- 1Laboratory of Microbiology and Bioprocesses, Federal University of Fronteira Sul, Erechim, Brazil
- 2Embrapa Swine and Poultry, Brazilian Agricultural Research Corporation (EMBRAPA), Concórdia, Brazil
- 3Agricultural Engineering Post-Graduate Programa (UNIOESTE), Department of Agricultural Science, Universidade Estadual do Oeste do Paraná, Cascavel, Brazil
This study evaluated the effect of the substrate:inoculum ratio and digestate recirculation conditions on the biogas and methane yield, free ammonia concentration and solid phase agronomic quality (after treatment) of poultry litter solid state anaerobic digestion (SSAD). Experimental tests were conducted using a central composite design (22), with four trials at the factorial points and three at the central points, using poultry litter as substrate that was collected after 12 cycles of broiler production [TS 76% (w.w−1)]. Three laboratory-scale reactors containing 3 kg of poultry litter each were operated at mesophilic conditions (37°C) and seven experimental runs were performed at retention time of 30 days each. There were three substrate:inoculum ratios (1:1; 1:1.66, and 1:3) and three daily recirculation intervals (2, 3, and 4 times per day), and each recirculation event lasted 15 min. The highest biogas and methane yields were183 LNbiogas. and 74 LNmethane., respectively, and they were obtained at the substrate:inoculum ratio of 1:3 with a digestate recirculation frequency of four times a day. Digestate recirculation was the variable that influenced the concentration of free ammonia; the most frequently recirculated tests had lower concentrations (below 60 mgNH3.L−1). After the SSAD process, we identified a transfer of nutrients (N, P, K) from the solid phase to the liquid phase. The nutrient concentrations in the solid phase (Nout = 11 g.kg−1, Kout = 5 g.kg−1, and Pout = 7 g.kg−1) indicated that the digested poultry litter still has potential for use in fertilization. In this study, it was estimated that 90 m3biogas. was generated using SSAD. Whereas 100 tons of poultry litter are produced after 12 production cycles, the biogas generation capacity reaches 9,000 m3/year. In this scenario, farmers can use biogas from poultry litter SSAD as an additional source of energy.
Introduction
The poultry industry in Brazil has gained worldwide prominence and has experienced a major evolution in the technological aspects related to the production of broilers. In 2016, Brazil produced 12.9 million tons of chicken meat, which ranked second in terms of world production (ABPA, 2017). According to the FAO, from 2000 to 2030, the world will have to increase the meat production by 20%, and the greatest potential growth will be in chicken meat (40.4%)1.
Considering the environmental impact of broiler production, poultry litter management must be closely examined. The major components of poultry litter include the bedding material, feathers, manure and wasted feed. The litter contains plant nutrients such as nitrogen (N), phosphorus (P), and potassium (K) (Bolan et al., 2010). This residue is often applied in agriculture, without prior treatment, as a fertilizer. Due to the organic composition of poultry litter, it is possible to use the litter to generate biogas via the anaerobic digestion (AD) process (Rajagopal and Massé, 2016).
The AD process can be classified according to the total solid (TS) concentration: wet digestion (WD) is typically characterized as TS concentrations below 20%, and dry anaerobic digestion or solid state anaerobic digestion (SSAD) has TS concentrations higher than 20% (Chiumenti et al., 2017).
A challenge for the digestion of poultry litter, when monodigestion is applied, is to avoid the use of the WD process because a large amount of water is used to adjust the litter to a concentration of TS under 15% (the TS concentration of poultry litter is approximately 70%), which generates a very diluted digestate and increases the costs of transport for agricultural use. The SSAD can operate up to 40% of TS, thus significantly reducing the necessity of liquid effluent (digestate) management (Di Maria et al., 2017). Thus, compared to WD, SSAD has several advantages, such as decreased energy requirements for heating, a smaller reactor volume and generation of less liquid wastewater; instead, it generates a digestate that is easier to handle and apply as an organic fertilizer (Ge et al., 2016).
This process was originally applied to treat the organic fraction of municipal solid waste (Li et al., 2011) and has recently been proposed for use in the treatment of agricultural, livestock and poultry wastes, mixed with energy crops, to obtain a solid-phase stabilized product (Chiumenti et al., 2017). Nonetheless, SSAD technology still has a series of aspects that must be improved and studied in greater depth for the maturation of the technology and to diversify the type of substrate used.
The SSAD is a complex process that is, in many cases, associated with the accumulation of ammonia and VFAs, which can lead to inefficient performance and even process failure. The low generation of biogas is a consequence of the complex phenomena occurring at high TS concentrations (Capson-Tojo et al., 2017). Abbassi-Guendouz et al. (2012) reported that a TS concentration of 30% is an obstacle to solid-liquid transfer and decreases the degradation efficiency and inhibits methanogenic activity. The methane yield was approximately 170 L. for up to 25% of TS and fell to below 50 L. for 35% of TS when using food waste (FW). Xu et al. (2014) reported a two-sided effect of TS concentration during AD, with an increase of up to 10%(TS) stimulated microbial growth and a consequent increase in methane generation. On the other hand, a TS concentration of 15–20% increased the resistance to mass diffusion, thereby inhibiting hydrolysis and methanogenesis. Some authors (Abbassi-Guendouz et al., 2012; Di Maria et al., 2013) also reported that a lower water content also caused a rapid accumulation of volatile fatty acids (VFA), which inhibited methanogenesis process.
For substrate dilution, one alternative is to recirculate the liquid digestate during SSAD to improve the results of the process. Recirculation influences the degradation of the solid material in two ways: the movement of moisture through the bed of biomass and the change in moisture content. Supplemental water also dilutes inhibitory substances. It was found that leaching is favorable for the anaerobic decomposition of food waste. However, depending on the frequency of recirculation, it can increase acidogenesis, which may result in the inhibition of methanogenesis (Kusch et al., 2012).
From these studies, we can verify that there is a variability referring to substrate type, substrate:inoculum ratio, TS concentration and dilution influence on biogas and methane yield, thus confirming the need for new investigations to optimize the performance of the digester and apply the SSAD to the poultry litter (Ge et al., 2016). In this context, to use a substrate, such as the litter of poultry in an SSAD process, it is necessary to study the poultry litter: inoculum ratio and the recirculation range, which will allow the biological process to be balanced.
Thus, the main objectives of the present study were to evaluate the influence of the substrate:inoculum ratio and digestate recirculation in biogas and methane production, as well as evaluate the characteristics of poultry after anaerobic treatment.
Materials and Methods
Poultry Litter Sampling and Characterization
The poultry litter after 12 cycles of broiler production was collected at a farm near Concordia, SC, Brazil (27° 8′19.86″latitude and51°43′8.10″longitude). Each cycle housed 14,500 broilers during 42 days in a 1,200 m2 [12 m (wide) × 100 m (length)] aviary (Miele et al., 2010). Samples were characterized in the Laboratory of Embrapa Swine and Poultry, Concordia, SC, Brazil.
Inoculum
The inoculum used for SSAD was collected from a standardized mesophilic acclimated inoculum from a lab-scale CSTR (continuous stirred-tank reactor), according to the methodology described by Steinmetz et al. (2016). The acclimated inoculum was a mixture of equal volumes of anaerobic sludge from two independent large-scale UASB (Upflow Anaerobic Sludge Blanket) reactors (swine wastewater; gelatin manufactory effluent) and from fresh dairy cattle manure. Before use, the inoculum was sifted (2 mm mesh) prior to SSAD reactor inoculation.
Experimental Design and Planning
Three SSAD reactors were used for this study, and each was composed of a vertical cylindrical plastic drum with an internal diameter of 25 cm and measuring 52 cm in height (Figure 1). All reactors were filled with 3 kg of poultry litter and acclimated with anaerobic inoculum. The amount of inoculum added in each reactor varied according to the desired substrate:inoculum ratio (Table 1). The reactors were operated in batch mode at 37°C for 30 days. The fractions (solid and liquid) were separated by a stainless steel plate (1 mm mesh) fixed 15 cm from the bottom of the reactor. The digestate stored at the bottom of the reactor had no contact with the solid fraction. The percolate was pumped from the bottom to the top of the reactor using a peristaltic pump (Provitec AWG 5000, Brazil) at a flow rate of 600 mL.min−1, according to the desired recirculation frequency (Table 1). A top sprinkler (Agrojetp3, Brazil) was used to distribute the liquid on the substrate surface.
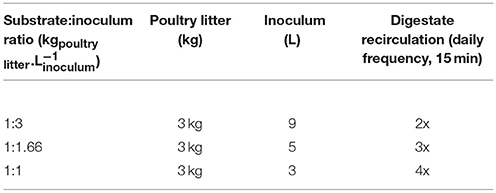
Table 1. Poultry litter and inoculum added according to the desired substrate:inoculum ratio and daily digestate recirculation frequency.
In this study, the experimental planning methodology was applied to evaluate the effects of dilution (substrate:inoculum ratio) and digestate recirculation on biogas and methane production using a CCD (22) with triplicates of the central point (Rodrigues and Iemma, 2014). A statistical analysis was performed by calculating the effects, standard error, t- and p-values. The experimental results were statistically evaluated with 95% of significance (p < 0.05).
Analytical Methods
The TS and VS were analyzed according to APHA (2012). For the analysis of the phosphorus concentration, the samples are first digested in nitro-perchloric solution in a digester block. For this colorimetry methods was used (by the molybdovanadate method) with UV-VIS spectrophotometer model Cary50 (Agilent). Total ammoniacal nitrogen (TAN) was analyzed by colorimetric method (APHA, 2012) using a flow injection analysis system (FIAlab-2500, FIAlab), and the results were expressed as NH3-N. Free ammonia (FA) concentration was calculated according to Equation (1) (Kunz and Mukhtar, 2016).
Biogas produced was measured using a milligascounter (MGC-1 V3.2 PMMA, Ritter Apparatebau). The biogas was collected periodically in bag samplers (plastic/aluminum foil, Hermann Nawrot AG, Germany) and analyzed in portable infrared BIOGAS5000 (Geotech, UK).
Biogas Generation in a Poultry Farm
Energy Demanded From Firewood and Biogas
The estimated biogas energy potential (Equation 2) was calculated considering the methane concentration in biogas (here, 40%) with the lower calorific value (LCV) and methane density. The data used for the calculations can be found in Table 2.
Where:
EBE: estimated biogas energy (kJ.m−3)
LCVmethane: lower calorific value (kJ.kg−1)
ɤmethane: methane density (kg.m−3)
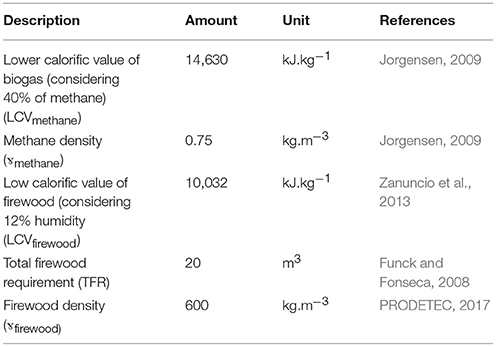
Table 2. Technical coefficient adopted to estimate the firewood and biogas requirement for a broiler rearing (1 cycle).
The firewood energy content (Equation 3) was obtained according to the methodology described by Funck and Fonseca (2008) using firewood's lower calorific value and the total amount of wood used to produce 1 broiler cycle; the data used are presented in Table 2.
Where:
ERfirewood: energy required using firewood (kJ)
TFR: total firewood required (for each cycle) (m3)
FC: firewood density (kg.m−3)
LCVfirewood: lower calorific value of firewood (kJ.kg−1)
The biogas volume required to supply the energy demanded using firewood was calculated according Equation 4 (Funck and Fonseca, 2008).
Where:
BV: biogas volume (m3)
ECfirewood: energy requirement using firewood (kJ)
EBE: estimated biogas energy (kJ.m−3)
Results and Discussion
Characteristics of the Feed Material and Inoculum
The Table 3 presents the characteristics of poultry litter and the liquid inoculum 236 used. The poultry litter presented concentration de 222 (g.kg−1) the C and 24 (g.kg1) 237 the N.
Previous reports suggested that using a feedstock C/N ratio from 27 to 32 promotes steady digester operation at optimum ammonia nitrogen levels and feedstock with a C/N ratio of 32 producing a lower concentration of ammonia nitrogen. Because a high C/N ratio results in the rapid consumption of nitrogen by methanogens, which results in lower gas yield. On the other hand, a low C/N ratio causes accumulation of ammonia at pH above 8.5, causing inhibition of methanogenic activity, and consequently decreasing or even ceasing gas production (Weiland, 2006). The optimum C/N ratio depends on the digestibility of carbon and nitrogen sources (Fernandez et al., 2008). The poultry litter presents C/N ratios ranging from 7 to 10, which are considered low for the anaerobic digestion process (Kelleher et al., 2002; Singh et al., 2010). However, an interesting finding by Kirchmann and Witter (1992) is that during the anaerobic process of poultry manure in uncovered vessels the C/N ratio increases. So if bacteria could withstand the inhibition caused by excessive free ammonia production the system will experience a positive feedback cycle in which an optimum C/N ratio can be achieved (Singh et al., 2010).
Results Obtained From Central Composite Design for Effect of Substrate:Inoculum Ration and Digestate Recirculation in Biogas and Methane Yield
The factorial planning matrix with the experimental variables studied show the proportion of poultry litter: inoculum (X1) and recirculation of the digestion (X2) (Table 4).
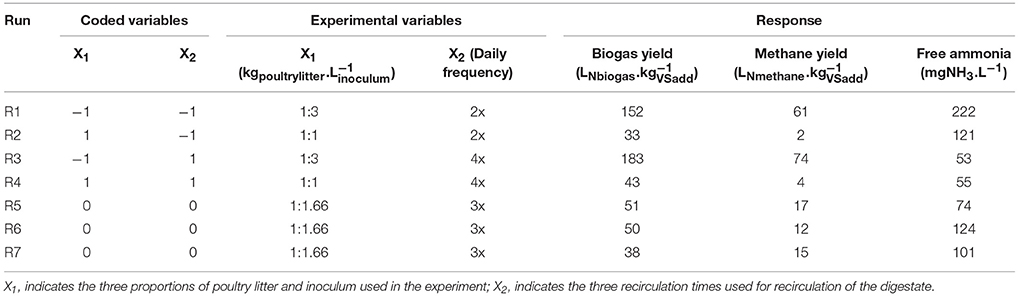
Table 4. Matrix of the composite central design (coded and real values) for the experimental variables poultry litter:inoculum ratio (X1) and digestate recirculation (X2) and respective responses in terms of biogas and methane yield and free ammonia.
For X1, three feed ratios were tested and three recirculation times were tested for the X2 variable, all with a frequency of 15 min. From these variables, the process responses were obtained, which were the biogas and the methane yield and the free ammonia concentration. The Table 5 presents the statistical results found for the effects of the variables poultry litter: inoculum ratio and digestate recirculation on the biogas yield. Parameters with p-values less than 5% (p < 0.05) were considered significant.
The Table 5 presents the statistical results found for the effects of the variables poultry litter: inoculum ratio and digestate recirculation on the biogas yield. The p-value column indicates whether the studied variable was significant for the process. Analyzing the results, we can see that the poultry litter: inoculum ratio had a p-value of 0.002, and we concluded that this variable had a significant effect on the response, with a negative value of t(2); thus, the highest dilutions (1:3) presented higher biogas production.
The other variable studied was the recirculation of the digestate (p-value of 0.095), it had no influence on the response. However, due to great variability inherent in bioprocesses involving biological processes, a 90% confidence level could be adopted (Rodrigues and Iemma, 2014). In this case, the recirculation of the digestate was significant because it had a p-value of less than 0.1. It showed a positive effect in the process, indicating that the digestate treatments that were recirculated four times a day for 15 min had promising results in this study.
Table 6, which show the effects of the variables on methane yield, it can be concluded that the variables presented the same behaviors found for biogas yield. The proportion of poultry litter: inoculum had a p-value of 0.001, which is less than 0.05 and a negative t(2), which is considered significant for the process and indicates that the 1:3 (kgpoultrylitter.L) ratio presented a better condition for methane yield.
The results showed that the poultry litter:inoculum ratio was a crucial parameter. Within the values tested (1:3,1:1.66 and 1:1 kgpoultrylitter.), the initial feed condition of 1:3 showed better yields. It is still the case that digestate recirculation also played an important role in biogas yield and that the frequency of four times a day had a significant effect. Under these conditions, the best yield was found for run R3 183 LNbiogas. and 74 LNmethane. (Table 4). The lowest yields were found for run R2, a poultry litter:inoculum ratio of 1:1 and recirculation twice a day, with a biogas and methane yield of 33 LNbiogas. and 2 LNmethane., respectively.
These biogas and methane production potentials were obtained with a hydraulic retention time (HRT) of 30 days. This HRT was chosen due to the experimental conditions and the management of poultry litter that was already performed by poultry farmers in the study region. However, it has been found that digestion can continue after 30 days, so optimizing the digestion time may improve the recovery and biogas quality of poultry litter.
Other SSAD studies also concluded that with a low substrate:inoculum ratio (1:3 or up to 25% TS), they obtained a higher methane yield, and low methane yields were observed at higher TS concentrations (Abbassi-Guendouz et al., 2012; Di Maria et al., 2012; Sun et al., 2016; Capson-Tojo et al., 2017). The TS content affects the mass transfer among gas-liquid-solid phases and, consequently, the substrate degradation rate. Humidity decrease can also directly affect the microbial metabolism by the diffusion limitation related above and cause an accumulation of inhibitory agents (Ge et al., 2016).
The positive effects of this practice (recirculation and dilution) have been reported by Di Maria et al. (2013), showing a methane production of 211.14 LNmethane. for batch runs with digestate recirculation and 60.14 LNmethane..
Thus, when evaluating the recirculation allied with the dilution factor (Table 4), it was concluded that these factors influenced the efficiency of the process. The biogas yield increased by 18% in run R3 when digestate was recirculated four times per day compared to run R1, with two recirculations per day, when both assays used the same substrate: inoculum ratio.
Good conditions of the mixture in the reactor are important, as they give microorganisms access to the nutrients present in the substrate. To achieve this contact of microorganisms with the substrate and to guarantee adequate humidity conditions, the digestate recirculation process is carried out in the SSAD process because the agitation cannot be carried out in the substrate due to the high total solid concentration (Deutsche GesellschaftfürInternationaleZusammenarbeit, 2010).
Free Ammonia Concentration Effect on SSAD
Variation of FA and pH during the experimental period are shown in Figures 2A,B.
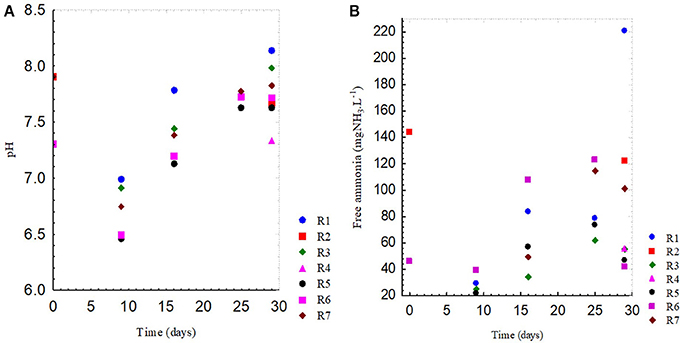
Figure 2. pH values (A) and free ammonia concentration (B) for seven experimental runs. R5, R6 and R7 are the experiments with an substrate:inoculum ratio of 1:1.66 with digestate recirculated 3 times a day. R1 had a substrate:inoculum ratio of 1:3, and R2 had a substrate:inoculum ratio of 1:1; digestate was recirculated 2 times a day in both runs. R3, with a substrate:inoculum ratio of 1:3, and R4, with 1:1, both had digestate recirculated 4 times a day.
The pH was demented from the beginning of reactor operation until 10 days thereafter, probably because of the hydrolysis stage. During this step, high-molecular-mass compounds are degraded to low-molecular-weight compounds. Degradation rate depends on the characteristics of the substrate, so hydrolysis is highly important for the overall speed of the digestion process (Li et al., 2011).
When evaluating the effects of dilution and recirculation on the concentration of free ammonia (Figure 2B), it was observed that an adequate recirculation frequency can contribute to the dilution of inhibitory substances. It was statistically proven that the recirculation variable of the digestate has a significant effect (p < 0.05) on the FA concentration, with 95% confidence (Figure 3). Recirculation had a negative effect, indicating that higher recirculation frequencies had lower concentrations of free ammonia; therefore, trial runs R3 and R4 (recirculated 4 times per day) had the lowest free ammonia values (55 mg.L−1).
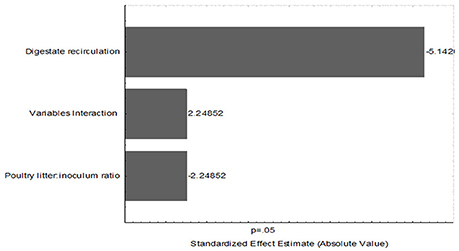
Figure 3. Pareto diagram of the main effects and interaction of the variables evaluated on the concentration of free ammonia in poultry litter SSAD.
The ammonia formation by the degradation of organic nitrogen and the liberation of free ammonia can harm the population of microorganisms in the biodigestion processes, since the ammonia in its free state has high biocidal action (Vinnerås, 2013). As far as the biocidal mechanism of action of free ammonia is concerned, this product has high solubility, not only in water, but also in lipids, which facilitates its entry and diffusion by the cells, and can act in cell destabilization, membrane destruction and protein denaturation, causing cell damage due to the rapid alkalization of the cytoplasm (Diez-Gonzalez et al., 2000).
Characteristics of Poultry Litter Before and After Anaerobic Treatment
The solid fraction (poultry litter) was characterized before and after the SSAD process (Table 7). Run R2 showed the lowest reduction (28%) in addition to a lower yield of biogas and methane. For the other runs, the VS reductions ranged from 19 to 23%. The runs that presented higher humidity at the end of the process, R1 and R3, with a19% reduction in SV concentration, presented the highest yields of biogas and methane, thus contributing to the results found in the present study, which are statistically confirmed.
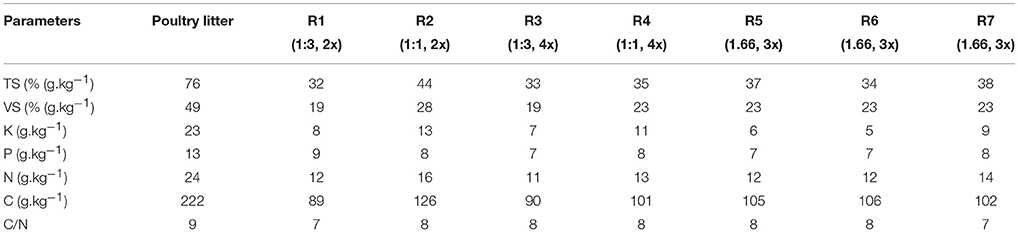
Table 7. Parameters analyzed from poultry litter (before treatment) runs R1–R7 are the parameters analyzed in the solid digestate after 30 days of SSAD.
There was also a decrease in the amount of nutrients (N, P, K) after the SSAD process. Potassium showed a higher reduction after all runs, reaching a lower concentration for R6 and decreasing by 78% (Kin = 23 g.kg−1). This effect is mainly due to the very high solubility of this cation in the water phase (LENNTECH, 2018). The values of nitrogen in the solid fraction after SSAD ranged from 11 to 16(g.kg−1), resulting in a reduction of approximately 55% over the initial value (Nin = 24 g.kg1). This reduction, as described above, can explained by the fact that the nitrogen species present in fresh manure are mainly in organic form, such as urea and protein. Depending on the environmental conditions, a large percentage of this organic nitrogen is converted into TAN, which is soluble in water (Kelleher et al., 2002).
TAN is produced during the ammonification process via the degradation of organic nitrogen, mainly urea and proteins. Ammonium (NH4+) and free ammonia (NH3) are the predominant forms of inorganic nitrogen. It has been shown that free ammonia is the more toxic of the two because it can pass through the cell membrane, thereby causing proton imbalance and potassium deficiency (Apples et al., 2008).
Phosphorus was the nutrient that showed the lowest reduction after the SSAD process. This is because phosphorus can be present in poultry litter in two forms inorganic (35–41%) and organic (58–65%) (Bolan et al., 2010). The P concentration in the solid fraction after SSAD treatment was between 9 and 7 g.kg−1, showing a reduction of45%fromthe initial concentration. The inorganic phosphate species include dibasic calcium phosphate, amorphous calcium phosphate and soluble phosphates; these species are water soluble (Sato et al., 2005); the organic P is mainly in the form of phytic acid salts, which are poorly soluble in water (Turner and Leytem, 2004). This explains why there was a smaller reduction of P: because only the inorganic phosphorus forms exhibit solubility and because they are present in smaller proportions, only the recirculation liquid leached them. However, they could still be recovered, as they are retained in the liquid, and after the process, they can be reused as fertilizer (Nkemka and Hao, 2016).
At the end of the SSAD process, when using the highest dilution factor 1:3 (up to 20% TS), there was an average generation of 3 l of liquid digestate per 1 kg of solid digestate. Compared with the liquid digestion process (with 8% TS), we would have a generation of 6 l of liquid digestate for every 1 kg of solid digestate (Orrico Junior et al., 2010).
Digestate, final effluent, has high potential as a biofertilizer and is used in soil recovery and agricultural production (Massé et al., 2015).
Energy Recovery From Poultry Litter as Biogas for Aviary Heating
During the first days of life, a bird's thermoregulatory system is not yet fully developed. For this reason, especially in winter (from May to August in South America), the farmers'main concern is to provide the necessary thermal comfort conditions for young birds. During this period, the aviary temperature is below ideal conditions, particularly in the southern region of Brazil, thus forcing farms to provide a supplementary heating source for their poultries (Tabler et al., 2014).
There are several heating systems used in poultry production. In this case, the wood-heating system was adopted as a reference because of its importance as a biomass source for heating systems in Brazil, mainly due to its low cost (Coradi et al., 2016). However, it also presents some drawbacks, such as the in complete burning of wood in a precarious heating system, aromatic and soot emissions that can cause environmental impacts and occupational health damage to farmers (Oanh et al., 2005).
Considering an aviary of 100 m × 12 m with the capacity to house 14,500 broilers, the poultry litter was reused for up to 12 production cycles, estimating that the aviary has the capacity to produce 100 tons of poultry litter (De Carli, 2018). According to the results observed after poultry litter SSAD, the best condition can generate 183LNbiogas. (Table 4). The composition of poultry litter presents 490 gVS.(Table 1); therefore, the capacity of biogas production can be estimated as 90 m3biogas., considering that with 100 tons of poultry litter (after 12 cycles), we will have an estimated biogas generation of 9,000 m3biogas.
For the analysis of energy equivalence of firewood and biogas, we considered the heating of 1 cycle of chicken rearing, with 21 days of heating during the winter period (Palhares, 2012). The results of Equations (2)–(4) are presented in Table 8. In order to supply the energy demand of the system a biogas volume (BV) of 11,000 m3. In this study, the percentage of methane found in biogas was 40%, with a low calorific power (14,212 kJ) compared with the already tabulated data, which describe a potential of 20,900–29,290 kJ of biogas with carbon dioxide remaining in its composition (Jorgensen, 2009). Thus, the scenario would not be favorable for the total replacement of firewood with biogas.
However, in addition to the substitution, biogas can be used as a supplementary source of heating because in Brazil, two energy sources, firewood and liquefied petroleum gas (LPG) (Migliavacca and Yanagihara, 2017), are already used in many facilities. The study presented satisfactory results, concluding that commercial burners for LPG can be adapted for biogas, with heating efficiencies similar to those obtained with the original fuel. Thus, by using biogas for heating poultry, we will be taking advantage of the energy potential of the waste produced by the activity itself, thus reducing the expenses from the use of other energy sources.
In Brazil, the states in the southern region are responsible for the largest producers of broilers. However, other regions, such as the midwest, already present growth rates in the production of broilers (ABPA, 2017). In this region, the average temperatures throughout the year are approximately 20–24°C (Instituto Nacional de Meteorologia, 2018). With this in mind, the use of biogas for aviary heating systems can be more attractive than for the southern part of Brazil. The consumption of wood for heating and aviaries considering this condition, is approximately 12 m3 of wood per cycle of poultry litter (Miele et al., 2010). Thus, using Equations (3) and (4) and the coefficients presented in Table 4, a volume of 6,583 m3 of biogas is necessary to suppress the demand for energy from firewood. These conditions are more favorable for replacing firewood with biogas, as heating occurs only when the temperatures decrease, mainly at night (Palhares, 2012).
In addition, warmer regions are more favorable for the development of the anaerobic digestion process, especially for anaerobic digesters without heating systems and with more stable processes and a better quality of biogas and digestate (Babaee et al., 2013).
Conclusions
The solid state anaerobic digestion of poultry litter is a promising process that has advantages as a good, robust method with low-water consumption and, consequently, low-liquid digestate production.
Based on the results, the recirculation in SSAD had an influence on the free ammonia concentration, indicating that those tests with a higher recirculation frequency presented lower concentrations of free ammonia (55 mg.L−1). After the SSAD process, there was a decrease in the nutrients N, P, and K to values 11, 7, and 5 (g.kg−1), respectively, in the solid phase. However, the solid digestate still had a satisfactory quantity of nutrients for use in agriculture as fertilizer.
Biogas can be used as a supplementary source of energy, and this method is already used by poultry farmers in Brazil with wood and LPG. This situation is even more favorable when considering regions with tropical conditions.
The biogas and methane recovery results are relevant to the study because of the reactor configuration and retention time adopted. Other studies that optimize the retention time and reactor configuration can improve the biogas recovery, the quality of the biogas and, therefore, the economic viability.
Author Contributions
VM contributed in the accomplishment of the experiments, treatment and discussion of the data and writing of the article. RS assisted with the execution of the experiments, treatment and discussion of the data and writing of the article. AdA assisted with conducting the experiments, processing and discussing the data and writing the article. TG contributed to the accomplishment of the experiments, treatment and discussion of the data and writing of the article. HT assisted with the accomplishment of the experiments, treatment and discussion of the data and writing of the article. AK also assisted in the execution of experiments, treatment and discussion of the data and writing of the article.
Funding
This work was financially supported by the project Eletrosul Centrais Elétricas S.A Uirapuru transmissora de Energia S.A [projeto N° 1110130054(N°14/2012 ANEEL)] and BiogásFert Network (Project N°453 021280400-02).
Conflict of Interest Statement
The authors declare that the research was conducted in the absence of any commercial or financial relationships that could be construed as a potential conflict of interest.
Acknowledgments
We are thankful for the support from CNPQ.
Footnotes
1. ^USDA–United States Department Of Agriculture Livestock, and Poultry: World Markets and Trade (2013). Available in: http://www.thepoultrysite.com/reports/?id=1788 (Access in: 14, set.2017).
References
Abbassi-Guendouz, A., Brockmann, D., Trably, E., Dumas, C., Delgenés, J. F., Steyer, J. F., et al. (2012). Total solids content drives high solid anaerobic digestion via mass transfer limitation. Bioresour. Technol. 111, 55–61. doi: 10.1016/j.biortech.2012.01.174
ABPA (Brazilan Asssociation of Animal Protein) (2017). Annual Report. Available online at: http://abpa-br.com.br/storage/files/final_abpa_relatorio_anual_2017_ingles_web.pdf (Acessoem 15, set.2017).
APHA AWWA, WEF. (2012). Standard Methods for the Examination of Water and Wastewater, 22nd Edn. Washington, DC: APHA.
Apples, L., Baeyens, J., Degrève, J., and Dewil, R. (2008). Principles and potential of the anaerobic digestion of waste-activated sludge. Prog. Energy Combust. Sci. 34, 755–781. doi: 10.1016/j.pecs.2008.06.002
Babaee, A., Shayegan, J., and Roshani, A. (2013). Anaerobic slurry co-digestion of poultry manure and straw: effect of organic loading and temperature. J. Environ. Health Sci. Eng. 11:15. doi: 10.1186/2052-336X-11-15
Bolan, N. S., Szogi, A. A., Chuasavathi, T., Seshdri, B., Rothrock, M. J., and Panneerselvam, P. (2010). Uses and management of poultry litter. World Poult. Sci. J. 66, 673–698. doi: 10.1017/s0043933910000656
Capson-Tojo, G., Trably, E., Rouez, M., Crest, M., Steyer, J. F., Delgenés, J. F., et al. (2017). Dry anaerobic digestion of food waste and cardboard at different substrate loads, solid contents and co-digestion proportions. Bioresour. Technol. 233, 166–175. doi: 10.1016/j.biortech.2017.02.126
Chiumenti, A., de Borso, F., and Limina, S. (2017). Dry anaerobic digestion of cow manure and agriculture products in a full-scale plant: efficiency and comparison with wet fermentation. Waste Manag. 71, 704–710. doi: 10.1016/j.wasman.2017.03.046
Coradi, P. C., Martins, M. A., da Costa, D. R., Vigoderis, R. B., and de Tinôco, I. F. F. (2016). Computational fluid dynamics (cfd) simulating heated air from wood burning inside a poultries barn. Engenharia Agricola J. Braz. Assoc. Agric. Eng. 36, 768–778. doi: 10.1590/1809-4430-Eng.Agric.v36n5p768-778/2016
De Carli, S. (2018). Personal Information. Ferticel - Estimated production of chicken bed in aviary of 100 meters.
Deutsche GesellschaftfürInternationaleZusammenarbeit, GIZ. (2010). Guide to Biogas: From Production to Use. Dag-Hammarskjöld-Weg 1-5 65760, Eschborn.
Di Maria, F., Barratta, M., Biaconi, F., Placidi, P., and Passeri, D. (2017). Solid anaerobic digestion batch with liquid digestate recirculation and wet anaerobic digestion of organic waste: comparison of system performances and identification of microbial guilds. Waste Manag. 59, 172–180. doi: 10.1016/j.wasman.2016.10.039
Di Maria, F., Gigliotti, G., Sordi, A., Micale, C., Zadra, C., and Massaccesi, L. (2013). Hybrid solid anaerobic digestion batch: biomethane production and mass recovery from the organic fraction of solid waste. Waste Manag. Res. 31, 869–873. doi: 10.1177/0734242X13477902
Di Maria, F., Sordi, A., and Micale, C. (2012). Optimization of solid state anaerobic digestion by inoculum recirculation: the case of an existing mechanical biological treatment plant. Appl. Energy. 97, 462–469. doi: 10.1016/j.apenergy.2011.12.093
Diez-Gonzalez, F., Jarvis, G. N., Adamovich, D. A., and Russel, J. B. (2000). Use of carbonate and alkali to eliminate Escherichia coli from dairy cattle manure. Environ. Sci. Technol. 34, 1275–1279. doi: 10.1021/es9910356
Fernandez, J., Perez, M., and Romero, L. I. (2008). Effect of substrate concentration on dry mesophilic anaerobic digestion of organic fraction of municipal solid waste (OFMSW) (OFMSW). Bioresour. Technol. 99, 6075–6080. doi: 10.1016/j.biortech.2007.12.048
Funck, S. R., and Fonseca, R. A. (2008). Energetic evaluation and performance of broilers with automatic heating using gas and wood. Braz. J. Agric. Environ. Eng. 12, 91–97. doi: 10.1590/S1415-43662008000100014
Ge, X., Xu, F., and Li, Y. (2016). Solid-state anaerobic digestion of lignocellulosic biomass: recent progress and perspectives. Bioresour. Technol. 205, 238–249. doi: 10.1016/j.biortech.2016.01.050
Instituto Nacional de Meteorologia, INMET. (2018). INMET–Instituto Nacional de Meteorologia. Available online at: http://www.inmet.gov.br/portal/index.php?r=tempo/graficos (Accessed January 10, 2018).
Jorgensen, P. J. (2009). Jorgensen, P. J. (2009). Biogas: Green Energy. PlanEnergi and Researcher for a Day. Tjele, DK: Faculty of Agricultural Sciences; Aarhus University.
Kelleher, B. P., Leahy, J. J., Henihaan, A. M., O'Dwyer, T. F., Sutton, D., and Leahy, M. J. (2002). Advances in poultry litter disposal technology-a review. Bioresour. Technol. 83, 27–26. doi: 10.1016/S0960-8524(01)00133-X
Kirchmann, H., and Witter, E. (1992). Composition of fresh, aerobic and anaerobic farm animal dungs. Biores. Techonol. 40, 137–142. doi: 10.1016/0960-8524(92)90199-8
Kunz, A., and Mukhtar, S. (2016). Hydrophobic membrane technology for ammonia extraction from wastewaters. EngenhariaAgrícola. 32, 377–386. doi: 10.1590/1809-4430-Eng.Agric.v36n2p377-386/2016
Kusch, S., Oschsner, H., and Jungbluth, T. (2012). Effect of various leachate recirculation strategies on batch anaerobic digestion of solid substrates. J. Environ. Waste Manag. 9, 69–88. doi: 10.1504/IJEWM.2012.044161
LENNTECH (2018). Potassium and Water: Reaction Mechanisms, Environmental Impact 588 and Health Effects. Available online at: https://www.lenntech.com/periodic/water/potassium/potassium-and590Water.htm#ixzz56nxQ3OTY (Accessed 11 fev.2018).
Li, Y., Park, S. Y., and Zhu, J. (2011). Solid-state anaerobic digestion for methane production from organic waste. Renew. Sustain. Energy Rev. 15, 821–826. doi: 10.1016/j.rser.2010.07.042
Massé, D. I., Saady, N. M. C., and Gilbert, Y. (2015). Psychrophilic dry anaerobic digestion of cow feces and wheat straw: feasibility studies. Biomass Bioenergy 77, 1–8. doi: 10.1016/j.biombioe.2015.03.017
Miele, M., Abreu, P. G., De Abreu, V. M. N., Jaenisch, F. R. F., Martins, F. M., Mazzuco, H., et al. (2010). Coeficientes técnicos para o cálculo do custo de produção de frango de corte. Comunicado Técnico 483, Embrapa Suínos e Aves.
Migliavacca, A., and Yanagihara, J. I (2017). Mass balance applied to brazilian conventional broiler houses during one production cycle. Braz. J. Poult. Sci. 19, 75–86. doi: 10.1590/1806-9061-2016-0365
Nkemka, V. N., and Hao, X. (2016). Start-up of a sequential dry anaerobic digestion of paunch under psychrophilic and mesophilic temperatures. Waste Manag. 74, 144–149. doi: 10.1016/j.wasman.2016.06.022
Oanh, N. T. K., Albina, D. O., Ping, L., and Wang, X. (2005). Emission of particulate matter and polycyclic aromatic hydrocarbons from select cookstove-fuel systems in Asia. Biomass Bioenergy 28, 579–590. doi: 10.1016/j.biombioe.2005.01.003
Orrico Junior, M. A. P., Orrico, A. C. A., and Júnior de Lucas, J. (2010). Anaerobic biodigestion of waste from aviculturalproduction:browser of broilers and carcass. Engenharia Agrícola 30, 546–554. doi: 10.1590/S0100-69162010000300017
Palhares, J. C. (2012). Use of the Chicken Bed in Biogas Production. Ergomix. Available online at: https://pt.engormix.com/avicultura/artigos/cama-de-frango-producao-de-biogas-t37681.htm (Accessed January 10, 2018).
PRODETEC (2017). Specific Weight of Materials. Available online at: http://www.prodetec.com.br/downloads/pesos_especificos.pdf (Access in: 19.out.2017).
Rajagopal, R., and Massé, D. (2016). Start-up of dry anaerobic digestion system for processing solid poultry litter using adapted liquid inoculum. Process Saf. Environ. Prot. 102, 495–502. doi: 10.1016/j.psep.2016.05.003
Rodrigues, M. I., and Iemma, A. F. I. (2014). Experimental Design Process Optimization. Boca Raton, FL: CRC Press.
Sato, S., Solomon, D., Hyland, C., Ketterings, Q. W., and Lehmann, J. (2005). Phosphorus speciation in manure and manure-amended soils using XANES spectroscopy. Environ. Sci. Technol. 39, 7485–7491. doi: 10.1021/es0503130
Singh, K., Lee, K., Worley, J., Risse, M. L., and Das, K. C. (2010). Anaerobic digestion of poultry litter: a review. Appl. Eng. Agric. 26, 677–688. doi: 10.13031/2013.32061
Steinmetz, R. L. R., Mezzari, M. P., da Silva, M. L. B., Kunz, A., do Amaral, C., Tápparo, D. C., et al. (2016). Enrichment and acclimation of an anaerobic mesophilic microorganism's inoculum for standardization of BMP assys. Bioresour. Technol. 219, 21–28. doi: 10.1016/j.biortech.2016.07.031
Sun, C., Cao, W., Banks, C. J., Heaven, S. O., and Liu, R. (2016). Biogas production from undiluted chicken manure and maize silage: a study of ammonia inhibition in high solids anaerobic digestion. Bioresour. Technol. 219, 1215–1223. doi: 10.1016/j.biortech.2016.07.082
Tabler, T., Liang, Y., Moyle, J. R., Clark, F. D., and Farnell, M. M Wells, J. (2014). Managing Heat and Minimum Ventilation Systems in the Broiler House. the Poultry Site. Available online at: http://www.thepoultrysite.com/articles/3297/managing-heat-and-minimum-ventilation-systems-in-the-broiler-house/ (Accessed January 5, 2018).
Turner, B. L., and Leytem, A. B. (2004). Phosphorus compounds in sequential extracts of animal manures:chemical speciation and novel fractionation procedure. Environ. Sci. Technol. 38, 6101–6108. doi: 10.1021/es0493042
Vinnerås, B. (2013). “Sanitation and hygiene in manure management,” in Animal Waste – Recycling, Treatment and Management, eds S. G. Sommer, L. S. Jensen, M. L. Christensen, and T. Schmidt (Oxford: Wiley-Blackwell).
Weiland, P. (2006). “State of the art of solid-satate digestion - recente developmentes,” in Solid-Satatedigestion- State of the Art and Further Randd Requirements, 24, ed F. N. Rohstoffe (Gülzow-Prüzen: Gulzower Fachgespräche), 22–38.
Xu, F., Wang, Z. W., Tang, L., and Li, Y. (2014). A mass diffusion-based interpretation of the effect of total solids content on solid-state anaerobic digestion of cellulosic biomass. Bioresour. Technol. 167, 178–185. doi: 10.1016/j.biortech.2014.05.114
Keywords: biogas yield, methane yield, central composite design, poultry litter, free ammonia concentration
Citation: Marchioro V, Steinmetz RLR, do Amaral AC, Gaspareto TC, Treichel H and Kunz A (2018) Poultry Litter Solid State Anaerobic Digestion: Effect of Digestate Recirculation Intervals and Substrate/Inoculum Ratios on Process Efficiency. Front. Sustain. Food Syst. 2:46. doi: 10.3389/fsufs.2018.00046
Received: 20 April 2018; Accepted: 17 July 2018;
Published: 07 August 2018.
Edited by:
Barbara Amon, Leibniz-Institut für Agrartechnik und Bioökonomie (ATB), GermanyReviewed by:
Jun Zhu, University of Arkansas, United StatesAriel A. Szogi, Agricultural Research Service (USDA), United States
Copyright © 2018 Marchioro, Steinmetz, do Amaral, Gaspareto, Treichel and Kunz. This is an open-access article distributed under the terms of the Creative Commons Attribution License (CC BY). The use, distribution or reproduction in other forums is permitted, provided the original author(s) and the copyright owner(s) are credited and that the original publication in this journal is cited, in accordance with accepted academic practice. No use, distribution or reproduction is permitted which does not comply with these terms.
*Correspondence: Airton Kunz, YWlydG9uLmt1bnpAZW1icmFwYS5icg==