- 1School of Construction and Environmental Engineering, Tampere University of Applied Sciences, Tampere, Finland
- 2Finnish Environment Institute SYKE, Helsinki, Finland
The growing demand for food and the increasing costs of cultivation are posing a challenge for agriculture. Diminishing phosphorus reserves, as well as the energy intensive method of producing nitrogen fertilisers are drivers for more intensive reuse of different organic fertilisers, such as manures and excreta. Source separation and fertilisation with human urine can be one option for nutrient reuse. Urine contains all the main nutrients as well as micronutrients in soluble form, but it also contains chemicals, like pharmaceuticals and hormones. The aim of this study was to examine the efficiency and safety of the use of source separated human urine as a fertiliser for barley (Hordeum vulgare). The fertiliser efficiency of source-separated urine was examined in field-scale experiments for the first time in Finland. Two separate cultivation experiments in two fields and barley varieties were conducted. The efficiency of urine as a fertiliser was compared to corresponding amount of mineral fertiliser. No fertiliser was applied to one plot in order to create a reference treatment. The two experiments were conducted using variety Wolmari with 54 kg N ha−1 and variety Harbinger with 100 kg N ha−1. The barley grain and straw yield grown with urine fertiliser was equivalent to the yield in mineral fertilised plots. The growth of barley in both fertiliser treatments was slightly faster, compared to non-fertilised treatment. There were no significant differences between the treatments in terms of protein content of the grain although the results varied in terms of the thousand grain weight (TGW) and germination. The urine analyses indicated that there were no pathogen indicators, nor heavy metal concentrations, exceeding the limit values set by legislation. The main nutrient concentrations (N, P, K) would also meet the requirements for a fertiliser product according to Finnish legislation. Pharmaceuticals and hormones were found from the urine, but apart from progesterone, all of them presented extractable values in soil below the detection limits, and they were not detected in measurable amounts in barley grain at the end of the growing season. These results suggest that source separated urine could be an efficient fertiliser in crop cultivation.
Introduction
Phosphorus is an essential nutrient in crop cultivation. It is estimated, that there are affordable and relatively easily extractable phosphate rock reserves left for only another 50–100 years. Also, the quality of the phosphorus mineral is diminishing. Yet there is an ever increasing demand for phosphorus in agriculture (Cordell et al., 2009, 2011). The production of nitrogen fertilisers—another crucial element in food production - in turn is highly energy intensive. Therefore, in the current need for increasing resource efficiency, we need to look for more intensive nutrient reuse for agricultural purposes. Source separation of human urine offers one option for nutrient reuse from human waste. Urine can be diverted from the solid excreta and it can be used as a liquid fertiliser as such. This diversion enables reuse of waste for agriculture purposes and at the same time protects the natural water bodies from waste pollution and eutrophication (Vinnerås and Jönsson, 2002). Source separated human urine is a nutrient-rich liquid where the main nutrients (N, P, K) occur in water-soluble ionic form and are therefore readily available for plant uptake (Schönning, 2006; Udert et al., 2006). The use of source separated human urine could be one option to complete the demand of nitrogen fertilisation and to close the nutrient cycle.
Source separated urine has been found to be a safe and efficient fertiliser for many crops and vegetables and has been studied in many different contexts since the late 1990s (Kirchmann and Pettersson, 1995; WHO, 2006; Heinonen-Tanski et al., 2007; Mnkeni et al., 2007; Pradhan et al., 2007; Chowdhury and Islam, 2008; Viskari et al., 2009; Pradhan, 2010). However, in Finland and in many other European countries, urine is not accepted as a fertiliser, and there are many institutional constraints in its use.
Source separated urine has the potential to be used as a fertiliser because of its nutrient content, availability and easy application to soils. The main nutrients (nitrogen, phosphorus, potassium and sulphur) occur in water-soluble ionic form and are therefore readily available for plant uptake (Schönning, 2006). The majority of the nutrients are excreted via urine (Schouw et al., 2002; Rose et al., 2015). From nitrogen about 90%, phosphorus 50–65% and potassium, 50–80% is excreted in urine (Lapid, 2008). In addition, urine contains Cl−, Na, Mg, Cu and other organic and inorganic compounds, which can be utilised for plant growth. The amount of nitrogen and phosphorus in urine varies depending on the diet, person and time of the day. For example, nitrogen present in urine can reach a concentration of up to 9 g N L−1, the concentration of phosphorus is around 0.7 g P L−1 (Winker et al., 2009). In comparison with other household waste, urine contains considerably more nutrients than faeces, greywater and biodegradable suspended solids (Vinnerås and Jönsson, 2002; Pradhan, 2010). Table 1 shows the estimated amounts of nitrogen, phosphorus and BOD produced in urine and faeces per capita annually. The estimated amounts vary, however, depending on the collection and analysis method. For example, Vinnerås and Jönsson (2002) estimated that about 3.7 kg of nitrogen, 0.34 kg of phosphorus and 1.2 kg of potassium per capita/a could be recovered if all urine could be fully separated from other wastewaters. Nitrogen in urine is mostly in the form of urea and/or ammonium (Kirchmann and Pettersson, 1995; Richert Stintzing et al., 2001). Phosphorus (P) and potassium (K) in turn are almost entirely in inorganic, ionic form (Lentner, 1981), which is directly plant-available (Kirchmann and Pettersson, 1995), although inorganic P in soil may be rapidly, and extensively adsorbed to Al, Fe minerals, or precipitated with Ca.
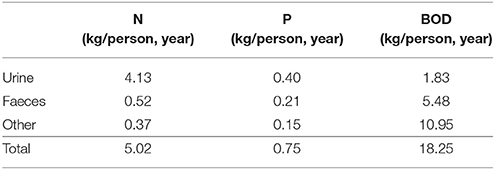
Table 1. The estimated amount of nutrients produced in excreta and greywater by one person per year (Weckman, 2005; Udert et al., 2006; Ministry of the Environment Finland, 2017).
Concerning the hygienic issues, the urine of a healthy person contains only a small amount of pathogens, originating mostly from faecal contamination (Höglund et al., 1998). Storage is an easy method for the urine disinfection in case of any contamination (Höglund, 2001). The current recommendation for urine storage is 6 months at a temperature over 22°C (Jönssön et al., 2004; Schönning, 2006; WHO, 2006). However, research on the disinfecting effects of urea showed that no E. coli or Salmonella spp. were found after 5 days of storage. Significant reduction in phage was observed after 21 days and no viruses were found after 50 days. The study indicates that the storage time for safe urine reuse is 2 months at a temperature over 20°C when the nitrogen concentration in the urine is greater than 2 g N L−1 and pH is over 8.8. (Vinnerås et al., 2003, 2008; Winker et al., 2009).
Concerns about the use of urine as a fertiliser have also arisen. The potential risks of various pharmaceutical and hormone residues as well as other potential micro-pollutants as contaminants are seen as an obstacle. Knowledge of their presence in urine and their behaviour in the soil is still limited. Humans are exposed to different contaminants, like heavy metals, in our environment through skin contact, respiration and diet and the contaminants are excreted in sweat, urine and faeces (e.g., Schouw et al., 2002; Genuis et al., 2011). Compared with animal manure and industrial fertilisers the heavy metal concentrations of urine are lower (Jönssön et al., 2004; Winker et al., 2009). On the other hand, on average, 2/3 of the drugs used by humans are excreted via urine and about 1/3 in the faeces (Lienert et al., 2007). The potential health risks associated with the use of urine and faeces as fertilisers have been extensively studied, and WHO guidelines have been developed for the urine treatment and use (WHO, 2006).
There are existing technologies for nutrient recovery, such as separating dry toilets. There are many factors, however, that are preventing or hindering the nutrient recovery on a larger scale. These are for example public opinion toward the use of urine as a fertiliser, missing storage facilities and logistical chains, as well as legislative barriers (Magid et al., 2006; Lienert and Larsen, 2010).
The aim of this study was to acquire scientific data on the use and efficiency of urine in crop cultivation. For the first time in Finland, field scale experiments using urine as a fertiliser were conducted using barley as a test crop.
Materials and Methods
The feasibility of source separation of urine, management and potential as a fertiliser was examined in this study. The fertiliser efficiency of source-separated urine was tested in two field-scale experiments using barley (Hordeum vulgare, var. Wolmari and Harbinger) as a test plant. The experiments were implemented in cooperation with two farms within 80 kilometres from Tampere (Central Finland). Urine fertilisation was compared with corresponding levels of mineral fertiliser and non-fertilised sites were used as a reference within the experimental fields. The amount of urine spread was defined by farmers, and was based on the field-specific requirements in terms of nitrogen requirement. Other environmental requirements, originating from farming subsidies and environmental regulations were also determining the fertilisation levels in the fields. Experiments were carried out in ways that would cause the least disturbance to the farmers.
Ethics Review
According to the guidelines of Finnish National Board on Research Integrity and statement from the Academic Ethics Committee of the Tampere Region, the body dealing with ethical statements of Tampere University of Applied Sciences, ethics approval and further consent was not required in this kind of research study. Written and informed consent concerning the implementation of experiments and publication of the results was obtained from the farmers that participated this study. The urine used in the study was collected from thousands of anonymous participants of a festival in August 2015. No oral or written consent were asked from them. Anonymity and discretion, however, was assured at every step in the urine management during this study. From the private household, that also donated urine for the project an oral and informed consent was obtained and the anonymity assured by keeping the detailed information of the donors only at the attention of the authors.
Urine as Fertiliser
Urine was collected from two different sources. The largest amount, total of 4.8 m3 of urine, was collected from male urinals of a Weekend Music Festival with about 40,000 participants in Helsinki in August 2015. Another set of urine (1.5 m3) was collected with a separating dry toilet from a private household with a family of four persons. Both of the urine batches were stored over winter in sealed 1 m3 IBC-containers until used in spring 2016. The storage fulfilled the criteria for safe management and use of human excreta in agriculture (WHO, 2006). The microbial quality, nutrient and element content as well as pharmaceuticals and hormone concentrations were analysed from the urine. Summary of different analysis methods of urine is presented in Table 2.
Field Experiments
The potential of urine as a fertiliser was studied by comparing it with a corresponding amount of nitrogen in mineral fertiliser and using non-fertilised plots as a reference. The experimental fields were divided into three different plots with the different fertiliser treatments (Figures 1A,B). Monitoring was conducted in and samples taken from square shaped sampling plots sized 50*50 cm in each fertiliser treatment plot. In Field 1 there were seven sampling plots per fertiliser treatment, resulting in 21 sampling plots in total and in Field 2 10 sampling plots, resulting in 30 sampling plots in total. The sampling plots were placed to the different fertiliser treatment sites using systematic placing into lines. The soil physico-chemical characteristics and main nutrient content of the soil in experimental plots were analysed. The analysis methods are summarised in Table 2 and results are presented in Table 3.
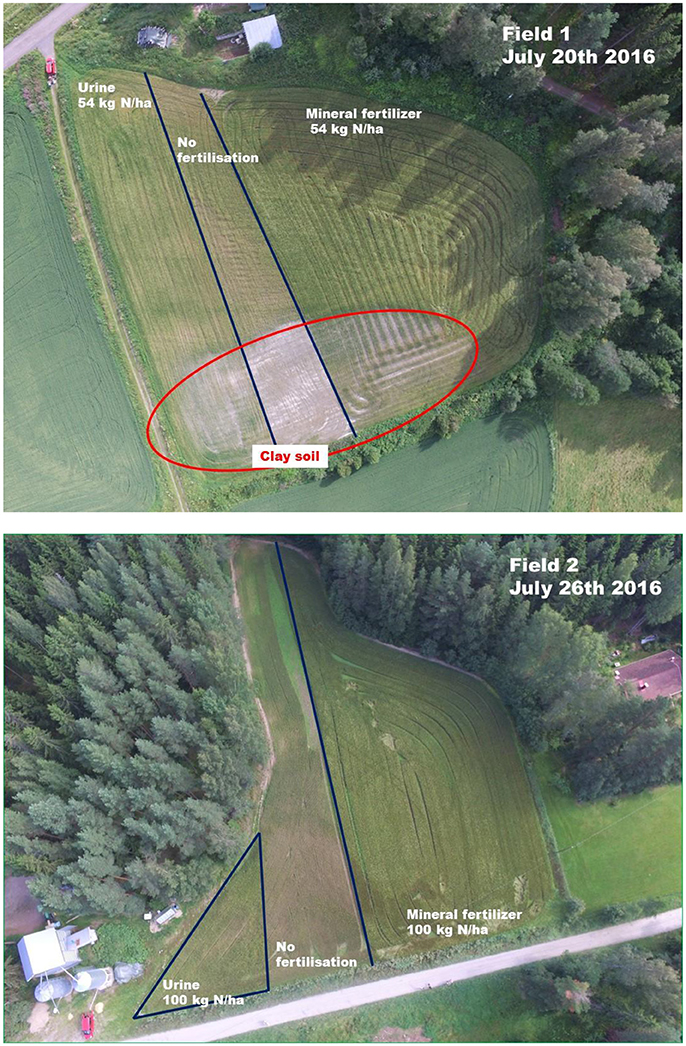
Figure 1. Aerial views of the experimental fields. (A) Field 1 with fertiliser treatment 54 kg N ha−1 on July 20th 2016. (B) Field 2 with fertiliser treatment 100 kg N ha−1 on July 26th 2016.
There were two different barley varieties, Wolmari and Harbinger, and two nitrogen fertilisation levels (54 kg N ha−1 in the field 1 and 100 kg N ha−1 in the field 2) were used in the experiments respectively (Table 4). The urine was spread about 2 weeks after sowing to ensure the efficient uptake and use of the nutrients in the beginning of the growth. In field 1, urine was spread using a slurry tanker equipped with a disc injector while in field 2 urine was spread manually by simulating deep injection. The simulation was carried out using a sharpened metal tube attached to a 10 l watering can and by pressing the tip of the tube into the soil while pouring the urine into the tube. When injecting urine directly into the soil, nitrogen losses are reduced and nutrient uptake enhanced (Johansson, 2000). Mineral fertiliser was amended simultaneously with the seeds. Table 4 summarises the experimental setup in different fields.
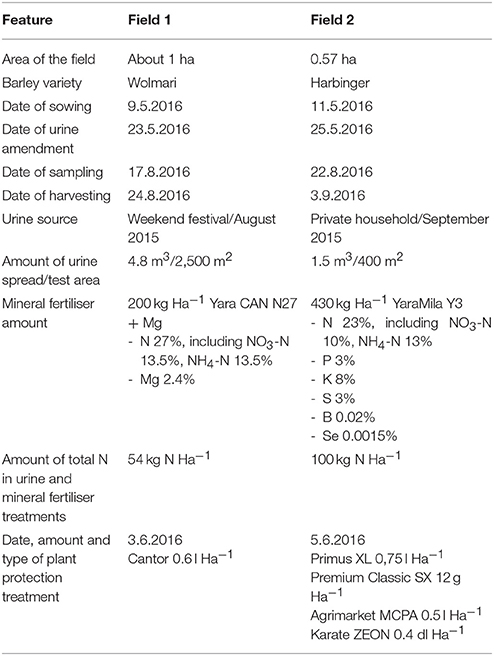
Table 4. Summary about the experimental setup and implementation of the fertilisation test in the two test fields.
Barley growth and condition was monitored every second week by recording the growth stages of the stems using Zadoks cereal development scale with 99 different stages (e.g., Boys and Geary, 2015), which describes the growth and ripening of the cereal. At the end of the growing season both the barley grains and straw were harvested from the sampling plots and biomass, protein content of the grain, germination and weight of thousand grains (TGW) measured. These results were compared between the different fertiliser treatments. Comparison of the results were also made with the official variety test results made by the Natural Resources Institute Finland (Luke). Official variety tests are implemented cultivating the different crop varieties “using common cultivation methods” and in areas suitable for the cultivation of the crop in question (Laine et al., 2016). This means that fertilisation needed is defined by, for example, the soil quality, desired yield and the level of nitrogen required. The yield results from the 10 sampling plots (0.25 m2) were extrapolated to hectare level. Furthermore, pharmaceutical and hormone concentrations of the soil and barley grains were analysed at the end of the growing season to see the possible accumulation of these micro-pollutants in the soil or yield.
Statistical Analyses
Descriptive statistical analyses of barley yield and characteristics were carried out using IBM SPSS Statistics software (version 22). Mean values and standard deviations are presented from the yield data. No comparison or statistical tests were made between the fields, because of different locations, barley variety and fertilisation levels used.
Results
Urine as Fertiliser
The urine contained relatively high amounts of nitrogen and other macro and micro-nutrients (Table 5). Overall average NPK-ratio of all urine collected (20-1.2-4) was almost equivalent to a commercial mineral fertiliser, for example YaraMila Y1 (27-1.3-4). The NPK ratio of urine in Field 1 with 54 kg N ha−1 was 28-1.6-4.9 and Field 2 with 100 kg N ha−1 25-2.3-7.3 respectively. Stored urine contained no pathogen indicators Salmonella or E. coli and the concentrations of harmful metals were significantly below the limit values given in the Finnish legislation (Ministry of Agriculture and Forestry Finland, 2011).
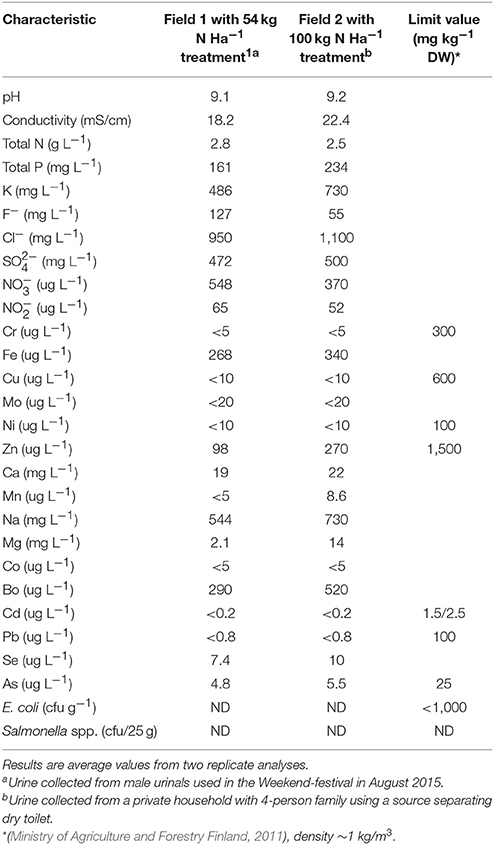
Table 5. Average pH, conductivity, element, anion and pathogen amounts in urine used in the fertiliser experiments at different fields and corresponding limit values for fertiliser products.
A total of 55 pharmaceuticals and hormones were analysed and total of 16 drugs, especially anti-inflammatory drugs, were found in the urine (Table 6). However, none of the analysed extractable pharmaceuticals were found in soil or barley grains at the end of the growing season.
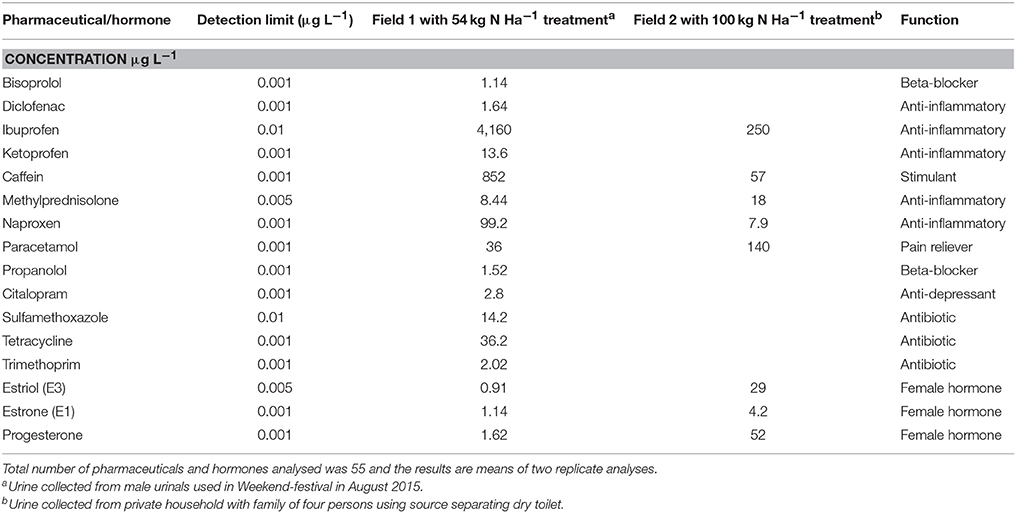
Table 6. Concentrations of pharmaceuticals and hormones found in urine samples used in different field experiments.
All extractable hormone concentrations, except progesterone, were below the detection limits of the analysis methods both in the soil and grain sample. Exactly the same amount of progesterone was found from every grain sample, 3 μg kg−1 DM, regardless of the fertiliser treatment. It was calculated that 3.1 μg and 195 μg progesterone per m2 were spread into the test fields 1 and 2 respectively. The detection limit for progesterone was 1 μg kg−1 DM.
Barley Growth, Yield, and Quality
The differences in the barley growth with the different fertiliser treatments are shown in Figure 2. It demonstrates the late phase of the growing season in the test fields at the end of July 2016. Based on the harvest, urine was found to be as efficient as a mineral fertiliser. The yield with urine was markedly higher compared with the yield without fertilisation (Figure 2). The barley yield of variety Wolmari in the 54 kg N ha−1 treatment was on average 6,200 kg ha−1 with urine fertiliser, 6,800 kg ha−1 with mineral fertiliser and 4,500 kg ha−1 for the non-fertilised treatment. With the variety Harbinger in the 100 kg N ha−1 treatment, the yield was 7,600, 7,200, and 4,400 kg ha−1 respectively. For straw yield, the trend was exactly the same as with the grain yield with variety Wolmari. With variety Harbinger the straw yield was higher in mineral fertilisation than urine fertilisation (Figure 3). At fertiliser rate 54 kg N ha−1, the straw yields were on average 2,800, 3,300, and 1,800 kg ha−1 in the urine fertilised site, mineral fertilised site and in non-fertilised site. At fertiliser rate 100 kg N ha−1, the straw yields were 3,900, 4,900, and 2,400 kg ha−1, respectively.
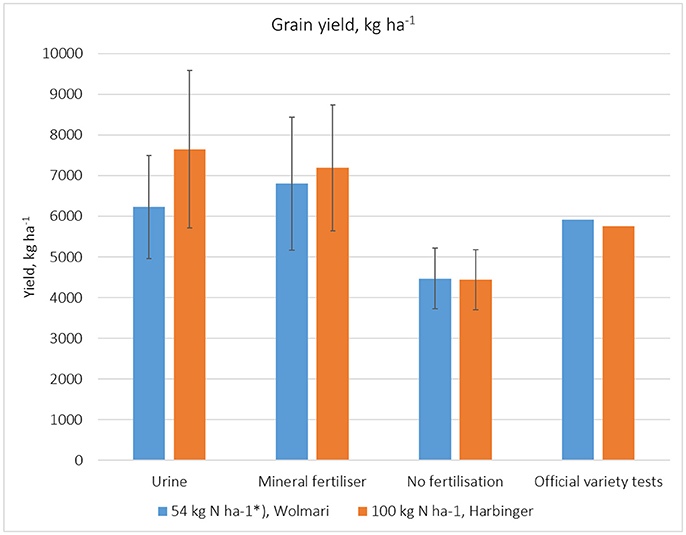
Figure 2. Total grain yield (±SD) of barley (Hordeum vulgare, var. Wolmari and Harbinger) (kg ha−1) with urine, mineral fertiliser and non-fertilised treatments. N = 30 *Samples located in clay soil were not included in the analyses, N = 21.
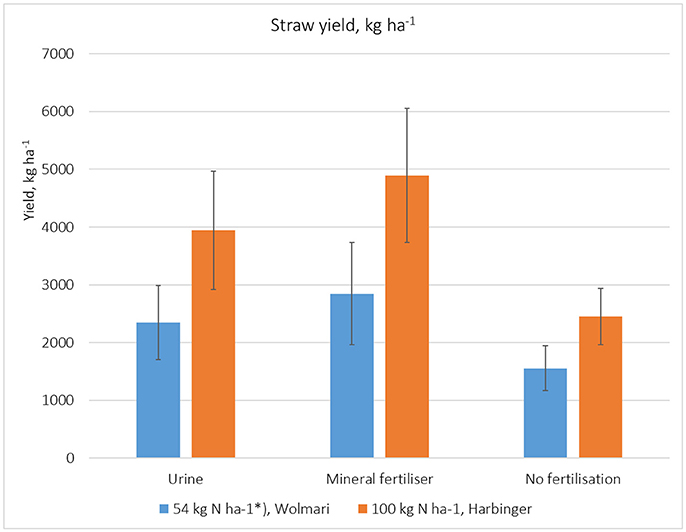
Figure 3. Total straw yield of barley (±SD) (Hordeum vulgare, var. Wolmari and Harbinger) (kg ha−1) with urine, mineral fertiliser and non-fertilised treatments. N = 30. *Samples located in clay soil were not included in the analyses, N = 21.
Thousand-grain weight (TGW) is an indicator of the size of the grain (e.g., Škarpa, 2006). It is a commonly used parameter in describing the quality of yield and varies typically between 20 and 40 g. In Figure 4, the results of TGW in the different fertiliser treatments are presented. In the lower nitrogen fertilisation (54 kg N ha−1) TGW of fertilised barley was higher than non-fertilised barley, but in the higher nitrogen fertilisation (100 kg N ha−1) it was the opposite (Figure 4).
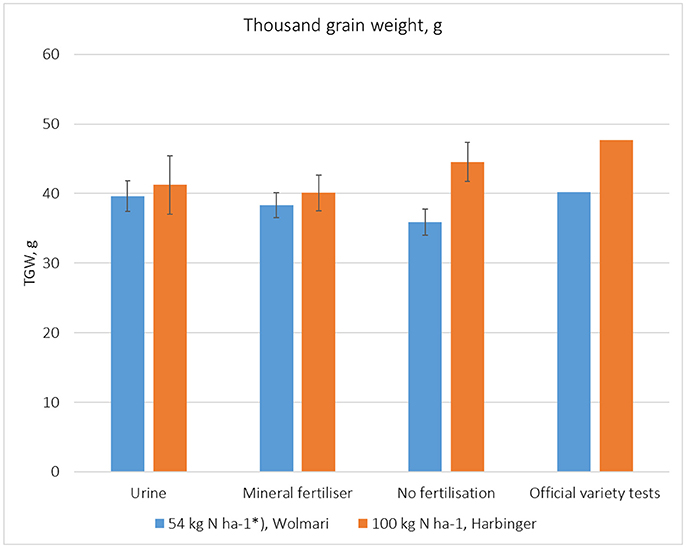
Figure 4. Thousand grain weight (TGW) of barley (±SD) (Hordeum vulgare, var. Wolmari and Harbinger) (kg ha−1) with urine, mineral fertiliser and non-fertilised treatments. N = 30 *Samples located in clay soil were not included in of analyses, N = 21.
Fertiliser treatment had no effect on the protein content or germination of the grain at either fertiliser rate or varieties used (Figures 5, 6). The total protein content of the grains with variety Wolmari was 96–98 g kg−1 in different treatments, i.e., clearly lower than expected, also reflecting the lower fertilisation level. With variety Harbinger the total protein content in this study was 100–105 g kg−1 in different fertiliser treatments. Both results were lower than the results from official variety tests. For the variety Wolmari, the expected protein content of the grain was 119 g kg−1 and for Harbinger 117 g kg−1 (Laine et al., 2016) (Figure 5).
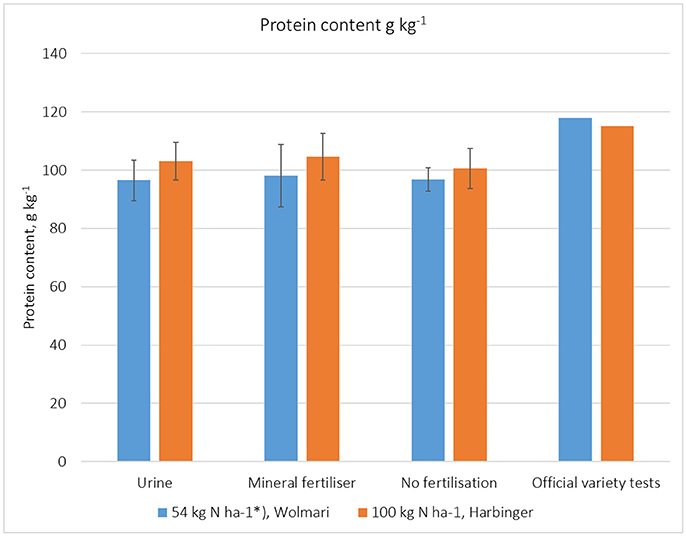
Figure 5. Total protein content of barley (±SD) (Hordeum vulgare, var. Wolmari and Harbinger) (kg ha−1) with urine, mineral fertiliser and non-fertilised treatments. N = 30 *Samples located in clay soil were not included in the analyses, N = 21.
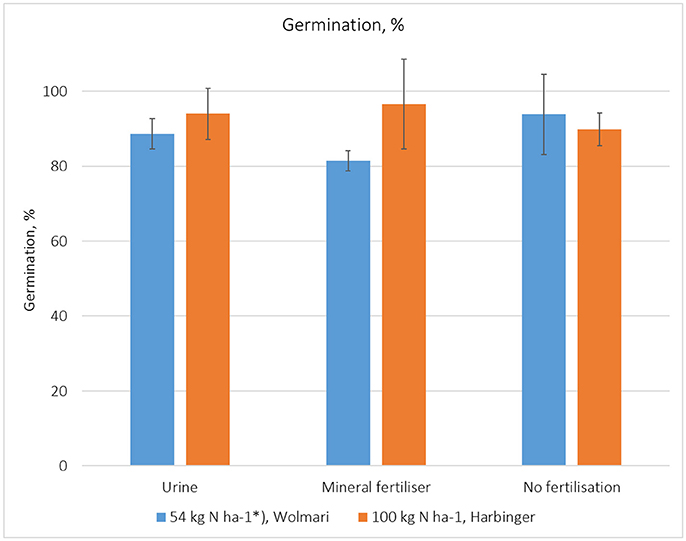
Figure 6. Germination of barley (±SD) (Hordeum vulgare, var. Wolmari and Harbinger) (kg ha−1) with urine, mineral fertiliser and non-fertilised treatments. N = 30. *Samples located in clay soil were not included in the analyses, N = 21.
At both fertiliser rates and treatments, the Zadoks growth stage (Boys and Geary, 2015) of barley was slightly slower in the non-fertilised sampling plots compared with the urine- and mineral-fertilised plots. In field 1 (54 kg N ha−1) with variety Wolmari the growth without fertiliser was clearly slower at first, but at the ripening stage the barley reached the same growth stage as the barley with urine and mineral fertiliser treatment. In field 2 (100 kg N ha−1) with higher nitrogen levels, the growth stage of barley without fertiliser was slightly behind compared with the fertilised treatments throughout the whole growing season (Figures 7, 8).
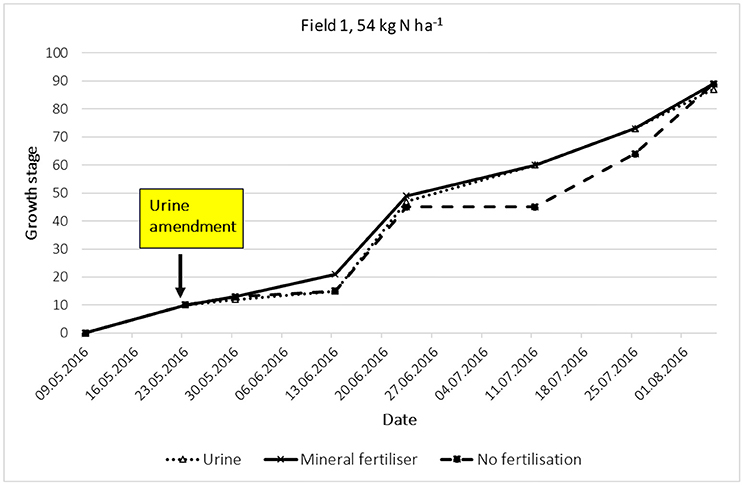
Figure 7. Zadoks' growth stage (Boys and Geary, 2015) of barley (Hordeum vulgare, var. Wolmari) in Field 1, 54 kg N ha−1 treatment during the growing season 2016.
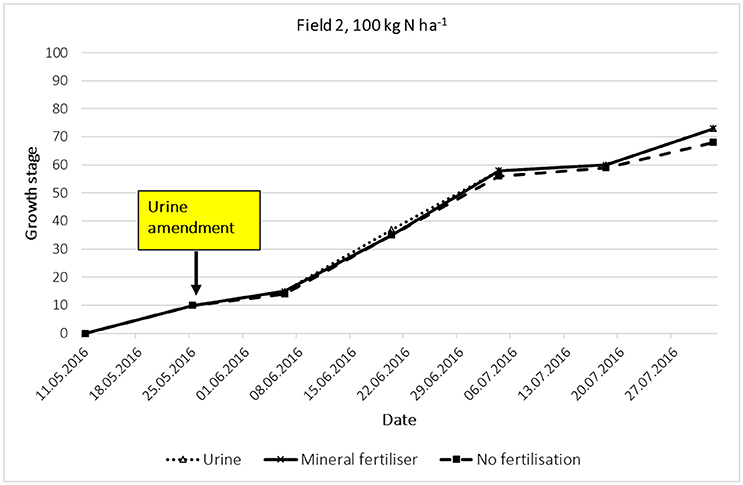
Figure 8. Zadoks' growth stage (Boys and Geary, 2015) of barley (Hordeum vulgare, var. Harbinger) in Field 2, 100 kg N ha−1 treatment during the growing season 2016.
Discussion
Urine Quality and Characteristics
The urine analysis results of this study show that source separated urine fulfils the criteria of the fertiliser products according to the Finnish legislation (Ministry of Agriculture and Forestry Finland, 2011) in terms of microbiological quality (E. coli and Salmonella) and harmful metal concentrations. All studied parameters were below the limit values defined in the legislation. The results indicate that there is no increased heavy metal input from the use of urine. This is in accordance with earlier findings of Kirchmann et al. (2017) and EEA (2018), which state that the overall heavy metal exposure in the environment has decreased remarkably during the past decades and therefore, the concentrations in wastewaters have also decreased. The results supported also the findings and recommendations in the WHO guidelines (2006).
There is, however, variation in the nutrient concentration depending on the source and the way of collecting the urine. For example, nitrogen concentration of urine can vary from 1 to 9 mg L−1, depending on the diet and time of collection (Pradhan, 2010). Based on the nutrient content and the fertiliser efficiency source separated urine meets the criteria for fertiliser products that can be used as such as soil improvers (Ministry of Agriculture and Forestry Finland, 2011). Therefore, in principle, there are no restraints from the quality point of view, to accept source separated urine as a fertiliser, as long as it is correctly stored and managed (WHO, 2006).
Urine contains significant amounts of salts, like sulphate and chloride. In this study, the sulphate concentration was on average about 500 mg L−1 and chloride concentration about 1,000 mg L−1. In long-term fertiliser use, high salt concentrations could be a risk in terms of soil salinization and therefore needs further investigation and long-term field trials.
When urine is stored according to WHO guidelines (2006), the pH of urine increases to a level of between 9 and 10. In this process, urea is hydrolysed to ammonia. Ammonia and other substances in urine are causing an unpleasant odour. Therefore, storage in sealed containers is very important. When using the urine as fertiliser, deep injection is crucial to prevent nitrogen losses into the atmosphere. This also helps preventing odours spreading to the environment. In this study odour was detected for only a few minutes after injection to the soil. According to the studies by Johansson, 2000), there is on average about 2–10% atmospheric loss of nitrogen from the field, when urine fertiliser is applied using band spread with trailing hoses. The variation in nitrogen losses to the atmosphere can be high, however, from a few per cent up to 30–40%.
Barley Growth Stages, Yield, and Quality
In the long term field trials made in Denmark (Magid et al., 2007), nitrogen uptake and mineral fertiliser equivalent (MFE) of urine fertilisation to cereal crops, like barley, oats and wheat, was found to be very good and in some years even a better fertiliser than NPK-fertiliser or cattle slurry. Cattle slurry as well as mineral NPK-fertilisers are widely used and accepted fertilisers, while source separated urine is not. Based on the trials urine was found to be a “very reliable fertiliser” (Magid et al., 2007). In this study, results are limited to only one growing season, which is not enough to make long term conclusions. The results indicate, however, a trend, which has been confirmed in other long term cultivation experiments (e.g., Magid et al., 2007).
The barley yield results showed that barley grown with urine fertiliser was equivalent to the mineral fertilised barley. Total grain yield was at the same level with both varieties (Wolmari and Harbinger) and nitrogen fertiliser rates (54 and 100 kg N ha−1). Yields were approximately 60 and 70% higher than the unfertilised treatment for the higher and lower fertiliser application rates, respectively. The trend was exactly same with straw yield with variety Wolmari, where the straw yield was the same in fertilised treatments. With variety Harbinger, straw yield in mineral fertilisation was higher than urine fertilisation. These results are in accordance with the previous findings with grain crops like barley indicating the efficiency of urine as fertiliser (Kirchmann and Pettersson, 1995; Johansson, 2000). Factors affecting the significant differences in both grain and straw yield in different fields are the different fertilisation rates, different variety and slight differences in the soil type. There were no significant differences in the weather conditions in the different fields, since they were located about 50 km apart.
In Figures 2, 4, 5 the official variety test results, which are regularly implemented by Natural Resources Institute Finland, are indicated for comparison to the results of this study (Laine et al., 2016). The total grain yield was clearly higher than the yield indicated in the official variety tests. This might be due to the extrapolation from the small sampling plots to hectare, which magnifies any minor errors in the accuracy of the harvesting. The differences between the treatments, which was the focus in this study, however, were statistically significant. They indicate that urine as a fertiliser is as efficient as mineral fertiliser. The thousand-grain weight (TWG) and the protein content of barley were on the same order of magnitude as the official tests indicated (Figures 4, 5). There was also variation in the results of TGW. The lower TGW in 100 kg N ha−1 fertilised treatments compared to the non-fertilised treatment might indicate the enhanced growth of total biomass of grain and straw, leaving the grain smaller in size. In the lower nitrogen fertilisation 54 kg N ha−1 in turn the grains were bigger in both fertilisation treatments compared with the non-fertilised plot, which could indicate allocation of available nitrogen to the growth of the grain.
In addition to the protein content of the grain, the quality of barley yield was also measured by analysing the germination of the seeds. The protein content of variety Wolmari was 9.7–9.8% in all the different fertiliser treatments. This is about 83% of the official variety test results. This could be due to low nitrogen fertilisation level (54 kg N ha−1) in the test field. With variety Harbinger the total protein content varied between 10 and 10.5% in all the treatments, which is about 91% of the official variety test results. Fertilisation treatment had no effect on the total protein content of barley in either of the fertilisation levels or barley varieties, not even with non-fertilised treatment. Especially with the variety Wolmari, low protein content might indicate the use of soil nitrogen in the growth phase, leaving less nitrogen to the formation of protein in the grain. There was also no difference in the germination (90–97%) between the different fertiliser treatments and non-fertilised treatment. Previous studies indicate that that nitrogen fertilisation or nitrogen concentration of grain does not affect the germination rate of barley (Ellis and Marshall, 1998).
The fertiliser efficiency of urine depends greatly on the environmental conditions, such as soil type and weather conditions during the growing season. In the experiments made in Sweden in 1996–1998, urine as a barley fertiliser was found almost equally efficient compared to the commercial fertilisers used in the study (Johansson, 2000). The barley yield was 80–90% of that for the commercial fertilisers. Slightly lower total yield with urine fertilisation was explained by the nitrogen losses to the atmosphere. These losses were 2–10% depending on the year and fertiliser amount used. Urine can be spread without any dilution to cereal crops, which was also done in this study. It is highly recommended to use slurry tankers equipped with either disk or hose injectors to avoid nitrogen losses (Johansson, 2000).
Pharmaceuticals and Hormones in Urine, Soil, and Yield
Substances that have been orally digested and metabolised are excreted mostly via urine. To some extent, also substances exposed through skin exposure or inhalation are excreted via urine. The substances remain partly unmetabolised. About two thirds of all unmetabolised pharmaceuticals and drugs used are excreted via urine and about one third via faeces (Lienert et al., 2007).
Several different pharmaceuticals and hormones were found in the urine samples. In the urine collected from the festival, in total 16 pharmaceuticals and hormones were found. From the urine collected from the private household, only eight different pharmaceuticals and hormones were found. The range of different pharmaceuticals was greater in the urine collected from the festival. This is obvious, because the number of people using different medication is greater. The groups of pharmaceuticals with the highest concentrations in the urine were anti-inflammatory drugs, like ibuprofen, ketoprofen and naproxen, and pain relievers like paracetamol. Other pharmaceutical groups found were antibiotics (sulfamethoxazole, tetracycline, trimetoprim), allergy drugs (methylprednisolone), beta-blockers (bisoprolol, propanolol), anti-depressants (citalopram) and caffeine. The single largest amount of pharmaceutical found was ibuprofen, the concentration being 4,160 μg L−1 in the urine collected from the festival. The urine also contained on average 852 μg L−1 of caffeine, which was included in the pharmaceuticals analysed.
Pharmaceuticals in urine can be of concern if any accumulation or other disturbance in soil and plant growth takes place. Previous research studies have shown that plants can uptake via roots certain persistent pharmaceuticals from soils, such as carbamazepine. These pharmaceuticals can accumulate in the roots and foliage of the plants (Winker et al., 2010; Bartha, 2012; Carter et al., 2014), but the amounts have been so small that it has not been considered as a health risk (Winker et al., 2010). Degradation of certain pharmaceuticals have been studied. Many pharmaceuticals, especially antibiotics, are both biodegradable (Winker et al., 2009) and photodegradable (Doll and Frimmel, 2003). For example anti-inflammatory drug naproxen (Topp et al., 2008), and antibiotics triclosane and triclocarbane (Prosser et al., 2014) degrade in soil almost completely and do not accumulate to plants or disturb their growth. Furthermore, several pharmaceuticals have, in fact, been found to degrade quite rapidly (Carter et al., 2014; Song and Guo, 2014), but there are groups of pharmaceuticals which are more persistent in soils. These are for example carbamazepine, diphenhydramine and fluoxetine, which might accumulate and cause risks to the soil environment (Wu et al., 2010). Antibiotics in soils are of special concern, because in the long run, they might cause increased resistance to antibiotics in soil microbes.
The accumulation of pharmaceuticals to plants depends on the characteristics of the substance, especially the biodegradability and adsorption, but also soil characteristics, such as organic matter content and pH (Jjemba, 2002; Song and Guo, 2014). Our knowledge on the fate and degradation of pharmaceuticals in agricultural soils is still limited, especially in the Finnish context.
Since there was no extractable progesterone found in the soil at the end of the growing season, and the concentrations in urine varied greatly in different fields, it is more likely that the question is about the plants' own formation of progesterone. In the literature, there are indications that plants can form progesterone also naturally (Janeczko, 2012; Janeczko et al., 2013). The significance of endogenic progesterone to the plants, however, is unknown.
At the end of the growing season, two replicate mixed soil and barley grain samples were taken from both fields. The traces of extractable pharmaceuticals and hormones was analysed. Apart from the progesterone traces found in the grain (3 μg kg−1 DM), the concentrations of all extractable pharmaceuticals and hormones remained below the detection limit. This suggests that pharmaceuticals are likely to degrade during the growing season and do not accumulate in the barley, or the concentrations have been below the detection limit of the analysis method. Therefore, there seems to be no risk with pharmaceutical accumulation, which is supported by earlier findings (Topp et al., 2008; Carter et al., 2014; Prosser et al., 2014; Song and Guo, 2014). Our findings, however, are suggestive. There are no long-term trials about the potential accumulation of pharmaceuticals and there might be also other micropollutants, such as microplastics, pesticides etc., to which we are also exposed and which might be excreted via urine. In terms of progesterone, it is possible that there is endogenic production of it in cereal crops. According to Janeczko (2012), plants can produce progesterone without external accumulation and endogenic progesterone production has been found for example from wheat (Janeczko et al., 2013).
Pharmaceuticals in urine can be a limiting factor for the fertiliser use, if they are found in large amounts. Most of the pharmaceuticals do not degrade during storage (Schürmann et al., 2012). Many pharmaceuticals in urine do not accumulate in struvite, which can be precipitated from urine (Schürmann et al., 2012; Kemacheevakul et al., 2014). Also zeolite treatment can be a promising technology in the removal of antibiotics from wastewaters (Malakootian et al., 2016). There are some pharmaceuticals, however, like tetracycline-antibiotics that accumulate particularly in struvite (Kemacheevakul et al., 2012). In this study, tetracycline was also found in urine, the concentration being about 36 μg L−1. Tetracycline is one of the antibiotics that are used in treating farm animals and therefore can also be found in soils where manure is used as a fertiliser (Brambilla et al., 2007). Since the biodegradability and fate of different pharmaceuticals vary, there is a need for more research for example of risks of developing antibiotic resistance and disturbance in soil microbiological processes.
Among the public, there are strong opinions for and against the use of urine as a fertiliser. The environmental risks in terms of pharmaceutical accumulation or harmful heavy metal exposure to soil or crops could not be indicated in this study. Furthermore, the hygienic safety and fertiliser efficiency of urine in terms of barley yield and quality was clearly shown. No pathogen indicators were found and the barley yield was equally good compared with a mineral fertiliser and would meet the requirements of Finnish legislation. Therefore, the urine should be accepted as a fertiliser and the use of source separation and fertiliser techniques could be taken into consideration.
Author Contributions
E-LV designed and was in charge of the implementation of the experiments. She also carried out the data analysis and wrote most of the manuscript. GG, KK, and AG were assisting in the practical field work, laboratory analyses and data collection. RV and SL contributed to the data analysis, manuscript writing and discussion.
Funding
This study was financially supported by the Finnish Ministry of Environment, Programme to promote the recycling of nutrients and to improve the status of the Archipelago Sea, during years 2015-2016 (Project code: RAKI-YM167/481/2014-BIOUREA).
Conflict of Interest Statement
The authors declare that the research was conducted in the absence of any commercial or financial relationships that could be construed as a potential conflict of interest.
Acknowledgments
We wish to thank Sipilä and Mikkola farms and Global Dry Toilet Association of Finland for participation, expertise and good cooperation during the study. The linguistic corrections made by Ms. Taru Owston from Tampere University of Applied Sciences are gratefully acknowledged.
References
Bartha, B. (2012). Uptake and Metabolism of Human Pharmaceuticals in Plants. Identification of Metabolites and Specification of the Defense Enzyme Systems under Pharmaceutical Exposure. Doctoral Dissertation. Technical University of Münich, Münich. Available online at: http://nbn-resolving.de/urn/resolver.pl?urn:nbn:de:bvb:91-diss-20120718-1099038-1-4
Boys, E., and Geary, F. (2015). Barley Growth Guide. Kenilworth: Agriculture and Horticulture Development Board (AHDB).
Brambilla, G., Patrizii, M., De Filippis, S. P., Bonazzi, G., Mantovi, P., Barchi, D., et al. (2007). Oxytetracycline as environmental contaminat in arable lands. Anal. Chim. Acta 586, 326–329. doi: 10.1016/j.aca.2006.11.019
Carter, L. J., Harris, E., Williams, M., Ryan, J., Kookana, R., and Boxall, A. (2014). Fate and uptake of pharmaceuticals in soil-plant systems. J. Agric. Food Chem. 62, 816–825. doi: 10.1021/jf404282y
Chowdhury, M. H., and Islam, M. T. (2008). Study on effect of urine as fertilizer through vegetable production in Bangladesh. Asia-Pac. J. Rural Dev. XVIII, 2.
Cordell, D., Drangert, J. O., and White, S. (2009). The story of phosphorus: global food security and food for thought. Glob. Env. Change 19, 292–305. doi: 10.1016/j.gloenvcha.2008.10.009
Cordell, D., Rosemarin, A., Schröder, J. J., and Smit, A. L. (2011). Towards global phosphorus security: a systems framework for phosphorus recovery and reuse options. Chemosphere 84, 747–758. doi: 10.1016/j.chemosphere.2011.02.032
Doll, T. E., and Frimmel, F. H. (2003). Fate of pharmaceuticals - photodegradation by simulated solar UV-light. Chemosphere 52, 1757–1769. doi: 10.1016/S0045-6535(03)00446-6
EEA (2018). Environmental Pressures of Heavy Metal Releases from Europe's Industry. European Environment Agency. Briefing no. 3/2018. doi: 10.2800/501322
Ellis, R. P., and Marshall, B. (1998). Growth, yield and grain quality of barley (Hordeum vulgare L.) in response to nitrogen uptake. II. Plant development and rate of germination. J. Exp. Bot. 49, 1021–1029.
Genuis, S. J., Birkholz, D., Rodushkin, I., and Beesoon, S. (2011). Blood, Urine, and Sweat (BUS) study: monitoring and elimination of bioaccumulated toxic elements. Arch. Environ. Contam. Toxicol. 61, 344–357. doi: 10.1007/s00244-010-9611-5
Heinonen-Tanski, H., Sjöblom, A., Fabritius, H., and Karinen, P. (2007). Pure human urine is a good fertilizer for cucumbers. Biores Tech. 8, 214–217. doi: 10.1016/j.biortech.2005.11.024
Höglund, C. (2001). Evaluation of the Microbial Health Risks Associated with the Reuse of Source-Separated Human Urine. Ph.D. thesis, Stockholm, KTH.
Höglund, C., Stenström, T. A., Jönsson, H., and Sundin, A. (1998). Evaluation of faecal contamination and microbial die-off in urine separating systems. Water Sci. Tech. 38, 17–25. doi: 10.2166/wst.1998.0232
Ikkala, J. (2001). Correctly Implemented Germination Test (for Barley). Available online at: https://www.farmit.net/node/159780 (in Finnish).
Janeczko, A. (2012). The presence and activity of progesterone in the plant kingdom. Steroids 77, 169–173. doi: 10.1016/j.steroids.2011.10.012
Janeczko, A., Okleštková, J., Siwek, A., Dziurka, M., Pociecha, E., Kocurek, M., et al. (2013). Endogenous progesterone and its cellular binding sites in wheat exposed to drought stress. J. Steroid Biochem. Mol. Biol. 138, 384–394. doi: 10.1016/j.jsbmb.2013.07.014
Jjemba, P. (2002). The potential impact of veterinary and human terapeutic agents in manure and biosolids on plants grown on arable land: a review. Agric. Ecosyst. Environ. 93, 267–278. doi: 10.1016/S0167-8809(01)00350-4
Johansson, M. (2000). Urine Separation – Closing the Nutrient Cycle. Final report on the R&D project: source separated human urine – a future source of fertilizer for agriculture in the Stockholm region. Stockholm: Stockholm Vatten, Stockholmshem & HSB National Federation.
Jönssön, H., Stinzing, A., Vinnerås, B., and Salomon, E. (2004). Guidelines on the Use of Urine and Feces on Crop Production. Stockholm: Stockholm Environment Institute.
Kemacheevakul, P., Chuangchote, S., Otani, S., Matsuda, T., and Shimizu, Y. (2014). Phosphorus recovery: minimization of amount of pharmaceuticals and improvement of purity in struvite recovered from hydrolysed urine. Environ. Technol. 35, 3011–3019. doi: 10.1080/09593330.2014.929179
Kemacheevakul, P., Otani, S., Matsuda, T., and Shimizu, Y. (2012). Occurrence of micro-organic pollutants on phosphorus recovery from urine. Wat. Sci. Technol. 66, 2194–2201. doi: 10.2166/wst.2012.452
Kirchmann, H., Börjesson, G., Kätterer, T., and Cohen, Y. (2017). From agricultural use of sewage sludge to nutrient extraction: a soil science outlook. Ambio 46, 143–154. doi: 10.1007/s13280-016-0816-3
Kirchmann, H., and Pettersson, S. (1995). Human urine chemical composition and fertilizer use efficiency. Fertil. Res. 40, 149–154. doi: 10.1007/BF00750100
Laine, A., Högnäsbacka, M., Kujala, M., Niskanen, M., Jauhiainen, L., and Nikander, H. (2016). Virallisten Lajikekokeiden Tulokset 2008-2015. Helsinki: Natural Resources Institute Finland. Available online at: http://jukuri.luke.fi/bitstream/handle/10024/531720/luke-luobio_3_2016.pdf (In Finnish).
Lapid, D. (2008). Ecological Sanitation: A Hope for a Sustainable Future. Available online at: http://www.irbnet.de/daten/iconda/CIB_DC26761.pdf
Lentner, C. (1981). Units of Measurement, Body Fluids, Composition of the Body, Nutrition, 8th Edn. West Caldwell, NJ: Division of Medical Education, Ciba-Geigy Corp.
Lienert, J., Bürki, T., and Escher, B. (2007). Reducing micropollutants with source control: substance flow analysis of 212 pharmaceuticals in feces and urine. Wat. Sci. Tech. 56, 87–96. doi: 10.2166/wst.2007.560
Lienert, J., and Larsen, T. A. (2010). High acceptance of urine source separation in seven European countries: a review. Environ. Sci. Technol. 44, 556–566. doi: 10.1021/es9028765
Magid, J., Eilersen, A. M., Wrisberg, S., and Henze, M. (2006). Possibilities barriers for recirculation of nutrients organic matter from urban to rural areas: a technical theoretical framework applied to the medium-sized town Hillerød, Denmark. Ecol. Eng. 28, 44–54. doi: 10.1016/j.ecoleng.2006.03.009
Magid, J., Luxhøi, J., Jensen, L. S., Møller, J., and Bruun, S. (2007). “Establishment of a long-term field trial with urban fertilizers – is recycling of nutrients from urban areas to peri-urban organic farms feasible?” in Long-term Field Experiments in Organic Farming, Edited by 2006. International Society of Organic Agriculture Research (ISOFAR), ISOFAR Scientific Series 1, eds J. Raupp, C. Pekrun, M. Oltmanns, and U. Köpke (Berlin: Verlag Dr Köster), 204.
Malakootian, M., Nori Sepehr, M., Bahraini, S., and Zarrabi, M. (2016). Capacity of natural and modified zeolite with cationic surfactant in removal of antibiotic tetracycline from aqueous solutions. Koomesh 17, 779–788.
Ministry of Agriculture and Forestry Finland (2011). Decree of the Ministry of Agriculture and Forestry on Fertilizer Products. Helsinki, 24/11.
Ministry of the Environment Finland (2017). Government Decree on Treating Domestic Wastewater in Areas Outside Sewer Networks. Helsinki, 157/2017.
Mnkeni, P. N., Kutu, F. R., Muchaonyerwa, P., and Austin, L. M. (2007). Evaluation of human urine as a source of nutrients for selected vegetables and maize under tunnel house conditions in the Eastern Cape, South Africa. Waste Manag. Res. 26, 132–139. doi: 10.1177/0734242X07079179
Pradhan, S. (2010). Yield and Quality of Vegetables Fertilized with Human Urine and Wood ash. Faculty of Science and Forestry/Department of Environmental Sciences. Dissertation, publications of the University of Eastern Finland. Dissertations in Forestry and Natural Sciences, no 6.
Pradhan, S., Nerg, A., Sjöblom, A., Holopainen, J. K., and Heinonen-Tanski, H. (2007). Use of human urine as fertilizer in cultivation of cabbage (Brassica oleracea). Impacts on chemical, microbial and flavor quality. J Agric. Food Chem. 55, 8657–8663. doi: 10.1021/jf0717891
Prosser, R. S., Lissemore, L., Solomon, K., and Sibley, P. (2014). Toxicity of biosolids-derived triclosan and triclocarban to six crop species. Environ. Toxicol Chem. 33, 1840–1848. doi: 10.1002/etc.2624
Richert Stintzing, A., Rodhe, L., and Åkerhielm, H. (2001). Humanurin Som Gödselmedel I Växtnäring, Spridningsteknik Och Miljöeffekter. JTI-report Lantbruk & Industri 278. Uppsala: Swedish Institute of Agricultural Engineering (JTI).
Rose, C., Parker, A., Jefferson, B., and Cartmell, E. (2015). The characterization of feces and urine: a review of the literature to inform advance treatment technology. Crit. Rev. Environ. Sci. Technol. 45, 1827–1879. doi: 10.1080/10643389.2014.1000761
Schönning, C. (2006). Urine Diversion – Hygienic Risks and Microbial Guidelines for Reuse. Report Background paper for Guidelines for the safe use of wastewater, excreta and greywater of WHO, WHO, Solna, Sweden.
Schouw, N. L., Danteravanich, S., Mosbaeck, H., and Tjell, J. C. (2002). Composition of human excreta—a case study from Southern Thailand. Sci. Tot. Environ. 286, 155–166. doi: 10.1016/S0048-9697(01)00973-1
Schürmann, B., Everding, W., Montag, D., and Pinnekamp, J. (2012). Fate of pharmaceuticals and bacteria in stored urine during precipitation and drying of struvite. Water Sci. Technol. 65, 1774–1780. doi: 10.2166/wst.2012.041
Škarpa, P. (2006). Effect of soil nutrients reserves and level of fertilisation on production parameters of spring barley (Hordeum vulgare L.) Acta Univ. Agric. Silvic. Mendelianae Brun. 54, 91–98. doi: 10.11118/actaun200654040091
Song, W., and Guo, M. (2014). “Residual veterinary pharmaceuticals in animal manures and their environmental behaviors in soils,” in Applied Manure and Nutrient Chemistry for Sustainable Agriculture and Environment, eds Z. He and H. Zhang (Dordrecht: Springer Science + Business Media), 23–52.
Topp, E., Hendel, J., Lapen, D., and Chapman, R. (2008). Fate of the nonsteroidal anti-inflammatory drug naproxen in agricultural soil receiving liquid municipal biosolids. Environ. Toxicol Chem. 27, 2005–2010. doi: 10.1897/07-644.1
Udert, K. M., Larsen, T. A., and Gujer, W. (2006). Fate of major compounds in source-separated urine. Wat. Sci. Tech. 54, 413–420. doi: 10.2166/wst.2006.921
Vinnerås, B., Holmquist, A., Bagge, E., Albihn, A., and Jönsson, H. (2003). The potential for disinfection of separated feacal matter by urea and by peracetic acid for hygienic nutrient recycling. Biores. Tech. 89, 155–161. doi: 10.1016/S0960-8524(03)00044-0
Vinnerås, B., and Jönsson, H. (2002). The performance and potential of faecal separation and urine diversion to recycle plant nutrients in household wastewater. Biores. Tech. 84, 275–282. doi: 10.1016/S0960-8524(02)00054-8
Vinnerås, B., Nordin, A., Niwabaga, C., and Nyberg, K. (2008). Inactivation of bacteria and viruses in human urine depending on temperature and dilution rate. Wat. Res. 42, 4067–4074. doi: 10.1016/j.watres.2008.06.014
Viskari, E.-L., Haapamäki, S., Hamdine, S., Mwakangale, J., Pradhan, S., and Heinonen-Tanski, H. (2009). “Dry toilet compost and separated urine as fertilisers – experiences from Finland,” in Third International Dry Toilet Conference, August 12-14 2009, Extended Abstract in Proceedings (Tampere).
WHO (2006). Guidelines for the Safe Use of Wastewater, Excreta and Greywater - Volume 4. Excreta and Greywater Use in Agriculture. World Health Organisation. Available online at: http://www.who.int/water_sanitation_health/publications/gsuweg4/en/
Weckman, A. (2005). Nutrients from Toilets to Fields. Luopioinen: Guide of the Finnish Garden Association.
Winker, M., Clemens, J., Reich, M., Gulyas, H., and Otterpohl, R. (2010). Ryegrass uptake of carbamazepine and ibuprofen applied by urine fertilization. Sci. Total Environ. 408, 1902–1908. doi: 10.1016/j.scitotenv.2010.01.028
Winker, M., Vinnerås, B., Muskolus, A., Arnold, U., and Clemens, J. (2009). Fertilizer products from new sanitation systems: their potential values and risks. Biores. Technol. 100, 4090–4096. doi: 10.1016/j.biortech.2009.03.024
Keywords: urine, fertiliser, barley, source separation, pharmaceuticals, microbiological quality, yield
Citation: Viskari E-L, Grobler G, Karimäki K, Gorbatova A, Vilpas R and Lehtoranta S (2018) Nitrogen Recovery With Source Separation of Human Urine—Preliminary Results of Its Fertiliser Potential and Use in Agriculture. Front. Sustain. Food Syst. 2:32. doi: 10.3389/fsufs.2018.00032
Received: 31 January 2018; Accepted: 11 June 2018;
Published: 28 June 2018.
Edited by:
Tom Misselbrook, Rothamsted Research (BBSRC), United KingdomReviewed by:
Paolo Mantovi, Centro Ricerche Produzioni Animali, ItalyJoão Coutinho, University of Trás-os-Montes and Alto Douro, Portugal
Harald Menzi, Federal Office for the Environment, Switzerland
Copyright © 2018 Viskari, Grobler, Karimäki, Gorbatova, Vilpas and Lehtoranta. This is an open-access article distributed under the terms of the Creative Commons Attribution License (CC BY). The use, distribution or reproduction in other forums is permitted, provided the original author(s) and the copyright owner are credited and that the original publication in this journal is cited, in accordance with accepted academic practice. No use, distribution or reproduction is permitted which does not comply with these terms.
*Correspondence: Eeva-Liisa Viskari, ZWV2YS1saWlzYS52aXNrYXJpQHRhbWsuZmk=