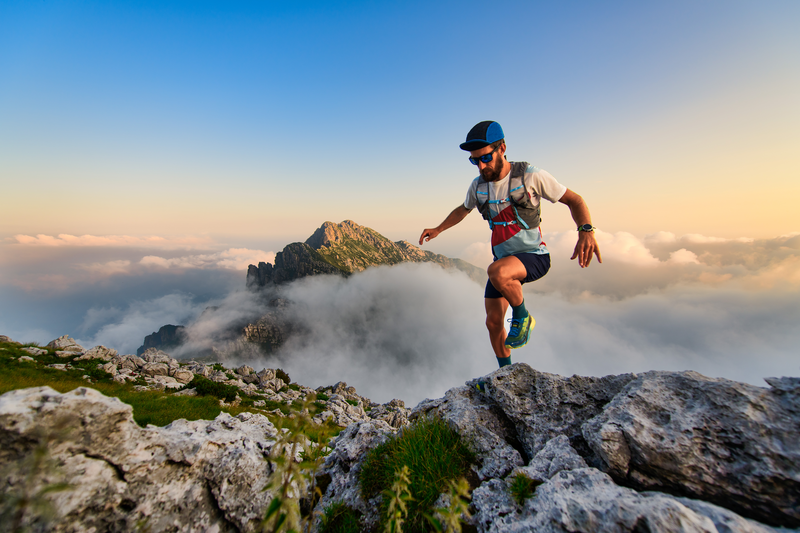
94% of researchers rate our articles as excellent or good
Learn more about the work of our research integrity team to safeguard the quality of each article we publish.
Find out more
ORIGINAL RESEARCH article
Front. Sustain. Food Syst. , 19 June 2018
Sec. Waste Management in Agroecosystems
Volume 2 - 2018 | https://doi.org/10.3389/fsufs.2018.00027
This article is part of the Research Topic RAMIRAN 2017: Sustainable Utilisation of Manures and Residue Resources in Agriculture View all 20 articles
New technology makes it possible to apply organic fertilizers with higher precision, and organic producers want to know how to exploit these new possibilities to make their production more efficient. This study investigated the effect of band application (in different positions) of pelleted organic fertilizer, compared with broadcasting, on grain yield and weed density in spring oats (Avena sativa L.). Six microplot field experiments were carried out on silty clay and sandy loam in Sweden during the growing season of 2014–2016. In oats seeded at 25 cm row spacing, pelleted meat bone meal was band-applied at one of three distances from the crop row (0, 4, and 12.5 cm) and at two or three incorporation depths (1 and 4 cm on silty clay and 1, 4, and 6 cm on loamy sand). These treatments were compared with broadcast spreading, mineral nitrogen fertilizer, and an unfertilized control. On both soil types, fertilizer placement 4 cm from the crop and 4–6 cm incorporation depth gave the highest yield and crop nitrogen uptake. Yield in this treatment was 800 kg ha−1 higher on clay soil and 1,100 kg ha−1 higher on sandy loam compared with the same organic fertilizer applied by broadcasting, an 80–150% yield increase. On the sandy loam, distance from the crop row had a more significant effect on grain yield (p < 0.001) than soil incorporation depth (p = 0.07). On the silty clay, crop yield was significantly influenced by incorporation depth (p = 0.003) and distance from the crop row (p = 0.04). In five experiments, mineral N fertilizer equivalent (MFE) increased from on average 63% with broadcasting to 85% with placement 4 cm from the crop row and 4 cm incorporation depth. Weed biomass was significantly affected by fertilizer placement on the clay soil, with higher weed biomass with deeper incorporation (p = 0.045) and greater distance from the crop row (p = 0.049). On the sandy loam, there was a tendency for larger weed plants at greater distance from the crop row (p = 0.13) except when seeds and pellets were placed together, which gave the highest weed weight, probably due to lower competition from the crop in this treatment.
Due to their physical properties, organic fertilizers are usually difficult to apply with good precision. Use of pelleted organic fertilizers is therefore an attractive alternative for organic farmers. Pellets can be applied with machines that provide a uniform distribution in the field and are not as limited in time to perfect soil conditions as fertilizer products that require heavy machinery. If the pellets are sufficiently robust, they can be applied with a seeder and incorporated in rows with the same precision as seeds or granulated mineral fertilizers. The dose, time of application, and placement can thereby be much better optimized than for other organic fertilizers, which are often heavy, sticky, and difficult to distribute. Modern machinery equipped with RTK GPS and designed for seeding and weed hoeing with high precision is becoming more frequent in Swedish organic production. This gives farmers the possibility to place the pellets with high precision and many farmers want to know more about optimal placement. Meat and bone meal (MBM) is currently a common pelleted fertilizer in Sweden, although products with chicken manure were more common in the past. In the future, and in other countries, other organic materials could be used.
When pellets are broadcast, soil incorporation is often performed with a harrow to mix the pellets with a large volume of soil, but this typically leaves a large fraction of the pellets close to the soil surface. Application in bands with a seeder can achieve deep incorporation of all pellets, and also keeps the pellets more concentrated and less mixed with the soil. The way in which organic fertilizers are placed in soil during application can affect the biological turnover of nitrogen (Sørensen and Jensen, 1995; Sørensen and Amato, 2002). For example, if the fertilizer is mixed with a larger volume of soil, immobilization of nitrogen will be faster (Sørensen and Jensen, 1995), since both the energy source (carbon) and the nitrogen will be available to a larger number of microorganisms. If the fertilizer is instead concentrated to narrow bands in the soil, microbial access to carbon and nitrogen, and thus nitrogen immobilization, will be more limited. Baitilwake et al. (2012) found greater immobilization and nitrification after soil incorporation than surface application of chicken and cattle manure. However, Delin and Strömberg (2011) did not find any differences in net mineralization of nitrogen between surface-applied and incorporated chicken manure and cattle slurry, as long as the manure was kept in concentrated lumps, although when the manure was mixed with a larger soil volume both net immobilization and nitrification increased.
Apart from the potential advantages listed above, band application also means that the fertilizer can be applied at an optimal distance from the crop row. In organic farming, wide row spacing is commonly used to facilitate mechanical weed control (Hiltbrunner et al., 2005). This justifies pellet placement close to the row, instead of broadcasting or placement mid-way between rows, as this can increase nutrient availability for the crop and reduce it for weeds (Rasmussen et al., 1996). This has previously been observed with mineral fertilizers (Rasmussen et al., 1996; Blackshaw, 2005) and animal slurry on corn (Schröder et al., 1997; Rasmussen, 2002; Petersen, 2005; Bittman et al., 2012). In the case of animal slurry, placement close to the seed row has been shown to increase yield in spring cereals (Petersen, 2005) and phosphorus (P) uptake in corn (Schröder et al., 1997; Bittman et al., 2012). Rasmussen et al. (1996) observed 55% less weed biomass and 28% higher yield of spring barley after application of fertilizer close to the crop row compared with broadcasting. In a 4-year experiment, Blackshaw (2005) found that subsurface-banded mineral nitrogen fertilizer applied to spring wheat often led to lower weed nitrogen uptake and biomass and higher grain yield than when nitrogen was surface-applied. However, no study performed to date on placement of pelleted organic fertilizers to spring cereals has sought to identify the optimal placement of pellets in relation to seed and quantify the production benefits.
The objective of the present study was to investigate how crop nitrogen (N) uptake, grain yield, and weed density in spring cereals on two different soils are affected by band application of pelleted organic fertilizer at different incorporation depths and distances from the crop row, in comparison with broadcast pellets with very shallow incorporation. The hypotheses are that (i) the nitrogen use efficiency of pelleted fertilizers increases with incorporation depth, but the effect decreases with precipitation, (ii) with row spacing of 25 cm or more, the effect on yield of nitrogen in pelleted fertilizers is higher and the weed pressure lower if the fertilizer is placed less than 6 cm from the seed row than if it is broadcast or placed at greater distance from the row, and (iii) any yield loss caused by 25 cm row spacing instead of the conventional 12.5 cm will be smaller than the yield increase caused by band application with optimized placement. In the experiments, a spring oats crop seeded at 25 cm row distance was fertilized with pelleted MBM, as an example relevant for Swedish organic production.
Six microplot field experiments were carried out in spring oats (Avena sativa L.) on silty clay and sandy loam soils (Table 1) in Sweden (58°N, 13°E) during the growing season of 2014-2016. The sandy loam was on a farm with regular addition of farmyard manure, whereas on the silty clay soil manure has not been applied for a long time. Mean monthly precipitation and temperature in the period May-August 2014–2016 were recorded at a location <20 km from all study sites (Table 2). In 2014, the weather was warm and dry in July, followed by heavy precipitation in August, while 2015 had a cool growing season and 2016 had rather dry weather in May (Table 2).
Table 2. Mean monthly air temperature and precipitation at the experimental sites in April–August, 2014–2016, compared with *mean climate for the period 1960–1990.
In randomized block experiments, four blocks and 12 treatments (treatments 1–2, 6–15) were established on silty clay and an additional three treatments (treatments 3–5) on sandy loam (Table 3). Apart from two control treatments, one of which received no fertilizer (treatment 1) and one 60 kg N ha−1 as mineral fertilizer (Axan; 27% N and 4% sulfur (S); Yara Sweden) (treatment 2), all treatments received 60 kg N ha−1 as pelleted meat bone meal (MBM) (Ekoväx 8-3-5-3; Ekoväx Sweden). The nitrogen in the pelleted MBM was 95% organic, of which 60% was expected to be plant-available within 1–2 months after application (Delin and Engström, 2010; Delin et al., 2012). The pelleted MBM also contained 23 kg P ha−1, 38 kg potassium (K) ha−1, and 23 kg S ha−1. Placement at different depths and distances from the crop row was compared with shallow incorporation of broadcast pellets. A 25 cm row spacing was used, since it is common practice in organic farming to enable mechanical weeding between crop rows. For comparison, one additional treatment with broadcasting involved the conventional row spacing of 12.5 cm (treatment 13). To test the effects of placement and incorporation under moist conditions, two extra treatments with irrigation, one in plots with broadcasting (treatment 14) and one in plots with 4 cm incorporation and placement in rows (treatment 15) were included in the experiments.
Table 3. Incorporation depth and distance from the crop row of Axan (NH4NO3) and pelleted meat bone meal (MBM) placement, crop row spacing, and irrigation in different treatments (T) in six experiments on silty clay (C) and sandy loam (S) during 2014–2016.
Each microplot was 0.7 m2 and was seeded and fertilized by hand, with four (or eight in treatment 13) 70 cm long crop rows per plot. During seeding, two 70 cm long iron plates were knocked into the ground to form a trench, into which seeds and/or pellets were poured. The plates were then removed and soil was drawn from the sides to close the trenches. Seeds were placed at 4 cm depth (and thereby together with pellets in treatment 6). The amount of seeds planted in each plot was 550 seeds m−2, according to general recommendations. In the broadcasting treatments (12–14), pellets were only gently incorporated by mixing by hand into the upper 1-cm layer of soil. The irrigated treatments (14–15) received around 5 L water per plot, corresponding to 7 mm, directly after sowing and pellet application. To ensure weed pressure, white mustard (Sinapis alba L.) was sown in a diagonal across each microplot in 2014 (14 seeds per plot). This was repeated only on the silty clay soil in 2015 and 2016, since the weed pressure from the naturally occurring weed flora was considered to be high enough on the sandy loam.
Weed density and biomass and crop yield were measured within a net area of 50 × 50 cm, i.e., 50 cm of the two (or four in treatment 13) middle crop rows. The number of weed plants was counted on two occasions, at stem elongation of the spring oat crop (growth stage (GS) 30) and at panicle emergence (GS 55) (Zadoks et al., 1974). At panicle emergence, the weeds were harvested as close to ground level as possible, dried, and weighed plot-wise. The nitrogen content was analyzed treatment-wise. The oat crop was harvested at ripening (GS 92) by cutting 1-2 cm above the ground, and dried at 60°C for 24 h. The crop samples were then threshed and grains and straw were weighed separately. The plant material was milled and subsamples were analyzed for water and nitrogen content. Nitrogen analyses were performed with a Leco TruMac CN (LECO Corporation, St. Joseph, MI, USA).
Grain yield (15% water content, kg ha−1), nitrogen offtake with grain yield (kg N ha−1), and total aboveground nitrogen (kg N ha−1) in treatments 3-11 had the corresponding values from treatments 1 and 12 deducted, to give the net increase for different placements compared with no fertilization and broadcasting, respectively. These effects, together with weed numbers (no. m−2) and weed biomass (dry matter, g m−2), were statistically analyzed with a two-way ANOVA, including the factors incorporation depth, distance from the crop row and their interaction. Year was treated as a random factor. Experiments on clay soil and sandy soil were analyzed separately. Effects of irrigation were analyzed by one-way ANOVA including treatments 7, 12, 14, and 15, in order to determine whether irrigation increased yield more for broadcasting (treatment 14 compared with 12) than for row incorporation (treatment 15 compared with 7). Effect of crop row spacing was analyzed by comparing treatments 12 and 13. All models were fitted using the general linear model in Minitab 16 Statistical Software (Minitab Inc. 2010).
Nitrogen offtake with grain in treatments 3–15 was compared against the mineral N fertilizer response, in order to calculate the mineral fertilizer equivalent (MFE), i.e., the fraction of total N as available to plants as N applied as ammonium nitrate (Delin et al., 2012; Jensen, 2013).
The spring oat crop produced on average comparatively high grain yield in treatment 1 without fertilization (around 4,400 kg ha−1) on the sandy loam, whereas on the silty clay the average grain yield in the unfertilized plots was very low (1,300–2,600 kg ha−1) (Table 4). Fertilization with mineral nitrogen fertilizer (treatment 2) resulted in around a 30-40 kg ha−1 yield increase per additional kg N in most experiments, but only 15 kg ha−1 per additional kg N on the silty clay in 2014 (calculated from Table 4). Yield was very low in all treatments in this experiment (around 2,000 kg ha−1), whereas yield was normal (moderate) in the other two experiments on silty clay (4,500–5,000 kg ha−1) and rather high on the sandy loam (6,000–7,000 kg ha−1). Straw yield also differed between soils, with on average 5,200 kg dry matter (DM) ha−1 on the sandy loam and 3,000 kg DM ha−1 on the silty clay (data not shown). Straw yield was linearly correlated with grain yield and of similar magnitude, with a straw/grain ratio of 0.9 on the sandy loam and 1.2 on the silty clay.
Table 4. Grain yield (15% water content, kg ha−1) in treatments 1–15 (see Table 3) on two different soils (sandy loam and silty clay) in 2014–2016.
Nitrogen offtake with grain yield on the silty clay ranged from on average 26 kg N ha−1 in the unfertilized treatment to 48 kg N ha−1 in the treatment with mineral fertilizer. It was 42 kg N ha−1 in the highest yielding treatment with pelleted MBM (treatment 7). On the sandy loam, nitrogen offtake was on average 62 kg N ha−1 in the unfertilized treatment, 85 kg N ha−1 in the treatment with mineral fertilizer, and 89 kg N ha−1 in the highest yielding treatment with pelleted MBM (treatment 4) (data not shown).
The effects on yield of band placement of MBM pellets (treatments 3–11) compared with broadcasting (treatment 12) varied depending on placement distance from the crop row and incorporation depth in the soil (Figure 1). On both soil types, fertilizer placement 4 cm from the crop row with 4–6 cm incorporation depth gave the highest yield, with on average 800 kg ha−1 higher yield on clay soil and 1,100 kg higher yield on sandy loam compared with broadcasting (Figure 1). This represents an 80–150% yield increase. Some other placement options reduced yield compared with broadcasting, for instance placement together with the seed on loamy sand (Figure 2) or shallow incorporation and placement far from the crop row on silty clay (Figure 1).
Figure 1. Grain yield increase in treatments with placement of pelleted meat bone meal (MBM) in rows compared with broadcasting, as a function of distance from crop row and incorporation depth, on (left) silty clay and (right) sandy loam (3-year average, error bars indicate standard error).
Figure 2. Increase in above-ground crop nitrogen in treatments with placement of pelleted meat bone meal (MBM) in rows compared with broadcasting, as a function of distance from crop row and incorporation depth, on (left) silty clay and (right) sandy loam (3-year average, error bars indicate standard error).
Analysis of the differences in yield increase with the different placement of band-applied pellets compared with broadcast revealed that the interaction between incorporation depth and distance from the crop row was not negligible (p = 0.02 on sandy loam, p = 0.09 on silty clay) and was thus included in the model. On the sandy loam, there were statistically significant differences between different placement distances from the crop row (p < 0.001), with on average 600 kg ha−1 higher yield and 7 kg N ha−1 higher nitrogen offtake for 4 cm compared with 12.5 cm distance from the crop row, but not between different incorporation depths (p = 0.07). Treatment 6, where pellets and seeds were placed at the same position in soil, gave the lowest yield effect on the sandy loam soil.
On the silty clay, there were statistically significant differences in crop yield between incorporation depths (p = 0.003) and distances from the crop row (p = 0.04). On this soil, crop yield was on average 450 kg ha−1 higher and nitrogen offtake on average 4 kg N ha−1 higher when pellets were incorporated to 4 cm compared with 1 cm depth, and on average 460 kg ha−1 and 4 kg N ha−1 higher, respectively, when placed at 4 cm compared with 12.5 cm from the crop row. Combining 4 cm incorporation with placement 4 cm from the crop row gave 1,200 kg ha−1 higher yield (Figure 1) and 11 kg N ha−1 higher nitrogen offtake than the lowest yielding option (1 cm incorporation, 12 cm from crop row).
The differences in above-ground crop nitrogen (in both straw and grain; Figure 2) showed a similar pattern to the differences in grain yield (Figure 1). On the silty clay, incorporation depth had a significant impact on crop nitrogen (p = 0.01), with on average 5 kg ha−1 more nitrogen in the crop when pellets were incorporated to 4 cm depth compared with 1 cm. The differences in crop nitrogen depending on pellet distance from the crop row were not statistically significant (p = 0.095), but the trend was similar to that observed for yield, with decreasing nitrogen uptake with increasing distance from the crop row (Figure 2). On the sandy loam, the differences were larger (Figure 2) and statistically significant for distance from crop row (p = 0.018), but not for incorporation depth (p = 0.062) or interaction (p = 0.12).
Irrigation had no statistically significant effects on yield differences between treatments with placement (treatments 7, 15) compared with broadcasting (12, 14) on either soil type in any year. In the irrigated treatments, placement increased yield by on average 1,300 kg ha−1 on the sandy loam and 600 kg ha−1 on the silty clay, which is similar to the yield increase in their unirrigated counterparts (1100 kg ha−1 and 800 kg ha−1, respectively).
Yield was on average 300 kg ha−1 (p = 0.034) higher in the treatment with conventional row spacing (12.5 cm) and broadcast MBM pellets than in the corresponding treatment with double row spacing. The difference varied between years and sites, and was on average larger on the silty clay (440 kg ha−1) than on the sandy loam (120 kg ha−1).
On average for both soils, the MFE for pelleted MBM (Table 5) was 79% in treatment 6, i.e. with similar placement of pellets and the mineral fertilizer used for comparison. However, in one experiment (on sandy loam), the MFE values in several treatments were above 100%, indicating that nutrients other than nitrogen probably limited crop yield. In the other five experiments, MFE increased from on average 62% in the treatment with broadcasting to 85% in the highest yielding treatment (placement 4 cm from crop row, 4 cm incorporation depth). Placement of pellets together with the crop seeds was not a good alternative (average MFE 56%).
Table 5. Mineral nitrogen fertilizer equivalents (MFE) of meat bone meal (MBM) pellets, calculated from nitrogen offtake (Equation 1) in treatments 3–15 (see Table 3) on sandy loam and silty clay in 2014–2016.
The weed flora on the silty clay soil was dominated by the planted Sinapis alba L. and the naturally occurring weed species Chenopodium album, L., Elymus repens (L.) Gould and Sinapis arvensis L. The weed flora on the sandy loam comprised many species, including Fumaria officinalis L., Viola arvensis Murr., and Myosotis arvensis (L.) Hill.
The number of weeds was approximately twice as high on the sandy loam (300–400 plants m−2) as on the silty clay soil (150–200 plants m−2) at the first count (GS 30). On the sandy loam, the number of weeds declined by on average 20% from the first (GS 30) to the second count (GS 55) and there were no significant differences in the weed decrease depending on pellet incorporation (p = 0.52) or pellet distance from the crop row (p = 0.79). Average weed plant weight (Table 6) on the sandy loam was 0.16 g DM plant−1, with a tendency for larger weed plants with greater distance from crop rows (p = 0.13). An exception was treatment 6 (seeds and pellets placed together), which had the largest weed weight (0.21 g DM plant−1). On the silty clay, weed numbers increased, from on average 155 plants m−2 at the first count (GS 30) to 210 plants m−2 at the second count (GS 55), with no significant differences in the weed increase depending on pellet incorporation (p = 0.53) or pellet distance from the crop row (p = 0.70). On the silty clay, weed plant weight (Table 6) was significantly higher (p = 0.001) when pellets were incorporated to 4 cm (0.075 g DM plant−1) compared with 1 cm (0.003 g DM plant−1), with a tendency (p = 0.066) for larger weed plants when pellets were placed 12.5 cm from the crop row.
Table 6. Weed dry weight (g m−2) in treatments 1–15 (see Table 2) on sandy loam and silty clay in 2014–2016.
Average weed biomass at panicle emergence (GS 55) of the oat crop was similar on the two soil types (Table 5). On the silty clay, weed biomass was significantly affected by pellet placement, with higher weed biomass with deeper incorporation of the fertilizer (p = 0.045) and greater distance from the crop row (p = 0.049) (Figure 3). On the sandy loam, there were no statistically significant effects of placement (p = 0.7), but for the treatments with 1 cm incorporation (treatments 9-11) there was a tendency for higher weed pressure with greater distance from the crop row, whereas no such tendency was observed at deeper incorporation depths.
Figure 3. Difference in weed biomass with placement of pelleted meat bone meal (MBM) in rows compared with broadcasting, as a function of distance from crop row and incorporation depth, on (left) silty clay, and (right) sandy loam (3-year average, error bars indicate standard error).
The effects of placement differed between the two soils studied, which were chosen to represent a clay and a sandy loam soil. However, the sandy soil had received regular doses of farmyard manure over time, whereas the clay soil had not received any farmyard manure during the previous 50 years. The difference in nitrogen offtake in the unfertilized treatment on these soils indicated that the sandy loam delivered more than twice as much nitrogen as the silty clay. The sandy loam also had larger crop biomass, on average 10,400 kg DM ha−1 for all years and treatments, compared with only 5,800 kg DM ha−1 on the silty clay. This means that competition for water and light between crop and weeds was tougher on the sandy loam, which could explain why the larger amounts of weeds on the sandy loam did not result in higher weed biomass than on the silty clay. The tougher competition with the crop probably also affected differences in weed biomass between treatments, as weed biomass tended to be larger in treatments where yield was lower, such as treatment 6 where yield was suppressed and weed biomass elevated. On the clay soil, both weeds and crop were favored by incorporation of fertilizer, indicating that competition was not important for the outcome. The soil nutrient status of the sandy loam is more typical of organic farms in Sweden, and the results for that soil are therefore more applicable for making fertilizer recommendations for organic grain crops.
Fertilizer experiments with organic residues containing several nutrients are often designed to study the effect of one element at a time. This is usually achieved by adding excess amounts of the other nutrients, to ensure that they do not limit crop growth in any treatment. This was not done in this experiment, since we wanted to study the total effect of different pellet placements. However, we assumed that the main limiting nutrient would be nitrogen and we therefore chose a nitrogen fertilizer (without P and K) for comparison (treatment 2). However, the high MFE values (>100%) in some experiments (Table 5) indicate that other elements may have limited yield in treatment 2. This was especially the case in the experiment on sandy loam in 2015, where treatment 10, with the same pellet placement as the mineral fertilizer in treatment 2, had a MFE value of 160%. That field had a low potassium value (Table 1), suggesting there was a potassium fertilization effect from the pellets in addition to the nitrogen effect. The higher MFE value in treatments other than treatment 10 could be partly attributable to better placement of fertilizer in these treatments than in the control (treatment 2).
No weed hoeing was conducted at early growth stages in this study, since we wanted to see how weeds were affected by crop fertilization. However, weeds were removed at panicle emergence, so they did not affect subsequent yield. In addition to weed density, weed hoeing could affect pellet incorporation, as pellets placed mid-row at shallow depth could be incorporated into soil, which could potentially affect nutrient availability. Other studies have examined weed survival after hoeing depending on fertilization, with variable results. For example, Melander et al. (2002) obtained higher yield of winter wheat when nitrogen fertilizer was incorporated into soil, but no effect on weeds surviving hoeing. Rasmussen (2002) studied the effect of weed control depending on slurry application strategy to spring cereals and found that both mechanical and chemical weed control were more efficient if the slurry was injected rather than surface-applied. In barley, weed numbers were reduced with slurry injection and no additional weed control measures, which could be explained by earlier crop development with injection.
According to Petersen (2005), rapid and high N utilization by the crop and low N uptake by weeds can be achieved by high seed density, short distance between crop row and band-applied slurry, and/or early seeding. The treatment with conventional crop row spacing (13: 12.5 cm) in this study confirmed that it often gave higher yield than the double spacing when fertilization was performed in the same way (treatment 12). However, this difference was much smaller than that caused by fertilizer placement, especially on the sandy loam. If pellets were subsurface-banded to oats with 12.5 cm spacing, distance from the crop row should be less important since it can never be more than 6.25 cm. Band application would probably still be as successful as in 25 cm spacing, as long as pellets are not put together with the seeds. The benefit could be because of more efficient incorporation and lower immobilization (Sørensen and Jensen, 1995; Delin and Strömberg, 2011).
Incorporation of organic fertilizers into soil is often justified by its reducing effect on ammonia emissions (Webb et al., 2010). However, ammonia emissions are not considered a risk with pelleted fertilizer (Adeli et al., 2012), and incorporation is motivated instead by the assumption that the pellets need close contact with moist soil for the nutrients to be released and accessible to crop roots. We suspected that incorporation would not be needed in wetter conditions, since the pellets would enter moist soil even with surface broadcasting, so we included two treatments with irrigation directly after fertilization. However, we found that the effect of subsurface banding persisted in irrigated soil. The effect of subsurface banding can therefore not be explained by incorporation into moister soil, but rather to a better position for crop roots to reach the nutrients.
The results confirm that placement of pelleted MBM benefits yield compared with broadcasting. Similar findings have been made for mineral fertilizers, e.g., a study by Rasmussen et al. (1996) found 28% higher yield in spring barley with placement compared with broadcasting. Distance of placement of mineral fertilizer from the row did not have a consistent effect on final yield in previous investigations (Petersen, 2001), although crop nitrogen uptake was delayed by 0.5 day for every 1 cm increase in distance from the crop row. The magnitude of the delay and whether it makes a difference for crop nutrient uptake probably vary depending on soil properties, climate conditions, and competition for nutrients from weeds and microorganisms. In our experiments, yield was reduced by on average 60 kg ha−1 per cm increase in distance when comparing yield in treatments with placement 4 and 12.5 cm from crop row (Figure 1), but the variation depending on year, site, and incorporation depth was 20–200 kg ha−1. Previous studies examining the effects of placement of organic fertilizers to spring cereals have mainly focused on animal slurry. Petersen (2003) reported an increase in crop N recovery in spring barley from 45 to 50 % when using band injection of pig slurry instead of broadcasting. A similar increase in N uptake was observed in the present study, where about 5–15 kg more N was taken up in the crop with optimal placement compared with broadcasting (Figure 3), which corresponds to around 10% of the total crop N uptake. Similarly to Rasmussen et al. (1996), we found that the effects on weeds were primarily secondary, i.e., that a well-fertilized crop competed better against weeds. In contrast, Blackshaw (2005), who studied yield of spring wheat and weed density of different weeds, found significant effects on weed biomass in some cases, without any significant effects on crop yield. The effects on weeds are most likely a combination of fertilization and competition with the crop.
Placement of organic fertilizers with centimeter precision may sound impracticable at farm level. However, with modern technology such as System Cameleon (Gothia Redskap, Fornåsa Sweden), a multifunctional system designed for precision seeding, fertilization, and weeding in organic crop production, this is now a reality. The findings in this study should be useful to guide the technological development of such machinery in the right direction and to assist farmers using such systems. In parallel with our microplot experiments, we performed field experiments with some selected treatments on organic farms using their field equipment. We also performed a few field experiments with a combidrill in experimental plots at a silty clay site. These experiments confirmed that grain yield increases with subsurface banding compared with broadcast, as shown in the microplots. The effect of placement distance from the crop row was more difficult to evaluate in these experiments, with few treatments to compare and with different set-ups in different experiments. However, although not statistically significant, placement 4 cm from the crop row tended to give higher yield than placement midway between rows, while placement under the crop row resulted in earlier crop nitrogen uptake than placement midway between rows. However, in one experiment in a farmer's field, placement midway between rows ultimately resulted in higher yield than placement under the crop row. This could be related to dry weather in that year, in combination with placement under the row negatively affecting moisture conditions for crop roots. Another possible explanation is that the weed hoeing performed by the farmer incorporated and mixed the pellets well into the soil and thus had a beneficial effect on nitrogen release from the pellets placed midway between the rows.
The results of this study show that farmers who use equipment for precision placement of pelleted fertilizers can double the grain yield effect from their pelleted MBM compared with using broadcasting and shallow incorporation with a harrow. To achieve this, farmers should aim at placement about 4 cm from the crop row and with at least 4 cm soil incorporation. These effects do not seem to be dependent on moisture conditions. The effect of pellet placement on weed density is small, with a highly competitive crop appearing to be more important for reducing weed plant size than limited weed access to nutrients.
The raw data supporting the conclusions of this manuscript will be made available by the authors, without undue reservation, to any qualified researcher.
AL, SD, and LE designed the study; LE and SD performed the experiments; SD and LE analyzed and/or interpreted the data. SD wrote the manuscript; LE and AL revised the manuscript for important intellectual content. All authors approved the final version of the manuscript and agree to be accountable for the content of the work.
The authors declare that the research was conducted in the absence of any commercial or financial relationships that could be construed as a potential conflict of interest.
The study was funded by SLU EkoFOrsk, Sweden. The authors wish to thank Per Ståhl, Emil Olsson, and Roland Höckert for valuable input from the farmers' viewpoint when setting up objectives and designing experiments. We also wish to thank the staff at Lanna Research station for providing field sites and practical assistance. Many thanks to Markus Delin, Johanna Wetterlind, Karin Wallin, and May Ibrahim for help with counting and harvesting weeds in a busy period.
Adeli, A., Tewolde, H., and Jenkins, J. N. (2012). Broiler litter type and placement effects on corn growth, nitrogen utilization, and residual soil nitrate-nitrogen in a no-till field. Agron. J. 104, 43–48. doi: 10.2134/agronj2011.0093
Baitilwake, M. A., Salomez, J., Mrema, J. P., and de Neve, S. (2012). Nitrogen mineralization of two manures as influenced by contrasting application methods under laboratory conditions. Commun. Soil Sci. Plant Anal. 43, 357–367. doi: 10.1080/00103624.2012.641473
Bittman, S., Liu, A., Hunt, D. E., Forge, T. A., Kowalenko, C. G., Chantigny, M. H., et al. (2012). Precision placement of separated dairy sludge improves early phosphorus nutrition and growth in corn (Zea mays L.). J. Environ. Qual. 41, 582–591. doi: 10.2134/jeq2011.0284
Blackshaw, R. E. (2005). Nitrogen fertilizer, manure, and compost effects on weed growth and competition with spring wheat. Agron. J. 97, 1612–1621. doi: 10.2134/agronj2005.0155
Delin, S., and Engström, L. (2010). Timing of organic fertilizer application to synchronise nitrogen supply with crop demand. Acta agriculturae scandinavica. Sec. B Soil Plant Sci. 60, 78–88. doi: 10.1080/09064710802631943
Delin, S., Stenberg, B., Nyberg, A., and Brohede, L. (2012). Potential methods for estimating nitrogen fertilizer value of organic residues. Soil Use Manag. 28, 283–291. doi: 10.1111/j.1475-2743.2012.00417.x
Delin, S., and Strömberg, N. (2011). Imaging-optode measurements of ammonium distribution in soil after different manure amendments. Eur. J. Soil Sci. 62, 295–304. doi: 10.1111/j.1365-2389.2010.01326.x
Hiltbrunner, J., Liedgens, M., Stamp, P., and Streit, B. (2005). Effects of row spacing and liquid manure on directly drilled winter wheat in organic farming. Eur. J. Agron. 22, 441–447. doi: 10.1016/j.eja.2004.06.003
Jensen, L. S. (2013). “Animal manure fertiliser value, crop utilisation and soil quality impacts,” in Animal Manure Recycling: Treatment and Management Wiley, eds S. G. Sommer, M. L.Christensen, T. Schnidt, and L. S Jensen (Somerset, NJ: John Wiley & Sons), 295–328.
Melander, B., Cirujeda, A., and Jørgensen, M. H. (2002). Effects of inter-row hoeing and fertilizer placement on weed growth and yield of winter wheat. Weed Res. 43, 428–438. doi: 10.1046/j.0043-1737.2003.00359.x
Petersen, J. (2001). Recovery of 15N-ammonium- 15N-nitrate in spring wheat as affected by placement geometry of the fertilizer band. Nutr. Cycl. Agroecosyst. 61, 215–221. doi: 10.1023/A:1013756715438
Petersen, J. (2003). Weed:spring barley competition for applied nitrogen in pig slurry. Weed Res. 43, 33–39. doi: 10.1046/j.1365-3180.2003.00314.x
Petersen, J. (2005). Competition between weeds and spring wheat for 15N-labelled nitrogen applied in pig slurry. Weed Res. 45, 103–113. doi: 10.1111/j.1365-3180.2004.00429.x
Rasmussen, K. (2002). Influence of liquid manure application method on weed control in spring cereals. Weed Res. 42, 287–298. doi: 10.1046/j.1365-3180.2002.00286.x
Rasmussen, K., Rasmussen, J., and Petersen, J. (1996). Effects of fertilizer placement on weeds in weed harrowed spring barley. Acta Agr. Scandinavica Sect. B 46, 192–196. doi: 10.1080/09064719609413133
Schröder, J. J., Ten Holte, L., and Brouwer, G. (1997). Response of silage maize to placement of cattle slurry. Netherlands J. Agr. Sci. 45, 249–261.
Sørensen, P., and Amato, M. (2002). Remineralization and residual effects of N after application of pig slurry to soil. Eur. J. Agron. 16, 81–95. doi: 10.1016/S1161-0301(01)00119-8
Sørensen, P., and Jensen, E. S. (1995). Mineralization-immobilization and plant uptake of nitrogen as influenced by the spatial distribution of cattle slurry in soils of different texture. Plant Soil 173, 283–291. doi: 10.1007/BF00011466
Webb, J., Pain, B., Bittman, S., and Morgan, J. (2010). The impacts of manure application methods on emissions of ammonia, nitrous oxide and on crop response—A review. Agr. Ecosyst. Environ. 137, 39–46. doi: 10.1016/j.agee.2010.01.001
Keywords: meat bone meal, organic fertilizer, fertilizer banding, soil incorporation, organic grain production
Citation: Delin S, Engström L and Lundkvist A (2018) Optimal Placement of Meat Bone Meal Pellets to Spring Oats. Front. Sustain. Food Syst. 2:27. doi: 10.3389/fsufs.2018.00027
Received: 01 February 2018; Accepted: 31 May 2018;
Published: 19 June 2018.
Edited by:
Claudia Wagner-Riddle, University of Guelph, CanadaReviewed by:
Eliana Lanfranca Tassi, Istituto per lo studio degli ecosistemi (ISE), ItalyCopyright © 2018 Delin, Engström and Lundkvist. This is an open-access article distributed under the terms of the Creative Commons Attribution License (CC BY). The use, distribution or reproduction in other forums is permitted, provided the original author(s) and the copyright owner are credited and that the original publication in this journal is cited, in accordance with accepted academic practice. No use, distribution or reproduction is permitted which does not comply with these terms.
*Correspondence: Sofia Delin, c29maWEuZGVsaW5Ac2x1LnNl
Disclaimer: All claims expressed in this article are solely those of the authors and do not necessarily represent those of their affiliated organizations, or those of the publisher, the editors and the reviewers. Any product that may be evaluated in this article or claim that may be made by its manufacturer is not guaranteed or endorsed by the publisher.
Research integrity at Frontiers
Learn more about the work of our research integrity team to safeguard the quality of each article we publish.