- 1Department of Poultry Science, University of Georgia, Athens, GA, United States
- 2United States Department of Agriculture, Agricultural Research Service, U.S. National Poultry Research Center, Egg Safety and Quality Research Unit, Athens, GA, United States
- 3Norel Animal Nutrition, Madrid, Spain
- 4Southern Poultry Research Group, Athens, GA, United States
The objective of this study was to determine the effects of protected sodium butyrate (SB), and protected sodium butyrate plus essential oils (carvacrol and ginger; SBEO) on the cecal microbiota of broilers challenged with Eimeria maxima and Clostridium perfringens. Birds were assigned to 4 treatments (8 replicates pens of 58 birds/pen): unchallenged control; challenged control; challenged and supplemented with SB; challenged and supplemented with SBEO. On d 13, challenged birds were orally inoculated with ~5,000 Eimeria maxima oocysts. On d 18–19, the same birds were exposed to Clostridium perfringens via drinking water (~8 log CFU/ml). Cecal excreta was collected at d 12, 18, 21, and 28 for microbiota analysis through 16s rRNA sequencing using Illumina MiSeq platform and analyzed using QIIME v. 1.9.1 The cecal microbiota was analyzed over time within each experimental group. The inclusion of SB alone or in combination with EO contributed to larger variations in the cecal microbiota over time than the unsupplemented treatments, as shown by the diversity indices. The community structure and abundance of the cecal microbiota were significantly different across ages, especially in the groups supplemented with SB and SBEO. As shown in the PCoA analysis, the supplementation of SB led to a more stable microbial community and lower between-sample variability over time. In the unchallenged control birds, Ruminococcus decreased (p = 0.006), whereas Bacteroides and Clostridiales increased (p ≤ 0.02) as the birds aged. In the challenged control group, however, the frequency of Coprococcus and Blautia decreased as birds aged (p ≤ 0.01), and, Clostridiales did not increase. Supplementation of SB, but not SBEO, increased the frequency of Lactobacillus (p = 0.01) on d 12 compared to d 18 and d 28, and prevented the reduction in the frequency of Blautia as the birds aged. Nevertheless, supplementation of SB and SBEO contributed to unique changes in the predicted functions of the cecal microbiota over time, which was not observed in the unsupplemented birds. SB and SBEO modulated the diversity, composition, and predictive function of the cecal microbiota which may have lowered the negative impact of necrotic enteritis (NE).
Introduction
Due to the recent restrictions in the use of antimicrobial growth promoters in the diets of broiler chickens, and move toward a more judicious antibiotic use, the search for gut health feed additives is becoming increasingly important as the incidence of enteric diseases has grown in commercial flocks submitted to antibiotic-free programs (Kaldhusdal et al., 2016; Broom, 2017). Several alternatives have been evaluated and different mechanisms of action have been proposed. However, there is still a lack of knowledge on how different feed additives could at least partially attenuate the impact of enteric diseases, such as coccidiosis and necrotic enteritis (NE) in broiler flocks.
Coccidiosis is one of the most important predisposing factors for the development of NE in chickens (Prescott et al., 2016). The increased mucus production that occurs due to the Eimeria infection (Collier et al., 2008), and leakage of plasma proteins into the intestinal lumen (Prescott et al., 2016) increase the proliferation of Clostridium perfringens, the causative agent of NE (Prescott et al., 2016). Besides increasing the susceptibility to NE, coccidiosis also leads to changes in the overall structure of the intestinal microbiota (Stanley et al., 2014; Wu et al., 2014; Zhou et al., 2017). Wu et al. (2014) demonstrated a reduction in the cecal microbial diversity following Eimeria infection, and reduction of many members of the family Ruminococcaceae. Yet, Eimeria infection caused changes in short chain fatty acids (SCFA) produced in the ceca of chickens (Stanley et al., 2014).
Clostridium perfringens infection is also associated with shifts in the intestinal microbiota (Stanley et al., 2012, 2014; Antonissen et al., 2016). C. perfringens can interact and compete with other microorganisms in the gut, which may alter the proliferation of C. perfringens, production of toxins and the severity of the disease (Antonissen et al., 2016). Stanley et al. (2012) reported that NE infection changed the abundance of important bacterial families in the gut, such as Clostridiales and Lactobacillales. Additionally, SCFA-producing bacteria as well as segmented filamentous bacteria, such as Candidatus Savagella, decreased due to NE challenge, demonstrating that the overall dysbiosis may also be related to the pathogenesis of the disease (Stanley et al., 2014). Thus, it is reasonable to hypothesize that different dietary supplements administered to NE infected flocks may directly influence the diversity and composition of the intestinal microbiota, mainly through growth and proliferation of commensal bacteria that play important roles on the general physiology of the host.
Butyrate has multiple effects on the intestine. It serves as an energy source for epithelial cells, stimulates mucus production, controls the intestinal barrier function, promotes pathogen control, and modulates the immune-system (Guilloteau et al., 2010). Therefore, it is believed that butyrate may alleviate the negative effects of NE in chickens by modulating the intestinal microbiota, immune-system, and the intestinal barrier function. We recently showed that dietary supplementation of chickens with a protected source of sodium butyrate (SB) modulated the diversity, composition, and predictive function of the cecal microbiota (Bortoluzzi et al., 2017). Indeed, SB may directly modulate the intestinal microbiota, through its bactericidal effect, or indirectly by stimulating the growth of beneficial lactic acid bacteria (Ahsan et al., 2016). Additionally, essential oils (EO) obtained from plant materials are known to possess antimicrobial, antioxidant, and anti-inflammatory properties (Brenes and Roura, 2010). Jerzsele et al. (2012) showed that a blend of ginger oils and carvacrol reduced the gross lesions caused by NE and had beneficial effects on the intestinal morphology.
Therefore, we hypothesized that supplementing SB alone or in combination with EO would beneficially modulate the cecal microbiota of broiler chickens challenged with E. maxima and C. perfringens, thereby alleviating the severity of challenge and improving birds performance. The objective of this study was to evaluate the effects of SB alone or in combination with EO on the balance (in terms of diversity, composition, and predicted function) of the cecal microbiota of broiler chickens challenged with E. maxima and C. perfringens.
Material and Methods
Housing, Birds and Treatments
The animal care and use procedures followed the Guide for the Care and Use of Agricultural Animals in Research and Teaching (Federation of Animal Science Societies, 2010) under supervision of a licensed poultry veterinarian. One thousand eight hundred fifty-six (1,856) one-day-old male Ross chicks were assigned to four (4) treatment groups with eight (8) replicate pens per treatment, and 58 birds per pen raised on new litter. The treatments were: non-supplemented non-challenged group (unchallenged control); non-supplemented and challenged control; challenged and supplemented with SB (70% of sodium butyrate protected with sodium salts of palm fatty acids; ChaSB; 0.1% inclusion); and challenged and supplemented with SB plus essential oils (70% of sodium butyrate protected with sodium salts of palm fatty acids, and carvacrol and ginger oil −0.5% each in the commercial product; ChaSBEO; 0.1% inclusion). Each experimental diet was mixed separately, and the feed additives were supplemented to replace kaolin in the unsupplemented diet. The products containing SB and SBEO were provided by Norel Animal Nutrition, Madrid, Spain.
Feed consisted of a non-medicated commercial-type broiler starter, grower, and finisher diets compounded according to NRC guidelines (NRC, 1994) and contained feedstuffs commonly used in the United States (Table 1). The feeds were available ad libitum from date of chick arrival as follows: Starter, d 0 until d 13; grower, d 14 to d 34; and finisher, d 34 to d 41 (study termination). Diets were fed as crumbles (starter feed) or pellets (grower and finisher feed).
The challenge model consisted of coccidial vaccine (Coccivac®-B52) administered at d 0 by spray cabinet, and individual inoculation with ~5,000 sporulated oocysts of Eimeria maxima by oral gavage on d 13, kindly provided by Dr. Lorraine Fuller, from the Department of Poultry Science, University of Georgia. On d 18, and 19, the same birds were challenged with C. perfringens, as follow: feed was withdrawn for 4 h and water was withdrawn for 2–3 h prior to administration of C. perfringens. A measured amount of water (~200 mL with C. perfringens) that was consumed within 30 min was used for each pen. A C. perfringens culture (~1 × 108 cfu/mL total) was added to this water and thoroughly mixed and given to birds in each challenge pen. Once the challenge water was consumed, treatment feed and water were returned to pen.
Sample Collection and Analysis Performed on Days 12, 18, 21, and 28
Two birds per pen were euthanized by cervical dislocation and the ceaca were collected into a sterile Ziploc bag, immediately put in ice, and brought to the lab. Thus, the cecal content of the two birds was pooled in a new sterile Ziploc bag, and thoroughly homogenized by hand for further microbiota analysis. The content was then diluted in a 1:10 sterile phosphate-buffered saline solution (PBS; ph of 7.4); 1 mL of the solution was transferred to a 2 mL micro-tube, centrifuged for 3 m at 3,270 g, the supernatant discarded, and 200 μg of the content used for DNA isolation.
DNA Extraction of the Cecal Microbiota
The DNA isolation was conducted following the manufacturer recommendations (PowerViral Environmental RNA/DNA Isolation Kit—Mo Bio; QIAGEN, Carlsbad, CA, USA), with a slight modification as described by Bortoluzzi et al. (2017). The quality and presence of DNA was verified by Nanodrop (NanoDrop 1000 Spectrophotometer; Thermo Fischer Scientific, Wilminton, DE, USA) and agarose gel electrophoresis (1.5%), respectively. Only the DNA with a 260:280 ratio of 1.80 to 2.00 in the Nanodrop was used for further analysis.
PCR Amplification and Sequencing
The V3-V4 hypervariable region of the 16S rRNA gene was amplified using the primer FwOvAd_341f and ReOvAd_785r as previously described (Klindworth et al., 2013). All the procedures followed a standardized protocol described previously (Bortoluzzi et al., 2017). The 16S rRNA gene was then sequenced using the Illumina Miseq platform.
Bioinformatics
All sequence data processing was performed using QIIME v. 1.9.1 software package (Caporaso et al., 2010). Sequences were paired-end and quality trimmed using Geneious (Newark, NJ). High-quality sequences were aligned against the SILVA database (Ribocon GmbH, Bremen, Germany) release 119 (Pruesse et al., 2007). UCHIME software (Tiburon, CA) was used to identify and remove chimeric sequences (Edgar et al., 2011). Number of sequences per sample was normalized based on the sample with the lowest number of reads for statistical comparison (Gihring et al., 2012). Operational taxonomic units (OTUs) were assigned at a 97% identity using SILVA database. Alpha (richness: Observed Species, Chao1; diversity: Shannon, and Phylogenetic Diversity (PD) of the Whole Tree), and beta diversity indices were calculated using QIIME v1.9.1. Nonparametric statistical tests PERMANOVA and ANOSIM were used to compare categories, and the similarity between the microbiota, respectively, using the weighted (quantitative) UniFrac metric measure. Principal Coordinates Analysis (PCoA) was used to visualize the data. Phylogenetic Investigation of Communities by Reconstruction of Unobserved States (PICRUSt) analysis was carried out using the KEGG and Clusters of Orthologous Groups of proteins (COGs) databases. For this, a closed-reference OTU table was normalized by the 16S rDNA copy number, the metagenome was predicted, and categorized by function based on Kyoto Encyclopedia of Genes and Genomes (KEGG; Uji, Kyoto, Japan) pathway (Kanehisa and Goto, 2000). The obtained biome file was processed by STAMP (Halifax, Nova Scotia, Canada) version 2.1.3 (Parks et al., 2014).
Statistical Analysis
The data was analyzed as a completely randomized design, with 8 replicates each treatment (n = 8). From each replicate, the cecal content of birds was pulled for microbiota analysis. The frequency of the main bacterial groups observed was submitted to a non-parametric one-way ANOVA (Kruskal-Wallis test) and, in case of significant difference (P ≤ 0.05), means were separated by Dunn test, using SAS 9.4 (SAS Institute, 2011). Welch's T-test was applied to compare the KEGG pathways (P ≤ 0.05).
Results
Alpha and Beta Diversity Indices of the Cecal Microbiota
Chao index (minimal number of OTU present in a sample), number of observed species (number of species present in a community), phylogenetic diversity (PD) of the whole tree (minimum total length of all the phylogenetic branches required to span a given set of taxa on the phylogenetic tree), and Shannon index (abundance and evenness of the species present in a sample) were evaluated as measures of alpha diversity indices (Tables 2, 3). Comparisons among timepoints were performed to evaluate the transition of the cecal microbiota whithin each experimental treatment. The different treatments differently changed the microbiota across ages, as shown by the diversity indices.
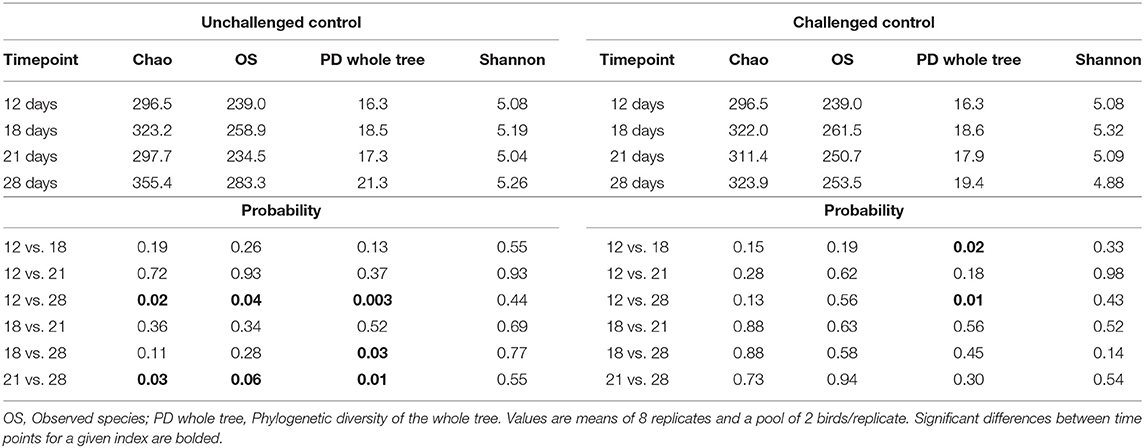
Table 2. Alpha diversity indexes of the cecal microbiota of broiler chickens at different timepoints considering the unsupplemented treatments and unchallenged or challenged with a necrotic enteritis infection model.
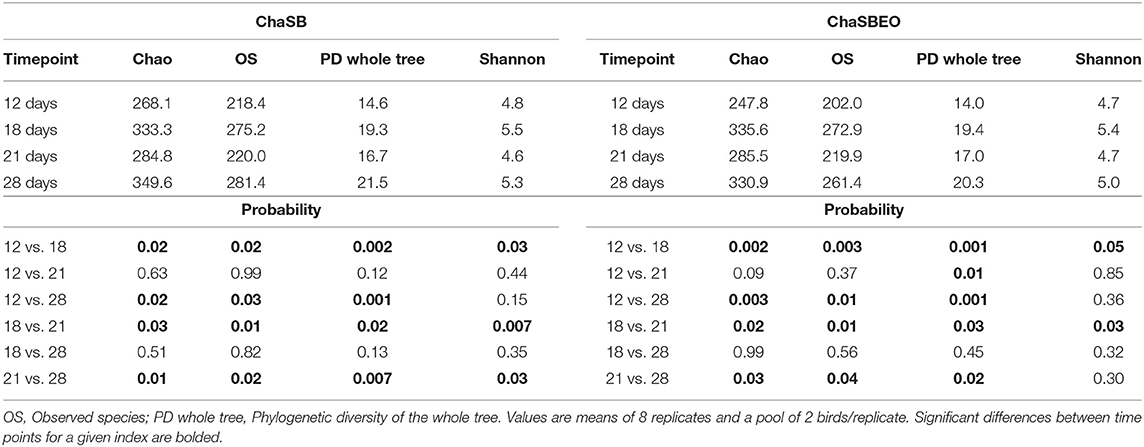
Table 3. Alpha diversity indexes of the cecal microbiota of broiler chickens at different timepoints considering the necrotic enteritis challenged birds supplemented with sodium butyrate (SB) or sodium butyrate plus essential oils (SBEO).
When considering the unchallenged control group (Table 2), it was observed that richness and evenness were higher, mainly on d 28 of age when compared to earlier ages. However, considering the challenged control group, only few differences were observed in terms of richness and evenness across ages (Table 2). On the other hand, when the birds were challenged and supplemented with either SB or SBEO (Table 3) many differences were observed, showing that the supplementation of these feed additives had a strong effect on the cecal microbiota across ages.
The community structure and abundance of the cecal microbiota were significantly different across the experimental groups and ages. Both community membership (unweighted) and structure (weighted) contributed to differences in cecal microbiota across ages. PERMANOVA analysis was conducted to describe the strength and significance that treatment groups and age had in determining the variation among distance matrixes (weighted UniFrac). These differences, that are shown using Principal Coordinated Analysis (PCoA) plots, were evident across ages within each experimental treatment (Figure 1). ANOSIM was used to compare the similarity among the cecal microbiota. Differences were observed across ages within different treatment groups (Table 4). The largest difference in the microbiota was observed on d 12 vs. 28 when birds were challenged and supplemented with SB (R = 0.97 and P = 0.001; “R” is the index of ANOSIM that indicates the similarity of comparison between group pairs. “R” ranges from −1 to 1: the pairs are more similar when the R index is closer to 0 and the pairs are different from each other when the R index is close to 1).
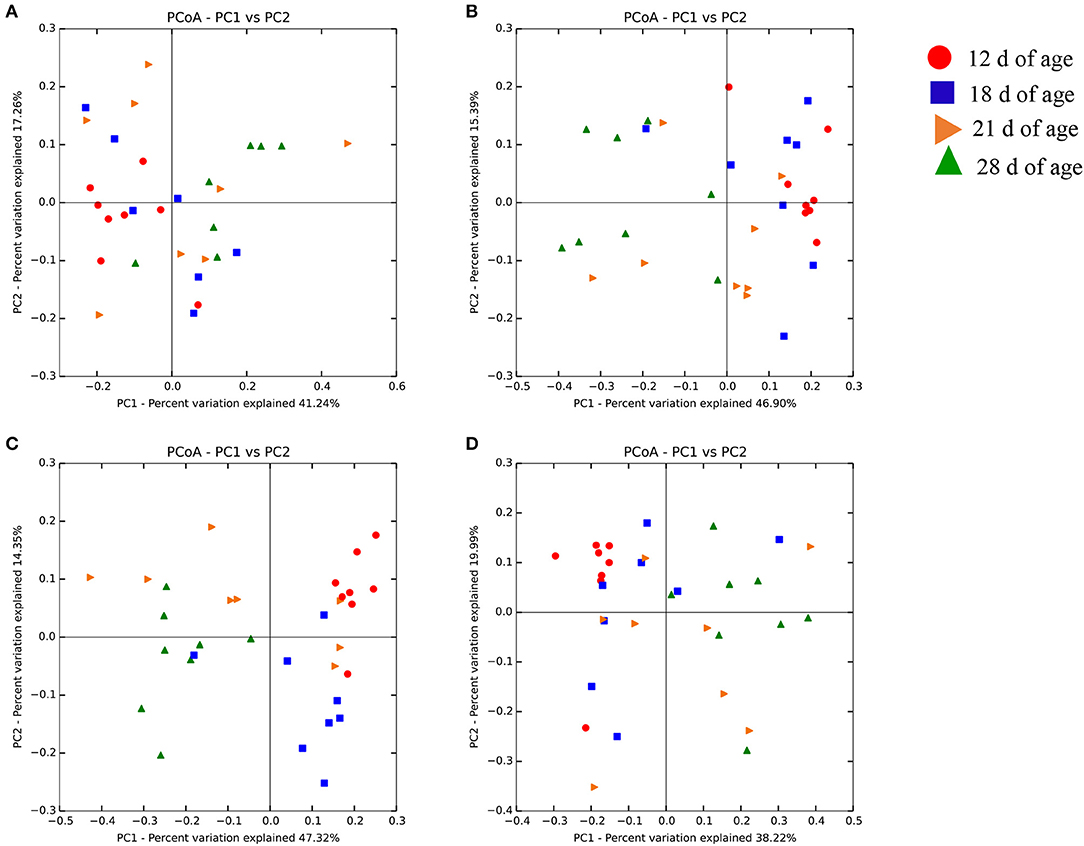
Figure 1. Weighted UniFrac PCoA of the cecal microbiota in the unchallenged control (A), challenged control (B), challenged and supplemented with sodium butyrate (C), and challenged and supplemented with sodium butyrate plus essential oils (D) according to the different moments of sample collection.
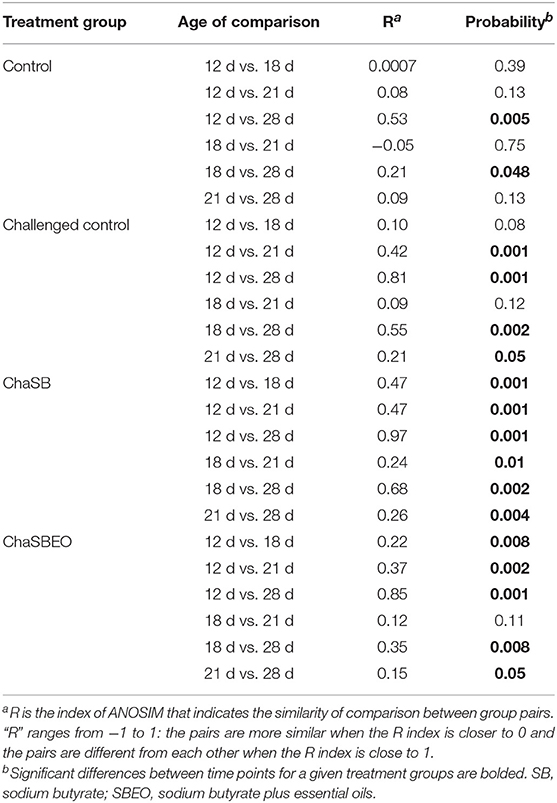
Table 4. ANOSIM analysis results comparing the different timepoints within each of the treatment groups.
Composition of the Cecal Microbiota
The cecal microbiota in the four ages evaluated was dominated by bacteria belonging to the Phylum Firmicutes, followed by Bacteroidetes and Proteobacteria (Table 5). The effect of age on the relative abundance of the main phylum was accessed for the four experimental groups. First, it was observed that the frequency of Firmicutes significantly decreased (87.1 vs. 61.9%; P = 0.0002), and Bacteroidetes increased (9.9 vs. 31.2%; P = 0.034) in the cecal microbiota of unchallenged control birds on d 28 compared to d 12. The same effect was observed within the challenged control group; however, a higher frequency of Proteobacteria (0.1% on d 12 vs. 1.7% on d 18; P = 0.002) was observed after the birds were challenged, which was not observed in the unchallenged control treatment. On the other hand, when the birds were challenged and supplemented with SB or SBEO, the differences in the frequency of Firmicutes and Bacteroidetes were observed at an early stage (d 21). The increase in the frequency of Proteobacteria due to the challenge when the birds were supplemented with SBEO was not as evident as in the other challenged treatments.
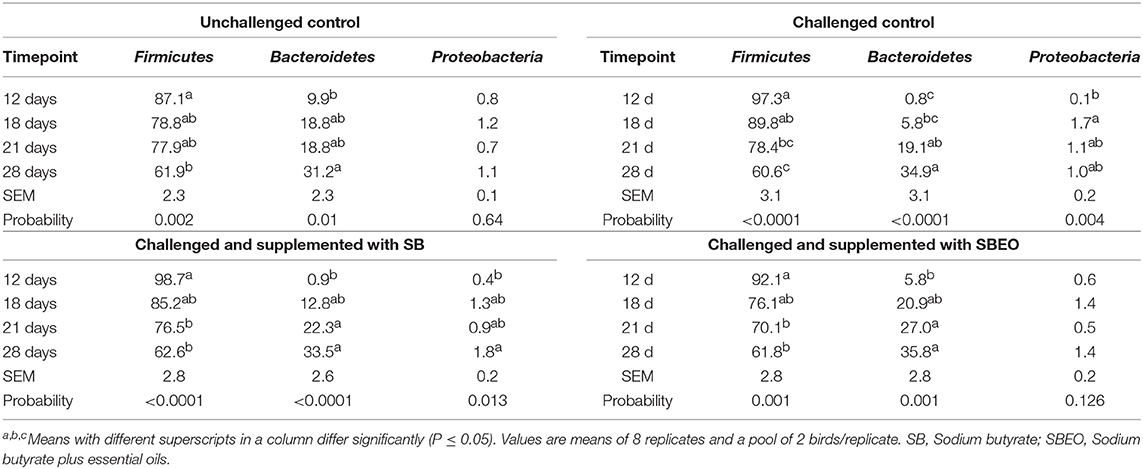
Table 5. Relative abundance (%) of the main phylum in the cecal microbiota of broiler chickens at different timepoints whithin each one of the experimental groups.
The most abundant genera observed in the cecal microbiota were Ruminococcus, Lactobacillus, and Bacteroides, followed by unclassified Ruminococcaceae and Clostridiales (Figure 2). Again, the effect across ages was evaluated within each experimental treatment. In the unchallenged control birds, it was observed that Ruminococcus decreased (34.1% on d 12 vs. 14.3% on d 18; P = 0.006), whereas Bacteroides (6% on d 12 vs. 23% on d 28; P = 0.02) and Clostridiales (3.4% on d 12 vs. 9.1% on d 28; P = 0.006) increased as the birds aged. In the challenged control group, however, besides similar effects on Ruminococcus (P = 0.001) and Bacteroides (P = 0.001), it was also observed that the frequency of Coprococcus (5.6% o d 12 vs. 0.9% on d 28; P = 0.01) and Blautia (4.7% on d 12 vs. 2% on 28; P = 0.02) decreased as birds aged. This was an effect caused by the challenge, as it was not observed in the unchallenged control group. Clostridiales did not increase as an effect of age in these challenged control birds (Figure 2).
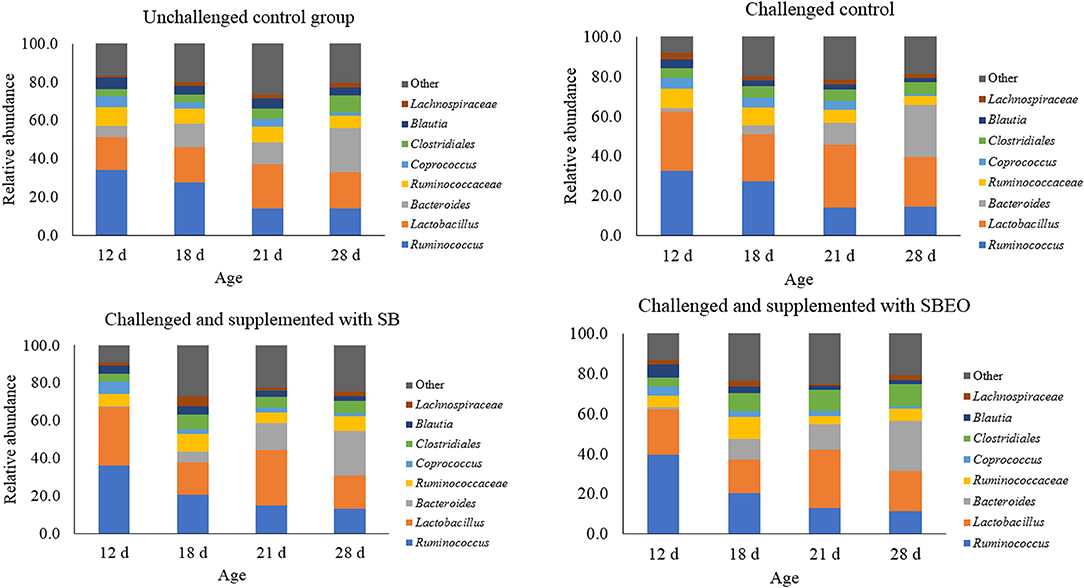
Figure 2. Relative abundance (%) of the main bacterial group present in the cecal microbiota of broiler chickens in different timepoints within each experimental treatment (Values are means of 8 replicates and a pool of 2 birds/replicate). SB, Sodium butyrate; SBEO, Sodium butyrate plus essential oils.
In the challenged SB supplemented birds, the effect of age was also evident on the frequency of Ruminococcus (P = 0.002), Lactobacillus (P = 0.01), Bacteroides (P = 0.001), and Coprococcus (P = 0.02). The frequency of Lachnospiraceae also tended to be affected by age (P = 0.07) in this treatment. The frequency of Lactobacillus was lower at d 18 (17.1%), but not at d 21 (29.5%), compared to d 12; Lachnospiraceae tended to have higher abundance on d 18 (5.1%), compared to the other ages, and Ruminococcus and Coprococcus followed the same pattern as observed in the challenged control group. Supplementation of SB to challenged birds did not reduce the abundance of Blautia, as observed in the challenged control group (Figure 2). Lastly, looking at the effects of age in the challenged and SBEO supplemented birds, similar results were observed as the challenged SB birds. Ruminococcus decreased (39.6% on d 12 vs. 11.5% on d 28; P = 0.001), Bacteroides increased (1.2% on d 12 vs. 25% on d 28; P = 0.001), and Blautia decreased (6.5% on d 12 vs. 2.3% on d 28; P = 0.02); additionally, Lactobacillus tended to be higher at d 21 (29.2%; P = 0.06), and Ruminococcaceae had a higher abundance at d 18 compared to d 21 (11% vs. 4.2%; P = 0.02; Figure 2).
Prediction of the Cecal Microbiota Functions
To predict microbial community functions from the microbiota data, phylogenetic investigation of communities by reconstruction of unobserved states (PICRUSt) analysis was carried out using the KEGG and Clusters of Orthologous Groups of proteins (COGs) databases. The focus of this analysis was to evaluate the changes in the predicted functions comparing d 12 (pre-challenge conditions) with d 21 (after coccidia and C. perfringens challenge) within each experimental treatment. A Venn diagram was constructed to visualize the shared and unique predicted functions among the treatments which were divided between upregulated (Figure 3A) and downregulated predictive functions (Figure 3B) on d 21 compared to d 12. Among the upregulated predicted functions, it was observed that 11, 11, and 9 were unique to the challenged, challenged supplemented with SB, and challenged supplemented with SBEO, respectively. Only few functions were downregualed on d 21 compared to d 12, wherein only 1, 4, and 2 were unique to the challenged, challenged supplemented with SB, and challenged supplemented with SBEO, respectively.
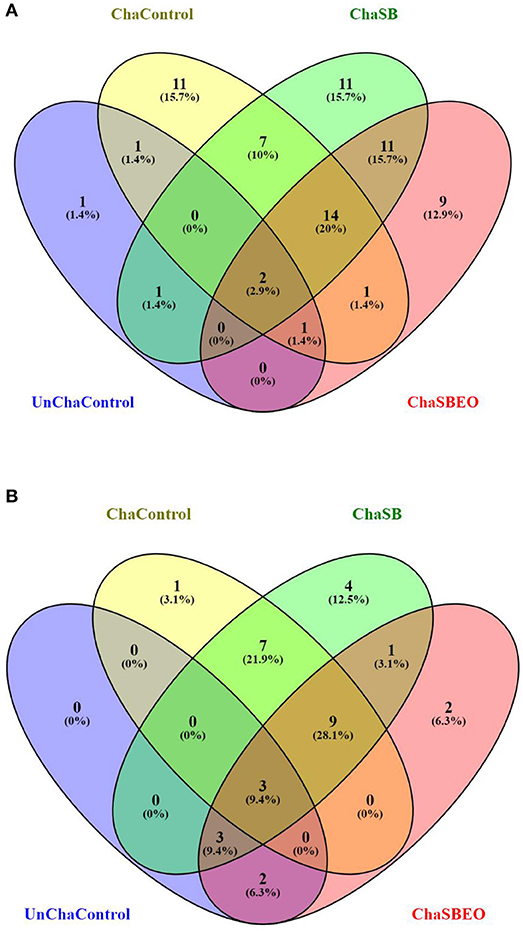
Figure 3. Venn diagram of the up (A) and downregulated (B) predicted functions on d 21 compared to d 12 according to each experimental group. Absolute number represent the number of different predicted functions among the groups, followed by its respective percentage. SB, Sodium butyrate; SBEO, Sodium butyrate plus essential oils.
When evaluating the effect of the challenge, the predicted functions common to the unchallenged control treatment were removed. When evaluating the effect of the supplementation of SB and SBEO, the predicted functions shared with the unchallenged and challenged control were also filtered out, thereby the remaining functions were unique to SB or SBEO supplementation. In the unchallenged control treatment (Figure 4A) few changes were observed in the predictive functions of the cecal microbiota as the birds aged. A total of 14 pathways were changed (P ≤ 0.01) comparing d 21 vs. d 12. A larger number of functional categories of the cecal microbiota in the challenged control treatment were changed on d 21 vs. d 12 (Figure 4B). These changes were due mainly to the challenge since the functions shared with the unchallenged control were filtered out. A total of 30 features were unique to the treatment supplemented with SB wherein most of them were related to metabolism, and one related to penicillin and cephalosporin biosynthesis (Figure 5A). Additionally, 23 functional categories were unique to the SBEO supplemented treatment which included, besides penicillin and cephalosporin biosynthesis, biosynthesis of vancomycin group of antibiotics, and streptomycin biosynthesis that were enriched on d 21 vs. d 12 (Figure 5B).
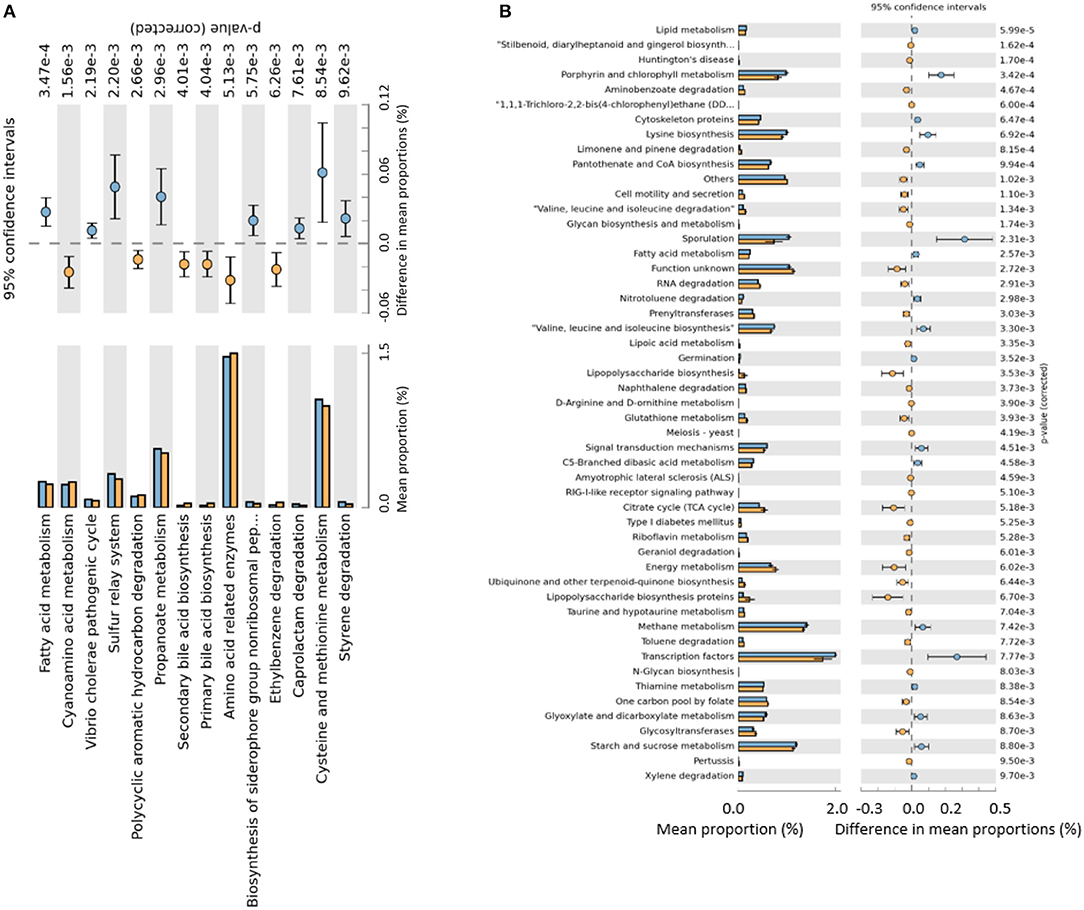
Figure 4. Predicted functions of the cecal microbiota unique to the unchallenged control birds (A) and unique to challenged control birds (B) comparing 12 d (blue bars) vs. 21 d (orange bars) with P ≤ 0.01.
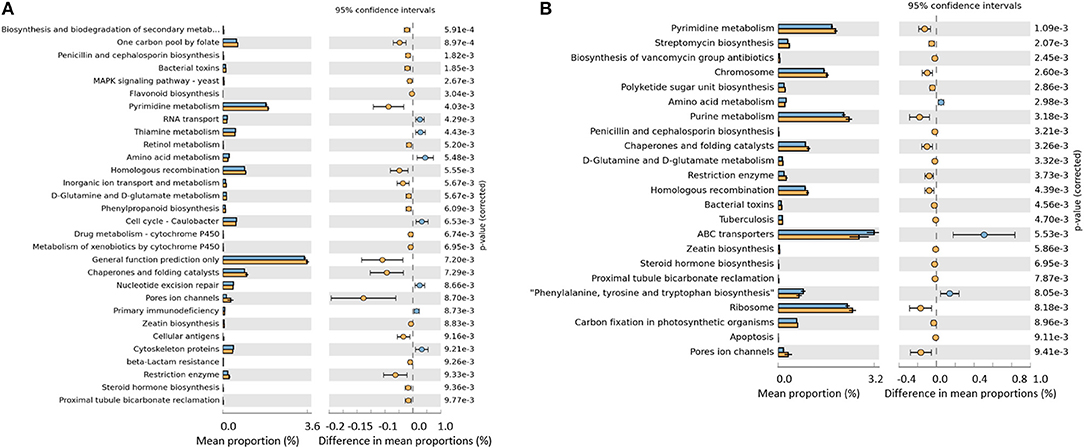
Figure 5. Predicted functions of the cecal microbiota unique to challenged and sodium butyrate supplemented birds (A) and unique to challenged and sodium butyrate plus essential oils supplemented birds (B) comparing 12 d (blue bars) vs. 21 d (orange bars) with P ≤ 0.01.
Discussion
In the present study, SB improved FCR of the chickens before the challenge (1–13 d), and both SB and SBEO completely recovered the performance of the birds in terms of FCR in the overall experimental period (1–41 d) compared to challenged control birds. Additionally, SB and SBEO partially attenuated the severity of NE lesions, and numerically decreased the oocyst shedding (this data will be published elsewhere). The search for feed additives contributing to the health and formation of the gut has become even more important due to the recent restrictions to the use of antibiotics for growth promotion by the poultry industry, which may increase the incidence of NE in broilers. The GIT acts as an interface between diet, host, and gut microbiota, and has vital role in the health status of an animal. In the present study, we evaluated the action of SB alone or in combination with EO in broiler chickens submitted to a NE infection model, and their effects on the establishment of the cecal microbiota. Nutrition affects the composition of the microbiota (Pan and Yu, 2014), and the functions that the microorganisms are going to perform on the host. Nutritional strategies targeting the modulation of the microbiota before and after a challenge have the potential to improve development and regeneration of the injured intestinal mucosa, digestive physiology, immune system and inflammation (Kogut, 2017).
Few studies have investigated the effect of Eimeria infection on the microbial community richness (Stanley et al., 2012; Macdonald et al., 2017; Zhou et al., 2017), and have observed that challenge itself does not have a strong effect on this parameter. Challenge with Eimeria plus C. perfringens, however, drastically changes the diversity and composition of the cecal microbiota (Stanley et al., 2014). Feed additives, such as SB and EO, may indirectly modulate the intestinal microbiota by their effects on the immune-system, or directly by their effects on the bacteria population. Indeed, supplementation of SB and SBEO, as well as the infection model used herein, modulated the diversity, composition, and predicted function of the cecal microbiota. The diversity of the cecal microbiota changed as the birds aged; however, SB and SBEO supplementation to challenged birds had an critical impact on the establishment of the microbiota over the rearing cycle of the birds. As shown in the PCoA plot (Figure 1), the supplementation of SB reduced the variability between samples and promoted a more stable microbiota over time, which was not observed in the unsupplemented or SBEO supplemented challenged birds.
At the phylum level, Firmicutes decreased and Bacteroidetes increased over time, regardless the treatment group; the induction to NE, on the other hand, increased the frequency of Proteobacteria-related species, but the supplementation of SBEO prevented this effect (Table 5). Additionally, NE challenge impaired the increase of Clostridiales-related bacteria over time, and led to a reduction of Blautia, but SB supplementation prevented the later effect. Non-pathogenic Clostridia species, such as Blautia, can use carbohydrates as a fermentable substrate and produce SCFA as the major end products of glucose fermentation which can reduce the incidence of inflammatory diseases (Sokol et al., 2008; Park et al., 2012; Fujimoto et al., 2013; Bai et al., 2016). Therefore, dietary SB modulated the cecal microbiota of infected chickens, which can also explain its beneficial effects on the performance of the birds.
Bortoluzzi et al. (2017) observed that dietary inclusion of SB per se did not have a significant impact on the cecal microbiota of broiler chickens. However, when the birds were fed a nutritionally-reduced diet, the composition and predicted function of the cecal bacteria community drastically varied, and the supplementation of SB reduced these variations. Similar findings have been observed by Zhou et al. (2017) wherein coated SB had no significant effect on the cecal microbiota of healthy chickens, but balanced the shifts of microbial composition caused by E. tenella infection. Additionally, the ANOSIM analysis revealed that the cecal microbiota showed more differences across ages when the birds were supplemented with SB or SBEO vs. the unsupplemented groups. Taken together, these results indicate that supplementation of SB mainly is beneficial in speeding the establishment of the cecal microbiota, which may be important in terms of resistance and recovery from enteric diseases.
In the absence of transcriptome data, PICRUSt was applied to predict the metagenome from 16S rRNA data and a reference genome database (Langille et al., 2013). Previously, SB supplementation was shown to reduce the variations of the predicted functions caused by a nutritional challenge (Bortoluzzi et al., 2017). In the present study, we observed that SB and SBEO led to many variations in the predicted functions of the cecal microbiota over time, besides the variations related to the normal aging of the birds and/or challenge. The changes in the predicted functions of the cecal microbiota attributed to the supplementation of SB and SBEO may have contributed to the better performance observed in these group of birds, even under challenge conditions. Functions related to metabolism and synthesis of antibiotics were enriched when the birds were supplemented with SB (1 category of antibiotic synthesis) or SBEO (3 categories of antibiotic synthesis). Indeed, the cecal microbiota of birds presenting better feed efficiency was enriched with functions related to glucometabolism, ion transportation and amino acid metabolism (Yan et al., 2017). The enrichment of functional categories related to antibiotic synthesis may have reduced the impact of NE, promoted better feed efficiency in these group of birds, and help explain the mechanism of action of these feed additves.
Conclusion
Although the interactions between commensal bacteria and nutrition in health and challenge situations are not fully understood, dietary nutrients, and feed additives are responsible for modulating the population of commensal and pathogenic microorganisms. As such, the understanding of these interactions, in both physiological and pathological situations, will allow the use of feed additives to promote a better growth during enteric pathogen challenges.
Overall, SB and SBEO supplementation to NE-challenged birds contributed to changes in the diversity, composition and predicted functions of the cecal microbiota. Older birds presented a cecal microbiota with lower and higher abundance of Firmicutes and Bacteroidetes, respectively, than younger birds. Additionally, Proteobacteria was observed in higher frequency after challenge, in challenged control vs. SBEO supplemented birds. NE challenge led to a decrease in the frequency of Blautia over time, but dietary SB prevented this effect. SB and SBEO modulated the predicted function of the many metabolic pathways of the cecal microbiota over time, which potentially explains the improvement in performance obtained with these feed additives. Therefore, these feed additves may be considered as potential replacements of the antimicrobial growth promoters in diets of broiler chickens.
Author Contributions
CB, JM, MP, CH, and TA were responsible for the experimental design. CH performed the animal trial. CB collected the samples and performed the laboratory analysis, and wrote the manuscript. BV assisted with the laboratory analysis. MR assisted with the bioinformatic analysis. All the authors read and approved the last version of the manuscript.
Funding
The study was financially supported by Norel Animal Nutrition.
Conflict of Interest Statement
JM, and MP are employed by Norel, Animal Nutrition who manufactures the products used in the present research. CB, MR, BV, CH, and TA have no conflict of interest to declare.
Acknowledgments
We would like to thank Dr. Lorraine Fuller from the Department of Poultry Science of University of Georgia for providing the Eimeria maxima oocysts.
References
Ahsan, U., Cengiz, O., Raza, I., Kuter, E., Chacher, M. F. A., Iqbal, Z., et al. (2016). Sodium butyrate in chicken's nutrition: the dynamics of performance, gut microbiota, gut morphology, and immunity. World's Poult. Sci. J. 72, 265–275. doi: 10.1017/S0043933916000210
Antonissen, G. V., Eeckhaut, K. V., Driessche, L., Onrust, F., Haesebrouck, R., et al. (2016). Microbial shifts associated with necrotic enteritis. Avian Pathol. 45, 308–312. doi: 10.1080/03079457.2016.1152625
Bai, G., Ni, K., Tsuruta, T., and Nishino, N. (2016). Dietary casein and soy protein isolate modulate the effects of raffinose and fructooligosaccharides on the composition and fermentation of gut microbiota in rats. J. Food Sci. 81, 2093–2098. doi: 10.1111/1750-3841.13391
Bortoluzzi, C., Pedroso, A. A., Mallo, J. J., Puyalto, M., Kim, W. K., and Applegate, T. J. (2017). Sodium butyrate improved performance while modulating the cecal microbiota and regulating the expression of intestinal-immune related genes of broiler chickens. Poult. Sci. 96, 3981–3993. doi: 10.3382/ps/pex218
Brenes, A., and Roura, E. (2010). Essential oils in poultry nutrition: main effects and modes of action. Anim. Feed Sci. Tech. 158, 1–14. doi: 10.1016/j.anifeedsci.2010.03.007
Broom, L. J. (2017). Necrotic enteritis: current knowledge and diet-related mitigation. World's Poult. Sci. J. 73, 281–292. doi: 10.1017/S0043933917000058
Caporaso, J. G., Kuczynski, J., Stombaugh, J., Bittinger, K., Bushman, F. D., Costello, E. K., et al. (2010). Qiime allows analysis of high-throughput community sequencing data. Nat. Meth. 7, 335–336. doi: 10.1038/nmeth.f.303
Collier, C. T., Hofacre, C. L., Payne, A. M., Anderson, D. B., Kaiser, P., Mackie, R. I., et al. (2008). Coccidia-induced mucogenesis promotes the onset of necrotic enteritis by supporting Clostridium perfringens growth. Vet. Immunol. Immunopathol. 122, 104–115. doi: 10.1016/j.vetimm.2007.10.014
Edgar, R. C., Haas, B. J., Clemente, J. C., Quince, C., and Knight, R. (2011). UCHIME improves sensitivity and speed of chimera detection. Bioinformatics 27, 2194–2200. doi: 10.1093/bioinformatics/btr381
Federation of Animal Science Societies (2010). Guide for the Care and Use of Agricultural Animals in Research and Teaching. 3rd edn. Champaign, IL.
Fujimoto, T., Imaeda, K., Takahashi, K., Kasumi, E., Bamba, S., Fujiyama, Y., et al. (2013). Decreased abundance of Faecalibacterium prausnitzii in the gut microbiota of Crohn's disease. J. Gastroenterol. Hepatol. 28, 613–619. doi: 10.1111/jgh.12073
Gihring, T. M., Green, S. J., and Schadt, C. W. (2012). Massively parallel rRNA gene sequencing exacerbates the potential for biased community diversity comparisons due to variable library sizes. Environ. Microbiol. 14, 285–290. doi: 10.1111/j.1462-2920.2011.02550
Guilloteau, P., Savary, G., Jaquelin-Peyrault, Y., Rome, V., Le Normand, L., and Zabielski, R. (2010). Dietary sodium butyrate supplementation increases digestibility and pancreatic secretion in young milk-fed calves. J. Dairy. Sci. 93, 5842–5850. doi: 10.3168/jds.2009-2751
Jerzsele, A., Szeker, K., Csizinszky, R., Gere, E., Jakab, C., Mallo, J. J., et al. (2012). Efficacy of protected sodium butyrate, a protected blend of essential oils, their combination, and Bacillus amyloliquefaciens spore suspension against artificially induced necrotic enteritis in broilers. Poult. Sci. 91, 837–843. doi: 10.3382/ps.2011-01853
Kaldhusdal, M., Benestad, S. L., and Lovland, A. (2016). Epidemiologic aspects of necrotic enteritis in broiler chickens—disease occurrence and production performance. Avian Pathol. 45, 271–274. doi: 10.1080/03079457.2016.1163521
Kanehisa, M., and Goto, S. (2000). KEGG: kyoto encyclopedia of genes and genomes. Nucleic Acids Res. 28, 27–30. doi: 10.1093/nar/28.1.27
Klindworth, A., Pruesse, E., Schweer, T., Peplies, J., Quast, C., Horn, M., et al. (2013). Evaluation of general 16S ribosomal RNA gene PCR primers for classical and next-generation sequencing-based diversity studies. Nucleic Acids Res. 41:e1. doi: 10.1093/nar/gks808
Kogut, M. H. (2017). Issues and consequences of using nutrition to modulate the avian immune response. J. Appl. Poult. Res. 26, 605–612. doi: 10.3382/japr/pfx028
Langille, M. G., Zaneveld, J., Caporaso, J. G., McDonald, D., Knights, D., Reyes, J. A., et al. (2013). Predictive functional profiling of microbial communities using 16S rRNA marker gene sequences. Nat. Biotechnol. 31, 814–821. doi: 10.1038/nbt.2676
Macdonald, S. E., Nolan, M. J., Harman, K., Boulton, K., Hume, D. A., Tomley, F. M., et al. (2017). Effects of Eimeria tenella infection on chickens caecal microbiome diversity, exploring variation associated with severity of pathology. PLoS ONE 12:e0184890. doi: 10.1371/journal.pone.0184890
Pan, D., and Yu, Z. (2014). Intestinal microbiota of poultry and its interaction with host and diet. Gut Microbes 5, 108–119. doi: 10.4161/gmic.26945
Park, S. K., Kim, M. S., Roh, S. W., and Bae, J. W. (2012). Blautia stercoris sp. nov., isolated from human faeces. Int. J. Syst. Evol. Microbiol. 62 (Pt 4), 776–779. doi: 10.1099/ijs.0.031625-0
Parks, D. H., Tyson, G. W., Hugenholtz, P., and Beiko, R. G. (2014). STAMP: statistical analysis of taxonomic and functional profiles. Bioinformatics 30, 3123–3124. doi: 10.1093/bioinformatics/btu494
Prescott, J. F., Parreira, V. R., Mehdizadeh Gohari, I., Lepp, D., and Gong, J. (2016). The pathogenesis of necrotic enteritis in chickens: what we know and what we need to know: a review. Avian Pathol. 45, 288–294. doi: 10.1080/03079457.2016.1139688
Pruesse, E., Quast, C., Knittel, K., Fuchs, B. M., Ludwig, W., Peplies, J., et al. (2007). SILVA: a comprehensive online resource for quality checked and aligned ribosomal RNA sequence data compatible with ARB. Nucleic Acids Res. 35, 7188–7196. doi: 10.1093/nar/gkm864
Sokol, H., Lay, C., Seksik, P., and Tannock, G. W. (2008). Analysis of bacterial bowel communities of IBD patients: what has it revealed? Inflamm. Bowel Dis. 14, 858–867. doi: 10.1002/ibd.20392
Stanley, D., Hughes, R. J., and Moore, R. J. (2014). Microbiota of the chicken gastrointestinal tract: influence on health, productivity and disease. Appl. Microbiol. Biotechnol. 98, 4301–4310. doi: 10.1007/s00253-014-5646-2
Stanley, D., Keyburn, A. L., Denman, S. E., and Moore, R. J. (2012). Changes in the caecal microflora of chickens following Clostridium perfringens challenge to induce necrotic enteritis. Vet. Microbiol. 159, 155–162. doi: 10.1016/j.vetmic.2012.03.032
Wu, S. B., Stanley, D., Rodgers, N., Swick, R. A., and Moore, R. J. (2014). Two necrotic enteritis predisposing factors, dietary fishmeal and Eimeria infection, induce large changes in the caecal microbiota of broiler chickens. Vet. Microbiol. 169, 188–197. doi: 10.1016/j.vetmic.2014.01.007
Yan, W., Sun, C., Yuan, J., and Yang, N. (2017). Gut metagenomic analysis reveals prominet roles of Lactobacillus and cecal microbiota in chicken feed efficiency. Sci. Rep. 7:45308. doi: 10.1038/srep45308
Keywords: butyrate, broilers, essential oils, microbiota, necrotic enteritis
Citation: Bortoluzzi C, Rothrock MJ, Vieira BS, Mallo JJ, Puyalto M, Hofacre C and Applegate TJ (2018) Supplementation of Protected Sodium Butyrate Alone or in Combination With Essential Oils Modulated the Cecal Microbiota of Broiler Chickens Challenged With Coccidia and Clostridium perfringens. Front. Sustain. Food Syst. 2:72. doi: 10.3389/fsufs.2018.00072
Received: 14 August 2018; Accepted: 17 October 2018;
Published: 06 November 2018.
Edited by:
Joshua B. Gurtler, Agricultural Research Service (USDA), United StatesReviewed by:
Cangliang Shen, West Virginia University, United StatesGovindaraj Dev Kumar, University of Maryland, United States
Copyright © 2018 Bortoluzzi, Rothrock, Vieira, Mallo, Puyalto, Hofacre and Applegate. This is an open-access article distributed under the terms of the Creative Commons Attribution License (CC BY). The use, distribution or reproduction in other forums is permitted, provided the original author(s) and the copyright owner(s) are credited and that the original publication in this journal is cited, in accordance with accepted academic practice. No use, distribution or reproduction is permitted which does not comply with these terms.
*Correspondence: Todd Jay Applegate, applegt@uga.edu