- 1Department of Engineering - Air Quality Engineering, Aarhus University, Aarhus, Denmark
- 2Faculty of Science and Engineering, Wolverhampton, United Kingdom
- 3Department of Agroecology, Aarhus University, Aarhus, Denmark
The largest source of ammonia (NH3) emissions to the atmosphere is NH3 from agriculture, the majority of which arise from livestock manure. The NH3 emitted is a threat to human health through the formation of fine particles, causes eutrophication of natural ecosystems and is a loss of fertilizer nitrogen (N). The Convention on Long-Range Transboundary Air Pollution (CLRTP) and the European Union National Emission Ceilings Directive (NECD) sets limits for national NH3 emissions and require the reporting of annual emission inventories to demonstrate compliance. The EMEP/EEA Air Pollutant Emission Inventory Guidebook provides emission factors (EFs) to support inventory compilation. Here we report the development of revised NH3 EFs for livestock housing, manure storage, field-applied manure and excreta deposited during grazing. Data from 276 studies were used with more than 70% of these data from peer reviewed journals, the remaining being from conference proceedings and scientific reports. For most sources, the new EFs are the weighted means of the emissions reported. The empirical ALFAM model was used to develop EFs for field-applied slurry. The standard deviation of the EFs were substantial, due to the breadth of the categories of livestock and management systems and because of variations in manure management and climate. The data collected will be available at http://www.alfam.dk.
Introduction
Ammonia (NH3) emissions from livestock manure and mineral fertilizers constitute a large loss of reactive nitrogen (N) (Amann et al., 2013). Agriculture is the largest source of NH3 in the atmosphere (Sailesh et al., 2013) and global agricultural NH3 emissions increased by 90% between 1970 and 2005.
The NH3 emitted is a threat to human health, because it reacts with acidic compounds in the atmosphere (Walker et al., 2006), forming fine particles (PM2.5) that cause lung diseases (Wang et al., 2017). Ammonia-N deposited to land or waters may exceed the critical N loads of the ecosystems causing eutrophication (e.g., Sutton et al., 2011).
Emissions of NH3 from livestock manure account for a large but very variable proportion of the plant-available N in the manure, from a few percent to 100% of TAN (e.g., Hafner et al., 2018, 2019). For farmers, the loss of fertilizer N due to NH3 emissions may significantly reduce N fertilizer efficiency, contributing to low overall use efficiency of applied N, i.e., <50% in the tropics and <70% in temperate areas (Malhi et al., 2001). Experiments on commercial farms in Denmark have shown that N use efficiency of the ammonium () in manure is highly variable (Olesen et al., 2009). Consequently, farmers tend to underestimate the fertilizer value of manure, so that the total available N applied (manure + mineral fertilizer) may exceed crop requirements. This increases fertilizer costs, contributes to nitrate pollution of drinking water and aquatic ecosystems, and increases emissions of NH3 (Sutton et al., 2011).
Concern over the harmful consequences of NH3 emissions led to the adoption in 1999 of the Gothenburg Protocol of the UNECE Convention on Long-range Transboundary Air Pollution (CLRTAP; UNECE, 2019). The European Union has since enacted the National Emission Ceilings Directive (NECD; EP, 2016), which brings CLRTAP into the EU's legal framework, including the NH3 emission ceilings applied to the Member States. Both CLRTAP and NECD require that the EU countries must submit an annual NH3 emission inventory and this calls for models that can be used for calculating national emissions.
The EMEP/EEA air pollutant emission inventory guidebook (“the Guidebook,” European Environment Agency, 2016) provides guidance to European emission inventory compilers on methods for estimating emissions of compounds encompassed by CLRTAP and NECD, including NH3. The methodologies are divided into Tiers of increasing complexity and detail and for key sources such as NH3, the use of a Tier 2 or higher methodology is mandatory. The emission factors (EFs) were last systematically reviewed in 2008 and with the limited resources available at the time, the EFs were based on the expert judgement of the EAGER group of researchers (http://www.eager.ch/). The aim of this review was to provide state of art EFs for the Tier 2 calculation of NH3 emission from the manure management chain. Emission factors have been derived for several livestock categories (e.g., dairy cows, sows, finishing pigs etc.) and for solid or liquid manures. This review was undertaken as part of the revision of the 2016 version of the Guidebook (European Environment Agency, 2016), and the EFs are primarily relevant for European livestock systems but may be applicable to equivalent systems in similar climates.
Method
Data were collected from articles published in peer reviewed journals and from proceeding articles presented at international conferences or published in national reports.
The EFs are expressed as a function of TAN to enable a mass-flow approach to calculation NH3 emissions so that calculations of emissions during manure storage and following manure application will take account of differences in emissions at early steps in manure management, e.g., housing and storage (Webb and Misselbrook, 2004).
Housing
The variation in the units used to report NH3 emissions from livestock housing complicates the summary of these data. Emissions may be reported as: a % of total ammoniacal nitrogen (TAN = NH3+) or total N excreted, g N per animal per day, g N per animal per hour, g N per livestock unit (LU) per day etc. The Supplementary Material lists emission data in a range of units as given in the original articles. For compatibility with the Guidebook, it was necessary to express the emission in % of TAN per year. To convert data from these formats, the following procedure was adopted:
• If data were already expressed in % of TAN excreted then data were used unchanged.
• For poultry, emissions expressed in % of N-total excreted were converted to TAN by calculating the fraction in excreta that is TAN or in a short time will be transformed to TAN (i.e., uric acid).
• If emission was related to number of head of livestock then the national value of N excretion per head (reference source) was used to obtain N excretion and TAN in the excreta.
• If livestock units (LU) were used then EF were related to the annual average weight of an animal in that livestock class and excretion then the emission was estimated using national excretion data.
Where N excretion by the livestock could not be derived from the paper and where national data on average annual N excretion for that class of livestock were not available, the excretion data from Velthof et al. (2015) were used to calculate the amount of Total N excreted per head of livestock for the livestock category. The rationale for using these data was that they report excretion calculated using the IPCC (2006) Guidelines, in which N-excretion is the difference between N intake and the N retention in livestock and livestock products. To calculate the TAN excretion, the ratio of TAN to total—N excreted in the Guidebook (European Environment Agency, 2016) was used.
If the emission was given per LU and number of animals per LU could not be calculated because average weight of animals in the livestock category were not given, then the EUROSTAT definition of number of animals per LU was used (Eurostat, 2013).
If emission was given in heat producing units then one HPU was taken to equal the animal's heat production of 1,000 W at environmental temperature of 20°C. For a dairy cow of 600 kg that produce about 30–35 L milk that will be ca. 1.45 HPU per LU (CIGR, 2002; Zhang et al., 2005).
There is a wide range of housing categories in Europe and emission from livestock housing is much affected by floor design, manure removal, cleaning etc. In the Tier 2 NH3 emission methodology (European Environment Agency, 2016) the calculation scheme is simplified in recognition that data on the number of livestock in each production system are often sparse. Therefore, EFs are only calculated for the main, indicative, categories of livestock production and manure management systems and are presented in tables with standard deviation of the mean (SD). In addition a weighted average for each category has been calculated to reflect the number of farms used in each study: i.e., if a study reported an emission from four farms then that mean emission was allocated four times the weight of a mean emission measured at a single farm. No other factors, e.g., duration of the measurement period, were used to weight the mean EF.
In contrast to non-ruminant livestock, it is common for ruminant livestock to be grazed. The length of the grazing season varies across Europe and within countries. Cattle will usually graze outdoors when the weather allows sufficient grass growth to feed the animals and when soil conditions are dry enough for the animals to graze the pasture and not damage the soil. Hence in general cattle will be grazing during the 'summer' period (April to October) and housed over winter (November to March). However, in regions where summers are too dry for grass growth cattle may be housed during that season. The purpose of the Guidebook (European Environment Agency, 2016) is to provide guidance and default EF for countries that have not developed their own methods and EF to enable the calculation of national NH3 emissions. Hence we based the default EF on “typical” cattle rearing systems with summer grazing and winter housing. If such systems do not represent those used in a country then that country is encouraged to develop appropriate EF.
The default EFs for NH3 emissions from buildings housing cattle reported in this paper were calculated on the basis that both dairy and beef cattle are outside for 180 days during summer. This assumption is based on expert judgement.
Data from housing and management systems that differ significantly from EU systems or from studies where the aim was to reduce NH3 emission have been omitted from the calculations, because the EF should contribute to calculations of NH3 emission from standard housing and management systems. In some cases we could not calculate the excretion of N and the data from these studies were not included in the calculations.
Manure Storage
In most publications the annual emission of NH3 from liquid manure stores was given as g m−2 y−1. Here the emission was calculated as a % of stored TAN, and related to a store with the depth 3 m and an average of TAN concentration in the studies from where data was collated.
In the calculation of NH3 emission from solid manure the EF was related to TAN in the manure at initiation of the storage period. Data from measurements of emission using small dynamic chambers in the laboratories provide useful information about the relative effect of manure treatments on emissions (Perazzolo et al., 2015; Owusu-Twum et al., 2017), but were omitted from the calculations on the grounds that the absolute values measured will not be representative of values found in practice.
Field Applied Manure
The EFs for slurry applied in the field were estimated using the ALFAM2 model, which takes into account the slurry dry matter (DM), application rate, temperature and rain at or immediately after the time of application. The development of Tier 2 EFs for these sources therefore requires an estimate of slurry characteristics and the seasonal distribution of applications, so the environmental conditions required as model inputs can be obtained. For slurry we concluded that many areas in Europe with medium to high intensity agriculture are designated Nitrate Sensitive Zones and so obliged to follow good agricultural practices. Consequently, slurry will be applied at times of the year when there is a crop present that can utilize the manure nutrients. EU regulation limits how early slurry can be applied but most closed periods end between mid-January and mid-February and max N-application rate is limited to 170 kg ha−1 (Webb et al., 2013), and the majority of liquid manure applied to arable crops will be in the spring. A small proportion is applied pre-sowing to winter arable crops that can take up the readily available inorganic N in slurry. Unlike arable crops, grassland is harvested several times per year, and slurry is applied to grassland throughout the growing season. However, the growth potential of grassland is greatest in the spring and the residual effects of manure applications can be utilized most effectively during the remainder of the growing season, so good agricultural practice would be to apply a large proportion of liquid slurry in the spring. Using expert judgement, the timing of manure applications was specified for Spring (March-May), Summer (June-August), Autumn (September to November), and Winter (December-February) (Table 1).
The distribution over the year to grassland and arable land is estimated knowing that ruminant livestock manure N accounts for about 60% of European production and non-ruminant 40% (European Union, 2018a,b) and it is assumed that 80% of ruminant manure is applied to grassland and the remaining 20% to arable (mainly silage maize). All non-ruminant manure is applied to arable land. This means that about 50% of all manure is applied to arable crops and 50% to grassland. And by weighing the application times slurry application can be distributed over the year (Table 2).
We distinguished between cool, temperate and warm climates, equating approximately to Scandinavia and the Baltic countries, the remainder of Europe north of the Alps/Carpathian mountains and Europe south of the Alps/Carpathian mountains, respectively. The conditions at the start of the growing season in the cool and temperate regions are likely to be similar but to occur later in the former than in the latter (Table 3). In the warm region, the situation is more complex. In areas where rainfall is adequate or irrigation is possible, there is likely to be double cropping. This may lead to more manure being applied in the autumn and for the spring application to be made when the air temperature is higher than would be the case in the other two regions. Where irrigation is not possible, the application pattern for grassland is likely to resemble that for arable cropping, since there will be relatively little summer production. To simplify the situation, we used the same spring/summer/autumn periods as for the two other regions but considered that the temperature is higher. The average air temperatures assumed are shown in Table 3.
Estimating the rainfall at these times is difficult. The annual precipitation in agricultural areas varies from >2,000 mm (W Norway) to 250–500 mm (E Spain) (European Environment Agency, 2018).
A reasonable value of precipitation for the main agricultural areas is about 750 mm per year. We took a simple approach here and use an annual average, based on 750 mm per year (0.085 mm per hour). Similarly, it is difficult to estimate appropriate wind speeds. Here again we took a simple approach; on the basis of a map of wind speed at 50 m (Wind Atlas, 2018), we considered a representative speed to be 5.5 m s−1. The NH3 emission calculations used wind at 2 m as input so the wind speed at 2 m height was calculated assuming a surface roughness length (z0; m) at 0.1 m as follows:
where v is the wind speed (m s−1) at height z (m), and reference wind speed vref (m s−1) at reference height zref (m). This gives an average wind speed at 2.7 m s−1.
Much of the data used to estimate of NH3 emissions from solid manure was obtained from a data file provided by Webb et al. (2012) and studies published since 2012. The values are from measurements made over more than 120 h and include those made using wind tunnels. The emission was given in % of applied TAN. Emission estimates higher than 100% have been omitted from the calculations.
Grazing
There are few studies where NH3 emission from grazing cattle are measured using micrometeorological measurements. Therefore, a few measurements was used in this study, where emissions was measured using wind tunnels with air flow adapted to the wind speed in the environment and where the wind tunnels was moved to include the effect of rain has been used in the calculations.
Results
Most of the studies of emission from buildings used in this study are published in peer reviewed papers (Table 4), while reports make up a large fraction of the data on emissions from stored and applied slurry. Emissions from applied solid manure contribute with most of the reported data. The few studies of emission from grazing have all been published in peer reviewed journals.
The number of studies decreased in the order; fattening finishing pigs in buildings with a slatted floor, dairy cows in buildings with a slatted floor, fatteners (solid floor), laying hen, and broilers (solid and slatted floor) (Table 5). There were data available from fewer than 10 studies for dairy cows (solid floor), tied dairy cows and non-dairy cows (solid floor), sows and piglets (solid floor), and laying hen and turkeys on solid floor (Table 5). The variation in measured EF is about 50% of the average in most studies with the exception of EF from pig buildings and turkey. The estimated EF for dairy cows (slurry), fatteners (slurry), fatteners (solid), and sows and piglets are similar to the factors in the current Guidebook (European Environment Agency, 2016). For the other categories the calculated EF deviate by more than 50% from the estimates given in the current Guidebook.
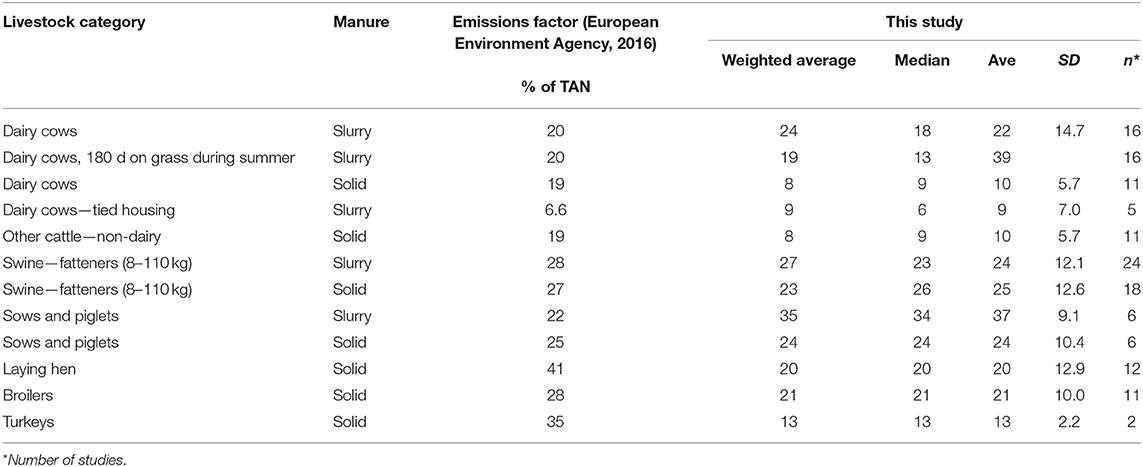
Table 5. Ammonia emission from livestock buildings given as a % of TAN content in the manure stored in the animal house (references to articles providing data for each category can be found in the Excel spreadsheets in the Supplementary Material).
As for building EF, the final means reported here were weighted according to the number of stores from which measurements were taken. The means were not weighted according to the length of the measurement period. There are more studies of emissions from stored solid manure than from stored liquid manure, respectively, 50 and 21 studies (Table 6). The larger number of studies of emission from stored solid manure is not reflected in a low SD in percent of the estimated EF, which with exception of the EF for biogas slurry was higher than 50%. The estimated EF deviated with more than 33% from the guidebook data for dairy cows (solid), other cattle, horses, mules, asses, and laying hen.
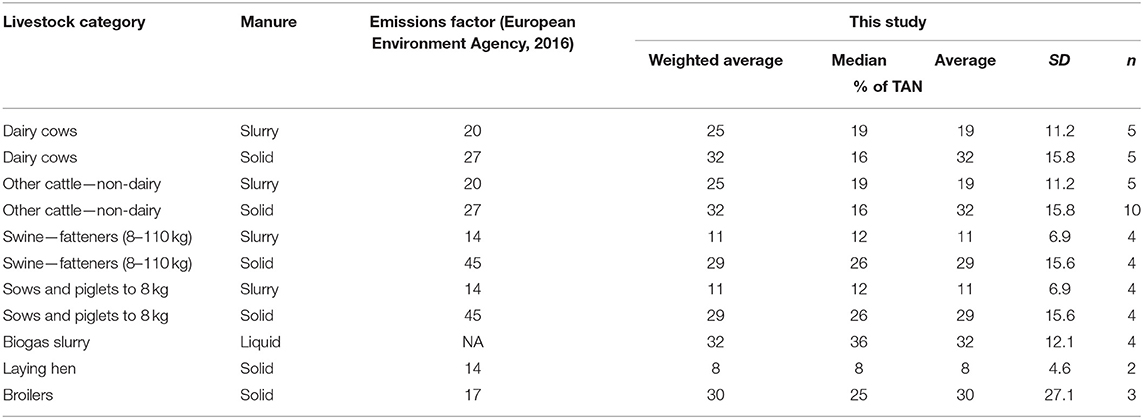
Table 6. Ammonia emission from stored manure given as a % of TAN content in the manure stored outside the animal house (references to articles providing data for each category can be found in the Excel spreadsheets in the Supplementary Material).
The NH3 EFs for pig and cattle slurry applied in the field were calculated for the seasonal temperatures shown in Table 3 and are shown in Table 7. The EF for each region were calculated as weighted averages, using the seasonal distribution shown in Table 2. Finally, the Tier 2 NH3 EFs were calculated using an assumed distribution of slurry between cool, temperate and warm regions of 25, 50, and 25%, respectively. This led to NH3 EFs of 19 and 25% of TAN for pig and cattle slurry, respectively.
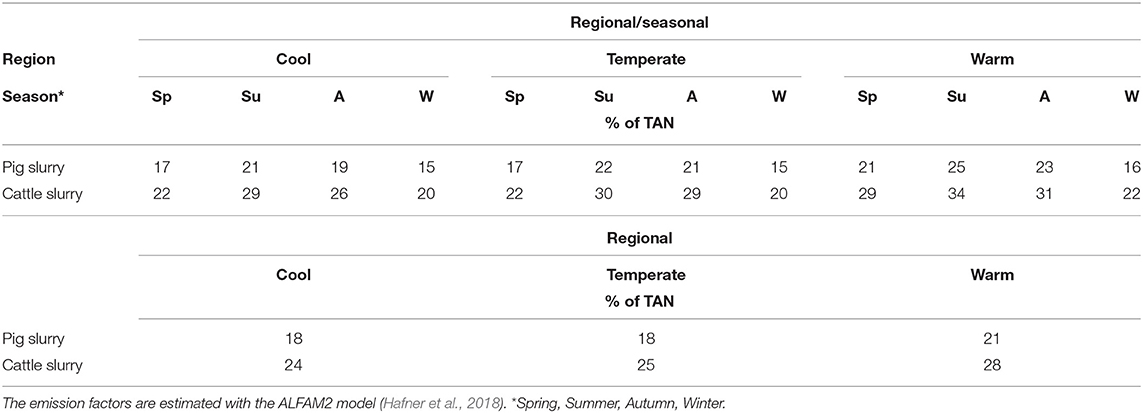
Table 7. Ammonia emission from animal slurry applied in the field given as a % of TAN content in the manure applied in the field.
There is a large number of data from studies measuring emission from solid manure applied in the field (Table 8). For dairy cows and non-dairy solid manure categories the SD is about 30% of the average EF, but for the other categories the SD is <53% of TAN irrespective that the number of studies are between 20 and 32. For the cattle categories the EF is near similar to the EF given in the Guidebook, but for the other categories it differs much more.
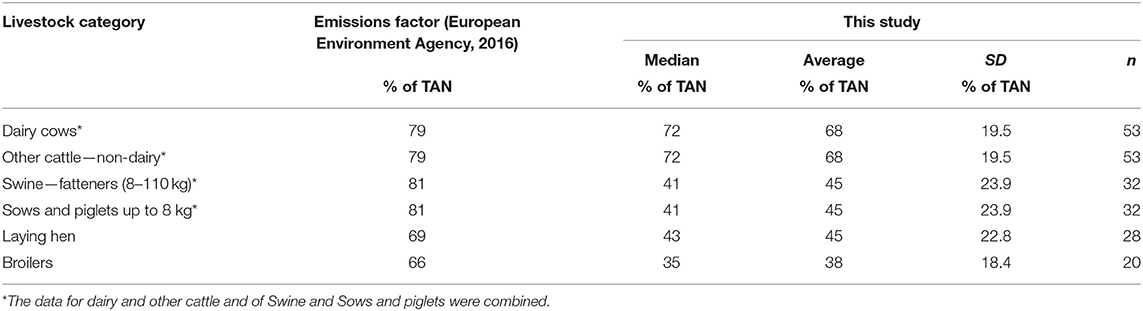
Table 8. Ammonia emission from solid manure applied in the field given as a % of TAN content in the manure applied in the field (references to articles providing data for each category can be found in the Excel spreadsheets in the Supplementary Material).
For grazing cattle the median NH3 emission was 6% of total N excreted, average emission was 9% and SD of the average emission 7% (n = 12). For sows with piglets on grassland the NH3 emission was 19% of N-total (n = 1). In the Guidebook the EF were 10% of TAN for dairy cows, 6% of TAN for non-dairy cattle and 25% of TAN for sows and piglets (European Environment Agency, 2016).
Discussion
This study shows that there is a large variation in the results from studies measuring NH3 emission from buildings, manure stores and following manure application to land. Consequently the EF given have SD that are around 50% of the estimated average for a category of livestock.
The SD of EF for housing may be due to the housing categories being only broadly defined and emission being affected by several factors which vary within each broad category. Those factors are: (1) the ratio of slatted floor to concrete floor area for pigs (Sommer et al., 2006), (2) floor opening area (Philippe et al., 2016), (3) distribution of excreta within the building, which is affected by positioning of feeders and drinkers, and behavior of pigs as related to age and temperature (Aarnink et al., 2006), (4) capacity of in-house storage, (5) age of animals, (6) climate, and (7) feeding practice.
In their review, Philippe et al. (2011) stated “The large range in published NH3 emissions may be attributed to differences in house design (e.g., type of slatted floor, ventilation system, manure management) and management practices (e.g., age and weight at weaning, diet formulation).” This variation in emission caused by livestock housing systems and management was also pointed out by Groot Koerkamp et al. (1998) and the effect of climate by Saha et al. (2014). The large SD is therefore due to a range of factors, which are unaccounted for when calculating an average for the broadly defined categories used to give EF that can be used throughout Europe considering that in many regions little is known about either the proportions of livestock housed with different types of building and manure management practices.
Emission factors from pig buildings in % of TAN have a smaller SD than most other livestock buildings due to the more precise measurements of emissions from the pig buildings with forced ventilation and to some extend less variation in housing systems, feeding practice, and manure management.
The SD for the estimated EF (NH3 in % of TAN) for stored manure was large for both stored slurry and stored solid manure. For stored slurry this may be due to different design of the store, i.e., the depth of stored slurry and climatic conditions and for solid manure it may be an effect of density and water content of the heap (Bernal et al., 2017). In most publications the annual emissions of NH3 from liquid manure stores are given as g NH3 m−2 y−1. This is a meaningful unit, because emission from stored slurry is related to the surface area, in addition to the effect of surface TAN concentration, emission is affected by surface pH, weather etc. The effect of surface to depth is illustrated by emission from a storage diameter 30 m and depth 1 m being similar to the emission from a storage diameter 30 m and depth 3 m, if the composition and climate are similar, but EF for the deep store should be 1/3 of the emission from the shallow one. For solid manure stores we present the EF in percent of TAN at initiation of the storage period, which is not an ideal solution, because transformations between organic N and TAN depend on a range of factors, including carbon to nitrogen (C:N), resilience of organic matter, oxygen content—porosity, size of heap, cover, turning etc. (Bernal et al., 2017). In future EFs should be related to the most important parameters which should include the transformation of organic N to TAN.
Emission from applied slurry is calculated using standard European conditions and is related to slurry DM and application rate, rain intensity and temperature (Hafner et al., 2018; Huijsmans et al., 2018). Soil characteristics will in practice have an effect on emissions (Chantigny et al., 2004; Seçer et al., 2018), but the statistical model does not include soil characteristics, because there was no clear effect of soil type in the analyses of emission data from the 12 countries that provided data for the model, which were from south to north of Europe (Hafner et al., 2018). Losses of NH3 from solid livestock manure applied to soil are not as well-understood as the emissions from slurry application due to the greater variation in the composition of solid manure and there is widely differing practices with respect to litter use and storage, which contribute to the high SD of the average EF.
Ammonia emission from cattle on grassland is highly variable and most of the emission originates from urine patches (Laubach et al., 2012, 2013; Nichols et al., 2018). Increasing addition of N in fertilizer to the grassland will cause an increase in NH3 emission due to increased grass N production, greater intake of N and increasing N excretion of which most ends up in the urine (Jarvis et al., 1989; Bussink, 1992; Voglmeier et al., 2018). Ammonia emissions increase with increasing soil moisture content (Bussink, 1992), and air temperature, wind speed, global radiation, and rainfall all influence emissions (Bell et al., 2017; Voglmeier et al., 2018). The same pattern is seen when studying NH3 emission from sows on grassland, which was related to the amount of feed given to the sows, incident solar radiation, air temperature and rain (Sommer and Hutchings, 2001). These variations are not accounted for with the average EF given in the Guidebook. The few studies carried out do not support a distribution in EF as affected by soil and climate.
The EFs presented here differ in many instances from those in the current Guidebook (European Environment Agency, 2016). This only partly reflects the availability of new results from studies published in the 10 years since the previous systematic update. The resources available for the earlier update were limited, reflecting the lack of appreciation in Europe of the negative effects of NH3 emissions in the past. As a result, the EFs were developed from the values used in the Tier 3 emission inventory methods of a small number of predominantly northern European countries. In the succeeding period, there has been an increase in awareness of the cost of atmospheric NH3 pollution, especially with regards to human health (Giannadaki et al., 2018), and the cost-effectiveness of abatement measures (Backes et al., 2016). This has led to the establishment of more restrictive limits on national NH3 emissions, within the scope of both CLRTP and NECD. This has prompted an awareness among regulators and policymakers of the need for more accurate and more detailed NH3 emission inventories, and to an increase in resources for methodological development.
Changes in Tier 2 EFs varied widely among the different stages of manure management and livestock types (see Tables 5–8), hence the impact of the changes on national Inventories will vary according to the different proportions of livestock classes and manure management systems in each country. The impact of the changes in Tier 2 EFs on total annual emissions from each livestock type can be estimated from the default Tier 1 EFs, expressed as kg(NH3) a−1 AAP−1 (Table 9). These Tier 1 defaults are produced by using the revised Tier 2 EFs from each stage of manure management to calculate NH3 emissions based on standard annual N excretion values for each class of livestock. These revised Tier 1 default EFs can be compared with the previous default Tier 1 EFs to give an estimate of the change in annual NH3 emissions from each class of livestock (Table 9).
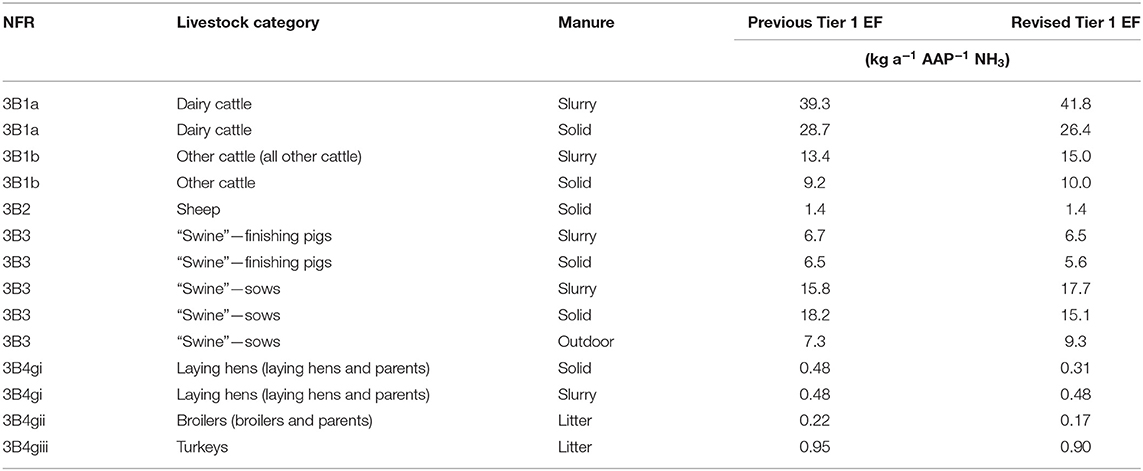
Table 9. Existing guidebook emission factors (European Environment Agency, 2016) and revised emission factors.
Most of the changes to the Tier 2 EFs lead to only small (c. 5–10%) changes in emissions expressed as kg(NH3) a−1 AAP−1 by the Tier 1 default EFs. Moreover, the changes include both increases, generally for livestock systems producing slurry, and decreases, generally for livestock systems producing FYM. There are some substantial reductions in Tier 1 EFs for poultry, but poultry are generally only a small item in national inventories.
Hence increases in total national emissions from countries with a large proportion of livestock systems producing slurry can be expected while total national emissions from countries in which livestock production is dominated by livestock systems producing FYM are expected to be small. For most countries NH3 emissions from livestock production are dominated by emissions from cattle production. Hence total national emissions are expected to increase for countries where cattle are housed in slurry producing systems. It should be noted that the Guidebook EFs are guidelines only and many countries estimate national emissions using a Tier 3 approach in which EFs for each stage of manure management are based on measurements of NH3 emissions from within that country. Hence changes to the Guidebook EFs will not impact on countries using such Tier 3 methodologies. Overall therefore the changes Tier 2 EFs reported here are not expected to make major changes to national emissions estimated using the Guidebook default methodology and none to national emissions estimated using national EFs.
Conclusion
The new NH3 EFs from manure management are derived as weighted means of studies from across Europe, of which most are published in peer-reviewed papers and the application of a model of emission from field-applied slurry. The SD of the average of mean was high, due to the very broad definition of livestock and management systems, and to variations in climate and management practices. As a consequence, national NH3 emission inventories developed using the Tier 2 methodology in the Guidebook will be associated with substantial uncertainty.
Data Availability Statement
The datasets for this study can be found in the ALFAM website (http://www.alfam.dk).
Author Contributions
SS and JW added data to the files used in the data analysis. Data analysis was carried out by SS, JW, and NH. SS wrote the first draft of the article which was then revised by the co-authors.
Funding
The authors wish to acknowledge the contribution made to the funding of this work from the European Environment Agency, under the Framework Contract No. EEA/ACC/13/003 Lot-2.
Conflict of Interest
The authors declare that the research was conducted in the absence of any commercial or financial relationships that could be construed as a potential conflict of interest.
Supplementary Material
The Supplementary Material for this article can be found online at: https://www.frontiersin.org/articles/10.3389/fsufs.2019.00101/full#supplementary-material
References
Aarnink, A. J. A., Schrama, J. W., Heetkamp, M. J. W., Stefanowska, J., and Huynh, T. T. T. (2006). Temperature and body weight affect fouling of pig pens. J. Anim. Sci. 84, 2224–2231. doi: 10.2527/jas.2005-521
Amann, M., Klimont, Z., and Wagner, F. (2013). “Regional and global emissions of air pollutants: recent trends and future scenarios,” in Annual Review of Environment and Resources, Vol. 38, eds A. Gadgil and D. M. Liverman, (Laxenburg: International Institute for Applied Systems Analysis), 31–55. doi: 10.1146/annurev-environ-052912-173303
Backes, A. M., Aulinger, A., Bieser, J., Matthias, V., and Quante, M. (2016). Ammonia emissions in Europe, part II: How ammonia emission abatement strategies affect secondary aerosols. Atmos. Environ. 126, 153–161. doi: 10.1016/j.atmosenv.2015.11.039
Bell, M., Flechard, C., Fauvel, Y., Häni, C., Sintermann, J., Jocher, M., et al. (2017). Ammonia emissions from a grazed field estimated by miniDOAS measurements and inverse dispersion modelling. Atmos. Meas. Tech. 10, 1875–1892. doi: 10.5194/amt-10-1875-2017
Bernal, M. P., Sommer, S. G., Chadwick, D., Qing, C., Guoxue, L., and Michel, F. C. Jr. (2017). Current approaches and future trends in compost quality criteria for agronomic, environmental, and human health benefits. Adv. Agron. 144. 143–233. doi: 10.1016/bs.agron.2017.03.002
Bussink, D. W. (1992). Ammonia volatilization from grassland receiving nitrogen fertilizer and rotationally grazed by dairy cattle. Nutr. Cycl. Agroecosys. 33, 257–265. doi: 10.1007/BF01050881
Chantigny, M. H., Rochette, P., Angers, D. A., Massé, D., and Côte, D. (2004). Ammonia volatilization and selected soil characteristics following application of anaerobically digested pig slurry. Soil Sci. Soc. Am. J. 68, 306–312. doi: 10.2136/sssaj2004.3060
CIGR (2002). Report of Working Group on Climatization of Animal Houses - Heat and Moisture Production at Animal and House Levels. CIGR Section II, Commission Internationale du Genie Rural (International Commission of Agricultural Engineering). Available online at: http://www.cigr.org/ (accessed April 1, 2019).
EP (2016). Directive (EU) 2016/2284 of the European Parliament and of the Council of 14 December 2016 on the Reduction of National Emissions of Certain Atmospheric Pollutants, Amending Directive 2003/35/EC and Repealing Directive 2001/81/EC. Brussels: European Council.
European Environment Agency (2016). EMEP/EEA Air Pollutant Emission Inventory Guidebook 2016 - Technical Guidance to Prepare National Emission Inventories. EEA Report No 21/2016.
European Environment Agency (2018). Average Annual Precipitation. Available online at: https://www.eea.europa.eu/data-and-maps/figures/average-annual-precipitation (accessed June 2019).
European Union (2018a). Livestock population in LSU in the EU-28 and Norway, 2013. png. Available online at: https://ec.europa.eu/eurostat/statistics-explained/index.php?title=File:Livestock_population_in_LSU_in_the_EU-28_and_Norway,_2013.png (accessed April 1, 2019).
European Union (2018b). National Inventory submission under UNFCCC, Table 3.B(b) of CRF. Available online at: https://unfccc.int/documents/65892 (accessed April 1, 2019).
Eurostat (2013). Eurostat Statistics Explained. Glossary Livestock Units (LSU). Available online at: https://ec.europa.eu/eurostat/statistics-explained/index.php/Glossary:Livestock_unit_(LSU) (accessed April 1, 2019).
Giannadaki, D., Giannakis, E., Pozzer, A., and Lelieveld, J. (2018). Estimating health and economic benefits of reductions in air pollution from agriculture. Sci. Total Environ. 622–623, 1304–1316. doi: 10.1016/j.scitotenv.2017.12.064
Groot Koerkamp, P., Metz, J., Uenk, G., Phillips, V., Holden, M., Sneath, R., et al. (1998). Concentrations and emissions of ammonia in livestock buildings in Northern Europe. J. Agric. Eng. Res. 70, 79–95. doi: 10.1006/jaer.1998.0275
Hafner, S. D., Pacholski, A., Bittman, S., Burchill, W., Bussink, W., Chantigny, M., et al. (2018). The ALFAM2 database on ammonia emission from field-applied manure: description and illustrative analysis. Agric. For. Meteor. 258, 66–79. doi: 10.1016/j.agrformet.2017.11.027
Hafner, S. D., Pacholski, A., Bittman, S., Carozzi, M., Chantigny, M., Génermont, S., et al. (2019). A flexible semi-empirical model for estimating ammonia volatilization from field-applied slurry. Atmos. Environ. 199, 474–484. doi: 10.1016/j.atmosenv.2018.11.034
Huijsmans, J. F. M., Vermeulen, G. D., Hol, J. M. G., and Goedhart, P. W. (2018). A model for estimating seasonal trends of ammonia emission from cattle manure applied to grassland in the Netherlands. Atmos. Environ. 173, 231–238. doi: 10.1016/j.atmosenv.2017.10.050
IPCC (2006). “IPCC guidelines for national greenhouse gas inventories, prepared by the National Greenhouse Gas Inventories Programme” in IGES, eds H. S. Eggleston, L. Buendia, K. Miwa, T. Ngara, and K. Tanabe.
Jarvis, S. C., Hatch, D. J., and Roberts, D. H. (1989). The effects of grassland management on nitrogen losses from grazed swards through ammonia volatilization; the relationship to excretal N returns from cattle. J. Agric. Sci. 112, 205–216. doi: 10.1017/S0021859600085117
Laubach, J., Taghizadeh-Toosi, A., Gibbs, S. J., Sherlock, R. R., Kelliher, F. M., and Grover, S. P. P. (2013). Ammonia emissions from cattle urine and dung excreted on pasture. Biogeosciences 10, 327–338. doi: 10.5194/bg-10-327-2013
Laubach, J., Taghizadeh-Toosi, A., Sherlock, R. R., and Kelliher, F. M. (2012). Measuring and modelling ammonia emissions from a regular pattern of cattle urine patches. Agric. Forest Meteor. 156, 1–17. doi: 10.1016/j.agrformet.2011.12.007
Malhi, S. S., Grant, C. A., Johnston, A. M., and Gill, K. S. (2001). Nitrogen fertilization management for no-till cereal production in the Canadian Great Plains: a review. Soil Tillage Res. 60, 101–122. doi: 10.1016/S0167-1987(01)00176-3
Nichols, K. L., Del Grosso, S. J., Derner, J. D., Follett, R. F., Archibeque, S. L., Delgado, J. A., et al. (2018). Nitrous oxide and ammonia emissions from cattle excreta on shortgrass steppe. J. Environ. Qual.47, 419–426. doi: 10.2134/jeq2017.12.0463
Olesen, J. E., Askegaard, M., and Rasmussen, I. A. (2009). Winter cereal yields as affected by animal manure and green manure in organic arable farming. Eur. J. Agron. 30, 119–128. doi: 10.1016/j.eja.2008.08.002
Owusu-Twum, M. Y., Polastre, A., Subedi, R., Santos, S. S., Ferreira, L. M. M., Coutinho, J., et al. (2017). Gaseous emissions and modification of slurry composition during storage and after field application: effect of slurry additives and mechanical separation. J. Environ. Manage. 200, 416–422. doi: 10.1016/j.jenvman.2017.06.004
Perazzolo, F., Mattachini, G., Tambone, F., Misselbrook, T., and Provolo, G. (2015). Effect of mechanical separation on emissions during storage of two anaerobically codigested animal slurries. Agric. Ecosys. Environ. 207, 1–9. doi: 10.1016/j.agee.2015.03.023
Philippe, F.-X., Cabaraux, J.-F., and Nicks, B. (2011). Ammonia emissions from pig houses: influencing factors and mitigation techniques. Agric. Ecosyst. Environ. 141, 245– 260. doi: 10.1016/j.agee.2011.03.012
Philippe, F. X., Laitat, M., Wavreille, J., and Nicks, B. (2016). Floor slat openings impact ammonia and greenhouse gas emissions associated with group-housed gestating sows. Livestock 10, 2027–2033. doi: 10.1017/S1751731116000938
Saha, C. K. L., Ammona, C., Berga, W., Fiedler, M., Loebsin, C., Sanftleben, P., et al. (2014). Seasonal and diel variations of ammonia and methane emissions from a naturally ventilated dairy building and the associated factors influencing emissions. Sci. Tot. Environ. 468–469, 53–62. doi: 10.1016/j.scitotenv.2013.08.015
Sailesh, N., Behera, S. N., Sharma, M., Aneja, V. P., and Balasubramanian, R. (2013). Ammonia in the atmosphere: a review on emission sources, atmospheric chemistry and deposition on terrestrial bodies. Environ. Sci. Pollut. Res. 20, 8092–8131. doi: 10.1007/s11356-013-2051-9
Seçer, M., Elmaci, Ö. L., and Erdemir, O. (2018). Does the potential ammonium fixation of soils have an impact on the optimum nitrogen fertilizer rate? Com. Soil Sci. Plant Anal. 49, 1522–1529. doi: 10.1080/00103624.2018.1474363
Sommer, S. G., and Hutchings, N. J. (2001). Ammonia emission from field applied manure and its reduction – invited paper. Eur. J. Agron. 15, 1–15. doi: 10.1016/S1161-0301(01)00112-5
Sommer, S. G., Zhang, G. Q., Bannink, A., Chadwick, D., Hutchings, N. J., Misselbrook, T., et al. (2006). Algorithms determining ammonia emission from livestock houses and manure stores. Adv. Agron. 89, 261 - 335. doi: 10.1016/S0065-2113(05)89006-6
Sutton, M. A., Oenema, O., Erisman, J. W., Leip, A., van Grinsven, H., and Winiwarter, W. (2011). Too much of a good thing. Nat. Comm. 472, 159–161. doi: 10.1038/472159a
UNECE (2019). Amendment of the Text and Annexes II to IX to the Protocol to the 1979 Convention on Long-range Transboundary Air Pollution to Abate Acidification, Eutrophication and Ground-Level Ozone and the Addition of New Annexes X and XI C.N.155.2013.TREATIES-XXVII-1-h of 28 February 2013 (Adoption of Amendments to Annexes II to IX to the Protocol and addition of annexes X and XI). Available online at: https://treaties.un.org/doc/Publication/CN/2013/CN.155.2013-Eng.pdf (accessed April 8, 2019).
Velthof, G. L., Hou, Y., and Oenema, O. (2015). Nitrogen excretion factors of livestock in the European Union: a review. J. Sci. Food Agric. 95, 3004–3014. doi: 10.1002/jsfa.7248
Voglmeier, K., Jocher, M., Häni, C., and Ammann, C. (2018). Ammonia emission measurements of an intensively grazed pasture. Biogeosciences 15, 4593–4608. doi: 10.5194/bg-15-4593-2018
Walker, J. T., Robarge, W. P., Shendrikar, A., and Kimball, H. (2006). Inorganic PM2.5 at a US agricultural site. Environ. Pollut. 139, 258–271. doi: 10.1016/j.envpol.2005.05.019
Wang, J. F., Yin, Q., Tong, S. L., Ren, Z. P., Hu, M. G., and Zhang, H. R. (2017). Prolonged continuous exposure to high fine particulate matter associated with cardiovascular and respiratory disease mortality in Beijing, China. Atmosph Environ. 168, 1–7. doi: 10.1016/j.atmosenv.2017.08.060
Webb, J., and Misselbrook, T. H. (2004). A mass-flow model of ammonia emissions from UK livestock production. Atmos. Environ. 38, 2163–2176. doi: 10.1016/j.atmosenv.2004.01.023
Webb, J., Sommer, S. G., Kupper, T., Groenestein, K., Hutchings, N. J., Eurich-Menden, B., et al. (2012). Emissions of ammonia, nitrous oxide and methane during the management of solid manures. Sust. Agric. Rev. 8, 67–107. doi: 10.1007/978-94-007-1905-7_4
Webb, J., Sørensen, P., Velthof, G., Amon, B., Pinto, M., Rodhe, L., et al. (2013). An assessment of the variation of manure nitrogen efficiency throughout Europe and an appraisal of means to increase manure N efficiency. Adv. Agron. 119, 371–442. doi: 10.1016/B978-0-12-407247-3.00007-X
Wind Atlas (2018). Global Wind Atlas. Available online at: https://www.globalwindatlas.info (accessed April 1, 2019).
Keywords: ammonia, housing, store, applied, grazing, inventories, slurry, manure
Citation: Sommer SG, Webb J and Hutchings ND (2019) New Emission Factors for Calculation of Ammonia Volatilization From European Livestock Manure Management Systems. Front. Sustain. Food Syst. 3:101. doi: 10.3389/fsufs.2019.00101
Received: 17 June 2019; Accepted: 18 October 2019;
Published: 14 November 2019.
Edited by:
Salvador Calvet Sanz, Polytechnic University of Valencia, SpainReviewed by:
Alberto Sanz Cobeña, Centro de Estudios e Investigación para la Gestión de Riesgos Agrarios y Medioambientales (CEIGRAM), SpainHarald Menzi, Federal Office for the Environment, Switzerland
Copyright © 2019 Sommer, Webb and Hutchings. This is an open-access article distributed under the terms of the Creative Commons Attribution License (CC BY). The use, distribution or reproduction in other forums is permitted, provided the original author(s) and the copyright owner(s) are credited and that the original publication in this journal is cited, in accordance with accepted academic practice. No use, distribution or reproduction is permitted which does not comply with these terms.
*Correspondence: Sven G. Sommer, c2dzQGVuZy5hdS5kaw==