibilities part-time. However, the long-term viability of business models premised on exploiting family labor remains uncertain. Below, we elucidate several ripple effects of differential compliance costs on socioeconomic sustainability and equity in Central Coast agriculture.
Access to Markets
Differential compliance costs pose a barrier for small farms specializing in direct-to-consumer sales (e.g., farmers markets) to scale-up or diversify their economic portfolio by accessing more lucrative wholesale market channels (Appendix F, Theme 2 in Supplementary Material). The abrupt increase in Produce Rule compliance cost at the $500,000 annual sales threshold, at which a farm shifts from qualified exempt to fully covered status, contributes to this barrier by imposing a constraining “glass ceiling” on small farms that might otherwise seek to expand. The cost of securing third-party food safety certification may also constrain small farm growth. As one berry farmer explained, sometimes he has too much fruit to sell just at his farmers markets, but without food safety certification he cannot sell the excess of his highly perishable crop to a local cooler, the first point of aggregation for berries: “We considered [food safety certification] because sometimes we can't sell all the berries… [at] the farmers markets…But it's too expensive, and right now we don't have enough money to afford it” (Respondent 9).
Food safety training requirements for farm workers can also pose a barrier for smaller-scale farms to access a very tight farm labor market, because they must compete with their larger-scale counterparts. Respondent 25 explained that, already, “It's really hard to maintain employees on a small farm because there's not a lot of labor. The labor that is there is going to gravitate toward where they get the most benefits (i.e., a large farm)…” Training costs also experience economies of scale, tipping the competitive edge in the labor market further toward large operations.
Barriers to Entry and Incentives to Exit
In the Central Coast region, high land values and rents already pose a significant barrier for beginning farmers to enter agriculture in the region, especially for farmers of color or immigrant farmers (Calo and De Master, 2016; Calo, 2018). Growers reported that food safety exacerbates barriers to entry. The operations manager for a small-farmer training center explained how his program has tried to defray these costs through a cooperative Group GAP model for food safety certification:
“We're trying to reduce the initial costs to getting started, reduce some of the barriers… Someone coming onto the land here with not a lot of resources and already a lot of fixed costs, startup costs, it's really hard to make a profit anyhow.” (Respondent 25)
However, these types of cooperative compliance initiatives remain rare in the Central Coast, and are difficult to maintain once farmers move out of the program and begin farming independently. At the same time, the additional cost, liability exposure, and bureaucratic time burden of food safety compliance may incentivize some farmers to exit agriculture altogether. For example, one third-generation farmer in his mid-60s expressed his frustration with what he saw as an overwhelming array of new requirements:
“We're one problem away from saying, you know what, then you farm it… I'm doing the best I can… Just tell me you don't want me to farm, and I'll leave” (Respondent 46).
When the additional costs of food safety compliance impel growers to exit agriculture, this process fuels consolidation in the Central Coast because the land tends to go to already-large growing operations that can afford the capital expenditure (see, e.g., Respondent 55 in Appendix F, Theme 3 in Supplementary Material).
“Safe” Land, Land-Use, and Land Access
Compounding the detrimental effects of both consolidation and the widespread preference for “clean” fields, many buyers now prefer crops grown on land far from areas perceived as potential food safety hazards, especially wetlands, riparian areas, and rangeland (Appendix F, Theme 4 in Supplementary Material). Larger growers have access to multiple land parcels, allowing them to flexibly shift planting strategies to grow low-risk crops on “problem” fields; they are also well-positioned in the land leasing market, and can shift their leases to “safer” locations. Smaller-scale growers, on the other hand, have less access to preferred land parcels (see Calo and De Master, 2016), and may face a significant financial loss if they have to abandon a parcel due to adjacent land-use concerns. Moreover, widespread intolerance for proximity to “unsafe” land uses—such as rangeland or conservation easements—can lead to conflicts with neighbors (Appendix F, Theme 5 in Supplementary Material) and may eventually catalyze further homogenization of the Central Coast at the landscape level as “safe” land uses cluster together.
Growers Face Disadvantages Due to Race, Ethnicity, and Language
Factors including race, ethnicity, and language may exacerbate market barriers and barriers to entry for growers who cannot access training resources (e.g., because English is not their primary language), social capital (e.g., do not know from whom to seek assistance), or who are actively discriminated against by auditors, inspectors, or buyers. While not a focus of our respective projects, some growers of color and immigrant growers described barriers they faced (Appendix F, Theme 6 in Supplementary Material):
“There are inspectors who are racist and they do make me struggle, and because it's like with everything else, I have to struggle with that. It's hard, but you have to do this. Wherever you go you have that, they put obstacles, they give a bigger chance to others than to you.” (Respondent 30)
Additionally, food safety standards, guidance, and training documents are written primarily in English. While efforts have been made to translate the materials into Spanish, there are reports of inadequate translations, as well as delays in the availability of translated materials (cooperative extension farm advisor, personal communication, March 7, 2018)9. Exposure to these structural discriminations can disadvantage some growers in navigating food safety requirements and staying in business.
Grower Agency and Decision-Making Power
Major outbreaks impact the entire supply chain, yet neither liability nor the power to decide how best to prevent future outbreaks is evenly distributed. In this section, we compare growers' decision-making power to the level of liability they bear in the case of a food safety incident.
Nationwide, food safety practices are driven by the demands of commercial buyers (Astill et al., 2019). Wholesale or contract growers sell to intermediary aggregators, such as shippers or packing houses, which themselves face pressures from retailers and foodservice companies who in turn face legal liability risk if a consumer gets sick. Buyer demands are reinforced by government regulatory enforcement, but also by growers' concerns over their own liability risk (Astill et al., 2019).
Growers in the Central Coast similarly report that their farm management practices are heavily influenced by the demands of their commercial buyers (Appendix G, Theme 1 in Supplementary Material). “The more they're in control of the crop,” said Respondent 55, “the more they're in control of safety.” Other growers indicated that representatives from their customers are on the ground “pretty much every day” (Respondent 49) to monitor food safety. As an exception that proves the rule, growers selling exclusively direct-to-customer reported facing few, if any, buyer demands for food safety (Appendix G, Theme 2 in Supplementary Material). According to Respondent 12a, “I think there's more direct traceability, because our customers know where they get their food.”
Not every grower is happy with the power that commercial customers wield over farm-level decisions, but as one grower phrased it, “If the retailers want it, we comply, we do it, but it's been a pain in the neck” (Respondent 22). To ease the management burden, growers also tend to maintain a uniform food safety protocol, regardless of crop and often regardless of customer, which means adopting the strictest food safety standard for their entire operation (Appendix G, Theme 3 in Supplementary Material): “It's just in our best interest to do the same standards for everything. We can sleep better at night” (Respondent 54).
Growers also feel the constant threat of liability if something does go wrong (Baur et al., 2017). A nationwide study conducted by USDA's Economic Research Service found that most growers “voiced concerns over the uncertainty regarding their potential liability in the event of a foodborne illness outbreak, even if they had passed third-party food safety audits or met Produce Rule standards. Growers were quick to point out that following best practices does not entirely eliminate the risk of contamination” (Astill et al., 2019). Growers in the Central Coast, at all scales and regardless of market channel or whether they had third-party certifications, expressed similar concerns (Appendix G, Theme 4 in Supplementary Material). Respondent 44a bitterly explained the intensity of the dilemma: “If we get a ****ing hit and somebody gets sick, we're going to get just as ****ing sued as if we didn't do anything and hadn't spent a dime on [food safety].”
The liability threat may be worsening as the latest round of food safety reforms—responding to the 2018 EHEC outbreaks in romaine lettuce—emphasize increased farm-level surveillance, citing a need for supply chain transparency. In a letter to the leafy greens industry following the April 2018 outbreak, FDA officials recommended that produce handlers “adopt traceability best practices and state-of-the-art technology” to quickly and easily trace the origins of leafy greens involved in an outbreak or recall and “determine which farm(s) and growing region are responsible” (Ostroff and Plaisier, 2018, p. 3–4). In September 2018, Walmart (one of the largest produce retailers in the world) announced that all of its leafy greens suppliers would henceforth be required to adhere to the company's new food traceability initiative (Redfield et al., 2018). Using blockchain technology, “All fresh leafy greens suppliers are expected to be able to trace their products back to farm(s) (by production lot) in seconds—not days” (Redfield et al., 2018, p. 1–2).
Central Coast growers are aware of the precarious position that this level of transparency puts them in:
“We have to tell all of our customers exactly where it came from, there's no mystery about where it comes from. So, nobody wants to get named in a lawsuit and you want to show everybody that you're doing as much as you can.” (Respondent 55)
Not only do these surveillance initiatives pass liability risk up the supply chain to growers (see also Appendix A, Theme 5 in Supplementary Material), but these initiatives may also further alienate growers from controlling land management decisions on their own farms. Moreover, this alienation is compounded by the disciplinary function of food safety regulation, which sets up a clear hierarchy between food safety knowledge-makers and knowledge-takers that devalues growers' practical knowledge about the specific risks and tradeoffs on their farm (see Parker et al., 2012). Taken together, these results indicate that growers bear disproportionate liability for food safety outcomes relative to their decision-making power.
Discussion
Building upon previous research on food safety impacts in California's Central Coast region, we have synthesized socioecological data from three related studies to address three questions: How do growers perceive the efficacy of current food safety standards in mitigating food safety risks? How do those perceptions compare to the state of scientific evidence? And, how do food safety requirements impact socioeconomic sustainability? By systematically analyzing social and ecological data together, we have addressed gaps in the literature that have heretofore emphasized studying components of food safety reform either abstractly or in relative isolation. By highlighting the perceptions and experiences of farmers implementing food safety reforms, we have also illuminated ecological and social contradictions that result from three disjunctures between food safety regulations and farming realities in practice: (1) Although some food safety requirements reduce risk, growers question the legitimacy and validity of other requirements which may fail to mitigate risk or even generate new food safety problems; (2) Although food safety standards might formally promote co-management of food safety and environmental protection, in practice food safety requirements can conflict with growers' management approaches, including those linked to environmental sustainability; and (3) While food safety reform arose to ensure the economic sustainability of the produce industry in the face of public health crises, these reforms have fostered impediments to socioeconomic sustainability.
The disjunctures we have identified point to a clear need to shift current food safety approaches toward a more integrated approach that better aligns with farming practices. Our findings suggest that by adhering to an implicit ideal of a zero-risk food supply, extant food safety policy perpetuates cycles of crisis and reform driven by “an impossible struggle to split the world in two—a purified inside protected against a dangerous outside” (DuPuis, 2015, p. 112). A more integrated approach would shift from a narrow emphasis on individual grower compliance and would place farmer knowledge and experience at the center of reforms to create a more effective, equitable, and sustainable food safety practice.
We suggest three specific shifts in the direction of current food safety approaches to address these disjunctures. First, as individual growers currently face a disproportionate share of the accountability for food safety outcomes relative to their economic means and decision-making agency, we contend that institutional mechanisms for covering the costs of compliance be integrated into both state and federal farm policies. Given the significant increased financial burdens on growers—including those associated with product recalls10 and additional labor time (including paperwork, required trainings, sanitation work)—subsidies to offset losses would more equitably distribute the monetary costs of safe food. We suggest that institutional supports be structured such that growers of higher-risk foods in particular become eligible for higher levels of subsidies to offset costs. The higher-risk foods designation should align with the much-anticipated FDA list of high-risk foods when it is released in 2020.
Second, certain legal protections should be provided to farmers to buffer them from liability in situations in which they have complied with applicable standards in good faith. Growers currently face disproportionate personal liability relative to their actual ability to mitigate food safety risks in open agricultural systems. In the 2011 Listeria outbreak in Colorado cantaloupe, for example, litigation costs quickly outstripped the $2.5 million in product coverage held by the farmers, the Jensen Brothers (Linden, 2012). The Jensens eventually declared bankruptcy in the face of this outbreak, in spite of what they claimed was inadequate information from their food safety auditor about their risks (Baur et al., 2017). Our findings suggest that the fear of liability drives much of what several respondents phrased as “overkill” that has been observed when food safety standards are implemented in practice. Producers are under tremendous pressure to “do more” in order to demonstrate their due diligence, regardless of whether that “more” is based in evidence. To alleviate this misdirected incentive and free farmers to concentrate on evidence-based risk mitigation, accountability for safe food (like costs) should be more equitably distributed, with liability protections for those who operate in good faith.
Third, we argue that a shift toward embracing a wider array of ecologically-based food safety practices is warranted. This shift in practices should be supported by a robust and ongoing research agenda that actively encourages participatory studies with farmers, to solicit farmers' experiential knowledge of agroecosystems. As an additional benefit, such a participatory approach may reassure growers that their needs are incorporated into food safety guidelines, which could also improve food safety education outcomes. Emerging research suggests that promoting natural pathogen suppression (that is, establishing beneficial soil microbe communities that resist invasion by pathogens) may offer promising alternatives to the current factory-logic model of farm management for food safety (e.g., see Jones et al., 2019a). Allowing for such alternatives would afford farmers the flexibility to utilize more diverse strategies to achieve food safety that also meet their management needs, rather than perpetuating an overemphasis on controlling biodiversity. The recognition of ecologically-based approaches as a suite of tools to mitigate food safety risks could elevate grower agency, especially for diversified farms that rely heavily on naturally-provided soil quality, pollination, and pest control services.
To support this integrated approach, we conclude with recommendations for future research to inform alternatives to the current approach to food safety reform.
Conclusion
Achieving the aims of conserving biodiversity, improving food safety, and improving farmer livelihoods is possible, but further study of the following research questions is critical to make this feasible:
First, can strategic application of compost and manures simultaneously improve soil quality and food safety? Soil amendments represent a key pathway by which pathogens can enter the farm-field environment [Franz and van Bruggen, 2008; California Leaf Green Products Handler Marketing Agreement (LGMA), 2019]; however, preliminary evidence suggests that soils with significant organic matter and diverse soil microbial communities may suppress pathogens (Jones et al., 2019a). Thus, establishing a sufficient waiting period between compost or manure application and crop harvest could represent a viable pathway for building healthy soils that rapidly suppress any pathogens introduced into farm fields. Which soil bacteria are most suppressive, how much/often soil amendments should be applied, and how long before harvest are all critical questions.
Second, how do insecticides affect food-safety risk? New research suggests that some insects (e.g., fruit flies) can facilitate pathogen spread onto fresh produce (Black et al., 2018). Yet, recent evidence also suggests coprophagous insects (e.g., dung beetles) reduce pathogen contamination and prevent pathogen spread, especially on organic farms where insects are more diverse and abundant (Jones et al., 2019a,b). Moreover, agrochemicals may depress the beneficial soil bacteria that compete with and suppress foodborne pathogens (Staley et al., 2014).
Third, could non-crop vegetation (e.g., hedgerows, grass strips, and adjacent semi-natural vegetation) actually improve food safety? Many growers have been pressured to remove non-crop vegetation and establish “bare-ground buffers” that prevent potential wildlife disease vectors from entering their farm fields (Karp et al., 2015a; Baur et al., 2016). But emerging evidence suggests that non-crop vegetation (i.e., hedgerows) may not promote wildlife intrusion (Sellers et al., 2018) and is not associated with elevated pathogen prevalence (Karp et al., 2015b). On the contrary, it is possible that non-crop vegetation may actually filter and sequester pathogens from runoff (Tate et al., 2006; Knox et al., 2007), mitigating on-farm risk.
Fourth, are there heightened food-safety risks associated with species that remain in more “sterile” farm field environments that follow the factory logic model? Ecological theory predicts that the species most resilient to disturbances may also be the most competent disease vectors (Keesing et al., 2010; Civitello et al., 2015). Thus, promoting biodiversity could actually reduce food safety risk if less competent vectors “dilute out” human commensal species more likely to vector diseases. There is some evidence that more diverse rodent communities are less risky from a food-safety perspective because competent vectors (e.g., deer mice) dominate disturbed, species-poor environments, and are reduced in abundance in more diverse communities (Kilonzo et al., 2013). Whether this observation holds for other taxa (e.g., birds and insects) and in other locations, however, is unknown. If new food safety standards disproportionately burden growers that use diversified practices supporting enhanced biodiversity (Kremen et al., 2012; Kremen and Miles, 2012), food safety reform could result in reduced diversity and resilience, increasing the vulnerability of food safety risks, thereby inducing new food safety crises. ' Fifth, how do trends of farm and market consolidation impact the implementation of food safety regulations, particularly for small-scale growers and beginning farmers? As Delind and Howard (2008) posit, the additional resources required to comply with food safety schemes can leave smaller operations vulnerable, causing them to disappear or to seek alternative markets. Current food safety regulations advantage larger-scale operations that have the greatest capacity to absorb the costs of compliance, further accentuating consolidation trends (Adalja and Lichtenberg, 2018b; Astill et al., 2018; Bovay et al., 2018). Yet research is also needed to examine whether smaller-scale operations may adapt more nimbly to evolving food safety risks, and thus be less vulnerable to various “lock-ins” (see Plumecocq et al., 2018).
Finally, how do food safety reforms differentially affect farmers with diverse ethnic, racial, and socioeconomic backgrounds? How might policy be adapted to address and mitigate any differential impacts? Further, how might equity concerns be better incorporated into a more integrated approach to food safety? Some studies suggest that race and ethnicity can condition land access in our study region (e.g., Calo and De Master, 2016). Our research suggests that race and ethnicity also disproportionately affect growers' interactions with food safety auditors, but more systematic research, particularly on differential implementation costs and management tradeoffs, is needed to assess the prevalence of these sociocultural factors.
Our work here seeks to contribute to ongoing food safety dialogues in ways that attend carefully to the perspectives and experiences of growers at the forefront of implementation of food safety reforms, while also illuminating the disjunctures evident in current food safety practices. As food safety reform increasingly penetrates global production and trade relations, in an effort to align international standards and multinational supply networks, we aim to inform an integrated approach to food safety regulations that is effective, equitable, and sustainable.
Data Availability Statement
All datasets generated for this study are included in the article/Supplementary Material.
Ethics Statement
The studies involving human participants were reviewed and approved by either the University of California, Santa Cruz Institutional Review Board or the University of California, Berkeley Institutional Review Board. Written informed consent for participation was not required for this study in accordance with national legislation and institutional requirements.
Author Contributions
EO, PB, and KD conceived the ideas for this manuscript, contributed interview data and analyses, and primary editors of the manuscript. EO and PB wrote the manuscript, with contributions from KD, DK, and DG, as well as key analytical insights from CK, AE, and AS. All authors reviewed the manuscript and gave approval for publication.
Conflict of Interest
CK is the Field Chief Editor for Frontiers in Sustainable Food Systems and PB is one of the Topic Editors for Conflicts and Compromises between Food Safety Policies and Environmental Sustainability. They were not involved in the peer review of this article.
The remaining authors declare that the research was conducted in the absence of any commercial or financial relationships that could be construed as a potential conflict of interest.
Acknowledgments
We are grateful to the many growers and food safety professionals that allowed us to interview them. We thank E. Cervantes and S. Drobny for assistance with interview transcription, S. Philpott and M. Fairbairn for guidance on one of our (EO) interview guides, and D. Weller for early feedback. This publication was made possible in part by support from USDA NIFA (#2017-67012-26092) and NSF DDRIG (#SES-1431490) to PB; USDA NIFA (#2017-67019-26293) to DK, KD, and DG; USDA Hatch (KY008079) to DG.
Supplementary Material
The Supplementary Material for this article can be found online at: https://www.frontiersin.org/articles/10.3389/fsufs.2019.00102/full#supplementary-material
Footnotes
1. ^In this article we use the term food safety narrowly to mean mitigating the risk that foodborne human pathogens may contaminate fruits and vegetables in the pre-harvest environment.
2. ^For instance, a conventional strawberry farm of 50 acres is expected to yield 4,000–10,000 trays of strawberries per acre, which at an average price of $10 per tray returns $40,000–$100,000 per acre (Bolda et al., 2016). Organic strawberries average $15 per tray, but yields are lower−3,500 to 5,000 trays per acre—and returns are thus between $50,000 and $75,000 per acre (Bolda et al., 2014). Thus a 10-acre strawberry farm, whether organic or conventional, can exceed $500,000 in annual sales, an important threshold for food safety regulations (see section Food Safety Regulatory Reforms Affecting California's Central Coast).
3. ^Marketing agreements are programs initiated by industry but facilitated by USDA and state departments of agriculture to “help producers and handlers work together to solve marketing problems that they cannot solve individually” (see www.ams.usda.gov). The LGMA marks a novel use of such agreements to regulate product safety, a proactive industry work-around reached during a time when federal authority over produce agriculture was virtually non-existent but threatening to rapidly expand (see Shekhar, 2010; Endres and Johnson, 2011). See the California LGMA website: https://lgma.ca.gov.
4. ^Farms with less than $25,000 in annual sales are excluded from the Produce Rule, and farms between $25,000 and $500,000 may be eligible for a qualified exemption (reducing their compliance requirements) if they sell more than half of their product either (a) directly to consumers or (b) to a restaurant or retail food establishment within the same state or within 275 miles from the farm (80 FR 74353).
5. ^The Produce Rule requires that covered farms maintain detailed records of worker trainings [§ 112.30(b)], results of laboratory tests for water samples [§ 112.50(b)], and treatment of biological soil amendments of animal origin [§ 112.60(b)]. Third-party audits require additional records related to all aspects of a farm's food safety management system, including, for example, logs related to monitoring animal intrusion and corrective actions taken.
6. ^By legitimacy we mean the extent to which growers (who are subject to food safety regulation) believe that government and industry regulators have the right and authority to govern what happens on the farm. By validity we mean the extent to which growers believe that the practices they are expected to implement will actually achieve the promised outcome, in this case, mitigating the risk that human pathogens will contaminate food.
7. ^These categories, which also include humans and tools/equipment/buildings, are identified for growers in the Produce Safety Alliance grower training course (Produce Safety Alliance, 2017). The Produce Safety Alliance administers the grower training program officially approved by the FDA for fulfillment of the Produce Safety Rule supervisor training requirements, Rule 21 CFR Subpart C §112.22(c).
8. ^The implementation cost of these measures has not yet been studied in detail. However, an April 2019 article in a local Central Coast newspaper quoted a grower saying, “It's [the new LGMA standard] absolutely necessary…” (Cimini, 2019). However, he added, “Sure, it's going to cost more. It's time, it's labor, it's testing, it's laboratories… It's going to affect the bottom line. This is the cost of doing business. This is the cost of safety.”
9. ^As an example of a typical delay, FDA first published an English-language flowchart to help farmers determine whether they are covered under the Produce Rule in November of 2015 (Coverage and Exemptions/Exclusions for 21 Part 112). The Spanish-language version was not published until July of 2018 (Cobertura y exenciones).
10. ^FDA has the authority to issue a mandatory product recall in the event of an outbreak, which imposes direct economic costs on growers. For example, the agricultural industry in Monterey County lost an estimated $160 million due to outbreak-associated recalls in 2018 (Cuevas and Cimini, 2019).
References
Adalja, A., and Lichtenberg, E. (2018a). Implementation challenges of the food safety modernization act: evidence from a national survey of produce growers. Food Control 89, 62–71. doi: 10.1016/j.foodcont.2018.01.024
Adalja, A., and Lichtenberg, E. (2018b). Produce growers' cost of complying with the Food Safety Modernization Act. Food Policy 74, 23–38. doi: 10.1016/j.foodpol.2017.10.005
Allende, A., and Monaghan, J. (2015). Irrigation water quality for leafy crops: a perspective of risks and potential solutions. Int. J. Environ. Res. Public. Health 12, 7457–7477. doi: 10.3390/ijerph120707457
Astill, G., Minor, T., Calvin, L., and Thornsbury, S. (2018). Before Implementation of the Food Safety Modernization Act's Produce Rule: A Survey of U.S. Produce Growers. Economic Research Service, USDA. Available online at: https://www.ers.usda.gov/publications/pub-details/?pubid=89720 (accessed May 10, 2019).
Astill, G., Minor, T., Thornsbury, S., and Calvin, L. (2019). U.S. Produce Growers' Decisionmaking Under Evolving Food Safety Standards. Economic Research Service, USDA. Available online at: https://www.ers.usda.gov/webdocs/publications/93242/eib-210.pdf (accessed June 11, 2019).
Atwill, E. R. (2008). “Implications of wildlife in E. coli outbreaks associated with leafy green produce,” in Proceedings of the 23rd Vertebrate Pest Conference, 5–6. doi: 10.5070/V423110666
Batáry, P., Báldi, A., Kleijn, D., and Tscharntke, T. (2011). Landscape-moderated biodiversity effects of agri-environmental management: a meta-analysis. Proc. Biol. Sci. 278, 1894–1902. doi: 10.1098/rspb.2010.1923
Baur, P., Driscoll, L., Genet, S., and Karp, D. (2016). Inconsistent food safety pressures complicate environmental conservation for California produce growers. Calif. Agric. 70, 142–151. doi: 10.3733/ca.2016a0006
Baur, P., Getz, C., and Sowerwine, J. (2017). Contradictions, consequences and the human toll of food safety culture. Agric. Hum. Values 34, 713–728. doi: 10.1007/s10460-017-9772-1
Bedard-Haughn, A., Tate, K. W., and van Kessel, C. (2004). Using nitrogen-15 to quantify vegetative buffer effectiveness for sequestering nitrogen in runoff. J. Environ. Qual. 33, 2252–2262. doi: 10.2134/jeq2004.2252
Bender, S. F., Wagg, C., and Heijden, M. G. A. V. D. (2016). An underground revolution: biodiversity and soil ecological engineering for agricultural sustainability. Trends Ecol. Evol. 31, 440–452. doi: 10.1016/j.tree.2016.02.016
Bengtsson, J., Ahnström, J., and Weibull, A. C. (2005). The effects of organic agriculture on biodiversity and abundance: a meta-analysis. J. Appl. Ecol. 42, 261–269. doi: 10.1111/j.1365-2664.2005.01005.x
Benjamin, L., Atwill, E. R., Jay-Russell, M., Cooley, M., Carychao, D., Gorski, L., et al. (2013). Occurrence of generic Escherichia coli, E. coli O157 and Salmonella spp. in water and sediment from leafy green produce farms and streams on the Central California coast. Int. J. Food Microbiol. 165, 65–76. doi: 10.1016/j.ijfoodmicro.2013.04.003
Bennett, S. D., Sodha, S. V., Ayers, T. L., Lynch, M. F., Gould, L. H., and Tauxe, R. V. (2018). Produce-associated foodborne disease outbreaks, USA, 1998–2013. Epidemiol. Infect. 146, 1397–1406. doi: 10.1017/S0950268818001620
Beretti, M. (2009). Challenges to Co-Management of Food Safety and Environmental Protection: A Grower Survey. Salinas, CA: Resource Conservation District of Monterey County.
Beretti, M., and Stuart, D. (2008). Food safety and environmental quality impose conflicting demands on Central Coast growers. Calif. Agric. 62, 1–8. doi: 10.3733/ca.v062n02p68
Beuchat, L. R. (2002). Ecological factors influencing survival and growth of human pathogens on raw fruits and vegetables. Microbes Infect. 4, 413–423. doi: 10.1016/S1286-4579(02)01555-1
Blaauw, B. R., and Isaacs, R. (2014). Flower plantings increase wild bee abundance and the pollination services provided to a pollination-dependent crop. J. Appl. Ecol. 51, 890–898. doi: 10.1111/1365-2664.12257
Black, E. P., Hinrichs, G. J., Barcay, S. J., and Gardner, D. B. (2018). Fruit flies as potential vectors of foodborne illness. J. Food Prot. 81, 509–514. doi: 10.4315/0362-028X.JFP-17-255
Blaikie, N. W. H. (2000). Designing Social Research: The Logic of Anticipation. Malden, MA: Polity Press.
Bolda, M. P., Laura, T., Murdock, J., and Summer, D. A. (2016). Sample Costs to Produce and Harvest Strawberries: Central Coast Region. Santa Cruz, CA; Monterey, CA: University of California Cooperative Extension.
Bolda, M. P., Tourte, L., Klonsky, K., De Moura, R. L., and Tumber, K. P. (2014) Sample Costs to Produce Organic Strawberries: Central Coast. Santa Cruz, CA; Monterey, CA; San Benito Counties: University of California Cooperative Extension.
Bovay, J., Ferrier, P., and Zhen, C. (2018). Estimated Costs for Fruit and Vegetable Producers To Comply With the Food Safety Modernization Act's Produce Rule. Economic Research Service, USDA. Available online at: https://www.ers.usda.gov/publications/pub-details/?pubid=89748 (accessed May 10, 2019).
Brady, M. J., McAlpine, C. A., Miller, C. J., Possingham, H. P., and Baxter, G. S. (2011). Mammal responses to matrix development intensity. Austral. Ecol. 36, 35–45. doi: 10.1111/j.1442-9993.2010.02110.x
California Department of Fish Wildlife (2019). Special Animals List. California Natural Diversity Database. Available online at: https://www.wildlife.ca.gov/Data/CNDDB/Plants-and-Animals (accessed October 31, 2019).
California Department of Food Agriculture (CDFA) (2018). California Agricultural Statistics Review 2017-2018. Sacramento, CA. Available online at: https://www.cdfa.ca.gov/statistics/PDFs/2017-18AgReport.pdf
California Department of Food Agriculture (CDFA) (2017–2018). California Agricultural Statistics Review, 2017–2018. Available online at: https://www.cdfa.ca.gov/statistics/PDFs/2017-18AgReport.pdf (accessed September 22, 2019).
California Food Emergency Response Team (2007). Investigation of an Escherichia coli O157: H7 Outbreak Associated With Dole Pre-Packaged Spinach. California Food Emergency Response Team.
California Leaf Green Products Handler Marketing Agreement (LGMA) (2019). Commodity Specific Food Safety Guidelines for the Production and Harvest of Lettuce and Leafy Greens, Version 16. Available online at: https://lgma-assets.sfo2.digitaloceanspaces.com/downloads/190419-CA-LGMA-Metrics_Accessible_0605.pdf (accessed July 17, 2019).
Callaway, T. R., Edrington, T. S., and Nisbet, D. J. (2014). Isolation of Escherichia coli O157:H7 and Salmonella from migratory brown-headed cowbirds (Molothrus ater), common grackles (Quiscalus quiscula), and cattle egrets (Bubulcus ibis). Foodborne Pathog. Dis. 11, 791–794. doi: 10.1089/fpd.2014.1800
Calo, A. (2018). How knowledge deficit interventions fail to resolve beginning farmer challenges. Agric. Hum. Values 35, 367–381. doi: 10.1007/s10460-017-9832-6
Calo, A., and De Master, K. T. (2016). After the incubator: factors impeding land access along the path from farmworker to proprietor. J. Agric. Food Syst. Commun. Dev. 6, 111–127. doi: 10.5304/jafscd.2016.062.018
Calvin, L., Jensen, H., Klonsky, K., and Cook, R. (2017). Food Safety Practices and Costs Under the California Leafy Greens Marketing Agreement. USDA Economic Research Service.
Cardinale, B. J., Duffy, J. E., Gonzalez, A., Hooper, D. U., Perrings, C., Venail, P., et al. (2012). Biodiversity loss and its impact on humanity. Nature 486, 59–67. doi: 10.1038/nature11148
Chaplin-Kramer, R., de Valpine, P., Mills, N. J., and Kremen, C. (2013). Detecting pest control services across spatial and temporal scales. Agric. Ecosyst. Environ. 181, 206–212. doi: 10.1016/j.agee.2013.10.007
Chen, H., Grieneisen, M. L., and Zhang, M. (2016). Predicting pesticide removal efficacy of vegetated filter strips: a meta-regression analysis. Sci. Total Environ. 548, 122–130. doi: 10.1016/j.scitotenv.2016.01.041
Chen, Z., and Jiang, X. (2014). Microbiological safety of chicken litter or chicken litter-based organic fertilizers: a review. Agriculture 4, 1–29. doi: 10.3390/agriculture4010001
Cimini, K. (2019). More Stringent Groundwater Testing Aims to Lower Risk of E. coli Infections From Romaine. Salinas, CA. Available online at: https://www.thecalifornian.com/story/news/2019/04/24/california-regulations-groundwater-aim-reduce-e-coli-romaine-lettuce/3551811002/ (accessed July 26, 2019).
Civitello, D. J., Cohen, J., Fatima, H., Halstead, N. T., Liriano, J., McMahon, T. A., et al. (2015). Biodiversity inhibits parasites: broad evidence for the dilution effect. Proc. Natl. Acad. Sci. U.S.A. 112, 8667–8672. doi: 10.1073/pnas.1506279112
Cooley, M. B., Jay-Russell, M., Atwill, E. R., Carychao, D., Nguyen, K., Patel, R., et al. (2013). Development of a robust method for isolation of shiga toxin-positive Escherichia coli (STEC) from fecal, plant, soil and water samples from a leafy greens production region in California. PLoS ONE 8:e65716. doi: 10.1371/journal.pone.0065716
County of Monterey Agricultural Commissioner (2018). Monterey County Crop Report. Salinas, CA. Available online at: https://www.co.monterey.ca.us/government/departments-a-h/agricultural-commissioner/forms-publications/crop-reports-economic-contributions#ag (accessed September 3, 2019).
County of San Benito Agricultural Commissioner (2017). Crop and Livestock Report. Hollister, CA. Available online at: https://www.cosb.us/county-departments/agriculture/crop-report/ (accessed September 3, 2019).
County of Santa Cruz Office of the Agricultural Commissioner (2017). Santa Cruz County Agricultural Crop and Livestock Report. Watsonville, CA. Available online at: https://www.agdept.com/AgriculturalCommissioner/AnnualCropandLivestockReports.aspx (accessed September 3, 2019).
Crohn, D. M., and Bianchi, M. L. (2008). Research priorities for coordinating management of food safety and water quality. J. Environ. Qual. 37, 1411–1418. doi: 10.2134/jeq2007.0627
Cuevas, E., and Cimini, K. (2019). Monterey County's Ag Industry Sees $160M Loss After E. coli Outbreaks. Salinas, CA. Available online at: https://www.thecalifornian.com/story/news/2019/06/25/monterey-county-agricultural-crop-values-decline-due-e-coli-outbreaks-romaine-lettuce/1563060001/ (accessed July 17, 2019).
Delind, L. B., and Howard, P. H. (2008). Safe at any scale? Food scares, food regulation, and scaled alternatives. Agric. Hum. Values 25, 301–317. doi: 10.1007/s10460-007-9112-y
Drinkwater, L. E., Wagoner, P., and Sarrantonio, M. (1998). Legume-based cropping systems have reduced carbon and nitrogen losses. Nature 396, 262–265. doi: 10.1038/24376
DuPuis, M. E. (2015). Dangerous Digestion: The Politics of American Dietary Advice. Oakland, CA: University of California Press. doi: 10.1525/california/9780520275478.001.0001
Eisler, R. (1998). Copper Hazards to Fish, Wildlife, and Invertebrates: A Synoptic Review (Biological Sciences Report No. USGS/BRD/BSR–1997-0002). U.S. Geological Survey, Biological Resources Division. Washington, DC.
Elliott, J. E., Hindmarch, S., Albert, C. A., Emery, J., Mineau, P., and Maisonneuve, F. (2014). Exposure pathways of anticoagulant rodenticides to nontarget wildlife. Environ. Monit. Assess. 186, 895–906. doi: 10.1007/s10661-013-3422-x
Endres, A. B., and Johnson, N. R. (2011). United States Food Law Update: The FDA Food safety modernization act, obesity and deceptive labeling enforcement. Food Law Policy 7, 135–168.
Ferens, W. A., and Hovde, C. J. (2011). Escherichia coli O157:H7: animal reservoir and sources of human infection. Foodborne Pathog. Dis. 8, 465–487. doi: 10.1089/fpd.2010.0673
Frank, C., Kapfhammer, S., Werber, D., Stark, K., and Held, L. (2008). Cattle density and Shiga toxin-producing Escherichia coli infection in Germany: increased risk for most but not all serogroups. Vectorborne Zoonotic Dis. 8, 635–643. doi: 10.1089/vbz.2007.0237
Franz, E., and van Bruggen, A. H. C. (2008). Ecology of E. coli O157:H7 and Salmonella enterica in the primary vegetable production chain. Crit. Rev. Microbiol. 34, 143–161. doi: 10.1080/10408410802357432
Fraser, D., Mouton, A., Serieys, L. E. K., Cole, S., Carver, S., Vandewoude, S., et al. (2018). Genome-wide expression reveals multiple systemic effects associated with detection of anticoagulant poisons in bobcats (Lynx rufus). Mol. Ecol. 27, 1170–1187. doi: 10.1111/mec.14531
Fuchs, J. G. (2010). “Interactions between beneficial and harmful microorganisms: from the composting process to compost application,” in Microbes at Work: From Wastes to Resources, eds H. Insam, I. Franke-Whittle, and M. Goberna (Berlin; Heidelberg: Springer), 213–229. doi: 10.1007/978-3-642-04043-6_11
Gardner, T., and McLaughlin, J. (2008). Campylobacteriosis Outbreak due to Consumption of Raw Peas — Alaska. Available online at: http://citeseerx.ist.psu.edu/viewdoc/download?doi=10.1.1.504.1489&rep=rep1&type=pdf (accessed July 29, 2019).
Gelting, R. J., Baloch, M. A., Zarate-Bermudez, M. A., and Selman, C. (2011). Irrigation water issues potentially related to the 2006 multistate E. coli O157: H7 outbreak associated with spinach. Agric. Water Manag. 98, 1395–1402. doi: 10.1016/j.agwat.2011.04.004
Gennet, S., Howard, J., Langholz, J., Andrews, K., Reynolds, M. D., and Morrison, S. A. (2013). Farm practices for food safety: an emerging threat to floodplain and riparian ecosystems. Front. Ecol. Environ. 11, 236–242. doi: 10.1890/120243
Gonthier, D., Sciligo, A., Karp, D., Lu, A., Garcia, K., Juarez, G., et al. (2019). Bird services and disservices to strawberry farming in Californian agricultural landscapes. J. Appl. Ecol. 56, 1948–1959. doi: 10.1111/1365-2664.13422
Gonthier, D. J., Ennis, K. K., Farinas, S., Hsieh, H.-Y., Iversona, L., Batary, P., et al. (2014). Biodiversity conservation in agriculture requires a multi-scale approach. Proc. R. Soc. Biol. Sci. 281, 20141358–20141358. doi: 10.1098/rspb.2014.1358
Gottlieb, S. (2018). Statement From FDA Commissioner Scott Gottlieb, M.D., On Developments in the Romaine Outbreak Investigation, Recent Outbreaks and the Use of Modern Tools to Advance Food Safety. Washington, DC: US Food and Drug Administration. Available online at: https://www.fda.gov/NewsEvents/Newsroom/PressAnnouncements/ucm612187.htm (accessed March 25, 2019).
Guthman, J. (2004). Agrarian Dreams: The Paradox of Organic Farming in California. Berkeley, CA: University of California Press.
Haddaway, N. R., Brown, C., Eggers, S., Josefsson, J., Kronvang, B., Randall, N., et al. (2016). The multifunctional roles of vegetated strips around and within agricultural fields. A systematic map protocol. Environ. Evid. 5:18. doi: 10.1186/s13750-016-0067-6
Harter, T., Lund, J., Darber, J., Fogg, G., Howitt, R., Jessoe, K., et al. (2012). Addressing Nitrate in California's Drinking Water With a Focus on Tulare Lake Basin and Salinas Valley Groundwater. Report for the State Water Resources Control Board Report to the Legislature. Davis, CA.
Hassanein, N. (2011). Matters of scale and the politics of the Food Safety Modernization Act. Agric. Hum. Values 28, 577–581. doi: 10.1007/s10460-011-9338-6
Havelaar, A. H., Vazquez, K. M., Topalcengiz, Z., Muñoz-Carpena, R., and Danyluk, M. D. (2017). Evaluating the U.S. food safety modernization act produce safety rule standard for microbial quality of agricultural water for growing produce. J. Food Protect. 80, 1832–1841. doi: 10.4315/0362-028X.JFP-17-122
Heath, S. K., Soykan, C. U., Velas, K. L., Kelsey, R., and Kross, S. M. (2017). A bustle in the hedgerow: woody field margins boost on farm avian diversity and abundance in an intensive agricultural landscape. Biol. Conserv. 212, 153–161. doi: 10.1016/j.biocon.2017.05.031
Hornick, M. (2013). LGMA food safety metrics updated. The Packer. Available online at: http://www.thepacker.com/fruit-vegetable-news/marketing-profiles/LGMA-food-safety-metrics-updated-230510191.html (accessed March 29, 2017).
Hosea, R. C. (2000). “Exposure of non-target wildlife to anticoagulant rodenticides in California,” in Proceedings of 19th Vertebr. Pest Conference, 236–244. doi: 10.5070/V419110029
Hutton, S. A., and Giller, P. S. (2003). The effects of the intensification of agriculture on northern temperate dung beetle communities. J. Appl. Ecol. 40, 994–1007. doi: 10.1111/j.1365-2664.2003.00863.x
Islam, M., Doyle, M. P., Phatak, S. C., Millner, P., and Jiang, X. (2004). Persistence of enterohemorrhagic Escherichia coli O157:H7 in soil and on leaf lettuce and parsley grown in fields treated with contaminated manure composts or irrigation water. J. Food Prot. 67, 1365–1370. doi: 10.4315/0362-028X-67.7.1365
Jakes, A. F., Jones, P. F., Paige, L. C., Seidler, R. G., and Huijser, M. P. (2018). A fence runs through it: a call for greater attention to the influence of fences on wildlife and ecosystems. Biol. Conserv. 227, 310–318. doi: 10.1016/j.biocon.2018.09.026
Jay, M. T., Cooley, M., Carychao, D., Wiscomb, G. W., Sweitzer, R. A., Crawford-miksza, L., et al. (2007). Escherichia coli in swine near spinach fields and cattle, Central California Coast. Emerg. Infect. Dis. 13, 1908–1911. doi: 10.3201/eid1312.070763
Jiang, X., Morgan, J., and Doyle, M. P. (2003). Fate of Escherichia coli O157:H7 during composting of bovine manure in a laboratory-scale bioreactor. J. Food Prot. 66, 25–30. doi: 10.4315/0362-028X-66.1.25
Jones, M. S., Fu, Z., Reganold, J. P., Karp, D. S., Besser, T. E., Tylianakis, J. M., et al. (2019a). Organic farming promotes biotic resistance to foodborne human pathogens. J. Appl. Ecol. 56, 1117–1127. doi: 10.1111/1365-2664.13365
Jones, M. S., Tadepalli, S., Bridges, D. F., Wu, V. C. H., and Drummond, F. (2015). Suppression of Escherichia coli O157:H7 by dung beetles (Coleoptera: Scarabaeidae) using the lowbush blueberry agroecosystem as a model system. PLoS ONE 10:e0120904. doi: 10.1371/journal.pone.0120904
Jones, M. S., Wright, S. A., Smith, O. M., Besser, T. E., Headrick, D. H., Reganold, J. P., et al. (2019b). Organic farms conserve a dung beetle species capable of disrupting fly vectors of foodborne pathogens. Biol. Control 2019:104020. doi: 10.1016/j.biocontrol.2019.104020
Jung, Y., Jang, H., and Matthews, K. R. (2014). Effect of the food production chain from farm practices to vegetable processing on outbreak incidence. Microb. Biotechnol. 7, 517–527. doi: 10.1111/1751-7915.12178
Karp, D., Baur, P., Atwill, E., Master, K. D., Gennet, S., Iles, A., et al. (2015a). The unintended ecological and social impacts of food safety regulations in California's Central Coast Region. BioScience 65, 1173–1183. doi: 10.1093/biosci/biv152
Karp, D., Gennet, S., Kilonzo, C., Partyka, M., Chaumont, N., Atwill, E., et al. (2015b). Comanaging fresh produce for nature conservation and food safety. Proc. Natl. Acad. Sci. U.S.A. 112, 11126–11131. doi: 10.1073/pnas.1508435112
Karp, D. S., Mendenhall, C. D., Sandí, R. F., Chaumont, N., Ehrlich, P. R., Hadly, E. A., et al. (2013). Forest bolsters bird abundance, pest control and coffee yield. Ecol. Lett. 16, 1339–1347. doi: 10.1111/ele.12173
Karp, D. S., Moses, R., Gennet, S., Jones, M. S., Joseph, S., M'Gonigle, L. K., et al. (2016). Agricultural practices for food safety threaten pest control services for fresh produce. J. Appl. Ecol. 53, 1402–1412. doi: 10.1111/1365-2664.12707
Keesing, F., Belden, L. K., Daszak, P., Dobson, A., Harvell, C. D., Holt, R. D., et al. (2010). Impacts of biodiversity on the emergence and transmission of infectious diseases. Nature 468, 647–652. doi: 10.1038/nature09575
Kilonzo, C., Li, X., Vivas, E. J., Jay-Russell, M. T., Fernandez, K. L., and Atwill, E. R. (2013). Fecal shedding of zoonotic food-borne pathogens by wild rodents in a major agricultural region of the Central California Coast. Appl. Environ. Microbiol. 79, 6337–6344. doi: 10.1128/AEM.01503-13
Klein, A.-M., Brittain, C., Hendrix, S. D., Thorp, R., Williams, N., and Kremen, C. (2013). Wild pollination services to California almond rely on semi-natural habitat. J. Appl. Ecol. 49, 723–732. doi: 10.1111/j.1365-2664.2012.02144.x
Knox, A. K., Tate, K. W., Dahlgren, R. A., and Atwill, E. R. (2007). Management reduces E. coli in irrigated pasture runoff. Calif. Agric. 61, 159–165. doi: 10.3733/ca.v061n04p159
Kremen, C., Iles, A., and Bacon, C. (2012). Diversified farming systems: an agroecological, systems-based alternative to modern industrial agriculture. Ecol. Soc. 17:44. doi: 10.5751/ES-05103-170444
Kremen, C., and Miles, A. (2012). Ecosystem services in biologically diversified versus conventional farming systems: benefits, externalities, and trade-offs. Ecol. Soc. 17, 40–40. doi: 10.5751/ES-05035-170440
Kremen, C., Williams, N. M., Bugg, R. L., Fay, J. P., and Thorp, R. W. (2004). The area requirements of an ecosystem service: crop pollination by native bee communities in California. Ecol. Lett. 7, 1109–1119. doi: 10.1111/j.1461-0248.2004.00662.x
Kross, S. M., Kelsey, T. R., McColl, C. J., and Townsend, J. M. (2016). Field-scale habitat complexity enhances avian conservation and avian-mediated pest-control services in an intensive agricultural crop. Agric. Ecosyst. Environ. 225, 140–149. doi: 10.1016/j.agee.2016.03.043
Langholz, J. A., and Jay-Russell, M. T. (2013). Potential role of wildlife in pathogenic contamination of fresh produce. Hum. Wildl. Interact. 7, 140–157. doi: 10.26077/e5gg-r037
Lejeune, J., Homan, J., Linz, G., and Pearl, D. L. (2007). “Role of the European starling in the transmission of E. coli O157 on dairy farms,” in Proceedings of 23rd Vertebrate Pest Conference, 31–34. doi: 10.5070/V423110392
Letourneau, D. K., Allen, S. G. B., and Stireman, J. O. III. (2012). Perennial habitat fragments, parasitoid diversity and parasitism in ephemeral crops. J. Appl. Ecol. 49, 1405–1416. doi: 10.1111/1365-2664.12001
Lewis Ivey, M. L., and Miller, S. A. (2013). Assessing the efficacy of pre-harvest, chlorine-based sanitizers against human pathogen indicator microorganisms and Phytophthora capsici in non-recycled surface irrigation water. Water Res. 47, 4639–4651. doi: 10.1016/j.watres.2013.04.037
Li, M. I. N., Baker, C. A., Danyluk, M. D., Belanger, P., Boelaert, F., Cressey, P., et al. (2018). Identification of biological hazards in produce consumed in industrialized countries: a review. J. Food Prot. 81, 1171–1186. doi: 10.4315/0362-028X.JFP-17-465
Linden, T. (2012). Liability Insurance: How Much Is Enough? Prod. News - Cover. Fresh Prod. Globe 1897. Available online at: http://producenews.com/news-dep-menu/test-featured/7945-liability-insurance-how-much-is-enough (accessed July 29, 2019).
Loos, J., Abson, D. J., Chappell, M. J., Hanspach, J., Mikulcak, F., Tichit, M., et al. (2014). Putting meaning back into “sustainable intensification.” Front. Ecol. Environ. 12, 356–361. doi: 10.1890/130157
López-Gálvez, F., Sampers, I., Gil, M. I., and Allende, A. (2017). Modelling of E. coli inactivation by chlorine dioxide in irrigation water. Agric. Water Manag. 192, 98–102. doi: 10.1016/j.agwat.2017.07.001
Lowell, K., Langholz, J. J., and Stuart, D. (2010). Safe and Sustainable: Co-Managing for Food Safety and Ecological Health in California's Central Coast Region. San Francisco, CA; Washington, DC.
Lynch, M. F., Tauxe, R. V., and Hedberg, C. W. (2009). The growing burden of foodborne outbreaks due to contaminated fresh produce: risks and opportunities. Epidemiol. Infect. 137, 307–315. doi: 10.1017/S0950268808001969
Lytton, T. D. (2019). Outbreak: Foodborne Illness and the Struggle for Food Safety. Chicago, IL; London: University of Chicago Press. doi: 10.7208/chicago/9780226611716.001.0001
M'Gonigle, L. K., Ponisio, L. C., Cutler, K., and Kremen, C. (2015). Habitat restoration promotes pollinator persistence and colonization in intensively managed agriculture. Ecol. Appl. 25, 1557–1565. doi: 10.1890/14-1863.1
Millner, P., and Reynolds, S. (2009). High tunnel and organic horticulture: compost, food safety, and crop quality. HortScience 44, 242–245. doi: 10.21273/HORTSCI.44.2.242
Minor, T., Hawkes, G., McLaughlin, E. W., Park, K. S., Calvin, L., Hawkes, G., et al. (2019). Food Safety Requirements for Produce Growers: Retailer Demands and the Food Safety Modernization Act, EIB-206. Available online at: https://www.ers.usda.gov/webdocs/publications/92761/eib-206.pdf?v=6869.6
Morandin, L. A., Long, R. F., and Kremen, C. (2016). Pest control and pollination cost-benefit analysis of hedgerow restoration in a simplified agricultural landscape. J. Econ. Entomol. 109, 1020–1027. doi: 10.1093/jee/tow086
Nguyen-The, C., Bardin, M., Berard, A., Berge, O., Brillard, J., Broussolle, V., et al. (2016). Agrifood systems and the microbial safety of fresh produce: Trade-offs in the wake of increased sustainability. Sci. Total Environ. 562, 751–759. doi: 10.1016/j.scitotenv.2016.03.241
Olaimat, A. N., and Holley, R. A. (2012). Factors influencing the microbial safety of fresh produce: a review. Food Microbiol. 32, 1–19. doi: 10.1016/j.fm.2012.04.016
Oliveira, M., Usall, J., Viñas, I., Solsona, C., and Abadias, M. (2011). Transfer of Listeria innocua from contaminated compost and irrigation water to lettuce leaves. Food Microbiol. 28, 590–596. doi: 10.1016/j.fm.2010.11.004
Ostroff, S., and Plaisier, M. K. (2018). Letter to State Officials and the Leafy Greens Industry on Environmental Assessment of Romaine Lettuce Outbreak. Silver Spring, MD: US Food and Drug Administration. Available online at: https://www.fda.gov/media/117511/download (accessed June 7, 2019).
Pachepsky, Y., Shelton, D., Dorner, S., and Whelan, G. (2016). Can E. coli or thermotolerant coliform concentrations predict pathogen presence or prevalence in irrigation waters? Crit. Rev. Microbiol. 42, 384–393. doi: 10.3109/1040841X.2014.954524
Painter, J. A., Hoekstra, R. M., Ayers, T., Tauxe, R. V., Braden, C. R., Angulo, F. J., et al. (2013). Attribution of foodborne illnesses, hospitalizations, and deaths to food commodities by using outbreak data, United States, 1998-2008. Emerg. Infect. Dis. 19, 407–415. doi: 10.3201/eid1903.111866
Park, S., Navratil, S., Gregory, A., Bauer, A., Srinath, I., Jun, M., et al. (2013). Generic Escherichia coli contamination of spinach at the preharvest stage: effects of farm management and environmental factors. Appl. Environ. Microbiol. 79, 4347–4358. doi: 10.1128/AEM.00474-13
Parker, J. S., Wilson, R. S., LeJeune, J. T. III., and Doohan, D. (2012). An expert guide to understanding grower decisions related to fresh fruit and vegetable contamination prevention and control. Food Control 26, 107–116. doi: 10.1016/j.foodcont.2011.12.025
Penrod, K., Hunter, R., and Merrifield, M. (2001). “Missing linkages: restoring connectivity to the California landscape,” in Proceedings of Conference on San Diego Zoo (San Diego, CA).
Phillips, B. M., Anderson, B. S., Hunt, J. W., Siegler, K., Voorhees, J. P., Tjeerdema, R. S., et al. (2012). Pyrethroid and organophosphate pesticide-associated toxicity in two coastal watersheds (California, USA). Environ. Toxicol. Chem. 31, 1595–1603. doi: 10.1002/etc.1860
Plumecocq, G., Debril, T., Duru, M., Magrini, M.-B., Sarthou, J. P., and Therond, O. (2018). The plurality of values in sustainable agriculture models: diverse lock-in and coevolution patterns. Ecol. Soc. 23:21. doi: 10.5751/ES-09881-230121
Poessel, S. A., Breck, S. W., Fox, K. A., and Gese, E. M. (2015). Anticoagulant rodenticide exposure and toxicosis in coyotes (Canis latrans) in the Denver Metropolitan Area. J. Wildl. Dis. 51, 265–268. doi: 10.7589/2014-04-116
Pollack, S. (2001). “Consumer demand for fruit and vegetables: the U.S. example,” in Changing Structure of Global Food Consumption and Trade. Agricultural Trade Report WRS-01-1, ed A. Regmi (Washington, DC: U.S. Department of Agriculture), 49–54.
Ponisio, L. C., de Valpine, P., M'Gonigle, L. K., and Kremen, C. (2019). Proximity of restored hedgerows interacts with local floral diversity and species' traits to shape long-term pollinator metacommunity dynamics. Ecol. Lett. 22, 1048–1060. doi: 10.1111/ele.13257
Ponisio, L. C., M'Gonigle, L. K., and Kremen, C. (2016). On-farm habitat restoration counters biotic homogenization in intensively managed agriculture. Glob. Change Biol. 22, 704–715. doi: 10.1111/gcb.13117
Produce Safety Alliance (2017). Grower Training Course Manual, Version 1.1. Available online at: https://producesafetyalliance.cornell.edu/curriculum/ (accessed July 24, 2019).
Redfield, C., Mundo, M., Mounce, R., and Yiannas, F. (2018). Walmart Food Traceability Initiative, Letter fo Fresh Leafy Green Suppliers. Available online at: https://corporate.walmart.com/media-library/document/leafy-greens-food-safety-traceability-requirements-supplier-letter/_proxyDocument?id=00000166-0c8a-d96e-a3ff-8ffe51e30001 (accessed March 25, 2019).
Ribera, L. A., Yamazaki, F., Paggi, M., and Seale, J. L. (2016). The Food safety modernization act and production of specialty crops. Choices 31, 1–7. Available online at: http://www.jstor.org/stable/choices.31.1.06
Schulte, L. A., Niemi, J., Helmers, M. J., Liebman, M., Arbuckle, J. G., James, D. E., et al. (2017). Prairie strips improve biodiversity and the delivery of multiple ecosystem services from corn – soybean croplands. Proc. Natl. Acad. Sci. U.S.A. 114, 11247–11252. doi: 10.1073/pnas.1620229114
Sellers, L. A., Long, R. F., Jay-Russell, M. T., Li, X., Atwill, E. R., Engeman, R. M., et al. (2018). Impact of field-edge habitat on mammalian wildlife abundance, distribution, and vectored foodborne pathogens in adjacent crops. Crop Prot. 108, 1–11. doi: 10.1016/j.cropro.2018.02.005
Serieys, L. E. K., Foley, J., Owens, S., Woods, L., Boydston, E. E., Lyren, L. M., et al. (2013). Serum chemistry, hematologic, and post-mortem findings in free-ranging bobcats (Lynx rufus) with notoedric mange. J. Parasitol. 99, 989–996. doi: 10.1645/12-175.1
Shekhar, V. (2010). Produce exceptionalism: examining the leafy greens marketing agreement and its ability to improve food safety. J. Food Law Policy 6, 267.
Staley, Z. R., Rohr, J. R., Senkbeil, J. K., and Harwood, V. J. (2014). Agrochemicals indirectly increase survival of E. coli O157:H7 and indicator bacteria by reducing ecosystem services. Ecol. Appl. 24, 1945–1953. doi: 10.1890/13-1242.1
Strawn, L. K., Gröhn, Y. T., Warchocki, S., Worobo, R. W., and Bihn, E. A. (2013). Risk factors associated with Salmonella and Listeria monocytogenes contamination of produce fields. Appl. Environ. Microbiol. 79, 7618–7627. doi: 10.1128/AEM.02831-13
Stuart, D. (2008). The illusion of control: industrialized agriculture, nature, and food safety. Agric. Hum. Values 25, 177–181. doi: 10.1007/s10460-008-9130-4
Stuart, D. (2009). Constrained choice and ethical dilemmas in land management: environmental quality and food safety in California agriculture. J. Agric. Environ. Ethics 22, 53–71. doi: 10.1007/s10806-008-9129-2
Stuart, D., and Worosz, M. R. (2012). Risk, anti-reflexivity, and ethical neutralization in industrial food processing. Agric. Hum. Values 29, 287–301. doi: 10.1007/s10460-011-9337-7
Suslow, T. V., Oria, M. P., Beuchat, L. R., Garrett, E. H., Parish, M. E., Harris, L. J., et al. (2003). Production practices as risk factors in microbial food safety of fresh and fresh-cut produce. Compr. Rev. Food Sci. Food Saf. 2, 38–77. doi: 10.1111/j.1541-4337.2003.tb00030.x
Tate, K. W., Atwill, E. R., Bartolome, J. W., and Nader, G. (2006). Significant Escherichia coli attenuation by vegetative buffers on annual grasslands. J. Environ. Qual. 35, 795–805. doi: 10.2134/jeq2005.0141
Thorne, J. H., Dick, C., and Quinn, J. F. (2006). A conservation design for the Central coast of California and the evaluation of mountain lion as an umbrella species. Natural Areas J. 26, 137–148. doi: 10.3375/0885-8608(2006)26137:ACDFTC2.0.CO;2
Topalcengiz, Z., Strawn, L. K., and Danyluk, M. D. (2017). Microbial quality of agricultural water in Central Florida. PLoS ONE 12:e0174889. doi: 10.1371/journal.pone.0174889
Truchado, P., Gil, M. I., Suslow, T., and Allende, A. (2018). Impact of chlorine dioxide disinfection of irrigation water on the epiphytic bacterial community of baby spinach and underlying soil. PLoS ONE 13:e199291. doi: 10.1371/journal.pone.0199291
Turner, K., Moua, C. N. O. U., Hajmeer, M., Barnes, A., and Needham, M. (2019). Overview of leafy greens–related food safety incidents with a California link: 1996 to 2016. J. Food Prot. 82, 405–414. doi: 10.4315/0362-028X.JFP-18-316
United States Department of Agriculture National Agricultural Statistics Service. (2019). “Chapter 2: County level data,” in 2017 Census of Agriculture, Vol. 1. Available online at: https://www.nass.usda.gov/Publications/AgCensus/2017/Full_Report/Volume_1,_Chapter_2_County_Level/California/ (accessed September 4, 2019).
US Centers for Disease Control (CDC) (2008). Multistate Outbreak of E. coli O157:H7 Infections Linked to Romaine Lettuce (Final Update, June 28, 2019). Available online at: https://www.cdc.gov/ecoli/2018/o157h7-04-18/index.html (accessed September 6, 2019).
US Centers for Disease Control (CDC) (2018). Multistate Outbreak of E. coli O157:H7 Infections Linked to Romaine Lettuce (Final Update, June 28, 2018). Available online at: https://www.cdc.gov/ecoli/2018/o157h7-04-18/index.html (accessed September 6, 2019).
US Centers for Disease Control (CDC) (2019). Outbreak of E. coli Infections Linked to Romaine Lettuce (Final Update, January 9, 2019). Available online at: https://www.cdc.gov/ecoli/2018/o157h7-11-18/index.html (accessed June 7, 2019).
US Food Drug Administration (FDA) (1998). Guidance for Industry: Guide to Minimize Microbial Food Safety Hazards for Fresh Fruits and Vegetables. Available online at: http://www.fda.gov/food/guidanceregulation/guidancedocumentsregulatoryinformation/produceplantproducts/ucm064574.htm
US Food Drug Administration (FDA) (2019). Investigation Summary: Factors Potentially Contributing to the Contamination of Romaine Lettuce Implicated in the Fall 2018 Multi-State Outbreak of E. coli O157:H7. Available online at: https://www.fda.gov/Food/RecallsOutbreaksEmergencies/Outbreaks/ucm631243.htm (accessed March 27, 2019).
Uyttendaele, M., Jaykus, L. A., Amoah, P., Chiodini, A., Cunliffe, D., Jacxsens, L., et al. (2015). Microbial hazards in irrigation water: standards, norms, and testing to manage use of water in fresh produce primary production. Compr. Rev. Food Sci. Food Saf. 14, 336–356. doi: 10.1111/1541-4337.12133
Valcour, J. E., Michel, P., McEwen, S., and Wilson, J. (2002). Associations between indicators of live- stock farming intensity and incidence of human Shiga toxin-producing Escherichia coli infection. Emerg. Infect. Dis. 8, 252–257. doi: 10.3201/eid0803.010159
Verbruggen, P., and Havinga, T. (2016). The rise of transnational private meta-regulators. Tilburg Law Rev. 21, 116–143. doi: 10.1163/22112596-02102003
Ward, A. (2019). New, More Stringent Food Safety Practices Adopted to Prevent Outbreaks. Calif. LGMA. Available online at: https://lgma.ca.gov/news/new-more-stringent-food-safety-practices-adopted-to-prevent-outbreaks (accessed October 29, 2019).
Weil, R. R., and Magdoff, F. (2004). “Significance of soil organic matter to soil quality and health,” in Soil Organic Matter in Sustainable Agriculture, eds F. Magdoff and R. R. Weil (Boca Raton, FL: CRC Press), 1–42. doi: 10.1201/9780203496374.ch1
Wells, S. J., Fedorka-Cray, P. J., Dargatz, D. A., Ferris, K., and Green, A. (2001). Fecal shedding of Salmonella spp. by dairy cows on farm and at cull cow markets. J. Food Prot. 64, 3–11. doi: 10.4315/0362-028X-64.1.3
Wilkes, G., Edge, T., Gannon, V., Jokinen, C., Lyautey, E., Medeiros, D., et al. (2009). Seasonal relationships among indicator bacteria, pathogenic bacteria, Cryptosporidium oocysts, Giardia cysts, and hydrological indices for surface waters within an agricultural landscape. Water Res. 43, 2209–2223. doi: 10.1016/j.watres.2009.01.033
Keywords: food safety, California, agriculture, human pathogen, sustainability, socio-ecological system
Citation: Olimpi EM, Baur P, Echeverri A, Gonthier D, Karp DS, Kremen C, Sciligo A and De Master KT (2019) Evolving Food Safety Pressures in California's Central Coast Region. Front. Sustain. Food Syst. 3:102. doi: 10.3389/fsufs.2019.00102
Received: 30 July 2019; Accepted: 21 October 2019;
Published: 12 November 2019.
Edited by:
Christos Damalas, Democritus University of Thrace, GreeceReviewed by:
Karl Matthews, Rutgers, The State University of New Jersey, United StatesKalmia Kniel, University of Delaware, United States
Copyright © 2019 Olimpi, Baur, Echeverri, Gonthier, Karp, Kremen, Sciligo and De Master. This is an open-access article distributed under the terms of the Creative Commons Attribution License (CC BY). The use, distribution or reproduction in other forums is permitted, provided the original author(s) and the copyright owner(s) are credited and that the original publication in this journal is cited, in accordance with accepted academic practice. No use, distribution or reproduction is permitted which does not comply with these terms.
*Correspondence: Elissa M. Olimpi, ZW9saW1waSYjeDAwMDQwO2dtYWlsLmNvbQ==; Patrick Baur, cGJhdXImI3gwMDA0MDtiZXJrZWxleS5lZHU=
†These authors have contributed equally to this work