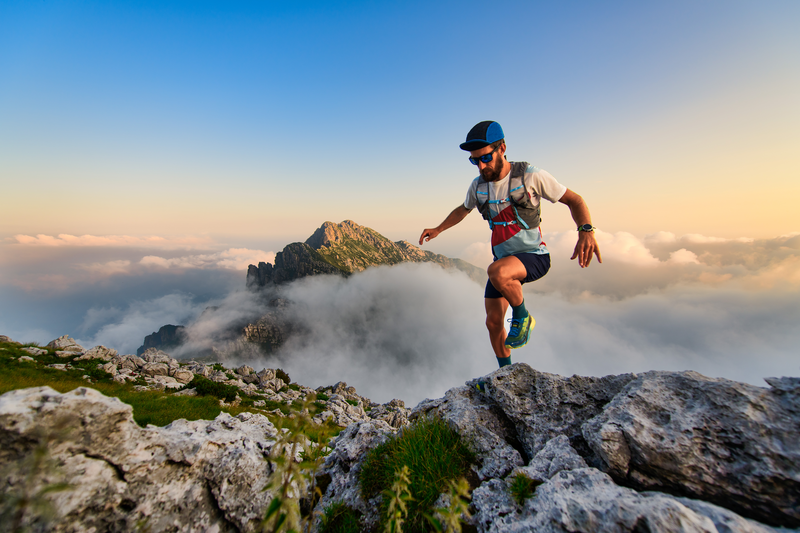
94% of researchers rate our articles as excellent or good
Learn more about the work of our research integrity team to safeguard the quality of each article we publish.
Find out more
ORIGINAL RESEARCH article
Front. Sustain. Food Syst. , 29 October 2018
Sec. Water-Smart Food Production
Volume 2 - 2018 | https://doi.org/10.3389/fsufs.2018.00071
Urban agriculture is undergoing a contemporary global renaissance, providing fresh food for growing urban populations and vital environmental benefits for cities. Despite urban agriculture's social-environmental importance, a rural bias in agricultural research has left critical gaps in our understanding of how urban agroecosystem management can sustainably produce food in the future. Specifically, there is a need to study urban agriculture water management due to recent drought events, likely increases in urban water scarcity, and higher temperatures. Gardeners can play a decisive role in increasing urban agriculture's sustainability through their water, soil, and vegetation management. Here, we examined water use, vegetation, and soil management in the California Central Coast—a region facing drought—to better understand how urban agriculture management affects water use sustainability. We worked with gardeners to study their water management decisions using citizen science, where volunteer gardeners collected their own water use behavior data and participated in a survey to describe their behaviors around water use, water conservation, and plot level management. We found that water use varies by gardener, and water use is positively related to mulching and crop cover in plots. Contrary to expectations, gardeners that reported high levels of concern over current environmental conditions (drought) and water conservation in the survey tended to use high amounts of water, suggesting that environmental worldviews do not necessarily translate into everyday practices. On the other hand, gardeners in gardens with more rules and regulations around water use tended to use less water, highlighting the practicality of enforcing rules and regulations during drought periods. Gardeners reported interest in adapting gardening practices to more sustainably use water regardless of their current practices. The combination of education and rules and regulations will help improve the sustainability of garden systems in times of environmental change.
More than half of the world's population now lives in cities (United Nations Department of Economic Social Affairs PD., 2015), and urban agriculture is a resource to enhance urban food system sustainability (Wiskerke, 2015). Urban agriculture is broadly described as the production of crop and livestock on small parcels of land within cities (Zezza and Tasciotti, 2010; Ackerman et al., 2014), and encompasses home/private gardens, production-focused farms, community/allotment gardens, roof-top gardens, and community orchards (Mougeot, 2000). Urban agriculture may be an essential social resource for food security and nutrition in food desert neighborhoods (Walker, 2007; Alaimo et al., 2008), but also an ecological resource in cities experiencing loss of natural habitat and related environmental issues including biodiversity loss and storm water run-off (Lovell, 2010; Mandel, 2013; Ackerman et al., 2014; Gittleman et al., 2017). Urban agroecosystems are thus critical social-ecological systems for urban food systems and ecosystem services broadly (Barthel et al., 2015; Wiskerke, 2015). Despite urban agriculture's growing relevance as agroecosystems in cities and as components of urban food systems, a rural focus in agricultural management has left a gap in our understanding of how urban agroecosystem resource management influences sustainable food production in urban systems (Lin et al., 2015). Urban gardeners may lack appropriate agroecological knowledge of practices to promote sustainable resource management of plants, soils, and water (Gregory et al., 2015). This is important because plants in agroecosystems are carefully maintained through soil nutrient management and water supplementation based on plant ecophysiology and environmental conditions (Prasad et al., 2008; Daryanto et al., 2017), but current and future patterns of extreme drought and heat in cities are increasing the need for natural resource inputs while simultaneously reducing access to them (Milly et al., 2008; Hunt et al., 2013). This could limit the sustainability of urban agriculture due to negative effects of drought on crop production (Tardieu et al., 2000) and for natural resource conservation in cities (Eriksen-Hamel and Danso, 2010).
Water access and availability often challenge urban gardeners because both environmental limitations and city policy mechanisms regulate the continuous access to water in gardens and therefore crop productivity (Gregory et al., 2015). In California, a recent 5 year-long drought spurred cities to implement severe watering restrictions for urban community gardens (Community Gardens Program, 2016), limiting the number of days and hours that gardeners could access and use water. Gardeners may not have experience or the resources to adapt their growing practices to changes in temperatures and water availability to reduce their water consumption while still supporting plant productivity (Oberholtzer et al., 2014). Plant sensitivity to the negative effects of drought-induced high temperatures on soil moisture retention (Blum, 1996; Prasad et al., 2008; Monneveux et al., 2011) may prompt gardeners to reevaluate soil and water management. Some gardeners may mulch beds to retain soil moisture and change watering methods in attempts to reduce water use and maintain plant survivorship (Gregory et al., 2015), while few change the types of plants that they grow (Egerer et al., 2019). Some gardens may implement rules and regulations in attempts to reduce water use (Turner, 2011), but it is unclear if rules reduce overall water use. There is a critical need for research in urban agriculture on limited resources that links scientists with practitioners to improve learning and actionable science (Lin and Egerer, 2017; Ossola et al., 2017). Urban gardeners can play a valuable role in urban agriculture research and in the production of knowledge that gardeners can utilize to use water more sustainably—reducing water use and increasing efficiency through behavioral change. Involving gardeners in the design and undertaking of research can be an approach that promotes sustainable water management through gardener learning and behavior adaptation to overall improve food system sustainability. This can bolster garden resilience—the ability of the social-ecological system to respond to environmental and social disturbances (Carpenter and Folke, 2006)—in a changing, increasingly more extreme climate.
Learning and knowledge exchange is key to building resilience and garden sustainability (Westley et al., 2013; Schultz et al., 2015). Adaptation of management practices through experience and learned behavior can affect the short and long term resilience of gardens to resource scarcity and environmental change (Barthel and Isendahl, 2013). Experimentation, behavioral adaptation, and learning in agroecosystem management prepares gardeners for current and future disturbances and therefore their ability to handle change (Krasny and Tidball, 2009; Barthel et al., 2010, 2015). Participation in resource management can empower gardeners to make management changes through their learning and interacting with natural resources and garden social networks (Okvat and Zautra, 2011). Including gardeners in scientific research on agroecosystem resource management has great potential to co-produce knowledge on sustainable management (Gregory, 2018) in systems experiencing environmental change (Childers et al., 2015). Research approaches like citizen science that are shaped by civic ecology frameworks can better incorporate ecology, stakeholder experience, and policy for “action-based” research to build community resilience (Shirk et al., 2012; Krasny et al., 2014). Citizen science entails public participation in organized research project data collection (Louv et al., 2012); the knowledge generated from the research can be collaboratively produced by citizen scientists and researchers, informed by diverse experiences and perspectives, and directly applied by practitioners involved in knowledge production (Shirk et al., 2012; Grove et al., 2016). Thus using citizen science under a civic ecology framework integrates the realms of science, engagement and education (Shirk et al., 2012; Krasny et al., 2014), and sits at the nexus between scientific discovery and social change (Krasny and Tidball, 2015).
In this research, we draw from civic ecology frameworks and citizen science approaches in order to understand drivers of water use in urban gardens to improve the sustainability and resiliency of urban agricultural systems under environmental change. In addition to the social learning benefits described above, citizen science lends itself to urban agriculture research because of the practical challenges of the research (e.g., site access, difficulty of measuring inputs and outputs) (Pollard et al., 2017). We worked in a drought-stricken region—the California Central Coast—and asked three questions: (1) What are the reported biophysical variables and social variables that influence gardener water use behavior in urban gardens? (2) Do plot-level biophysical variables and social variables predict self-reported water use by gardeners? (3) What do gardeners learn and how do they report changing their water, soil, and vegetation management to be more sustainable through research participation? To answer these questions we used a contributory citizen science model with collaboratory model features (sensu Shirk et al., 2012): scientists designed the research questions and asked for volunteer gardeners to contribute data, but scientists and gardeners collaboratively collected and interpreted data on management. Both groups reviewed the results, provided iterative feedback, and together brainstormed solutions to water access and availability challenges facing gardens. With this research, we aimed to uncover relative unknowns in urban agricultural research: water usage and drivers of water use behavior.
Our study had three hypotheses and we had a priori expectations informed by past research. (H1) Water use varies by biophysical factors that influence gardeners; here we expected that gardeners will water more in hotter temperatures and when having more crops. (H2) Water use varies by social factors; here we expected that gardeners that convey more concern about environmental changes occurring in the region (drought, water scarcity) will be more conservative in their water use as will gardeners in gardens with more watering rules. (H3) Gardeners will learn about water use through participation; here we expected that gardeners would report knowledge of water use and types of changes to their practices.
We worked in four urban community gardens in the Central California Coast, one in Monterey Co. (36.2400° N, 121.3100° W), two in Santa Clara Co. (37.3600° N, 121.9700° W), and one in Santa Cruz Co. (37.0300° N, 122.0100° W). The cities range in size, with estimated population densities of 50, 274, and 232 people/sq. km, respectively (2010–2014 U.S. census period) (US Census Bureau, 2014). The current populations of major cities included in the survey range from 1.03 million (San Jose) to 22,000 (Marina). The study took place from August to September 2017 (Aug 1–Sept 15, 2017), a time of year typically characterized by little to no rainfall, periodic heat waves, and drought conditions (Rippey, 2017). In some counties there were water restrictions, and garden bylaws for some gardens had influenced or required the garden management to impose watering restrictions, limiting the number of days in the week and time of day that gardeners were allowed to water (Community Gardens Program, 2016). It is within these gardens that water is particularly a resource concern for the management. In each of the four gardens, we monitored five individually managed plots, for a total of 20 plots across all gardens. Plots had similar irrigation infrastructure; participants had either a personal or shared spigot with hose attachment at or near to their plot. In each plot, we collected data on vegetation characteristics, ground cover characteristics, soil properties, ambient temperature, rainfall, and on water used by gardeners. For each gardener, we collected data on their perceptions of water use and learning. We collected a portion of the data (vegetation, ground cover, temperature, etc.) and then worked with volunteer citizen scientists in each garden to collect other data (water use, rainfall). We describe both of these data collection processes in detail below.
We used a citizen science approach with volunteer gardeners (henceforth “participants”) as it is an appropriate and effective research tool to collect urban gardener data (Pollard et al., 2017). The gardens in which we worked were interested in understanding their water usage and wanted to make efforts to reduce water. For this reason, participants wanted to work collaboratively with us to inform the design of the data collection by providing feedback on data collection feasibility and by providing feedback and self-assessment throughout the study period. The collaborative aspects of the research aimed to better provide participants the opportunity to produce knowledge that influences their agroecological practices and resource management (Sharp et al., 2011).
For our study, we solicited five participants per garden to participate in the study using paper fliers hung in the garden and through communication with management. Participants had to be active gardeners (i.e., growing plants, regularly visiting the garden) and be willing to collect data for the project's entire duration. Participants either contacted the researchers if they were interested in participating or the researchers asked the gardeners if they were interested in participating during site visits. We included three interested gardeners that had participated in our pilot study the previous year (Lin et al., 2018) in the present study. Our sample size was limited by logistical feasibility and efforts to maintain high quality communication with all participants across the counties, and introduces a limitation to our study.
Each participant was given a Gardena water meter to measure their water use (Gardena Brand Electronic Garden Hose Water Meters), and we demonstrated as well as provided instructions on how to use these meters. The meters were installed directly to each of their faucets at the plot level so that they only recorded their own water use. We provided participants a clipboard and a data sheet to record the amount of water they used each time they watered, at what time of day, precipitation at their plots and the weather conditions at the time of the watering. We encouraged other notes on their watering experience. At the end of the 6 weeks, the participant gave data sheets to the research team. One simple rainfall gauge was installed onsite to confirm that there was no rainfall during the experimental period. Participants checked on this when they were taking their measurements.
We reviewed data quality for the participant-collected data before data analysis. We eliminated from the analysis two individuals who ceased watering because of crop death from extreme heat events (we qualitatively discuss their survey responses below). We calculated three water use variables for each participant for the analysis: (1) water use per watering event (i.e., each time a participant watered) per meter square of gardening surface area (L/m2); (2) mean water use across all watering events per meter square area (L/m2); and (3) coefficient of variation in water use across all events (CV). Thus in this study, water use is the amount of water withdrawn by gardeners (i.e., not crop consumptive use). Water use variables were standardized for area because garden plots were of slightly differing sizes, and rescaled because water use was quite variable across participants and therefore rescaling was needed for optimal model convergence.
We measured garden plot characteristics on vegetation cover, ground cover, soil properties, and temperature at each of the 20 plots to assess the types of management used at the plot level. We did this to determine whether there were certain biophysical characteristics of the plots that would be predictive of water usage aside from what participants self-reported (described below). For plot vegetation, we measured the number of crops in the plots and the percent cover of crops, weeds, and herbaceous plants in the plots. For ground cover, we similarly measured the percent cover of mulch, straw, rocks, grass, and bare soil in the plots. For both vegetation and ground cover, we used a visual assessment and an estimate of the percentage of total plot surface of each type of vegetation and ground cover covering the plot. In addition, we reviewed the crops growing in the participants plots and classified the crop species by their water use needs using the US Farmers Almanac watering guidelines (https://www.almanac.com/), and the University of California Agricultural Extension Service (http://cagardenweb.ucanr.edu/). We then created general classifications for the entire crop community as needing low, medium or high water users based on these “ideal” guidelines (similar methods to Pataki et al., 2013) in relation to the percent crop cover measured in participants' plots. We use these classifications to determine whether crop water needs predicted water use.
We collected baseline data on soils in the plots, including two bulk density cores for each plot as well as five soil cores up to 15–20 cm in depth to analyze for soil organic matter, water holding capacity, bulk density, and texture. This was done in part to provide participants with information about their soils. We followed Wilke's (2005) standardized methods for soil property measurements that are used for assessing amended soils in urban gardens (e.g., Grewal et al., 2011). To determine soil organic matter (SOM), we used the Loss on Ignition (LOI) method (500°C, 4 h) with dried soils to calculate the percent SOM. To determine bulk density, we weighed fresh soil, dried samples at 105°C, for 24 h, and then calculated bulk density with the final dried weight. To determine soil water holding capacity (WHC), we followed the standardized method that determines the maximum amount of water retained by the soil against gravity by saturating soil samples, draining soils of free water, and evaluating only the water held by the soil (Wilke, 2005). We filled 2 × 2″ cylinders with a perforated base with sieved, fresh soil, and placed them in a water bath overnight. We then capped and placed cylinders on a tray of sand for ~6 h, allowing soils to drain, and then removed and dried soils (105°C, 24 h) to calculate WHC. For soil texture, the proportion of sand, silt and clay were determined through a particle size analysis by A&L Western Agricultural Laboratories (Modesto, CA, USA).
For the analysis (section Self-Reported Watering Data and Stated Influences on Watering Behavior), we selected only one soil variable due to significant correlation among soil properties. We chose the soil organic matter content (%) because it not only had the best fit for the model and was correlated to other soil properties (e.g., WHC), but many participants reported that they use mulch and compost in their plots which is likely reflected in the soil organic matter content in this system (Egerer et al., 2017).
We monitored the ambient temperature of each participant's plot with a temperature logger (Onset HOBO UA-001-08) placed 1.3 m above the plot to record temperatures (°C) directly at the plot. The loggers took temperature readings every hour over the study period. We checked the temperature loggers throughout the study period to ensure that they were in good working order. We downloaded and collected the data at the end of the study period, and quality checked and cleaned the data. For each plot, the temperature at the time of the reported watering event was taken for each event to examine if ambient plot temperatures predicted water use.
We took a four step approach to understand participant's perceptions of their water use behavior. The first step was to understand whether participants understood their water use before the project, and we distributed paper survey questionnaires to participants at the beginning and end of the study period. In the pre-study survey we asked them how much water they think that they use each time they water, including an option of “I don't know.” This question was important because it provided baseline information on participant knowledge. The pre-study survey also asked participants what crops they are growing, their watering frequency, what variables influence their water use (e.g., rules, weather, plants), what they add to their soils (e.g., compost), and their perceptions of current drought and climatic conditions. We also asked the three participants that participated in our pilot study the previous year (Lin et al., 2018) whether participation had changed their watering since. The second step was to monitor the amounts of water that participants use based on the self-reported water data for each participant. The third step was to ask participants again in a post-study survey how much water they think that they use after their research participation and data collection. The post-study survey asked participants how much water they think that they use each time (given their data collection), if and how useful they found participating in the project (1–5 scale and open response), what learning outcomes they gained from the project, what they discussed/shared with others through their participation, and the influence of participation on their water management decisions and behaviors. In addition, the post-study survey asked participants to describe if and how they changed their water management in the research process. We used this survey data to compare how much participants know about and whether they gained a better understanding of their water use.
The fourth step was to send participants their results in the form of a tabulated water use summary and personalized soil profile six weeks after the study's end. In this report we provided summary statistics across all participants so that they could gauge how their use fits within the range of data collected. To collect qualitative data and to provide an opportunity for participants to suggest how to improve the study, we included a survey form with the summary results soliciting further thoughts on their experience and on their results after six weeks. We also asked participants what could be improved from the research/data collection design. We used this questionnaire to collect any additional information not captured by the pre- and post-study surveys, to gain more insight into post-study impact, and for participants to inform future research design. We calculated summary statistics for the pre- and post-survey responses, and reviewed all qualitative responses to inform our analysis and interpretation.
We used the collected survey responses to help inform which explanatory variables would go into water use models. Information from the survey suggested that: (1) self-reported watering behavior of participants may be influenced by biophysical variables such as the local weather and by the plants in plots; (2) self-reported watering behavior may be influenced by a social variable: garden rules and regulations on watering; (3) participants are heavily amending the soils and ground cover in their plots; and (4) participants are divided on views on environmental conditions with those voicing high concern to those voicing little to no concern for drought and water scarcity in the region. We therefore used four quantitative biophysical non-correlated explanatory variables informed by the survey to model water use behavior across four categories: one climate/weather variable (plot-level ambient temperature (°C) at the time of the reported watering event), one crop management variable (percent crop plant cover per 1 m2), one soil management variable (percent organic matter), and one ground cover management variable (percent mulch and straw cover). We also selected the presence of garden rules and the level of environmental concern reported as social variables for the model. For the rules variable, we classified participants as: (1) having rules in their garden, where the garden limits the number of days and times gardeners can water, or (2) having no restrictions on water use. For the environmental concern variable, we coded participant pre-study survey responses by either conveying high concern for regional drought and water scarcity, or having little or no concern.
To determine whether variables strongly predict water use, we modeled the three water use response variables in our analysis at the scale of the participant [mean water use (L/m2), water use CV, and water use per event (L/m2)]. For the mean water use and water use CV analysis, we built generalized linear regression models (GLMs) assuming log-normal error structure with the four biophysical variables and two social variables (section Self-Reported Watering Data and Stated Influences on Watering Behavior) and their potential interaction because rules and environmental concern may interact to influence watering outcomes. We used GLMs because it is a flexible multivariate approach (Zuur et al., 2009) that could test what biophysical variables and social variables best predict self-reported water use. We used the glmulti package and function (Calcagno and De Mazancourt, 2010) in the R statistical environment (R Development Core Team, 2016) to determine best fit models. Models with the lowest Akaike Information Criterion (AICc) values were selected as the best fit models and model assumptions were checked with Shapiro–Wilks tests (Bolker et al., 2009).
For the water use per event analysis, we used generalized linear mixed models (GLMM) with a link log function and Gaussian error distribution and repeated measures to model the liters of water used per event (L/m2). Participant nested within garden site were treated as random effects, and the biophysical and social explanatory variables (section Self-Reported Watering Data and Stated Influences on Watering Behavior) were treated as fixed effects. This approach allowed us to: fully examine the distribution of the data; test the importance of each and combinations of variables for predicting amount of water used at an event; and include participant and site as random effects (Zuur et al., 2009). We rescaled the response variable due to the wide range in water use amounts per event (e.g., 1–1,000 L). We used model comparison with AICc to evaluate the best model fit, considering the best model with the lowest AICc score (Burnham and Anderson, 2002). GLMM analyses were performed using the lme4 package (Bates et al., 2012) in R.
To examine whether crop water needs predicted water use, we used GLM to compare mean water use among participants for crop water need groups. We fit the model and ran a post-hoc test using the glht function in the multcomp package (Hothorn et al., 2008) to assess difference in water use. Mean water use per event (L/m2) was the response variable, and low, medium and high crop water needs were the predictor variables.
To evaluate whether gardener understanding of their water use matched gardener watering management practices, we reviewed the pre- and post-study survey responses in relation to the water use data that participants collected. We compared pre- and post-study survey responses to the survey question asked in both surveys, “How much water do you think that you use each time?,” to evaluate differences in water use behavior understanding and thus potential learning outcomes. In both surveys, participants could provide an estimated numerical value (in gallons or liters) or could select “I don't know” as a response. Based on the reported responses we categorized participants as those that provided a numerical response, and those that replied, “I don't know” or did not reply. Of those that reported a response, we categorized participants that (1) approximated their water use and it was close to their actual measured mean water use (±20 L, 10% of the average water used over the study period) as having a better understanding, (2) approximated and overestimated their use (>20 L), (3) approximated and underestimated their water use (<20 L).
We qualitatively analyzed the participant responses to the open-ended question of “What have you learned from participating in this study?” on the post-study survey to examine if and how participants had a better understanding of their watering practices. We reviewed participant responses to open ended questions on what they learned, how they found the study useful, and their experiences and thoughts on project participation. We summarized scaled (1–5) values for the question “How useful did you find participating in this project?” Moreover, we reviewed participant responses on how to improve their water conservation, and put it in the context of our own experience as researchers working with participants through the course of the study.
All participants gave their informed verbal and written consent for inclusion before they participated in the study. The study was conducted in accordance with the University of California-Santa Cruz (UCSC) Office of Research Compliance Administration. The research was exempted from Institutional Review Board under #HS2569. The UCSC operates under a Federalwide Assurance approved by the DHHS Office for Human Research Protections, FWA00002797.
Self-reported water use behavior varied by participant with participants using on average 202 L or 31 L/m2 for each watering event (i.e., time that they watered) over the course of the 6 weeks (Table 1). A majority of participants watered their plots 1–2 times per week (53%). The other participants that watered their plots more frequently (daily or 3–4 times per week) tended to use on average less water per event.
Table 1. Summary statistics for the plot-level variables measured in the garden plots by gardener citizen scientists (A) and by researchers (B–D) over the duration of the study.
In the pre-surveys, garden-set rules, regulations, and limits were the most frequently cited influences on participant watering behavior (65%). This was followed by the weather (40%), plant needs (35%), other gardeners (15%), and the news/media (e.g., reports on drought and climate) (10%). Survey responses also mentioned that participants manage their soils and ground cover by adding compost, straw and mulches to their plots, and how this may influence their watering because they assess the soil (e.g., “I eyeball the soil [dryness] to know how much water to use”). Participants documented little to no rainfall, just dew and fog at their plots, and this was confirmed by regional climatic data during this period that recorded just 2 mm of precipitation in Santa Cruz, California and 1 mm of precipitation recorded in San Jose, California for the entire study period (US Climate Data, 2017).
The best model predicting mean water use over the study period included mulch/straw ground cover, soil organic matter, garden rules, and environmental concern variables (Table 2). Mean water use was significantly higher with greater mulch/straw cover in plots (Table 2; Figure 1). The best model predicting water use CV included garden rules, environmental concern and their interaction, with water use CV significantly increasing among participants in gardens with rules and with low environmental concern (Table 2; Figure 2).
Table 2. Generalized linear models (GLMs) that best predict amount of mean water used (A) and variation (CV) in water use (B) over the 6-week study period [ΔAICc to null model: (A) 16.5; (B) 23.0].
Figure 1. Relationship between garden biophysical variables (measured in the garden plots) and the three water use variables examined over the 6-week study period: mean water use (a–c), water use CV (d–f), and water used per watering event (g–i). Gray shading and fitted line show model fits in Tables 2, 3 (i.e., GLM or GLMM).
Figure 2. Relationships between social variables (garden rules; participant's environmental concern) and water use variables examined over the 6-week study period: mean water use (a,b), water use CV (c,d), and water used per watering event (e,f).
The best models predicting water use per event included the percent mulch/straw cover, temperature at watering, and environmental concern (Table 3), followed by models also including garden rules and soil organic matter. Participants that expressed high concern for environmental changes in the region like drought, heat, and water scarcity tended to use more water per event than those that expressed little to no concern, and participants with garden plots with more mulch ground cover and more crop cover tended to use more water (Figures 1, 2). Participants tended to use more water when watering at higher ambient temperatures (Figure 1). At this scale, the interaction between environmental concern and garden rules was relatively insignificant, and did not improve model fit (Table 3).
Table 3. Generalized linear mixed models (GLMM) predicting water used by participants per watering event over the 6-week study period, with participant nested within garden as a random effect.
Gardeners had crops across the range of water use needs including crops that have low water use needs (deep-rooted crops; e.g., asparagus, tomato, melon) as well as crops with high water use needs (shallow-rooted crops; e.g., lettuce, corn, cabbage) (Supplementary Table 1). Water use did not vary depending on recommended watering needs of plants in the post-hoc comparison.
Gardener expectations of their water use often differed from their measured water use. In the pre-study survey, 70% of participants reported that they did not know how much water they use each time. In the post-study survey after project participation, 65% of participants estimated an amount of water that they use each time. Yet 35% did not know or did not respond indicating that they did not learn about their water use through participation. Of the 65% that estimated, more than half (69%) of the participants had a better understanding of their water use, estimating close (±20 L) to their mean water use while 8% of participants overestimated and 23% underestimated mean water use. These quantitative values were further supported by qualitative survey responses in the post-survey. One participant clearly articulated the general difficulty of assessing their water usage even after 6 weeks of monitoring: “I [still] do not have a concept of what the amount (the actual number) of water I am using looks like.”
Nevertheless, a majority of gardeners found participation in the study to be useful, rating a 4 (47%) or 5 (26%) out of 5 for the post-study survey question “How useful did you find participating in this project?” In the analysis of the post-survey response to the question “What have you learned from participating in this study?” participants reported that they learned several valuable lessons through their participation. We identified two main learning processes from the participant responses: (1) monitoring water use is a learning process through which participants better understand how to identify how much water is used (more technical/ mechanical); and (2) monitoring water use is a learning process through which participants learn about themselves and the people around them (observational).
Gardeners that learned about the technical facts of water use included those that simply stated “I learned it takes a lot of water to grow food” or “I learned about water usage.” Another participant reported: “I learned that the pressure of the water reflects how much water is used and how long I have to water.” Other participants in this group associated their learning about water use specifically with the study methods, design and equipment. Stated by one participant: “I learned I need to watch the length of time it takes to water, the water flow rate, and how frequently I am watering. Keeping a record helped a lot with this.” Another participant described how “the materials used, the equipment to track your water—how much going out—is really interesting. I use 10 times more than I thought I was.” This participant also reflected learning about themselves, and about other gardener's practices through observation, and reported that “[the study] told me that I can look at others' plots and see what they are doing (and what they are not).” Here, the participant related learning about sustainable water use in community gardens to direct observations of others, and they then linked this back to self-reflections on their behavior. Another participant that indicated learning about themselves stated: “I change my watering a lot daily and weekly depending on weather and seasonal plantings.”
Some participants reported a “ripple effect” both within the garden and an effect beyond the garden. Stated by one participant: “I like participating in a group effort. Everyone participating has learned something from it. There's a ripple effect.” Another participant highlighted that: “This project helps me serve as an example to other community gardeners. The collected water measurements strengthen my case for water conservation [in the garden].” Expanding impacts beyond the garden, one participant said that participation “has and will change my habits at home, too.” Participants brought forth deeper realizations on their experience and on broader implications with passing time in the weeks after the study. For example, one participant voiced that “the garden can use less water than what it is using. We could further limit the number of days [that we are allowed to water].”
Participants reported that they are changing their management to improve the characteristics of their soil and reduce their water use. One participant actually implemented a drip irrigation system toward the end of the study in order to better control her use, illustrating a change in water use behavior. Several other participants also voiced a plan to implement drip irrigation in their plots. Participants reported plans to improve their soils after receiving their summary results. Stated by one participant: “I am making a plan to improve my soil profile and texture by using more compost and also reduce watering…I would like to amend my soil and hopefully be able to have it retested to see if my soil profile and soil texture improve.” However, this was not universally voiced by participants, and a few participants—particularly those in gardens with rules and regulations—stated that they did not change their watering practices since the study's completion. Other participants did relay that the soil results provided new information for the whole garden: “I was surprised to see the sand, silt, and clay proportions in the soil. At the garden, we generally talk of our soil as clay but we actually have more sand than clay and are unaware of silt content.”
Last, participants expressed their opinions on how to improve the study's design, data presentation, and tangible management outcomes of the study. Participants conveyed that they still require more information to understand the results, specifically in the management of “healthy” soils. Participants wished for specific recommendations regarding soil properties that they believe are necessary for healthy soil improvement. For example, one participant stated: “I would like to know more about what is included in [soil] “organic matter” and how this relates to fertilizers and compost. I am also aware that there needs to be a healthy microbe population in the soil but not much more than that. Mostly I'm interested in how I can tell the soil is healthy—what to look for in texture, smell, water retention, ease of planting, etc.” Other participants reported interests in learning about best methods of watering without erosion and more information about the specific implications of certain soil properties (texture, nutrients) for water management.
We worked with urban gardeners in a region (California) experiencing environmental change to better understand current urban agriculture water use, and to generate knowledge needed for urban agriculture's future sustainability. In our research, we found that citizen science gardeners use water conservatively and generously over the season depending on the agroecological characteristics in their garden plots and the rules in place at their gardens, but not necessarily with their conveyed environmental concerns. Gardener narratives revealed that water and garden soils are generally poorly understood, and that gardeners misperceive the amount of water that they think that they use. Although most participants water based on what they think their plants need, the lack of relationships between plant watering needs and water used may point to gaps in information on water use, and that gardeners may be using more or less water than is necessary for growing the plants. Water seems to be an intangible component of urban agroecosystems that is challenging to quantify and to conserve by individuals. Garden implemented rules could reduce water use, and gardeners can improve their understanding of their water use by participating in data collection. Many of the participants could estimate their water usage post-study, and most reported research participation useful and conveyed specific learning outcomes. Many of these participants are also eager to adapt their management—particularly of their soils—to be more sustainable.
We explore two key patterns that we see in our analysis of participant water use in these gardens: (1) gardeners generally misperceive their water use and can use high amounts of water in their plot systems regardless of plant needs. But these generous water users also use water-conserving practices (mulching with woodchips and straw) and report strong concerns about environmental changes occurring around them (extreme heat and drought); and (2) gardeners with garden-implemented rules on water usage tended to use less water, suggesting that governance systems could regulate water use if people will not reduce water use willingly. Reports from the participants suggest that education and learning through, for example, voluntary water monitoring can also be an effective tool toward water conservation in absence of rules, although it may take more time and effort.
Participants in the study used over double or triple the recommended amount of water for gardening in the region (~25 L/m2/week) (UCCE Master Gardener Program, 2014). Plant needs influenced 35% of participant watering, even though many of the participants that cultivated plants with low water needs used greater amounts of water. Although we did not ask about plant selection or measure indicators of plant performance, a lack of a pattern among crop types and water use suggest that gardeners could be using more water than necessary for crop survival and production. Counter to expectations, low water use was not evident in environmentally concerned participants. Participants that expressed concerns about the climate and environmental changes like drought and extreme heat did not overall use less water, rather their use was variable and many used high amounts of water per event.
A contradiction in worldview and watering behavior supports theories in environmental psychology. Theories of planned behavior (Ajzen, 1991) and Values-Belief-Norm theory (Stern and Dietz, 1994; Harland et al., 1999; Armitage and Conner, 2001; Steg and Vlek, 2009) support that worldview beliefs do not necessarily predict consistent behaviors because other normative beliefs and attitudes also affect behaviors (Ives and Kendal, 2014). Behaviors are multiple and changeable, thus different value “orientations” (e.g., nature centered vs. self-centered) that shape people's beliefs may produce similar environmental behaviors and vice versa (i.e., similar value orientations may produce quite different behaviors) (Ives and Kendal, 2014). Studies on household water consumption show that attitudes expressed toward water conservation are not representative of water consumption (Aitken et al., 1994). In domestic gardens, residents' practices, attitudes, and beliefs often contradict because gardens are leisure spaces, rather than spaces where environmentally sensitive practices are enforced (Askew and McGuirk, 2004). Everyday practices like water use reveal inconsistencies between beliefs and practice due to conflicts among worldview beliefs and urban place-making processes that are associated with habitual behaviors (Allon and Sofoulis, 2006). Water use may be better explained by habits related to differences in the perceived necessity of caring for plants than by water-saving beliefs conveyed by people.
Participants using high amounts of water in our study are actually using more sustainable practices including adding straw cover and compost that would likely warrant less water use. Research in urban agriculture soils encourages composting, cover cropping, and straw mulching to improve soil fertility and water holding capacity (Beniston and Lal, 2012; Gregory et al., 2015) because ground cover and soil management methods can reduce the rate of soil moisture loss (de Pascale et al., 2011). Increasing the application of water-saving ground cover and soil amendments should suggest reduced water use. Yet we found that participants are adding a lot of inputs to their plots across a number of management factors and may be misunderstanding the synergies among inputs. These participants seem to use more inputs—using more water, more straw, and more compost—despite their crop types. Such results also support the above assumption that many gardeners manage for plant care without fully understanding the interactions among their sustainable management decisions.
Considering whether to focus on the practice or the process through which management decisions are made, the results suggest that while gardeners may be eager to focus changes on particular practices, gardeners may need to focus on the process through which they make water use decisions to make impactful changes. Conveyed environmentally concerned worldviews and intentions to use less water may not translate to behaviors and are therefore not a good proxy for water conservation outcomes.
The participants that had rules at their gardens as to what days and hours they could water used relatively less water. This suggests that rules and regulations on water usage can reduce gardener water use by reducing the frequency of intensive watering or by instilling a notion of shared norms around water where one are expected to use less by the greater social community (Seligman and Finegan, 1990). Community expectations and governance systems in place to conserve water may reduce water use through “good citizenship” notions (Holmes, 1999). The effect of rules may be explained by indirect effects through relationships among watering practices, plant needs, and temperature. Gathered from their water use logs, the participants in the gardens with rules were watering at cooler times of the day—in the morning and evening as required by the management—and are therefore potentially subconsciously using less water because of the cooler ambient temperatures. By influencing when gardeners water, rules may have a more indirect effect on water use through the effect of cooler temperatures on water use behavior. In addition, these participants happened to be growing fewer water-intensive plants in their plots (i.e., tomatoes rather than lettuce), although this was not required by the management and probably due to chance. Thus they may perceive lower water use requirements and water less. These participants also had less crop cover in their plots, and we observed several of them targeting water usage at the specific plants rather than the overhead spraying that we observed by participants with highly vegetated plots.
We caution against universalizing watering rules and regulations for all gardens, however, because rules may have short-term impacts but not produce long-term effects on water conservation, and because water governance is complex itself. First, the participants in gardens with rules did not report adjusting their practices after project participation. Second, there are nevertheless problems with high water use at these gardens: the garden managers in gardens with specific garden-level rules disclosed information that the rules do not universally reduce water use by all gardeners, and that there are instances when other gardeners (those not participating in the study) use high amounts of water on watering days to compensate for reduced water access. This occurs especially for plots with high water need crops such as corn. Water governance can elicit grievances among garden members and management (Turner, 2011; Egerer and Fairbairn, 2018) and should be carefully considered and implemented. Managers and garden organizations should design water use rules and regulations based on the watering needs of the plants in the garden, but importantly also on the social context of their gardens and knowledge of water management held by their gardener communities because there are differences in the cultures and conceptualizations of water and plant needs (Allon and Sofoulis, 2006; Jackson, 2006; Head and Muir, 2007; Turner, 2011).
How can urban gardeners adapt their agroecological practices to improve the sustainability of resource use in changing climates and during times of resource stress (e.g., water shortage, drought)? In this study in four community gardens, we show gardeners variably use water across environmental concerns and crop regimes, and that these gardeners differ in their perception of their water use. The relatively small sample size limits the predictive power of our statistical models, and the study design limits exploring other interaction effects because we did not select gardeners based on their backgrounds or beliefs, rather on their interest to participate. Yet we show that water usage is individualized based on the gardener's perceptions of climate, needs of plants, and the water governance structures of their gardens. Our findings further suggest that gardeners may not fully understand their water use and the water requirements of the plants that they are growing. Water is difficult to conceive for gardeners as indicated by the disparity in actual water used, the perception of use and the recommended needs of the plants. This is similar to findings in domestic gardens and landscapes that argue that water is a misunderstood natural resource (Kolokytha et al., 2002) that supports the pleasures of everyday domestic life but is in tension with environmental worldviews on conservation (Head and Muir, 2007).
Monitoring water usage through participation in research can build the capacity of urban gardeners to learn about their water use through multiple learning processes and adapt their management practices with changing environments. We suggest that research engagement and monitoring may reveal to participants the inconsistencies in their water use behavior in relation to their worldviews, and may provoke better understandings of water use that may generate long-lasting changes that improve garden resource use sustainability. Management can incorporate opportunities for learning about water use as well as water use conservation techniques and strategies for gardeners into their governance plans. Education could be an effective way to have gardeners willingly reduce water use over time, with more knowledge sharing among gardeners as a way to build long-term sustainable water use networks in gardens, rather than rules and regulations that may only inhibit water use short term during drought events.
Gardeners are eager to learn and adapt their gardening through their learned experience in tandem with more “formal” assessments and recommendations provided by researchers (Gregory et al., 2015). In our study we found that while some participants' learning processes were more technical or straightforward (e.g., “I learned I use more water than I expected”), others learned about themselves and about how their behaviors relate to others' through observation. Participating in the research project had an overall positive impact on the garden community and catalyzed conversations on sustainable water use and conservation. Participants expressed that they were excited to be a part of a team effort to reduce water use, or that they have suggestions for the entire garden after their participation. As evidenced in participant narratives after the study, participants are using the soil analysis paired with their water usage numbers provided by the researchers to make management changes in their plots (“I am making a plan to improve my soil profile and texture by using more compost and also reduce watering”).
Gardeners also want to know how other gardeners performed and if and why they used water differently to give them a better understanding of their own management. Stated by one participant: “I would have liked to have seen some of the other gardeners results to compare if the purchased soil originally added to the boxes is holding fertility.” And another participant reported: “I was hoping to see more of an analysis of water use by [each] participant…It would be informative to see if our overall water use is average, or, differs from the other gardens studied (and why). Is our water holding capacity lower, average or high compared to other gardens? Is the percentage of organic matter and soil type roughly the same or vastly different? These would be useful to know in managing the garden.” Thus, educational outreach activities that will be of interest to gardeners can focus on soil properties in relation to management suggestions, and this is similar to findings by Gregory et al. (2015) in New York City gardens. In addition, there was no relationship exhibited between plant water needs and water use. This highlights missing knowledge of plant needs, and outreach activities should convey information to gardeners about crop needs and crop/variety selection to reduce water use.
To reflect on managing the study and working with the participants, we experienced and observed both the challenges and the rewards of citizen science research. Maintaining consistent and clear communication with participants on project expectations, materials and methods (i.e., working the water use meter), and interpreting results was time consuming, as was cleaning and proofing the data for potential errors once it was collected. Nevertheless, our conversations with participants both during and after the study illuminated that gardeners are genuinely inquisitive, are experimental in their agroecological practices, and are motivated to learn about how to better their gardening for water conservation aims—even if their behaviors do not indicate such. As researchers, participants taught us about resourceful water conservation methods, and about how to creatively share results with practitioners. Research that engages both researchers and gardeners together can improve knowledge exchange between groups and facilitate actionable science (Ossola et al., 2017). Collaborations based on scientific integration and knowledge co-production can be a platform for research toward global sustainability (Mauser et al., 2013).
In this study, we investigated the biophysical and social variables that affect water use in urban gardens, what gardeners learn from water research participation, and how gardeners adapt their management to be more sustainable. Our results suggest that water use behavior requires a nuanced understanding by managers and researchers because gardens are socioecological systems in which interactions between biophysical variables, governance systems, and human behavior together shape water use. The inconsistency between human belief and behaviors is part of the complexity of working in socioecological systems, and this complexity argues for the necessity to work in citizen science platforms where there is co-learning among researchers and resource managers. Working with gardeners in research expands an understanding of urban agriculture water use that can have on-the-ground positive effects on resource management and urban welfare through gardener learning with critical implications for the sustainability and resiliency of urban food systems.
All authors conceived of and designed the study. ME and BL performed the data collection. ME and BL analyzed the data. ME drafted the manuscript. All authors provided feedback on and contributed significantly to the manuscript.
The authors declare that the research was conducted in the absence of any commercial or financial relationships that could be construed as a potential conflict of interest.
We sincerely thank all of the 20 community gardeners that participated in the research, and the garden organizations for supporting the research: Charles Street Community Garden, Coyote Creek Community Garden, Marina Tree and Garden Club and Goodwill Community Garden, Trescony Community Garden. Thank you to Z Jordan for assistance with data collection and plant identification. Thank you to the Philpott lab group, D. Kendal, K. Lee, A. Marshall, A. Ossola, and A. Singh for helpful discussions on methodology, data analysis and interpretation that improved the study. Thank you to Graham MacDonald and two reviewers for comments that further strengthened the manuscript. This work was supported by the U.S. Department of Agriculture National Institute of Food and Agriculture (grant # 2016-67019-25185 to SP, BL), the National Science Foundation and an international travel allowance through the Graduate Research Opportunities Worldwide (GROW) (grant # 2016-174835 to ME), and the Carbon Fund at the University of California, Santa Cruz, CA. Any opinions, findings, and conclusions or recommendations expressed in this material are those of the authors and do not necessarily reflect the views of funding organizations.
The Supplementary Material for this article can be found online at: http://journal.frontiersin.org/article/10.3389/fsufs.2018.00071/full#supplementary-material
Ackerman, K., Conard, M., Culligan, P., Plunz, R., Sutto, M.-P., and Whittinghill, L. (2014). Sustainable food systems for future cities: the potential of urban agriculture. Econ. Soc. Rev. 45, 189–206. Available online at: https://www.esr.ie/article/view/136
Aitken, C. K., McMahon, T. A., Wearing, A. J., and Finlayson, B. L. (1994). Residential water use: predicting and reducing consumption. J. Appl. Soc. Psychol. 24, 136–158. doi: 10.1111/j.1559-1816.1994.tb00562.x
Ajzen, I. (1991). The theory of planned behavior. Organ. Behav. Hum. Decis. Process. 50, 179–211. doi: 10.1016/0749-5978(91)90020-T
Alaimo, K., Packnett, E., Miles, R. A., and Kruger, D. J. (2008). Fruit and vegetable intake among urban community gardeners. J. Nutr. Educ. Behav. Educ. Behav. 40, 94–101. doi: 10.1016/j.jneb.2006.12.003
Allon, F., and Sofoulis, Z. (2006). Everyday water: cultures in transition. Aust. Geogr. 37, 45–55. doi: 10.1080/00049180500511962
Armitage, C. J., and Conner, M. (2001). Efficacy of the theory of planned behavior: a meta-analytic review. Br. J. Soc. Psychol. 40, 471–499. doi: 10.1348/014466601164939
Askew, L. E., and McGuirk, P. M. (2004). Watering the suburbs: distinction, conformity and the suburban garden. Aust. Geogr. 35, 17–37 doi: 10.1080/0004918024000193702
Barthel, S., Folke, C., and Colding, J. (2010). Social-ecological memory in urban gardens - retaining the capacity for management of ecosystem services. Glob. Environ. Chang. 20, 255–265. doi: 10.1016/j.gloenvcha.2010.01.001
Barthel, S., and Isendahl, C. (2013). Urban gardens, agriculture, and water management: sources of resilience for long-term food security in cities. Ecol. Econ. 86, 224–234. doi: 10.1016/j.ecolecon.2012.06.018
Barthel, S., Parker, J., and Ernstson, H. (2015). Food and green space in cities: a resilience lens on gardens and urban environmental movements. Urban Stud. 52, 1321–1338. doi: 10.1177/0042098012472744
Bates, D., Maechler, M., and Bolker, B. (2012). Package ‘lme4'. Linear mixed-effects models using s4 classes. Available online at: http://cran.R-project.org/package=lme4
Beniston, J., and Lal, R. (2012). “Improving soil quality for urban agriculture in the North Central US,” in Carbon Sequestration in Urban Ecosystems (Dordrecht: Springer), 279–313. doi: 10.1007/978-94-007-2366-5
Blum, A. (1996). Crop responses to drought and the interpretation of adaptation. Plant Growth Regul. 20, 135–148. doi: 10.1007/BF00024010
Bolker, B. M., Brooks, M. E., Clark, C. J., Geange, S. W., Poulsen, J. R., Stevens, M. H., et al. (2009). Generalized linear mixed models: a practical guide for ecology and evolution. Trends Ecol. Evol. 24, 127–135. doi: 10.1016/j.tree.2008.10.008
Burnham, K. P., and Anderson, D. R. (2002). Model Selection and Multimodel Inference: a Practical Information—Theoretic Approach. New York, NY: Springer.
Calcagno, V., and De Mazancourt, C. (2010). glmulti: an R Package for easy automated model selection with generalized linear models. J. Stat. Softw. 34, 1–29. doi: 10.18637/jss.v034.i12
Carpenter, S. R., and Folke, C. (2006). Ecology for transformation. Trends Ecol. Evol. 21, 309–315 doi: 10.1016/j.tree.2006.02.007
Childers, D. L., Cadenasso, M. L., Grove, J. M., Marshall, V., McGrath, B., and Pickett, S. T. A. (2015). An ecology for cities: a transformational nexus of design and ecology to advance climate change resilience and urban sustainability. Sustainability 7, 3774–3791. doi: 10.3390/su7043774
Community Gardens Program (2016). Community Gardens Program 2016 Rules and Regulations. San Jose, CA.
Daryanto, S., Wang, L., and Jacinthe, P. A. (2017). Global synthesis of drought effects on cereal, legume, tuber and root crops production: a review. Agric. Water Manag. 179, 18–33. doi: 10.1016/j.agwat.2016.04.022
de Pascale, S., Costa, L. D., Vallone, S., Barbieri, G., and Maggio, A. (2011). Increasing water use efficiency in vegetable crop production: from plant to irrigation systems efficiency. Horttechnology 21, 301–308.
Egerer, M., and Fairbairn, M. (2018). Gated gardens: effects of urbanization on community formation and commons management in community gardens. Geoforum 96, 61–69 doi: 10.1016/j.geoforum.2018.07.014
Egerer, M. H., Lin, B. B., Threlfall, C. G., and Kendal, D. (2019). Temperature variability influences urban garden plant richness and gardener water use behavior, but not planting decisions. Sci Total Environ. 646, 111–120. doi: 10.1016/j.scitotenv.2018.07.270
Egerer, M. H., Philpott, S. M., Liere, H., Jha, S., Bichier, P., and Lin, B. B. (2017). People or place? Neighborhood opportunity influences community garden soil properties and soil-based ecosystem services. Int. J. Biodivers. Sci. Ecosyst. Serv. Manag. 14, 32–44. doi: 10.1080/21513732.2017.1412355
Eriksen-Hamel, N., and Danso, G. (2010). Agronomic considerations for urban agriculture in southern cities. Int. J. Agric. Sustain. 8, 86–93. doi: 10.3763/ijas.2009.0452
Gittleman, M., Farmer, C. J. Q., Kremer, P., and McPhearson, T. (2017). Estimating stormwater runoff for community gardens in New York City. Urban Ecosyst. 20, 129–139. doi: 10.1007/s11252-016-0575-8
Gregory, M. M. (2018). Enhancing Urban Food Production, Ecosystem Services, and Learning in New York City Gardens Through Cover Cropping And Participatory Action Research. Cornell.
Gregory, M. M., Leslie, T. W., and Drinkwater, L. E. (2015). Agroecological and social characteristics of New York city community gardens: contributions to urban food security, ecosystem services, and environmental education. Urban Ecosyst. 19, 763–794. doi: 10.1007/s11252-015-0505-1
Grewal, S. S., Cheng, Z., Masih, S., Wolboldt, M., Huda, N., Knight, A., et al. (2011). An assessment of soil nematode food webs and nutrient pools in community gardens and vacant lots in two post-industrial American cities. Urban Ecosyst. 14, 181–194. doi: 10.1007/s11252-010-0146-3
Grove, J. M., Childers, D. L., Galvin, M., Hines, S., Muñoz-erickson, T., Svendsen, E. S., et al. (2016). Linking science and decision making to promote an ecology for the city: practices and opportunities. Ecosyst. Heal. Sustain. 2:e01239. doi: 10.1002/ehs2.1239
Harland, P., Staats, H., and Wilke, H. A. M. (1999). Explaining proenvironmental intention and behavior by personal norms and the theory of planned behavior. J. Appl. Soc. Psychol. 29, 2505–2528. doi: 10.1111/j.1559-1816.1999.tb00123.x
Head, L., and Muir, P. (2007). Changing cultures of water in eastern Australian backyard gardens. Soc. Cult. Geogr. 8, 889–905 doi: 10.1080/14649360701712651
Holmes, K. (1999). ‘Gardens', imaginary homelands: the dubious cartographies of australian identity. J. Aust. Stud. 61, 152–162.
Hothorn, T., Bretz, F., and Westfall, P. (2008). Simultaneous inference in general parametric models. Biometr. J. 50, 346–363. doi: 10.1002/bimj.200810425
Hunt, J. C., Timoshkina, Y. V., Bohnenstengel, S. I., and Belcher, S. (2013). Implications of climate change for expanding cities worldwide. Proc. Inst. Civ. Eng. Urban Des. Plan. 166, 241–254 doi: 10.1680/udap.10.00062
Ives, C. D., and Kendal, D. (2014). The role of social values in the management of ecological systems. J. Environ. Manage. 144, 67–72. doi: 10.1016/j.jenvman.2014.05.013
Jackson, S. (2006). Compartmentalising culture: the articulation and consideration of indigenous values in water resource management. Aust. Geogr. 37, 19–31 doi: 10.1080/00049180500511947
Kolokytha, E., Mylopoulos, Y., and Mentes, A. (2002). Evaluating demand management aspects of urban water policy—A field survey in the city of Thessaloniki, Greece. Urban Water 4, 391–400. doi: 10.1016/S1462-0758(02)00024-9
Krasny, M. E., Russ, A., Tidball, K. G., and Elmqvist, T. (2014). Civic ecology practices: participatory approaches to generating and measuring ecosystem services in cities. Ecosyst. Serv. 7, 177–186. doi: 10.1016/j.ecoser.2013.11.002
Krasny, M. E., and Tidball, K. G. (2009). Community gardens as contexts for science, stewardship, and civic action learning. Cities Environ. 2, 1–18. doi: 10.15365/cate.2182009
Krasny, M. E., and Tidball, K. G. (2015). Civic Ecology: Adaptation and Transformation From the Ground Up. Cambridge, MA: MIT Press.
Lin, B. B., and Egerer, M. (2017). “Urban agriculture: an opportunity for biodiversity and food provision in urban landscapes,” in Urban Biodiversity: From Research to Practice, eds A. Ossola and J. Niemelä (London: Taylor & Francis/Routledge), 71–86.
Lin, B. B., Egerer, M. H., Liere, H., Jha, S., Bichier, P., and Philpott, S. M. (2018). Local- and landscape-scale land cover affects microclimate and water use in urban gardens. Sci. Total Environ. 610–611, 570–575. doi: 10.1016/j.scitotenv.2017.08.091
Lin, B. B., Philpott, S. M., and Jha, S. (2015). The future of urban agriculture and biodiversity-ecosystem services: challenges and next steps. Basic. Appl. Ecol. 16, 189–201. doi: 10.1016/j.baae.2015.01.005
Louv, R., Fitzpatrick, J. W., Dickenson, J. L., and Bonney, R. (2012). Citizen Science: Public Participation in Environmental Research. Ithica, NY: Cornell University Press.
Lovell, S. T. (2010). Multifunctional urban agriculture for sustainable land use planning in the United States. Sustainability 2, 2499–2522. doi: 10.3390/su2082499
Mandel, L. (2013). Eat Up: The Inside Scoop on Rooftop Agriculture. Gabriola Island, BC: New Society Publishers.
Mauser, W., Klepper, G., Rice, M., Schmalzbauer, B. S., Hackmann, H., Leemans, R., et al. (2013). Transdisciplinary global change research: the co-creation of knowledge for sustainability. Curr. Opin. Environ. Sustain. 5, 420–431 doi: 10.1016/j.cosust.2013.07.001
Milly, P. C., Betancourt, J., Falkenmark, M., Hirsch, R. M., Kundzewicz, Z. W., Lettenmaier, D. P., et al. (2008). Stationarity is dead: stationarity whither water management? Science 319, 573–574. doi: 10.1126/science.1151915
Monneveux, P., Jing, R., and Misra, S. C. (2011). Phenotyping Wheat for Adaptation to Drought. Drought Phenotyping Crop. From Theory to Pract. Montpellier: CGIAR Generation Challenge Programme.
Mougeot, L. J. A. (2000). “Urban agriculture: definition, presence, potentials and risks, and policy challenges,” in Growing Cities, Growing Food: Urban Agriculture on the Policy Agenda. A Reader on Urban Agriculture, eds N. Bakker, M. Dubbeling, S. Gündel, U. Sabel-Koschella, and H. De Zeeuw (Feldafing: DSE/ETC), 99–117.
Oberholtzer, L., Dimitri, C., and Pressman, A. (2014). Organic Agriculture in U.S. Urban Areas. Istanbul: Building Bridges between Organic Farms and Education.
Okvat, H. A., and Zautra, A. J. (2011). Community gardening: a parsimonious path to individual, community, and environmental resilience. Am. J. Commun. Psychol. 47, 374–387. doi: 10.1007/s10464-010-9404-z
Ossola, A., Irlich, U., and Niemelä, J. (2017). “Bringing urban biodiversity research into practice,” in Urban Biodiversity: From Research to Practice, eds A. Ossola and J. Niemelä (London: Taylor & Francis/Routledge), 1–17.
Pataki, D. E., McCarthy, H. R., Gillespie, T., Jenerette, G. D., and Pincetl, S. (2013). A trait based ecology of the Los Angeles urban forest. Ecosphere 4, 1–20. doi: 10.1890/ES13-00017.1
Pollard, G., Roetman, P., and Ward, J. (2017). The case for citizen science in urban agriculture research. Futur. Food J. Food. Agric. Soc. 5, 9–20.
Prasad, P. V., Staggenborg, S. A., and Ristic, Z. (2008). “Impacts of drought and/or heat stress on physiological, developmental, growth and yield processes of crop plants,” in Response of Crops to Limited Water: Understanding and Modeling Water Stress Effects on Plant Growth Processes, eds L. R. Ahuja, V. R. Reddy, S. A. Saseendran, and Q. Yu (Madison, WI: American Society of Agronomy, Inc; Crop Science Society of America, Inc; Soil Science Society of America, Inc), 301–355.
Schultz, L., Folke, C., Österblom, H., and Olsson, P. (2015). Adaptive governance, ecosystem management, and natural capital. Proc. Natl. Acad. Sci. U.S.A. 112, 7369–7374. doi: 10.1073/pnas.1406493112
Seligman, C., and Finegan, J. E. (1990). “A two-factor model of energy and water conservation,” in Social Influence Processes and Prevention: Social Psychological Applications to Social Issues, Vol 1, eds J. Edwards, R. S. Tindale, L. Heath, and E. J. Posavac (Boston, MA: Springer), 279–299. doi: 10.1007/978-1-4899-2094-2_12
Sharp, L., Mcdonald, A., Sim, P., Knamiller, C., Sefton, C., and Wong, S. (2011). Positivism, post-positivism and domestic water demand : interrelating science across the paradigmatic divide. Transact. Inst. Br. Geogr. 36, 501–515. doi: 10.1111/j.1475-5661.2011.00435.x
Shirk, J. L., Ballard, H. L., Wilderman, C. C., Phillips, T., Wiggins, A., and Jordan, R. (2012). Public participation in scientific research: a framework for deliberate design. Ecol. Soc. 17, 29. doi: 10.5751/ES-04705-170229
Steg, L., and Vlek, C. (2009). Encouraging pro-environmental behaviour: an integrative review and research agenda. J. Environ. Psychol. 29, 309–317. doi: 10.1016/j.jenvp.2008.10.004
Stern, P. C., and Dietz, T. (1994). The value basis of environmental concern. J. Soc. Issues 50, 65–84. doi: 10.1111/j.1540-4560.1994.tb02420.x
Tardieu, F., Reymond, M., Hamard, P., Granier, C., and Muller, B. (2000). Spatial distributions of expansion rate, cell division rate and cell size in maize leaves: a synthesis of the effects of soil water status, evaporative demand and temperature. J. Exp. Bot. 51, 1505–1514. doi: 10.1093/jexbot/51.350.1505
Turner, B. (2011). Embodied connections: sustainability, food systems and community gardens. Local Environ. 16, 509–522 doi: 10.1080/13549839.2011.569537
United Nations Department of Economic and Social Affairs PD. (2015). World Urbanization Prospects: The 2014 Revision. New York, NY.
US Climate Data. (2017). California Climate Data. Available online at: http://www.usclimatedata.com/climate.php (Accessed January 11, 2017).
Westley, F. R., Tjornbo, O., Schultz, L., Olsson, P., Folke, C., and Crona, B. (2013). A theory of transformative agency in linked social-ecological systems. Ecol. Soc. 18, 27. doi: 10.5751/ES-05072-180327
Wilke, B. M. (2005). Determination of chemical and physical soil properties. Monit. Assess. Soil Bioremediat. 5, 47–95 doi: 10.1007/3-540-28904-6_2
Wiskerke, J. S. C. (2015). “Urban food systems,” in Cities and Agriculture - Developing Resilient Urban Food Systems, eds H. de Zeeuw and P. Drechsel (London; New York, NY: Routledge), 1–25.
Keywords: urban agriculture, citizen science, water management, drought, California
Citation: Egerer MH, Lin BB and Philpott SM (2018) Water Use Behavior, Learning, and Adaptation to Future Change in Urban Gardens. Front. Sustain. Food Syst. 2:71. doi: 10.3389/fsufs.2018.00071
Received: 13 June 2018; Accepted: 09 October 2018;
Published: 29 October 2018.
Edited by:
Graham K. MacDonald, McGill University, CanadaReviewed by:
Cristina Patanè, Consiglio Nazionale Delle Ricerche (CNR), ItalyCopyright © 2018 Egerer, Lin and Philpott. This is an open-access article distributed under the terms of the Creative Commons Attribution License (CC BY). The use, distribution or reproduction in other forums is permitted, provided the original author(s) and the copyright owner(s) are credited and that the original publication in this journal is cited, in accordance with accepted academic practice. No use, distribution or reproduction is permitted which does not comply with these terms.
*Correspondence: Monika H. Egerer, bWVnZXJlckB1Y3NjLmVkdQ==
Disclaimer: All claims expressed in this article are solely those of the authors and do not necessarily represent those of their affiliated organizations, or those of the publisher, the editors and the reviewers. Any product that may be evaluated in this article or claim that may be made by its manufacturer is not guaranteed or endorsed by the publisher.
Research integrity at Frontiers
Learn more about the work of our research integrity team to safeguard the quality of each article we publish.