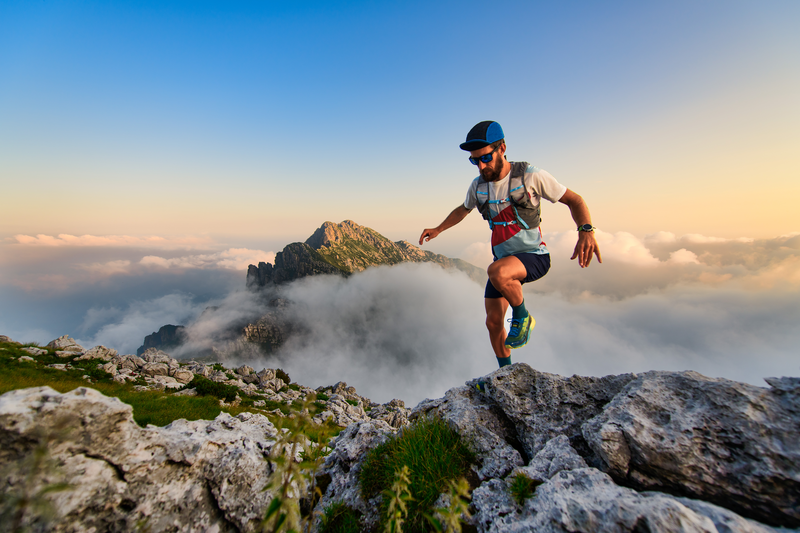
95% of researchers rate our articles as excellent or good
Learn more about the work of our research integrity team to safeguard the quality of each article we publish.
Find out more
ORIGINAL RESEARCH article
Front. Nutr.
Sec. Food Chemistry
Volume 12 - 2025 | doi: 10.3389/fnut.2025.1573987
This article is part of the Research Topic Applications of metabolomics in the formation of food flavor View all articles
The final, formatted version of the article will be published soon.
You have multiple emails registered with Frontiers:
Please enter your email address:
If you already have an account, please login
You don't have a Frontiers account ? You can register here
Stewing is a traditional processing method, commonly used for crayfish meat (Procambarus clarkii). In this study, we used a novel method called quantitative marinating (QM) to reduce industrial waste during crayfish meat processing. The taste, flavor, and aroma of crayfish meat processed by boiling (CON), stewing (SG), and QM were investigated. The results showed that crayfish meat in both SG and QM had higher L* and b* values (P < 0.05). Crayfish meat subjected to QM exhibited significantly greater hardness, gumminess, and chewiness than SG (P < 0.05), which was associated with tightly packed muscle fibers, as observed via scanning electron microscopy. Both QM and SG exhibited lower bitterness and astringency compared with CON, as tested by electronic tongue. A total of 25 types of FAAs content showed significant changes in QM and SG (P < 0.05), with the umami amino acids and total amino acids in QM increased by 19.47% and 52.97%, respectively, compared with SG.The results of flavor 5'-nucleotides showed that GMP, AMP, and IMP in QM increased by 72.87%, 48.78% and 51.98% compared with SG, respectively (P < 0.05).Headspace-gas chromatography-ion mobility spectrometry (HS-GC-IMS) identified 31 compounds, with QM having more volatile compounds such as anethole, linalool, and 1-octanol than SG. The levels of biogenic amines of tryptamine, phenethylamine, and histamine in QM decreased significantly compared with SG (P < 0.05). In conclusion, QM significantly improved the meat color, texture profile and taste-related qualities of crayfish meat while reducing the biogenic amines in crayfish meat.
Keywords: crayfish meat, Quantitative marinating, texture, Flavor compounds, biogenic amine
Received: 10 Feb 2025; Accepted: 31 Mar 2025.
Copyright: © 2025 Kang, Zhang, Fu, Ouyang, Wei, Lin, Jiang, Yu and Yang. This is an open-access article distributed under the terms of the Creative Commons Attribution License (CC BY). The use, distribution or reproduction in other forums is permitted, provided the original author(s) or licensor are credited and that the original publication in this journal is cited, in accordance with accepted academic practice. No use, distribution or reproduction is permitted which does not comply with these terms.
* Correspondence:
Meijuan Yu, Hunan Academy of Agricultural Sciences (CAAS), Changsha, China
Hui Yang, Hunan Academy of Agricultural Sciences (CAAS), Changsha, China
Disclaimer: All claims expressed in this article are solely those of the authors and do not necessarily represent those of their affiliated organizations, or those of the publisher, the editors and the reviewers. Any product that may be evaluated in this article or claim that may be made by its manufacturer is not guaranteed or endorsed by the publisher.
Research integrity at Frontiers
Learn more about the work of our research integrity team to safeguard the quality of each article we publish.