- 1Department of Food Science and Technology, University of California, Davis, Davis, CA, United States
- 2Department of Animal and Food Sciences, University of Delaware, Newark, DE, United States
- 3Department of Veterinary Biosciences, College of Veterinary Medicine, The Ohio State University, Columbus, OH, United States
Human norovirus (NoV) is a major cause of fresh produce associated outbreaks. In this study a non-thermal process, high pressure processing (HPP), was evaluated for efficacy against a human NoV GII.4 strain, and Tulane virus (TV). Virus survival following HPP treatment was determined using direct quantitative reverse transcriptase PCR (RT-qPCR), the porcine gastric mucin magnetic bead (PGM-MB) binding assay followed by RT-qPCR, and plaque assay. HPP (400 MPa) inactivation of human NoV and TV was enhanced in simple mediums at a low initial temperature (4°C) and neutral pH (pH 7). HPP treatment of human NoV and TV in strawberry puree at 400 MPa did not significantly affect the level of RNA detected using the PGM-MB binding assay; increasing the pressure level to 600 MPa lead to inactivation of both human NoV and TV. Overall, HPP inactivation of human NoV GII.4 and TV is enhanced at lower initial temperature and neutral pH, and viruses can be inactivated in the food matrix at commercially acceptable pressure levels.
Introduction
Human norovirus (NoV) is a major cause of non-bacterial acute gastroenteritis responsible for over 21 million cases of illness annually (U. S. Centers for Disease Control Prevention., 2013). Additionally, human NoV is a major cause of foodborne illness in the US, as 5 million cases of human NoV food related illness are estimated to occur each year (Scallan et al., 2011; Hall et al., 2012; Hoffmann et al., 2012). Fresh produce is the leading simple food type confirmed to be the causative agent of human NoV foodborne outbreaks from 2001 to 2008 (Hall et al., 2012). Human NoV contamination of produce can occur during production, harvesting, processing, transport, and handling (Seymour and Appleton, 2001; Steele and Odemeru, 2004; Butot et al., 2007; Baert et al., 2008; Heaton and Jones, 2008). It has been demonstrated that human NoV and other foodborne viruses can become internalized in growing produce. Foodborne pathogens found internally in edible produce structures present a unique problem in terms of inactivation. An intervention that can target viruses found within the food matrix must be utilized to inactive foodborne viruses while maintaining the fresh quality of produce.
High pressure processing (HPP) is a non-thermal food processing technology that is widely used in the food industry to increase the shelf life and safety of foods. During HPP treatments, pressure is distributed uniformly through the food product. This allows for inactivation of pathogens located within the food matrix as well as on the surface of food. HPP has been shown to be effective for control of human NoV in different food matrices. For example, HPP has been shown effective to control for human NoV in oysters, a major high risk food for human NoV outbreaks. Human NoV GI.1 seeded oysters treated by HPP (600 MPa at 6°C for 5 min) did not lead to infection in human subjects who consumed the oysters following HPP treatment (Leon et al., 2011). It has been established that the efficacy of HPP is impacted significantly by the sample matrix with differences in pH, salt concentration, fat composition, and protein composition all effecting pathogen inactivation. This has also been shown to hold true for viral inactivation (Kingsley and Chen, 2008, 2009; Lou et al., 2011a; Li et al., 2013a). In addition, several studies have found that different viruses, including human NoV, hepatitis A virus (HAV), human rotavirus (RV), murine norovirus (MNV-1), feline calicivirus (FCV), and Tulane virus (TV) all require different processing parameters such as pressure level, initial temperature, and holding time for optimal inactivation (Kingsley et al., 2002, 2005, 2006, 2007; Grove et al., 2008; Kingsley and Chen, 2008, 2009; Tang et al., 2010; Lou et al., 2011a; Lou et al., 2011b; Li et al., 2013a).
Human NoV does not grow robustly in cell culture systems, therefore determining efficacy of food processing technologies to inactive the virus has remained a challenge. RNA based detection methods are the current standard methodology used for human NoV detection in foods. A major negative aspect of reverse transcriptase PCR (RT-PCR) based methods is the inability to determine whether RNA detected is from an infectious or non-infectious viral particle. These results of these methods therefore may not accurately reflect the risk of human NoV disease development upon consumption. Recently, methods have been developed to assess human NoV capsid integrity coupled with genomic RNA detection. In theory, these methods detect viral RNA from intact viral capsid, which is a more accurate estimate of viral infectivity compared to RNA detection alone. One such method is the porcine gastric mucin (PGM) binding assay.
PGM conjugated to magnetic beads (PGM-MBs) capture human NoV particles capable of binding to their putative cellular receptor, the histo-blood group antigens (HBGAs) (Tian et al., 2005, 2008, 2010; Dancho et al., 2012). The PGM-MB binding assay was used to assess human NoV GI.1 infectivity in oysters after HPP treatment and the results of the PGM-MB assay were similar to the results obtained from a human volunteer study (Leon et al., 2011; Dancho et al., 2012). Tulane virus (TV) is a widely used surrogate for human NoV research. TV utilizes HBGAs as cellular receptors (Farkas et al., 2010) similar to human NoV. TV can therefore be used as a control to determine whether the PGM-MB binding assay has the ability to discriminate between infectious and non-infectious virus particles.
The objective of this study is to evaluate use HPP to inactivate a human NoV GII.4 strain and TV in simple mediums and strawberry puree. In addition, the use of the PGM-MB binding assay for detection on human NoV inactivation will be compared to plaque assay using TV as a surrogate.
Materials and Methods
Viruses and Cell Culture
Viruses and cell culture methods followed those previously described (Hall et al., 2012; U. S. Centers for Disease Control Prevention., 2013). MK2-LLC (ATCC, Manassas, VA) cells were cultured in low serum Eagle's minimum essential medium (Opti-MEM, Invitrogen), supplemented with 2% fetal bovine serum (FBS), at 37°C under a 5% CO2 atmosphere. Confluent monolayers of cells were cultured in T-150 flasks with a cell density of ~4.6 × 106 cells per 150 cm2 flask. For growing TV stock, MK2-LLC cells were washed with Hank's balanced salt solution (HBSS) and subsequently infected with TV at a multiplicity of infection (MOI) of 0.1. After 1 h incubation at 37°C, 15 ml of Opti-MEM with 2% FBS was added. The virus was harvested 2 days post inoculation and subjected to three freeze-thaw cycles to lyse cells, followed by centrifugation at 1,000 × g for 30 min to remove cellular debris.
Characterization of a Human Norovirus GII.4 Strain
Human NoV clinical isolate 5 M was originally isolated from an outbreak of acute gastroenteritis in Ohio. Positive human NoV samples were sent to the Ohio Department of Health (ODH) as part of the norovirus surveillance program. Anonymous patient stool samples were provided for this study by ODH, the study was exempted from Institutional Research Board oversight. Samples were prepared as previously described (U. S. Centers for Disease Control Prevention., 2013). The stool samples were diluted 1:10 in phosphate buffered saline (PBS), shaken vigorously for 10 min at 4°C and centrifuged for 10 min at 5,000 × g. The sample was filtered through a 0.45 μm filter, aliquoted and stored at −80°C until use. The entire genomic cDNA of the human NoV strain 5 M was amplified by RT-PCR using five to six overlapping fragments. The PCR products were then purified and cloned into a pGEM-T-easy vector (Promega), and sequenced at the Plant Microbe Genetics Facility at The Ohio State University. The full-length genome of the viral isolate was 7,558 nt in length and has been deposited into GeneBank at accession number JQ798158. Sequence comparison found that the strain belongs to the norovirus genotype GII.4. The genomic RNA was then quantified by real-time RT-PCR (RT-qPCR) and the GII.4 isolate 5 M was found to have 6.7 × 106 genomic RNA copies/ml.
Virus Enumeration by Plaque Assay
Virus plaque assay was conducted as previously described (U. S. Centers for Disease Control Prevention., 2013). A standard plaque assay was used to quantify TV in LLC-MK2 cells. Briefly, cells were seeded into six-well plates (Corning Life Sciences, Wilkes-Barre, PA) at a density of 2 × 106 cells per well. When cells were ~80% confluent (24 h incubation), cell monolayers were infected with 400 μl of a 10-fold dilution series of TV. Plates were incubated for 1 h at 37°C with gentile agitation every 10 min. The cells were overlaid with 3 ml of Eagle minimum essential medium (MEM) containing 1% agarose, 2% FBS, 1% sodium bicarbonate, 0.1 mg of kanamycin/ml, 0.05 mg of gentamicin/ml, 15 mM HEPES (pH 7.7), and 2 mM L-glutamine. After incubation at 37°C and 5% CO2 for 2 days, the plates were fixed with 10% formaldehyde. The plaques were visualized by staining with 0.05% (w/v) crystal violet. Viral titer was expressed as mean log10 plaque forming unit (PFU)/ml ± standard deviation.
Enumeration of Viral Genomic RNA by Real Time Reverse Transcriptase PCR (RT-qPCR)
Determination of viral genome copy was conducted as previously described (Kingsley and Chen, 2009; Hoffmann et al., 2012). Total RNA was extracted from samples using an RNeasy Kit (Qiagen), following the manufacturer's instructions. First strand cDNA was synthesized by SuperScriptase III (Invitrogen) using the primers for human NoV and TV in Table 1. The VP1 gene of both viruses was then quantified by real-time PCR using custom Taqman primers and probes (Table 1) on a StepOne real-time PCR machine (Applied Biosystems, Foster City, CA). TaqMan Fast Universal Master Mix was used for all reactions. Cycling parameters were as follows: a holding stage at 95°C was maintained for 20 s prior to cycling, followed by 50 cycles of 95°C for 1 s for annealing and 60°C for 20 s for extension. Standard curves and were used to quantify genomic RNA copies. Viral RNA was expressed as mean log10 genomic RNA copies/ml ± standard deviation.
Porcine Gastric Mucin Conjugated Magnetic Bead Binding Assay
The PGM-MB assay was conducted as previously described (U. S. Centers for Disease Control Prevention., 2013). Type III porcine gastric mucin (PGM) (Sigma, St. Louis, MI) was cross-linked to MagnaBind carboxyl derivatized beads (MB) (Pierce Biotechnology, Rockford, IL) following the manufacturer's instructions. Briefly, 1 ml of MBs was washed three times with PBS. Subsequently, 1 ml of 10 mg/ml Type III PGM in 0.1M 2-(N-morpholino) ethanesulfonic acid (MES) buffer (0.9% NaCl, pH 4.7) and 0.1 ml of 10 mg/ml 1-ethyl-3-[3-dimethlaminopropyl]carbodiimide hydrochloride (EDC) in MES buffer were added to the MBs and incubated for 30 min at room temperature rotating at 8 rpm. Following incubation, beads were isolated from the PGM solution using magnetic separation followed by washing three times with PBS. The PGM-MBs were re-suspended in 1 ml of PBS containing 0.05% sodium azide and stored at 4°C until use. The level of PGM binding to MBs was determined by measuring the initial protein concentration (absorbance at 280 nm) in the 10 mg/mL PGM solution and the protein concentration in the PGM solution removed from beads prior to washing. Following HPP treatment, 100 μl of untreated or treated viruses were combined with 100 μl of PGM-MBs and 800 μl PBS was added for a final volume of 1 ml. The virus-PGM-MB solution was incubated for 30 min at room temperature rotating at 8 rpm. The beads were separated and washed three times with PBS, and finally re-suspended in 100 μl of PBS for RNA extraction using the RNeasy kit (Qiagen). Human NoV and TV RNA levels were determined by RT-qPCR.
HPP Treatment of Viruses in PBS and Strawberry Puree
Strawberry puree was prepared by blending 25 g of fresh strawberries with 25 ml of deionized H2O. The original pH of the strawberry puree was 4.05. Human NoV and TV were diluted ~1:10 in strawberry puree to achieve a titer of 1 × 106 RNA copy/ml. In addition the pH of the strawberry puree was adjusted to seven using 0.1 M NaOH and viruses were similarly diluted in pH 7 strawberry puree. Human NoV and TV were diluted ~1:10 in PBS with a pH of seven and also PBS adjusted with 0.1 M HCl to pH 4 to achieve a level of 1 × 106 RNA copy/ml. Five hundred microliter of each virus diluted in strawberry puree pH 4, strawberry puree pH 7, PBS pH 7, and PBS pH 4 was placed in a sterile polyethylene stomacher bag (Fisher Scientific, Pittsburg, PA) and heat sealed with an AIE-200 impulse sealer (American International Electric, Whittier, CA). All samples were prepared in triplicate, double bagged, and shipped on ice to the University of Delaware for high pressure treatment using a lab scale HPP unit (model Avure PT-1; Avure Technologies, Kent, WA) with water as the hydrostatic fluid. Samples were treated at 400 MPa at an initial temperature of 4°C or 20°C with a holding time of 2 min. The 2 min holding time did not include the come-up and release time (22 MPa/s, 4 s, respectively). In addition, strawberry puree at original pH was treated at 500 and 600 MPa for 2 min at an initial temperature of 4°C and at 600 MPa for 5 min at an initial temp of 4°C. Following treatment, the level of human NoV RNA was determined using the PGM-MB binding assay followed by RT-qPCR. TV levels were determined using the PGM-MB binding assay in addition to standard plaque assay.
Statistical Analysis
All experiments were performed in triplicate. Statistical analysis was performed by one-way ANOVA analysis using Tukey's family comparisons using Minitab 16 statistical analysis software (Minitab Inc., State College, PA). A P value of <0.05 was considered statistically significant.
Results
HPP Inactivation of Human NoV GII.4 in PBS and Strawberry Puree
Using the PGM-MB binding assay followed by RT-qPCR the level of human NoV RNA detected in PBS at pH 4 was 5.46 ± 0.19 RNA log10 copy/ml (Figure 1A). Treatment of 400 MPa for 2 min at 4°C resulted in a reduction in the level of RNA detected to 3.32 ± 0.385 log10 RNA copy/ml (2.14 log10 reduction) and treatment at 20°C resulted in a reduction to 4.38 ± 0.28 log10 RNA copy/ml (1.08 log10 reduction) (Figure 1A). PBS at pH 7 had an initial human NoV RNA level detected using the PGM-MB assay of 5.31 ± 0.25 log10 RNA copy/ml (Figure 1A). Following treatment at 4°C the RNA level was 2.25 ± 0.14 log10 RNA copy/ml (3.06 log10 reduction) and treatment at 20°C resulted in a reduction to 4.07 ± 0.04 log10 RNA copy/ml (1.24 log10 reduction) (Figure 1A). These results indicate that human NoV was more easily inactivated at 4 than at 20°C in PBS. In addition, human NoV inactivation was enhanced at pH 7 compared to pH 4.0.
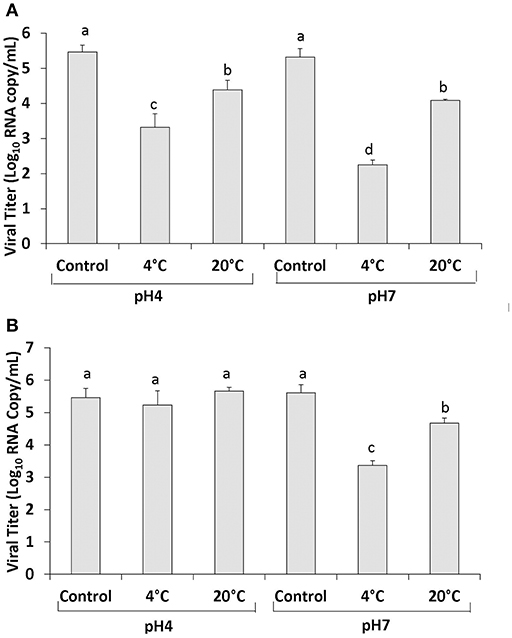
Figure 1. Human NoV GII.4 inactivation by HPP in PBS (A) and strawberry puree (B). Each bar represents average of three replicates ±1 standard deviation. Groupings (a, b, c, d) are based on one-way ANOVA analysis using Tukey's comparisons, with a 95% C.I. Values with different lowercase letters (a, b, c, d) are significantly different (p < 0.05) and values sharing the same lowercase letters (a, b, c, d) are not significantly different (p > 0.05).
In strawberry puree pH adjusted to seven, the initial level of human NoV RNA detected using the PGM-MB assay was 5.61 ± 0.25 log10 RNA copy/ml (Figure 1B). Following 400 MPa treatment for 2 min at 4°C the level of RNA was reduced to 3.32 ± 0.15 log10 RNA copy/ml (2.29 log10 reduction) (Figure 1B). Strawberry puree pH 7 treated at 20°C resulted in an ~1 log reduction in viral RNA to 4.67 ± 0.16 RNA log10 copy/ml (Figure 1B). In strawberry puree pH 4 (original pH), the level of human NoV RNA detected using the PGM-MB assay was 5.46 ± 0.28 RNA log10 copy/ml (Figure 1B). Treatment at 400 MPa for 2 min at 4 and 20°C resulted in RNA levels of 5.23 ± 0.44 and 5.66 ± 0.12 log10 RNA copy/ml, respectively (Figure 1B).
To achieve inactivation of human NoV in strawberry puree at natural pH (pH 4) samples were treated at 500 MPa and 600 MPa for 2 min at an initial temperature of 4°C. A slight reduction in human NoV was detected at 500 MPa (Figure 2). No human NoV RNA was detected at a pressure level of 600 MPa, indicating this pressure level is sufficient to inactivate the virus (Figure 2).
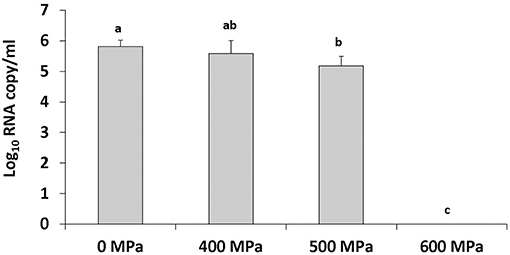
Figure 2. Human NoV GII.4 inactivation in strawberry puree (pH 4) by increasing pressure levels. Treatment is at an initial temperature of 4°C with a holding time of 2 min. Each bar represents average of three replicates ±1 standard deviation.
HPP Inactivation of TV in PBS and Strawberry Puree
The level of infectious TV in PBS at pH 7 was 5.48 ± 0.06 log10 PFU/ml and following 400 MPa treatment at 4°C for 2 min no infectious virus was detected, however at a holding temperature of 20°C 3.05 ± 0.14 log10 PFU/ml of TV remained (2.43 log10 reduction) (Figure 3A). In PBS at pH 4 the titer of TV was 5.55 ± 0.08 log10 PFU/ml and treatment at 400 MPa at 4°C again resulted in no infectious virus recovered, and similar to pH 7 at an initial temperature of 20°C 2.86 ± 0.08 log10 PFU/ml TV was detected (2.69 log10 reduction) (Figure 3A). Using the PGM-MB binding assay followed by RT-qPCR the level of TV RNA detected in PBS pH 4 was 6.10 ± 0.39 log10 RNA copy/ml (Figure 3C). Following treatment at 400 MPa for 2 min at 4°C the RNA level decreased to 3.07 ± 0.66 log10 RNA copy/ml (3.03 log10 reduction) and at 20°C the level detected using the PGM-MB assay was 4.02 ± 0.31 log10 RNA copy/ml (2.08 log10 reduction) (Figure 3C). In PBS at pH 7 the initial TV RNA level detected was 6.35 ± 0.19 log10 RNA copy/ml, and 400 MPa treatment for 2 min at 4 and 20°C reduced the level of RNA detected to 2.91 ± 0.16 (3.44 log10 reduction) and 3.77 ± 0.05 (2.58 log10 reduction) log10 RNA copy/ml, respectively (Figure 3C). This data showed that TV in PBS was efficiently inactivated by HPP and 4°C was more easily inactivated than at 20°C. At same treatment condition at 4°C, no infectious TV was detected by plaque assay whereas 3-log RNA was still detected by real-time RT-PCR, consistent with the fact that real-time RT-PCR is more sensitive than plaque assay and it is capable of detecting RNA from infectious and non-infectious virus particles.
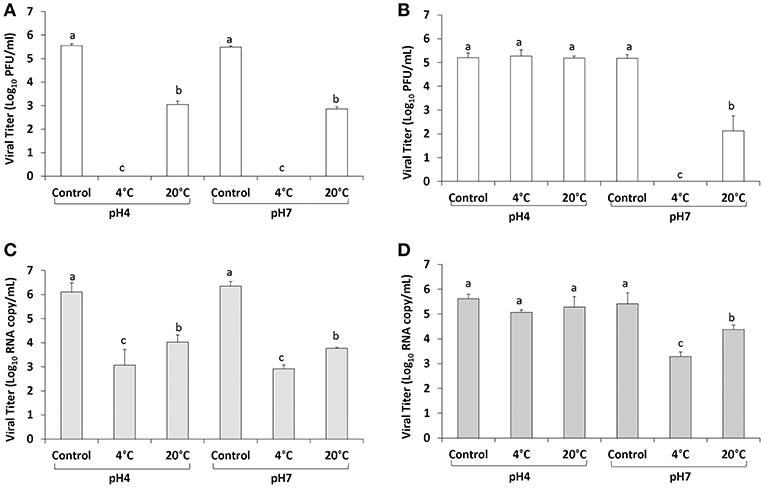
Figure 3. TV inactivation by HPP in PBS (A,B) and strawberry puree (C,D). Each bar represents average of three replicates ±1 standard deviation. Groupings (a, b, c, d) are based on one-way ANOVA analysis using Tukey's comparisons, with a 95% C.I. Values with different lowercase letters (a, b, c, d) are significantly different (p < 0.05) and values sharing the same lowercase letters (a, b, c, d) are not significantly different (p > 0.05).
In strawberry puree with the pH adjusted to seven, the initial titer of TV was 5.17 ± 0.16 log10 PFU/ml and following treatment at 4°C for 2 min, no infectious virus was detected (Figure 3B). In strawberry puree pH adjusted to seven, the level of TV RNA detected using the PGM-MB assay was 5.42 ± 0.44 log10 RNA copy/ml (Figure 3D). Following treatment at 4°C the level of RNA detected was 3.29 ± 0.18 log10 RNA copy/ml (2.13 log10 reduction) and treatment at 20°C resulted in an RNA level of 4.37 ± 0.18 log10 RNA copy/ml (1.05 log10 reduction) (Figure 3D). In strawberry puree at pH 4 (original pH) the initial TV RNA level detected using the PGM-MB assay was 5.62 ± 0.17 log10 RNA copy/ml (Figure 3D). Following treatment of 400 MPa for 2 min at 4°C the level of TV RNA detected was 5.06 ± 0.10 log10 RNA copy/ml and at 20°C was 5.28 ± 0.41 log10 RNA copy/ml (Figure 3D). No significant reduction in TV RNA was observed, similar to the TV titer reduction determined by plaque assay. At a holding temperature of 20°C the level of infectious TV detected in strawberry puree at pH 7 was 2.12 ± 0.63 log10 PFU/ml (Figure 3B). At pH 7.0 in strawberry puree, TV was more easily inactivated at 4 than at 20°C. Surprisingly, no significant TV titer reduction was observed at either 4 or 20°C when TV was diluted in strawberry puree at pH 4.0 (natural pH) treated by 400 MPa for 2 min (Figure 3B). Therefore, TV inactivation was enhanced at pH 7.0 compared to pH 4.0 and the food matrix played a key role in protecting TV from inactivation.
To completely inactivate TV in strawberry puree at its original pH (pH 4), samples were treated at 500 and 600 MPa for 2 min at an initial temperature of 4°C. There was a significant (1.57 log10) reduction observed in TV infectivity at 500 MPa and a 1.67 log10 reduction detected using the PGM-MB binding assay (Figures 4A,B). TV was not detected using plaque assay or the PGM-MB binding assay following 600 MPa of treatment (Figures 4A,B).
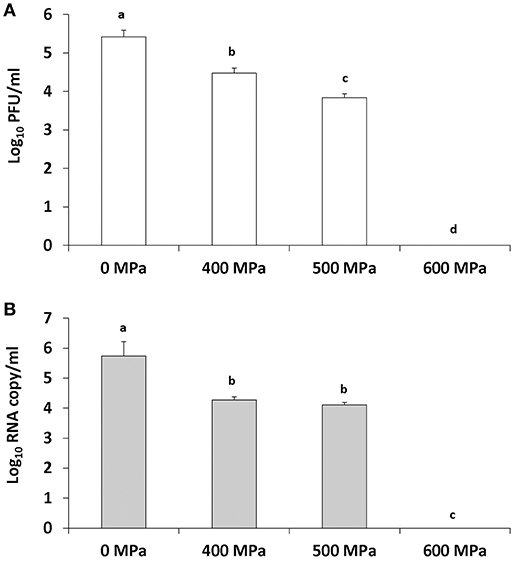
Figure 4. TV inactivation in strawberry puree (pH 4) by increasing pressure levels as detected by plaque assay (A) and PGM-MB coupled with RT-qPCR (B). Treatment is at an initial temperature of 4°C with a holding time of 2 min. Each bar represents average of three replicates ± 1 standard deviation.
Discussion
Fresh produce is a major vehicle of human NoV outbreaks. Several studies have shown that viruses can become internalized in growing produce (Wei et al., 2010, 2011; Dicaprio et al., 2012; Erickson, 2012; Esseili et al., 2012; Hirneisen and Kniel, 2013). Internalized viruses would be protected from all surface disinfectants. Therefore, treatments that can target viruses within the food matrix, as well as maintain the quality of fresh produce must be developed. In this study, we evaluated HPP for the ability to inactivate human NoV in simple mediums at different pHs and incorporated in the food matrix. Overall, were found commercially acceptable pressure levels are capable of inactivating both human NoV and TV in both PBS and strawberry puree under optimized conditions.
Comparison of TV Infectivity as Detected by Plaque Assay and TV Receptor Binding Ability Detected by the PGM-MB Assay Following HPP Treatment
PGM-MBs were initially used for the purpose of extracting human NoV from complex foods to decrease PCR inhibition and lower the limit of detection (Tian et al., 2008, 2011, 2012). PGM-MBs were subsequently used to discriminate between infectious and non-infectious human NoV particles following treatments with HPP, UV, and heat (Dancho et al., 2012; Kingsley et al., 2014).
We found HPP treatment of TV in pH 7 PBS at 20°C resulted in a 2.62 log10 PFU reduction in infectivity and a 2.58 log10 reduction in TV RNA using the PGM-MB binding assay. However, under treatment conditions where no infectious virus was detected using plaque assay (400 MPa, 4°C, 2 min; PBS pH 4, PBS pH 7, puree pH 7), TV viral RNA was still detected using the PGM-MB binding assay although the level of RNA was significantly reduced (p < 0.05) compared to untreated controls. Correlation between TV PGM-MB detection and infectivity was good when reductions were ~2 log, but diverged with high log reductions in another study (Li and Chen, 2015). However, the PGM-MB is still an improved method over direct RT-qPCR to estimate viral survival.
Human NoV GII.4 and TV Inactivation by HPP
The primary mechanism of HPP inactivation of viruses was found to be disruption of the viral capsid and receptor binding activity but not degradation of viral RNA (Lou et al., 2011a). Thus, intact virus particles that possess receptor binding activity should be able to bind to PGM-MB and subsequently detected by RT-qPCR. The PGM-MB binding assay has been employed in several studies to estimate the survival of human NoV following HPP treatment (Dancho et al., 2012; Li et al., 2013b; Ye et al., 2014; Li and Chen, 2015). We found that treatment of 400 MPa at 4°C of human NoV GII.4 in PBS at pH 4 resulted in a 2.14 log10 reduction in viral RNA using the PGM-MB binding assay compared to a 1.08 log10 reduction at 20°C. Similarly human NoV in PBS at pH 7 at 4°C resulted in a 2.88 log10 reduction in viral RNA using the PGM-MB assay while at 20°C only a 1.24 log10 reduction was observed. It has been observed that human NoV inactivation, as well as human NoV surrogates, is favored at a low initial temperature and neutral pH compared to higher temperatures and acidic pHs (Lou et al., 2011a,b; Li et al., 2013a). Our data is consistent with other studies indicating that human NoV inactivation by HPP is favored at lower treatment holding temperatures and neutral pH.
The complexity of the suspension medium provides protection to viruses during HPP treatment (Kingsley et al., 2005; Kingsley and Chen, 2008, 2009; Lou et al., 2011a). No significant reductions in human NoV GII.4 RNA were detected in strawberry puree (pH 4) by the PGM-MB binding assay at 400 MPa for 2 min at temperatures of 4 or 20°C. However, a significant reduction in RNA was detected in puree when the pH was adjusted to seven and the initial temperature was 4°C. Similarly, there was no reduction in TV infectivity in strawberry puree at its original pH (pH 4), when treated at 400 MPa for 2 min at either 4 or 20°C. However, when the pH of the puree was adjusted to pH 7, no infectious TV was detected following 400 MPa treatment for 2 min at 4°C (>5 log10 reduction) and a 3 log10 reduction in TV infectivity was achieved at an initial temperature of 20°C. Interestingly, it was reported that a 3.7 log RNA reduction was achieved when human NoV GII.4 strain was inoculated into oysters (natural pH ~7.0) treated by 350 MPa for 2 min at 4°C whereas only 1.2 log10 RNA reduction was observed when the virus-inoculated oyster was treated by 350 MPa for 2 min at 20°C. These results indicate that human NoV and TV inactivation is enhanced at higher initial temperature and neutral pH, as well as that the food matrix provides a protective effect to the virus during HPP treatment.
It has been reported that the complexity of the food matrix can protect viruses from inactivation by HPP. Previous studies have shown that the presence carbohydrates, fats, salts, and proteins limits the effectiveness of HPP against viruses (Gross and Jaenicke, 1994; Balny et al., 2002; Baert et al., 2009; Kingsley and Chen, 2009). In this study we found that human NoV and TV HPP inactivation was enhanced in PBS compared to strawberry puree when both matrices had a pH of 4. This indicates that other components of the strawberry puree provided a baroprotective effect. In fresh meat products, HPP treatment is known to promote lipid oxidation (Beltran et al., 2004; Bolumar et al., 2011, 2014; Alves et al., 2012). It has been proposed that lipid oxidation in caused by free radical formation due to HPP treatment (Mariutti et al., 2008; Bolumar et al., 2011). Compounds present in strawberries, such as polyphenols (anthocyanins, flavanols, flavonols) or phenolic acids (hydroxybenzoic acid, hydroxycinnamic acid) are known antioxidants. Therefore, the presence of these antioxidants in strawberries could minimize capsid damage caused by free radicals leading to enhanced resistance of viruses to HPP. In the same studies of meat and poultry, free radical formation was found to be enhanced at pressures above 500 MPa (Beltran et al., 2004; Mariutti et al., 2008; Bolumar et al., 2011, 2014; Alves et al., 2012). In this study there was a dramatic increase in virus inactivation in strawberry puree when the pressure level was increased from 500 to 600 MPa. It is possible that higher levels of free radicals were formed during 600 MPa treatment and effectively overwhelmed the antioxidant potential of the system, leading to enhancement of virus inactivation.
The results of this study indicate that (i) the PGM-MB binding assay coupled with RT-qPCR is an improved method for detecting inactivation of human NoV by HPP compared to direct RT-qPCR alone; (ii) HPP inactivation of human NoV GII.4 and TV is enhanced at lower initial temperature (4°C) and neutral pH (pH 7) and the food matrix provides a protective effect to the virus; (iii) HPP is capable of inactivating human NoV GII.4 and TV at commercially acceptable pressure level.
Author Contributions
All authors listed have made a substantial, direct and intellectual contribution to the work, and approved it for publication.
Conflict of Interest Statement
The authors declare that the research was conducted in the absence of any commercial or financial relationships that could be construed as a potential conflict of interest.
Acknowledgments
This work was supported in part by a grant (2015-69003-23410) and a NoroCORE project grant (2011-68003-30395) from the U.S. Department of Agriculture.
References
Alves, A. B., Bragagnolo, N., da Silva, M. G., Skibsted, L. H., and Orlien, V. (2012). Antioxidant protection of high-pressure processed minced chicken meat by industrial tomato products. Food Bioprod. Process. 90, 499–505. doi: 10.1016/j.fbp.2011.10.004
Baert, L., Debevere, J., and Uyttendaele, M. (2009). The efficacy of preservation methods to inactivate foodborne viruses. Int. J. Food Microbiol. 131, 83–94. doi: 10.1016/j.ijfoodmicro.2009.03.007
Baert, L., Uyttendaele, M., Vermeersch, M., Van Coillie, E., and Debevere, J. (2008). Survival and transfer of murine norovirus 1, a surrogate for human noroviruses, during the production process of deep-frozen onions and spinach. J. Food Prot. 71, 1590–1597. doi: 10.4315/0362-028X-71.8.1590
Balny, C., Masson, P., and Heremans, K. (2002). High pressure effects on biological macromolecules: from structural changes to alteration of cellular processes. Biochim. Biophys. Acta 1595, 3–10. doi: 10.1016/S0167-4838(01)00331-4
Beltran, E., Pla, R., Yuste, J., and Mor-Mur, M. (2004). Use of antioxidants to minimize rancidity in pressurized and cooked chicken slurries. Meat Sci. 66, 719–725. doi: 10.1016/j.meatsci.2003.07.004
Bolumar, T., Andersen, M. L., and Orlien, V. (2011). Antioxidant active packaging for chicken meat processed by high pressure treatment. Food Chem. 129, 1406–1412. doi: 10.1016/j.foodchem,.2011.05.082
Bolumar, T., Andersen, M. L., and Orlien, V. (2014). Mechanisms of radical formation in beef and chicken meat during high pressure processing evaluated by electron spin resonance detection and the addition of antioxidants. Food Chem. 150, 422–428. doi: 10.1016/j.foodchem.2013.10.161
Butot, S., Putallaz, T., and Sánchez, G. (2007). Procedure for rapid concentration and detection of enteric viruses from berries and vegetables. Appl. Environ. Microbiol. 73, 186–192. doi: 10.1128/AEM.01248-06
Dancho, B. A., Chen, H., and Kingsley, D. H. (2012). Discrimination between infectious and non-infectious human norovirus using porcine gastric mucin. Int. J. Food Microbiol. 155, 222–226. doi: 10.1016/j.ijfoodmicro.2012.02.010
Dicaprio, E., Ma, Y., Purgianto, A., Hughes, J., and Li, J. (2012). Internalization and dissemination of human norovirus and animal caliciviruses in hydroponically grown romaine lettuce. Appl. Environ. Microbiol. 78, 6143–6152. doi: 10.1128/AEM.01081-12
Erickson, M. C. (2012). Internalization of fresh produce by foodborne pathogens. Annu. Rev. Food Sci. Technol. 3, 283–310. doi: 10.1146/annurev-food-022811-101211
Esseili, M. A., Wang, Q., Zhang, Z., and Saif, L. J. (2012). Internalization of sapovirus, a surrogate for norovirus, in romaine lettuce and the effect of lettuce latex on virus infectivity. Appl. Environ. Microbiol. 78, 6271–6279. doi: 10.1128/AEM.01295-12
Farkas, T., Cross, R. W., Hargitt, E., Lerche, N. W., Morrow, A. L., and Sestak, K. (2010). Genetic diversity and histo-blood group antigen interactions of rhesus enteric caliciviruses. J. Virol. 84, 8617–8625. doi: 10.1128/JVI.00630-10
Gross, M., and Jaenicke, R. (1994). Proteins under pressure - the influence of high hydrostatic-pressure on structure, function and assembly of proteins and protein complexes. Eur. J. Biochem. 221, 617–630. doi: 10.1111/j.1432-1033.1994.tb18774.x
Grove, S. F., Lewis, T., Ross, T., Forsyth, S., Wan, J., Coventry, J., et al. (2008). Inactivation of hepatitis A virus, poliovirus and a norovirus surrogate by high pressure processing. Innov. Food Sci. Emerg. 9, 206–210. doi: 10.1016/j.ifset.2007.07.006
Hall, A. J., Eisenbart, V. G., Etingüe, A. L., Gould, L. H., Lopman, B. A., and Parashar, U. D. (2012). Epidemiology of foodborne norovirus outbreaks, United States, 2001-2008. Emerg. Infect. Dis. 18, 1566–1573. doi: 10.3201/eid1810.120833
Heaton, J. C., and Jones, K. (2008). Microbial contamination of fruit and vegetables and the behaviour of enteropathogens in the phyllosphere: a review. J. Appl. Microbiol. 104, 613–626. doi: 10.1111/j.1365-2672.2007.03587.x
Hirneisen, K. A., and Kniel, K. E. (2013). Comparative uptake of enteric viruses into spinach and green onions. Food Environ. Virol. 5, 24–34. doi: 10.1007/s12560-012-9093-x
Hoffmann, S., Batz, M. B., and Morris, J. G. (2012). Annual cost of illness and quality-adjusted life year losses in the United States due to 14 foodborne pathogens. J. Food Prot. 75, 1292–1302. doi: 10.4315/0362-028X.JFP-11-417
Kingsley, D. H., and Chen, H. (2008). Aqueous matrix compositions and pH influence feline calicivirus inactivation by high pressure processing. J. Food Prot. 71, 1598–1603. doi: 10.4315/0362-028X-71.8.1598
Kingsley, D. H., and Chen, H. (2009). Influence of pH, salt, and temperature on pressure inactivation of hepatitis A virus. Int. J. Food Microbiol. 130, 61–64. doi: 10.1016/j.ijfoodmicro.2009.01.004
Kingsley, D. H., Guan, D., and Hoover, D. G. (2005). Pressure inactivation of hepatitis A virus in strawberry puree and sliced green onions. J. Food Prot. 68, 1748–1751. doi: 10.4315/0362-028X-68.8.1748
Kingsley, D. H., Guan, D., Hoover, D. G., and Chen, H. (2006). Inactivation of hepatitis A virus by high-pressure processing: the role of temperature and pressure oscillation. J. Food Prot. 69, 2454–2459. doi: 10.4315/0362-028X-69.10.2454
Kingsley, D. H., Holliman, D. R., Calci, K. R., Chen, H., and Flick, G. J. (2007). Inactivation of a norovirus by high-pressure processing. Appl. Environ. Microbiol. 73, 581–585. doi: 10.1128/AEM.02117-06
Kingsley, D. H., Hoover, D. G., Papafragkou, E., and Richards, G. P. (2002). Inactivation of hepatitis A virus and a calicivirus by high hydrostatic pressure. J. Food Prot. 65, 1605–1609. doi: 10.4315/0362-028X-65.10.1605
Kingsley, D. H., Vincent, E. M., Meade, G. K., Watson, C. L., and Fan, X. (2014). Inactivation of human norovirus using chemical sanitizers. Int. J. Food Microbiol. 171, 94–99. doi: 10.1016/j.ijfoodmicro.2013.11.018
Leon, J. S., Kingsley, D. H., Montes, J. S., Richards, G. P., Lyon, G. M., Abdulhafid, G. M., et al. (2011). Randomized, double-blinded clinical trial for human norovirus inactivation in oysters by high hydrostatic pressure processing. Appl. Environ. Microbiol. 77, 5476–5482. doi: 10.1128/AEM.02801-10
Li, X., and Chen, H. (2015). Evaluation of the porcine gastric mucin binding assay for high-pressure-inactivation studies using murine norovirus and tulane virus. Appl. Environ. Microbiol. 81, 515–521. doi: 10.1128/AEM.02971-14
Li, X., Chen, H., and Kingsley, D. H. (2013b). The influence of temperature, pH, and water immersion on the high hydrostatic pressure inactivation of GI.1 and GII.4 human noroviruses. Int. J. Food Microbiol. 167, 138–143. doi: 10.1016/j.ijfoodmicro.2013.08.020
Li, X., Ye, M., Neetoo, H., Golovan, S., and Chen, H. (2013a). Pressure inactivation of Tulane virus, a candidate surrogate for human norovirus and its potential application in food industry. Int. J. Food Microbiol. 162, 37–42. doi: 10.1016/j.ijfoodmicro.2012.12.016
Lou, F., Neetoo, H., Chen, H., and Li, J. (2011a). Inactivation of a human norovirus surrogate by high-pressure processing: effectiveness, mechanism, and potential application in the fresh produce industry. Appl. Environ. Microbiol. 77, 1862–1871. doi: 10.1128/AEM.01918-10
Lou, F., Neetoo, H., Li, J., and Chen, H. (2011b). Lack of correlation between virus barosensitivity and the presence of a viral envelope during inactivation of human rotavirus, vesicular stomatitis virus, and avian metapneumovirus by high-pressure processing. Appl. Environ. Microbiol. 77, 8538–8547. doi: 10.1128/AEM.06711-11
Mariutti, L. R. B., Orlien, V., Bragagnolo, N., and Skibsted, L. H. (2008). Effect of sage and garlic on lipid oxidation in high-pressure processed chicken meat. Eur. Food Res. Technol. 227, 337–344. doi: 10.1007/s00217-007-0726-5
Scallan, E., Hoekstra, R. M., Angulo, F. J., Tauxe, R. V., Widdowson, M. A., Roy, S. L., et al. (2011). Foodborne illness acquired in the United States–major pathogens. Emerg. Infect. Dis. 17, 7–15. doi: 10.3201/eid1701.P11101
Seymour, I. J., and Appleton, H. (2001). Foodborne viruses and fresh produce. J. Appl. Microbiol. 91, 759–773. doi: 10.1046/j.1365-2672.2001.01427.x
Steele, M., and Odemeru, J. (2004). Irrigation water as a source of foodborne pathogens on fruit and vegetables. J. Food Prot. 67, 2839–2849. doi: 10.4315/0362-028X-67.12.2839
Tang, Q., Li, D., Xu, J., Wang, J., Zhao, Y., Li, Z., et al. (2010). Mechanism of inactivation of murine norovirus-1 by high pressure processing. Int. J. Food Microbiol. 137, 186–189. doi: 10.1016/j.ijfoodmicro.2009.10.033
Tian, P., Brandl, M., and Mandrell, R. (2005). Porcine gastric mucin binds to recombinant norovirus particles and competitively inhibits their binding to histo-blood group antigens and Caco-2 cells. Lett. Appl. Microbiol. 41, 315–320. doi: 10.1111/j.1472-765X.2005.01775.x
Tian, P., Engelbrektson, A., and Mandrell, R. (2008). Two-log increase in sensitivity for detection of norovirus in complex samples by concentration with porcine gastric mucin conjugated to magnetic beads. Appl. Environ. Microbiol. 74, 4271–4276. doi: 10.1128/AEM.00539-08
Tian, P., Yang, D., Jiang, X., Zhong, W., Cannon, J. L., Burkhardt, W., et al. (2010). Specificity and kinetics of norovirus binding to magnetic bead-conjugated histo-blood group antigens. J. Appl. Microbiol. 109, 1753–1762. doi: 10.1111/j.1365-2672.2010.04812.x
Tian, P., Yang, D., and Mandrell, R. (2011). A simple method to recover Norovirus from fresh produce with large sample size by using histo-blood group antigen-conjugated to magnetic beads in a recirculating affinity magnetic separation system (RCAMS). Int. J. Food Microbiol. 147, 223–227. doi: 10.1016/j.ijfoodmicro.2011.04.013
Tian, P., Yang, D., Pan, L., and Mandrell, R. (2012). Application of a receptor-binding capture quantitative reverse transcription-PCR assay to concentrate human norovirus from sewage and to study the distribution and stability of the virus. Appl. Environ. Microbiol. 78, 429–436. doi: 10.1128/AEM.06875-11
Wei, J., Jin, Y., Sims, T., and Kniel, K. E. (2010). Manure- and biosolids-resident murine norovirus 1 attachment to and internalization by Romaine lettuce. Appl. Environ. Microbiol. 76, 578–583. doi: 10.1128/AEM.02088-09
Wei, J., Jin, Y., Sims, T., and Kniel, K. E. (2011). Internalization of murine norovirus 1 by Lactuca sativa during irrigation. Appl. Environ. Microbiol. 77, 2508–2512. doi: 10.1128/AEM.02701-10
Keywords: high pressure processing, norovirus, Tulane virus, porcine gastric mucin, inactivation
Citation: DiCaprio E, Ye M, Chen H and Li J (2019) Inactivation of Human Norovirus and Tulane Virus by High Pressure Processing in Simple Mediums and Strawberry Puree. Front. Sustain. Food Syst. 3:26. doi: 10.3389/fsufs.2019.00026
Received: 11 October 2018; Accepted: 04 April 2019;
Published: 30 April 2019.
Edited by:
Karl Matthews, Rutgers University, The State University of New Jersey, United StatesReviewed by:
Matthew D. Moore, University of Massachusetts Amherst, United StatesShiowshuh Sheen, United States Department of Agriculture, United States
Copyright © 2019 DiCaprio, Ye, Chen and Li. This is an open-access article distributed under the terms of the Creative Commons Attribution License (CC BY). The use, distribution or reproduction in other forums is permitted, provided the original author(s) and the copyright owner(s) are credited and that the original publication in this journal is cited, in accordance with accepted academic practice. No use, distribution or reproduction is permitted which does not comply with these terms.
*Correspondence: Erin DiCaprio, ZWxkaWNhcHJpb0B1Y2RhdmlzLmVkdQ==