- Scripps Institution of Oceanography, University of California San Diego, San Diego, CA, United States
A Viewpoint on the Frontiers in Science Lead Article
Emerging signals of climate change from the equator to the poles: new insights into a warming world
Key points
- Atmospheric circulation patterns are important for inducing extreme weather/climate events.
- Improved understanding of how atmospheric circulation responds to a warming climate is necessary to sharpen the accuracy of regional climate change projections.
- Coupled ocean–atmosphere feedback is an important part of shaping regional climate change patterns.
Each summer, record-shattering heat extremes are reported around the world. On 25 July 2019, Paris recorded an all-time high temperature of 42.6°C. On 28 June 2021, Portland, Oregon, set a new temperature record of 47°C. The background warming caused by the greenhouse effect is the culprit behind the increased frequency and severity of heat waves. Hot and dry conditions are conducive to wildfires. Widespread bushfires ravaged Australia from June 2019 to January 2020, driving koalas and kangaroos away from their habitats. Smoke from the fires darkened the skies of New Zealand. In 2020, fires in California burned a record 10.2 million acres, equivalent in area to the US state of Rhode Island, while explosive fires on Maui Island, Hawaii, shocked the world in August 2023. Such heat-related extreme events have severe socio-economic and health impacts, contributing to a growing public perception and awareness of the threats posed by ongoing global warming.
Global mean surface temperature increase is the direct consequence of increased concentrations of heat-trapping greenhouse gases (GHGs) in the atmosphere. It is a good approximation of regional temperature responses, especially for local summer climates when the atmospheric circulation effect is small and heat extremes have a large impact on the world around us. Heat extremes emerge quickly—the probability of exceeding an extreme temperature threshold increases exponentially with mean warming. In addition, so-called thermodynamic variables (such as humidity) are increasing functions of temperature, and these are expected to increase further in a warming climate. Other thermodynamic effects include extreme rainfall due to atmospheric rivers impinging on coastal mountain ranges and snowpacks, which are an important source of fresh water for the state of California, for example. In a warmer climate, atmospheric rivers and the associated rainfall on the West Coast of the United States are likely to become more intense. Meanwhile, the snowpacks in the western mountains are likely to become smaller and melt earlier, resulting in a longer dry season that exacerbates wildfires.
Vapor pressure deficit is defined as the difference between saturation and measured water vapor pressure (e), VPD ≡ es – e = (1 – RH) es, where RH is the relative humidity. It is a measure of dryness and is highly correlated with wildfire risk. An increase in temperature results in an increase in vapor pressure deficit,
as relative humidity tends to decrease over land in response to the greenhouse warming. Here α = 0.07 K−1. Thus, the risk of fire rises as a direct and indirect consequence of warming.
Extreme events such as heat waves are usually associated with deep and long-lasting blocking highs in the westerly flow while atmospheric rivers are part of extratropical cyclones. The vertically integrated vapor transport, IVT = [vq], in atmospheric rivers increases with a warming climate (thermodynamic Δq effect) but also responds to atmospheric wind change (dynamic Δv effect), ΔIVT = [vΔq + qΔv], where the brackets denote the vertical atmospheric column integral. Flow dynamics are complex and influenced by multiple factors: while the thermodynamic effect of the slow background warming is robust and elevates the risk of extreme heat, fires, and heavy rainfall, the way the flow field that gives rise to the extreme events will change in warmer climates is not as obvious. A dynamic response to global warming is thus key to addressing important questions such as “How does the Walker circulation change?”, “Will El Niño–Southern Oscillation (ENSO) become stronger?”, “Will there be more hurricanes in the North Atlantic?”, and “How do extratropical storm tracks change?”
Collins et al. (1) highlighted several robust changes in atmospheric circulation that have emerged from recent studies in the United Kingdom and elsewhere. Extratropical cyclones are important for the United Kingdom and many extratropical countries. The Southern Hemisphere storm tracks are projected to shift poleward because of an increased poleward temperature gradient in both the upper and lower troposphere due respectively to elevated tropical warming and muted surface warming in the upwelling zone of the Southern Ocean. In the Northern Hemisphere, storm track change is complex because the amplified surface warming in the Arctic opposes the increased temperature gradient in the upper troposphere. Robust poleward shifts in storm tracks are limited to the North Pacific (2).
Tropical cyclones (TCs), known as typhoons in the Northwest Pacific and hurricanes in the North Atlantic and Northeast Pacific, are highly organized deep convection. The maximum intensity TCs can reach is an increasing function of sea surface temperature (SST), and the most intense TCs are thus expected to become stronger and more destructive in a warmer climate. The frequency of occurrences (basin counts or track densities) is regulated by local convective instability or SST relative to the tropical mean (3) because of the weak horizontal temperature gradient in the free troposphere in the deep tropics.
Observations of year-to-year variability over the past century illustrate that the tropical SST pattern is important for tropical rainfall and TCs as well as for extratropical storm tracks: for example, the southwestern United States is rainier and stormier during El Niño than La Niña winters. The so-called El Niño-like SST change is a robust pattern induced by increased atmospheric CO2 over several generations of global climate models with a reduced East–West gradient across the equatorial Pacific (Figure 1). This SST pattern results from reduced evaporative damping over the equatorial cold tongue (due to low SSTs and weak surface winds in the background state) and is associated with weakened easterly trades, which is consistent with the Bjerknes feedback. The enhanced eastern equatorial Pacific warming has further been shown to amplify ENSO and its remote influence on southwestern United States winter rainfall in a warmer climate (4).
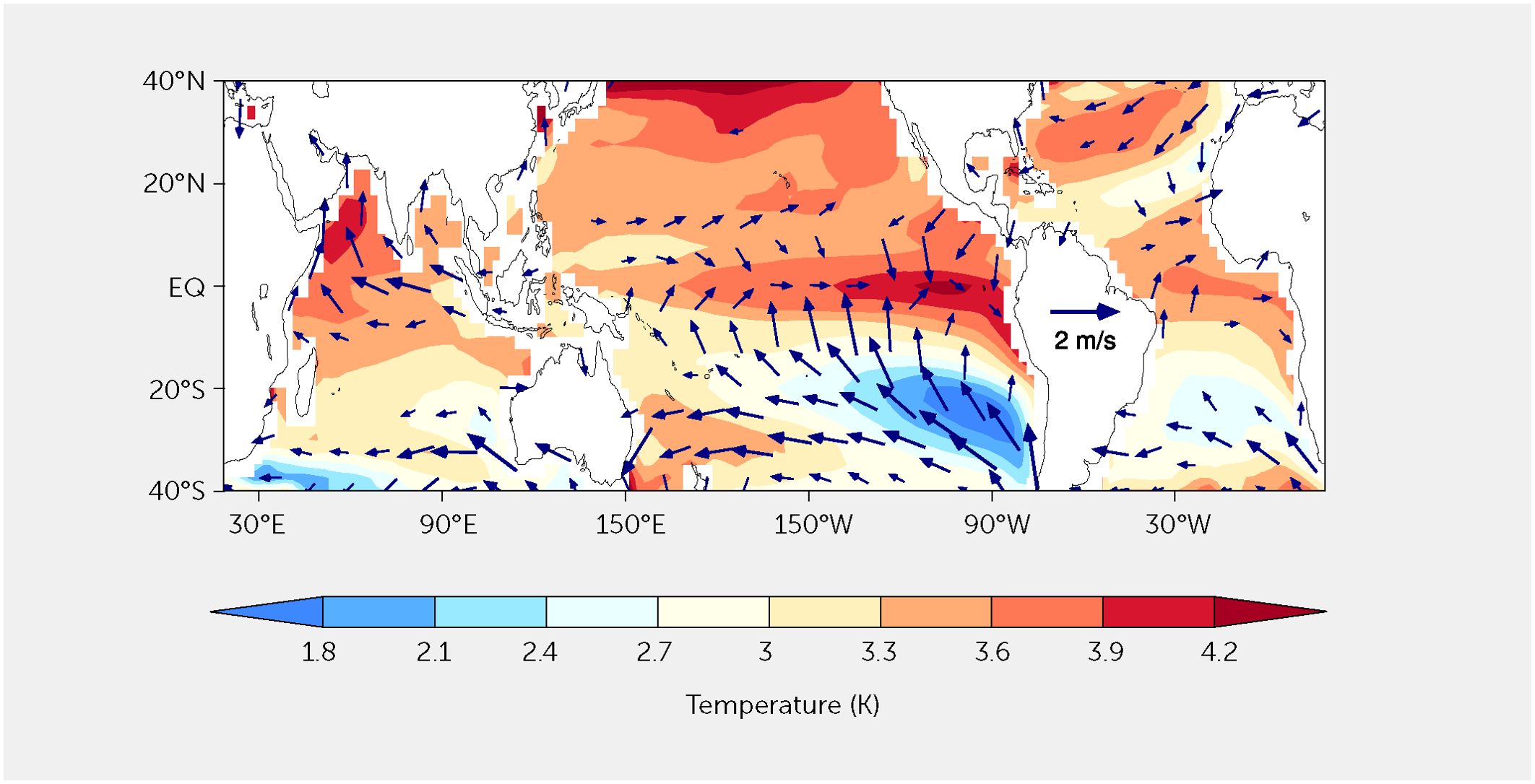
Figure 1. Patterns of climate change at the air–sea interface. Sea surface temperature (°C) and surface wind (m/s) change in the 1% carbon dioxide (CO2) run at year 140 (the time of 4xCO2) based on the 31-model ensemble mean of the Coupled Model Intercomparison Project, phase 6. Adapted from (12).
Satellite-based remote sensing fills important gaps in in situ observations, especially over remote oceans. During the satellite era (which nominally started in 1980), the Eastern Tropical Pacific Ocean did not warm up above the noise of large internal variability (e.g., ENSO) against a backdrop of robust warming over the rest of the tropics. The apparent discrepancy of the El Niño-like warming simulated by climate models could be due to several reasons, ranging from insufficient representation of the effect of ocean upwelling damping on SST increase (5), anthropogenic aerosol cooling (6), freshwater discharges from melting Antarctic ice sheets (7), and internal Pacific multi-decadal variability. This is a hot topic under intense debate (8). Much hangs in the balance as a large portion of the projected change in the literature is based on the El Niño-like warming pattern, including tropical and extratropical cyclones (5), ENSO, and ENSO’s teleconnection. Coincidentally, many of these changes are associated with atmospheric flows and interactions with the ocean.
Just a decade ago, climate models mutually disagreed on how ENSO would respond to global warming. The Intergovernmental Panel on Climate Change Fifth Assessment Report concluded that “confidence in any specific projected change in (ENSO) variability in the 21st century remains low” (9). Now, a consensus has started forming from model simulations that ENSO is likely to strengthen through the middle of this century and then weaken if GHG emissions were not mitigated (10, 11), and useful diagnostic tools have been developed to explain the non-monotonic ENSO change. This model projection, however, is being confronted with a discrepancy, for the satellite era at least, in the warming pattern and Walker circulation change between models and observations. The approach that views the ocean and atmosphere as a coupled system spurred rapid advances during the 1980s in our understanding and modeling of climate variability, culminating in successful predictions of ENSO with dynamical models. Research for the past decade has led to the recognition that coupled ocean–atmospheric feedback is key to determining regional patterns of climate change (Figure 1) in response to spatially “uniform” GHG forcing (12). With expanding observations and advanced modeling, the coupled feedback view could help identify key ocean–atmospheric processes and accelerate progress in regional climate dynamics, sharpening the accuracy of projected changes in the atmospheric circulation and modes of climate variability such as ENSO.
Statements
Author contributions
SX: Writing – review & editing, Writing – original draft, Conceptualization.
Funding
The author(s) declare financial support was received for the research, authorship, and/or publication of this article. This study received funding from the National Science Foundation (AGS 2105654). The funder was not involved in the study design, collection, analysis, interpretation of data, the writing of this article or the decision to submit it for publication.
Conflict of interest
The author declares that the research was conducted in the absence of financial relationships that could be construed as a potential conflict of interest.
The author declared a past co-authorship with the lead article authors AS, PH to the handling editor.
Publisher’s note
All claims expressed in this article are solely those of the authors and do not necessarily represent those of their affiliated organizations, or those of the publisher, the editors and the reviewers. Any product that may be evaluated in this article, or claim that may be made by its manufacturer, is not guaranteed or endorsed by the publisher.
References
1. Collins M, Beverley JD, Bracegirdle TJ, Catto J, McCrystall M, Dittus A, et al. Emerging signals of climate change from the equator to the poles: new insights on a warming world. Front Sci (2024) 2:1340323. doi: 10.3389/fsci.2024.1340323
2. Priestley MDK, Catto JL. Future changes in the extratropical storm tracks and cyclone intensity, wind speed, and structure. Weather Clim Dynam (2022) 3(1):337–60. doi: 10.5194/wcd-3-337-2022
3. Vecchi GA, Soden BJ. Effect of remote sea surface temperature change on tropical cyclone potential intensity. Nature (2007) 450(7172):1066–70. doi: 10.1038/nature06423
4. Zhou Z-Q, Xie S-P, Zheng X-T, Liu Q, Wang H. Global warming-induced changes in El Niño teleconnections over the North Pacific and North America. J Clim (2014) 27(24):9050–64. doi: 10.1175/JCLI-D-14-00254.1
5. Sobel AH, Lee CY, Bowen SG, Camargo SJ, Cane MA, Clement A, et al. Near-term tropical cyclone risk and coupled Earth system model biases. Proc Natl Acad Sci USA (2023) 120(33):e2209631120. doi: 10.1073/pnas.2209631120
6. Kang SM, Xie S-P, Deser C, Xiang B. Zonal mean and shift modes of historical climate response to evolving aerosol distribution. Sci Bull (2021) 66(23):2405–11. doi: 10.1016/j.scib.2021.07.013
7. Dong Y, Armour KC, Battisti DS, Blanchard-Wrigglesworth E. Two-way teleconnections between the Southern Ocean and the tropical Pacific via a dynamic feedback. J Clim (2022) 35(19):6267–82. doi: 10.1175/JCLI-D-22-0080.1
8. Watanabe M, Kang SM, Collins M, Hwang YT, McGregor S, Stuecker MF. Possible shift in controls of the tropical Pacific surface warming pattern. Nature (2024) 630(8016):315–24. doi: 10.1038/s41586-024-07452-7
9. Christensen JH, Krishna Kumar K, Aldrian E, An SI, Cavalcanti IFA, de Castro M, et al. Climate phenomena and their relevance for future regional climate change. In: Stocker TF, Qin D, Plattner GK, Tignor M, Allen SK, Boschung J, et al, editors. Climate Change 2013: The physical science basis. Contribution of Working Group I to the fifth assessment report of the Intergovernmental Panel on Climate Change. Cambridge: Cambridge University Press (2013) 1217–308. doi: 10.1017/CBO9781107415324.028
10. Peng Q, Xie S-P, Deser C. Collapsed upwelling projected to weaken ENSO under sustained warming beyond the twenty-first century. Nat Clim Chang (2024) 14(8):815–22. doi: 10.1038/s41558-024-02061-8
11. Geng T, Cai W, Jia F, Wu L. Decreased ENSO post-2100 in response to formation of a permanent El Niño-like state under greenhouse warming. Nat Commun (2024) 15(1):5810. doi: 10.1038/s41467-024-50156-9
Keywords: regional climate change, thermodynamic change, atmospheric circulation change, heat extremes, cyclones, El Niño, coupled ocean–atmosphere dynamics
Citation: Xie S-P. Chase the changing winds. Front Sci (2024) 2:1430737. doi: 10.3389/fsci.2024.1430737
Received: 10 May 2024; Accepted: 04 September 2024;
Published: 01 October 2024.
Edited and Reviewed by:
Moetasim Ashfaq, Oak Ridge National Laboratory (DOE), United StatesCopyright © 2024 Xie. This is an open-access article distributed under the terms of the Creative Commons Attribution License (CC BY). The use, distribution or reproduction in other forums is permitted, provided the original author(s) and the copyright owner(s) are credited and that the original publication in this journal is cited, in accordance with accepted academic practice. No use, distribution or reproduction is permitted which does not comply with these terms.
*Correspondence: Shang-Ping Xie, c3hpZUB1Y3NkLmVkdQ==