- 1Department of Environmental Science, Policy, and Management, University of California, Berkeley, Berkeley, CA, United States
- 2Department of Natural Resources Environmental Sciences, University of Illinois at Urbana-Champaign, Urbana, IL, United States
- 3Department of Zoology, Institute for Resources, Environment and Sustainability, and Biodiversity Research Centre, University of British Columbia, Vancouver, BC, Canada
Agricultural practices can either contribute to pollinator decline or provide opportunities to support pollinator communities. At the landscape-scale, agriculture can have negative impacts on pollinators, especially pollinators that specialize on limited floral or nesting resources. While increasing floral resources at the field-scale is positive for pollinator communities, little is known about how it affects specialist bees that depend on a specific pollen source (oligoleges). We studied pollinators on small-scale farms that contrasted in crop diversity (monocultures vs. polycultures), embedded in the intensively managed agriculture region of the San Joaquin Valley in California, to understand how wild bee communities and specialist bees would respond to field-scale diversification practices. We used squash (Cucurbita pepo) as our focal crop, because it is visited by both specialist pollinators, “squash bees” in the genera Peponapis and Xenoglossa, and by generalist bees like those in the genera Apis and Agapostemon. We hypothesized that there would be a greater number of squash bees on monoculture farms, which have abundant squash flowers, than on polyculture farms. Contrary to our predictions, we found that increasing the number of non-squash floral resources at the field-scale in agroecosystems supports a greater abundance of squash bees but has no effect on the diversity of bees visiting squash flowers. This pattern of increased abundance was consistent for other wild bees and the total number of bees (i.e., including honey bees), but not for honey bee abundance alone. Further, the abundance of pollinators increased or remained the same on polyculture farms throughout the morning while decreasing on monoculture farms, suggesting that as squash flowers start to close in midmorning, bees on the monocultures go elsewhere because no other floral resources co-occur. However, they remain on the polycultures where other resources co-occur. Thus, on-farm diversification may be an important refuge for both specialist bees and other pollinator species that are vulnerable to floral resource simplification as a result of development, especially through monoculture agriculture.
Introduction
Global change patterns, including habitat alteration and climate change, are transforming ecosystems with negative effects on biodiversity and, thus, reducing ecosystem functioning and compromising ecosystem services (IPBES, 2019). Agriculture, nature's double-edge sword, can either contribute to biodiversity loss or provide opportunities to support biodiversity (Kremen and Merenlender, 2018). Large-scale agriculture intensification in landscapes, including (semi-) natural habitat loss and agrochemical use, have caused insect pollinator decline (Kremen et al., 2002; Biesmeijer et al., 2006; Carvell et al., 2006; Rundlöf et al., 2008; Brittain et al., 2010; Williams et al., 2010; Dupont et al., 2011; Bartomeus et al., 2013; Grab et al., 2019) whereas diversification practices in agricultural landscapes have been shown to support wild bees by providing nesting and foraging resources (Steffan-Dewenter and Leschke, 2003; Morandin and Kremen, 2013; Sardiñas and Kremen, 2015; Kovács-Hostyánszki et al., 2017; Quinn et al., 2017). On-farm diversification practices that provide spatiotemporal floral resource continuity can support pollinator communities (Westphal et al., 2003; Ullmann, 2015), yet little is known about how it affects the diversity and abundance of rarer specialist bees.
Wild bees play a huge role in the pollination of agricultural crops and can be used as a supplement or replacement to managed Apis mellifera (honey bee) colonies, reducing risk associated with honey bee decline or increasing expense for honey bee rentals (Klein et al., 2007; Garibaldi et al., 2013). Specialist wild bees, more notably Peponapis and Xenoglossa spp. (the squash bees), Nomia melanderi (the alfalfa bee), and Diadasia spp. (the sunflower bees), rely on specific crops for floral resources (Cane and Sipes, 2006; Patrício-Roberto and Campos, 2014). In fact, squash bees, specifically Peponapis spp., expanded their range with the domestication and cultivation of squash throughout North America (Giannini et al., 2010; López-Uribe et al., 2016). These squash bees are solitary ground-nesting species that require ground space near to their preferred pollen source for suitable nesting habitat (Hurd et al., 1974). Male bees will sometimes sleep in the flower, while females exclusively rely on Cucurbita spp. in order to provide their offspring an adequate pollen source throughout development (Hurd et al., 1971). Squash plants also depend on squash bees. While both the generalist A. mellifera (non-native to the U.S.) and specialist Peponapis pruinosa (native) are effective pollinators of squash plants, P. pruinosa females synchronize their crepuscular emergence with the opening of the flower, unlike honey bees allowing for sufficient pollination of squash plants without honey bee colonies (Tepedino, 1981). While the importance of specialist pollinators to pollination has long been questioned (e.g., Waser et al., 1996; Larsson, 2005; Maldonado et al., 2013), studies indicate that bees with a narrow pollen diet (oligolecty) are rarer on agroecosystems (Wood et al., 2016; Amy et al., 2018).
Mass-flowering crops are large monocultures that bloom concurrently, providing a bountiful, albeit time-limited, source of nectar and pollen. Research has shown that mass-flowering crops can attract and support their associated specialist and generalist pollinators (Westphal et al., 2003), perhaps even more than surrounding floral resources. Other studies suggest that floral diversity in surrounding landscapes, such as semi-natural areas, or in the immediate field margins, such as hedgerows, are still important in promoting specialist pollinators (Garibaldi et al., 2014; Stanley and Stout, 2014; Kremen and M'Gonigle, 2015; M'Gonigle et al., 2015). Yet, increasing floral diversity in the surrounding landscape to supplement mass-flowering crops may be impossible in landscapes dominated by agriculture or depauperate semi-natural habitats, and on-farm restoration of floral diversity, such as hedgerows, can be difficult for farmers that lack capital to pay for the labor, supplies, and equipment, and time involved in their implementation (Brodt et al., 2006; Garbach and Long, 2017).
A small number of studies of on-farm or field-scale diversification practices across space and time demonstrate that increasing floral resources support a more abundant and diverse pollinator community (Kennedy et al., 2013; Pereira et al., 2015; Venturini et al., 2016; Lindborg et al., 2017; Quinn et al., 2017). However, little is known about how diversification at the field-scale and, thus, greater floral resource diversity, would specifically influence specialist pollinator species of crops. Thus, the lack of previous research provides inconclusive information and point to the importance of investigating whether mass-flowering crops or a diverse crop mixture would better support more specialized crop pollinators.
We therefore explored the role of on-farm diversification in an agriculturally dominated landscape on pollinator communities, with an emphasis on specialists. We compared farms that only provide one floral resource (i.e., squash flowers) and farms that provide a wide range of floral resources (i.e., squash and other flowers). Specifically, we asked: How does farm type (polyculture vs. monoculture) affect specialist pollinators and other groups of bees? How do pollinator communities visiting squash flowers change throughout the day on these two types of farms? We predicted that we would observe a higher number of squash bees on farms that provide a greater amount of squash flowers (i.e., monoculture farms) than those that provide a limited amount of squash flowers (i.e., polyculture farms). We further predicted that the monoculture farms would host lower numbers and diversity of other wild bees (non-squash specializing) compared to polyculture farms, and these trends would be consistent throughout the morning. Lastly, we expected that proportion of surrounding agricultural land would have a minimal effect on the pollinator abundance and diversity given the high proportion of agriculture in the landscape and the low variability of the surrounding landscape among sites.
Materials and Methods
Study System
Field sites were located in Fresno County in California's San Joaquin Valley, an agriculturally dominated region containing a wide range of annual and perennial crops, including row crops and orchards. Natural or semi-natural habitats are sparse or nearly non-existent, except in the foothills of the Coastal Range and Sierra Nevada Range in this region (Kelly et al., 2005). While this region is dominated by large-scale farms, our research focused on small-scale farms (<40 acres) embedded in this landscape. Some of these small-scale farms grow a high diversity of specialty crop together (e.g., squash, Thai peppers, jujube, bittermelon; Molinar, 2012) and frequently rotate these crops over space and time (i.e., “polycultures”). Other small-scale farmers grow only one crop per season of squash or other row crops (i.e., “monocultures”). We sampled on 10 farm sites (5 polyculture farms and 5 monoculture farms) during the late spring of 2018 (May 28 to June 14, 2018; Figures 1A,B). Farms were considered polycultures if they grew 2 or more different crop types and monoculture if they grew 1 crop type at the time of data collection.
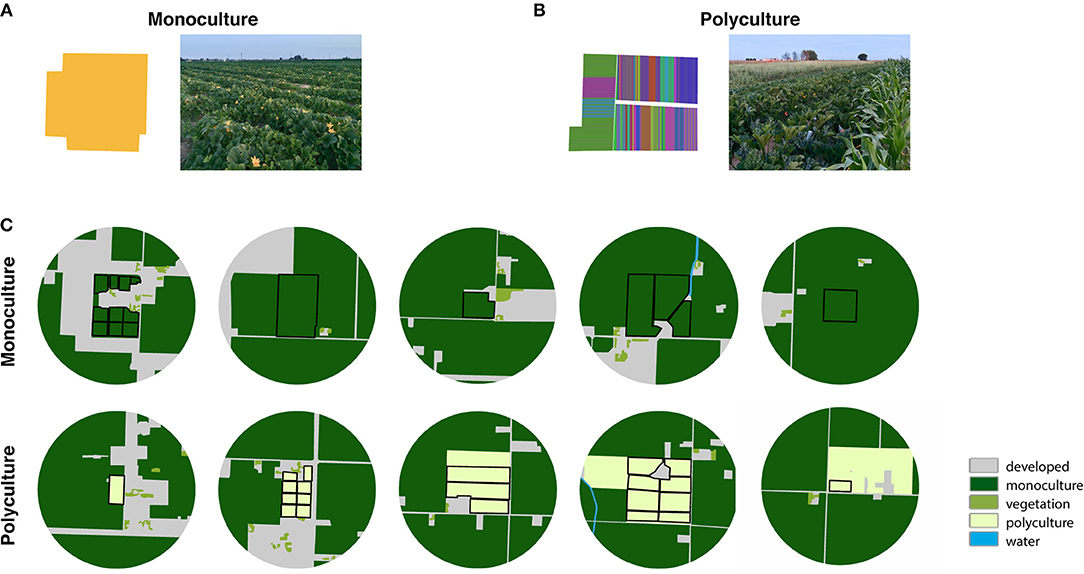
Figure 1. Example photos and hand-digitized crop maps, in which each color represents a different crop type, of (A) a monoculture farm and (B) a polyculture farm to visualize the field-scale variation in the (C) landscape-scale maps (hand-digitized aerial imagery at 500 m buffer from the farm center) of the monoculture and polyculture sites.
Farm sites were selected if they grew at least two rows of a dominant summer squash variety (zucchini, Cucurbita pepo var. cylindrica) and were at minimum 1 km apart from each other, to maintain independence relative to typical bee foraging distances (Greenleaf et al., 2007). Squash plants are entirely dependent on insect pollination (but see possibility of parthenocarpy, Knapp et al., 2017), and have specialist pollinators, known as squash bees (Peponapis spp. and Xenoglossa spp.), allowing us to study the effect of on-farm diversification on specialist and generalist pollinators, separately. In addition, all farm sites had grown squash in monoculture or polyculture the previous year (2017).
Sampling Methods
At each site, we established two parallel 30 m transects perpendicular to the field edge, running along adjacent rows of squash, ~2 m apart (Figure S1). We were limited in where and how far apart our transects could be since some polyculture farms only contained two rows of summer squash and, therefore, the two transects were always on adjacent rows across all sites. In all other cases, all transects were located 5 m from the edge.
We surveyed pollinators during two periods in the morning: just after sunrise (~05:30 a.m.; hereafter “early morning”), when squash bees first become active, and later in the morning (~08:30 a.m.; hereafter “late morning”). Each site was sampled once on 1 day, during squash bloom, on clear days with low wind speeds (~0.5 mph) and cool to warm temperatures (~60 to ~70°F, early to late mornings, respectively). We surveyed bees for 8 min along each transect, not including time needed for voucher specimen handling. Our survey only recorded floral visitors that contacted the anthers and stigmas of squash flowers (i.e., potential pollinators). While we also surveyed other non-bee floral visitors, we did not include them in these analyses. Bees were identified as squash bees (Peponapis spp. and Xenoglossa spp.), honey bees (A. mellifera), or within six categories for observational surveys of wild bees following Kremen et al. (2011). Additionally, we did not distinguish between feral and managed honey bees as there is no physical marker that permits the distinction of these two categories. We netted voucher bee specimens to confirm in-field identification. Voucher specimens were identified by M. Chase.
Landscape Classification
The surrounding landscape at each farm was characterized using the following land-use categories: developed (including home developments, animal feeding operations, warehouses and packing houses, and roads), water (including irrigation canals and streams), vegetation (including home gardens with flowering plants and trees and wind breaks), polyculture agriculture, and monoculture agriculture (Figure 1C). We hand-digitized National Agriculture Imagery Program (NAIP) 2016 imagery for Fresno County within a 500m buffer around farm sites in ArcGIS 10.6.1 (ESRI, 2011), and classified each polygon according to one of these five categories. To determine the effect of agricultural intensity on pollinator communities visiting squash flowers across different farm types, we examined the proportion of agriculture (including both polyculture and monoculture categories) within each buffer per farm site. The proportion of agriculture in the surrounding landscape at the farm sites varied from ~60 to 95%, with a median of 84% (Figure 1B). In this study, we did not choose farm sites based on landscape context.
Statistical Analyses
We analyzed the influence of farm type (polyculture and monoculture farms), landscape context (proportion of agriculture in the surrounding landscape), and time of day (early and late morning) on the abundance and diversity of bee groups using general linear mixed effects models with a random effect of farm site to account for site differences. Specifically, for each of these bee groups [squash bees, wild bees (except honey and squash bees), honey bees, and total bees], we used visits to squash flowers within 8 min to estimate abundances and diversity indices, which included richness (using Chao1 estimator; Chao, 1987), evenness (Evar; Petchey and Gaston, 2006), and Shannon diversity (Magurran, 1988). Bee abundance models assumed a Poisson error distribution and diversity models assumed a Gaussian error distribution. We transformed the diversity indices responses by ln(d+ 1) to ensure residuals of fitted models met assumptions of homoscedasticity and normality. In addition, the residuals of the fitted models had no strong pattern of over-dispersion.
We developed two candidate model sets to test the importance of fixed effects on all response variables: (1) “landscape models”—farm type and landscape context (proportion of agricultural land) as fixed effects (Table S1) and (2) “time of day models”—time of day and farm type as fixed effects (Table S2). The full models included both main effects and a two-way interaction term between both main effects and were compared to simpler models without the interaction, and with one of the two fixed effects alone (Tables S1, S2). All competing models were ranked based on Akaike Information Criterion (AICc). The model from each set with the lowest AICc (i.e., ΔAICc = 0) was considered to be the overall best-fit model. Additionally, models with ΔAICc < 2 were considered important and competing models. For all models, AICc values were used to calculate associated Akaike weights (w), which represent the likelihood the model would be the best-fit model in repeated runs of the experiment (Anderson and Burnham, 2002). We report statistics and Akaike weights for covariates of the overall best-fit models and competing models. All statistical analyses were performed using R software version 3.5.2 (R Core Team, 2018) and model selection for mixed models was conducted using a combination of the “lme4” package (Bates et al., 2017) and the “MuMIn” package for model averaging of coefficients (Barton and Barton, 2015).
Results
Bee Abundance
A total of 590 bees, of which 31% were squash bees, 13% were other wild bees, and 56% were honey bees, were observed across all farm sites and survey rounds (early and late morning). Squash bee abundance ranged from 0 to 37, other wild bee abundance ranged from 0 to 50, and honey bee abundance ranged from 0 to 58 across all farm sites and survey rounds.
Landscape Models
Bee abundances were affected by farm type, proportion of agriculture land, and their interaction. Squash bee abundance was best explained by the model that included only farm type (w = 0.506) and a competing model that included the interaction between farm type and the proportion of agriculture (w = 0.331). There was a greater number of squash bees visiting squash flowers on polyculture farms than monoculture farms (farm type: β = 2.126 ± 0.541, P < 0.001; Figure 2A). In addition, as the proportion of agriculture in the surrounding landscape increased, more squash bees visited squash flowers on polyculture farms than monoculture farms (farm type x proportion of agricultural land:: β = 8.122 ± 3.427, P = 0.018; Figure 3A).
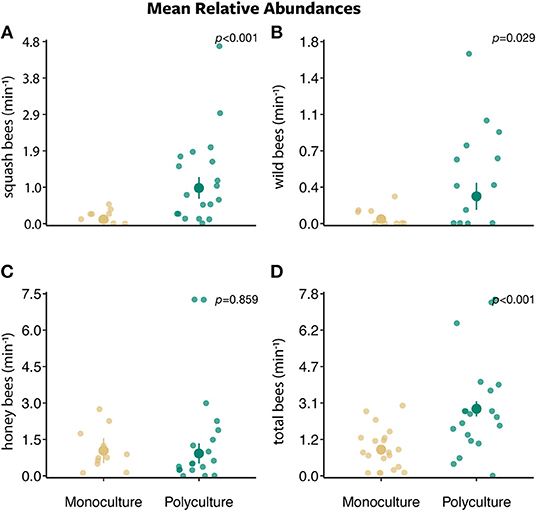
Figure 2. The mean relative abundances ± standard error of (A) squash bees, (B) wild bees, (C) honey bees, and (D) total bees per minute along a 30 m transect between two farm types (polyculture and monoculture).
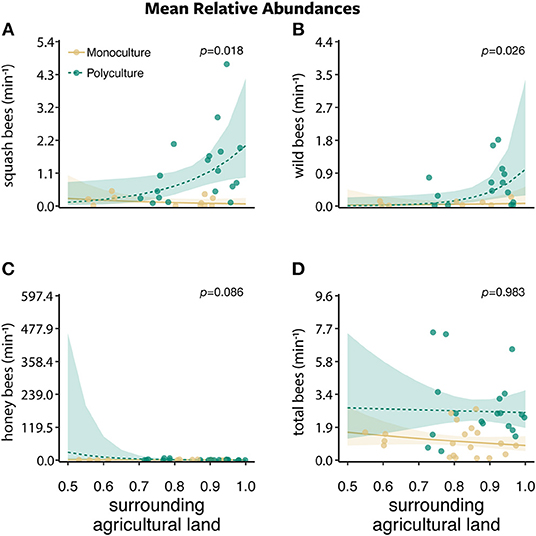
Figure 3. The mean relative abundances ± standard error of (A) squash bees, (B) wild bees, (C) honey bees, and (D) total bees per minute along a 30 m transect between two farm types (polyculture and monoculture) across the proportion of surrounding agricultural land.
For wild bees (i.e., all bees except squash and honey bees), all models in the wild bee model set were strongly supported (ΔAICc = 0–1.730; w = 0.121–0.287; Figures 2–4 and Figures S2, S3). The top model included main effects of both farm type and proportion of agricultural land (w = 0.326; Table S1), but no interaction. There was a greater number of wild bees visiting squash flowers on polyculture farms (farm type: β = 1.488 ± 0.745, P = 0.046; Figure 2B) and a positive marginal effect of the proportion of agricultural land on the number of wild bees (proportion of agricultural land: β = 6.356 ± 3.442, P = 0.065; Figure S2B).
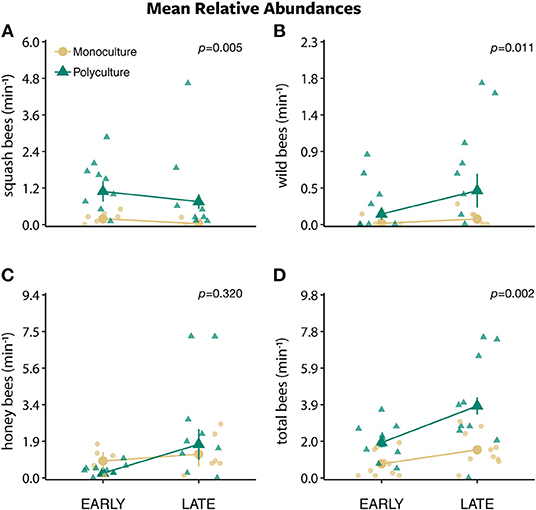
Figure 4. The mean relative abundances ± standard error of (A) squash bees, (B) wild bees, (C) honey bees, and (D) total bees per minute along a 30 m transect between two farm types (polyculture and monoculture) across early morning (sunrise) and late morning (~8:30 a.m.).
Honey bee abundance was best supported by both the null model (w = 0.373) and the model with proportion of agricultural land only (w = 0.339; Table S1). However, the effect of the proportion of agricultural land on honey bee abundance was marginal at best (proportion of agricultural land: β = −4.079 ± 2.586, P = 0.115; Figure S2C).
The models that best explained total bee abundance included the effect of farm type only (w = 0.635; Table S1). The top model demonstrated a strong effect of farm type on total bee abundance, in which the number of total bees visiting squash flowers was greater on polyculture farms than monoculture farms (farm type: β = 0.839 ± 0.217, P < 0.001; Figure 2D). A competing model with both main effects of farm type and the proportion of agricultural land (w = 0.264; Table S1) also provided support that total bee abundance was greater on polyculture than monoculture farm sites (farm type: β = 0.906 ± 0.219, P < 0.001).
Time of Day Models
The models that included the effect of time of day and farm type plus their interaction best predicted squash bee (w = 0.672), honey bee (w = 1.000), and total bee (w = 0.969) but not wild bee (w = 0.165) abundances (Table S2). The number of squash bees remained the same throughout out the morning (from early to late morning) on polyculture farms while decreasing in numbers on monoculture farms (interaction: β = 1.428 ± 0.764, P = 0.062; Figure 4A). The number of honey bees and total bees remained the same from early to late morning on monoculture farms, while increasing on polyculture farms (interaction: β = 1.634 ± 0.277, P < 0.001 and β = 0.654 ± 0.206, P = 0.002, respectively; Figures 4C,D). Wild bee abundance was best explained by the model with time of day only (w = 0.636; Table S2), which predicted that there was no difference in the number of wild bees from early to late morning (time of day: β = −0.015 ± 0.124, P = 0.905; Figure S3B).
Bee Community Diversity
Apart from squash bees and honey bees, a total of six different groups of wild bees were observed in the pollinator survey conducted across farm sites. Based on these groups, Shannon diversity ranged from 0.245 to 0.836, richness ranged from 2 to 4, and evenness ranged from 0.332 to 0.998 across all farm sites surveyed.
Landscape Models
The effect of farm type, proportion of agricultural land, or the interaction had little support when compared to the null models for richness, Shannon diversity, and evenness (respectively, w = 0.564, w = 0.663, w = 0.615; Table S2). For the richness and evenness of bees visiting squash flowers, the model testing only the effect proportion of agricultural land was within two AICc units of the null model although this effect was not significant (proportion of agricultural land: β = 0.426 ± 0.366, P = 0.841 and β = −0.002 ± 0.638, P = 0.808, respectively).
Time of Day Models
For time of day models, we did not find support for the effect of time of day, farm type, and the interaction when compared to the null model on richness, Shannon diversity, and evenness (respectively, w = 0.798, w = 0.809, w = 0.864; Table S2). In addition, none of the models were within two AICc units of the null model (Table S2).
Discussion
Although we hypothesized that squash monoculture fields would provide a substantial amount of pollen resources for specialist squash bees and thus have high squash bee abundances, in contrast, our results suggest that on-farm diversification supports a higher abundance of squash specialist bees (Peponapis spp. and Xenoglossa spp.), other wild bees, and honey bees. Even in an intensively managed landscape like California's San Joaquin Valley, on-farm diversification may support pollinator communities, including native bees, despite the absence of natural habitats and the high dependence on managed honey bees for pollination (Kremen et al., 2002; Durant, 2018; Goodrich, 2019). Most likely a combination of factors, including floral resource availability through space and time, potentially other management practices such as reduced tillage and agrochemical use, promoted pollinator abundances on polyculture farms.
Our results suggest that multiple floral resources through space and time, as exhibited in our study system on squash polyculture farms, is beneficial even for rarer, specialist bees. These findings confirm a previous meta-analysis showing that agriculture intensity decreases specialist pollinator communities (Williams et al., 2010); our results, to the best of our knowledge, further demonstrate that on-farm diversification, despite high agricultural intensification in the surrounding landscape promotes pollinator abundance for squash in polycultures. The polyculture farms, in this study system, consist of many non-squash flowering crops intercropped during the same growing season (diversity in space) and across growing seasons (diversity in time). Therefore, while polycultures have less squash plants per farm area potentially aggregating squash bees, polycultures also provide squash bees and other wild bees other non-squash flower resources. Pereira et al. (2015) and Norris et al. (2017) did not study specialist pollinator communities, but the authors did show that intercropping increases the abundance of pollinators by providing additional floral resources. Furthermore, Moeller (2004) proposed that a more temporally continuous supply of diverse floral resources promote pollinator community diversity and stability. This has been supported in several other observational (Burkle et al., 2013; Leong et al., 2015; Ponisio et al., 2015; Aleixo et al., 2017; Kremen et al., 2018) and experimental studies (e.g., Venjakob et al., 2016). Thus, higher and continuous diversity of non-squash floral resources on polycultures could explain our finding that a greater abundance of bees but not diversity are visiting squash flowers.
While a continuous supply of floral resources supports the abundance and diversity of pollinator communities, temporal shifts of the specialized floral resource may still pose challenges for oligolectic bees (Mayer and Kuhlmann, 2004; Minckley et al., 2013; Ogilvie and Forrest, 2017). Instead, some studies suggest that the constancy of a specialized floral resource through time could be important to the stability of specialist pollinators (Greenleaf and Kremen, 2006; Minckley et al., 2013; Scheper et al., 2014; Ullmann, 2015). For example, Ullmann (2015) found higher densities of squash bees (i.e., P. pruinosa) at farm sties with increased connectivity to other squash fields between years. In our study system, both squash monocultures and polycultures should have experienced a boost in the squash bee population given that squash was grown on each farm site the prior year. Yet, we still observe a higher abundance of squash bees and other wild bees visiting squash flowers on polycultures. Therefore, we do not expect that a population boost effect may be playing a role in the result that a higher abundance of squash and other wild bees are visiting squash flowers on polycultures than monocultures.
Throughout the morning, we observed that the number of pollinators (i.e., squash, wild, honey, and total bees) increased or remained the same on polyculture farms, while decreasing on monoculture farms. This suggests that as squash flowers close on monoculture farms, bees travel elsewhere for floral resources, but on polyculture farms, bees stay on the farm and visit other flowers. Bees seek and travel to floral resources because it is important for bees to have sufficient resources (pollen and nectar) to support their caloric plus dietary needs and provide for their young (Fewell and Winston, 1992; Roulston et al., 2000; Francis et al., 2016; Arenas and Kohlmaier, 2019). Similar to our results, other studies have found that bees in more diversified landscapes travel less to obtain the needed resources and conversely, they travel further in more simplified landscapes (Steffan-Dewenter et al., 2002; Jha and Kremen, 2013; Ogilvie and Forrest, 2017; Pope and Jha, 2017). Floral diversity of non-squash crops on polyculture farms likely underlie the positive of effect of polycultures on the abundance of squash bees and other wild pollinators visiting squash plants.
In our study, we also found an interactive effect of the surrounding landscape (i.e., the proportion of agricultural land) and farm type (i.e., polyculture vs. monoculture) on squash bees and honey bees. While our study was not designed to vary surrounding landscape, our models accounted for this variable. We would have included landscape explicitly in the experimental design, but the region of our research, the San Joaquin Valley in California, does not have expansive patches of natural habitat and lacks landscape complexity (Kelly et al., 2005). Thus, the farm at ~60% of surrounding agricultural land was notably exceptional given that the non-agricultural components in that landscape were largely chicken broilers (i.e., a concentrated chicken feeding operations), largely paved and lacked vegetation, and a horse barn, neither agriculture nor housing development. While studies on the effect of landscape composition on bees are substantial (e.g., Kennedy et al., 2013; Motzke et al., 2016), in this study we treat the effect of landscape on the pollinator measurements with caution and caveats because of this potential limitation.
Farm management is important in order to maintain and support biodiversity in agricultural landscapes. In this study, we found a strong effect of diversification in farm practices (i.e., intercropping with squash) on the number of wild pollinators. While we speculate that the driving force in these results is the positive effect of on-farm diversification on pollinator communities, other farming practices such as agrochemical use and tillage could have also been shown to influence the composition of pollinator communities and nesting habitats (Shuler et al., 2005; Bernauer et al., 2015; Goulson et al., 2015; Ullmann, 2015; Hladik et al., 2016; Potts et al., 2016; Kratschmer et al., 2018) including Peponapis (Shuler et al., 2005; Williams et al., 2010; Ullmann et al., 2016). Although our study did not explore these farming practices, polyculture farms in our squash study system tended to disturb the soil less (i.e., reduced tillage) as a result of their intercropping practice. Further, polyculture farmers spot-treated pest outbreaks with pesticides and hand-weeded whereas the monoculture farmers used broadcast pesticide application for pests and weeds.
Agricultural intensification from the field- to the landscape-scale is on the rise (Rudel et al., 2009) and will continue to have adverse effects on pollinator communities. Our results show that on-farm diversification may provide important floral resources for squash specialist bees, and other wild bee communities that face challenges in landscapes dominated by agriculture. In order to reconcile biodiversity conservation and agriculture, agroecosystem management approaches are needed across varying scales to support ecosystem functioning and ecosystem services (Kremen and Merenlender, 2018). Importantly, our results provide evidence that diversifying farmlands in an agriculturally-dominated landscape creates a more suitable matrix for pollinator communities. These findings support that agricultural diversification benefits bee communities, however, few studies, including this study (but see Kremen and M'Gonigle, 2015; M'Gonigle et al., 2015), have examined how wild bee communities differentially respond to a range of agricultural practices, from simplification to diversification, across varying landscapes contexts.
Data Availability Statement
The datasets generated for this study are available on request to the corresponding author.
Author Contributions
AG and CK designed the study. AG and MC collected the data. MC identified the specimens. AG analyzed the data and wrote the first draft of the manuscript. All authors contributed to the final version of the manuscript.
Funding
This research was funded by NSF Graduate Research Fellowship (to AG), Annie's Sustainable Agriculture Scholarship (to AG), UCANR Graduate Student in Extension Fellowship (to AG), and grants from the Army Research Office (W911NF-17-1-0231 to CK) and C.S. Fund (173-010 to CK). Publication made possible in part by support from the Berkeley Research Impact Initiative (BRII) sponsored by the UC Berkeley Library.
Conflict of Interest
The authors declare that the research was conducted in the absence of any commercial or financial relationships that could be construed as a potential conflict of interest.
Acknowledgments
We are grateful to the farmers for collaborating on this project and so graciously sharing their expertise, time, and resources. We are also thankful for the field and lab assistance provided by Gisel DeLaCerda, Marisol Montes, and Paula Yang as well as the support of University of California Kearney Agricultural Research and Extension Center, and Dr. Kent Daane and Dr. Ruth Dahlquist-Willard. The authors are also grateful for the help and valuable comments provided by Dr. Emily Kearney.
Supplementary Material
The Supplementary Material for this article can be found online at: https://www.frontiersin.org/articles/10.3389/fsufs.2019.00087/full#supplementary-material
Table S1. Model parameter estimates (β ± SE) and model selection statistics (ΔAICc and Akaike weights, w) for the “landscape” candidate model set for each response variable (bee group abundances and diversity indices). The model from each set with the lowest AICc (i.e., ΔAICc = 0) was considered to be the overall best-fit model. Akaike weights (w) indicate the likelihood the model would be the best-fit model in repeated runs of the experiment. Bolded values represent overall best-fit model and competing models (ΔAICc < 2). *P < 0.1, **P < 0.05, ***P < 0.001.
Table S2. Model parameter estimates (β ± SE) and model selection statistics (ΔAICc and Akaike weights, w) for the “time of day” candidate model set for each response variable (diversity indices and bee group abundances). The model from each set with the lowest AICc (i.e., ΔAICc = 0) was considered to be the overall best-fit model. Akaike weights (w) indicate the likelihood the model would be the best-fit model in repeated runs of the experiment. Bolded values represent overall best-fit model and competing models (ΔAICc < 2). *P < 0.1, **P < 0.05, ***P < 0.001.
Figure S1. (A,B) illustrate the sampling scheme, which consistent of two transects ~2 m apart, depicted by black arrows, on two rows of squash between two farm types (polyculture and monoculture).
Figure S2. The mean relative abundances ± standard error of (A) squash bees, (B) wild bees, (C) honey bees, and (D) total bees per minute along a 30 m transect across the proportion of surrounding agricultural land.
Figure S3. The mean relative abundances ± standard error of (A) squash bees, (B) wild bees, (C) honey bees, and (D) total bees per minute along a 30 m transect across early morning (sunrise) and late morning (~8:30 a.m.).
References
Aleixo, K. P., Menezes, C., Imperatriz Fonseca, V. L., and da Silva, C. I. (2017). Seasonal availability of floral resources and ambient temperature shape stingless bee foraging behavior (Scaptotrigona aff. depilis). Apidologie 48, 117–127. doi: 10.1007/s13592-016-0456-4
Amy, C., Noël, G., Hatt, S., Uyttenbroeck, R., Van de Meutter, F., Genoud, D., et al. (2018). Flower strips in wheat intercropping system: effect on pollinator abundance and diversity in Belgium. Insects 9:114. doi: 10.3390/insects9030114
Anderson, D. R., and Burnham, K. P. (2002). Avoiding pitfalls when using information-theoretic methods. J. Wildl. Manage. 66, 912–918. doi: 10.2307/3803155
Arenas, A., and Kohlmaier, M. G. (2019). Nectar source profitability influences individual foraging preferences for pollen and pollen-foraging activity of honeybee colonies. Behav. Ecol. Sociobiol. 73:34. doi: 10.1007/s00265-019-2644-5
Bartomeus, I., Ascher, J. S., Gibbs, J., Danforth, B. N., Wagner, D. L., Hedtke, S. M., et al. (2013). Historical changes in northeastern US bee pollinators related to shared ecological traits. Proc. Natl. Acad. Sci. U.S.A. 110, 4656–4660. doi: 10.1073/pnas.1218503110
Barton, K., and Barton, M. K. (2015). Package ‘MuMIn.' Version 1. Available online at: http://brieger.esalq.usp.br/CRAN/web/packages/MuMIn/MuMIn.pdf
Bates, D., Bolker, B., Walker, S., Singmann, H., Dai, B., Scheipl, F., et al. (2017). Package ‘lme4.' Available online at: http://dk.archive.ubuntu.com/pub/pub/cran/web/packages/lme4/lme4.pdf
Bernauer, O. M., Gaines-Day, H. R., and Steffan, S. A. (2015). Colonies of bumble bees (Bombus impatiens) produce fewer workers, less bee biomass, and have smaller mother queens following fungicide exposure. Insects 6, 478–488. doi: 10.3390/insects6020478
Biesmeijer, J. C., Roberts, S. P. M., Reemer, M., Ohlemüller, R., Edwards, M., Peeters, T., et al. (2006). Parallel declines in pollinators and insect-pollinated plants in Britain and the Netherlands. Science 313, 351–354. doi: 10.1126/science.1127863
Brittain, C., Bommarco, R., Vighi, M., Settele, J., and Potts, S. G. (2010). Organic farming in isolated landscapes does not benefit flower-visiting insects and pollination. Biol. Conserv. 143, 1860–1867. doi: 10.1016/j.biocon.2010.04.029
Brodt, S., Feenstra, G., Kozloff, R., Klonsky, K., and Tourte, L. (2006). Farmer-community connections and the future of ecological agriculture in California. Agric. Hum. Values 23, 75–88. doi: 10.1007/s10460-004-5870-y
Burkle, L. A., Marlin, J. C., and Knight, T. M. (2013). Plant-pollinator interactions over 120 years: loss of species, co-occurrence, and function. Science 339, 1611–1615. doi: 10.1126/science.1232728
Cane, J. H., and Sipes, S. (2006). “Characterizing floral specialization by bees: analytical methods and a revised lexicon for oligolecty,” in Plant–Pollinator Interactions: From Specialization to Generalization, eds N. M. Waser and J. Ollerton (Chicago, IL: University of Chicago Press, 99–122.
Carvell, C., Roy, D. B., Smart, S. M., Pywell, R. F., Preston, C. D., and Goulson, D. (2006). Declines in forage availability for bumblebees at a national scale. Biol. Conserv. 132, 481–489. doi: 10.1016/j.biocon.2006.05.008
Chao, A. (1987). Estimating the population size for capture-recapture data with unequal catchability. Biometrics 43, 783–791. doi: 10.2307/2531532
Dupont, Y. L., Damgaard, C., and Simonsen, V. (2011). Quantitative historical change in bumblebee (Bombus spp.) assemblages of red clover fields. PLoS ONE 6:e25172. doi: 10.1371/journal.pone.0025172
Durant, J. L. (2018). Where have all the flowers gone? Honey bee declines and exclusions from floral resources. J. Rural Stud. 65, 161–171. doi: 10.1016/j.jrurstud.2018.10.007
Fewell, J. H., and Winston, M. L. (1992). Colony state and regulation of pollen foraging in the honey bee, Apis mellifera L. Behav. Ecol. Sociobiol. 30, 387–393. doi: 10.1007/BF00176173
Francis, J. S., Muth, F., Papaj, D. R., and Leonard, A. S. (2016). Nutritional complexity and the structure of bee foraging bouts. Behav. Ecol. 27, 903–911. doi: 10.1093/beheco/arv229
Garbach, K., and Long, R. F. (2017). Determinants of field edge habitat restoration on farms in California's Sacramento Valley. J. Environ. Manage. 189, 134–141. doi: 10.1016/j.jenvman.2016.12.036
Garibaldi, L. A., Carvalheiro, L. G., Leonhardt, S. D., Aizen, M. A., Blaauw, B. R., Isaacs, R., et al. (2014). From research to action: enhancing crop yield through wild pollinators. Front. Ecol. Environ. 12, 439–447. doi: 10.1890/130330
Garibaldi, L. A., Steffan-Dewenter, I., Winfree, R., Aizen, M. A., Bommarco, R., Cunningham, S. A., et al. (2013). Wild pollinators enhance fruit set of crops regardless of honey bee abundance. Science 339, 1608–1611. doi: 10.1126/science.1230200
Giannini, T. C., Saraiva, A. M., and Alves-dos-Santos, I. (2010). Ecological niche modeling and geographical distribution of pollinator and plants: a case study of Peponapis fervens (Smith, 1879) (Eucerini: Apidae) and Cucurbita species (Cucurbitaceae). Ecol. Inform. 5, 59–66. doi: 10.1016/j.ecoinf.2009.09.003
Goodrich, B. K. (2019). Do more bees imply higher fees? Honey bee colony strength as a determinant of almond pollination fees. Food Policy 83, 150–160. doi: 10.1016/j.foodpol.2018.12.008
Goulson, D., Nicholls, E., Botías, C., and Rotheray, E. L. (2015). Bee declines driven by combined Stress from parasites, pesticides, and lack of flowers. Science 347:1255957. doi: 10.1126/science.1255957
Grab, H., Branstetter, M. G., Amon, N., Urban-Mead, K. R., Park, M. G., Gibbs, J., et al. (2019). Agriculturally dominated landscapes reduce bee phylogenetic diversity and pollination services. Science 363, 282–284. doi: 10.1126/science.aat6016
Greenleaf, S. S., and Kremen, C. (2006). Wild bees enhance honey bees' pollination of hybrid sunflower. Proc. Natl. Acad. Sci. U.S.A. 103, 13890–13895. doi: 10.1073/pnas.0600929103
Greenleaf, S. S., Williams, N. M., Winfree, R., and Kremen, C. (2007). Bee foraging ranges and their relationship to body size. Oecologia 153, 589–596. doi: 10.1007/s00442-007-0752-9
Hladik, M. L., Vandever, M., and Smalling, K. L. (2016). Exposure of native bees foraging in an agricultural landscape to current-use pesticides. Sci. Total Environ. 542, 469–477. doi: 10.1016/j.scitotenv.2015.10.077
Hurd, P. D., Linsley, E. G., and Michelbacher, A. E. (1974). Ecology of the Squash and Gourd Bee, Peponapis pruinosa, on Cultivated Cucurbits in California (Hymenoptera: Apoidea). Washington, DC: Smithsonian Institution Press.
Hurd, P. D., Linsley, E. G., and Whitaker, T. W. (1971). Squash and gourd bees (Peponapis, Xenoglossa) and the origin of the cultivated Cucurbita. Evolution 25, 218–234. doi: 10.1111/j.1558-5646.1971.tb01874.x
IPBES (2019). Intergovernmental Platform on Biodiversity and Ecosystem Services. Available online at: https://www.ipbes.net/. (accessed April 12, 2019).
Jha, S., and Kremen, C. (2013). Resource diversity and landscape-level homogeneity drive native bee foraging. Proc. Natl. Acad. Sci. U.S.A. 110, 555–558. doi: 10.1073/pnas.1208682110
Kelly, P. A., Phillips, S. E., and Williams, D. F. (2005). “Documenting ecological change in time and space: the San Joaquin Valley of California,” in Mammalian Diversification: From Chromosomes to Phylogeography (A Celebration of the Career of James L. Patton), eds E. A. Lacey and P. Myers (Berkeley; Los Angeles, CA: University of California Press), 57–78.
Kennedy, C. M., Lonsdorf, E., Neel, M. C., Williams, N. M., Ricketts, T. H., Winfree, R., et al. (2013). A global quantitative synthesis of local and landscape effects on wild bee pollinators in agroecosystems. Ecol. Lett. 16, 584–599. doi: 10.1111/ele.12082
Klein, A.-M., Vaissiere, B. E., Cane, J. H., Steffan-Dewenter, I., Cunningham, S. A., Kremen, C., et al. (2007). Importance of pollinators in changing landscapes for world crops. Proc. R. Soc. B Biol. Sci. 274, 303–313. doi: 10.1098/rspb.2006.3721
Knapp, J. L., Bartlett, L. J., and Osborne, J. L. (2017). Re-evaluating strategies for pollinator-dependent crops: how useful is parthenocarpy? J. Appl. Ecol. 54, 1171–1179. doi: 10.1111/1365-2664.12813
Kovács-Hostyánszki, A., Espíndola, A., Vanbergen, A. J., Settele, J., Kremen, C., and Dicks, L. V. (2017). Ecological intensification to mitigate impacts of conventional intensive land use on pollinators and pollination. Ecol. Lett. 20, 673–689. doi: 10.1111/ele.12762
Kratschmer, S., Pachinger, B., Schwantzer, M., Paredes, D., Guernion, M., Burel, F., et al. (2018). Tillage intensity or landscape features: what matters most for wild bee diversity in vineyards? Agric. Ecosyst. Environ. 266, 142–152. doi: 10.1016/j.agee.2018.07.018
Kremen, C., and Merenlender, A. M. (2018). Landscapes that work for biodiversity and people. Science 362:eaau6020. doi: 10.1126/science.aau6020
Kremen, C., and M'Gonigle, L. K. (2015). EDITOR'S CHOICE: small-scale restoration in intensive agricultural landscapes supports more specialized and less mobile pollinator species. J. Appl. Ecol. 52, 602–610. doi: 10.1111/1365-2664.12418
Kremen, C., M'Gonigle, L. K., and Ponisio, L. C. (2018). Pollinator community assembly tracks changes in floral resources as restored hedgerows mature in agricultural landscapes. Front. Ecol. Evol. 6, 1–10. doi: 10.3389/fevo.2018.00170
Kremen, C., Ullman, K. S., and Thorp, R. W. (2011). Evaluating the quality of citizen-scientist data on pollinator communities. Conserv. Biol. 25, 607–617. doi: 10.1111/j.1523-1739.2011.01657.x
Kremen, C., Williams, N. M., and Thorp, R. W. (2002). Crop pollination from native bees at risk from agricultural intensification. Proc. Natl. Acad. Sci. U.S.A. 99, 16812–16816. doi: 10.1073/pnas.262413599
Larsson, M. (2005). Higher pollinator effectiveness by specialist than generalist flower-visitors of unspecialized Knautia arvensis (Dipsacaceae). Oecologia 146, 394–403. doi: 10.1007/s00442-005-0217-y
Leong, M., Roderick, G. K., Kremen, C., Ponisio, L. C., and Thorp, R. W. (2015). Temporal dynamics influenced by global change: bee community phenology in urban, agricultural, and natural landscapes. Glob. Chang. Biol. 22, 1046–1053. doi: 10.1111/gcb.13141
Lindborg, R., Gordon, L. J., Malinga, R., Bengtsson, J., Peterson, G., Bommarco, R., et al. (2017). How spatial scale shapes the generation and management of multiple ecosystem services. Ecosphere 8:e01741. doi: 10.1002/ecs2.1741
López-Uribe, M. M., Cane, J. H., Minckley, R. L., and Danforth, B. N. (2016). Crop domestication facilitated rapid geographical expansion of a specialist pollinator, the squash bee Peponapis pruinosa. Proc. R. Soc. B Biol. Sci. 283:20160443. doi: 10.1098/rspb.2016.0443
Magurran, A. E. (1988). Ecological Diversity and Its Measurement. Princeton, NJ: Princeton University Press.
Maldonado, M. B., Lomáscolo, S. B., and Vázquez, D. P. (2013). The Importance of pollinator generalization and abundance for the reproductive success of a generalist plant. PLoS ONE 8:e75482. doi: 10.1371/journal.pone.0075482
Mayer, C., and Kuhlmann, M. (2004). Synchrony of pollinators and plants in the winter rainfall area of South Africa—observations from a drought year. Trans. R. Soc. South Africa 59, 55–57. doi: 10.1080/00359190409519162
M'Gonigle, L. K., Ponisio, L. C., Cutler, K., and Kremen, C. (2015). Habitat restoration promotes pollinator persistence and colonization in intensively managed agriculture. Ecol. Appl. 25, 1557–1565. doi: 10.1890/14-1863.1
Minckley, R., Roulston, T. H., and Williams, N. (2013). Resource assurance predicts specialist and generalist bee activity in drought. Proc. R. Soc. London, Biol. 280:1759. doi: 10.1098/rspb.2012.2703
Moeller, D. A. (2004). Facilitative interactions among plants via shared pollinators. Ecology 85, 3289–3301. doi: 10.1890/03-0810
Molinar, R. H. (2012). Indigenous Asian specialty vegetables in the central valley of California. HortScience 47, 835–838. doi: 10.21273/HORTSCI.47.7.835
Morandin, L. A., and Kremen, C. (2013). Bee preference for native versus exotic plants in restored agricultural hedgerows. Restor. Ecol. 21, 26–32. doi: 10.1111/j.1526-100X.2012.00876.x
Motzke, I., Klein, A. M., Saleh, S., Wanger, T. C., and Tscharntke, T. (2016). Habitat management on multiple spatial scales can enhance bee pollination and crop yield in tropical homegardens. Agric. Ecosyst. Environ. 223, 144–151. doi: 10.1016/j.agee.2016.03.001
Norris, S. L., Blackshaw, R. P., Critchley, C. N. R., Dunn, R. M., Smith, K. E., Williams, J., et al. (2017). Intercropping flowering plants in maize systems increases pollinator diversity. Agric. For. Entomol. 20, 246–254. doi: 10.1111/afe.12251
Ogilvie, J. E., and Forrest, J. R. (2017). Interactions between bee foraging and floral resource phenology shape bee populations and communities. Curr. Opin. Insect Sci. 21, 75–82. doi: 10.1016/j.cois.2017.05.015
Patrício-Roberto, G. B., and Campos, M. J. O. (2014). Aspects of landscape and pollinators-what is important to bee conservation? Diversity 6, 158–175. doi: 10.3390/d6010158
Pereira, A. L. C., Taques, T. C., Valim, J. O. S., Madureira, A. P., and Campos, W. G. (2015). The management of bee communities by intercropping with flowering basil (Ocimum basilicum) enhances pollination and yield of bell pepper (Capsicum annuum). J. Insect Conserv. 19, 479–486. doi: 10.1007/s10841-015-9768-3
Petchey, O. L., and Gaston, K. J. (2006). Functional diversity: back to basics and looking forward. Ecol. Lett. 9, 741–758. doi: 10.1111/j.1461-0248.2006.00924.x
Ponisio, L. C., M'Gonigle, L. K., and Kremen, C. (2015). On-farm habitat restoration counters biotic homogenization in intensively managed agriculture. Glob. Chang. Biol. 22, 704–715. doi: 10.1111/gcb.13117
Pope, N. S., and Jha, S. (2017). Seasonal food scarcity prompts long-distance foraging by a wild social bee. Am. Nat. 191, 45–57. doi: 10.1086/694843
Potts, S. G., Imperatriz-Fonseca, V., Ngo, H. T., Aizen, M. A., Biesmeijer, J. C., Breeze, T. D., et al. (2016). Safeguarding pollinators and their values to human well-being. Nature 540, 220–229. doi: 10.1038/nature20588
Quinn, N. F., Brainard, D. C., and Szendrei, Z. (2017). Floral strips attract beneficial insects but do not enhance yield in cucumber fields. J. Econ. Entomol. 110, 517–524. doi: 10.1093/jee/tow306
R Core Team (2018). A Language and Environment for Statistical Computing. R Found. Stat. Comput. Available online at: https://www.r-project.org. (accessed April 12, 2019).
Roulston, T. H., Cane, J. H., and Buchmann, S. L. (2000). What governs protein content of pollen: pollinator preferences, pollen-pistil interactions, or phylogeny? Ecol. Monogr. 70, 617–643. doi: 10.1890/0012-9615(2000)070[0617:WGPCOP]2.0.CO;2
Rudel, T. K., Schneider, L., Uriarte, M., Turner, B. L., DeFries, R. S., Lawrence, D., et al. (2009). Agricultural intensification and changes in cultivated areas, 1970-2005. Proc. Natl. Acad. Sci. U.S.A. 106, 20675–20680. doi: 10.1073/pnas.0812540106
Rundlöf, M., Nilsson, H., and Smith, H. G. (2008). Interacting effects of farming practice and landscape context on bumble bees. Biol. Conserv. 141, 417–426. doi: 10.1016/j.biocon.2007.10.011
Sardiñas, H. S., and Kremen, C. (2015). Pollination services from field-scale agricultural diversification may be context-dependent. Agric. Ecosyst. Environ. 207, 17–25. doi: 10.1016/j.agee.2015.03.020
Scheper, J., Reemer, M., van Kats, R., Ozinga, W. A., van der Linden, G. T. J., Schaminée, J. H. J., et al. (2014). Museum specimens reveal loss of pollen host plants as key factor driving wild bee decline in The Netherlands. Proc. Natl. Acad. Sci. U.S.A. 111, 17552–17557. doi: 10.1073/pnas.1412973111
Shuler, R. E., Roulston, T. H., and Farris, G. E. (2005). Farming practices influence wild pollinator populations on squash and pumpkin. J. Econ. Entomol. 98, 790–795. doi: 10.1603/0022-0493-98.3.790
Stanley, D. A., and Stout, J. C. (2014). Pollinator sharing between mass-flowering oilseed rape and co-flowering wild plants: implications for wild plant pollination. Plant Ecol. 215, 315–325. doi: 10.1007/s11258-014-0301-7
Steffan-Dewenter, I., and Leschke, K. (2003). Effects of habitat management on vegetation and above-ground nesting bees and wasps of orchard meadows in Central Europe. Biodivers. Conserv. 12, 1953–1968. doi: 10.1023/A:1024199513365
Steffan-Dewenter, I., Münzenberg, U., Bürger, C., Thies, C., and Tscharntke, T. (2002). Scale-dependant effects of landscape context on three pollinator guilds. Ecology 83, 1421–1432. doi: 10.1890/0012-9658(2002)083[1421:SDEOLC]2.0.CO;2
Tepedino, J. (1981). The pollination efficiency of the squash bee (Peponapis pruinosa) and the honey bee (Apis mellifera) on summer squash (Cucurbita pepo). J. Kansas Entomol. Soc. 54, 359–377.
Ullmann, K. S. (2015). The Role of Connectivity and Disturbance in Dynamic Landscapes: Squash Bees (Peponapis pruinosa) in Yolo County, California (ProQuest dissertations and Theses). University of California, Davis, CA, United States.
Ullmann, K. S., Meisner, M. H., and Williams, N. M. (2016). Impact of tillage on the crop pollinating, ground-nesting bee, Peponapis pruinosa in California. Agric. Ecosyst. Environ. 232, 240–246. doi: 10.1016/j.agee.2016.08.002
Venjakob, C., Klein, A.-M., Ebeling, A., Tscharntke, T., and Scherber, C. (2016). Plant diversity increases spatio-temporal niche complementarity in plant-pollinator interactions. Ecol. Evol. 6, 2249–2261. doi: 10.1002/ece3.2026
Venturini, E. M., Drummond, F. A., Hoshide, A. K., Dibble, A. C., and Stack, L. B. (2016). Pollination reservoirs in lowbush blueberry (Ericales: Ericaceae). J. Econ. Entomol. 110:tow285. doi: 10.1093/jee/tow285
Waser, N. M., Chittka, L., Price, M. V., Williams, N. M., and Ollerton, J. (1996). Generalization in pollination systems, and why it matters. America 77, 1043–1060. doi: 10.2307/2265575
Westphal, C., Steffan-Dewenter, I., and Tscharntke, T. (2003). Mass flowering crops enhance pollinator densities at a landscape scale. Ecol. Lett. 6, 961–965. doi: 10.1046/j.1461-0248.2003.00523.x
Williams, N. M., Crone, E. E., Roulston, T. H., Minckley, R. L., Packer, L., and Potts, S. G. (2010). Ecological and life-history traits predict bee species responses to environmental disturbances. Biol. Conserv. 143, 2280–2291. doi: 10.1016/j.biocon.2010.03.024
Keywords: on-farm diversification, polycultures, specialist pollinators, Peponapis spp., Xenoglossa spp.
Citation: Guzman A, Chase M and Kremen C (2019) On-Farm Diversification in an Agriculturally-Dominated Landscape Positively Influences Specialist Pollinators. Front. Sustain. Food Syst. 3:87. doi: 10.3389/fsufs.2019.00087
Received: 27 May 2019; Accepted: 19 September 2019;
Published: 15 October 2019.
Edited by:
Lynn V. Dicks, University of East Anglia, United KingdomReviewed by:
Ilias Travlos, Agricultural University of Athens, GreeceEmma Louise Burns, Australian National University, Australia
Copyright © 2019 Guzman, Chase and Kremen. This is an open-access article distributed under the terms of the Creative Commons Attribution License (CC BY). The use, distribution or reproduction in other forums is permitted, provided the original author(s) and the copyright owner(s) are credited and that the original publication in this journal is cited, in accordance with accepted academic practice. No use, distribution or reproduction is permitted which does not comply with these terms.
*Correspondence: Aidee Guzman, aideeguzman@berkeley.edu