Explore article hub
- 1Faculty of Medicine, Allergy and Clinical Immunology, Transilvania University of Brasov, Brasov, Romania
- 2Swiss Institute of Allergy and Asthma Research (SIAF), University of Zurich, Davos, Switzerland
- 3Christine Kühne-Center for Allergy Research and Education (CK-CARE), Davos, Switzerland
- 4Crisis Decision Support Program, Environment and Life Sciences Research Center, Kuwait Institute for Scientific Research, Safat, Kuwait
- 5Institute Debrest of Epidemiology and Public Health, UMR1318 INSERM and Montpellier University, Montpellier, France
- 6Department of Pulmonology, Allergy and Thoracic Oncology, University Hospital of Montpellier, Montpellier, France
- 7Department of Medicine, University of California, San Francisco, San Francisco, CA, United States
- 8School of Public Health, University of California at Berkeley, Berkeley, CA, United States
- 9SOS Allergy and Clinical Immunology, USL Toscana Centro, Prato, Italy
- 10Terrestrial Ecology and Climate Change, Department of Ecology, School of Biology, Faculty of Sciences, Aristotle University of Thessaloniki, Thessaloniki, Greece
- 11Skin and Allergy Hospital, Helsinki University Hospital, University of Helsinki, Helsinki, Finland
- 12Department of Environmental Health, Harvard T.H. Chan School of Public Health, Harvard University, Boston, MA, United States
- 13Channing Division of Network Medicine, Department of Medicine, Brigham and Women’s Hospital, Harvard Medical School, Boston, MA, United States
- 14Department of Clinical Immunology, Wroclaw Medical University, Wroclaw, Poland
- 15ALL-MED Medical Research Institute, Wroclaw, Poland
- 16Department of Pediatrics, Kilimanjaro Clinical Research Institute, Kilimanjaro Christian Medical Centre, Moshi, Tanzania
- 17Kilimanjaro Christian Medical University College, Moshi, Tanzania
- 18Department of Pediatrics, Hanyang University College of Medicine, Seoul, Republic of Korea
- 19Department of Health in Emergencies and Disasters, School of Public Health, Tehran University of Medical Sciences, Tehran, Iran
- 20Department of Pediatrics, Nippon Medical School, Tokyo, Japan
- 21Institute of Laboratory Medicine and Pathobiochemistry, Philipps-University Marburg, Marburg, Germany
- 22Universities of Giessen and Marburg Lung Center (UGMLC), German Center for Lung Research (DZL), Giessen, Germany
- 23Pulmonary, Critical Care and Sleep Medicine, Department of Medicine, Beth Israel Deaconess Medical Center, Harvard Medical School, Boston, MA, United States
- 24Department of Pediatrics, Federal University of Parana, Curitiba, Brazil
- 25Department of Respiratory Medicine, Eastern Health & Monash University, Melbourne, VIC, Australia
- 26Environmental Medicine, Faculty of Medicine, University of Augsburg, Augsburg, Germany
- 27Institute of Environmental Medicine, Helmholtz Center Munich, German Research Center for Environmental Health, Augsburg, Germany
- 28Department of Pediatrics, Chinese University of Hong Kong, Hong Kong, Hong Kong SAR, China
Abstract
Global warming and climate change have increased the pollen burden and the frequency and intensity of wildfires, sand and dust storms, thunderstorms, and heatwaves—with concomitant increases in air pollution, heat stress, and flooding. These environmental stressors alter the human exposome and trigger complex immune responses. In parallel, pollutants, allergens, and other environmental factors increase the risks of skin and mucosal barrier disruption and microbial dysbiosis, while a loss of biodiversity and reduced exposure to microbial diversity impairs tolerogenic immune development. The resulting immune dysregulation is contributing to an increase in immune-mediated diseases such as asthma and other allergic diseases, autoimmune diseases, and cancer. It is now abundantly clear that multisectoral, multidisciplinary, and transborder efforts based on Planetary Health and One Health approaches (which consider the dependence of human health on the environment and natural ecosystems) are urgently needed to adapt to and mitigate the effects of climate change. Key actions include reducing emissions and improving air quality (through reduced fossil fuel use), providing safe housing (e.g., improving weatherization), improving diets (i.e., quality and diversity) and agricultural practices, and increasing environmental biodiversity and green spaces. There is also a pressing need for collaborative, multidisciplinary research to better understand the pathophysiology of immune diseases in the context of climate change. New data science techniques, biomarkers, and economic models should be used to measure the impact of climate change on immune health and disease, to inform mitigation and adaptation efforts, and to evaluate their effectiveness. Justice, equity, diversity, and inclusion (JEDI) considerations should be integral to these efforts to address disparities in the impact of climate change.
Key points
- Climate change is driving an increase in immune-mediated diseases such as asthma, allergies, autoimmune diseases, and cancers.
- Anthropogenically driven increases in pollen, wildfires, sand and dust storms, thunderstorms, and heatwaves—with concomitant increases in air pollution, heat stress, and flooding—are altering the human exposome and worsening human health.
- Multilevel, multisectoral adaptation and mitigation actions are vital to reduce emissions and improve air quality, provide safe housing, improve diets and agricultural practices, and increase environmental biodiversity and peoples’ exposure to natural environments.
- New biomarkers, data science approaches, and economic models are vital to better measure the impact of climate change on health.
- Mitigation and adaptation efforts need to be global, equitable, and recognize that the health of the planet is integrally connected to human health.
Introduction
Increased anthropogenic activity, including deforestation, urbanization, modern agricultural practices, manufacture of synthetic chemicals, overuse of fertilizers and antibiotics, and the use of fossil fuels and biomass for energy, have altered Earth’s environment. Global temperatures and the frequency and severity of climate change events such as heatwaves, wildfires, sand and dust storms (SDSs), and thunderstorms have all increased over recent decades (1). These events further increase global warming. For example, wildfires release large amounts of greenhouse gases (GHGs) further contributing to air pollution and global warming, and accelerating the vicious climate feedback loop. Climate change events lead to increases in pollen burden, air pollution, flooding, and heat stress, which physically, chemically, and biologically alter the human exposome (i.e., the cumulative environmental exposures encountered by an individual in a lifetime). Wider environmental determinants of health are also affected by climate change, including biodiversity loss and access to nutritious food, clean drinking water, and secure shelter (Figure 1).
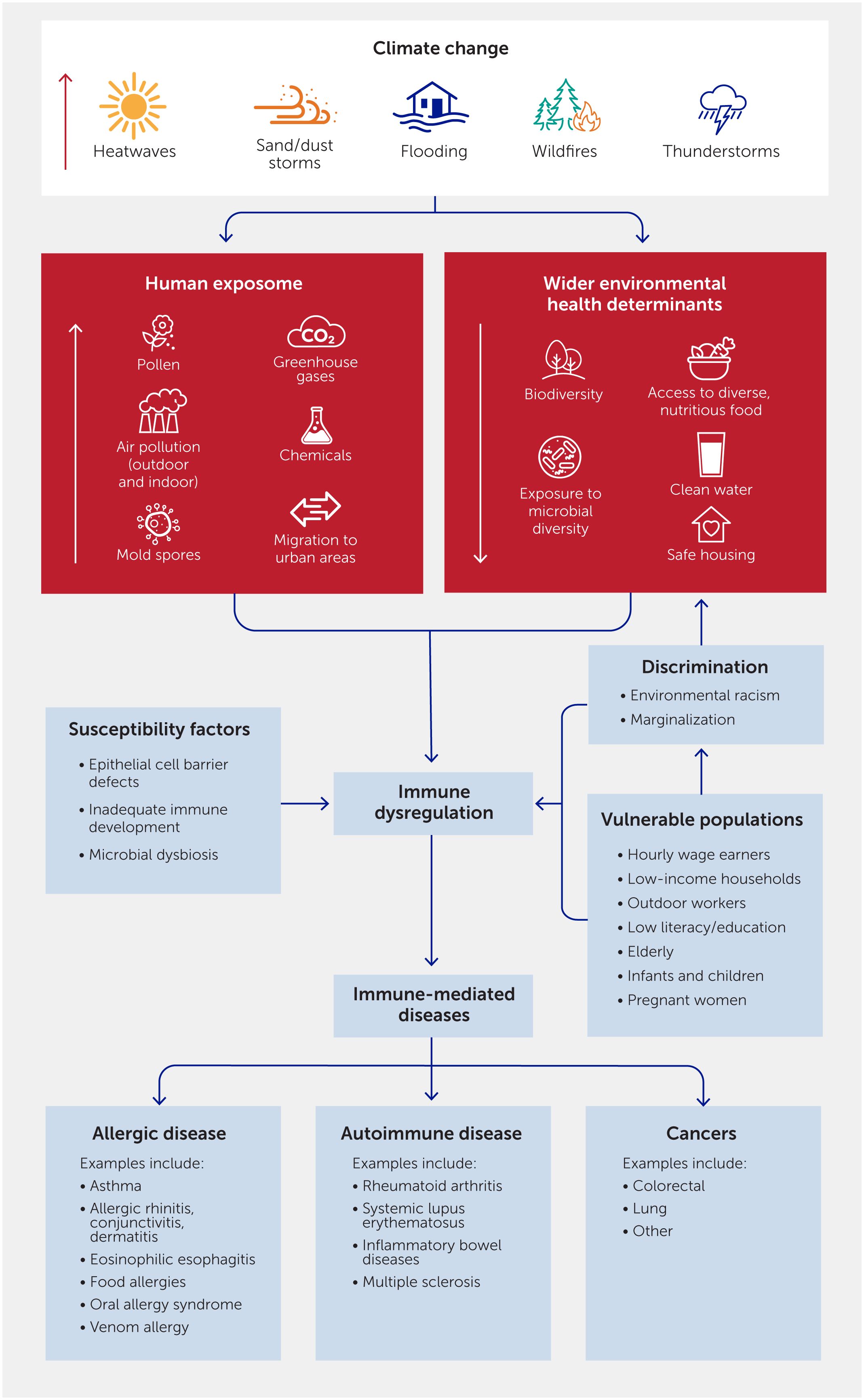
Figure 1 Effects of climate change-related events on immune dysregulation and human health through immune-mediated conditions. Climate change increases the frequency and severity of various types of events that affect the human exposome (the totality of a person’s lifetime exposures). The resulting immune dysregulation can cause a variety of immune-mediated conditions such as allergies, asthma, autoimmune diseases, and cancers. These risks are increased by susceptibility factors in individuals and within vulnerable populations.
Humans have evolved an immune system to protect against environmental assaults and maintain health. The epithelial barriers of the gut, lungs, and skin are the body’s first line of defense. Immune cells within the epithelial layer determine the extent of the threat posed by foreign invaders and mount a response. However, overreaction and hypersensitivity of the immune system leads to immune dysregulation, which can result in allergic diseases, autoimmune diseases, and cancer—conditions whose prevalence rates have increased in recent decades (2–4). In recent decades, increased urbanization, antibiotic use, and exposure to toxic chemicals—coupled with decreased exposure to biodiverse environments, less diverse diets, and a lack of physical activity—have created conditions that increase the risks of skin and mucosal barrier disruption, microbial dysbiosis, and immune dysregulation in populations globally (5). The epithelial barrier hypothesis proposes that increases in exposure to air pollutants and other toxic substances in the air, water, and foodstuffs (such as detergents, household cleaners, food emulsifiers, preservatives, pesticides, and microplastics) damage the epithelial barrier. This damage increases the penetration of allergens and microbes, leading to increases in pro-inflammatory reactions (6). A defective epithelial barrier has been demonstrated in asthma and allergic diseases (7). In addition, gut barrier defects and microbial dysbiosis have been demonstrated in many allergic and autoimmune diseases and cancers (8, 9). In parallel, a loss of biodiversity and limited exposure to microbial diversity increasingly impair tolerogenic immune development.
Major allergic diseases include allergic asthma, allergic rhinitis, atopic dermatitis, and food allergy. The estimated prevalence of allergies varies by age group, allergy type, geographical region, and season, and has changed over time. Methodological differences between studies can explain some of the observed variance in rates. However, the consensus is that, overall, there has been an upward trend in the prevalence of asthma and allergies (10–14). These diseases have a serious impact on individuals’ quality of life and incur substantial direct healthcare costs and indirect socioeconomic costs (15). Based on Global Cancer Observatory (GLOBOCAN) and World Health Organization (WHO) sources, cancer is a leading and increasing cause of mortality and morbidity worldwide. Globally, cancer caused approximately 10 million deaths in 2020, and it is the first or second leading cause of death in many countries (16, 17). The incidence of cancer in adults aged 50 years or younger has increased worldwide since the 1900s (18) and cancer risk has been linked to environmental factors such as air pollution (19, 20). Autoimmune diseases include rheumatoid arthritis, systemic lupus erythematosus, inflammatory bowel diseases (such as ulcerative colitis and Crohn’s disease), and multiple sclerosis. The overall prevalence of autoimmunity is estimated to be approximately 3–5% in the general population. The incidence and prevalence of autoimmune diseases vary with age, gender, and ethnicity, with women being more at risk than men (21). These diverse conditions also incur a vast and rising disease burden globally (22) and accumulating evidence links their incidence to pollution (23).
Without mitigation of the environmental risk factors driven by climate change, further increases in allergic diseases, autoimmune diseases, and cancers are expected (24, 25). Global temperatures are 1.1°C higher than during prehistorical times and future global warming is projected to span a range from 1.4°C to 4.4°C by 2100, depending on different GHG emissions scenarios (26). The magnitude of the increase depends on the extent of human interventions aiming at (i) the reduction of fossil fuel use; (ii) the implementation of eco-friendly sustainable practices; and (iii) enforcing equitable socioeconomic factors. With the recognition of the imminent threats to health and the need for global cooperation, the 2015 Paris Agreement signed at the United Nations Climate Conference (COP) 21 aims to limit global warming to well below 2°C and as close to 1.5°C as possible by promoting transitions toward low-emission and climate-resilient development (27). Nevertheless, even an optimistic projected global temperature increase of 1.5°C will have major consequences for human health, including immune-mediated diseases. Many studies have shown that the adverse effects of climate change on disease risk are higher among those of a lower socioeconomic status, those of younger or older age, and those with comorbid diseases such as cardiovascular diseases, asthma, or other atopic diseases (28).
In this article, we review the links between climate change, environmental exposures, and immune dysregulation and its associated diseases. We then recommend adaptation and mitigation solutions to reduce the impact of climate change on human health via these conditions.
Inflammatory versus tolerogenic responses in allergy
Climate change-associated exposures can trigger a complex array of inflammatory or tolerogenic responses (Figure 2). In healthy individuals, the immune system mediates a tolerogenic response upon encountering common innocuous environmental factors. During immune dysregulation, the immune system mounts an inflammatory response, even to innocuous environmental factors or healthy cells in the body. The mechanisms involved in allergic reactions to pollen, food, or insect allergens are those best understood.
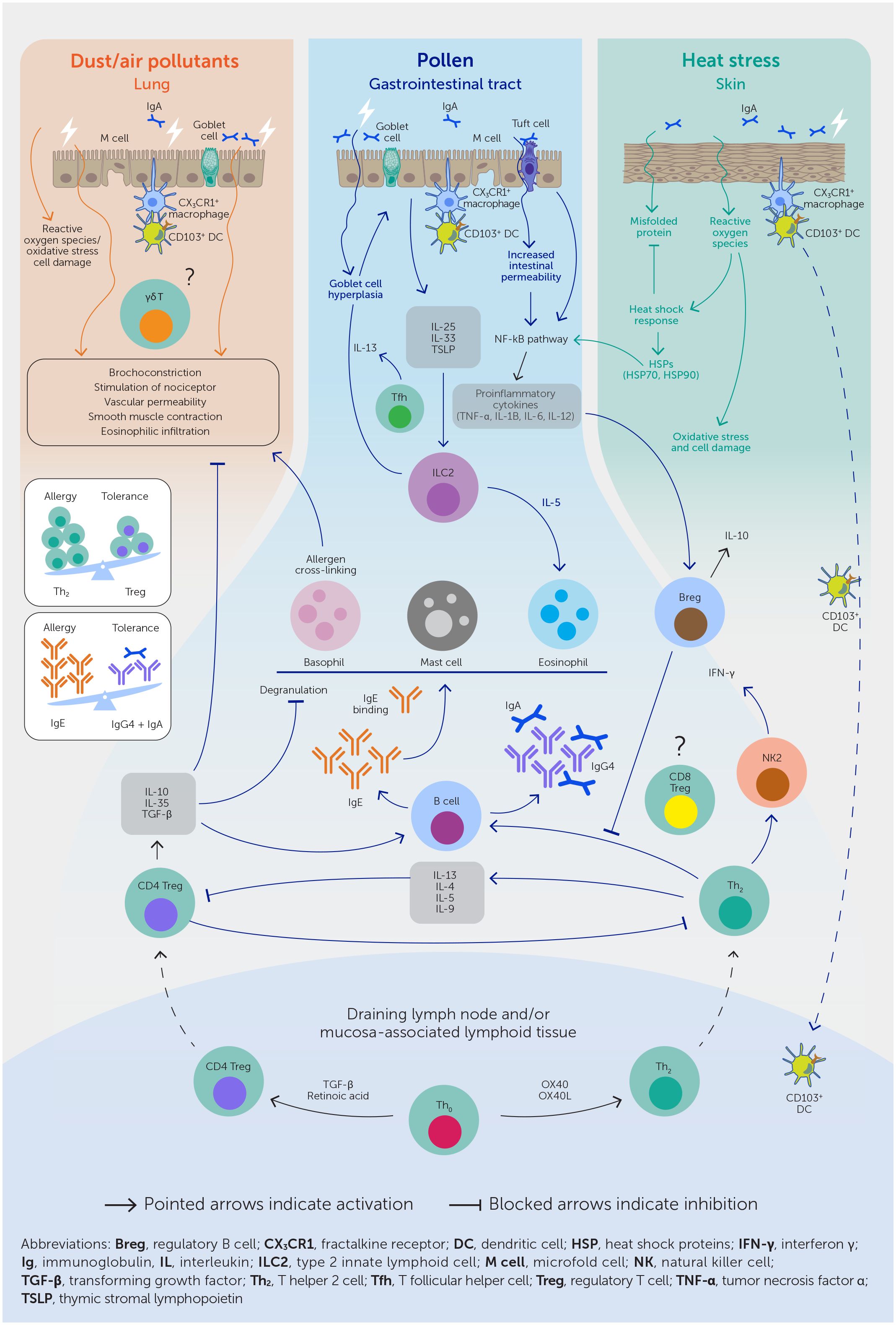
Figure 2 Climate change-associated exposures, such as pollen, air pollutants, and heat stress, trigger complex pathways mediating both inflammatory and tolerogenic responses. Penetration of allergens, pollutants, and other environmental stressors via a defective epithelial barrier leads to the release of pro-inflammatory cytokines such as interleukin (IL)-25, IL-33, and thymic stromal lymphopoietin (TSLP) and the skewing of T helper (Th) naïve cells to Th2 cells. Th2 cells mediate a pro-inflammatory cascade through cytokines IL-4, IL-5, IL-9, and IL-13, leading to a B cell isotype class switching to immunoglobulin (Ig)E. IgE bound to mast cells and basophils cross-link on subsequent allergen exposure, leading to the release of pro-inflammatory mediators such as histamine and prostaglandins. In allergies, these lead to enhanced vascular permeability, smooth muscle contraction, and eosinophilic infiltration, resulting in symptoms of bronchoconstriction. Heat stress and air pollutants work synergistically to mediate these pro-inflammatory effects. The immune system also actively mediates tolerogenic effects to commonplace environmental agents. Here, naïve Th cells are transformed into regulatory T cells (Tregs) which favor B cell isotype class switching to anti-inflammatory IgA and IgG4.
A defective epithelial barrier permits the entry of allergens, leading to the release of pro-inflammatory epithelium-derived cytokines such as interleukin (IL)-25, IL-33, and thymic stromal lymphopoietin (TSLP). These cytokines further prompt dendritic cells to transform naïve T helper (Th) cells into Th2 cells, which release pro-inflammatory cytokines such as IL-4, IL-5, IL-9, and IL-13. Epithelial cytokines also activate type 2 innate lymphoid cells (ILC2s) which also release pro-inflammatory cytokines IL-5 and IL-13. These cytokines favor B cell class switching to immunoglobulin (Ig)E. Allergic sensitization occurs when IgE binds to the high-affinity IgE receptor FcϵR1 on mast cells. In these individuals, subsequent exposure to allergens favors IgE cross-links and the release of preformed and de novo–synthesized pro-inflammatory mediators, such as histamine, prostaglandins, leukotrienes, and other cytokines. In allergies, these proinflammatory mediators enhance vascular permeability, smooth muscle contraction, and eosinophilic infiltration, resulting in symptoms of bronchoconstriction.
The immune system also mediates tolerogenic mechanisms. Here, naïve Th cells are transformed into tolerogenic regulatory T cells (Tregs) rather than inflammatory Th2 cells. Tregs favor B cell isotype class switching to IgA and IgG4, both of which block Th2 inflammatory responses (29). The environmental factors hypothesized to increase the likelihood of inflammatory rather than tolerogenic responses include microbial and epithelial barrier dysregulation, increased hygiene, early life avoidance of allergens, refined diet, and air pollution (30).
Climate change, pollen, and asthma
Changes in land use and climate, such as rising temperatures and nitrogen oxides (NOx), ozone (O3), and carbon dioxide (CO2) levels, have led to increased pollen quantity, quality, allergenicity, and duration of the pollen season (Table 1, Figure 3) (38, 41). In one study, the production of allergenic pollen by ragweed increased by 61% when CO2 concentrations were doubled (42). Greenhouse and field studies indicate that pollen concentrations are correlated with temperature and some estimates suggest that pollen concentrations may increase by 200% by the end of the century (37). Most pollen types have shifted toward earlier times of the year for pollen outputs (e.g., ragweed), possibly aggravating the burden on pollen-allergic patients (37, 38). Nitrogen dioxide (NO2) and O3 have also been shown to damage Platanus pollen cell membranes, increase the concentration of the Platanus pollen allergen a 3 released into the atmosphere, and alter its protein structure via nitrification and oxidation—enhancing its immunogenicity and stability (43).
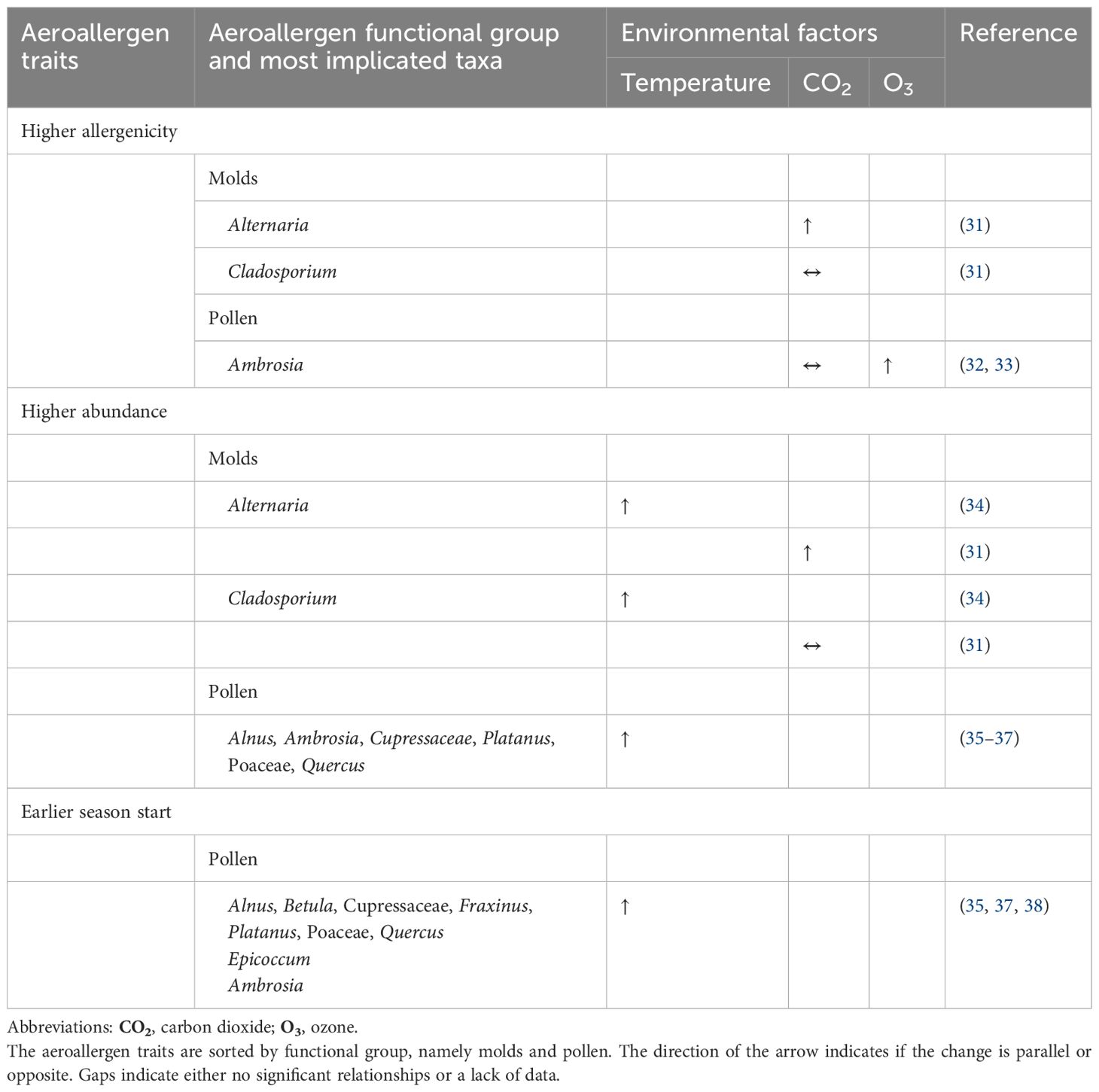
Table 1 Overview of the environmental factors influencing the allergenicity, abundance, and seasonality of aeroallergens (airborne pollen and fungal spores).
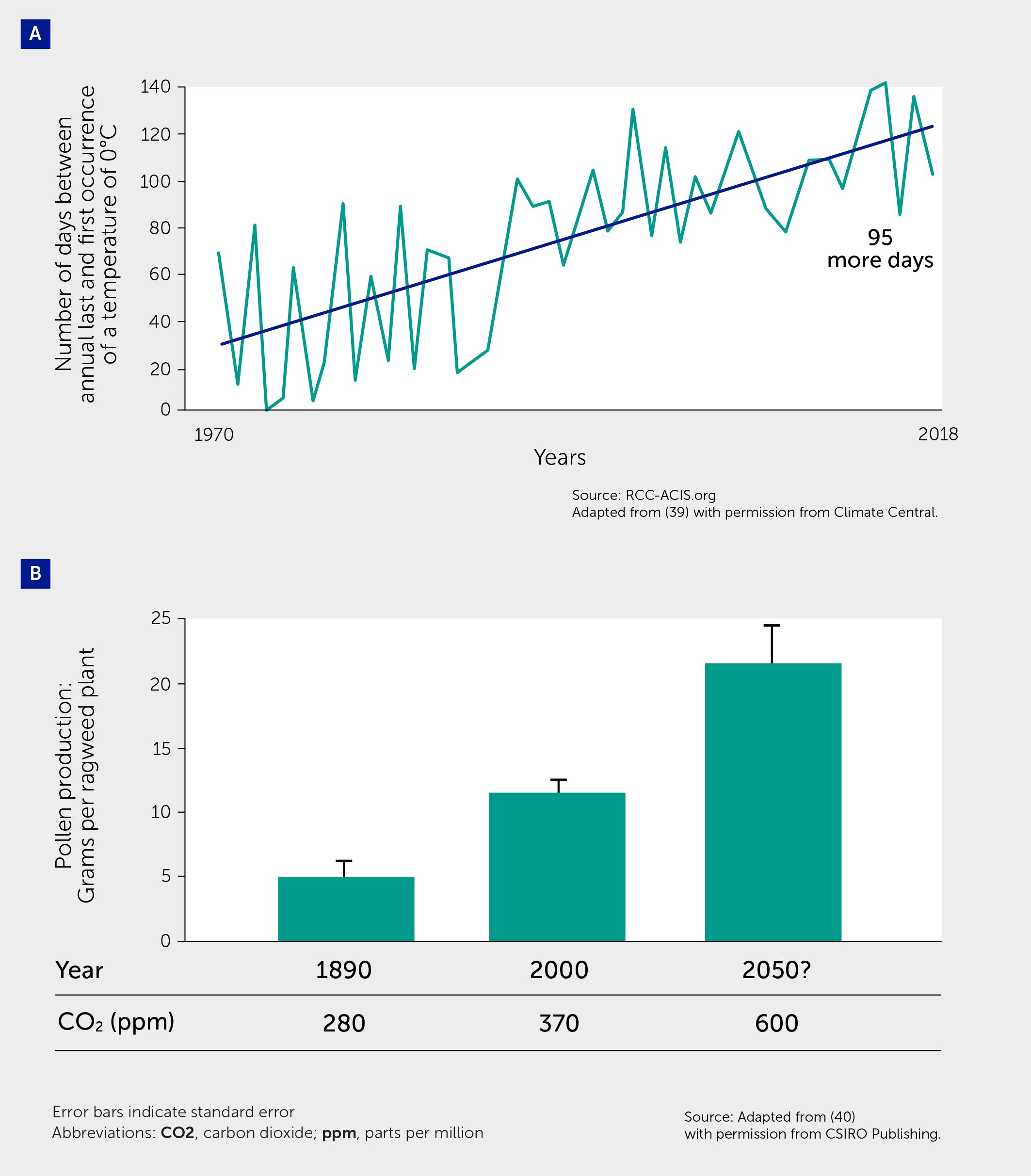
Figure 3 Climate change effects on pollen. (A) Global warming is causing longer growing seasons, which in turn leads to longer allergy seasons that start earlier in spring and last later into autumn. The figure shows data from 201 cities in the United States, plotting the number of days between the annual last and first occurrence of a temperature of 0°C (32°F), i.e., the first and last freezes of the year, over time—indicating a lengthening of the growing season. Adapted from (39) with permission from Climate Central. (B) Pollen concentrations increase with increases in atmospheric carbon dioxide (CO2). Figure shows pollen production in common ragweed grown at pre-industrial CO2 concentrations (280 ppm), current concentrations (370 ppm) and a projected 21st century concentration (600 ppm). Error bars indicate the standard error. The Student–Newman–Keuls test was used to determine differences among the CO2 treatments at the 0.05 significance level. Adapted from (40) with permission from CSIRO Publishing.
The intensification of pollen exposure has important implications for public health. Increased allergenicity of pollen results in more severe symptoms in allergic individuals (32). There is increasing evidence correlating high pollen levels (especially grass pollen) with higher rates of asthma exacerbations and associated emergency department visits and hospitalization (44–46).
Furthermore, pollen is also affected by thunderstorms, leading to a phenomenon called thunderstorm asthma (TA) characterized by severe asthma attacks and asthma-related deaths in patients with allergic rhinitis. First described nearly 40 years ago, TA has been reported in North America, Europe, the Middle East, and Australia. TA events result from a complex interaction of environmental and individual susceptibility factors (47). Environmental factors include high concentrations of an aeroallergen and rain and moisture that rupture pollen grains and fungal spores, leading to the release of fine allergen-bearing starch granules that are <2.5 µm in size (48). Individual susceptibility factors include (i) pre-sensitization to seasonal aeroallergens; (ii) history of seasonal allergic rhinitis; and (iii) low rates of inhaled corticosteroid use in patients with allergic asthma (49). TA is also associated with fungal spores, such as Alternaria, whose proliferation is increased by floods and storms (47).
Air pollution
The 2019 Global Burden of Disease (GBD) study found that air pollution (outdoor and indoor) is estimated to contribute to about 10% of all noncommunicable disease deaths and is among the top three risk factors for death, with the burden of deaths predominantly in Asia and Africa (50, 51). The Lancet Commission on Pollution and Health linked air pollution to multiple adverse health conditions in children, including low birth weight; noncommunicable diseases such as asthma, cancer, and chronic obstructive pulmonary disease (COPD); and neurodevelopmental disorders (52, 53).
Global warming contributes to air pollution via increases in GHGs and other pollutants released by wildfires and SDSs, with important implications for immune and non-immune diseases (54–61).
Air pollution impacts both the innate and adaptive immune function in various ways (62) (Figure 2). Adaptive T cells such as CD8+ T and CD4+ T cells (Tregs, Th1, and Th2) are altered as are innate immune cells such as innate lymphoid cells type 2 (ILC2), dendritic cells, toll-like receptors (TLRs) and natural killer (NK) cells (63). A study found that ozone-oxidized black carbon caused necroptosis in macrophages, increased levels of reactive oxygen species (ROS), and the expression of inflammatory factors and chemokines (64). Recently, age-related declines in immune function were linked to the accumulation of inhaled atmospheric particulate matter (PM) specifically contained within lung macrophages, which exhibited decreased activation, phagocytic capacity, and altered cytokine production. PM also disrupted the structures of B cell follicles and lymphatic drainage in lung-associated lymph nodes (65). Exposure to wildfire smoke and traffic-related air pollution has been associated with the activation of arylhydrocarbon receptors, toll-like receptors, and nuclear factor (NF)-κB signaling, and the upregulation of pro-inflammatory cytokines such as IL-22 (66). Firefighters have increased IL-6 and IL-12 and decreased IL-10 in their serum 12 hours after exposure to a wildfire (67). The activation of NLRP3 inflammasome and pyroptosis by environmental pollutants has been correlated to numerous diseases (68, 69). For example, perfluoroalkyl substance pollutants have been shown to activate the innate immune system through the absent in melanoma 2 (AIM2) inflammasome (70). In another study, the inflammasome was shown to be activated by micro- and nanoplastics, which are increasingly found in the air (71).
Chronic inflammation, including that triggered by air pollution, also promotes cancer progression. Inflammatory cytokines such as IL-6, TNF-α, and granulocyte macrophage colony-stimulating factor are mediated by air pollution, resulting in low-grade, chronic inflammation (72, 73). Low-grade inflammation fuels tumor progression by enabling immune evasion, angiogenesis, and metastatic dissemination. Air pollutants also mediate oxidative stress, which is characterized by an increase in ROS (74). Exposure to PM with an aerodynamic diameter of ≤2.5 mm (PM2.5) was found to promote lung cancer by acting on cells that harbor pre-existing oncogenic mutations in healthy lung tissue (75). The discovery of immune checkpoint proteins, such as programmed cell death protein 1 (PD-1)/programmed cell death ligand 1 (PD-L1) and cytotoxic T-lymphocyte associated protein 4 (CTLA-4), represents a significant breakthrough in the field of cancer immunotherapy (76).
Ambient outdoor air pollution
Globally, 7.8% of all deaths in 2019 were attributed to outdoor air pollution, with this fraction exceeding 12% in some countries (Figure 4) (77).
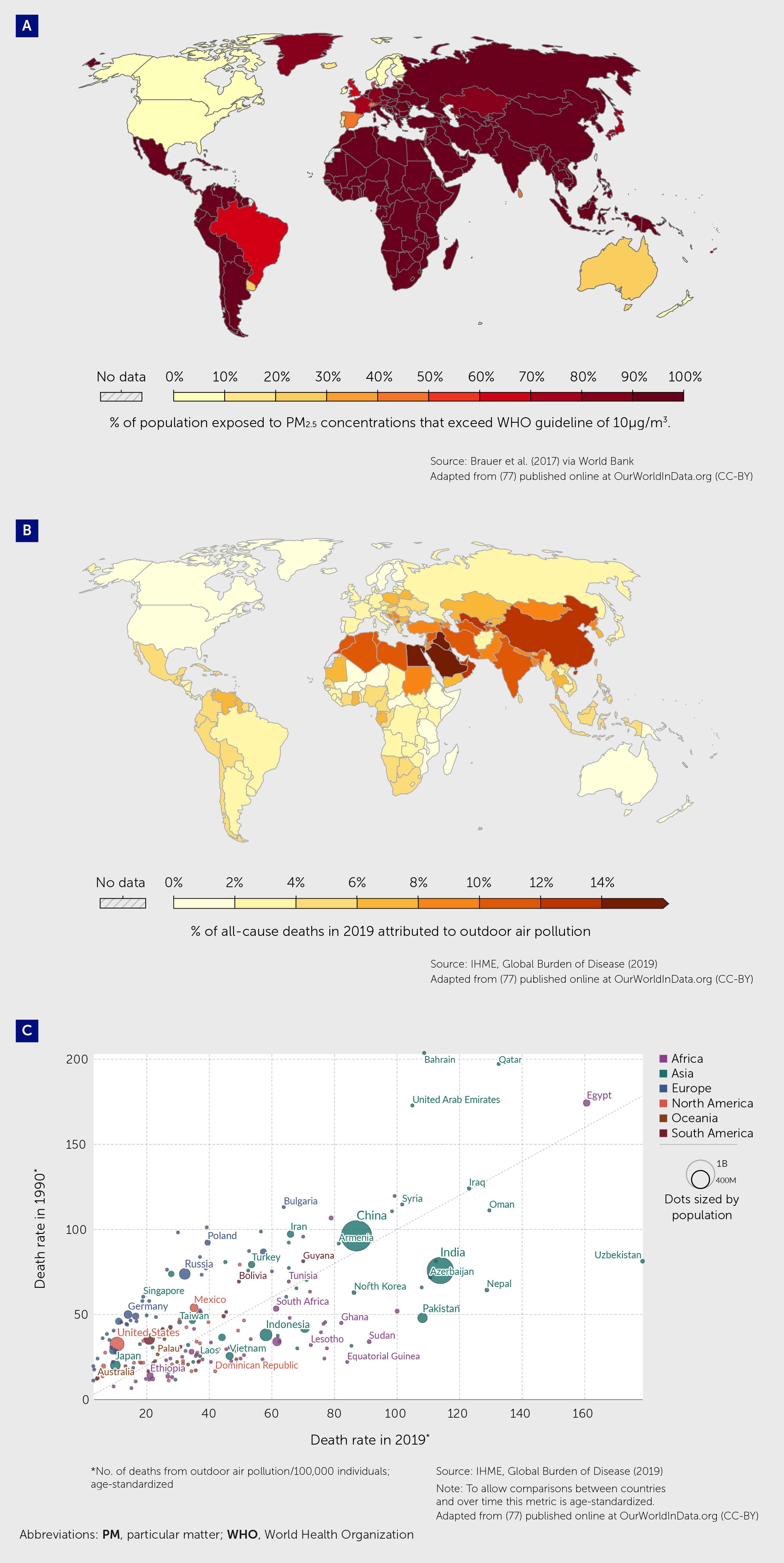
Figure 4 Contribution of air pollution to mortality rates globally. (A) Percentage of the population in 2017 exposed to mean annual ambient outdoor concentrations of particulate matter (PM2.5) that exceed 10 µg/m3/year, the guideline value recommended by the World Health Organization as the lower end of the range of concentrations over which adverse health effects due to PM2.5 exposure have been observed adapted from (77). (B) Percentage of deaths in 2019, from any cause, attributed to outdoor air pollution (from ambient particulate matter and ozone) as a risk factor adapted from (77). (C) Age-standardized death rates from outdoor air pollution (number of deaths per 100,000 individuals) in 1990 and 2019 adapted from (77).
Evidence suggests that exposure to numerous ambient air pollutants (e.g., PM, O3, NOx) is related to the incidence and exacerbation of asthma in both children and adults (78–82). A systematic review and meta-analysis of 67 studies found that short-term daily exposure to O3, NO2, and sulfur dioxide (SO2) was associated with increased emergency room visits and hospital admissions for asthma exacerbations. The pooled relative risk (RR) per 10 μg/m3 increase of ambient mean 24-hour concentrations was 1.008 [95% confidence interval (CI) 1.005–1.011)] for O3, 1.014 (95% CI 1.008–1.020) NO2, and 1.010 (95% CI 1.001–1.020) for SO2 (83). Another review evaluating 84 studies also concluded that short-term exposure (lag0 and lag1 exposure patterns) to outdoor pollutants increased asthma exacerbation risk (84). Other meta-analyses and systemic reviews have found an association between prenatal exposure to pollutants and the risk of asthma and wheezing in children (85, 86). A review conducted by the Health Effects Institute in the United States found that each 10 μg/m3 increase in NO2 was associated with a RR of asthma of 1.05 in children (12 studies) and 1.10 in adults (seven studies). Among children, each 5 μg/m3 increase in PM2.5 was associated with a relative risk of 1.33 (five studies). Similar effects were observed for associations with asthma prevalence among children (21 studies) (87). Another systematic literature review found compelling evidence that exposure to PM2.5, PM with an aerodynamic diameter of ≤10 mm (PM10), and NO2 contributes to the risk of asthma development in childhood (88). Inhaled PM2.5 are especially damaging to developing lungs as they are small enough to enter lung tissues (89). Exposure to O3 has also been associated with new-onset asthma and is a well-established trigger for asthma exacerbations. Ambient air pollution is also associated with other respiratory diseases such as COPD. Analysis of data from the large UK Biobank showed that ambient air pollution exposure was associated with lower lung function and increased COPD prevalence (90).
Air pollution is also associated with cancer and autoimmune disease. In 2017, it was estimated that 14% of total lung cancers could be attributed to air pollution (91). A recent systematic review and meta-analysis found that exposure to PM2.5 was associated with an increased risk of colorectal cancer: the odds ratios (OR) for incidence and mortality were 1.18 (95% CI 1.09–1.28) and 1.21 (95% CI 1.09–1.35), respectively. The risks of incidence and mortality were higher in the United States than in other countries studied (20). The International Agency for Research on Cancer (IARC) has classified outdoor air pollution as a Group 1 carcinogen (19).
A population-based cohort study found that every 10 µg/m3 increase in PM10 was associated with an incremental 7% risk of developing an autoimmune disease. Exposure to PM10 at >30 µg/m3 and PM2.5 at >20 µg/m3 was associated with a 12% and 13% higher risk of autoimmune disease, respectively (23). A study in Canada showed a link between fine PM and systemic autoimmune rheumatic diseases (SARDS) and found that the adjusted hazard ratio for SARDS related to one interquartile range increase in PM2.5 (3.97 µg/m3) was 1.12 (95% CI 1.08–1.15) (92).
Wildfires
Wildfires affect millions of people worldwide, causing a high incidence of attributable deaths from suffocation or injuries. Wildfires are growing in size and frequency as a result of climate change (93). Hundreds of thousands of hectares of forests and buildings/homes are destroyed worldwide each year by fire or human activities. These fires release large quantities of CO2, carbon monoxide, and PM into the atmosphere. Indeed, wildfires are estimated to contribute at least 25% of the PM in the atmosphere (94, 95). The resulting increase in ambient pollution risks a range of health issues, including respiratory diseases, as detailed in the previous section. Specifically, exposure to wildfire smoke has been shown to increase key pro-inflammatory markers, such as C-reactive protein (CRP) and IL-1β, which can worsen asthma and allergic disorders (96). Other studies have linked wildfires with increases in conjunctivitis (97).
In the United States, PM2.5 from wildfires have been detected at levels 10- to 15-fold higher than the 24-hour standard (35 μg/m3) even 1000 km away from the source (98). Decreases in respiratory peak flow rate have been documented 1 year after wildfire events (99). There was a significant increase in the use of dermatology clinics in San Francisco (~290 km downwind) for the treatment of atopic dermatitis and itch for a 2-week period during and after the Camp Fire in northern California in 2018 (100).
Sand and dust storms
The “global dust belt” refers to regions stretching from the Sahara Desert to the Gobi Desert in China and Mongolia. Globally, approximately 50% and 40% of all dust particles (≤20 μm in diameter) are from northern Africa and Asia, respectively. The remainder are from North America and the southern hemisphere (101).
SDSs can spread thousands of kilometers away from their origin: Saharan dust frequently reaches Europe and even North and South America. Asian dust is transported across the Pacific into North America. The long-term effects of drought, scarce vegetation, and human exploitation and land use, as well as variations in precipitation, temperature, and wind speed, are critical parameters in the context of climate change and its impact on dust emission and spread (102).
SDSs have a significant impact on hospital admissions for respiratory diseases such as asthma (103). Exposure to PM2.5 and PM10 during SDS outbreaks has been associated with respiratory and allergic diseases (104). Airborne dust can stay suspended in the air for days following SDS events. SDSs can also carry pollen, fungal spores, bacteria, and viruses, leading to respiratory infections, asthma, and allergies. Many microorganisms and pathogens can survive long-range transport by SDSs. For example in the southwestern United States, complex interactions between drought, heat, and increases in dust conditions have led to increased incidence of coccidiomycosis infections (105).
Indoor air pollution
Indoor sources of air pollution include cooking and heating with biomass such as coal and firewood, use of candles and incense sticks, cigarette smoke, volatile organic compounds (VOCs) from cleaning and other consumer products, molds, dust mites, and animal dander. Indoor air pollution contributed to 4.1% of global deaths in 2019, with the highest death rates among low-income countries (Figure 5).
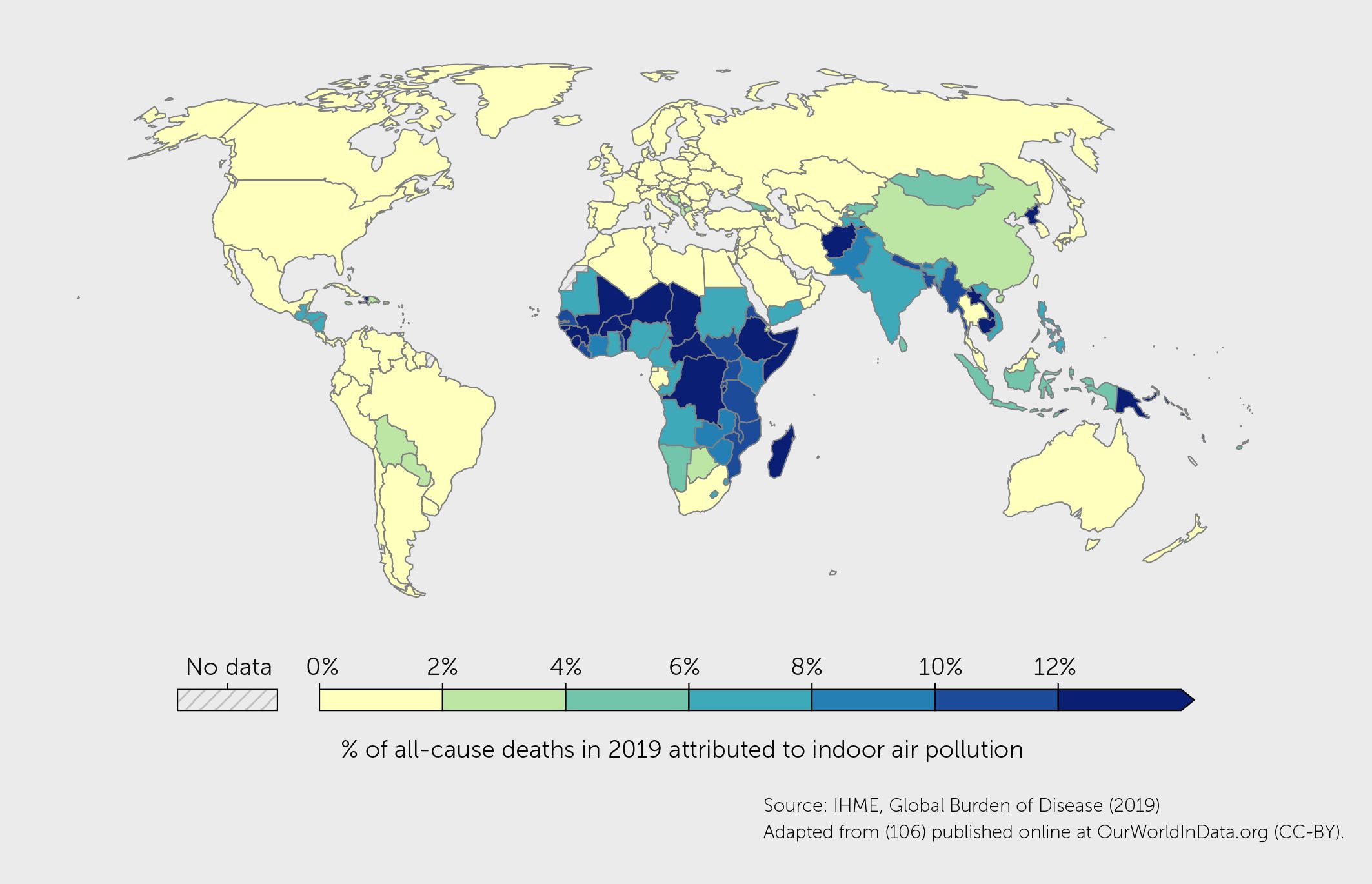
Figure 5 Percentage of deaths in 2019, from any cause, attributed to indoor air pollution (from burning solid fuels) as a risk factor (106).
Many forms of indoor pollution can impair human health. For example, NO2, which is generated by cooking and heating with gas, has been associated with an increased risk of asthma in children (107). A systematic review and meta-analysis estimated a 1.8-fold increase in pneumonia risk in young children due to exposure to unprocessed solid fuels (108). A systematic review of the effect of indoor PM and VOCs found that high VOCs were associated with upper airway and asthma symptoms in those with pre-existing lung disease (109). With decreased use of solid fuels and increased use of clean fuels for cooking, these rates have been decreasing since the 1990s and are expected to decrease further (102). Climate change has also led to increased flooding and extreme rainfall, which increases dampness in poorly weatherized homes, leading to indoor mold proliferation. Mold spores are indoor pollutants of concern as they increase asthma severity (110). A study observed a significant association between household mold and increased prevalence of asthma (OR = 2.399). Similarly, another study in China found that mold odor was associated with asthma (OR = 1.90) (111, 112).
Heat stress
Climate change is also increasing global temperatures, with increases in the severity and frequency of heatwaves and the associated morbidity and mortality (113).
During heat stress, high levels of ROS are released (Figure 2). ROS induce oxidative damage and degradation of biomolecules (e.g., proteins, lipids, carbohydrates, and DNA) leading to cellular death, while also increasing the production of heat shock proteins (HSPs), antioxidants, other metabolites, anti-inflammatory cytokines, and stress granules that mitigate the effects of heat stress (114) (115). There is increasing evidence that HSPs can influence the immune system, mediating both pro-inflammatory and anti-inflammatory pathways. For example, HSP70-B29, a bacterial HSP70-derived peptide, has been shown to induce HSP-specific Tregs that suppress arthritis by cross-recognition of their mammalian HSP70 homologs (116). However, HSPs have also been demonstrated to induce the release of pro-inflammatory cytokines such as IL-6 and IL-1 through the NF-kB pathway (117, 118). In primary human keratinocytes and mice, HSP90 inhibition robustly suppressed 12-O-tetradecanoylphorbol-13-acetate (TPA)-induced inflammation by targeting key pro-inflammatory cytokines and signaling pathways, suggesting it may be a potential topical treatment option for immune-mediated skin disease beyond psoriasis (119).
Heat stress has various direct and indirect effects on health and well-being. For example, a study in Australia found a significant increase in childhood asthma emergency department admissions during heatwaves (defined as three consecutive days of extreme heat). Male children and those aged 0–4 years were the most vulnerable (120). In China, exposure to ambient air pollution and high temperatures were independently and jointly associated with asthma risk in early childhood (121). Other direct effects of heat stress include cramps, stroke, increases in cardiovascular disease, and mental health conditions (122). Heat has been shown to cause ventricular-dependent changes that may undermine cardiac health, energy homeostasis, and function (123). Elderly individuals and those with comorbid conditions are at particular risk from heat stress (124).
Heat stress also indirectly affects health outcomes through disruption caused to infrastructures (e.g., power, water, and transport) and via increases in workplace injuries and drownings. Higher temperatures also increase the occurrence of harmful marine algal blooms that release toxins, and food and waterborne diseases (125).
Urban/rural environments and migration patterns
Climate change exacerbates shortages of food and water, which have led to human migration from rural to urban areas or from low- and middle-income countries to affluent countries. The United Nations predicts that close to 70% of the world’s population will live in urban areas by 2050 (126). These migration patterns are associated with increases in asthma and allergy in individuals displaced from a rural environment to a more crowded urban area. Rural areas have higher microbial diversity and exposure to animals, both of which are protective against allergy and asthma. However, migration also exposes individuals to new pollutants and allergens. A study in Brazil found that rural-to-urban migration contributes to the high burden of asthma in urban migrants (127).
The built environment, which encompasses all manufactured or modified structures that provide people with living, working, and recreational spaces, is a critical determinant of allergy and asthma (128) owing to higher levels of indoor pollutants and allergens (129). Climate change is exerting changes in built environments that are expected to drive immune-mediated disease. For example, increased temperatures lead to a lower quality of indoor air. Flooding events result in increased urban indoor dampness, humidity, and mold levels (110). An increase in temperate zones is expected to lead to an increase in rodent populations (130), a matter of concern because mouse allergen is also a critical driver of childhood asthma disparities (131).
Food and water safety
Global warming and climate change have substantially endangered food safety for humans and domestic animals. Chemical pollutants contained in foods, such as emulsifiers, coloring agents, and preservatives, can disrupt the epithelial barrier that protects against asthma and allergies (132). Residual detergents found after rinsing foods also damage lung tissue, as shown in human bronchial cell cultures (133). Dioxins and toxic metals such as lead, cadmium, and mercury found in contaminated water are also highly toxic and can lead to decreases in immunity (134, 135).
Biodiversity loss
The human body is an ecosystem or “holobiont”—a concept describing the host (animal or plant) as a community of species linked to other ecosystems and subject to continuous evolutionary pressure (136). Functioning ecosystems provide essential benefits to humanity (137)—sometimes referred to as ecosystem services. Biodiversity (the variety of life including genes, species, and ecosystems) is a key factor in maintaining a healthy and functioning ecosystem and for human health (138). Climate change, habitat destruction, and intensive land use have led to a startling loss of biodiversity, including microbial species (137, 139). Millions of species worldwide could face extinction as a result of climate change in the next few decades (140, 141).
Biodiversity loss associated with climate change can have a huge impact on human health, both directly (through disruption in the food supply or via emerging infectious diseases) and indirectly (by degrading ecosystem health and ecosystem services) (142).
In particular, exposure to a greater diversity of microbes is thought to be a key factor driving the early immune system away from the development of asthma and allergies (143). Maternal exposure to stables during pregnancy, for example, has been found to be associated with increased TNF-alpha and IFN-gamma levels in newborns (144).
Mental stress
Climate change is associated with increased mental health burden and stress (145). In individuals with persistent emotional stress and greater negative moods, allergy flares are more frequent (146). The stress hormones, including glucocorticoids, epinephrine, and norepinephrine, are thought to be involved in psychological stress-induced asthma exacerbation (147). A study of 763 Japanese mother-child pairs used 12 specific behavioral patterns as stress indicators and found that maternal chronic irritation and anger were associated with the risk of childhood asthma (148). In adolescents, stressors such as poverty, neighborhood stress, and school stress were individual predictors of emergency department visits for asthma (149). In 2021, a survey of children and young adults in 10 countries found that there was widespread climate anxiety and dissatisfaction with government responses to climate change (150).
Mitigation and adaptation to manage the effects of climate change on immune diseases
We have outlined the complex effects that climate change is having on environmental exposures that in turn cause immune dysregulation and its associated diseases, including asthma, allergies, autoimmune diseases, and cancers (Figure 1). Research into the pathophysiology of immune-mediated diseases has provided a greater understanding of their mechanisms and has led to the development of novel drugs (151). However, in addition to this individualized treatment approach, multisectoral, multidisciplinary, and multilevel efforts are urgently needed to mitigate and adapt to the effects of climate change-related exposures (Figure 6).
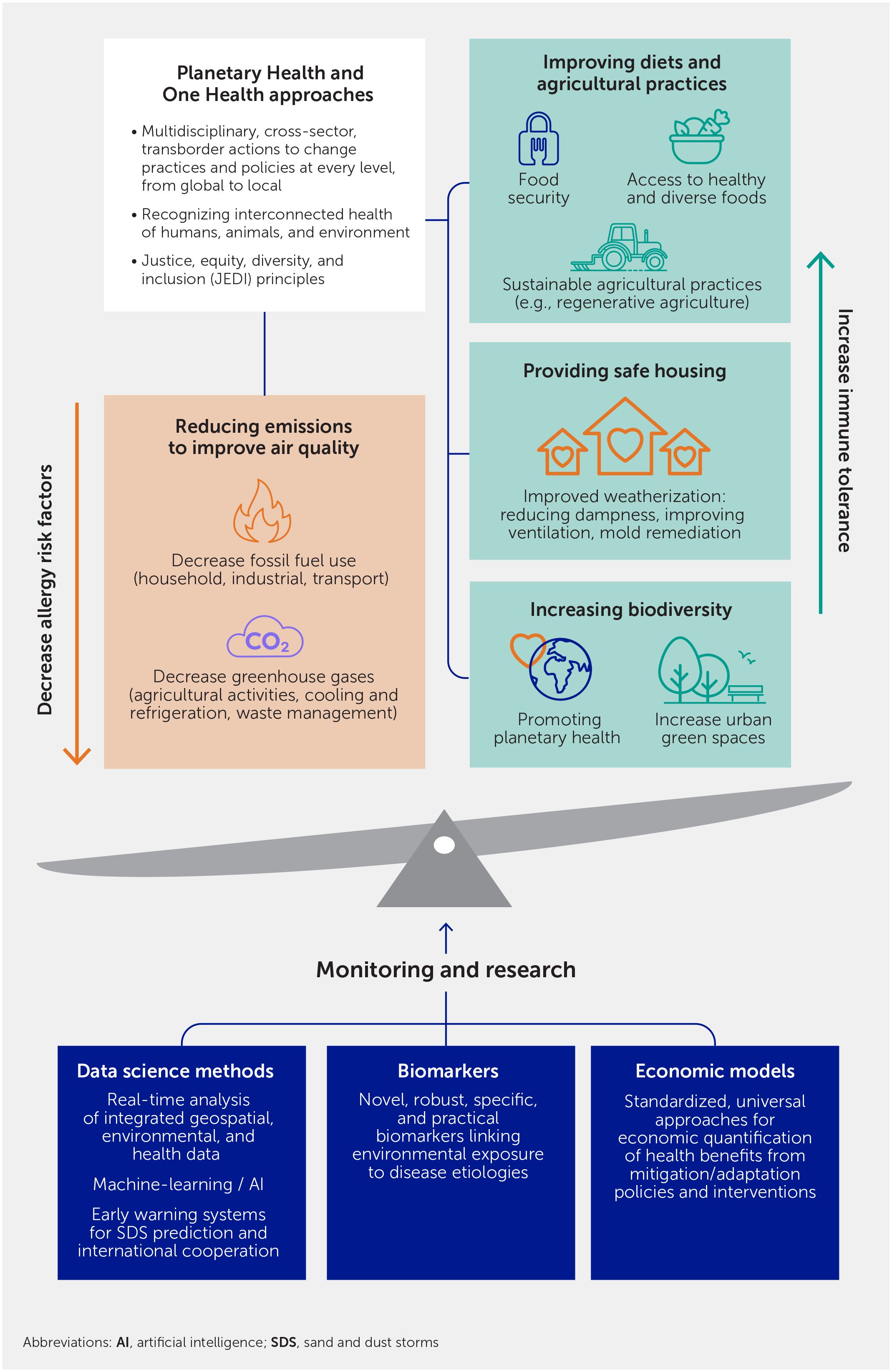
Figure 6 Mitigation and adaption actions to address increases in immune-mediated diseases associated with the exposome effects of climate change.
Mitigation refers to primary preventions intended to cut or prevent the emission of greenhouse gases (e.g., using an electric car or electrifying a housing unit) thus limiting the magnitude of future warming. Adaptation is a form of secondary prevention intended to reduce vulnerability to climate change impacts, such as going to cooling centers during heat stress (e.g., see www.marinhhs.org/cooling-centers) or using a filter in a home to decrease asthma and allergies (152).
Implementing Planetary Health and One Health approaches
Planetary Health has been defined as “the health of human civilization and the state of the natural systems on which it depends” (153). Planetary Health directs attention to the extensive degradation of our planet for the benefit of humans. The concept focuses on better balancing human needs with the health of Earth’s ecosystems to promote sustainability and protect the health and well-being of future generations. Reversing the degradation of the planet’s ecosystem will require collaborations between multiple disciplines and sectors in order to alter practices and policies at every level, from global to local, and drive sustainable solutions. One Health, an interdisciplinary approach stressing the interconnectedness of the health of humans, animals, and the environment, is an important approach for better planetary and human health (154, 155).
Environmental health professionals, specifically those specializing in asthma and allergy, can play an essential role in education, communication, and advocacy on climate change mitigation and adaptation measures. For example, the connection between climate change and asthma and allergy was emphasized during the 2008–2018 Finnish Allergy Programme (156). In the city of Lahti, the European Union Green Capital 2021, Natural Step to Health, a 2022–2032 Regional Health and Environment Programme focuses on asthma and allergy as one of the areas of interest (157). The goals also include mitigation of climate change and nature loss. In this program, a “Planetary Health Physician” connects the healthcare and environment sector to improve citizens’ diet (e.g., in day-care), physical exercise/mobility, housing environment, and nature connectedness (158), in the spirit of Planetary Health (153).
Reducing emissions and improving air quality
Widespread and fast action to reduce short-lived climate pollutants (SLCP; e.g., methane, hydrofluorocarbons, and black carbon) emissions has the potential to reduce global warming by as much as 0.6°C over the next few decades (159). At the community level, this can be achieved through actions targeting the reduction of air pollution emissions from agricultural activities, fossil fuel, waste management, household energy, industrial production, transport, cooling, and refrigeration. SLCP reduction will be beneficial for health. For example, reducing methane emissions by 40% by 2030 could prevent an estimated 540,000 emergency room visits globally from asthma (160). Investments to increase the usage of public transportation by expanding networks and lowering or eliminating fares, incentives for greater use of electric vehicles, and increasing pollution taxes can also improve air quality (161).
In the United States, Congress established much of the basic structure of the Clean Air Act in 1970 and made major revisions in 1977 and 1990 (162). Policies based on the Clean Air Act have led to decreases in air pollutant concentrations in southern California. Among children in southern California, decreases in ambient NO2 and PM2.5 between 1993 and 2014 were significantly associated with lower asthma incidence (163). A reduction in NO2 of 4.3 parts per billion reduced the risk of incident asthma by 20%.
The air quality, health, and economic benefits of emission reductions from 2002–2011 in the eastern United States were estimated for the month of July 2011. During this extremely hot month, these actions were estimated to have prevented 10–15 O3 exceedance days in the Ohio River Valley and 5–10 exceedance days in the United States mid-Atlantic region, saving 160–800 lives in the eastern United States and US$1.3–6.5 billion. Emission reductions were also estimated to have avoided 950 hospital admissions for respiratory symptoms, 370 hospital admissions for pneumonia, 570 emergency room visits for asthma symptoms, 922,020 minor restricted activity days, and 430,240 symptoms of asthma exacerbation (164). Therefore, surveillance of the impact of changes in air pollution on asthma and allergies would be useful in informing local and national government policies for managing environmental health risks. It would also allow systematic monitoring of the health impacts of policy decisions and plans implemented.
Emission reduction policies implemented in Seoul in 2007 also resulted in cleaner air, with significant decreases in PM10 (–17%) and NO2 (–5%) in the period 2008–2011 compared with 2003–2007. It was estimated that the policy prevented 500,000 (11.3%) hospital visits for asthma in the total population and 320,000 (15.5%) in the younger population during this period (165).
Improving air quality can have even more rapid and substantial health benefits. In 1996, in preparation for the Olympics, the city of Atlanta implemented a 17-day alternative transportation strategy involving 24-hour public transportation. Within a month, there was a 42% reduction in children seeking medical care for asthma and a 19% decrease in hospitalizations for asthma (166). This suggests that air quality policies can benefit asthma and allergy clinical outcomes within a month of their implementation and that these markers (across all age groups) could be used to monitor policy effectiveness (Table 2).
Providing safe housing
Housing is one of the central determinants of exposure to indoor asthma risk factors. Children living in historically marginalized urban homes near areas of high air pollution have higher rates of asthma and more severe and uncontrolled asthma (177–179). Consistently, there is strong evidence that improved weatherization (i.e., ventilation and mold remediation) decreases the risk of respiratory disease outcomes (129). However, the current provision of housing through for-profit markets creates barriers for large segments of the population, particularly racial and ethnic minorities, and working-class communities more generally, to access safe living conditions (180). Climate change worsens air quality and exacerbates indoor dampness and mold, thereby potentially widening existing race and class disparities in housing quality and concomitant asthma burden. Existing public housing stocks require repair, upgrade, and expansion to address this urgent threat. Randomized controlled trials provide strong evidence of the respiratory health benefits of high-quality public housing (181). Modeling studies estimate that repairing New York City’s public housing units would reduce asthma rates by a quarter among their 350,000 residents (182). In the private market, increases in regulatory health code requirements are needed, particularly with respect to mold remediation. An emerging evidence base is showing that proactive inspections are effective in this regard (183), particularly if informed by leading indicators of unhealthy or unsafe conditions (184).
Improving diets and agricultural practices
Asthma and allergies can be worsened by poor nutrition or inadequate diet interventions. Indeed, diversity in dietary proteins early in life has been shown to reduce the risk of asthma and allergies (185). Exploratory data suggest that improvements in diet quality could help reduce the inflammatory status among individuals with poorly controlled asthma (186). Dietary factors are also important in the development of food allergies, eosinophilic esophagitis, and other disorders resulting from chronic inflammation, such as autoimmune diseases and cancer. Therefore, focusing on food security, access to healthy foods, and diversity of food choices is important for the prevention of immune-mediated diseases.
The EAT-Lancet Commission on healthy diets from sustainable food systems established that global food production should stay within 5 Gt of CO2 equivalent per year from food systems by 2050 (187, 188). The commission recommended a healthy, plant-forward diet, high in whole grains, legumes, vegetables, fruits, and healthy fats with a very modest amount of animal protein—about 300 calories worth, or only 12% of the reference intake of 2,500 calories/day. The inclusion of a wide variety of plant-based proteins (including nuts) in the diet, especially in early life, can also help prevent asthma and allergies by developing immune tolerance (30, 189). Understanding how these revised diets will enable better control of an inflamed immune system, improve microbiota, and enhance overall health will be important for the future.
Agricultural practices need to be overhauled to become more sustainable. For example, regenerative agriculture is a holistic land-management practice that aims to sequester carbon in the soil while improving soil health, crop yields, water resilience, and nutrient density. It advocates for, among other practices, the use of cover crops, reducing tilling, rotating crops, spreading compost (as well as super-compost “inoculants”), and moving away from synthetic fertilizers, pesticides, herbicides, and factory farming (190).
Increasing biodiversity and exposure to natural environments
Growing up in a biodiverse environment is one of the strongest and most consistent protective factors against the development of asthma and related atopic conditions (191). According to the available evidence, it is highly probable that we could develop safe and effective primary preventive regimens for asthma and allergy by developing better practices for planetary health and promoting biodiversity and exposure to natural environments.
Indeed, green spaces can reduce all-cause mortality (192). In Toronto, a study found that green space structures can protect individuals aged 0–19 years from a high risk of developing asthma, and this direct protective effect can be enhanced by high tree diversity (193). Among adults residing in the five largest urban areas in Belgium, higher exposure to increased residential green space was associated with lower rates of non-accidental and respiratory mortality (194). In Metro Vancouver, a population-based birth cohort study found that increased exposure to residential greenspace might improve childhood development by reducing the adverse developmental effects of traffic-related exposures, especially NO2 air pollution (195).
Importantly, many greening practices are being established in cities worldwide to mitigate climate change effects (196). Planting trees and native plant species is an important part of creating better means to sequester carbon and reduce temperatures and CO2. Asthma and allergy experts should be included in policy and city planning discussions to promote biodiversity and reduce allergenicity. In fact, with proper planning and the inclusion of native dioecious plant species, it is possible to reduce major allergen spread (197).
Monitoring and research: data science, biomarkers, and economics
There is a pressing need for collaborative, multidisciplinary research to better understand the pathophysiology of immune diseases in the context of climate change. Moreover, the development and broad implementation of new data science techniques, biomarkers, and economic models are critical for measuring the impact of climate change on immune health and disease, informing the design of mitigation and adaptation policies and interventions, and evaluating their effectiveness.
Data science methods
Real-time analysis of integrated geospatial, environmental, and health data and the application of machine-learning techniques to better understand environmental-human interactions are currently some of the most important challenges in understanding the impact of global climate change on human health and disease. Big data analyses and artificial intelligence can be utilized to decipher the complex, multidimensional relationships between diverse parameters in the cause of diseases, with special attention to vulnerable populations, chronicity of cumulative effects over a lifetime, and multiple simultaneous exposures. The results of these studies are instrumental for the development of health resilience measures such as forecasting, adaptation of disease management, and prevention of noncommunicable diseases to manage climate change scenarios. The Integrated Clinical and Environmental Exposures Service (ICEES) provides regulation-compliant open access to electronic health record data that have been integrated with environmental exposure data, as well as analytic tools to explore the integrated data. Results suggest that the open-source ICEES can be used to replicate and extend published findings on factors that influence asthma exacerbations (198–200). The National Institutes of Health (NIH) is now looking to establish the Center for Exposome Research Coordination to accelerate precision environmental health. It aims to build upon the effort supported by the European Union to further extend, expedite, and coordinate exposome research efforts on a global scale (201). Another important program by the NIH is the Environmental Influences on Child Health Outcomes (ECHO) Program. ECHO combines observational and intervention research to answer important questions about how influences on early human development—even before birth—affect us throughout our lives and across generations. ECHO focuses on five pediatric health outcome areas: pre-, peri- and postnatal; upper and lower airways; obesity; neurodevelopment; and positive health, such as happiness and a sense of well-being (201). Examples of other exposome projects that have been established include the EU-funded Human Early-Life Exposome (HELIX) and the Health and Environment-wide Associations based on Large population Surveys (HEALS) (202). Big data monitoring methods are being used to evaluate air quality trends and vehicle traffic dynamics and monitor river water quality and wildfire pollutant composition (203–205). Big datasets and analytics that integrate statistics, computer science, biomedicine, and public health are being developed that can assist with understanding the complex dynamics between the environment and health.
Biomarkers
Biomarkers can provide evidence of exposure to pollutants, associate them with disease etiologies, and provide necessary data to inform policy decisions. For example, one study measured urinary concentrations of nine hydroxylated metabolites of polycyclic aromatic hydrocarbons (OH-PAHs) to assess wildland fire smoke exposure in wildland firefighters. Post-shift concentrations of OH-PAHs were 83–323% higher compared with pre-shift concentrations. Additionally, the increase in 4-hydroxy-phenanthrene urinary concentration was marginally associated with work shift exposure to PM2.5 and significantly associated with levoglucosan, a marker of wildland fire or vegetative biomass smoke. These results suggest that OH-PAHs, especially 4-hydroxy-phenanthrene, may be useful biomarkers of wildland fire smoke exposure (206). Another urinary marker, 1-hydroxypyrene, has been associated with occupational exposure to polycyclic aromatic hydrocarbons (PAHs) from urban pollution (207).
Recent studies have correlated CRP with air pollution, suggesting that low-grade inflammation may be a causative factor for the adverse health effects associated with exposure to air pollution. In a study by Prunicki et al, individuals exposed to wildfire smoke from the El Portal wildfire in California in 2014 had increased levels of the proinflammatory markers, CRP and IL-1β, compared with non-smoke exposed controls (96). A large population-based cohort study in the United Kingdom found that CRP levels were positively associated with NO, NO2, and PM2.5 (208). Similarly, short-term exposure to air pollution was associated with higher serum high-sensitivity CRP levels in adult residents of two urban areas in northern France (209). A metabolomics study identified lysophosphatidylcholine (LysoPC) P-20:0 and LysoPC P-18:1(9z), two products of phospholipid catabolism, as potential biomarkers of PM2.5 exposure (210).
Cancer biomarkers have also been associated with air pollution. For example, research in western China suggests that the lung cancer markers, cytokeratin 19 fragment (CYFRA211) and nerve-specific enolase (NSE), could be used as biomarkers for exposure to certain air pollutants (211).
While numerous studies have found associations between environmental exposures and biomarkers, robust, specific, and practical biomarkers are still lacking. In the future, omic approaches may provide comprehensive molecular signatures that can better assess the impact of climate change on disease development and progression. For example, a study found differences in metabolic profiles and alterations in the skin microbiome of women from polluted and less polluted cities (212). Omics has the potential to elucidate pathophysiological mechanisms and to facilitate patient stratification, disease prognosis, and prediction of treatment efficacy (213).
Economic models of climate change interventions
The adverse health consequences of climate change are significantly mitigated by policies that reduce GHG emissions, decreasing health burdens and healthcare costs.
In the United States, federal agencies have relied on a common damage function, the “social cost of GHG emissions” to economically quantify the societal benefits or harms of decreasing or increasing GHG emissions (214). From 2008 to 2022, this damage function did not fully consider the health effects of climate change beyond diarrhea and malaria morbidity and mortality (215). As a result, United States federal agencies have historically underestimated the social benefits of climate mitigation (216). In fact, the London School of Hygiene and Tropical Medicine and others have recently estimated that by achieving net zero in 16 major cities worldwide, 20,000 cases of childhood asthma, representing a quarter of the current asthma cases in these cities, could be avoided annually (217). Such interventions also offer a good economic return on investment: for every US$1 spent on climate mitigation, at least US$3 is saved from health benefits, largely through a reduction of diseases such as asthma and allergies (88, 218). Peer-reviewed cost-benefit analyses of policies and scenarios to reduce GHG emissions have generally concluded that the immediate health benefits of cleaner air (such as reduced asthma and allergy) outweigh the implementation costs of these interventions (219–221).
Standardized, universal approaches to quantify the health benefits of reducing GHG emissions in economic terms are urgently needed to i) motivate and guide climate policies around the world and ii) promote actions to decrease the public health burden of allergies and improve respiratory health.
Conclusion
Climate change presents an existential threat to the health of humans, animals, and the entire ecosystem. The changing exposome has led to an increased risk of major diseases associated with immune dysregulation, including asthma, allergies, cancers, and autoimmune diseases. While progress has been made in the development of therapeutic options to treat these disorders, these approaches are inadequate to meet the challenges posed by climate change.
We must fully recognize that planetary health is the basis of human health. Individuals, organizations, and nations need to work toward systematically mitigating the health effects of climate change, adapting to these changes, and promoting planetary health to improve immune health and decrease the immense burden of immune-mediated disease. Fundamentally, there is an urgent need to develop and implement sustainable practices and reduce the use of fossil fuels to benefit planetary health for succeeding generations. We suggest more collaborative research on the impact of climate change and policies for climate change mitigation on asthma and allergy outcomes.
Socioeconomically disadvantaged groups, such as immigrants with limited language proficiency, communities of color, indigenous groups, and outdoor workers on daily wages, face the brunt of the impact of climate change because of poor housing infrastructure, proximity to highly polluted areas, or poor access to medical care. Children, pregnant women, the elderly, individuals with preexisting conditions, and people with disabilities are also at greater risk from the adverse health effects of climate change. Justice, equity, diversity, and inclusion (JEDI) considerations should be integral within climate adaptation planning to address existing disparities exacerbated by climate change.
Statements
Author contributions
IA: Visualization, Writing – original draft, Writing – review & editing. MA: Visualization, Writing – original draft, Writing – review & editing. CA: Visualization, Writing – original draft, Writing – review & editing. AA-H: Visualization, Writing – original draft, Writing – review & editing. IA-M: Visualization, Writing – original draft, Writing – review & editing. JB: Visualization, Writing – original draft, Writing – review & editing. LC: Visualization, Writing – original draft, Writing – review & editing. AD: Visualization, Writing – original draft, Writing – review & editing. TH: Visualization, Writing – original draft, Writing – review & editing. AH: Visualization, Writing – original draft, Writing – review & editing. JH: Visualization, Writing – original draft, Writing – review & editing. MJ: Visualization, Writing – original draft, Writing – review & editing. YM: Visualization, Writing – original draft, Writing – review & editing. BM: Visualization, Writing – original draft, Writing – review & editing. J-WO: Visualization, Writing – original draft, Writing – review & editing. AO: Visualization, Writing – original draft, Writing – review & editing. RP: Visualization, Writing – original draft, Writing – review & editing. MJ: Visualization, Writing – original draft, Writing – review & editing. HR: Visualization, Writing – original draft, Writing – review & editing. MR: Writing – original draft, Writing – review & editing, Visualization. NR: Visualization, Writing – original draft, Writing – review & editing. VS: Visualization, Writing – original draft, Writing – review & editing. CS: Visualization, Writing – original draft, Writing – review & editing. FT: Visualization, Writing – original draft, Writing – review & editing. CT-H: Visualization, Writing – original draft, Writing – review & editing. GW: Visualization, Writing – original draft, Writing – review & editing. KN: Visualization, Writing – original draft, Writing – review & editing.
Data availability statement
The original contributions presented in the study are included in the article/supplementary material. Further inquiries can be directed to the corresponding author.
Funding
The author(s) declare financial support was received for the research, authorship, and/or publication of this article.
CA receives grants from the Swiss National Science Foundation, European Union (EU CURE), Novartis Research Institutes (Basel, Switzerland), Stanford University (Redwood City, CA, USA), and SciBase, Stockholm.
IA-M has received grants from EU and national public grants and has received support for attending the 2022 European Respiratory Society meeting in Barcelona from the society (€600).
TH has received honoraria for lecturing at Orion Pharma.
JEH receives grants from NIH/NIEHS P30 ES000002.
HR is supported by the German Lung Center (DZL) and the Universities Giessen and Marburg Lung Center (UGLMC), the MIRACUM Consortium of the Medical Informatics Initiative, the Stiftung Pathobiochemistry and DAAD and GIZ Hospital Partnership Programme. HR has received grants from the German Center for Lung Disease (DZL German Lung Center, no. 82DZL00502) and Universities Giessen and Marburg Lung Center (UGML).
MBR reports grants from the NIH and fees from the Conservation Law Foundation.
NARF received payment or honorarium from Sanofi, AstraZeneca, AbbVie, Novartis, Viatris, Chiesi, and Glenmark.
CS is supported by the Universities Giessen and Marburg Lung Center (UGMLC), the German Center for Lung Research (DZL), University Hospital Giessen and Marburg (UKGM) research funding according to article 2, section 3 cooperation agreement, and the Deutsche Forschungsgemeinschaft (DFG, German Research Foundation)-SFB 1021 (Project Number 197785619), KFO 309 (Project Number 284237345), and SK 317/1-1 (Project Number 428518790) as well as by the Foundation for Pathobiochemistry and Molecular Diagnostics.
GWKW received funding support from TS Lo Foundation, Hong Kong Research Grant Council Grant No. 14119320, and National Natural Science Foundation of China.
KCN was funded by C-CHANGE and the following NIH grants: P01HL152953, R01 ES032253, U01AI147462, U19AI167903, P01AI153559, and U19AI104209.
The funders had no role in the study design; in the collection, analysis, and interpretation of data; in the writing of the report; and in the decision to submit the paper for publication.
Conflict of interest
IA is Chair EAACI Guidelines on environmental science for allergic diseases and asthma and Deputy Editor of Allergy.
CA is chair of the European Academy of Allergy and Clinical Immunology Guidelines on Environmental Science in Allergic diseases and Asthma and is Editor-in-Chief of Allergy.
JB is the Physician Member of the California Air Resources Board a component agency of Cal/EPA.
CS receives consultancy and research funding, Bencard Allergie and Thermo Fisher Scientific; Research Funding, Mead Johnson Nutrition (MJN).
The remaining authors declare that the research was conducted in the absence of any commercial or financial relationships that could be construed as a potential conflict of interest.
The authors AD, RP, NARF, CS and KCN declared that they were an editorial board member of Frontiers, at the time of submission. This had no impact on the peer review process and the final decision.
Publisher’s note
All claims expressed in this article are solely those of the authors and do not necessarily represent those of their affiliated organizations, or those of the publisher, the editors and the reviewers. Any product that may be evaluated in this article, or claim that may be made by its manufacturer, is not guaranteed or endorsed by the publisher.
References
1. Lee AS, Aguilera J, Efobi JA, Jung YS, Seastedt H, Shah MM, et al. Climate change and public health: the effects of global warming on the risk of allergies and autoimmune diseases: the effects of global warming on the risk of allergies and autoimmune diseases. EMBO Rep (2023) 24(4):e56821. doi: 10.15252/embr.202356821
2. Lerner A, Jeremias P, Matthias T. The world incidence and prevalence of autoimmune diseases is increasing. Int J Celiac Dis (2016) 3(4):151–5. doi: 10.12691/ijcd-3-4-8
3. Torre LA, Siegel RL, Ward EM, Jemal A. Global cancer incidence and mortality rates and trends—an update. Cancer Epidemiol Biomarkers Prev (2016) 25(1):16–27. doi: 10.1158/1055-9965.EPI-15-0578
4. Savouré M, Bousquet J, Jaakkola JJK, Jaakkola MS, Jacquemin B, Nadif R. Worldwide prevalence of rhinitis in adults: a review of definitions and temporal evolution. Clin Transl Allergy (2022) 12(3):e12130. doi: 10.1002/clt2.12130
5. Huang Z, Li Y, Park H, Ho M, Bhardwaj K, Sugimura N, et al. Unveiling and harnessing the human gut microbiome in the rising burden of non-communicable diseases during urbanization. Gut Microbes (2023) 15(1):2237645. doi: 10.1080/19490976.2023.2237645
6. Sweeney A, Sampath V, Nadeau KC. Early intervention of atopic dermatitis as a preventive strategy for progression of food allergy. Allergy Asthma Clin Immunol (2021) 17(1):30. doi: 10.1186/s13223-021-00531-8
7. Celebi Sozener Z, Ozdel Ozturk B, Cerci P, Turk M, Gorgulu Akin B, Akdis M, et al. Epithelial barrier hypothesis: effect of the external exposome on the microbiome and epithelial barriers in allergic disease. Allergy (2022) 77(5):1418–49. doi: 10.1111/all.15240
8. Fujisaka S, Watanabe Y, Tobe K. The gut microbiome: a core regulator of metabolism. J Endocrinol (2023) 256(3):e220111. doi: 10.1530/JOE-22-0111
9. Li Q, von Ehrlich-Treuenstätt V, Schardey J, Wirth U, Zimmermann P, Andrassy J, et al. Gut barrier dysfunction and bacterial lipopolysaccharides in colorectal cancer. J Gastrointest Surg (2023) 27(7):1466–72. doi: 10.1007/s11605-023-05654-4
10. Nadeau KC. Approach to the patient with allergic or immunologic disease. In: Goldman L, Schafer A, editors. Cecil Medicine. Philadelphia, PA: Elsevier (2019).
11. Ha J, Lee SW, Yon DK. Ten-year trends and prevalence of asthma, allergic rhinitis, and atopic dermatitis among the Korean population, 2008-2017. Clin Exp Pediatr (2020) 63(7):278–83. doi: 10.3345/cep.2019.01291
12. Krishna MT, Mahesh PA, Vedanthan PK, Mehta V, Moitra S, Christopher DJ. The burden of allergic diseases in the Indian subcontinent: barriers and challenges. Lancet Glob Health (2020) 8(4):e478–9. doi: 10.1016/S2214-109X(20)30061-9
13. Asher MI, Rutter CE, Bissell K, Chiang CY, El Sony A, Ellwood E, et al. Worldwide trends in the burden of asthma symptoms in school-aged children: Global Asthma Network Phase I cross-sectional study. Lancet (2021) 398(10311):1569–80. doi: 10.1016/S0140-6736(21)01450-1
14. Gutowska-Ślesik J, Samoliński B, Krzych-Fałta E. The increase in allergic conditions based on a review of literature. Postepy Dermatol Alergol (2023) 40(1):1–7. doi: 10.5114/ada.2022.119009
15. Dierick BJH, van der Molen T, Flokstra-de Blok BMJ, Muraro A, Postma MJ, Kocks JWH, et al. Burden and socioeconomics of asthma, allergic rhinitis, atopic dermatitis and food allergy. Expert Rev Pharmacoecon Outcomes Res (2020) 20(5):437–53. doi: 10.1080/14737167.2020.1819793
16. Wild C, Weiderpass E, Stewart BW eds. World cancer report: cancer research for cancer prevention. Lyon: International Agency for Research on Cancer (2020). 611 pp.
17. Sung H, Ferlay J, Siegel RL, Laversanne M, Soerjomataram I, Jemal A, et al. Global cancer statistics 2020: GLOBOCAN estimates of incidence and mortality worldwide for 36 cancers in 185 countries. CA Cancer J Clin (2021) 71(3):209–49. doi: 10.3322/caac.21660
18. Ugai T, Sasamoto N, Lee HY, Ando M, Song M, Tamimi RM, et al. Is early-onset cancer an emerging global epidemic? Current evidence and future implications. Nat Rev Clin Oncol (2022) 19(10):656–73. doi: 10.1038/s41571-022-00672-8
19. International Agency for Research on Cancer. Air pollution and cancer (2013). Available at: https://www.iarc.who.int/wp-content/uploads/2018/07/AirPollutionandCancer161.pdf
20. Fu P, Li R, Sze SCW, Yung KKL. Associations between fine particulate matter and colorectal cancer: a systematic review and meta-analysis. Rev Environ Health (2023). doi: 10.1515/reveh-2022-0222
21. Wang L, Wang FS, Gershwin ME. Human autoimmune diseases: a comprehensive update. J Intern Med (2015) 278(4):369–95. doi: 10.1111/joim.12395
22. Cao F, He YS, Wang Y, Zha CK, Lu JM, Tao LM, et al. Global burden and cross-country inequalities in autoimmune diseases from 1990 to 2019. Autoimmun Rev (2023) 22(6):103326. doi: 10.1016/j.autrev.2023.103326
23. Adami G, Pontalti M, Cattani G, Rossini M, Viapiana O, Orsolini G, et al. Association between long-term exposure to air pollution and immune-mediated diseases: a population-based cohort study. RMD Open (2022) 8(1):e002055. doi: 10.1136/rmdopen-2021-002055
24. Hiatt RA, Beyeler N. Cancer and climate change. Lancet Oncol (2020) 21(11):e519–27. doi: 10.1016/S1470-2045(20)30448-4
25. Miller FW. The increasing prevalence of autoimmunity and autoimmune diseases: an urgent call to action for improved understanding, diagnosis, treatment, and prevention. Curr Opin Immunol (2023) 80:102266. doi: 10.1016/j.coi.2022.102266
26. Intergovernmental Panel on Climate Change. AR6 Synthesis Report (2023). Available at: https://www.ipcc.ch/report/ar6/syr/
27. United Nations sustainable development goals. UN Climate Change Conference Paris 2015 (2015). Available at: https://www.un.org/sustainabledevelopment/cop21/
28. Ziegler C, Muchira J. Climate change: the ultimate determinant of health. Prim Care (2023) 50(4):645–55. doi: 10.1016/j.pop.2023.04.010
29. Sampath V, Nadeau KC. Newly identified T cell subsets in mechanistic studies of food immunotherapy. J Clin Invest (2019) 129(4):1431–40. doi: 10.1172/JCI124605
30. Akdis CA, Akdis M, Boyd SD, Sampath V, Galli SJ, Nadeau KC. Allergy: mechanistic insights into new methods of prevention and therapy. Sci Transl Med (2023) 15(679):eadd2563. doi: 10.1126/scitranslmed.add2563
31. Wolf J, O’Neill NR, Rogers CA, Muilenberg ML, Ziska LH. Elevated atmospheric carbon dioxide concentrations amplify Alternaria alternata sporulation and total antigen production. Environ Health Perspect (2010) 118(9):1223–8. doi: 10.1289/ehp.0901867
32. Rauer D, Gilles S, Wimmer M, Frank U, Mueller C, Musiol S, et al. Ragweed plants grown under elevated CO2 levels produce pollen which elicit stronger allergic lung inflammation. Allergy (2021) 76(6):1718–30. doi: 10.1111/all.14618
33. Zhao F, Durner J, Winkler JB, Traidl-Hoffmann C, Strom TM, Ernst D, et al. Pollen of common ragweed (Ambrosia artemisiifolia L.): Illumina-based de novo sequencing and differential transcript expression upon elevated NO2/O3. Environ Pollut (2017) 224:503–14. doi: 10.1016/j.envpol.2017.02.032
34. Pashley C, Grinn-Gofron A, Nowosad J, Bosiacka B, Camacho I, Belmonte J, et al. Airborne Alternaria and Cladosporium fungal spores in Europe: forecasting possibilities and relationships with meteorological parameters. Sci Total Environ (2018) 653:938–46. doi: 10.1016/j.scitotenv.2018.10.419
35. Ziska LH, Makra L, Harry SK, Bruffaerts N, Hendrickx M, Coates F, et al. Temperature-related changes in airborne allergenic pollen abundance and seasonality across the northern hemisphere: a retrospective data analysis. Lancet Planet Health (2019) 3(3):e124–31. doi: 10.1016/S2542-5196(19)30015-4
36. Kurganskiy A, Creer S, De Vere N, Griffith GW, Osborne NJ, Wheeler BW, et al. Predicting the severity of the grass pollen season and the effect of climate change in northwest Europe. Sci Adv (2021) 7(13):eabd7658. doi: 10.1126/sciadv.abd7658
37. Zhang Y, Steiner AL. Projected climate-driven changes in pollen emission season length and magnitude over the continental United States. Nat Commun (2022) 13(1):1234. doi: 10.1038/s41467-022-28764-0
38. Paudel B, Chu T, Chen M, Sampath V, Prunicki M, Nadeau KC. Increased duration of pollen and mold exposure are linked to climate change. Sci Rep (2021) 11(1):12816. doi: 10.1038/s41598-021-92178-z
39. Climate Central. Your changing allergy season. ClimateCentral.org (2019). Available at: https://www.climatecentral.org/climate-matters/your-changing-allergy-season-2019.
40. Ziska LH, Caulfield FA. Rising CO2 and pollen production of common ragweed (Ambrosia artemisiifolia L.), a known allergy-inducing species: implications for public health. Funct Plant Biol (2000) 27(10):893–8. doi: 10.1071/PP00032
41. Anderegg WRL, Abatzoglou JT, Anderegg LDL, Bielory L, Kinney PL, Ziska L. Anthropogenic climate change is worsening North American pollen seasons. Proc Natl Acad Sci U.S.A. (2021) 118(7):e2013284118. doi: 10.1073/pnas.2013284118
42. Wayne P, Foster S, Connolly J, Bazzaz F, Epstein P. Production of allergenic pollen by ragweed (Ambrosia artemisiifolia L.) is increased in CO2-enriched atmospheres. Ann Allergy Asthma Immunol (2002) 88(3):279–82. doi: 10.1016/S1081-1206(10)62009-1
43. Zhou S, Wang X, Lu S, Yao C, Zhang L, Rao L, et al. Characterization of allergenicity of Platanus pollen allergen a 3 (Pla a 3) after exposure to NO2 and O3. Environ Pollut (2021) 278:116913. doi: 10.1016/j.envpol.2021.116913
44. Erbas B, Jazayeri M, Lambert KA, Katelaris CH, Prendergast LA, Tham R, et al. Outdoor pollen is a trigger of child and adolescent asthma emergency department presentations: a systematic review and meta-analysis. Allergy (2018) 73(8):1632–41. doi: 10.1111/all.13407
45. Shrestha SK, Lambert KA, Erbas B. Ambient pollen concentrations and asthma hospitalization in children and adolescents: a systematic review and meta-analysis. J Asthma (2021) 58(9):1155–68. doi: 10.1080/02770903.2020.1771726
46. Annesi-Maesano I, Cecchi L, Biagioni B, Chung KF, Clot B, Collaud Coen M, et al. Is exposure to pollen a risk factor for moderate and severe asthma exacerbations? Allergy (2023) 78(8):2121–47. doi: 10.1111/all.15724
47. D’Amato G, Annesi Maesano I, Molino A, Vitale C, D’Amato M. Thunderstorm-related asthma attacks. J Allergy Clin Immunol (2017) 139(6):1786–7. doi: 10.1016/j.jaci.2017.03.003
48. Suphioglu C, Singh MB, Taylor P, Bellomo R, Holmes P, Puy R, et al. Mechanism of grass-pollen-induced asthma. Lancet (1992) 339(8793):569–72. doi: 10.1016/0140-6736(92)90864-y
49. Girgis ST, Marks GB, Downs SH, Kolbe A, Car GN, Paton R. Thunderstorm-associated asthma in an inland town in south-eastern Australia. Who is at risk? Eur Respir J (2000) 16(1):3–8. doi: 10.1034/j.1399-3003.2000.16a02.x
50. GBD 2019 Risk Factors Collaborators. Global burden of 87 risk factors in 204 countries and territories, 1990-2019: a systematic analysis for the Global Burden of Disease Study 2019. Lancet (2020) 396(10258):1223–49. doi: 10.1016/S0140-6736(20)30752-2
51. Ritchie H, Roser M. Air pollution (2021). Available at: https://ourworldindata.org/air-pollution
52. Landrigan PJ, Fuller R, Acosta NJR, Adeyi O, Arnold R, Basu NN, et al. The Lancet Commission on pollution and health. Lancet (2018) 391(10119):462–512. doi: 10.1016/S0140-6736(17)32345-0
53. Fuller R, Landrigan PJ, Balakrishnan K, Bathan G, Bose-O’Reilly S, Brauer M, et al. Pollution and health: a progress update. Lancet Planet Health (2022) 6(6):e535–47. doi: 10.1016/S2542-5196(22)00090-0
54. Kahle JJ, Neas LM, Devlin RB, Case MW, Schmitt MT, Madden MC, et al. Interaction effects of temperature and ozone on lung function and markers of systemic inflammation, coagulation, and fibrinolysis: a crossover study of healthy young volunteers. Environ Health Perspect (2015) 123(4):310–6. doi: 10.1289/ehp.1307986
55. Arjomandi M, Balmes JR, Frampton MW, Bromberg P, Rich DQ, Stark P, et al. Respiratory responses to ozone exposure. MOSES (the multicenter ozone study in older subjects). Am J Respir Crit Care Med (2018) 197(10):1319–27. doi: 10.1164/rccm.201708-1613OC
56. Rebuli ME, Speen AM, Martin EM, Addo KA, Pawlak EA, Glista-Baker E, et al. Wood smoke exposure alters human inflammatory responses to viral infection in a sex-specific manner. A randomized, placebo-controlled study. Am J Respir Crit Care Med (2019) 199(8):996–1007. doi: 10.1164/rccm.201807-1287OC
57. Schwartz C, Bølling AK, Carlsten C. Controlled human exposures to wood smoke: a synthesis of the evidence. Part Fibre Toxicol (2020) 17(1):49. doi: 10.1186/s12989-020-00375-x
58. Hernandez ML, Ivins S, Chason K, Burbank AJ, Rebuli ME, Kobernick A, et al. Respiratory effects of sedentary ozone exposure at the 70-ppb national ambient air quality standard: a randomized clinical trial. Am J Respir Crit Care Med (2021) 203(7):910–3. doi: 10.1164/rccm.202006-2597LE
59. Alexis NE, Zhou LY, Burbank AJ, Almond M, Hernandez ML, Mills KH, et al. Development of a screening protocol to identify persons who are responsive to wood smoke particle-induced airway inflammation with pilot assessment of GSTM1 genotype and asthma status as response modifiers. Inhal Toxicol (2022) 34(11-12):329–39. doi: 10.1080/08958378.2022.2110334
60. Frampton MW, Balmes JR, Bromberg PA, Arjomandi M, Hazucha MJ, Thurston SW, et al. Effects of short-term increases in personal and ambient pollutant concentrations on pulmonary and cardiovascular function: a panel study analysis of the Multicenter Ozone Study in oldEr subjects (MOSES 2). Environ Res (2022) 205:112522. doi: 10.1016/j.envres.2021.112522
61. Hansson A, Rankin G, Uski O, Friberg M, Pourazar J, Lindgren R, et al. Reduced bronchoalveolar macrophage phagocytosis and cytotoxic effects after controlled short-term exposure to wood smoke in healthy humans. Part Fibre Toxicol (2023) 20(1):30. doi: 10.1186/s12989-023-00541-x
62. Skevaki C, Nadeau KC, Rothenberg ME, Alahmad B, Mmbaga BT, Masenga GG, et al. Impact of climate change on immune responses and barrier defense. J Allergy Clin Immunol (2024), S0091–6749(24)00119-2. doi: 10.1016/j.jaci.2024.01.016
63. Beerweiler CC, Masanetz RK, Schaub B. Asthma and allergic diseases: cross talk of immune system and environmental factors. Eur J Immunol (2023) 53(6):e2249981. doi: 10.1002/eji.202249981
64. Cui Y, Xiao Q, Yuan Y, Zhuang Y, Hao W, Jiang J, et al. Ozone-oxidized black carbon particles change macrophage fate: crosstalk between necroptosis and macrophage extracellular traps. Environ Pollut (2023) 329:121655. doi: 10.1016/j.envpol.2023.121655
65. Ural BB, Caron DP, Dogra P, Wells SB, Szabo PA, Granot T, et al. Inhaled particulate accumulation with age impairs immune function and architecture in human lung lymph nodes. Nat Med (2022) 28(12):2622–32. doi: 10.1038/s41591-022-02073-x
66. Ishihara Y, Kado SY, Bein KJ, He Y, Pouraryan AA, Urban A, et al. Aryl hydrocarbon receptor signaling synergizes with TLR/NF-κB-signaling for induction of IL-22 through canonical and non-canonical AhR pathways. Front Toxicol (2021) 3:787360. doi: 10.3389/ftox.2021.787360
67. Akdis CA, Nadeau KC. Human and planetary health on fire. Nat Rev Immunol (2022) 22(11):651–2. doi: 10.1038/s41577-022-00776-3
68. Hirota JA, Gold MJ, Hiebert PR, Parkinson LG, Wee T, Smith D, et al. The nucleotide-binding domain, leucine-rich repeat protein 3 inflammasome/IL-1 receptor I axis mediates innate, but not adaptive, immune responses after exposure to particulate matter under 10 μm. Am J Respir Cell Mol Biol (2015) 52(1):96–105. doi: 10.1165/rcmb.2014-0158OC
69. Mou Y, Liao W, Liang Y, Li Y, Zhao M, Guo Y, et al. Environmental pollutants induce NLRP3 inflammasome activation and pyroptosis: roles and mechanisms in various diseases. Sci Total Environ (2023) 900:165851. doi: 10.1016/j.scitotenv.2023.165851
70. Wang LQ, Liu T, Yang S, Sun L, Zhao ZY, Li LY, et al. Perfluoroalkyl substance pollutants activate the innate immune system through the AIM2 inflammasome. Nat Commun (2021) 12(1):2915. doi: 10.1038/s41467-021-23201-0
71. Alijagic A, Hedbrant A, Persson A, Larsson M, Engwall M, Särndahl E. NLRP3 inflammasome as a sensor of micro- and nanoplastics immunotoxicity. Front Immunol (2023) 14:1178434. doi: 10.3389/fimmu.2023.1178434
72. Pan WC, Wu CD, Chen MJ, Huang YT, Chen CJ, Su HJ, et al. Fine particle pollution, alanine transaminase, and liver cancer: a Taiwanese prospective cohort study (REVEAL-HBV). J Natl Cancer Inst (2016) 108(3):djv341. doi: 10.1093/jnci/djv341
73. Arias-Pérez RD, Taborda NA, Gómez DM, Narvaez JF, Porras J, Hernandez JC. Inflammatory effects of particulate matter air pollution. Environ Sci Pollut Res Int (2020) 27(34):42390–404. doi: 10.1007/s11356-020-10574-w
74. ArulJothi KN, Kumaran K, Senthil S, Nidhu AB, Munaff N, Janitri VB, et al. Implications of reactive oxygen species in lung cancer and exploiting it for therapeutic interventions. Med Oncol (2022) 40(1):43. doi: 10.1007/s12032-022-01900-y
75. Hill W, Lim EL, Weeden CE, Lee C, Augustine M, Chen K, et al. Lung adenocarcinoma promotion by air pollutants. Nature (2023) 616(7955):159–67. doi: 10.1038/s41586-023-05874-3
76. Shiravand Y, Khodadadi F, Kashani SMA, Hosseini-Fard SR, Hosseini S, Sadeghirad H, et al. Immune checkpoint inhibitors in cancer therapy. Curr Oncol (2022) 29(5):3044–60. doi: 10.3390/curroncol29050247
77. Ritchie H, Roser M. Outdoor air pollution (2022). Available at: https://ourworldindata.org/outdoor-air-pollution
78. Adamkiewicz G, Liddie J, Gaffin JM. The respiratory risks of ambient/outdoor air pollution. Clin Chest Med (2020) 41(4):809–24. doi: 10.1016/j.ccm.2020.08.013
79. Nanda A, Mustafa SS, Castillo M, Bernstein JA. Air pollution effects in allergies and asthma. Immunol Allergy Clin North Am (2022) 42(4):801–15. doi: 10.1016/j.iac.2022.06.004
80. Bi J, D’Souza RR, Moss S, Senthilkumar N, Russell AG, Scovronick NC, et al. Acute effects of ambient air pollution on asthma emergency department visits in ten U.S. States. Environ Health Perspect (2023) 131(4):47003. doi: 10.1289/EHP11661
81. Mukharesh L, Phipatanakul W, Gaffin JM. Air pollution and childhood asthma. Curr Opin Allergy Clin Immunol (2023) 23(2):100–10. doi: 10.1097/ACI.0000000000000881
82. Shaikh HS, Muhammad AIP, Ali A. Air pollution and increasing asthma hospitalizations: a looming healthcare crisis? J Pak Med Assoc (2023) 73(3):739. doi: 10.47391/JPMA.7545
83. Zheng XY, Orellano P, Lin HL, Jiang M, Guan WJ. Short-term exposure to ozone, nitrogen dioxide, and sulphur dioxide and emergency department visits and hospital admissions due to asthma: a systematic review and meta-analysis. Environ Int (2021) 150:106435. doi: 10.1016/j.envint.2021.106435
84. Huang J, Yang X, Fan F, Hu Y, Wang X, Zhu S, et al. Outdoor air pollution and the risk of asthma exacerbations in single lag0 and lag1 exposure patterns: a systematic review and meta-analysis. J Asthma (2022) 59(11):2322–39. doi: 10.1080/02770903.2021.2008429
85. Yan W, Wang X, Dong T, Sun M, Zhang M, Fang K, et al. The impact of prenatal exposure to PM2.5 on childhood asthma and wheezing: a meta-analysis of observational studies. Environ Sci Pollut Res Int (2020) 27(23):29280–90. doi: 10.1007/s11356-020-09014-6
86. Hua L, Ju L, Xu H, Li C, Sun S, Zhang Q, et al. Outdoor air pollution exposure and the risk of asthma and wheezing in the offspring. Environ Sci Pollut Res Int (2023) 30(6):14165–89. doi: 10.1007/s11356-022-23094-6
87. Boogaard H, Patton AP, Atkinson RW, Brook JR, Chang HH, Crouse DL, et al. Long-term exposure to traffic-related air pollution and selected health outcomes: A systematic review and meta-analysis. Environ Int (2022) 164:107262. doi: 10.1016/j.envint.2022.107262
88. Perera F, Ashrafi A, Kinney P, Mills D. Towards a fuller assessment of benefits to children’s health of reducing air pollution and mitigating climate change due to fossil fuel combustion. Environ Res (2019) 172:55–72. doi: 10.1016/j.envres.2018.12.016
89. Haikerwal A, Akram M, Sim MR, Meyer M, Abramson MJ, Dennekamp M. Fine particulate matter (PM2.5) exposure during a prolonged wildfire period and emergency department visits for asthma. Respirology (2016) 21(1):88–94. doi: 10.1111/resp.12613
90. Doiron D, de Hoogh K, Probst-Hensch N, Fortier I, Cai Y, De Matteis S, et al. Air pollution, lung function and COPD: results from the population-based UK Biobank study. Eur Respir J (2019) 54(1):1802140. doi: 10.1183/13993003.02140-2018
91. Turner MC, Andersen ZJ, Baccarelli A, Diver WR, Gapstur SM, Pope CA 3rd, et al. Outdoor air pollution and cancer: an overview of the current evidence and public health recommendations. CA Cancer J Clin (2020) 70(6):460–79. doi: 10.3322/caac.21632
92. Zhao N, Smargiassi A, Jean S, Gamache P, Laouan-Sidi EA, Chen H, et al. Long-term exposure to fine particulate matter and ozone and the onset of systemic autoimmune rheumatic diseases: an open cohort study in Quebec, Canada. Arthritis Res Ther (2022) 24(1):151. doi: 10.1186/s13075-022-02843-5
93. United States Environmental Protection Agency. Climate change indicators: wildfires (2022). Available at: https://www.epa.gov/climate-indicators/climate-change-indicators-wildfires
94. Burke M, Driscoll A, Heft-Neal S, Xue J, Burney J, Wara M. The changing risk and burden of wildfire in the United States. Proc Natl Acad Sci USA (2021) 118(2):e2011048118. doi: 10.1073/pnas.2011048118
95. Van der Werf GR, Morton DC, DeFries RS, Olivier JGJ, Kasibhatla PS, Jackson RB, et al. CO2 emissions from forest loss. Nat Geosci (2009) 2(11):737–8. doi: 10.1038/ngeo671
96. Prunicki MM, Dant CC, Cao S, Maecker H, Haddad F, Kim JB, et al. Immunologic effects of forest fire exposure show increases in IL-1β and CRP. Allergy (2020) 75(9):2356–8. doi: 10.1111/all.14251
97. Coronel G, Pastén M, Breuer N, Celeste A, Rejalaga L, Domecq FM, et al. Wildfires in Paraguay: environmental and human impacts. In: Leal Filho W, Azeiteiro UM, Setti AFF, editors. Sustainability in Natural Resources Management and Land Planning. Cham: Springer International Publishing (2021) 429–44. doi: 10.1007/978-3-030-76624-5_25
98. Xu R, Yu P, Abramson MJ, Johnston FH, Samet JM, Bell ML, et al. Wildfires, global climate change, and human health. N Engl J Med (2020) 383(22):2173–81. doi: 10.1056/NEJMsr2028985
99. Blando J, Allen M, Galadima H, Tolson T, Akpinar-Elci M, Szklo-Coxe M. Observations of delayed changes in respiratory function among allergy clinic patients exposed to wildfire smoke. Int J Environ Res Public Health (2022) 19(3):1241. doi: 10.3390/ijerph19031241
100. Fadadu RP, Grimes B, Jewell NP, Vargo J, Young AT, Abuabara K, et al. Association of wildfire air pollution and health care use for atopic dermatitis and itch. JAMA Dermatol (2021) 157(6):658–66. doi: 10.1001/jamadermatol.2021.0179
101. Kok JF, Adebiyi AA, Albani S, Balkanski Y, Checa-Garcia R, Chin M, et al. Contribution of the world’s main dust source regions to the global cycle of desert dust. Atmos Chem Phys (2021) 21(10):8169–93. doi: 10.5194/acp-21-8169-2021
102. Goudie AS. Dust storms: recent developments. J Environ Manage (2009) 90(1):89–94. doi: 10.1016/j.jenvman.2008.07.007
103. Thalib L, Al-Taiar A. Dust storms and the risk of asthma admissions to hospitals in Kuwait. Sci Total Environ (2012) 433:347–51. doi: 10.1016/j.scitotenv.2012.06.082
104. Hashizume M, Kim Y, Ng CFS, Chung Y, Madaniyazi L, Bell ML, et al. Health effects of Asian dust: a systematic review and meta-analysis. Environ Health Perspect (2020) 128(6):66001. doi: 10.1289/EHP5312
105. Pearson D, Ebisu K, Wu X, Basu R. A review of coccidioidomycosis in California: exploring the intersection of land use, population movement, and climate change. Epidemiol Rev (2019) 41(1):145–57. doi: 10.1093/epirev/mxz004
106. Ritchie H, Roser M. Indoor air pollution (2022). Available at: https://ourworldindata.org/indoor-air-pollution
107. Krasner A, Jones TS, Jones TS, LaRocque R. Cooking with gas, household air pollution, and asthma: little recognized risk for children. J Environ Health (2021) 83(8):14–8.
108. Dherani M, Pope D, Mascarenhas M, Smith KR, Weber M, Bruce N. Indoor air pollution from unprocessed solid fuel use and pneumonia risk in children aged under five years: a systematic review and meta-analysis. Bull World Health Organ (2008) 86(5):390–8C. doi: 10.2471/blt.07.044529
109. Maung TZ, Bishop JE, Holt E, Turner AM, Pfrang C. Indoor air pollution and the health of vulnerable groups: A systematic review focused on particulate matter (PM), volatile organic compounds (VOCs) and their effects on children and people with pre-existing lung disease. Int J Environ Res Public Health (2022) 19(14):8752. doi: 10.3390/ijerph19148752
110. D’Amato G, Chong-Neto HJ, Monge Ortega OP, Vitale C, Ansotegui I, Rosario N, et al. The effects of climate change on respiratory allergy and asthma induced by pollen and mold allergens. Allergy (2020) 75(9):2219–28. doi: 10.1111/all.14476
111. Wang J, Zhao Z, Zhang Y, Li B, Huang C, Zhang X, et al. Asthma, allergic rhinitis and eczema among parents of preschool children in relation to climate, and dampness and mold in dwellings in China. Environ Int (2019) 130:104910. doi: 10.1016/j.envint.2019.104910
112. Li S, Cao S, Duan X, Zhang Y, Gong J, Xu X, et al. Household mold exposure in association with childhood asthma and allergic rhinitis in a northwestern city and a southern city of China. J Thorac Dis (2022) 14(5):1725–37. doi: 10.21037/jtd-21-1380
113. García-Lledó A, Rodríguez-Martín S, Tobías A, Alonso-Martín J, Ansede-Cascudo JC, de Abajo FJ. Heat waves, ambient temperature, and risk of myocardial infarction: an ecological study in the Community of Madrid. Rev Esp Cardiol (Engl Ed) (2020) 73(4):300–6. doi: 10.1016/j.rec.2019.05.016
114. Medina E, Kim SH, Yun M, Choi WG. Recapitulation of the function and role of ROS Generated in response to heat stress in plants. Plants (Basel) (2021) 10(2):371. doi: 10.3390/plants10020371
115. Schieber M, Chandel NS. ROS function in redox signaling and oxidative stress. Curr Biol (2014) 24(10):R453–62. doi: 10.1016/j.cub.2014.03.034
116. van Eden W. Immune tolerance therapies for autoimmune diseases based on heat shock protein T-cell epitopes. Philos Trans R Soc Lond B Biol Sci (2018) 373(1738):20160531. doi: 10.1098/rstb.2016.0531
117. Heled Y, Fleischmann C, Epstein Y. Cytokines and their role in hyperthermia and heat stroke. J Basic Clin Physiol Pharmacol (2013) 24(2):85–96. doi: 10.1515/jbcpp-2012-0040
118. Martine P, Rébé C. Heat shock proteins and inflammasomes. Int J Mol Sci (2019) 20(18):4508. doi: 10.3390/ijms20184508
119. Ben Abdallah H, Seeler S, Bregnhøj A, Ghatnekar G, Kristensen LS, Iversen L, et al. Heat shock protein 90 inhibitor RGRN-305 potently attenuates skin inflammation. Front Immunol (2023) 14:1128897. doi: 10.3389/fimmu.2023.1128897
120. Xu Z, Huang C, Hu W, Turner LR, Su H, Tong S. Extreme temperatures and emergency department admissions for childhood asthma in Brisbane, Australia. Occup Environ Med (2013) 70(10):730–5. doi: 10.1136/oemed-2013-101538
121. Lu C, Zhang Y, Li B, Zhao Z, Huang C, Zhang X, et al. Interaction effect of prenatal and postnatal exposure to ambient air pollution and temperature on childhood asthma. Environ Int (2022) 167:107456. doi: 10.1016/j.envint.2022.107456
122. Sampath V, Shalakhti O, Veidis E, Efobi JAI, Shamji MH, Agache I, et al. Acute and chronic impacts of heat stress on planetary health. Allergy (2023) 78(8):2109–20. doi: 10.1111/all.15702
123. Roths M, Freestone AD, Rudolph TE, Michael A, Baumgard LH, Selsby JT. Environment-induced heat stress causes structural and biochemical changes in the heart. J Therm Biol (2023) 113:103492. doi: 10.1016/j.jtherbio.2023.103492
124. Kenny GP, Yardley J, Brown C, Sigal RJ, Jay O. Heat stress in older individuals and patients with common chronic diseases. CMAJ (2010) 182(10):1053–60. doi: 10.1503/cmaj.081050
125. World Health Organization. Heat and health. Available at: https://www.who.int/news-room/fact-sheets/detail/climate-change-heat-and-health
126. United Nations. World urbanization prospects. 2014 revision (2014). Available at: https://www.un.org/en/development/desa/publications/2014-revision-world-urbanization-prospects.html
127. Ponte EV, Lima A, Almeida PCA, de Jesus JPV, Souza-MaChado A, Barreto ML, et al. Rural to urban migration contributes to the high burden of asthma in the urban area. Clin Respir J (2019) 13(9):560–6. doi: 10.1111/crj.13058
128. Grant T, Croce E, Matsui EC. Asthma and the social determinants of health. Ann Allergy Asthma Immunol (2022) 128(1):5–11. doi: 10.1016/j.anai.2021.10.002
129. Gold DR, Adamkiewicz G, Arshad SH, Celedón JC, Chapman MD, Chew GL, et al. NIAID, NIEHS, NHLBI, and MCAN Workshop Report: the indoor environment and childhood asthma-implications for home environmental intervention in asthma prevention and management. J Allergy Clin Immunol (2017) 140(4):933–49. doi: 10.1016/j.jaci.2017.04.024
130. European Centre for Disease Prevention and Control. Rodent-borne diseases (2022). Available at: https://www.ecdc.europa.eu/en/climate-change/climate-change-europe/rodent-borne-diseases
131. Ahluwalia SK, Peng RD, Breysse PN, Diette GB, Curtin-Brosnan J, Aloe C, et al. Mouse allergen is the major allergen of public health relevance in Baltimore City. J Allergy Clin Immunol (2013) 132(4):830–5. doi: 10.1016/j.jaci.2013.05.005
132. Moghtaderi M, Hejrati Z, Dehghani Z, Dehghani F, Kolahi N. Sensitization to food additives in patients with allergy: A study based on skin test and open oral challenge. Iran J Allergy Asthma Immunol (2016) 15(3):198–203. Available at: https://ijaai.tums.ac.ir/index.php/ijaai/article/view/651
133. Wang M, Tan G, Eljaszewicz A, Meng Y, Wawrzyniak P, Acharya S, et al. Laundry detergents and detergent residue after rinsing directly disrupt tight junction barrier integrity in human bronchial epithelial cells. J Allergy Clin Immunol (2019) 143(5):1892–903. doi: 10.1016/j.jaci.2018.11.016
134. Emeny RT, Korrick SA, Li Z, Nadeau K, Madan J, Jackson B, et al. Prenatal exposure to mercury in relation to infant infections and respiratory symptoms in the New Hampshire Birth Cohort Study. Environ Res (2019) 171:523–9. doi: 10.1016/j.envres.2019.01.026
135. Roach K, Roberts J. A comprehensive summary of disease variants implicated in metal allergy. J Toxicol Environ Health B Crit Rev (2022) 25(6):279–341. doi: 10.1080/10937404.2022.2104981
136. Simon JC, Marchesi JR, Mougel C, Selosse MA. Host-microbiota interactions: from holobiont theory to analysis. Microbiome (2019) 7(1):5. doi: 10.1186/s40168-019-0619-4
137. Cardinale BJ, Duffy JE, Gonzalez A, Hooper DU, Perrings C, Venail P, et al. Biodiversity loss and its impact on humanity. Nature (2012) 486(7401):59–67. doi: 10.1038/nature11148
138. United Nations. United Nations Conference on Environment and Development (1992). Rio de Janeiro, Brazil. Available at: https://www.un.org/en/conferences/environment/rio1992
139. Wu L, Zhang Y, Guo X, Ning D, Zhou X, Feng J, et al. Reduction of microbial diversity in grassland soil is driven by long-term climate warming. Nat Microbiol (2022) 7(7):1054–62. doi: 10.1038/s41564-022-01147-3
140. Oliver TH, Isaac NJB, August TA, Woodcock BA, Roy DB, Bullock JM. Declining resilience of ecosystem functions under biodiversity loss. Nat Commun (2015) 6(1):10122. doi: 10.1038/ncomms10122
141. Moi DA, Lansac-Tôha FM, Romero GQ, Sobral-Souza T, Cardinale BJ, Kratina P, et al. Human pressure drives biodiversity–multifunctionality relationships in large neotropical wetlands. Nat Ecol Evol (2022) 6(9):1279–89. doi: 10.1038/s41559-022-01827-7
142. Pecl GT, Araújo MB, Bell JD, Blanchard J, Bonebrake TC, Chen IC, et al. Biodiversity redistribution under climate change: impacts on ecosystems and human well-being. Science (2017) 355(6332):eaai9214. doi: 10.1126/science.aai9214
143. Ege MJ, Mayer M, Normand AC, Genuneit J, Cookson WOCM, Braun-Fahrländer C, et al. Exposure to environmental microorganisms and childhood asthma. N Engl J Med (2011) 364(8):701–9. doi: 10.1056/NEJMoa1007302
144. Pfefferle PI, Büchele G, Blümer N, Roponen M, Ege MJ, Krauss-Etschmann S, et al. Cord blood cytokines are modulated by maternal farming activities and consumption of farm dairy products during pregnancy: the PASTURE Study. J Allergy Clin Immunol (2010) 125(1):108–115.e101–103. doi: 10.1016/j.jaci.2009.09.019
145. Patrick R, Snell T, Gunasiri H, Garad R, Meadows G, Enticott J. Prevalence and determinants of mental health related to climate change in Australia. Aust N Z J Psychiatry (2023) 57(5):710–24. doi: 10.1177/00048674221107872
146. Patterson AM, Yildiz VO, Klatt MD, Malarkey WB. Perceived stress predicts allergy flares. Ann Allergy Asthma Immunol (2014) 112(4):317–21. doi: 10.1016/j.anai.2013.07.013
147. Ohno I. Neuropsychiatry phenotype in asthma: psychological stress-induced alterations of the neuroendocrine-immune system in allergic airway inflammation. Allergol Int (2017) 66S:S2–8. doi: 10.1016/j.alit.2017.06.005
148. Kawamoto T, Miyake Y, Tanaka K, Nagano J, Sasaki S, Hirota Y. Maternal prenatal stress and infantile wheeze and asthma: the Osaka Maternal and Child Health Study. J Psychosom Res (2020) 135:110143. doi: 10.1016/j.jpsychores.2020.110143
149. Miadich SA, Everhart RS, Greenlee J, Winter MA. The impact of cumulative stress on asthma outcomes among urban adolescents. J Adolesc (2020) 80:254–63. doi: 10.1016/j.adolescence.2019.12.007
150. Hickman C, Marks E, Pihkala P, Clayton S, Lewandowski RE, Mayall EE, et al. Climate anxiety in children and young people and their beliefs about government responses to climate change: a global survey. Lancet Planet Health (2021) 5(12):e863–73. doi: 10.1016/S2542-5196(21)00278-3
151. Agache I, Akdis CA. Precision medicine and phenotypes, endotypes, genotypes, regiotypes, and theratypes of allergic diseases. J Clin Invest (2019) 129(4):1493–503. doi: 10.1172/JCI124611
152. Pacheco SE, Guidos-Fogelbach G, Annesi-Maesano I, Pawankar R, D’Amato G, Latour-Staffeld P, et al. Climate change and global issues in allergy and immunology. J Allergy Clin Immunol (2021) 148(6):1366–77. doi: 10.1016/j.jaci.2021.10.011
153. Whitmee S, Haines A, Beyrer C, Boltz F, Capon AG, de Souza Dias BF, et al. Safeguarding human health in the Anthropocene epoch: report of the Rockefeller Foundation-Lancet Commission on planetary health. Lancet (2015) 386(10007):1973–2028. doi: 10.1016/S0140-6736(15)60901-1
154. World Health Organization. One Health. Available at: https://www.who.int/health-topics/one-health#tab=tab_1
155. Mackenzie JS, Jeggo M. The one health approach-why is it so important? Trop Med Infect Dis (2019) 4(2):88. doi: 10.3390/tropicalmed4020088
156. Haahtela T, Valovirta E, Saarinen K, Jantunen J, Lindström I, Kauppi P, et al. The Finnish Allergy Program 2008-2018: society-wide proactive program for change of management to mitigate allergy burden. J Allergy Clin Immunol (2021) 148(2):319–326.e4. doi: 10.1016/j.jaci.2021.03.037
157. Hämäläinen RM, Halonen JI, Haveri H, Prass M, Virtanen SM, Salomaa MM, Sieppi P, Haahtela T. Nature step to health 2022-2032: interorganizational collaboration to prevent human disease, nature loss, and climate crisis. J Climate Change and Health (2023) 10:100194.
158. Thornton J. Prescriptions for the planet. Lancet (2022) 400(10365):1756. doi: 10.1016/S0140-6736(22)02355-8
159. California Air Resources Board. Short-lived climate pollutants. Available at: https://ww2.arb.ca.gov/our-work/programs/slcp/about
160. Climate and clean air coalition. Methane’s links to respiratory diseases strengthens the case for its rapid reduction (2021). Available at: https://www.ccacoalition.org/en/news/methane
161. Kelly FJ, Mudway IS, Fussell JC. Air pollution and asthma: critical targets for effective action. Pulm Ther (2021) 7(1):9–24. doi: 10.1007/s41030-020-00138-1
162. United States Environmental Protection Agency. Clean Air Act requirements and history (2022). Available at: https://www.epa.gov/clean-air-act-overview/clean-air-act-requirements-and-history
163. Garcia E, Berhane KT, Islam T, McConnell R, Urman R, Chen Z, et al. Association of changes in air quality with incident asthma in children in California, 1993-2014. JAMA (2019) 321(19):1906–15. doi: 10.1001/jama.2019.5357
164. Loughner CP, Follette-Cook MB, Duncan BN, Hains J, Pickering KE, Moy J, et al. The benefits of lower ozone due to air pollution emission reductions (2002-2011) in the Eastern United States during extreme heat. J Air Waste Manag Assoc (2020) 70(2):193–205. doi: 10.1080/10962247.2019.1694089
165. Kim H, Kim H, Lee JT. Effect of air pollutant emission reduction policies on hospital visits for asthma in Seoul, Korea; Quasi-experimental study. Environ Int (2019) 132:104954. doi: 10.1016/j.envint.2019.104954
166. Schraufnagel DE, Balmes JR, De Matteis S, Hoffman B, Kim WJ, Perez-Padilla R, et al. Health benefits of air pollution reduction. Ann Am Thorac Soc (2019) 16(12):1478–87. doi: 10.1513/AnnalsATS.201907-538CME
167. Hasunuma H, Ishimaru Y, Yoda Y, Shima M. Decline of ambient air pollution levels due to measures to control automobile emissions and effects on the prevalence of respiratory and allergic disorders among children in Japan. Environ Res (2014) 131:111–8. doi: 10.1016/j.envres.2014.03.007
168. Pope CA 3rd. Respiratory disease associated with community air pollution and a steel mill, Utah Valley. Am J Public Health (1989) 79(5):623–8. doi: 10.2105/ajph.79.5.623
169. Friedman MS, Powell KE, Hutwagner L, Graham LM, Teague WG. Impact of changes in transportation and commuting behaviors during the 1996 Summer Olympic Games in Atlanta on air quality and childhood asthma. JAMA (2001) 285(7):897–905. doi: 10.1001/jama.285.7.897
170. Russell AG, Tolbert P, Henneman L, Abrams J, Liu C, Klein M, et al. Impacts of regulations on air quality and emergency department visits in the Atlanta metropolitan area, 1999-2013. Res Rep (Health Eff Inst) (2018) (195):1–93. Available at: https://www.healtheffects.org/publication/impacts-regulations-air-quality-and-emergency-department-visits-atlanta-metropolitan
171. Li Y, Wang W, Kan H, Xu X, Chen B. Air quality and outpatient visits for asthma in adults during the 2008 Summer Olympic Games in Beijing. Sci Total Environ (2010) 408(5):1226–7. doi: 10.1016/j.scitotenv.2009.11.035
172. Mu L, Deng F, Tian L, Li Y, Swanson M, Ying J, et al. Peak expiratory flow, breath rate and blood pressure in adults with changes in particulate matter air pollution during the Beijing Olympics: a panel study. Environ Res (2014) 133:4–11. doi: 10.1016/j.envres.2014.05.006
173. Howden-Chapman P, Pierse N, Nicholls S, Gillespie-Bennett J, Viggers H, Cunningham M, et al. Effects of improved home heating on asthma in community dwelling children: randomised controlled trial. BMJ (2008) 337:a1411. doi: 10.1136/bmj.a1411
174. Marks GB, Ezz W, Aust N, Toelle BG, Xuan W, Belousova E, et al. Respiratory health effects of exposure to low-NOx unflued gas heaters in the classroom: a double-blind, cluster-randomized, crossover study. Environ Health Perspect (2010) 118(10):1476–82. doi: 10.1289/ehp.1002186
175. Pilotto LS, Nitschke M, Smith BJ, Pisaniello D, Ruffin RE, McElroy HJ, et al. Randomized controlled trial of unflued gas heater replacement on respiratory health of asthmatic schoolchildren. Int J Epidemiol (2004) 33(1):208–14. doi: 10.1093/ije/dyh018
176. James C, Bernstein DI, Cox J, Ryan P, Wolfe C, Jandarov R, et al. HEPA filtration improves asthma control in children exposed to traffic-related airborne particles. Indoor Air (2020) 30(2):235–43. doi: 10.1111/ina.12625
177. Grant TL, Wood RA. The influence of urban exposures and residence on childhood asthma. Pediatr Allergy Immunol (2022) 33(5):e13784. doi: 10.1111/pai.13784
178. Aris IM, Perng W, Dabelea D, Padula AM, Alshawabkeh A, Vélez-Vega CM, et al. Neighborhood opportunity and vulnerability and incident asthma among children. JAMA Pediatr (2023) 177(10):1055–64. doi: 10.1001/jamapediatrics.2023.3133
179. Pollack CE, Roberts LC, Peng RD, Cimbolic P, Judy D, Balcer-Whaley S, et al. Association of a housing mobility program with childhood asthma symptoms and exacerbations. JAMA (2023) 329(19):1671–81. doi: 10.1001/jama.2023.6488
180. Bryant-Stephens TC, Strane D, Robinson EK, Bhambhani S, Kenyon CC. Housing and asthma disparities. J Allergy Clin Immunol (2021) 148(5):1121–9. doi: 10.1016/j.jaci.2021.09.023
181. Colton MD, Laurent JGC, MacNaughton P, Kane J, Bennett-Fripp M, Spengler J, et al. Health benefits of green public housing: associations with asthma morbidity and building-related symptoms. Am J Public Health (2015) 105(12):2482–9. doi: 10.2105/AJPH.2015.302793
182. Cohen DA, Fleming B, McDonald K, Graetz N, Paul M, Lillehei A, et al. A green New Deal for New York City housing authority (NYCHA) communities. Data Prog (2019). Available at: https://www.filesforprogress.org/reports/green-new-deal-public-housing-nycha-summary.pdf
183. Korfmacher KS, Holt KD. The potential for proactive housing inspections to inform public health interventions. J Public Health Manag Pract (2018) 24(5):444–7. doi: 10.1097/PHH.0000000000000757
184. Samuels EA, Taylor RA, Pendyal A, Shojaee A, Mainardi AS, Lemire ER, et al. Mapping emergency department asthma visits to identify poor-quality housing in New Haven, CT, USA: a retrospective cohort study. Lancet Public Health (2022) 7(8):e694–704. doi: 10.1016/S2468-2667(22)00143-8
185. Nwaru BI, Takkinen HM, Kaila M, Erkkola M, Ahonen S, Pekkanen J, et al. Food diversity in infancy and the risk of childhood asthma and allergies. J Allergy Clin Immunol (2014) 133(4):1084–91. doi: 10.1016/j.jaci.2013.12.1069
186. Nygaard UC, Xiao L, Nadeau KC, Hew KM, Lv N, Camargo CA, et al. Improved diet quality is associated with decreased concentrations of inflammatory markers in adults with uncontrolled asthma. Am J Clin Nutr (2021) 114(3):1012–27. doi: 10.1093/ajcn/nqab063
187. Willett W, Rockström J, Loken B, Springmann M, Lang T, Vermeulen S, et al. Food in the Anthropocene: the EAT-Lancet Commission on healthy diets from sustainable food systems. Lancet (2019) 393(10170):447–92. doi: 10.1016/S0140-6736(19)30212-0
188. EAT. The EAT-Lancet Commission on food, planet, health. Available at: https://eatforum.org/eat-lancet-commission/
189. Logan K, Bahnson HT, Ylescupidez A, Beyer K, Bellach J, Campbell DE, et al. Early introduction of peanut reduces peanut allergy across risk groups in pooled and causal inference analyses. Allergy (2023) 78(5):1307–18. doi: 10.1111/all.15597
190. Natural Resources Defense Council. Regenerative Agriculture 101 (2022). Available at: https://www.nrdc.org/stories/regenerative-agriculture-101#whatis
191. Haahtela T, Alenius H, Lehtimäki J, Sinkkonen A, Fyhrquist N, Hyöty H, et al. Immunological resilience and biodiversity for prevention of allergic diseases and asthma. Allergy (2021) 76(12):3613–26. doi: 10.1111/all.14895
192. Rojas-Rueda D, Nieuwenhuijsen MJ, Gascon M, Perez-Leon D, Mudu P. Green spaces and mortality: a systematic review and meta-analysis of cohort studies. Lancet Planet Health (2019) 3(11):e469–77. doi: 10.1016/S2542-5196(19)30215-3
193. Dong Y, Liu H, Zheng T. Association between green space structure and the prevalence of asthma: a case study of Toronto. Int J Environ Res Public Health (2021) 18(11):5852. doi: 10.3390/ijerph18115852
194. Bauwelinck M, Casas L, Nawrot TS, Nemery B, Trabelsi S, Thomas I, et al. Residing in urban areas with higher green space is associated with lower mortality risk: a census-based cohort study with ten years of follow-up. Environ Int (2021) 148:106365. doi: 10.1016/j.envint.2020.106365
195. Jarvis I, Davis Z, Sbihi H, Brauer M, Czekajlo A, Davies HW, et al. Assessing the association between lifetime exposure to greenspace and early childhood development and the mediation effects of air pollution and noise in Canada: a population-based birth cohort study. Lancet Planet Health (2021) 5(10):e709–17. doi: 10.1016/S2542-5196(21)00235-7
196. Pereira P, Baró F. Greening the city: thriving for biodiversity and sustainability. Sci Total Environ (2022) 817:153032. doi: 10.1016/j.scitotenv.2022.153032
197. Ogren TL. Allergy-free gardening: The revolutionary guide to healthy landscaping. Berkeley: Ten Speed Press (2000).
198. Fecho K, Haaland P, Krishnamurthy A, Lan B, Ramsey SA, Schmitt PL, et al. An approach for open multivariate analysis of integrated clinical and environmental exposures data. Inform Med Unlocked (2021) 26:100733. doi: 10.1016/j.imu.2021.100733
199. Fecho K, Ahalt SC, Appold S, Arunachalam S, Pfaff E, Stillwell L, et al. Development and application of an open tool for sharing and analyzing integrated clinical and environmental exposures data: asthma use case. JMIR Form Res (2022) 6(4):e32357. doi: 10.2196/32357
200. Fecho K, Ahalt SC, Knowles M, Krishnamurthy A, Leigh M, Morton K, et al. Leveraging open electronic health record data and environmental exposures data to derive insights into rare pulmonary disease. Front Artif Intell (2022) 5:918888. doi: 10.3389/frai.2022.918888
201. National Institutes of Health. Center for exposome research coordination to accelerate precision environmental health (2023). Available at: https://grants.nih.gov/grants/guide/rfa-files/RFA-ES-23-010.html
202. Manrai AK, Cui Y, Bushel PR, Hall M, Karakitsios S, Mattingly CJ, et al. Informatics and data analytics to support exposome-based discovery for public health. Annu Rev Public Health (2017) 38:279–94. doi: 10.1146/annurev-publhealth-082516-012737
203. De Santis D, Amici S, Milesi C, Muroni D, Romanino A, Casari C, et al. Tracking air quality trends and vehicle traffic dynamics at urban scale using satellite and ground data before and after the COVID-19 outbreak. Sci Total Environ (2023) 899:165464. doi: 10.1016/j.scitotenv.2023.165464
204. Zhang Y, He X, Lian G, Bai Y, Yang Y, Gong F, et al. Monitoring and spatial traceability of river water quality using Sentinel-2 satellite images. Sci Total Environ (2023) 894:164862. doi: 10.1016/j.scitotenv.2023.164862
205. Jin X, Fiore AM, Cohen RC. Space-based observations of ozone precursors within California wildfire plumes and the impacts on ozone-NOx-VOC chemistry. Environ Sci Technol (2023) 57(39):14648–60. doi: 10.1021/acs.est.3c04411
206. Adetona O, Simpson CD, Li Z, Sjodin A, Calafat AM, Naeher LP. Hydroxylated polycyclic aromatic hydrocarbons as biomarkers of exposure to wood smoke in wildland firefighters. J Expo Sci Environ Epidemiol (2017) 27(1):78–83. doi: 10.1038/jes.2015.75
207. Ciarrocca M, Rosati MV, Tomei F, Capozzella A, Andreozzi G, Tomei G, et al. Is urinary 1-hydroxypyrene a valid biomarker for exposure to air pollution in outdoor workers? A meta-analysis. J Expo Sci Environ Epidemiol (2014) 24(1):17–26. doi: 10.1038/jes.2012.111
208. Cheng H, Narzo AD, Howell D, Yevdokimova K, Zhang J, Zhang X, et al. Ambient air pollutants and traffic factors were associated with blood and urine biomarkers and asthma risk. Environ Sci Technol (2022) 56(11):7298–307. doi: 10.1021/acs.est.1c06916
209. Darras-Hostens M, Achour D, Muntaner M, Grare C, Zarcone G, Garçon G, et al. Short-term and residential exposure to air pollution: associations with inflammatory biomarker levels in adults living in northern France. Sci Total Environ (2022) 833:154985. doi: 10.1016/j.scitotenv.2022.154985
210. Chu H, Huang FQ, Yuan Q, Fan Y, Xin J, Du M, et al. Metabolomics identifying biomarkers of PM2.5 exposure for vulnerable population: based on a prospective cohort study. Environ Sci Pollut Res Int (2021) 28(12):14586–96. doi: 10.1007/s11356-020-11677-0
211. Long L, Zhu LT, Huang Q. Correlation between lung cancer markers and air pollutants in western China population. Environ Sci Pollut Res Int (2022) 29(42):64022–30. doi: 10.1007/s11356-022-20354-3
212. Misra N, Clavaud C, Guinot F, Bourokba N, Nouveau S, Mezzache S, et al. Multi-omics analysis to decipher the molecular link between chronic exposure to pollution and human skin dysfunction. Sci Rep (2021) 11(1):18302. doi: 10.1038/s41598-021-97572-1
213. Radzikowska U, Baerenfaller K, Cornejo-Garcia JA, Karaaslan C, Barletta E, Sarac BE, et al. Omics technologies in allergy and asthma research: an EAACI position paper. Allergy (2022) 77(10):2888–908. doi: 10.1111/all.15412
214. United States Environmental Protection Agency. Technical support document: Technical update of the social cost of carbon for regulatory impact analysis under executive order 12866 (2016). Available at: https://www.epa.gov/sites/default/files/2016-12/documents/sc_co2_tsd_august_2016.pdf
215. Cromar K, Howard P, Vásquez VN, Anthoff D. Health impacts of climate change as contained in economic models estimating the social cost of carbon dioxide. GeoHealth (2021) 5(8):e2021GH000405:e2021. doi: 10.1029/2021GH000405
216. Cromar KR, Anenberg SC, Balmes JR, Fawcett AA, Ghazipura M, Gohlke JM, et al. Global health impacts for economic models of climate change: a systematic review and meta-analysis. Ann Am Thorac Soc (2022) 19(7):1203–12. doi: 10.1513/AnnalsATS.202110-1193OC
217. London School of Hygiene and Tropical Medicine. Global study shows cutting carbon emissions and air pollution in cities has the potential to dramatically improve child health (2022) (2021). Available at: https://www.lshtm.ac.uk/newsevents/news/2021/global-study-shows-cutting-carbon-emissions-and-air-pollution-cities-has
218. Coomes KE, Buonocore JJ, Levy JI, Arter C, Arunachalam S, Buckley L, et al. Assessment of the health benefits to children of a transportation climate policy in New York City. Environ Res (2022) 215(3):114165. doi: 10.1016/j.envres.2022.114165
219. West JJ, Smith SJ, Silva RA, Naik V, Zhang Y, Adelman Z, et al. Co-benefits of global greenhouse gas mitigation for future air quality and human health. Nat Clim Chang (2013) 3(10):885–9. doi: 10.1038/NCLIMATE2009
220. Buonocore JJ, Lambert KF, Burtraw D, Sekar S, Driscoll CT. An analysis of costs and health co-benefits for a U.S. power plant carbon standard. PloS One (2016) 11(6):e0156308. doi: 10.1371/journal.pone.0156308
Keywords: air pollution, immune diseases, allergy, asthma, biodiversity, climate change, mitigation, pollen
Citation: Agache I, Akdis C, Akdis M, Al-Hemoud A, Annesi-Maesano I, Balmes J, Cecchi L, Damialis A, Haahtela T, Haber AL, Hart JE, Jutel M, Mitamura Y, Mmbaga BT, Oh J-W, Ostadtaghizadeh A, Pawankar R, Prunicki M, Renz H, Rice MB, Filho NAR, Sampath V, Skevaki C, Thien F, Traidl-Hoffmann C, Wong GWK and Nadeau KC. Immune-mediated disease caused by climate change-associated environmental hazards: mitigation and adaptation. Front Sci (2024) 2:1279192. doi: 10.3389/fsci.2024.1279192
Received: 17 August 2023; Accepted: 07 March 2024;
Published: 04 April 2024.
Edited by:
Lisa A. Miller, University of California, Davis, United StatesReviewed by:
Valérie Siroux, INSERM U1209 Institut pour l’Avancée des Biosciences (IAB), FranceDavid Peden, University of North Carolina Hospitals, United States
Copyright © 2024 Agache, Akdis, Akdis, Al-Hemoud, Annesi-Maesano, Balmes, Cecchi, Damialis, Haahtela, Haber, Hart, Jutel, Mitamura, Mmbaga, Oh, Ostadtaghizadeh, Pawankar, Prunicki, Renz, Rice, Filho, Sampath, Skevaki, Thien, Traidl-Hoffmann, Wong and Nadeau. This is an open-access article distributed under the terms of the Creative Commons Attribution License (CC BY). The use, distribution or reproduction in other forums is permitted, provided the original author(s) and the copyright owner(s) are credited and that the original publication in this journal is cited, in accordance with accepted academic practice. No use, distribution or reproduction is permitted which does not comply with these terms.
*Correspondence: Kari C. Nadeau, a25hZGVhdUBoc3BoLmhhcnZhcmQuZWR1