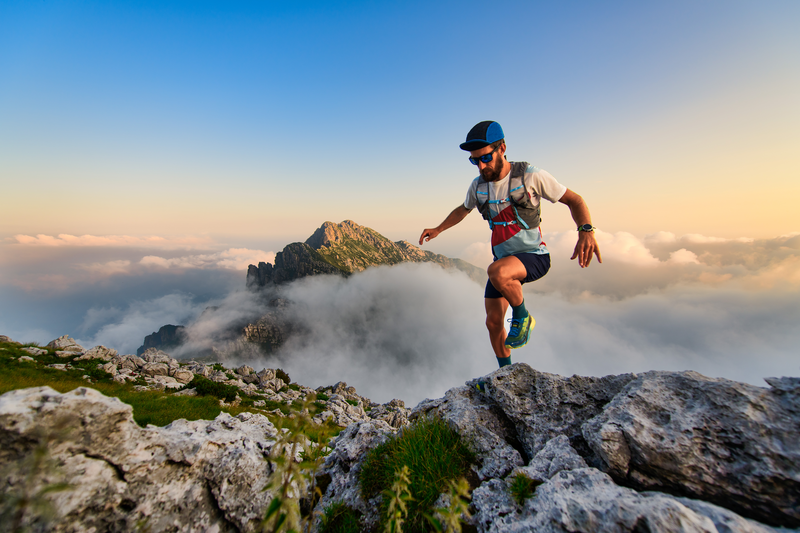
95% of researchers rate our articles as excellent or good
Learn more about the work of our research integrity team to safeguard the quality of each article we publish.
Find out more
ORIGINAL RESEARCH article
Front. Nutr. , 01 April 2025
Sec. Nutritional Epidemiology
Volume 12 - 2025 | https://doi.org/10.3389/fnut.2025.1551822
Background: While the role of specific nutrients in cancer is established, associations between comprehensive between dietary nutrient intake and cancer presence remain underexplored. This cross-sectional study investigates global dietary nutrient profiles in relation to solid and blood cancers.
Methods: A total of 42,732 mobile adults from the National Health and Nutrition Examination Survey (NHANES, 2001–2023) were enrolled in this study. The potential associations of dietary intakes of 34 nutrients and 4 common trace components with cancer presence were investigated by weighted logistic regression and restricted cubic spline.
Results: Higher intake of saturated fatty acid (OR = 1.1082, 95% CI: 1.0110–1.2146), β-carotene (OR = 1.0431, 1.0096–1.0777) and vitamin K (OR = 1.0370, 1.0094–1.0654) was positively associated with overall cancer presence, while phosphorus intake (OR = 0.9016, 0.8218–0.9892) showed a protective association. For solid tumors, dietary intakes of saturated fatty acid (OR = 1.1099), α-carotene (OR = 1.0353), β-carotene (OR = 1.0484), and vitamin K (OR = 1.0405) exhibited positive associations. Retinol intake was linked to blood carcinoma (OR = 1.0935, 1.0222–1.1698). Dose–response analyses revealed linear relationships without non-linear thresholds.
Conclusion: Specific dietary nutrients, notably saturated fats, carotenoids, and vitamin K, are associated with increased cancer presence, whereas phosphorus intake is associated with the reduced cancer presence. Due to the cross-sectional nature of the study and the measurement of dietary intake after cancer diagnosis, a causal relationship could not be established. These findings underscore the need for longitudinal studies to establish causality and inform dietary interventions in cancer management.
Cancer is the second leading cause of death on a global scale (1), with projections indicating a rising cancer-related disease burden over the coming decades (2). In 2024, the United States anticipates 2,001,140 new cancer cases and 611,720 deaths (3). By 2040, annual global cases may reach 29.9 million, with 15.3 million fatalities (4). Despite therapeutic advances, cancer biology remains complex (5), and the tumor microenvironment dynamically supports progression (6), complicating treatment. Diet critically influences tumor metabolism (7, 8), as nutrient adjustments alter tumor resource availability (9). Cancer cells adapt to nutrient scarcity by disrupting host homeostasis (10). Nutrient-deprivation therapies show broad efficacy (11–14), but benefits are short-lived due to tumor metabolic plasticity—for example, recruiting nerves to sustain growth under nutrient stress (14).
Solid tumors (e.g., breast, colorectal cancers) exploit stromal interactions and angiogenesis in nutrient-poor settings (14, 15). Conversely, blood cancers (e.g., leukemia, lymphoma) utilize systemic nutrients via bone marrow and circulation (16). Leukemia cells depend on lipid metabolism (17), while solid tumors reprogram glucose/glutamine pathways (18). These differences suggest cancer-specific nutritional strategies. Importantly, both cancer types share oxidative stress modulation and metabolic flexibility during dietary changes (19). Saturated fatty acids drive progression in solid tumors (breast, prostate, and colorectal) (20) and leukemia (21), urging cross-category dietary studies. Thus, understanding how combined nutrients regulate cancers is a translational priority.
Studies have investigated isolated nutrients: dietary fiber (22, 23), fatty acids (20, 24–26), β-carotene (27, 28), vitamin D (29–31), vitamin K (32, 33), caffeine (34, 35), selenium (36, 37), and others (38–40). Meta-analyses confirm nutrient-cancer links (41), but mechanisms lack consensus. Three gaps persist: First, research favors solid tumors (24, 42), neglecting blood cancers. Second, most studies focus on single nutrients, not dietary patterns. Third, cross-cancer analyses are methodologically limited—e.g., 30-nutrient studies on gynecological cancers (43), 15-micronutrient assessments in endometrial cancer (44), or 150-factor machine learning models in cervical cancer (45). While revealing specific associations, these lack systematic comparisons between solid and blood cancers.
In the present study, we sought to explore the association of global dietary nutrient intake with the presence of cancer, solid cancer, and blood cancers. To this end, the National Health and Nutrition Examination Survey (NHANES, 2001–2023) was utilized as a database, as it is recognized as an internationally authoritative, population-based survey. The analysis focused on the relationship between dietary nutrient intake and cancer among participating American populations. Furthermore, we sought to refine our understanding of the relationship between specific dietary nutrients and the presence of cancer, taking into account global dietary intake. A comprehensive understanding of the relationship between dietary nutrient intake and cancer, particularly solid and blood cancer, will provide a critical adjunct to subsequent cancer treatment.
The National Health and Nutrition Examination Survey (NHANES) is a nationally representative U.S. health survey that annually collects demographic, dietary, and clinical data from approximately 10,000 mobile adults (46). For this analysis, a total of 42,732 participants (2001–2023 cycles) meeting three criteria: For this analysis, we included 42,732 participants (2001–2023 cycles) meeting three criteria: (1) age ≥ 20 years; (2) complete cancer diagnosis records and dietary assessments; (3) full covariate data [including age, sex, race, immigration status, education level, poverty-income ratio (PIR), marital status, health insurance, Smoking status, BMI, physical activity level, and total energy intake]. Individuals lacking critical information were excluded. Study reporting followed STROBE guidelines (47), with participant selection detailed in Figure 1.
Nutrient intake was assessed using two consecutive 24-h dietary recalls from NHANES questionnaires (48). The geometric mean of both recordings was calculated to account for day-to-day variability (49). The first recall occurred in-person at Mobile Examination Centers (MEC), followed by a telephone-administered second recall (50).
Initially, the NHANES data from 2001 to 2023 was merged (comprising a total of 11 cycles). Subsequently, subjects were subjected to a multifaceted screening process, stratifying them based on their cancer-related information, dietary intake data, socio-demographic characteristics, lifestyle habits, and BMI. This comprehensive approach ultimately resulted in the enrollment of 42,732 subjects.
We analyzed 38 dietary components categorized as: Macronutrients: Protein, Carbohydrate, Total sugars, Dietary fiber, Total fat, Saturated fatty acids (SFA), Monounsaturated fatty acids (MUFA), Polyunsaturated fatty acids (PUFA), Cholesterol; Vitamins: Vitamin E, Retinol, Vitamin A, α-carotene, β-carotene, β-cryptoxanthin, Lycopene, Lutein + zeaxanthin, Thiamin (B1), Riboflavin (B2), Niacin, Folate, Vitamin B12, Ascorbic acid (C), Vitamin K; Minerals: Calcium, Phosphorus, Magnesium, Iron, Zinc, Copper, Sodium, Potassium, Selenium; Other: Caffeine, Theobromine, Moisture, Alcohol.
The presence of carcinoma was determined by the question: ‘Have you ever been told by a doctor or other health professional that you had cancer or a malignancy of any kind?’. The question ‘What kind of cancer was it?’ was used to determine whether the cancer was solid or blood. Participants reporting blood-related malignancies (‘Blood’, ‘Leukemia’, or ‘Lymphoma’) were classified as blood cancer cases (51). All other cancer types were categorized as solid tumors based on standardized classifications (52, 53).
We adjusted for covariates spanning three domains: Demographics: Age, sex, race/ethnicity (Hispanic, non-Hispanic White, non-Hispanic Black, other), and immigration status; Socioeconomic: Education level (<high school, high school, >high school), PIR (<1, 1–3, >3), marital status, and health insurance; Lifestyle/Clinical: Smoking status (never/former/current), BMI, physical activity level (sedentary/moderate/vigorous), total energy intake.
We performed weighted logistic regression to evaluate associations between nutrient intakes and the presence of cancer calculating adjusted odds ratios (ORs) with 95% confidence intervals (CIs). NHANES dietary weights were applied to ensure national representativeness. To explore potential non-linear relationships, restricted cubic splines (RCS) with four knots were modeled for significant nutrients. Continuous variables were summarized as median (interquartile range) and categorical variables as frequency (%). Between-group comparisons used Pearson’s χ2 test for categorical data and Kruskal–Wallis test for non-normally distributed continuous variables. Analyses were conducted using SPSS 29.0 (descriptive statistics), Stata/MP 18.0 (regression modeling), and R 4.4.2 (RCS analysis), with statistical significance set at two-tailed p < 0.05.
The baseline sociodemographic characteristics of all participants are summarized in Table 1. During the nearly 20-year period under consideration, the prevalence of cancer in the US population was 10.16% (4,342/42,732), with 9.85% (4,207/42,732) for solid cancers and 0.31% (135/42,732) for blood cancers. Compared with participants without cancer, those with cancer were more likely to be older (p < 0.001), to have lower energy intake (p < 0.001), to be female (p < 0.001), to be non-Hispanic White (p < 0.001), to live in the United States (p < 0.001), were more likely to be married or living with a partner (p = 0.012), had a higher proportion of PIR >3 (p < 0.001) and insurance (p < 0.001), were former smokers, and had moderate physical activity or sedentary habits (p < 0.001). These trends were almost identical for solid and blood cancers.
Table 1. Characteristics of participants stratified by any “Cancer Diagnosis,” “Solid Cancer Only” and “Blood Cancer Only.”
As illustrated in Table 2, a clear distinction emerges in the dietary nutrient intake patterns between cancer and non-cancer participants. Compared to non-cancer participants, patients with cancer consumed lower amounts of protein (p < 0.001), carbohydrates (p < 0.001), total sugars (p < 0.001), total fat (p < 0.001), SFA (p = 0.001), MUFA (p < 0.001), PUFA (p < 0.001), cholesterol (p = 0.001), lycopene (p < 0.001), vitamin B1 (p < 0.001), niacin (p < 0.001), vitamin B6 (p < 0.001), calcium (p < 0.001), phosphorus (p < 0.001), magnesium (p = 0.036), zinc (p < 0.001), sodium (p < 0.001), selenium (p < 0.001), and moisture (p < 0.001), whereas higher intakes of retinol (p < 0.001), vitamin A (p < 0.001), α-carotene (p < 0.001), β-carotene (p < 0.001), β-cryptoxanthin (p < 0.001),
Cross-sectional associations between 38 dietary nutrient intakes and cancer are presented in Table 3 and Supplementary Table S1. Following adjustment for all potential confounding variables, significant positive associations were observed for intakes of SFA [OR, 95% CI; 1.1082(1.0110, 1.2146), p = 0.028], β-carotene [1.0431(1.0096, 1.0777), p = 0.011], and vitamin K [1.0370(1.0094, 1.0654), p = 0.008] with the presence of cancer, whereas phosphorus intake [0.9016(0.8218, 0.9892), p = 0.029] was negatively associated with cancer presence. In addition, data analysis showed that intakes of SFA [1.1099(1.0113, 1.2180), p = 0.029], α-carotene [1.0353(1.0033, 1.0683), p = 0.030], β-carotene [1.0484(1.0146, 1.0833), p = 0.005], and vitamin K [1.0405(1.0098, 1.0722), p = 0.009] were all positively associated with the presence of solid cancers. For blood cancer, retinol intake [1.0935(1.0222, 1.1698), p = 0.009] demonstrated a positive association with the presence of blood cancer. These associations between specific nutrient intakes and the presence of cancer were also confirmed in the non-standardized data (Supplementary Table S1). There were no statistically significant associations between intake of other dietary nutrients and the presence of cancers, solid tumors and blood cancers (p > 0.05).
Table 3. Odds ratios (ORs) and 95% CIs of standardized dietary nutrient intakes associated with the presence of cancer, solid cancer, and blood cancer.
To further investigate the relationship between specific nutrient intake and cancer presence, curve fitting was performed after adjustment for all possible confounding factors. Figure 2 shows the trends of cancer, solid cancer and blood cancer presences with the intake of specific candidate nutrients according to the results of the correlation analyses. The intakes of SFA (cancer: p overall <0.0001, p for non-linear = 0.3895; solid cancer: p overall <0.0001, p for non-linear = 0.2235), β-carotene (p overall <0.0001, p for non-linear = 0.1009; p overall <0.0001, p for non-linear = 0.2730), and vitamin K (p overall <0.0001, p for non-linear = 0.8518; p overall <0.0001, p for non-linear = 0.7397) were overall positively associated with the presence of cancer and solid cancer, and no non-linear association was observed. Phosphorus intake (p overall <0.0001, p for non-linear = 0.1099) is negatively associated with cancer presence. Additionally, α-carotene intake (p overall <0.0001, p for non-linear = 0.6883) was positively associated with the presence of solid cancers, and retinol intake (p overall = 0.0003, p for non-linear = 0.6687) was also positively linked with the presence of blood cancers.
Figure 2. Dose–response curves between intake of candidate nutrients and the presence of cancer, solid cancer, and blood cancer. (a–i) The association between specific nutrient intake and cancer presence, subsequent to adjustment for all covariates. OR, odd ratio; 95% CI, confidence interval; SFA, saturated fatty acid.
This study aimed to systematically evaluate associations between 38 dietary nutrients and cancer presence, with particular focus on differential effects in solid tumors versus blood cancers. By analyzing comprehensive NHANES data, we sought to overcome limitations of prior single-nutrient or single-cancer-type studies, thereby identifying potential pan-cancer dietary risk modifiers. Our study provides novel evidence linking six dietary components—saturated fatty acids (SFA), retinol, α-carotene, β-carotene, vitamin K, and phosphorus—to cancer presence when analyzed through a comprehensive nutrient intake framework. Dose–response analyses revealed positive associations between elevated SFA, retinol, carotenoids, and vitamin K with cancer presence, while adequate phosphorus intake exerted protective associations. To our knowledge, this represents the first population-level investigation evaluating integrated dietary patterns across both solid and blood cancers.
Existing literature primarily examines isolated nutrients. Recent cohort data associate high SFA intake with colorectal cancer (54), corroborated by meta-analyses linking SFA to breast, prostate, and colorectal malignancies (20)—aligning with our observed SFA-cancer associations. Intriguingly, while meta-analyses suggest β-carotene inversely correlates with a reduced presence of breast cancer (55), null associations emerge for gastric cancer (56). Our findings contrast by demonstrating positive α/β-carotene-cancer links, paralleling a Singaporean case–control study showing elevated serum carotenoids with prostate cancer (57). These discrepancies likely stem from: (1) Cancer type specificity: Site-specific vs. pan-cancer analyses; (2) Exposure assessment: Serum biomarkers vs. dietary intake quantification; (3) Study design limitations in prior single-nutrient approaches. Resolving these contradictions requires large-scale RCTs integrating multi-omic biomarkers with longitudinal dietary monitoring.
Our analysis further identifies vitamin K intake as positively associated with both overall and solid cancer presence—a finding that contrasts with observational studies suggesting anti-cancer benefits of vitamin K supplementation (32, 58–61). Notably, a U.S. cohort study may align with our findings, reporting increased breast cancer incidence and mortality with higher vitamin K intake (62). This paradox may stem from: (1) source differentiation: Supplemental vs. dietary vitamin K forms (phylloquinone vs. menaquinones); (2) Cancer stage specificity: chemoprotective effects in early carcinogenesis vs. pro-tumor impacts in established malignancies. Regarding phosphorus, we observed protective associations at moderate intake levels, consistent with Zhu et al.’s (43) gynecological cancer findings. Preclinical studies further support phosphorus derivatives as promising anticancer nanocarriers (63), though epidemiological evidence remains conflicting (64, 65). The inverse association between phosphorus and cancer mirrors preclinical evidence of phosphate restriction slowing tumor growth (39, 66), suggesting a therapeutic avenue for dietary modulation. Mechanistic studies should clarify whether phosphorus exerts direct antineoplastic effects or serves as a biomarker for calcium-phosphate homeostasis.
The complex interplay between dietary nutrients and cancer likely operates through multiple synergistic biological pathways. Antioxidant nutrients such as vitamin C, vitamin E, and β-carotene exert protective effects by neutralizing reactive oxygen species (ROS) and reducing oxidative DNA damage—a hallmark of carcinogenesis (67–69). Selenium complements this defense by enhancing glutathione peroxidase activity, mitigating lipid peroxidation and genomic instability (70). However, pro-inflammatory dietary components like saturated fats may counteract these benefits by activating NF-κB signaling, which upregulates angiogenic cytokines (e.g., IL-6, TNF-α) to fuel tumor progression (71). Beyond direct oxidative mechanisms, nutrients modulate epigenetic landscapes: folate regulates DNA methylation patterns critical for tumor suppressor gene expression (72), while vitamin D induces cell cycle arrest through vitamin D receptor (VDR) activation (73). Dietary fibers further contribute to cancer prevention via gut microbiota-derived metabolites like butyrate, which selectively induce apoptosis in precancerous colonic cells (74, 75).
At the metabolic level, high saturated fat intake has been shown to epigenetically reprogram oncogenic pathways and activate pathological lipid metabolism in preclinical models (54). Vitamin K’s dual roles—modulating oxidative stress and regulating apoptosis through steroid/xenobiotic receptors—may explain its context-dependent associations with cancer outcomes (33, 76). Phosphorus’s potential anticancer effects, possibly mediated through redox balance restoration in the tumor microenvironment, remain mechanistically elusive but clinically suggestive (63). The paradoxical associations of carotenoids (α/β-carotene) with cancer could stem from their biphasic effects on inflammatory signaling, warranting single-cell resolution studies to delineate tissue-specific impacts (77–79). These mechanisms converge on cancer’s metabolic vulnerabilities. Emerging evidence suggests that strategic nutritional modulation—such as retinol’s regulation of ferroptosis (80) or phosphorus-mediated redox modulation—could enhance conventional chemotherapy by disrupting tumor metabolic dependencies (81, 82). Nevertheless, definitive causal attribution requires innovative models, particularly patient-derived organoids, to isolate nutrient effects from confounding lifestyle variables.
Furthermore, it is critical to recognize that cancer and its treatments may reciprocally alter nutrient intake patterns. Chemotherapy and radiation commonly induce anorexia, taste alterations (e.g., dysgeusia), and gastrointestinal toxicities (e.g., mucositis), significantly reducing dietary diversity and calorie consumption (83, 84). For instance, majority of patients report chemotherapy-induced taste changes that persist beyond treatment, preferentially reducing protein and vegetable intake (85, 86). Additionally, malignancies like pancreatic or gastrointestinal cancers directly impair nutrient absorption through mechanical obstruction or metabolic dysfunction (87, 88). These treatment- and disease-driven nutritional deficits may partly explain the lower macronutrient intake observed in cancer patients (Table 2). Recent clinical guidelines emphasize proactive nutritional support to mitigate these effects, highlighting the need for longitudinal studies disentangling causative dietary influences from treatment sequelae.
While this study identifies significant associations between nutrient profiles and cancer presence, several limitations warrant cautious interpretation. A primary constraint stems from the cross-sectional design, where dietary data collection occurred post-diagnosis. Cancer therapies—including chemotherapy and radiation—often reduce appetite and alter taste perception, likely contributing to observed nutritional disparities (e.g., lower macronutrient intake in cancer patients) rather than reflecting pre-disease dietary habits. Furthermore, self-reported cancer histories may introduce recall bias, and while NHANES protocols ensure methodological rigor, two 24-h dietary recalls might inadequately represent long-term consumption patterns. Critically, the temporal ambiguity inherent to observational designs prevents distinguishing whether dietary patterns influence cancer development or result from disease progression. Unaccounted interactions between nutrients and therapies further complicate causal attribution.
Despite these limitations, three key strengths bolster the findings’ validity: (1) Multivariable adjustments minimized confounding by sociodemographic, lifestyle, and energy intake variables; (2) restricted cubic spline analyses revealed non-linear dose–response relationships between specific nutrients (e.g., vitamin K, β-carotene) and cancer presence; (3) the nationally representative NHANES cohort (N = 42,732) offers robust statistical power and relevance to contemporary U.S. dietary practices. Future research should prioritize longitudinal designs with pre-diagnosis dietary assessments and clinical trials targeting nutrients showing threshold effects (e.g., vitamin K reduction trials), which could clarify causality and therapeutic applications. By evaluating 38 nutrients across cancer types, this study advances beyond reductionist approaches to reveal context-dependent dietary associations. The distinct associations for solid vs. blood cancers (e.g., retinol’s hematologic specificity) underscore the need for precision nutrition strategies tailored to cancer biology.
Our findings reveal significant nutrient-cancer associations, though the causal direction remains unclear due to potential treatment-induced dietary changes. While these patterns highlight promising targets for nutritional interventions, their clinical translation requires rigorous validation through multi-center longitudinal studies tracking pre-diagnosis diets across diverse populations. Priority should be given to randomized trials testing therapeutic modulation of threshold-effect nutrients (e.g., vitamin K reduction) before integrating such strategies into adjuvant therapies. This evidence hierarchy will determine whether observed associations reflect modifiable risk factors or secondary disease manifestations.
The datasets presented in this study can be found in online repositories. The names of the repository/repositories and accession number(s) can be found: NHANES repository (https://www.cdc.gov/nchs/nhanes/index.htm).
YQ: Software, Writing – original draft. LC: Visualization, Writing – original draft. ZZ: Data curation, Formal analysis, Writing – original draft. YL: Formal analysis, Writing – original draft. XT: Resources, Writing – original draft. MF: Funding acquisition, Methodology, Writing – original draft. JT: Funding acquisition, Investigation, Supervision, Writing – review & editing. KJ: Conceptualization, Funding acquisition, Project administration, Supervision, Writing – review & editing.
The author(s) declare that financial support was received for the research and/or publication of this article. KJ was supported by the Beijing Medical Award Foundation (Grant number: YXJL-2024-0299-0063). JT was supported by the Wu Jieping Medical Foundation (Grant number: 320.6750.2020-02-25) and Wuhan Knowledge Innovation Foundation (Grant number: 2024040801020361). MF was supported by the National Natural Science Foundation of China (Grant number: 32270992).
We extend our gratitude to the Public Instrument Platform of the College of Life Science and Technology at Huazhong Agricultural University.
The authors declare that the research was conducted in the absence of any commercial or financial relationships that could be construed as a potential conflict of interest.
The authors declare that no Gen AI was used in the creation of this manuscript.
All claims expressed in this article are solely those of the authors and do not necessarily represent those of their affiliated organizations, or those of the publisher, the editors and the reviewers. Any product that may be evaluated in this article, or claim that may be made by its manufacturer, is not guaranteed or endorsed by the publisher.
The Supplementary material for this article can be found online at: https://www.frontiersin.org/articles/10.3389/fnut.2025.1551822/full#supplementary-material
1. Kiri, S, and Ryba, T. Cancer, metastasis, and the epigenome. Mol Cancer. (2024) 23:154. doi: 10.1186/s12943-024-02069-w
2. Bray, F, Laversanne, M, Sung, H, Ferlay, J, Siegel, RL, Soerjomataram, I, et al. GLOBOCAN estimates of incidence and mortality worldwide for 36 cancers in 185 countries. CA Cancer J Clin. (2022) 74:229–63. doi: 10.3322/caac.21834
3. Statistics at a glance: the burden of Cancer in the United States National Cancer Institute (2024).
5. Xia, Y, Sun, M, Huang, H, and Jin, WL. Drug repurposing for cancer therapy. Signal Transduct Target Ther. (2024) 9:92. doi: 10.1038/s41392-024-01808-1
6. de Visser, KE, and Joyce, JA. The evolving tumor microenvironment: from cancer initiation to metastatic outgrowth. Cancer Cell. (2023) 41:374–403. doi: 10.1016/j.ccell.2023.02.016
7. Xiao, YL, Gong, Y, Qi, YJ, Shao, ZM, and Jiang, YZ. Effects of dietary intervention on human diseases: molecular mechanisms and therapeutic potential. Signal Transduct Target Ther. (2024) 9:59. doi: 10.1038/s41392-024-01771-x
8. Altea-Manzano, P, Cuadros, AM, Broadfield, LA, and Fendt, SM. Nutrient metabolism and cancer in the in vivo context: a metabolic game of give and take. EMBO Rep. (2020) 21:e50635. doi: 10.15252/embr.202050635
9. Mishra, A, Giuliani, G, and Longo, VD. Nutrition and dietary restrictions in cancer prevention. Biochim Biophys Acta Rev Cancer. (2024) 1879:189063. doi: 10.1016/j.bbcan.2023.189063
10. Papalazarou, V, and Maddocks, ODK. Supply and demand: cellular nutrient uptake and exchange in cancer. Mol Cell. (2021) 81:3731–48. doi: 10.1016/j.molcel.2021.08.026
11. Vernieri, C, Ligorio, F, Tripathy, D, and Longo, VD. Cyclic fasting-mimicking diet in cancer treatment: preclinical and clinical evidence. Cell Metab. (2024) 36:1644–67. doi: 10.1016/j.cmet.2024.06.014
12. Wang, X, Li, Y, Jia, F, Cui, X, Pan, Z, and Wu, Y. Boosting nutrient starvation-dominated cancer therapy through curcumin-augmented mitochondrial ca(2+) overload and obatoclax-mediated autophagy inhibition as supported by a novel nano-modulator GO-Alg@CaP/CO. J Nanobiotechnol. (2022) 20:225. doi: 10.1186/s12951-022-01439-0
13. Palm, W. Metabolic plasticity allows cancer cells to thrive under nutrient starvation. Proc Natl Acad Sci USA. (2021) 118:7118. doi: 10.1073/pnas.2102057118
14. Zhang, Y, Lin, C, Liu, Z, Sun, Y, Chen, M, Guo, Y, et al. Cancer cells co-opt nociceptive nerves to thrive in nutrient-poor environments and upon nutrient-starvation therapies. Cell Metab. (2022) 34:1999–2017.e10. doi: 10.1016/j.cmet.2022.10.012
15. Hanahan, D, and Weinberg, RA. Hallmarks of cancer: the next generation. Cell. (2011) 144:646–74. doi: 10.1016/j.cell.2011.02.013
16. Tabe, Y, Konopleva, M, and Andreeff, M. Fatty acid metabolism, bone marrow adipocytes, and AML. Front Oncol. (2020) 10:155. doi: 10.3389/fonc.2020.00155
17. Qin, H, Peng, M, Cheng, J, Wang, Z, Cui, Y, Huang, Y, et al. A novel LGALS1-depended and immune-associated fatty acid metabolism risk model in acute myeloid leukemia stem cells. Cell Death Dis. (2024) 15:482. doi: 10.1038/s41419-024-06865-6
18. Ying, M, You, D, Zhu, X, Cai, L, Zeng, S, and Hu, X. Lactate and glutamine support NADPH generation in cancer cells under glucose deprived conditions. Redox Biol. (2021) 46:102065. doi: 10.1016/j.redox.2021.102065
19. Rattigan, KM, Brabcova, Z, Sarnello, D, Zarou, MM, Roy, K, Kwan, R, et al. Pyruvate anaplerosis is a targetable vulnerability in persistent leukaemic stem cells. Nat Commun. (2023) 14:4634. doi: 10.1038/s41467-023-40222-z
20. Mei, J, Qian, M, Hou, Y, Liang, M, Chen, Y, Wang, C, et al. Association of saturated fatty acids with cancer risk: a systematic review and meta-analysis. Lipids Health Dis. (2024) 23:32. doi: 10.1186/s12944-024-02025-z
21. Stevens, BM, Jones, CL, Pollyea, DA, Culp-Hill, R, D’Alessandro, A, Winters, A, et al. Fatty acid metabolism underlies venetoclax resistance in acute myeloid leukemia stem cells. Nat Cancer. (2020) 1:1176–87. doi: 10.1038/s43018-020-00126-z
22. Islami, F, Marlow, EC, Thomson, B, McCullough, ML, Rumgay, H, Gapstur, SM, et al. Proportion and number of cancer cases and deaths attributable to potentially modifiable risk factors in the United States. CA Cancer J Clin. (2019) 74:405–32. doi: 10.3322/caac.21858
23. Arayici, ME, Basbinar, Y, and Ellidokuz, H. High and low dietary fiber consumption and cancer risk: a comprehensive umbrella review with meta-meta-analysis involving meta-analyses of observational epidemiological studies. Crit Rev Food Sci Nutr. (2025) 65:1617–1630. doi: 10.1080/10408398.2023.2298772
24. Lu, Y, Li, D, Wang, L, Zhang, H, Jiang, F, Zhang, R, et al. Comprehensive investigation on associations between dietary intake and blood levels of fatty acids and colorectal Cancer risk. Nutrients. (2023) 15:730. doi: 10.3390/nu15030730
25. Liu, Z, Huang, H, Xie, J, Xu, Y, and Xu, C. Circulating fatty acids and risk of hepatocellular carcinoma and chronic liver disease mortality in the UK biobank. Nat Commun. (2024) 15:3707. doi: 10.1038/s41467-024-47960-8
26. Ghamarzad Shishavan, N, Masoudi, S, Mohamadkhani, A, Sepanlou, SG, Sharafkhah, M, Poustchi, H, et al. Dietary intake of fatty acids and risk of pancreatic cancer: Golestan cohort study. Nutr J. (2021) 20:69. doi: 10.1186/s12937-021-00723-3
27. Zhang, Y, Yang, J, Na, X, and Zhao, A. Association between β-carotene supplementation and risk of cancer: a meta-analysis of randomized controlled trials. Nutr Rev. (2023) 81:1118–30. doi: 10.1093/nutrit/nuac110
28. Bates, CA, Vincent, MJ, Buerger, AN, Santamaria, AB, Maier, A, and Jack, M. Investigating the relationship between β-carotene intake from diet and supplements, smoking, and lung cancer risk. Food Chem Toxicol. (2024) 194:115104. doi: 10.1016/j.fct.2024.115104
29. Boot, IWA, Wesselius, A, Yu, EYW, White, E, Brustad, M, Marques, C, et al. Dietary vitamin D intake and the bladder cancer risk: a pooled analysis of prospective cohort studies. Clin Nutr. (2023) 42:1462–74. doi: 10.1016/j.clnu.2023.05.010
30. Zhao, S, Qian, F, Wan, Z, Chen, X, Pan, A, and Liu, G. Vitamin D and major chronic diseases. Trends Endocrinol Metab. (2024) 35:1050–61. doi: 10.1016/j.tem.2024.04.018
31. Venturelli, S, Leischner, C, Helling, T, Burkard, M, and Marongiu, L. Vitamins as possible Cancer biomarkers: significance and limitations. Nutrients. (2021) 13:3914. doi: 10.3390/nu13113914
32. Chen, A, Li, J, Shen, N, Huang, H, and Hang, Q. Vitamin K: new insights related to senescence and cancer metastasis. Biochim Biophys Acta Rev Cancer. (2024) 1879:189057. doi: 10.1016/j.bbcan.2023.189057
33. Welsh, J, Bak, MJ, and Narvaez, CJ. New insights into vitamin K biology with relevance to cancer. Trends Mol Med. (2022) 28:864–81. doi: 10.1016/j.molmed.2022.07.002
34. Saimaiti, A, Zhou, DD, Li, J, Xiong, RG, Gan, RY, Huang, SY, et al. Dietary sources, health benefits, and risks of caffeine. Crit Rev Food Sci Nutr. (2023) 63:9648–66. doi: 10.1080/10408398.2022.2074362
35. Stouth, DW, Lebeau, PF, and Austin, RC. Repurposing two old friends to fight Cancer: caffeine and statins. Cancer Res. (2023) 83:2091–2. doi: 10.1158/0008-5472.CAN-23-1066
36. Tu, K, Liu, K, Wang, Y, Jiang, Y, and Zhang, C. Association of dietary intake of zinc and selenium with breast cancer risk: a case-control study in Chinese women. Nutrients. (2023) 15:3253. doi: 10.3390/nu15143253
37. Carlisle, AE, Lee, N, Matthew-Onabanjo, AN, Spears, ME, Park, SJ, Youkana, D, et al. Selenium detoxification is required for cancer-cell survival. Nat Metab. (2020) 2:603–11. doi: 10.1038/s42255-020-0224-7
38. Yang, J, Qian, S, Na, X, and Zhao, A. Association between dietary and supplemental antioxidants intake and lung Cancer risk: evidence from a Cancer screening trial. Antioxidants. (2023) 12:338. doi: 10.3390/antiox12020338
39. Hoyt, M, Song, Y, Gao, S, O’Palka, J, and Zhang, J. Intake of calcium, magnesium, and phosphorus and risk of pancreatic Cancer in the prostate, lung, colorectal, and ovarian Cancer screening trial. J Am Nutr Assoc. (2022) 41:747–57. doi: 10.1080/07315724.2021.1970047
40. Martínez-Garay, C, and Djouder, N. Dietary interventions and precision nutrition in cancer therapy. Trends Mol Med. (2023) 29:489–511. doi: 10.1016/j.molmed.2023.04.004
41. Papadimitriou, N, Markozannes, G, Kanellopoulou, A, Critselis, E, Alhardan, S, Karafousia, V, et al. An umbrella review of the evidence associating diet and cancer risk at 11 anatomical sites. Nat Commun. (2021) 12:4579. doi: 10.1038/s41467-021-24861-8
42. Papavassiliou, KA, Sofianidi, AA, and Papavassiliou, AG. Anthocyanins in non-small cell lung Cancer (NSCLC) treatment and prevention. Nutrients. (2024) 16:1458. doi: 10.3390/nu16101458
43. Zhu, G, Li, Z, Tang, L, Shen, M, Zhou, Z, Wei, Y, et al. Associations of dietary intakes with gynecological cancers: findings from a Cross-sectional study. Nutrients. (2022) 14:5026. doi: 10.3390/nu14235026
44. Wang, X, Glubb, DM, and O’Mara, TA. Dietary factors and endometrial Cancer risk: a Mendelian randomization study. Nutrients. (2023) 15:603. doi: 10.3390/nu15030603
45. Nazari, E, Hasanzadeh, M, Rezvani, R, Rejali, M, Badpeyma, M, Delaram, Z, et al. Association of dietary intake and cervical cancer: a prevention strategy. Infect Agent Cancer. (2023) 18:42. doi: 10.1186/s13027-023-00517-8
46. Ji, K, Sun, M, Li, L, Hong, Y, Yang, S, and Wu, Y. Association between vitamin B2 intake and cognitive performance among older adults: a cross-sectional study from NHANES. Sci Rep. (2024) 14:21930. doi: 10.1038/s41598-024-72949-0
47. von Elm, E, Altman, DG, Egger, M, Pocock, SJ, Gøtzsche, PC, and Vandenbroucke, JP. The strengthening the reporting of observational studies in epidemiology (STROBE) statement: guidelines for reporting observational studies. Lancet. (2007) 370:1453–7. doi: 10.1016/S0140-6736(07)61602-X
48. Ji, K, Sun, M, Hong, Y, Li, L, Wang, X, Li, C, et al. Association of vitamin B1 intake with geriatric cognitive function: an analysis of the National Health and nutrition examination survey (NHANES) from 2011 to 2014. Heliyon. (2024) 10:e28119. doi: 10.1016/j.heliyon.2024.e28119
49. Kase, BE, Liese, AD, Zhang, J, Murphy, EA, Zhao, L, and Steck, SE. The development and evaluation of a literature-based dietary index for gut microbiota. Nutrients. (2024) 16:1045. doi: 10.3390/nu16071045
50. Ahluwalia, N, Dwyer, J, Terry, A, Moshfegh, A, and Johnson, C. Update on NHANES dietary data: focus on collection, release, analytical considerations, and uses to inform public policy. Adv Nutr. (2016) 7:121–34. doi: 10.3945/an.115.009258
51. Alaggio, R, Amador, C, Anagnostopoulos, I, Attygalle, AD, Araujo, IBDO, Berti, E, et al. The 5th edition of the World Health Organization classification of haematolymphoid tumours: lymphoid neoplasms. Leukemia. (2022) 36:1720–48. doi: 10.1038/s41375-022-01620-2
52. Dickie, L, Johnson, CH, Adams, S, and Negoita, S. Solid tumor rules. Rockville, MD: National Cancer Institute (2024).
53. Shete, AS, Gavhane, YN, Bhagat, AK, Shinde, VR, Bhong, KK, Khairnar, GA, et al. Solid tumors: facts, challenges and solutions. Int J Pharma Sci Res. (2011) 2:1–12.
54. Fan, L, Cai, Y, Wang, H, Zhang, H, Chen, C, Zhang, M, et al. Saturated fatty acid intake, genetic risk and colorectal cancer incidence: a large-scale prospective cohort study. Int J Cancer. (2023) 153:499–511. doi: 10.1002/ijc.34544
55. Dehnavi, MK, Ebrahimpour-Koujan, S, Lotfi, K, and Azadbakht, L. The association between circulating carotenoids and risk of breast Cancer: a systematic review and dose-response Meta-analysis of prospective studies. Adv Nutr. (2024) 15:100135. doi: 10.1016/j.advnut.2023.10.007
56. Deng, D, Zhang, Y, Zhang, R, Yi, J, Dong, J, Sha, L, et al. Circulating proteins and metabolite biomarkers in gastric Cancer: a systematic review and Meta-analysis. Arch Med Res. (2023) 54:124–34. doi: 10.1016/j.arcmed.2022.12.012
57. Loh, WQ, Yin, X, Kishida, R, Chia, SE, Ong, CN, and Seow, WJ. Association between vitamin a and E forms and prostate Cancer risk in the Singapore prostate Cancer study. Nutrients. (2023) 15:2677. doi: 10.3390/nu15122677
58. Yan, F, Eshak, ES, Arafa, A, Tamakoshi, A, and Iso, H. Vitamin K intake and risk of lung Cancer: the Japan collaborative cohort study. J Epidemiol. (2023) 33:536–42. doi: 10.2188/jea.JE20220063
59. Palmer, CR, Bellinge, JW, Dalgaard, F, Sim, M, Murray, K, Connolly, E, et al. Association between vitamin K(1) intake and mortality in the Danish diet, Cancer, and health cohort. Eur J Epidemiol. (2021) 36:1005–14. doi: 10.1007/s10654-021-00806-9
60. Yu, DW, Li, QJ, Cheng, L, Yang, PF, Sun, WP, Peng, Y, et al. Dietary vitamin K intake and the risk of pancreatic Cancer: a prospective study of 101,695 American adults. Am J Epidemiol. (2021) 190:2029–41. doi: 10.1093/aje/kwab131
61. Popa, DS, Bigman, G, and Rusu, ME. The role of vitamin K in humans: implication in aging and age-associated diseases. Antioxidants. (2021) 10:566. doi: 10.3390/antiox10040566
62. Wang, K, Wu, Q, Li, Z, Reger, MK, Xiong, Y, Zhong, G, et al. Vitamin K intake and breast cancer incidence and death: results from a prospective cohort study. Clin Nutr. (2021) 40:3370–8. doi: 10.1016/j.clnu.2020.11.009
63. Wolfram, A, Fuentes-Soriano, P, Herold-Mende, C, and Romero-Nieto, C. Boron- and phosphorus-containing molecular/nano platforms: exploiting pathological redox imbalance to fight cancer. Nanoscale. (2022) 14:17500–13. doi: 10.1039/D2NR03126D
64. Brown, RB, Bigelow, P, Dubin, JA, and Mielke, JG. High dietary phosphorus is associated with increased breast Cancer risk in a U.S. cohort of middle-aged women. Nutrients. (2023) 15:3735. doi: 10.3390/nu15173735
65. Lv, L, Ye, D, Chen, J, Qian, Y, Fu, AN, Song, J, et al. Circulating phosphorus concentration and risk of prostate cancer: a Mendelian randomization study. Am J Clin Nutr. (2022) 115:534–43. doi: 10.1093/ajcn/nqab342
66. Wilson, KM, Shui, IM, Mucci, LA, and Giovannucci, E. Calcium and phosphorus intake and prostate cancer risk: a 24-y follow-up study. Am J Clin Nutr. (2015) 101:173–83. doi: 10.3945/ajcn.114.088716
67. Valko, M, Rhodes, CJ, Moncol, J, Izakovic, M, and Mazur, M. Free radicals, metals and antioxidants in oxidative stress-induced cancer. Chem Biol Interact. (2006) 160:1–40. doi: 10.1016/j.cbi.2005.12.009
68. Jomova, K, Alomar, SY, Alwasel, SH, Nepovimova, E, Kuca, K, and Valko, M. Several lines of antioxidant defense against oxidative stress: antioxidant enzymes, nanomaterials with multiple enzyme-mimicking activities, and low-molecular-weight antioxidants. Arch Toxicol. (2024) 98:1323–67. doi: 10.1007/s00204-024-03696-4
69. Doseděl, M, Jirkovský, E, Macáková, K, Krčmová, LK, Javorská, L, Pourová, J, et al. Vitamin C-sources, physiological role, kinetics, deficiency, use, toxicity, and determination. Nutrients. (2021) 13:615. doi: 10.3390/nu13020615
70. Marković, R, Ćirić, J, Starčević, M, Šefer, D, and Baltić, M. Effects of selenium source and level in diet on glutathione peroxidase activity, tissue selenium distribution, and growth performance in poultry. Anim Health Res Rev. (2018) 19:166–76. doi: 10.1017/S1466252318000105
71. Shivappa, N, Godos, J, Hébert, JR, Wirth, MD, Piuri, G, Speciani, AF, et al. Dietary inflammatory index and colorectal Cancer risk-a Meta-analysis. Nutrients. (2017) 9:1043. doi: 10.3390/nu9091043
72. Fardous, AM, and Heydari, AR. Uncovering the hidden dangers and molecular mechanisms of excess folate: a narrative review. Nutrients. (2023) 15:4699. doi: 10.3390/nu15214699
73. Welsh, J. Vitamin D and breast cancer: insights from animal models. Am J Clin Nutr. (2004) 80:1721s–4s. doi: 10.1093/ajcn/80.6.1721S
74. Mathers, JC. Dietary fibre and health: the story so far. Proc Nutr Soc. (2023) 82:120–9. doi: 10.1017/S0029665123002215
75. Kurtz, RC, and Zhang, ZF. Gastric cardia cancer and dietary fiber. Gastroenterology. (2001) 120:568–70. doi: 10.1053/gast.2001.22365
76. Hirschhorn, T, and Stockwell, BR. Vitamin K: a new guardian against ferroptosis. Mol Cell. (2022) 82:3760–2. doi: 10.1016/j.molcel.2022.10.001
77. Baeza-Morales, A, Medina-García, M, Martínez-Peinado, P, Pascual-García, S, Pujalte-Satorre, C, López-Jaén, AB, et al. The antitumour mechanisms of carotenoids: a comprehensive review. Antioxidants. (2024) 13:1060. doi: 10.3390/antiox13091060
78. Park, YM, Lilyquist, J, Van’t Erve, TJ, O’Brien, KM, Nichols, HB, Milne, GL, et al. Association of dietary and plasma carotenoids with urinary F(2)-isoprostanes. Eur J Nutr. (2022) 61:2711–23. doi: 10.1007/s00394-022-02837-8
79. Varghese, R, Efferth, T, and Ramamoorthy, S. Carotenoids for lung cancer chemoprevention and chemotherapy: promises and controversies. Phytomedicine. (2023) 116:154850. doi: 10.1016/j.phymed.2023.154850
80. Bi, G, Liang, J, Shan, G, Bian, Y, Chen, Z, Huang, Y, et al. Retinol Saturase mediates retinoid metabolism to impair a Ferroptosis defense system in Cancer cells. Cancer Res. (2023) 83:2387–404. doi: 10.1158/0008-5472.CAN-22-3977
81. Kanarek, N, Petrova, B, and Sabatini, DM. Dietary modifications for enhanced cancer therapy. Nature. (2020) 579:507–17. doi: 10.1038/s41586-020-2124-0
82. Tajan, M, and Vousden, KH. Dietary approaches to Cancer therapy. Cancer Cell. (2020) 37:767–85. doi: 10.1016/j.ccell.2020.04.005
83. He, Y, Zheng, J, Ye, B, Dai, Y, and Nie, K. Chemotherapy-induced gastrointestinal toxicity: pathogenesis and current management. Biochem Pharmacol. (2023) 216:115787. doi: 10.1016/j.bcp.2023.115787
84. Zhu, X, Potterfield, R, Gruber, KA, Zhang, E, Newton, SD, Norgard, MA, et al. Melanocortin-4 receptor antagonist TCMCB07 alleviates chemotherapy-induced anorexia and weight loss in rats. J Clin Invest. (2024) 135:1305. doi: 10.1172/JCI181305
85. Bischoff, SC, Bager, P, Escher, J, Forbes, A, Hébuterne, X, Hvas, CL, et al. ESPEN guideline on clinical nutrition in inflammatory bowel disease. Clin Nutr. (2023) 42:352–79. doi: 10.1016/j.clnu.2022.12.004
86. Arends, J, Bachmann, P, Baracos, V, Barthelemy, N, Bertz, H, Bozzetti, F, et al. ESPEN guidelines on nutrition in cancer patients. Clin Nutr. (2017) 36:11–48. doi: 10.1016/j.clnu.2016.07.015
87. Ryan, AM, Power, DG, Daly, L, Cushen, SJ, Ní Bhuachalla, Ē, and Prado, CM. Cancer-associated malnutrition, cachexia and sarcopenia: the skeleton in the hospital closet 40 years later. Proc Nutr Soc. (2016) 75:199–211. doi: 10.1017/S002966511500419X
Keywords: cancer, solid cancer, blood cancer, dietary nutrient intake, NHANES
Citation: Qin Y, Chen L, Zhao Z, Li Y, Tian X, Feng M, Tang J and Ji K (2025) Dietary nutrient intake and cancer presence: evidence from a cross-sectional study. Front. Nutr. 12:1551822. doi: 10.3389/fnut.2025.1551822
Received: 26 December 2024; Accepted: 20 March 2025;
Published: 01 April 2025.
Edited by:
Olesya A. Kharenko, Syantra Inc., CanadaReviewed by:
Jamie Sheard, Independent Researcher, Chattanooga, TN, United StatesCopyright © 2025 Qin, Chen, Zhao, Li, Tian, Feng, Tang and Ji. This is an open-access article distributed under the terms of the Creative Commons Attribution License (CC BY). The use, distribution or reproduction in other forums is permitted, provided the original author(s) and the copyright owner(s) are credited and that the original publication in this journal is cited, in accordance with accepted academic practice. No use, distribution or reproduction is permitted which does not comply with these terms.
*Correspondence: Jing Tang, dGFuZ2ppbmd0b3BAMTYzLmNvbQ==; Kangkang Ji, a3lyaWVAbWFpbC51c3RjLmVkdS5jbg==
Disclaimer: All claims expressed in this article are solely those of the authors and do not necessarily represent those of their affiliated organizations, or those of the publisher, the editors and the reviewers. Any product that may be evaluated in this article or claim that may be made by its manufacturer is not guaranteed or endorsed by the publisher.
Research integrity at Frontiers
Learn more about the work of our research integrity team to safeguard the quality of each article we publish.