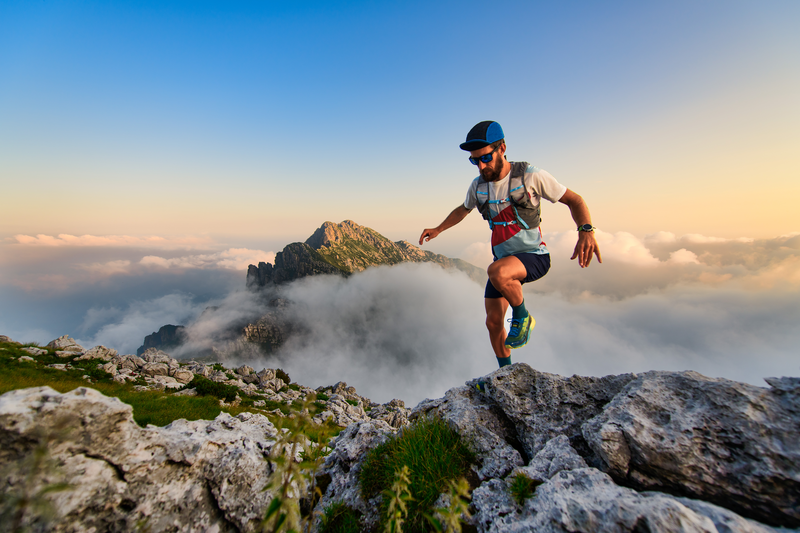
95% of researchers rate our articles as excellent or good
Learn more about the work of our research integrity team to safeguard the quality of each article we publish.
Find out more
ORIGINAL RESEARCH article
Front. Nutr. , 10 January 2025
Sec. Nutritional Epidemiology
Volume 11 - 2024 | https://doi.org/10.3389/fnut.2024.1521826
Background: Salt usage patterns have been associated with a risk of multiple diseases; however, their relationship with heavy metal exposure has not been extensively studied.
Methods: This study analyzed survey data from 11,574 NHANES participants. Weighted linear regression models were used to examine the relationship between the type of salt used by participants, the frequency of adding salt at the table, and the frequency of adding regular or seasoned salt to cooking or food preparation, and urinary concentrations of 10 heavy metals. Multiple sensitivity analyses were also performed.
Results: The weighted regression analysis indicated that participants’ salt usage patterns were associated with an increased urinary excretion of certain heavy metals. Specifically, regarding the type of salt used, compared to regular salt, the use of salt substitutes was significantly positively correlated with urinary molybdenum (Mo) levels, while not using salt or substitutes at the table was significantly positively correlated with urinary levels of both Mo and arsenic (As). In terms of the frequency of adding regular salt at the table, frequent addition compared to rarely adding salt was significantly positively correlated with urinary levels of cadmium (Cd), and antimony (Sb), while showing a significant negative correlation with urinary Mo levels. Additionally, when examining the frequency of using regular salt during cooking or food preparation, those who occasionally or very often added regular salt had significantly higher urinary levels of barium (Ba), cesium (Cs), and thallium (Tl) compared to those who never added regular salt during cooking. These associations remained stable in sensitivity analyses.
Conclusion: Our analysis revealed that participants’ salt usage patterns are associated with increased excretion of certain heavy metals, suggesting possible increased exposures to these metals. While these findings are concerning, they require validation in other populations and should be confirmed through prospective studies designed based on this hypothesis.
With industrialization’s progression, the impact of environmental pollutants on human health has become increasingly severe. It is reported that the global disease burden attributed to environmental factors accounts for approximately 8–9% of the total disease burden (1), with even higher proportions observed in developing countries (2). Heavy metals play a particularly significant role in this regard (3). These metals are widely present in the surrounding environment and primarily enter the human body through food, drinking water, inhalation, and skin contact (4). Consuming contaminated food and water is a major source of human heavy metal exposure. Long-term excretion of heavy metals is associated with an increased risk of various diseases, including cardiovascular disease (5), diabetes (6), respiratory diseases (7), dermatological disorders (8), and certain cancers (9). Even at low concentrations, prolonged excretion of heavy metals can adversely affect human health (4, 10). Studies show a significant positive association between excretion of heavy metals and their mixtures and all-cause mortality in U.S. populations (11). In 2019 alone, nearly 1 million deaths globally were attributed to lead (Pb) exposure, accounting for approximately 50% of deaths linked to known chemical exposures (12). This has led to widespread concern regarding heavy metal exposure.
Salt is a common ingredient in food preparation and seasoning; its usage patterns and quantities can significantly impact human health. Adding salt to food is directly linked to an individual’s preference for a salty diet and habitual salt intake (13). The frequency of adding salt can serve as an indicator of long-term preferences for salty flavors and overall sodium intake, which is directly associated with 24-h urinary sodium excretion (14).
Numerous studies have explored the relationship between salt consumption patterns and health outcomes. There is a strong and consistent association between salt intake, consumption of salty foods, and the incidence of gastric cancer and other precancerous conditions, possibly due to heavy metal contamination in salt (15, 16). Previous research has shown that, compared to those who never add salt at the table, individuals who always or frequently do so face an increased risk of gastric, lung, testicular, and bladder cancers. The consumption of processed meats, which generally have high sodium contents, is also significantly associated with an elevated risk of gastrointestinal cancers, urinary system cancers, and malignancies of the lung, prostate, testicles, and blood (17). Research indicates that high sodium intake is strongly associated with increased risks of cardiovascular diseases and related mortality (18). Further studies suggest that the frequency of salt addition to food is closely linked to cardiovascular disease risk, with lower frequencies of salt addition reducing the risk of heart failure and ischemic heart disease (IHD) (14). Moreover, higher frequencies of salt use are significantly associated with increased risks of multiple conditions, including psoriasis (19), chronic kidney disease (20), sleep apnea (21), and type 2 diabetes (22). One study found that individuals who frequently add extra salt to prepared meals have twice the risk of developing type 2 diabetes compared to those who never add salt (23). Furthermore, salt consumption habits have been causally linked to cognitive impairment (24).
In addition to the quantity and frequency of salt used, the type of salt may also influence health outcomes. Recent studies suggest that substituting regular salt with salt alternatives may lower blood pressure and reduce the incidence of stroke, major cardiovascular events, and all-cause mortality (25). However, salt may also pose additional health risks due to contamination with heavy metals (26–29). The cooking process may alter the metal content in food. For instance, Devesa et al. observed a significant increase in arsenic levels in salted cod after cooking (30). Similarly, other studies have reported elevated concentrations of heavy metals—including lead (Pb), cadmium (Cd), arsenic (As), and mercury (Hg)—in salted fish (31). Many researchers attribute the health risks associated with salt consumption primarily to high sodium intake, often overlooking the potential role of contaminant exposure.
Some studies suggest that contaminants in salt may mediate the relationship between salt use and adverse health outcomes (20). This is because some studies have found that the use of 24-h urinary sodium alone may not fully explain the association between the frequency of salt addition and certain health outcomes (32). This raises the question of whether salt usage patterns might influence health outcomes, in part, by altering heavy metal exposure in the body. Could heavy metal exposure act as an intermediary in the complex relationship between salt use and health outcomes? While the effects of salt usage patterns on cardiovascular and other diseases have been widely studied, the relationship between salt consumption patterns and heavy metal exposure remains largely unexplored.
To address these questions and fill this research gap, this study aims to explore the association between salt usage patterns and heavy metal exposure using data from the National Health and Nutrition Examination Survey (NHANES). Specifically, we will examine the relationships between the types of salt regularly used by participants, the frequency of adding salt at the table, and the frequency of adding regular or seasoned salt during cooking or food preparation, with the urinary concentrations of 10 heavy metals: barium (Ba), Cd, cobalt (Co), cesium (Cs), molybdenum (Mo), Pb, antimony (Sb), thallium (Tl), tungsten (W), and As. The findings will provide a deeper understanding of the role of salt usage patterns in certain health outcomes and offer valuable insights into how dietary habits may influence heavy metal exposure. Additionally, this research will provide a crucial reference for future studies investigating the complex interactions among diet, environmental pollutants, and human health.
The data for this study are sourced from NHANES, a cross-sectional survey conducted by the National Center for Health Statistics (NCHS) within the Centers for Disease Control and Prevention (CDC). NHANES is designed to assess the health and nutritional status of U.S. adults and children. Using a complex, multistage, probability sampling design, the survey provides a representative sample of the U.S. population across all age groups. To generate reliable statistical data, NHANES oversampled individuals aged 60 and older, as well as African Americans and Hispanics. The NHANES study protocol was approved by the NCHS Ethics Review Board, and all participants provided informed consent. The NHANES data are fully anonymized public data, collected and utilized in accordance with NHANES privacy protection policies and relevant laws and regulations. All data used in this study are derived from publicly available datasets and do not involve any personally identifiable information; therefore, no additional ethical review is required.
Since 2003, NHANES has conducted continuous surveys on salt usage patterns among the U.S. population. Therefore, this study selected participants aged 20 and older from eight consecutive NHANES cycles between 2003 and 2018. We filtered the data by first excluding participants under 20 years old (N = 35,522), then excluding those missing urinary metal data (N = 30,871), followed by excluding those lacking data on the type of salt added at the table and salt usage habits during cooking (N = 1,235). Finally, we excluded participants with missing covariate data (N = 1,110), resulting in a total of 11,574 participants included in the analysis. The detailed data filtering process is illustrated in Figure 1.
Participants were instructed to collect spot urine samples at the Mobile Examination Center (MEC), which were then processed, stored, and transported to the Division of Laboratory Sciences, National Center for Environmental Health, Centers for Disease Control and Prevention in Atlanta, GA, for analysis. All heavy metals were analyzed using inductively coupled plasma dynamic reaction cell mass spectrometry (ICP-DRC-MS). This study included 10 urinary heavy metal elements with detection rates exceeding 76.19%, namely Ba, Cd, Co, Cs, Mo, Pb, Sb, Tl, W, and As, with Sb having the lowest detection rate. For metals with concentrations below the limit of detection (LOD), NHANES replaced the values with LOD divided by the square root of 2. Detailed information on the detection rates of metal elements can be found in Supplementary Table 1. Urinary creatinine levels were also measured to account for urine dilution.
Since the 2003–2004 cycle, NHANES has continuously collected data on participants’ salt usage patterns through dietary interviews. During these interviews, participants are asked, “What type of salt do you usually add to your food at the table?” They can choose one answer from four options: 1. Ordinary salt including regular iodized salt, sea salt, and seasoning salts made with regular salt; 2. Lite salt; 3. Salt products or salt substitutes; 4. Does not use or add salt products at the table. So-called lite salt was recorded as such and has a reduced sodium content. Salt substitutes do not contain sodium (33). Low salt is usually a mixture containing NaCl and KCl (34), while salt substitutes usually contain KCl (35). If participants select one of the first three options, they are subsequently asked, “How often do you add ordinary salt to your food at the table?” They can choose one answer from three options: 1. Rarely; 2. Occasionally; 3. Very often. Following this, all participants are asked, “How often is ordinary salt or seasoned salt added in cooking or preparing foods in your household?” They select one answer from four options: 1. Never; 2. Rarely; 3. Occasionally; 4. Very often. Participants who refuse to answer, select “other,” or respond with “I do not know” are assigned a missing value.
In this study, several potential confounding factors were considered in the statistical analysis, including demographic characteristics such as age (continuous), gender (male and female), and race/ethnicity (Mexican American, Non-Hispanic White, Non-Hispanic Black, Other Hispanic, and Other groups). Socioeconomic status (SES) indicators included educational attainment (Less than 9th Grade, 9th-11th Grade, High School Graduate/GED or Equivalent, Some College or AA Degree, and College Graduate or Above) and the poverty-to-income ratio (PIR) (≤1, 1–4, and ≥ 4). Additionally, body mass index (BMI) was categorized as underweight (<18.5), normal (18.5 to <25), overweight (25 to <30), and obese (30 or greater), along with smoking status (categorized as never smoker, former smoker, and current smoker).
Continuous variables are presented as medians (IQR), while categorical data are expressed as percentages. To minimize the impact of urine dilution, urinary metal concentrations were adjusted using their ratios to urinary creatinine, units in μg/mg creatinine. Since all metal elements exhibited significant skewness, their natural logarithms were used in regression analyses to enhance normality. Taking into account the design of NHANES and following the analysis guidelines published by the National Center for Health Statistics, the two-year metal subsample weights were incorporated in the analysis to ensure that the results are nationally representative.
The primary analysis employed a weighted multivariable linear regression model to examine the association between heavy metal levels and salt usage patterns. The models were adjusted for age, gender, race/ethnicity, educational attainment, PIR, BMI, and smoking status. Results are presented as regression coefficients (β) along with their 95% confidence intervals.
Firstly, as the consumption of fish and shellfish is a significant source of human exposure to certain heavy metals, such as As and Cd (36–38), we further adjusted for participants’ fish and shellfish consumption over the past 30 days in the sensitivity analysis.
Secondly, given that smoking is a significant source of heavy metals in the body (4, 39), we further excluded current smokers and repeated the analysis on the remaining population, adjusting for fish and shellfish intake.
All analyses were conducted using R version 4.4.1, with weighted regression analysis performed using the “survey” package. Our study aims to provide preliminary exploratory insights into the relationship between salt use patterns and heavy metal exposure and to propose potential directions for future prospective research. To maintain sensitivity to the results, no multiple corrections were applied to p-values, and a two-sided p-value of <0.05 was considered statistically significant (40–43).
As shown in the baseline characteristics in Table 1, the median age of participants was 46.0 years (IQR: 33.0, 59.0), with females comprising 52% of the sample. The majority racial/ethnic group was Non-Hispanic White (69%). Most participants had higher educational attainment (Some College or AA degree: 32%) and a high PIR (> 1: 86%), 54% reported never smoking, and 37% were classified as obese.
As shown in Table 1, participants categorized by the type of salt typically added to food exhibit the following age distributions: those using Lite salt and Salt substitute have median ages of 53.0 (33.0, 66.0) and 56.0 (38.0, 67.0), respectively, while participants using Ordinary salt and those who reported not using salt at the table have median ages of 45.0 (32.0, 57.0) and 49.0 (35.0, 62.0), respectively. The proportion of females was significantly higher than that of males across all four types of salt usage (p = 0.027). Non-Hispanic Black participants were more likely to use lite salt (15%), salt substitutes (15%), or to avoid adding salt at the table (14%), while Non-Hispanic White participants more commonly used ordinary salt (71%) and salt substitutes (65%). Obese participants were more likely to opt for lite salt (44%) and salt substitutes (44%). Current smokers predominantly chose ordinary salt (23%), possibly influenced by an impaired sense of taste, whereas never-smokers more frequently reported not using table salt (58%).
Figure 2 illustrates the distribution of urinary metals and the results of the correlation analysis. Among the overall participants, the levels of As and Mo are the highest, while the levels of Sb, Tl, and W are the lowest. After adjusting for urine dilution, the Pearson correlation analysis of log-transformed urinary metals revealed significant positive associations among all metals. The strongest correlation was observed between Cs and Tl (r = 0.58), followed by Ba and Co (r = 0.41) and Cd and Pb (r = 0.40).
Figure 2. The distribution and correlations of 10 urinary metals. (A) Violin plot of urine concentration distribution of 10 heavy metals; (B) Pearson correlation analysis of log-transformed urine metals after adjusting for urine dilution, the depth of the red color represents the correlation’s strength.
Table 1 and Supplementary Table 2 present the differences in urinary metal levels categorized by the type of salt used, frequency of adding salt at the table, and frequency of using salt during cooking. As shown in Table 1, the differences in urinary levels of Cd, Mo, and As between the four groups of participants were significant, while the differences in the other metals were not significant.
As shown in Supplementary Table 2, among participants categorized by the frequency of adding salt at the table, those who responded “Very Often” have significantly higher levels of Cd, Pb, and Sb in their urine compared to those who responded “Rarely” and “Occasionally.” For the other metals, the differences among the three groups were not statistically significant. Among the participants divided according to the frequency of salt use during cooking, there were significant differences in urinary levels of Cd, Mo, and Pb among the four groups of participants.
The association between urinary metal levels and the type of salt used is presented in Table 2. After adjusting for confounding factors, a correlation was found between the type of salt used and urinary heavy metals. Specifically, the use of salt substitutes was significantly positively correlated with urinary Mo levels compared to the use of ordinary salt, while not using salt or substitutes at the table was significantly positively correlated with urinary Mo and As levels.
The association between the frequency of adding salt at the table and urinary metal levels is shown in Table 3. Compared to rarely adding salt at the table, frequently adding salt was significantly positively correlated with urinary Cd, Pb, and Sb levels and significantly negatively correlated with urinary Mo levels. Trend analysis indicates a significant positive trend in urinary Cd, Pb, and Sb excretion levels with increasing frequency of table salt use, while urinary Mo levels show a significant negative trend.
Table 3. Association between urinary levels of 10 metals and the frequency of adding salt at the table.
The association between the frequency of using salt during cooking and urinary metal levels is shown in Table 4. Compared to never adding salt during cooking, occasionally or very often adding salt is significantly positively correlated with urinary levels of Ba, Cs, Pb, and Tl. Trend analysis indicates a significant positive trend in urinary exposure levels of Cs, Pb, Tl, and As with increasing frequency of salt use during cooking, while urinary Mo levels exhibit a significant negative trend.
Table 4. Association between urinary levels of 10 metals and the frequency of using salt during cooking or preparing foods.
After adjusting for fish and shellfish consumption over the past 30 days, the analysis results regarding the association between the type of salt used, the frequency of adding salt at the table, and heavy metals are consistent with the previous findings (Supplementary Tables 3, 4). Compared to never adding salt during cooking, occasionally or very often adding salt is significantly positively correlated with urinary levels of Ba, Cs, Pb, and Tl. Trend analysis indicates a significant positive trend in urinary exposure levels of Cs, Pb, and Tl with increasing frequency of salt use during cooking. Urinary Mo levels continue to exhibit a significant negative trend (Supplementary Table 5).
After excluding current smokers, the analysis results regarding the association between the type of salt used and urinary heavy metals remain consistent with the previous findings (Supplementary Table 6). Compared to rarely adding salt at the table, frequently adding salt is significantly positively correlated with urinary levels of Cd, Pb, and Sb, while it shows a significant negative correlation with urinary Mo levels. Trend analysis indicates a significant positive trend in urinary exposure levels of Pb and Sb with increasing frequency of adding salt at the table. Urinary Mo levels continue to exhibit a significant negative trend (Supplementary Table 7). Compared to never adding salt during cooking, occasionally or very often adding salt is significantly positively correlated with urinary levels of Ba, Cs, and Tl. Trend analysis suggests that with increasing frequency of salt use in cooking, the urinary excretion levels of Cs, Pb, and Tl exhibit a significant positive trend (Supplementary Table 8).
The association between urinary metal levels and the use of antihypertensive prescription medications is shown in Supplementary Table 9. After adjusting for covariates, including age, gender, race/ethnicity, education attainment, smoking status, PIR, BMI, and consumption of shellfish and fish in the past 30 days, the analysis indicates a significant negative correlation between the use of antihypertensive medications and urinary levels of Ba, Cd, Cs, and Pb, while a significant positive correlation was found with urinary W levels. No significant associations were observed between antihypertensive medication use and other metals.
Given the potential impact of antihypertensive medication use, we included the use of antihypertensive drugs as a confounding factor in our sensitivity analysis and conducted a regression analysis to further validate whether the association between salt usage patterns and heavy metal exposure remained stable. The analysis of the association between the type of salt used and urinary metal levels showed that, compared to ordinary salt, the use of salt substitute was significantly positively associated with urinary Mo levels, while not using salt or substitutes at the table was significantly positively associated with urinary Mo and As levels (Supplementary Table 10). In the association between the frequency of salt use at the table and urinary metal levels, frequent use of salt at the table was significantly positively correlated with urinary Cd, Pb, and Sb levels, and negatively correlated with Mo levels, compared to rarely adding salt at the table. Trend tests remained significant (Supplementary Table 11). In the association between salt use during cooking and urinary metal levels, compared to never adding salt during cooking, rare, occasionally, or very often adding salt during cooking was significantly positively associated with urinary Ba, Cs, Pb, and Tl levels (Supplementary Table 12).
To our knowledge, this is the first report on the association between salt usage patterns and heavy metal excretion in the general population. In this cross-sectional study involving 11,574 participants from NHANES, we found that participants’ patterns of salt usage are associated with an increased urinary excretion of certain heavy metals. Specifically, regarding the type of salt used, compared to ordinary salt, which is high in sodium, the use of salt substitutes, which are high in potassium is significantly positively correlated with urinary levels of Mo, while not using salt or substitutes at the table is significantly positively correlated with urinary levels of both Mo and As. Concerning the frequency of adding ordinary salt at the table, frequently adding salt compared to rarely doing so is significantly positively associated with urinary levels of Cd, Pb, and Sb, while being negatively associated with urinary levels of Mo. In terms of the frequency of using ordinary salt during cooking, those who occasionally or very often add salt are significantly positively correlated with urinary levels of Ba, Cs, Pb, and Tl compared to participants who never add salt while cooking.
We found a significant positive correlation between the frequency of adding ordinary salt at the table and/or during cooking and urinary levels of Ba, Cd, Cs, Sb, Pb, and Tl. This association remained stable in sensitivity analyses. We suggest several possible explanations for this correlation: (1) Salt contains trace amounts of heavy metals (44, 45), and with increasing frequency of salt usage, the accumulation of heavy metals in the body may also rise. (2) Salt use may facilitate the release of heavy metal elements from food (46, 47). (3) The dietary habits of these participants may result in higher levels of heavy metals in the food and water they consume. (4) The use of sodium and potassium may influence the absorption and excretion of certain metal elements.
Ba, Cd, Pb, Sb, Tl, and Cs are commonly occurring heavy metal elements that are known to exhibit bioaccumulation and magnification effects (48–52). Some studies have detected the presence of heavy metals in ordinary salt (such as Pb, As, Hg, Cd, and Ba), although the levels are generally considered safe and acceptable (44, 45). Despite significant individual variation in salt intake, the cumulative daily consumption of salt can be substantial (53), potentially contributing to the bioaccumulation and magnification of trace if the salt contains metal contaminants.
The impact of salt use during cooking on the release of heavy metals remains a relatively under-researched area. Although studies have shown that cooking methods and food preparation techniques can influence the bioavailability and release of heavy metals (54, 55), there is a notable lack of in-depth exploration regarding the specific role of salt. Salt serves not only as a seasoning in cooking but may also influence the migration of heavy metals by altering the chemical environment of food and the moisture content. For instance, studies have found that the levels of Hg, Pb, As, and Cd in salted sardines are significantly higher than those in fresh or canned sardines (46). Similarly, salted meat products tend to have relatively high levels of Cs (47). Additionally, some studies have found higher levels of Pb and Cd in certain spices (56). Since salt and spices are often used together, particularly in certain regions or dietary habits, this could further increase the metal burden from dietary intake. Furthermore, changes in pH, salinity, and temperature during cooking may affect the leaching of heavy metals from cooking utensils (57). High temperatures and acidic conditions during cooking can potentially promote the leaching of Tl from aluminum food contact materials, thereby increasing the sources of heavy metal exposure in food (58, 59).
Higher frequencies of salt use at the table and during cooking are associated with increased consumption of processed foods, red meat, and processed meats, along with lower intake of vegetables and fruits (60). More studies have found generally high levels of heavy metals in numerous processed foods (61), including grains, tubers (62, 63), meats (64), and algae (65). Additionally, food packaging materials represent another significant pathway for heavy metal exposure (66, 67). Moreover, higher heavy metal exposure from primary processed foods should not be overlooked (68, 69). Furthermore, excessive salt intake may indirectly lead to increased water consumption (70), and the intake of water contaminated with metals is also a critical pathway for heavy metal exposure.
Our study found that Mo is positively correlated with the use of salt substitutes and not using salt at the table (Table 2), while it shows a negative correlation with the frequency of adding ordinary salt at the table (Table 3) and a significant negative trend with the frequency of using ordinary salt during cooking (Table 4). It is worth noting that in this study, the proportion of participants using salt substitutes was relatively low (1.4%). Despite the limited sample size, we still observed highly significant effects. Currently, there is a lack of reports on the Mo content in salt substitutes. The primary component of salt substitutes is potassium chloride along with some spices and additives. Some studies have found that Mo levels in spices are generally high (71). This may represent an important source of Mo, but further investigation is needed, as the origin and preparation of different spices can significantly affect their Mo content (72). However, participants who tend to choose salt substitutes may primarily follow a lighter vegetarian diet (73), and research indicates that vegetarians have significantly higher Mo intake compared to those on mixed diets (74). This may explain the observed negative trend between the frequency of adding salt at the table and during cooking and Mo excretion. Another possible explanation relates to the types of foods consumed. Participants who frequently add salt during meals and cooking may consume more foods and beverages that are lower in Mo. This demographic often has lower socioeconomic status (75). It has been reported that the main dietary sources of Mo include legumes, grain products, and nuts, with Mo content in legumes ranging from approximately 2.99 to 3.49 μg/g (74). Mo is an essential trace element involved as a cofactor in several key enzymes, such as xanthine oxidase and sulfite oxidase, playing a role in uric acid metabolism and maintaining normal neuronal activity (76). Most Mo intake in the human body comes from food and water (77). Individuals with normal diets and digestive function rarely suffer from Mo deficiency, as sufficient amounts can typically be obtained from food (78). In the body, Mo acts as an antagonist to copper, and excess Mo may lead to various diseases due to copper homeostasis disruption, the interaction of Cu and Mo could similarly mirror an interaction of Na or K and Mo. Additionally, studies have shown that high blood levels of Mo are closely associated with reduced sperm quality in men (79). Regarding hypertension, high airborne Mo exposures are significantly linked to a reduced risk of hypertension (80), and prior research has indicated that using salt substitutes can lower blood pressure (25).
As exposure primarily originates from drinking water, tobacco consumption, and the ingestion of As-contaminated foods (81). Our study found a significant positive correlation between As excretion and not using salt at the table, while no correlation was observed with the frequency of adding ordinary salt at the table or during cooking. This association remained significant even after adjusting for fish and shellfish consumption and excluding smoking participants. It should be noted, however, that the above explanations regarding Mo and As are speculative and currently lack direct supporting evidence. Therefore, targeted studies are needed to further explore and validate these findings.
Whether the use of table salt could influence the absorption and excretion of certain metal elements remains uncertain, as, to the best of our knowledge, no directly relevant studies have been reported. Despite the lack of direct evidence, we propose the following hypothetical mechanisms: (1) Salt intake may affect the intestinal absorption of certain metals. A high-salt diet could potentially damage gastrointestinal epithelial cells or mucosa (82), thereby facilitating the entry of metal ions into the bloodstream. (2) High-salt diets have been found to significantly alter the composition of the gut microbiota (83). Changes in the gut microbiota may, in turn, influence the absorption and excretion of heavy metals by modifying gastrointestinal physiological conditions (e.g., pH), intestinal permeability, and the activity of enzymes involved in heavy metal metabolism (84). (3) The intake levels of sodium and potassium may influence the absorption and excretion of certain metal ions (85–90).
In this study, we analyzed spot urine sample data provided by NHANES to assess metal exposure levels, as it was the only biomarker dataset containing information on multiple metal levels in participants. Urine is one of the most common biomarkers for metal exposure, offering the advantages of being non-invasive and easily obtainable. It has also been widely applied as a marker of human metal exposure in numerous studies (8, 91). However, urinary metal detection does not always provide an accurate or comprehensive reflection of overall metal exposure in the body. Urinary metal levels are closely related to the absorption, biotransformation, and excretion processes of metals within the human body (92). Specifically, urinary Cd is a good biomarker for total body burden and long-term cumulative exposure (93), but urinary Pb is not an effective biomarker for assessing lead exposure in the general population (94). Total As in urine reflects both organic and inorganic As exposure. However, in populations with high fish and seafood intake, urinary total As primarily indicates organic As content rather than the more toxic inorganic As (95). Urinary Ba concentrations mainly reflect recent exposure, typically within the past 3 days to 2 weeks (96). Since Mo is primarily excreted through urine, urinary Mo serves as a good biomarker for short-term Mo exposure, but it is more sensitive to dietary Mo intake (97, 98). Sb primarily enters the body through diet. A study on populations exposed to electronic waste found that occupationally exposed individuals had higher median urinary Sb levels, while blood Sb levels were similar between exposed and unexposed groups (99). Co is also primarily excreted through urine, making urinary Co a critical biomarker for environmental Co exposure (100). Previous studies have shown an association between respiratory and urinary Co levels in workers with occupational Co exposure (101). Cs is excreted approximately 85% through urine, making it a reliable biomarker for Cs exposure (102). Similarly, Tl levels in urine and other biological samples are significantly correlated with environmental Tl exposure (103). For W, its distribution and excretion in mammals are similar to those of Mo, with the majority being filtered by the kidneys and ultimately excreted in urine (104). These findings underscore the objective limitations of using urinary metal elements as biomarkers for human metal exposure. Such limitations pose challenges to the interpretation of our results and highlight the need for caution when inferring associations between salt usage patterns and metal exposure levels based on these findings. Specifically, the associations observed in this study between salt use patterns and Ba, Cd, Cs, Mo, Sb, Tl, and As are more likely to reflect recent or long-term metal exposure levels in participants. However, the association with Pb may not necessarily reflect the participants’ true exposure level.
This study has several unique strengths. First, this study is the first to reveal the potential association between heavy metal urinary excretion and salt consumption patterns. Second, the research assesses various aspects of salt consumption habits, including the type of salt used, the frequency of adding salt at the table, and the frequency of salt use during cooking, in relation to the risk of heavy metal exposure. This study has some limitations. First, the consumption patterns of salt was based on self-reports from participants, which may introduce potential bias. Second, while we adjusted for potential confounding factors, we could not eliminate all influences on heavy metal exposure. Third, the data were sourced from the general population in the United States, and further confirmation is needed regarding their representativeness for other populations. Fourth, due to restricted access to geographic coding data, we were unable to adjust for this information in the regression analyses. However, the potential impacts of dietary patterns and heavy metal exposure levels resulting from geographic differences should not be overlooked. Fifth, the low detection rates for some metal elements may affect statistical power or introduce bias in the results (105, 106). Sixth, this study is based on a cross-sectional design. Although we identified significant associations, whether these associations are causal remains to be further verified.
In summary, our analysis of 11,574 NHANES participants revealed associations between participants’ salt usage patterns and urinary excretion concentrations of certain heavy metals. Specifically, compared to using regular table salt, which is based on NaCl, the use of salt substitutes, which are typically based on KCl was significantly positively correlated with urinary Mo levels, while the frequency of adding regular salt at the table or during cooking was significantly positively correlated with urinary levels of Ba, Cd, Cs, Sb, and Tl. These findings have uncovered some intriguing interactions between salt use patterns and urinary metal excretion, while also highlighting the complexity of the interplay between dietary habits, metal metabolism, and human health. They also provide public health agencies with critical information that must be taken into account when formulating dietary recommendations concerning salt and salt substitute use. While these findings are potentially concerning, they require validation in other populations and should be confirmed through prospective studies designed based on this hypothesis.
Publicly available datasets were analyzed in this study. This data can be found at: https://wwwn.cdc.gov/nchs/nhanes/.
The studies involving humans were approved by the National Center for Health Statistics Ethics Review Board. The studies were conducted in accordance with the local legislation and institutional requirements. The participants provided their written informed consent to participate in this study.
SZ: Conceptualization, Data curation, Methodology, Resources, Visualization, Writing – original draft, Writing – review & editing. HT: Formal analysis, Methodology, Writing – original draft, Writing – review & editing. MZ: Methodology, Writing – review & editing. LP: Writing – review & editing.
The author(s) declare that no financial support was received for the research, authorship, and/or publication of this article.
Thanks to the editors and reviewers for their precious time and constructive comments. The authors thank the participants and staff of NHANES.
The authors declare that the research was conducted in the absence of any commercial or financial relationships that could be construed as a potential conflict of interest.
The authors declare that no Gen AI was used in the creation of this manuscript.
All claims expressed in this article are solely those of the authors and do not necessarily represent those of their affiliated organizations, or those of the publisher, the editors and the reviewers. Any product that may be evaluated in this article, or claim that may be made by its manufacturer, is not guaranteed or endorsed by the publisher.
The Supplementary material for this article can be found online at: https://www.frontiersin.org/articles/10.3389/fnut.2024.1521826/full#supplementary-material
1. Briggs, D. Environmental pollution and the global burden of disease. Br Med Bull. (2003) 68:1–24. doi: 10.1093/bmb/ldg019
2. Naddafi, K, Mesdaghinia, A, Abtahi, M, Hassanvand, MS, Beiki, A, Shaghaghi, G, et al. Assessment of burden of disease induced by exposure to heavy metals through drinking water at national and subnational levels in Iran, 2019. Environ Res. (2022) 204:112057. doi: 10.1016/j.envres.2021.112057
3. Zhou, N, Huang, Y, Li, M, Zhou, L, and Jin, H. Trends in global burden of diseases attributable to lead exposure in 204 countries and territories from 1990 to 2019. Front Public Health. (2022) 10:1036398. doi: 10.3389/fpubh.2022.1036398
4. Järup, L. Hazards of heavy metal contamination. Br Med Bull. (2003) 68:167–82. doi: 10.1093/bmb/ldg032
5. Pan, Z, Gong, T, and Liang, P. Heavy metal exposure and cardiovascular disease. Circ Res. (2024) 134:1160–78. doi: 10.1161/CIRCRESAHA.123.323617
6. Wang, X, Mukherjee, B, and Park, SK. Associations of cumulative exposure to heavy metal mixtures with obesity and its comorbidities among U.S. adults in NHANES 2003-2014. Environ Int. (2018) 121:683–94. doi: 10.1016/j.envint.2018.09.035
7. Yan, Z, Xu, Y, Li, K, and Liu, L. Heavy metal levels and flavonoid intakes are associated with chronic obstructive pulmonary disease: an NHANES analysis (2007-2010 to 2017-2018). BMC Public Health. (2023) 23:2335. doi: 10.1186/s12889-023-17250-x
8. Chen, Y, Pan, Z, Shen, J, Wu, Y, Fang, L, Xu, S, et al. Associations of exposure to blood and urinary heavy metal mixtures with psoriasis risk among U.S. adults: A cross-sectional study. Sci Total Environ. (2023) 887:164133. doi: 10.1016/j.scitotenv.2023.164133
9. Lim, JT, Tan, YQ, Valeri, L, Lee, J, Geok, PP, Chia, SE, et al. Association between serum heavy metals and prostate cancer risk - A multiple metal analysis. Environ Int. (2019) 132:105109. doi: 10.1016/j.envint.2019.105109
10. Navas-Acien, A, Guallar, E, Silbergeld, EK, and Rothenberg, SJ. Lead exposure and cardiovascular disease--a systematic review. Environ Health Perspect. (2007) 115:472–82. doi: 10.1289/ehp.9785
11. Duan, W, Xu, C, Liu, Q, Xu, J, Weng, Z, Zhang, X, et al. Levels of a mixture of heavy metals in blood and urine and all-cause, cardiovascular disease and cancer mortality: A population-based cohort study. Environ Pollut. (2020) 263:114630. doi: 10.1016/j.envpol.2020.114630
12. WHO (2021). Lead poisoning. Available at: https://www.who.int/news-room/fact-sheets/detail/lead-poisoning-and-health [Accessed October 25, 2024]
13. Van der Veen, JE, De Graaf, C, Van Dis, SJ, and Van Staveren, WA. Determinants of salt use in cooked meals in the Netherlands: attitudes and practices of food preparers. Eur J Clin Nutr. (1999) 53:388–94. doi: 10.1038/sj.ejcn.1600737
14. Ma, H, Wang, X, Li, X, Heianza, Y, and Qi, L. Adding salt to foods and risk of cardiovascular disease. J Am Coll Cardiol. (2022) 80:2157–67. doi: 10.1016/j.jacc.2022.09.039
15. Sharif, R, Ghazali, AR, Rajab, NF, Haron, H, and Osman, F. Toxicological evaluation of some Malaysian locally processed raw food products. Food Chem Toxicol. (2008) 46:368–74. doi: 10.1016/j.fct.2007.08.010
16. Yamaguchi, N, and Kakizoe, T. Synergistic interaction between Helicobacter pylori gastritis and diet in gastric cancer. Lancet Oncol. (2001) 2:88–94. doi: 10.1016/S1470-2045(00)00225-4
17. Hu, J, La Vecchia, C, Morrison, H, Negri, E, and Mery, L. Canadian Cancer registries epidemiology research group. Salt, processed meat and the risk of cancer. Eur J Cancer Prev. (2011) 20:132–9. doi: 10.1097/CEJ.0b013e3283429e32
18. Micha, R, Peñalvo, JL, Cudhea, F, Imamura, F, Rehm, CD, and Mozaffarian, D. Association between dietary factors and mortality from heart disease, stroke, and type 2 diabetes in the United States. JAMA. (2017) 317:912–24. doi: 10.1001/jama.2017.0947
19. Zhou, G, Gan, L, Zhao, B, Fang, F, Liu, H, Chen, X, et al. Adding salt to foods and risk of psoriasis: A prospective cohort study. J Autoimmun. (2024) 147:103259. doi: 10.1016/j.jaut.2024.103259
20. Tang, R, Kou, M, Wang, X, Ma, H, Li, X, Heianza, Y, et al. Self-reported frequency of adding salt to food and risk of incident chronic kidney disease. JAMA Netw Open. (2023) 6:e2349930. doi: 10.1001/jamanetworkopen.2023.49930
21. Li, T, Song, L, Li, G, Li, F, Wang, X, Chen, L, et al. Eating habit of adding salt to foods and incident sleep apnea: a prospective cohort study. Respir Res. (2023) 24:5. doi: 10.1186/s12931-022-02300-6
22. Wang, X, Ma, H, Kou, M, Tang, R, Xue, Q, Li, X, et al. Dietary sodium intake and risk of incident type 2 diabetes. Mayo Clin Proc. (2023) 98:1641–52. doi: 10.1016/j.mayocp.2023.02.029
23. Radzeviciene, L, and Ostrauskas, R. Adding salt to meals as a risk factor of type 2 diabetes mellitus: A case-control study. Nutrients. (2017) 9:67. doi: 10.3390/nu9010067
24. Zhou, R, Chen, F, Zhang, L, Sun, Y, Hu, R, Yan, J, et al. Association between adding salt in food and dementia in European descent: A mendelian randomization study. Brain Behav. (2024) 14:e3516. doi: 10.1002/brb3.3516
25. Neal, B, Wu, Y, Feng, X, Zhang, R, Zhang, Y, Shi, J, et al. Effect of salt substitution on cardiovascular events and death. N Engl J Med. (2021) 385:1067–77. doi: 10.1056/NEJMoa2105675
26. Chan, MWH, Hasan, KA, Balthazar-Silva, D, Mirani, ZA, and Asghar, M. Evaluation of heavy metal pollutants in salt and seawater under the influence of the Lyari River and potential health risk assessment. Mar Pollut Bull. (2021) 166:112215. doi: 10.1016/j.marpolbul.2021.112215
27. Shariatifar, N, Mozaffari Nejad, AS, and Ebadi Fathabad, A. Assessment of heavy metal content in refined and unrefined salts obtained from Urmia. Iran Toxin Reviews. (2016) 36:89–93. doi: 10.1080/15569543.2016.1262875
28. Di Salvo, E, Tardugno, R, Nava, V, Naccari, C, Virga, A, Salvo, A, et al. Gourmet table salts: the mineral composition showdown. Toxics. (2023) 11:705. doi: 10.3390/toxics11080705
29. Amorim, FAC, and Ferreira, SLC. Determination of cadmium and lead in table salt by sequential multi-element flame atomic absorption spectrometry. Talanta. (2005) 65:960–4. doi: 10.1016/j.talanta.2004.08.027
30. Devesa, V, Macho, ML, Jalón, M, Urieta, I, Muñoz, O, Súñer, MA, et al. Arsenic in cooked seafood products: study on the effect of cooking on total and inorganic arsenic contents. J Agric Food Chem. (2001) 49:4132–40. doi: 10.1021/jf010274l
31. Morshdy, AEMA, Tharwat, AE, Maarouf, H, Moustafa, M, Darwish, WS, El-Ghareeb, WR, et al. Heavy metal contents in salted fish retailed in Egypt: dietary intakes and health risk assessment. Open Vet J. (2023) 13:1738. doi: 10.5455/OVJ.2023.v13.i12.22
32. Kronsteiner-Gicevic, S, Thompson, AS, Gaggl, M, Bell, W, Cassidy, A, and Kühn, T. Adding salt to food at table as an indicator of gastric cancer risk among adults: a prospective study. Gastric Cancer. (2024) 27:714–21. doi: 10.1007/s10120-024-01502-9
33. Henney, JE, Taylor, CL, and Boon, CS. Background on the National Health and nutrition examination surveys and data analysis methods In: Strategies to reduce sodium intake in the United States. US: National Academies Press (2010).
34. Yin, X, Liu, H, Webster, J, Trieu, K, Huffman, MD, Miranda, JJ, et al. Availability, formulation, labeling, and Price of low-sodium salt worldwide: environmental scan. JMIR Public Health Surveillance. (2021) 7:e27423. doi: 10.2196/27423
35. Murphy, MM, Scrafford, CG, Barraj, LM, Bi, X, Higgins, KA, Jaykus, L-A, et al. Potassium chloride-based replacers: modeling effects on sodium and potassium intakes of the US population with cross-sectional data from NHANES 2015–2016 and 2009–2010. Am J Clin Nutr. (2021) 114:220–30. doi: 10.1093/ajcn/nqab020
36. Olmedo, P, Pla, A, Hernández, AF, Barbier, F, Ayouni, L, and Gil, F. Determination of toxic elements (mercury, cadmium, lead, tin and arsenic) in fish and shellfish samples. Risk assessment for the consumers. Environ Int. (2013) 59:63–72. doi: 10.1016/j.envint.2013.05.005
37. Foran, JA, Hites, RA, Carpenter, DO, Hamilton, MC, Mathews-Amos, A, and Schwager, SJ. A survey of metals in tissues of farmed Atlantic and wild Pacific salmon. Environ Toxicol Chem. (2004) 23:2108–10. doi: 10.1897/04-72
38. Mielcarek, K, Nowakowski, P, Puścion-Jakubik, A, Gromkowska-Kępka, KJ, Soroczyńska, J, Markiewicz-Żukowska, R, et al. Arsenic, cadmium, lead and mercury content and health risk assessment of consuming freshwater fish with elements of chemometric analysis. Food Chem. (2022) 379:132167. doi: 10.1016/j.foodchem.2022.132167
39. Ghaderi, A, NasehGhafoori, P, Rasouli-Azad, M, Sehat, M, Mehrzad, F, Nekuei, M, et al. Examining of thallium in cigarette smokers. Biol Trace Elem Res. (2017) 182:224–30. doi: 10.1007/s12011-017-1107-y
40. Cao, J, and Zhang, S. Multiple comparison procedures. JAMA. (2014) 312:543. doi: 10.1001/jama.2014.9440
41. Boyle, M, Buckley, JP, and Quirós-Alcalá, L. Associations between urinary organophosphate ester metabolites and measures of adiposity among U.S. children and adults: NHANES 2013-2014. Environ Int. (2019) 127:754–63. doi: 10.1016/j.envint.2019.03.055
42. Li, T, Yu, L, Yang, Z, Shen, P, Lin, H, Shui, L, et al. Associations of diet quality and heavy metals with obesity in adults: A cross-sectional Study from National Health and nutrition examination survey (NHANES). Nutrients. (2022) 14:4038. doi: 10.3390/nu14194038
43. Zhu, R, Wang, R, He, J, Wang, L, Chen, H, Niu, X, et al. Prevalence of cardiovascular-kidney-metabolic syndrome stages by social determinants of health. JAMA Netw Open. (2024) 7:e2445309. doi: 10.1001/jamanetworkopen.2024.45309
44. Wang, Z, Zhou, J, and Jia, X. Is the cooking salt safe in China? Assessment of chemical contaminants in cooking salt. Biol Trace Elem Res. (2019) 191:512–6. doi: 10.1007/s12011-019-1646-5
45. Cheraghali, AM, Kobarfard, F, and Faeizy, N. Heavy metals contamination of table salt consumed in iran. Iran J Pharm Res (2010) 9:129–132. doi: 10.22037/ijpr.2010.847
46. Sabala, RF, Zakaria, AI, Imre, K, Morar, A, Herman, V, and Sallam, KI. Potential cancer risks associated with the consumption of raw, salted, and canned sardine contaminated by mercury, arsenic, lead, and cadmium in Egypt. J Food Compos Anal. (2024) 134:106516. doi: 10.1016/j.jfca.2024.106516
47. Nabeshi, H, Tsutsumi, T, Hachisuka, A, and Matsuda, R. Reduction of radioactive cesium content in pond smelt by cooking. Food Hyg Safe Sci. (2013) 54:303–8. doi: 10.3358/shokueishi.54.303
48. Peana, M, Pelucelli, A, Chasapis, CT, Perlepes, SP, Bekiari, V, Medici, S, et al. Biological effects of human exposure to environmental cadmium. Biomol Ther. (2022) 13:36. doi: 10.3390/biom13010036
49. Peana, M, Medici, S, Dadar, M, Zoroddu, MA, Pelucelli, A, Chasapis, CT, et al. Environmental barium: potential exposure and health-hazards. Arch Toxicol. (2021) 95:2605–12. doi: 10.1007/s00204-021-03049-5
50. Barwick, M, and Maher, W. Biotransference and biomagnification of selenium copper, cadmium, zinc, arsenic and lead in a temperate seagrass ecosystem from Lake Macquarie estuary, NSW. Australia Mar Environ Res. (2003) 56:471–502. doi: 10.1016/S0141-1136(03)00028-X
51. Natasha, SM, Khalid, S, Dumat, C, Pierart, A, and Niazi, NK. Biogeochemistry of antimony in soil-plant system: ecotoxicology and human health. Appl Geochem. (2019) 106:45–59. doi: 10.1016/j.apgeochem.2019.04.006
52. Harmelin-Vivien, M, Bodiguel, X, Charmasson, S, Loizeau, V, Mellon-Duval, C, Tronczyński, J, et al. Differential biomagnification of PCB, PBDE, hg and Radiocesium in the food web of the European hake from the NW Mediterranean. Mar Pollut Bull. (2012) 64:974–83. doi: 10.1016/j.marpolbul.2012.02.014
53. Bibbins-Domingo, K, Chertow, GM, Coxson, PG, Moran, A, Lightwood, JM, Pletcher, MJ, et al. Projected effect of dietary salt reductions on future cardiovascular disease. N Engl J Med. (2010) 362:590–9. doi: 10.1056/NEJMoa0907355
54. Domingo, JL. Influence of cooking processes on the concentrations of toxic metals and various organic environmental pollutants in food: a review of the published literature. Crit Rev Food Sci Nutr. (2010) 51:29–37. doi: 10.1080/10408390903044511
55. Chera-Anghel, I-A, and Stefan-van Staden, R-I. Extraction of heavy metals by cooking/preserving of seafood, tuna and poultry from Romania - A source of contamination with heavy metals. Food Chem. (2023) 407:135158. doi: 10.1016/j.foodchem.2022.135158
56. Alawadhi, N, Abass, K, Khaled, R, Osaili, TM, and Semerjian, L. Heavy metals in spices and herbs from worldwide markets: A systematic review and health risk assessment. Environ Pollut. (2024) 362:124999. doi: 10.1016/j.envpol.2024.124999
57. Zubaidy, EAHA, FathiaS, M, and Bassioni, G. Effect of pH, salinity and temperature on aluminum cookware leaching during food preparation. Int J Electrochem Sci. (2011) 6:6424–41. doi: 10.1016/S1452-3981(23)19691-X
58. Sander, S, Kappenstein, O, Ebner, I, Fritsch, K-A, Schmidt, R, Pfaff, K, et al. Release of aluminium and thallium ions from uncoated food contact materials made of aluminium alloys into food and food simulant. PLoS One. (2018) 13:e0200778. doi: 10.1371/journal.pone.0200778
59. Weidenhamer, JD, Fitzpatrick, MP, Biro, AM, Kobunski, PA, Hudson, MR, Corbin, RW, et al. Metal exposures from aluminum cookware: An unrecognized public health risk in developing countries. Sci Total Environ. (2017) 579:805–13. doi: 10.1016/j.scitotenv.2016.11.023
60. Ma, H, Xue, Q, Wang, X, Li, X, Franco, OH, Li, Y, et al. Adding salt to foods and hazard of premature mortality. Eur Heart J. (2022) 43:2878–88. doi: 10.1093/eurheartj/ehac208
61. Morgan, JN. Effects of processing of heavy metal content of foods. Adv Exp Med Biol. (1999) 459:195–211. doi: 10.1007/978-1-4615-4853-9_13
62. Román-Ochoa, Y, Choque Delgado, GT, Tejada, TR, Yucra, HR, Durand, AE, and Hamaker, BR. Heavy metal contamination and health risk assessment in grains and grain-based processed food in Arequipa region of Peru. Chemosphere. (2021) 274:129792. doi: 10.1016/j.chemosphere.2021.129792
63. Pearson, AJ, and Ashmore, E. Risk assessment of antimony, barium, beryllium, boron, bromine, lithium, nickel, strontium, thallium and uranium concentrations in the New Zealand diet. Food Addit Contam Part A Chem Anal Control Expo Risk Assess. (2019) 37:451–64. doi: 10.1080/19440049.2019.1704445
64. Sarlak, Z, Hosseini, H, Garavand, F, Mohammadi, R, and Rouhi, M. The occurrence of Lead in animal source foods in Iran in the 2010s decade: A systematic review. Biol Trace Elem Res. (2021) 200:1917–36. doi: 10.1007/s12011-021-02787-y
65. Cavallo, G, Lorini, C, Garamella, G, and Bonaccorsi, G. Seaweeds as a “palatable” challenge between innovation and sustainability: A systematic review of food safety. Sustain For. (2021) 13:7652. doi: 10.3390/su13147652
66. Bandara, R, and Indunil, GM. Food packaging from recycled papers: chemical, physical, optical properties and heavy metal migration. Heliyon. (2022) 8:e10959. doi: 10.1016/j.heliyon.2022.e10959
67. Nguyen, V-T, Linh, TTT, Vo, T-K, Nguyen, QH, and Van, T-K. Analytical techniques for determination of heavy metal migration from different types of locally made plastic food packaging materials using ICP-MS. Food Sci Nutr. (2023) 11:4030–7. doi: 10.1002/fsn3.3391
68. Collado-López, S, Betanzos-Robledo, L, Téllez-Rojo, MM, Lamadrid-Figueroa, H, Reyes, M, Ríos, C, et al. Heavy metals in unprocessed or minimally processed foods consumed by humans worldwide: A scoping review. Int J Environ Res Public Health. (2022) 19:8651. doi: 10.3390/ijerph19148651
69. Liu, J, Luo, X, Wang, J, Xiao, T, Chen, D, Sheng, G, et al. Thallium contamination in arable soils and vegetables around a steel plant-A newly-found significant source of Tl pollution in South China. Environ Pollut. (2017) 224:445–53. doi: 10.1016/j.envpol.2017.02.025
70. He, FJ, Markandu, ND, Sagnella, GA, and MacGregor, GA. Effect of salt intake on renal excretion of water in humans. Hypertension. (2001) 38:317–20. doi: 10.1161/01.hyp.38.3.317
71. Seifert, M, Dorn, W, Mueller, R, Holzinger, S, and Anke, M. The biological and toxicological importance of molybdenum in the environment and in the nutrition of plants, animals and man part iii. Molybdenum content of the food. Acta Aliment Hung. (2009) 38:471–81. doi: 10.1556/AAlim.38.2009.4.7
72. Palou, L, Usall, J, Smilanick, JL, Aguilar, M-J, and Viñas, I. Evaluation of food additives and low-toxicity compounds as alternative chemicals for the control of Penicillium digitatum and Penicillium italicum on citrus fruit. Pest Manag Sci. (2002) 58:459–66. doi: 10.1002/ps.477
73. Gajari, D, Rumbak, I, Ranilović, J, and Tomić-Obrdalj, H. Application of a salt substitute in bitter taste suppression and toward better acceptance of cruciferous vegetables in diet. Appetite. (2022) 173:105996. doi: 10.1016/j.appet.2022.105996
74. Foteva, V, Fisher, JJ, Qiao, Y, and Smith, R. Does the micronutrient molybdenum have a role in gestational complications and placental health? Nutrients. (2023) 15:3348. doi: 10.3390/nu15153348
75. de Mestral, C, Mayén, A-L, Petrovic, D, Marques-Vidal, P, Bochud, M, and Stringhini, S. Socioeconomic determinants of sodium intake in adult populations of high-income countries: A systematic review and Meta-analysis. Am J Public Health. (2017) 107:e1–e12. doi: 10.2105/AJPH.2016.303629
76. Jomova, K, Makova, M, Alomar, SY, Alwasel, SH, Nepovimova, E, Kuca, K, et al. Essential metals in health and disease. Chem Biol Interact. (2022) 367:110173. doi: 10.1016/j.cbi.2022.110173
77. Smedley, PL, and Kinniburgh, DG. Molybdenum in natural waters: A review of occurrence, distributions and controls. Appl Geochem. (2017) 84:387–432. doi: 10.1016/j.apgeochem.2017.05.008
78. Gharibzahedi, SMT, and Jafari, SM. The importance of minerals in human nutrition: bioavailability, food fortification, processing effects and nanoencapsulation. Trends Food Sci Technol. (2017) 62:119–32. doi: 10.1016/j.tifs.2017.02.017
79. Meeker, JD, Rossano, MG, Protas, B, Diamond, MP, Puscheck, E, Daly, D, et al. Cadmium, lead, and other metals in relation to semen quality: human evidence for molybdenum as a male reproductive toxicant. Environ Health Perspect. (2008) 116:1473–9. doi: 10.1289/ehp.11490
80. Xu, J, White, AJ, Niehoff, NM, O’Brien, KM, and Sandler, DP. Airborne metals exposure and risk of hypertension in the sister study. Environ Res. (2020) 191:110144. doi: 10.1016/j.envres.2020.110144
81. Chung, J-Y, Yu, S-D, and Hong, Y-S. Environmental source of arsenic exposure. J Prev Med Public Health. (2014) 47:253–7. doi: 10.3961/jpmph.14.036
82. Fox, JG, Dangler, CA, Taylor, NS, King, A, Koh, TJ, and Wang, TC. High-salt diet induces gastric epithelial hyperplasia and parietal cell loss, and enhances Helicobacter pylori colonization in C57BL/6 mice. Cancer Res. (1999) 59:4823–8.
83. Yan, X, Jin, J, Su, X, Yin, X, Gao, J, Wang, X, et al. Intestinal Flora modulates blood pressure by regulating the synthesis of intestinal-derived corticosterone in high salt-induced hypertension. Circ Res. (2020) 126:839–53. doi: 10.1161/CIRCRESAHA.119.316394
84. Arun, KB, Madhavan, A, Sindhu, R, Emmanual, S, Binod, P, Pugazhendhi, A, et al. Probiotics and gut microbiome - prospects and challenges in remediating heavy metal toxicity. J Hazard Mater. (2021) 420:126676. doi: 10.1016/j.jhazmat.2021.126676
85. Newton, GL, Fontenot, JP, Tucker, RE, and Polan, CE. Effects of high dietary potassium intake on the metabolism of magnesium by sheep. J Anim Sci. (1972) 35:440–5. doi: 10.2527/jas1972.352440x
86. Kies, C, and Harms, JM. Copper absorption as affected by supplemental calcium, magnesium, manganese, selenium and potassium. Adv Exp Med Biol. (1989) 258:45–58. doi: 10.1007/978-1-4613-0537-8_4
87. Massey, LK. Dietary salt, urinary calcium, and kidney stone risk. Nutr Rev. (2009) 53:131–4. doi: 10.1111/j.1753-4887.1995.tb01536.x
88. Lemann, J, Pleuss, JA, Gray, RW, and Hoffmann, RG. Potassium administration reduces and potassium deprivation increases urinary calcium excretion in healthy adults [corrected]. Kidney Int. (1991) 39:973–83. doi: 10.1038/ki.1991.123
89. Wapnir, RA, and Stiel, L. Intestinal absorption of copper: effect of sodium. Proc Soc Exp Biol Med. (1987) 185:277–82. doi: 10.3181/00379727-185-42545
90. Handy, RD, Eddy, FB, and Baines, H. Sodium-dependent copper uptake across epithelia: a review of rationale with experimental evidence from gill and intestine. Biochim Biophys Acta. (2002) 1566:104–15. doi: 10.1016/s0005-2736(02)00590-4
91. Tao, C, Li, Z, Fan, Y, Li, X, Qian, H, Yu, H, et al. Independent and combined associations of urinary heavy metals exposure and serum sex hormones among adults in NHANES 2013-2016. Environ Pollut. (2021) 281:117097. doi: 10.1016/j.envpol.2021.117097
92. Martinez-Morata, I, Sobel, M, Tellez-Plaza, M, Navas-Acien, A, Howe, CG, and Sanchez, TR. A state-of-the-science review on metal biomarkers. Curr Environ Health Rep. (2023) 10:215–49. doi: 10.1007/s40572-023-00402-x
93. Hsieh, C-Y, Wang, S-L, Fadrowski, JJ, Navas-Acien, A, and Kuo, C-C. Urinary concentration correction methods for arsenic, cadmium, and mercury: a systematic review of practice-based evidence. Curr Environ Health Rep. (2019) 6:188–99. doi: 10.1007/s40572-019-00242-8
94. Sommar, JN, Hedmer, M, Lundh, T, Nilsson, L, Skerfving, S, and Bergdahl, IA. Investigation of lead concentrations in whole blood, plasma and urine as biomarkers for biological monitoring of lead exposure. J Expo Sci Environ Epidemiol. (2013) 24:51–7. doi: 10.1038/jes.2013.4
95. Navas-Acien, A, Francesconi, KA, Silbergeld, EK, and Guallar, E. Seafood intake and urine concentrations of total arsenic, dimethylarsinate and arsenobetaine in the US population. Environ Res. (2011) 111:110–8. doi: 10.1016/j.envres.2010.10.009
96. Harrison, GE, Carr, TE, Sutton, A, and Rundo, J. Plasma concentration and excretion of calcium-47, strontium-85, barium-133 and radium-223 following successive intravenous doses to a healthy man. Nature. (1966) 209:526–7. doi: 10.1038/209526b0
97. Novotny, JA, and Turnlund, JR. Molybdenum intake influences molybdenum kinetics in men. J Nutr. (2007) 137:37–42. doi: 10.1093/jn/137.1.37
98. Turnlund, JR, Keyes, WR, and Peiffer, GL. Molybdenum absorption, excretion, and retention studied with stable isotopes in young men at five intakes of dietary molybdenum. Am J Clin Nutr. (1995) 62:790–6. doi: 10.1093/ajcn/62.4.790
99. Julander, A, Lundgren, L, Skare, L, Grandér, M, Palm, B, Vahter, M, et al. Formal recycling of e-waste leads to increased exposure to toxic metals: an occupational exposure study from Sweden. Environ Int. (2014) 73:243–51. doi: 10.1016/j.envint.2014.07.006
100. Scansetti, G, Botta, GC, Spinelli, P, Reviglione, L, and Ponzetti, C. Absorption and excretion of cobalt in the hard metal industry. Sci Total Environ. (1994) 150:141–4. doi: 10.1016/0048-9697(94)90141-4
101. Lison, D, Buchet, JP, Swennen, B, Molders, J, and Lauwerys, R. Biological monitoring of workers exposed to cobalt metal, salt, oxides, and hard metal dust. Occup Environ Med. (1994) 51:447–50. doi: 10.1136/oem.51.7.447
102. Rw, L, Lr, W, Dr, M, and Jl, L. A physiologically based biokinetic model for cesium in the human body. Sci Total Environ. (2003) 317:235–55. doi: 10.1016/S0048-9697(03)00333-4
103. Campanella, B, Onor, M, D’Ulivo, A, Giannecchini, R, D’Orazio, M, Petrini, R, et al. Human exposure to thallium through tap water: A study from Valdicastello Carducci and Pietrasanta (northern Tuscany, Italy). Sci Total Environ. (2016) 548-549:33–42. doi: 10.1016/j.scitotenv.2016.01.010
104. Wang, Y, Nie, B, Zheng, S, Wu, H, Chen, N, and Wang, D. Emerging activated tungsten dust: source, environmental behaviors, and health effects. Environ Int. (2024) 188:108774. doi: 10.1016/j.envint.2024.108774
105. Richardson, DB, and Ciampi, A. Effects of exposure measurement error when an exposure variable is constrained by a lower limit. Am J Epidemiol. (2003) 157:355–63. doi: 10.1093/aje/kwf217
Keywords: salt, heavy metals, dietary patterns, exposure risk, NHANES
Citation: Zhang S, Tang H, Zhou M and Pan L (2025) Salt use patterns and heavy metal urinary excretion. Front. Nutr. 11:1521826. doi: 10.3389/fnut.2024.1521826
Received: 03 November 2024; Accepted: 30 December 2024;
Published: 10 January 2025.
Edited by:
Bei Gao, Nanjing University of Information Science and Technology, ChinaReviewed by:
Rui Wang, Shanghai Jiao Tong University, ChinaCopyright © 2025 Zhang, Tang, Zhou and Pan. This is an open-access article distributed under the terms of the Creative Commons Attribution License (CC BY). The use, distribution or reproduction in other forums is permitted, provided the original author(s) and the copyright owner(s) are credited and that the original publication in this journal is cited, in accordance with accepted academic practice. No use, distribution or reproduction is permitted which does not comply with these terms.
*Correspondence: Shuai Zhang, c2Vub21lZ2FAaG90bWFpbC5jb20=
†These authors have contributed equally to this work
Disclaimer: All claims expressed in this article are solely those of the authors and do not necessarily represent those of their affiliated organizations, or those of the publisher, the editors and the reviewers. Any product that may be evaluated in this article or claim that may be made by its manufacturer is not guaranteed or endorsed by the publisher.
Research integrity at Frontiers
Learn more about the work of our research integrity team to safeguard the quality of each article we publish.