- Department of Crop Sciences, University of Illinois, Urbana, IL, United States
To meet sustainable intensification goals in the US Midwest, strategies which maintain or increase yields while minimizing negative impacts on water quality are needed. In this study maize yield response and potential nitrogen (N) leaching losses were simultaneously quantified to test the hypothesis that N rates which produced maximum yields would result in minimum yield-scaled N leaching potential. Field experiments were conducted at two sites in 2015 and 2016 to determine the effect of N rate (0, 79, 179, 269, kg N ha−1) on yield, crop N uptake, potential N leaching losses, and post-harvest soil N concentrations. Results show that a significant yield response (up to 179 kg N ha−1) occurred in all years compared to the control. Nitrogen leaching potential increased at 269 kg N ha−1 compared to the control in 2015 but not 2016. Yield-scaled N leaching potential was not statistically different among treatments in three of four site-years. There was no improvement in crop N uptake or N recovery efficiency for 269 kg N ha−1 compared to 179 kg N ha−1 in three of four site-years, which coincided with a trend of increasing post-harvest soil N concentrations, further escalating the risk of environmental N losses. These results did not support our hypothesis that yield-scaled N leaching potential is minimized at N rates that optimize yields (on a normalized basis yield-scaled N leaching potential increased by 28% compared to the control). However, normalized data indicate that 179 kg N ha−1, the N rate most closely aligned with current recommendations in this region, resulted in 96% of maximum yield while preventing a 25% increase in yield-scaled N leaching potential compared to 269 kg N ha−1, underscoring the potential for achieving high yields while avoiding increased N leaching potential on an environmental efficiency basis.
Introduction
The US Midwest is an important global production region for maize (Zea mays L.) and soybean (Glycine max L.). However, there is increasing awareness of the economic and environmental costs associated with high-yielding crop production practices in this region, including nitrogen (N) fertilizer use (David et al., 2010; Sobota et al., 2015). Although N inputs are important for sustaining crop yields and maintaining soil quality (Poffenbarger et al., 2017), N fertilizer contributes to enhanced nitrate leaching losses (David et al., 2010). Nitrate leaching losses from agricultural fields have been identified as a leading contributor to hypoxic conditions in the Gulf of Mexico, negatively impacting freshwater quality (Mississippi River/Gulf of Mexico Watershed Nutrient Task Force, 2008). In response, Illinois and other states in the US Midwest have developed nutrient loss reduction strategies to promote in-field and edge-of-field-practices for reducing nitrate losses from agriculture. The Illinois strategy established short- and long-term nitrate loss reduction targets of 25 and 45%, respectively, compared to a 1980–1996 baseline (Illinois Nutrient Loss Reduction Strategy (IL NLRS), 2015). Despite N application rates being highlighted in several strategies, few studies have specifically assessed N rate strategies for minimizing the risk of water quality impacts via N leaching losses without negatively impacting yields.
A number of approaches have been developed to identify optimum N rates for maize production (see Morris et al., 2018 for a thorough review). In Illinois, current university recommendations for maize following soybean are based on the maximum return to N (MRTN) calculator, where the N rate resulting in the highest average return to N fertilizer is determined based on yield response data across many sites (Sawyer et al., 2006). Regional recommendations for the MRTN rate are also accompanied by a range of profitable N rates (e.g., 180–212 kg N ha−1 for maize following soybean in central Illinois). However, due to variability in soil conditions, weather, and crop management as well as potential interactions between these factors, optimum N rates can vary greatly (Morris et al., 2018). Thus agronomic research is often focused on characterizing the impacts of N rate on yield under a range of conditions, with little emphasis given to quantifying environmental losses.
It is well documented that increased N inputs correspond with greater N losses in maize systems (Jaynes et al., 2001; Helmers et al., 2012; Christianson and Harmel, 2015). Lawlor et al. (2008) synthesized experimental data and found exponentially increasing drainage N concentrations in response to increasing N rate. Yet, similar to crop yield, N losses can be highly variable due to differences in soil and environmental conditions (Eagle et al., 2017), indicating there may be benefits to simultaneously assessing these relationships within a site. For example, given the relatively high organic matter content of soils in the US Midwest, N losses in some years can be similar from unfertilized and fertilized plots due to rapid soil N mineralization rates. Precipitation is also an important factor, as reported by Christianson and Harmel (2015) who showed that the rate of N losses at higher N rates increased more rapidly in years with more than 850 mm of precipitation. Considering that variability in soil and climate factors not only drives N leaching losses but also crop productivity, the development of balanced N management strategies will depend on linking agronomic and environmental outcomes within individual field experiments to identify positive outcomes as well as potential tradeoffs.
Sustainable intensification is promoted as a means of meeting future food security goals while minimizing adverse environmental impacts (Garnett et al., 2013). Within this context, improved N fertilizer management is often emphasized due to its influence on crop productivity and economic profitability, as well as its wide-ranging effects on soil, water, and air quality (Tilman et al., 2011; Zhang et al., 2015; McLellan et al., 2018). One metric that is increasingly used to assess strategies for maintaining crop yields while minimizing N losses is the yield-scaled approach, where N losses are expressed per unit crop production.
A recent assessment integrating drainage N losses with maize yield determined that yield-scaled N loads remained low at moderate N rates, but increased rapidly when N rates exceeded crop demand (Zhao et al., 2016). Similarly, Zhou and Butterbach-Bahl (2014) identified an average optimum N rate (178 kg N ha−1) for minimizing yield-scaled nitrate leaching in maize in their global study, with this N rate producing 90% of maximum yields. Yet further research is necessary to evaluate the yield-scaled concept in the US Midwest. An important question is the degree to which N rates above MRTN recommendations may increase yield-scaled N losses without further benefiting yield. Prior field studies indicate that N rates in excess of crop demand can reduce N use efficiency and lead to elevated soil N concentrations following harvest, contributing to subsequent increases in N leaching losses (Andraski et al., 2000; Jaynes et al., 2001). At the same time, variability in environmental conditions discussed above can have differential impacts on crop yield response, optimum N rates, and potential N losses across sites (Hong et al., 2007; Eagle et al., 2017).
The knowledge gap addressed in this research is that few studies in Illinois have evaluated maize yield and N leaching potential in the same experiment to determine if N rates exceeding crop demand increase yield-scaled N losses without further benefiting yield. The novelty of the current investigation was to address the hypothesis that when N leaching potential is expressed on a yield-scaled basis, N rates which produce maximum yields will result in minimum yield-scaled N leaching losses, representing a win-win strategy for balancing crop production and environmental goals. The specific objectives of this study were to assess crop yield, N leaching potential, postharvest soil nitrate, and yield-scaled N losses along a gradient of N fertilizer inputs for maize agroecosystems.
Materials and Methods
Site Description and Experimental Design
Field trials were conducted in 2015 and 2016 at two locations: the University of Illinois Crop Science Research and Education Center (CSREC) in Urbana, Illinois (88.23°W, 40.05°N) and the Northwestern Research Station (NWRC) near Monmouth, Illinois (90.72°W, 40.93°N). These sites have similar climates with average long-term (1981–2010) annual temperatures of 10.8 and 10.0°C and precipitation of 1,008 and 1,001 mm, respectively (Illinois Climate Network, (http://www.isws.illinois.edu/warm/datatype.asp). All experimental fields utilized in this study were in a maize and soybean rotation with soybean as the previous crop, meaning trials were located in a new field each year. Soils at the Urbana field in 2015 were classified as a Raub silt loam (Fine-silty, mixed, superactive, mesic Aquic Argiudolls) with 0–2% slope. The Monmouth field in 2015 was an Osco silt loam (Fine-silty, mixed, superactive, mesic Typic Argiudolls) with 2–5% slope. In 2016, the Urbana field was a Drummer silty clay loam (Fine-silty, mixed, superactive, mesic Typic Endoaquolls) with 0–2% slope, and the Monmouth field was a Muscatune silt loam (Fine-silty, mixed, superactive, mesic Aquic Hapludolls) with 0–2% slope.
At each site, N response trials consisting of four rates (0, 90, 179, 269, kg N ha−1) were established in the spring prior to planting. This range of N rates was selected because it aligned with the typical range of rates used in previous MRTN field studies (Sawyer et al., 2006). Plots were 3 × 6 m at Urbana and 3 × 9 m at Monmouth. Each plot included four maize rows at 76 cm spacing. Nitrogen was applied as urea ammonium nitrate (UAN) solution containing 28% N immediately prior to maize planting as a broadcast solution, followed by soil incorporation. Each trial was arranged as a randomized complete block design with four replications. Both sites were managed with conventional tillage which included primary tillage using a deep chisel plow in the fall. Secondary tillage was performed in the spring with a one-pass shallow field cultivator before planting. For all experiments, Pioneer Hybrid 1221 was planted at population of 84,000 plants ha−1 except at Monmouth in 2016 which had Pioneer Hybrid 1311.
Soil N Measurements
Inorganic N leaching potential was measured using ion exchange resin lysimeters following the design and methods described in Susfalk and Johnson (2002); Langlois et al. (2003), and McIsaac et al. (2010). Lysimeters consisted of 5.1 cm diameter polyvinylchloride pipe and a coupling section for a total length of 7 cm. A layer of ion exchange resin was placed inside the lysimeter to absorb nitrate and ammonium dissolved in soil solution as water drained downward through the soil profile. Inside the lysimeter, the ion exchange resin was held in place between a layer of washed sand and a permeable nylon membrane (Nitrex Cloth 153 um, Wildco.com).
Lysimeters were installed at 50 cm soil depth prior to N application and planting each spring (Table 1). In each plot 6 lysimeters were placed under non-disturbed soil between the center crop rows (two lysimeters were placed on opposing sides of three holes set 1.5 m apart). Previous work in Illinois has demonstrated the utility of this method for estimating movement of N below this soil depth (McIsaac et al., 2010; Behnke et al., 2012). Lysimeters were removed following crop harvest (Table 1) and N absorbed on the resin was extracted using 2 M KCl. Nitrate and ammonium concentrations were determined colorimetrically with a Smartchem 170 discrete wet chemistry auto-analyzer (Unity Scientific).
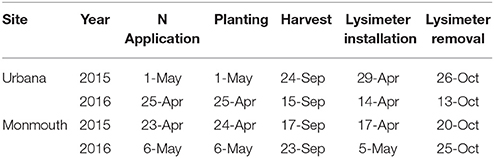
Table 1. Dates for crop management, N application, and lysimeter installation and removal at Monmouth and Urbana each year.
Following maize harvest each fall, deep soil cores were collected from all plots to evaluate the potential for subsequent N leaching. Three cores (2.8 cm diameter) were collected per plot to a depth of 90 cm using a tractor mounted hydraulic probe (Giddings Machine Company). Cores were divided into 0–30, 30–60, 60–90 cm depths and composited. Samples were placed on ice until laboratory analysis. Soil ammonium and nitrate were extracted from field moist soil using 2 M KCl. Extracts were filtered and analyzed for nitrate and ammonium colorimetrically as described above.
Crop Yield and N Uptake
At harvest, maize ears were collected from the center two rows of each plot in an area 1.5 × 3 m long. Maize grain was shelled from ears using an Almaco ECS. Total grain weight and moisture were recorded to calculate yield. Yields were adjusted to 15% moisture. To determine maize grain and biomass N concentrations and total crop N uptake, 6 whole maize plant samples were obtained from each plot at physiological maturity. Plants were cut at ground level from the center two rows and ears were separated from biomass. Biomass was shredded in a wood chipper and subsamples were dried at 60°C to a constant weight before grinding with a Wiley mill (Arthur H. Thomas Co., Philadelphia, PA) to pass through a 2-mm sieve. Maize grain weight and moisture were recorded (Dickey-John meter model GAC2100). Analysis of grain and biomass total N concentration was performed via combustion on an elemental analyzer by Brookside Labs (New Bremen, OH). Cob N uptake was estimated as 4.8% of total N uptake in plant and grain. Following Ciampitti and Vyn (2012), N recovery efficiency (NRE) was calculated as the increase in crop N uptake compared to the control, divided by N application rate (kg N ha−1).
Data Analysis
Analysis of variance was performed using linear mixed effects models and the restricted maximum likelihood method in the nlme package in R (version 3.1.1, Pinheiro et al., 2014; R Core Team, 2014). Response variables for each site were modeled with treatment designated as a fixed effect and block as a random effect. When necessary, data were log-transformed to meet assumptions. Significant differences between treatments were determined based on Tukey's pairwise comparisons (P < 0.05) using the multcomp package in R (Hothorn et al., 2008). For the analysis of post-harvest soil N concentrations, sample depth was included as nested variable. Treatment means for post-harvest soil N concentrations are reported by depth due to a significant treatment by depth interaction. Where appropriate, means are presented as de-transformed values.
To reduce between-site variability when interpreting overall trends, normalized values were calculated for each site-year following Zhou and Butterbach-Bahl (2014). Individual observations for yield, N leaching potential, and yield-scaled N leaching potential were expressed on a relative basis (i.e., ranging from 0 to 1) as a fraction of the highest mean observed in each site-year.
Results and Discussion
Weather and Crop Yield
In 2015, weather conditions were dry during the early spring period at both locations followed by wetter growing season conditions. Urbana received 17.2 cm above average rainfall during May and June of 2015. Monmouth had 22.7 cm rainfall above average during June and July in 2015. In 2016 relatively wet conditions occurred at both locations during the growing season. Urbana had 7.1 cm above average rainfall in June and Monmouth had 14.7 cm above average rainfall (cumulative) during June, July, and August.
For crop yield, a significant response to N fertilization occurred in all years (Table 2). While 179 kg N ha−1 was always greater than the control, no significant yield increase was observed when increasing from 179 to 269 kg N ha−1. As noted by Morris et al. (2018), applying the correct N rate to maximize returns for maize remains a challenge, as optimum N rate can vary greatly due to the complex interactions between soil conditions, N loss potential, weather, and crop management at various spatial and temporal scales. In previous work, Pittelkow et al. (2017) reported no significant yield increase moving from 156 to 234 kg N ha−1 over a 3 year study, with their range of N rate treatments being lower than our study but the relative increase between N rates being the same (50%). The fact that yields did not further increase at 269 kg N ha−1 is in agreement with the range of profitable MRTN rates recommended for maize following soybean in this region (180–212 kg N ha−1; http://cnrc.agron.iastate.edu/). However, MRTN is a regional average, thus by definition a wider range of yield response would be expected if a larger population of sites were included, with some sites requiring less N and others requiring more N (Morris et al., 2018). During a 16 year study for maize-maize and maize-soybean rotations in Iowa, Puntel et al. (2016) showed that higher optimum N rates (i.e., where a positive yield response was found at N rates above those comparable to MRTN) occurred in years with above normal spring precipitation. These authors used models to demonstrate that high April–June cumulative precipitation reduced soil organic matter mineralization and contributed to exponential increases in N losses (denitrification and leaching), thereby reducing soil N supply.
The fact that application of 269 kg N ha−1 did not further increase yield is important for farmers in the US Midwest searching for opportunities to generate additional economic returns. The current state of economics in this region is dominated by low crop prices, which can result in negative budgets if operating and overhead prices are too high and/or yield levels are too low. Under these conditions farmers may feel increased pressure to raise yields, thus an increasingly common question is whether additional N (beyond MRTN) will generate additional economic returns. Continued growth over previous decades in yield trends has also raised the question of whether increased crop nutrient inputs are necessary. Finally, the introduction of digital decision support tools from the private sector has contributed to the concern that regional MRTN rates may not be sufficient, as a primary focus of these tools is to ensure yield potential is not limited by soil N supply.
N Leaching Potential
Results show that 269 kg N ha−1 significantly increased leaching potential compared to the control in 2015 at both sites (Table 3). However, application of N fertilizer did not significantly increase N leaching potential at either site in 2016. In the context of sustainable intensification, potential yield gains related to N rate must be weighed against potential N losses if progress is to be made in meeting goals outlined in various state-level nutrient loss strategies. An important finding is that in both years of our study, yields were significantly increased with 179 kg N ha−1 without significant increases in N leaching potential. Our work aligns with other recent studies (Puntel et al., 2016; Eagle et al., 2017; Sela et al., 2017) highlighting the need for approaches to link crop productivity and environmental N losses to assess the consequences of applying N fertilizer at rates beyond current MRTN recommendations.
The majority of previous research in the US Midwest has shown a positive response of N leaching losses to increasing N rate (Andraski et al., 2000; Jaynes et al., 2001; Dinnes et al., 2002; Lawlor et al., 2008; Pittelkow et al., 2017). Helmers et al. (2012) assessed drainage N losses over 4 years in continuous maize and maize–soybean rotations and found that flow-weighted N concentrations increased with N rate in both systems. In a synthesis of 40 years of tile drainage N losses, Christianson and Harmel (2015) found N load exponentially increased at N rates above 150 kg N ha−1 and in years with >850 mm precipitation. Notable values in Table 3 are the relatively low N leaching potential at Monmouth in 2016 and the relatively high N leaching potential for Urbana in 2016. While Christianson and Harmel (2015) determined an average N load of 23 kg N ha−1 for Iowa, Illinois, and Minnesota in their synthesis, 19 site-years had N loads >90 kg N ha−1. The variability observed in our study is common, both considering results for the control and higher N rates. Due to elevated soil organic matter mineralization rates, studies have shown that N leaching concentrations can be similar from unfertilized and fertilized plots in some years (Lawlor et al., 2008). Similarly, a recent meta-analysis of N leaching in North America did not find N rate as a significant predictor in hierarchical regression models when analysis was restricted to observations between 110 and 270 kg N ha−1 (Eagle et al., 2017). This may be owing to the often greater importance of regional-scale environmental drivers, as reported in Eagle et al. (2017).
Instead of solely comparing treatment means for the limited number of site-years in our study, N leaching potential was also expressed on a normalized basis following Zhou and Butterbach-Bahl (2014). This approach showed that N leaching potential increased by 41% moving from zero N to 179 kg N ha−1. An additional increase in N rate from 179 to 269 kg N ha−1 further increased N leaching potential by 27%. Few differences in treatment means were observed in this study among fertilizer N treatments due to the high variability of N leaching potential. However, when linear models were used to evaluate results across the gradient of fertilizer N inputs, there was clearly an increase in N leaching potential as N rate increased. This trend provides empirical support for current nutrient loss reduction efforts promoting the application of MRTN rates to reduce N losses while maintaining yields. While changes in N rate do not provide the magnitude of N loss reductions compared to other practices such as cover crops, from a practical standpoint they are stackable, meaning they can be easily combined with other practices which may facilitate greater adoption in this region. Recently, Christianson et al. (2018) evaluated the effectiveness and stack-ability of three nutrient loss reduction strategies including Illinois and reported that N management was among the most stackable and cost effective practices.
Yield-Scaled N Leaching Potential
The yield-scaled metric can help identify sustainable intensification strategies for maintaining yields while limiting environmental impacts per unit production. In the present study, the only significant increase in yield-scaled N leaching potential was for 269 kg N ha−1 compared to the control at Monmouth in 2015 (Table 4). The remaining three of four site years showed no difference in yield-scaled N leaching among N application rates. Zhao et al. (2016) conducted a meta-analysis of 31 studies and concluded that drainage N losses expressed on a yield-scaled basis had a weak response to fertilizer N application rate, likely due to the high degree of year-to-year and site-to-site variability in precipitation and N losses. The highest value for yield-scaled N leaching potential in Table 4 was 7.2, which is generally higher than the range of means (3.6–4.5 kg N Mg−1) reported by Zhao et al. (2016) in response to increasing N rate. In another meta-analysis of 48 studies in North America, Eagle et al. (2017) found that N fertilizer rate had a positive effect on both area- and yield-scaled N losses, but noted that the exact relationship was highly variable and specific to site-year characteristics.
Our results suggest that the MRTN strategy is effective in producing close to maximum yield without leading to increased environmental impacts per unit crop production (i.e., yield-scaled N leaching potential). The normalized data across sites show that 179 kg N ha−1 resulted in more than 96% of maximum yield but prevented a 27% increase in N leaching potential and 25% increase in yield-scaled N leaching potential compared to 269 kg N ha−1. While this is a positive outcome, a slightly more nuanced interpretation is necessary, because these results contrast with the win-win scenario reported by Zhou and Butterbach-Bahl (2014). In their meta-analysis, yield-scaled N leaching was minimized at the N rate producing 90% of maximum yields (178 kg N ha−1), which implies that crop production goals and water quality concerns were not conflicting.
Our results do not support this concept, instead showing that it may be inherently difficult to minimize tradeoffs between crop production and water quality goals related to nitrate losses in this study system. While yield-scaled N leaching potential did not significantly increase at 179 kg N ha−1, yield-scaled N leaching potential was not minimized for this treatment either. In fact, normalized yield-scaled N leaching potential increased 28% compared to the control. In this regard, there was no evidence to support our hypothesis that yield-scaled N leaching potential is minimized at N rates that optimize yields. To meet targets recently established by nutrient loss reduction strategies in this region, other conservation practices will likely play an increasingly important role in overcoming this challenge, which could include cover crops, the use of nitrification inhibitors, delaying fall N application until soil temperatures are cooler, shifting fall N applications to spring, splitting N applications, and edge-of-field practices such as bioreactors, end of tile wetlands, and buffers (Illinois Nutrient Loss Reduction Strategy (IL NLRS), 2015).
It is recognized that a limited number of site-years were investigated in this study, but these results raise an important question about the yield-scaled concept, namely whether it can be used at sites with high soil N supply to balance gains in crop yield with corresponding environmental N losses. Unlike many studies showing a relatively large yield response to N fertilizer inputs, we found that normalized control yields were 69% of maximum yield, indicating a significant amount of N was supplied via soil N mineralization. Accordingly, moving from the control to 179 kg N ha−1 only resulted in a 27% yield increase (Figure 1). However, in order for yield-scaled N losses to decrease at higher N rates where maximum yield is achieved, yields must increase at a similar or faster rate than N losses. Therefore, the comparatively lower yield response in our study combined with the consistent trend of increasing N leaching potential resulted in three of four site years having similar yield-scaled N leaching potential among rates. As highlighted above, crop yield and N leaching potential are strongly influenced by climate and soil conditions, thus it is difficult to extend these results to other regions. The goal of this research was to demonstrate the concept of integrating agronomic and environmental goals when evaluating N rate strategies for maize production and the authors urge for potential environmental tradeoffs to be quantified in further site-specific studies. In particular, because soil N supply heavily influences yields in control plots, it is necessary to evaluate the yield-scaled framework in soils with high N supply where the relative rate of yield increase may be lower than the relative rate of increasing N losses.
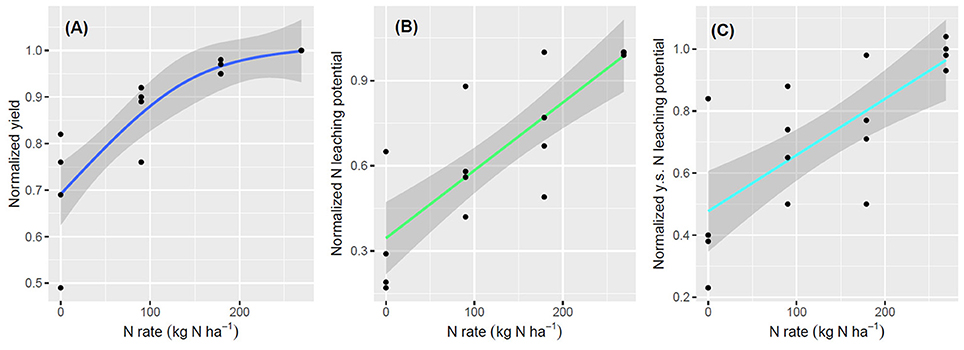
Figure 1. Normalized results for the effects of N rate on (A) yield, (B) N leaching potential, and (C) yield-scaled N leaching potential across the four site-years. Observations were expressed on a relative basis (i.e., ranging from 0 to 1) as a fraction of the highest mean value observed in each site-year. Shaded areas represent 90% confidence intervals.
Several comments regarding the advantages and disadvantages of the lysimeter methodology are necessary when interpreting results. The major transport mechanism for nitrate losses in this region is artificial subsurface drainage systems which are typically installed at around 1–1.2 m depth. However, monitoring drainage discharge and nutrient concentrations in a replicated field experiment requires major resources, thus lysimeters represent a useful indicator of N leaching potential, especially for comparing multiple treatments at multiple sites. As discussed in McIsaac et al. (2010) and Behnke et al. (2012), estimation of N leaching potential using lysimeters does not include root uptake or other possible loss pathways (e.g., denitrification) occurring below 50 cm, meaning values tend to be somewhat larger than field-scale N losses through subsurface drainage. On a related note, the major source of error in this study was most likely related to lysimeter installation. As water drains through the soil profile, dissolved nutrients are adsorbed onto resin contained inside the lysimeter. Therefore, lysimeter placement in soil must occur without influencing soil drainage characteristics in order to realistically reflect N movement below the root zone. As it was not possible to monitor changes in hydrology in the current study, the potential impacts of lysimeter installation on soil drainage conditions would be of interest to consider in future investigations.
Crop N Uptake and Post-harvest Soil N
Crop N uptake consistently increased across sites with additional N application. At Monmouth in 2015, significantly higher N uptake occurred at 269 kg N ha−1 compared to 179 kg N ha−1 (Table 5), whereas no increases were observed between these treatments in other site-years. Despite a decreasing trend in NRE with increasing N rate, no significant differences for NRE were observed. Values for NRE decreased at Monmouth in 2015 due to a lower crop yield response compared to the other three site-years. Variability in NRE is common and our results fall within the range reported in a large synthesis of maize N uptake dynamics (50% interquartile range: 26–61% NRE, n = 1,102), (Ciampitti and Vyn, 2012). The fact that improvements in yield and N uptake occurred without resulting in significant changes in NRE in our study is consistent with Burzaco et al. (2014), who found similar NRE values across three site-years and two N rates (90 and 180 kg N ha−1) in Indiana.
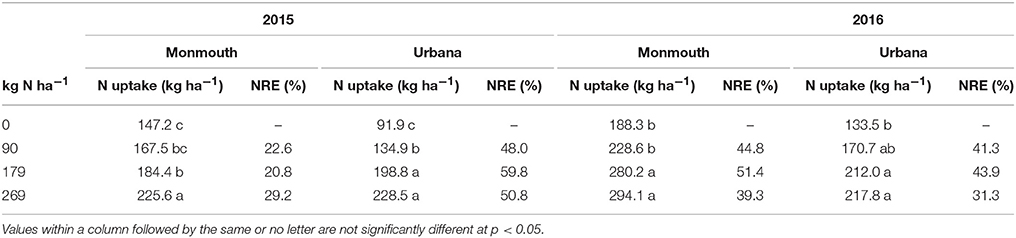
Table 5. Effects of N rate on crop N uptake and N recovery efficiency (NRE) at Monmouth and Urbana each year.
In the US Midwest there are growing efforts to balance N fertilizer inputs with crop demand as a primary means of decreasing environmental N losses (McLellan et al., 2018). By combining field study results in Iowa and New York with model simulations, Sela et al. (2016) showed that adaptive sidedress N rates strategies can help avoid unnecessary N inputs compared to conventional practice, reducing combined leaching and gaseous N losses by 38%. Similarly, Zhao et al. (2016) demonstrated that large increases in yield-scaled N losses can be avoided if N fertilizer rate matches crop demand, representing a potential win-win for producers and environment. While identifying N application rates which simultaneously increase yield and NRE is desirable from both an environmental and economic standpoint, our results only show a trend that average NRE was highest at 179 kg N ha−1 across site-years, without significant differences among N rates.
When applied N fertilizer is in excess of crop demand, increased residual soil N concentrations following harvest can represent an environmental risk (Dinnes et al., 2002; Hong et al., 2007). Elevated levels of soil residual nitrate are subject to loss the following year via leaching, particularly if above average precipitation occurs (Randall and Mulla, 2001; Gentry et al., 2009). In this study it was observed that post-harvest soil N concentrations increased with higher N application rates, particularly for 269 kg N ha−1 compared to the control (Table 6). Inorganic N concentrations generally decreased with depth, likely due to soil N mineralization being concentrated in surface soil layers. Monmouth in 2015 was the only site without significant differences among N rates for mean soil N concentration (0–90 cm). Jaynes et al. (2001) noted high variability of end-of-season soil N concentrations between replications within a treatment, such that the highest N rate only had significantly greater end-of-season soil N concentrations compared to lower N rates in 1 of 4 years. Similarly, increases for 269 kg N ha−1 were observed compared to 90 kg N ha−1 in 2 of 4 years.
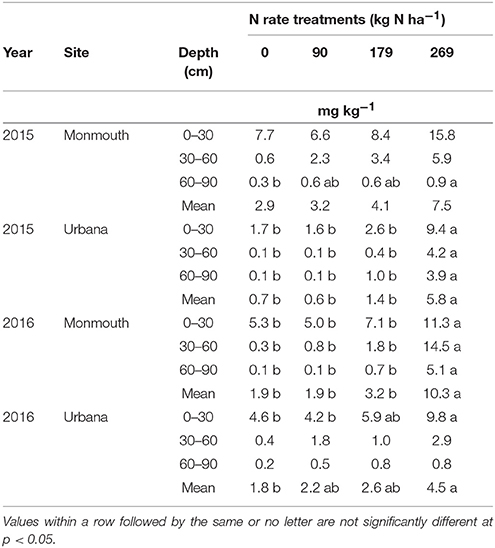
Table 6. Effects of N rate on postharvest soil N concentration by soil depth at Monmouth and Urbana each year.
Considering end-of-season crop and soil N dynamics together, our results show that increasing N application from 179 to 269 kg N ha−1 did not increase crop N uptake in three of four site years, but contributed to a trend of decreasing NRE and increasing post-harvest soil N concentrations. These findings are supported by Hong et al. (2007), who determined that residual soil N is consistently higher with N rates above the economic optimum in a multi-location study in Missouri. Previous research in this region has also documented the direct link between higher post-harvest soil N concentrations and subsequent negative impacts on water quality via enhanced nitrate losses. In a 6 year study, David et al. (1997) found that an average of 49% (ranging from 25 to 85%) of the pool of residual nitrate remaining after harvest was leached through tile drains. Jaynes and Colvin (2006) showed that higher postharvest soil N concentrations with higher N rates applied to maize contributed to elevated N losses from soybean the following year. Hence, in addition to N leaching potential which was quantified in this study during each growing season using lysimeters, results for postharvest soil N concentrations further highlight the likelihood for negative water quality impacts associated with N application rates above current MRTN recommendations.
Conclusion
The aim of this study was to develop balanced N rate strategies for minimizing N leaching potential per unit crop production while simultaneously increasing crop productivity. For the N rate falling within the range of current MRTN recommendations (179 kg N ha−1), normalized data across the four site-years show that more than 96% of maximum yield was achieved while preventing a 27 and 25% increase in N leaching potential and yield-scaled N leaching potential, respectively, compared to the highest N rate (269 kg N ha−1). Increasing N application from 179 to 269 kg N ha−1 did not generally increase crop N uptake but contributed to a trend of decreasing NRE and increasing post-harvest soil N concentrations, further escalating the risk of environmental N losses during the subsequent winter. These results support nutrient loss reduction efforts in this region promoting MRTN as a tool to generate high yields while reducing the potential for environmental N losses compared to higher N rates. At the same time, our results did not support the hypothesis that yield-scaled N leaching potential was minimized at N rates that optimize yields. Normalized yield-scaled N leaching potential increased by 28% compared to the control, largely due to the relatively high yields in control plots and a limited yield response to added N fertilizer compared to previous work. This finding contrasts with several prior studies and suggests that inherent tradeoffs may exist when trying to minimize conflict between crop production and water quality goals related to nitrate losses in this system.
Author Contributions
KG and CP contributed to all phases of this research including study design, data collection and analysis, results interpretation, and writing the paper.
Funding
This material is based upon work supported by the National Institute of Food and Agriculture, US Department of Agriculture, Hatch project (No. ILLU-802–949).
Conflict of Interest Statement
The authors declare that the research was conducted in the absence of any commercial or financial relationships that could be construed as a potential conflict of interest.
Acknowledgments
The authors gratefully acknowledge the contributions of Cheri Esgaer, Ben Baechle, Alexandre Pedrinho, Joe Edwards, Juan Burjel, Xu Zhao, Sara Riccetto, and Rebecca Graham for assisting with field data collection and laboratory analysis.
References
Andraski, T. W., Bundy, L. G., and Brye, K. R. (2000). Crop management and corn nitrogen rate effects on nitrate leaching. J. Environ. Qual. 29, 1095–1103. doi: 10.2134/jeq2000.00472425002900040009x
Behnke, G. D., David, M. B., and Voigt, T. B. (2012). Greenhouse gas emissions, nitrate leaching, and biomass yields from production of Miscanthus x giganteus in Illinois, USA. Bioenerg. Res. 5, 801–813. doi: 10.1007/s12155-012-9191-5
Burzaco, J. P., Ciampitti, I. A., and Vyn, T. J. (2014). Nitrapyrin impacts on maize yield and nitrogen use efficiency with spring-applied nitrogen: field studies vs. meta-analysis comparison. Agron. J. 106, 753–760. doi: 10.2134/agronj2013.0043
Christianson, L. E., and Harmel, R. D. (2015). 4R water quality impacts: an assessment and synthesis of forty years of drainage nitrogen losses. J. Environ. Qual. 44, 1852–1860. doi: 10.2134/jeq2015.03.0170
Christianson, R., Christianson, L., Wong, C., Helmers, M., McIsaac, G., Mulla, D., et al. (2018). Beyond the nutrient strategies: common ground to accelerate agricultural water quality improvement in the upper Midwest. J. Environ. Manage. 206, 1072–1080. doi: 10.1016/j.jenvman.2017.11.051
Ciampitti, I. A., and Vyn, T. J. (2012). Physiological perspectives of changes over time in maize yield dependency on nitrogen uptake and associated nitrogen efficiencies: a review. Field Crops Res. 133, 48–67. doi: 10.1016/j.fcr.2012.03.008
David, M. B., Drinkwater, L. E., and Mclsaac, G. F. (2010). Sources of nitrate yields in the Mississippi River basin. J. Environ. Qual. 39, 1657–1667. doi: 10.2134/jeq2010.0115
David, M. B., Gentry, L. E., Kovacic, D. A., and Smith, K. M. (1997). Nitrogen balance in and export from an agricultural watershed. J. Environ. Qual. 26, 1038–1048. doi: 10.2134/jeq1997.00472425002600040015x
Dinnes, D. L., Karlen, D. L., Jaynes, D. B., Kaspar, T. C., Hatfield, J. L., Colvin, T. S., et al. (2002). Nitrogen management strategies to reduce NO3 leaching in tile-drained midwestern soils. Agron. J. 94, 153–171. doi: 10.2134/agronj2002.1530
Eagle, A. J., Olander, L. P., Locklier, K. L., Heffernan, J. B., and Bernhardt, E. S. (2017). Fertilizer management and environmental factors drive N2O and NO3 losses in corn: a meta-analysis. Soil. Sci. Soc. Am. J. 81, 1191–1202. doi: 10.2136/sssaj2016.09.0281
Garnett, T., Appleby, M. C., Balmford, A., Bateman, I. J., Benton, T. G., Bloomer, P., et al. (2013). Sustainable intensification in agriculture: premises and policies. Science 341, 33–34. doi: 10.1126/science.1234485
Gentry, L. E., David, M. B., Below, F. E., Royer, T. V., and McIsaac, G. F. (2009). Nitrogen mass balance of a tile-drained agricultural watershed in east-central Illinois. J. Environ. Qual. 38, 1841–1847. doi: 10.2134/jeq2008.0406
Helmers, M. J., Zhou, X., Baker, J. L., Melvin, S. W., and Lemke, D. W. (2012). Nitrogen loss on tile drained Mollisols as affected by nitrogen application rate under continuous corn and corn-soybean rotations. Can. J. Soil Sci. 92, 493–499. doi: 10.4141/cjss2010-043
Hong, N., Scharf, P. C., Davis, J. G., Kitchen, N. R., and Sudduth, K. A. (2007). Economically optimal nitrogen rate reduces soil residual nitrate. J. Environ. Qual. 36, 354–362. doi: 10.2134/jeq2006.0173
Hothorn, T., Bretz, F., and Westfall, P. (2008). Simultaneous inference in general parametric models. Biom. J. 50, 346–363. doi: 10.1002/bimj.200810425
Illinois Nutrient Loss Reduction Strategy (IL NLRS) (2015). Illinois Environmental Protection Agency. Available online at: www.epa.illinois.gov/Assets/iepa/water-quality/watershed-management/nlrs/nlrs-final-revised-083115.pdf (Accessed February 8, 2017).
Jaynes, D. B., and Colvin, T. S. (2006). Corn yield and nitrate loss in subsurface drainage from midseason nitrogen fertilizer application. Agron. J. 98, 1479–1487. doi: 10.2134/agronj2006.0046
Jaynes, D. B., Colvin, T. S., Karlen, D. L., Cambardella, C. A., and Meek, D. W. (2001). Nitrate loss in subsurface drainage as affected by nitrogen fertilizer rate. J. Environ. Qual. 30, 1305–1314. doi: 10.2134/jeq2001.3041305x
Langlois, J. L., Johnson, D. W., and Mehuys, G. R. (2003). Adsorption and recovery of dissolved organic phosphorus and nitrogen by mixedbed ion-exchange resin. Soil Sci. Soc. Am. J. 67, 889–894. doi: 10.2136/sssaj2003.0889
Lawlor, P. A., Helmers, M. J., Baker, J. L., Melvin, S. W., and Lemke, D. W. (2008). Nitrogen application rate effect on nitrate-N concentration and loss in subsurface drainage for a corn-soybean rotation. Trans. ASABE 51, 83–94. doi: 10.13031/2013.24229
McIsaac, G. F., David, M. B., and Mitchell, C. A. (2010). Miscanthus and switchgrass production in central Illinois: impacts on hydrology and inorganic nitrogen leaching. J. Environ. Qual. 39, 1790–1799. doi: 10.2134/jeq2009.0497
McLellan, E. L., Cassmann, K. G., Eagle, A. J., Woodbury, P. B., Sela, C. T., Majerison, R. D., et al. (2018). The nitrogen balancing act: tracking the environmental performance of food production. BioScience 68, 194–203. doi: 10.1093/biosci/bix164
Mississippi River/Gulf of Mexico Watershed Nutrient Task Force (2008). Gulf Hypoxia Action Plan 2008 for reducing, mitigating, and controlling hypoxia in the Northern Gulf of Mexico and improving water quality in the Mississippi River Basin. USEPA Office of Wetlands, Oceans, and Watersheds, Washington, DC.
Morris, T. F., Murrell, T. S., Beegle, D. B., Camberato, J. J., Ferguson, R. B., Grover, J., et al. (2018). Strengths and limitations of nitrogen rate recommendations for corn and opportunities for improvement. Agron. J. 110, 1–37. doi: 10.2134/agronj2017.02.0112
Pinheiro, J., Bates, D., DebRoy, S., Sarkar, D., and and, R. (2014). nlme: Linear and Nonlinear Mixed Effects Models. R package version 3.1-117. Available online at: http://CRAN.R-project.org/package=nlme
Pittelkow, C. M., Clover, M. W., Hoeft, R. G., Nafziger, E. D., Warren, J. J., Gonzini, L. C., et al. (2017). Tile drainage nitrate losses and corn yield response to fall and spring nitrogen management. J. Environ. Qual. 46, 1057–1064. doi: 10.2134/jeq2017.03.0109
Poffenbarger, H. J., Barker, D. W., Helmers, M. J., Miguez, F. E., Olk, D. C., Sawyer, J. E., et al. (2017). Maximum soil organic carbon storage in Midwest U.S. cropping systems when crops are optimally nitrogen-fertilized. PLoS ONE 12:e0172293. doi: 10.1371/journal.pone.0172293
Puntel, L. A., Sawyer, J. E., Barker, D. W., Dietzel, R., Poffenbarger, H., Castellano, M. J., et al. (2016). Modeling long-term corn yield response to nitrogen rate and crop rotation. Front. Plant Sci. 7:1630 doi: 10.3389/fpls.2016.01630
Randall, G. W., and Mulla, D. J. (2001). Nitrate nitrogen in surface waters as influenced by climatic conditions and agricultural practices. J. Environ. Qual. 30, 337–344. doi: 10.2134/jeq2001.302337x
R Core Team (2014). R: A language and Environment for Statistical Computing. R Foundation for Statistical Computing, Vienna. Available online at: http://www.R-project.org
Sawyer, J. E., Nafziger, E. D., Randall, G., Bundy, L., Rehm, G., and Joern, B. (2006). Concepts and Rationale for Regional Nitrogen Rate Guidelines for Corn. Publication PM 2015. Iowa State University, Ames, IA.
Sela, S., van Es, H. M., Moebius-Clune, B. N., Marjerison, R., Melkonian, J., Moebius-Clune, D., et al. (2016). Adapt-N outperforms grower-selected nitrogen rates in Northeast and Midwestern United States strip trials. Agron. J. 108, 1726–1734. doi: 10.2134/agronj2015.0606
Sela, S., van Es, H. M., Moebius-Clune, B. N., Marjerison, R., Moebius-Clune, D., Schindelbeck, R., et al. (2017). Dynamic model improves agronomic and environmental outcomes for maize nitrogen management over static approach. J. Environ. Qual. 46, 311–319. doi: 10.2134/jeq2016.05.0182
Sobota, D. J., Compton, J. E., McCrackin, M. L., and Singh, S. (2015). Cost of reactive nitrogen release from human activities to the environment in the United States. Environ. Res. Lett. 10:025006. doi: 10.1088/1748-9326/10/2/025006
Susfalk, R. B., and Johnson, D. W. (2002). Ion exchange resin based soil solution lysimeters and snowmelt solution collectors. Commun. Soil. Sci. Plant. Anal. 33, 1261–1275. doi: 10.1081/CSS-120003886
Tilman, D., Balzer, C., Hill, J., and Befort, B. L. (2011). Global food demand and the sustainable intensification of agriculture. Proc. Natl. Acad. Sci. U.S.A. 108, 20260–20264. doi: 10.1073/pnas.1116437108
Zhang, X., Davidson, E. A., Mauzerall, D. L., Searchinger, T. D., Dumas, P., and Shen, Y. (2015). Managing nitrogen for sustainable development. Nature 528, 51–59. doi: 10.1038/nature15743
Zhao, X., Christianson, L. E., Harmel, D., and Pittelkow, C. M. (2016). Assessment of drainage nitrogen losses on yield-scaled basis. Field Crops Res. 199, 156–166. doi: 10.1016/j.fcr.2016.07.015
Keywords: N application rate, maize yield, yield-scaled N losses, N recovery efficiency, US Midwest
Citation: Greer KD and Pittelkow CM (2018) Linking Nitrogen Losses With Crop Productivity in Maize Agroecosystems. Front. Sustain. Food Syst. 2:29. doi: 10.3389/fsufs.2018.00029
Received: 13 April 2018; Accepted: 04 June 2018;
Published: 26 June 2018.
Edited by:
Ali Shahnazari, Sari Agricultural Sciences and Natural Resources University, IranReviewed by:
Mohammad Valipour, Payame Noor University, IranJorge A. Zegbe, Instituto Nacional de Investigaciones Forestales, Agrícolas y Pecuarias (INIFAP), Mexico
Copyright © 2018 Greer and Pittelkow. This is an open-access article distributed under the terms of the Creative Commons Attribution License (CC BY). The use, distribution or reproduction in other forums is permitted, provided the original author(s) and the copyright owner are credited and that the original publication in this journal is cited, in accordance with accepted academic practice. No use, distribution or reproduction is permitted which does not comply with these terms.
*Correspondence: Kristin D. Greer, a2dyZWVyQGlsbGlub2lzLmVkdQ==