- 1Arkansas Children’s Nutrition Center and Department of Pediatrics, University of Arkansas for Medical Sciences, Little Rock, AR, United States
- 2Department of Pediatrics, Larsson-Rosenquist Foundation Mother-Milk-Infant Center of Research Excellence (MOMI CORE), and the Human Milk Institute (HMI), University of California, San Diego, La Jolla, CA, United States
Introduction: Maternal diet modifies profiles of human milk oligosaccharides (HMOs), carotenoids, and polyphenols in human milk (HM). However, substantial variability in profiles exists between women, highlighting the complexity of non-dietary factors modulating these profiles. The objective of this study was to carry out a secondary analysis exploring the effect of maternal diet on HM carotenoids and polyphenols and relationships between dietary modulation of HM bioactives (carotenoids, polyphenols, and oligosaccharides) and maternal α1,2-fucosyltransferase 2 (FUT2) secretor phenotype.
Methods: In this pilot study, 16 exclusively breastfeeding women with obesity were enrolled between 4 and 5 months postpartum. The women were provided a 4-week meal plan consistent with the 2020 Dietary Guidelines for Americans (DGA). HM was collected for 24 h at baseline and post-intervention. Maternal FUT2 secretor phenotype was determined by 2′-fucosyllactose concentration in HM (non-secretor: < 100 nmol/ml; secretor: ≥100 nmol/ml). Concentrations of carotenoids and HMOs were determined by LC and polyphenol metabolites by UPLC–MS/MS.
Results: Thirteen women completed the study (6 secretors, 7 non-secretors). The change in HM concentrations of the HMOs lacto-N-tetraose (LNT, p = 0.007), lacto-N-fucopentaose II (LNFP II, p = 0.02), difucosyllacto-N-tetraose (DFLNT, p = 0.003), and disialyllacto-N-tetraose (DSLNT, p = 0.003) and polyphenol metabolites 4-hydroxybenzoic acid (4-HBA, p = 0.08) and ferulic acid (p = 0.02) over the intervention time frame was differentially associated with maternal secretor status. 4-HBA and ferulic acid positively correlated with HMOs LNT and DSLNT (rrm = 0.82–0.90, p = 0.03–0.06) for secretors but not for non-secretors. Only secretors demonstrated a negative correlation between 4-HBA and DFLNT (rrm = −0.94, p = 0.001).
Discussion: The influence of maternal diet on composition of HMOs and polyphenol metabolites in HM differs based on maternal secretor status. Consideration of non-dietary factors is needed to evaluate differences in response of HM bioactives to dietary modulation.
1 Introduction
Human milk (HM) is recognized as the optimal source of infant nutrition by the World Health Organization (WHO) (1) and the American Academy of Pediatrics (AAP) (2). Human milk has a unique composition of various bioactive components, including HM oligosaccharides (HMOs) (3, 4), carotenoids (5, 6), and polyphenols (6–8). Growing clinical evidence suggests that intake of HMOs (9–12), carotenoids (13–16), and polyphenols (17, 18) by breastfed infants supports gut and potentially cognitive development. Since carotenoids and polyphenols are not produced endogenously, they must be supplied by maternal dietary intake, which has been identified as a key factor driving concentrations of both bioactives in HM (7, 17, 19–23). Although HMOs are not derived directly from dietary intake, maternal diet has also been demonstrated to modify or associate with profiles of HMOs (24–27).
While the primary driver of carotenoid profiles in HM is maternal diet (19–22), there are multiple drivers of the variability observed in HMOs (25, 28). Other than maternal diet, known drivers of HMO variability include maternal genetics, such as α1,2-fucosyltransferase 2 (FUT2) secretor status (29, 30), and lactation duration (30–32). Variability is also observed in HM polyphenol profiles, despite similar maternal polyphenol intake (17, 18, 33), highlighting the fact that, like HMOs, profiles could be modified by non-dietary factors. Recent observational studies have suggested that maternal polyphenol intake is associated with HM microbiome composition (24) and HMO profiles (25). Associations between polyphenol intake and HMO profiles may depend on maternal secretor status (25), which could be due to differences in HM microbiome composition associated with maternal secretor status (34). Because it is well-established that dietary polyphenols undergo extensive gut microbial metabolism (35, 36), it is possible that a relationship between maternal polyphenol intake, secretor status, and composition of HM microbiota, polyphenols, and oligosaccharides exists. Integrated approaches are needed to improve our understanding of factors driving composition of HM bioactives that are diet-derived or diet-driven, such as oligosaccharides and polyphenols in HM. Thus far, no human studies have explored the role of maternal diet on diet-driven HM bioactive components when considering maternal secretor status as a factor. The present study was a secondary analysis of a within-subject pilot intervention study that was designed to determine if adherence to a healthy diet during lactation would influence the macronutrient and bioactive (hormone, HMO, cytokine) composition of HM in women with obesity, as described previously (26). There were two objectives of this secondary analysis: (1) explore the effect of maternal diet on carotenoids and polyphenols in HM and (2) carry out an exploratory analysis of relationships between dietary modulation of HM bioactives (HMOs, carotenoids, polyphenols) and FUT2 secretor phenotype.
2 Materials and methods
2.1 Chemicals and reagents
Ammonium acetate and LC–MS grade acetonitrile, methanol, formic acid, and water were purchased from Fisher Scientific (Waltham, MA, USA) and Alfa Aesar (Ward Hill, MA, USA). Potassium hydroxide (KOH) and HPLC-grade ethyl acetate were purchased from Sigma-Aldrich (St. Louis, MO, USA). ACS-grade petroleum ether and acetone were purchased from Thermo Scientific (Waltham, MA, USA) and butylated hydroxytoluene (BHT) from MP Biomedicals (Santa Ana, CA, USA). Authentic reference standards were purchased from Fisher Scientific, Sigma-Aldrich, Alfa Aesar, Toronto Research Chemicals (Toronto, Ontario, Canada), Apollo Chemical (Burlington, NC, USA), and TCI Chemicals (Portland, OR, USA). These include β-carotene; lutein; lycopene; 4-hydroxybenzoic acid (4-HBA); 3,4-dihydroxybenzoic acid; 4-hydroxycinnamic acid; 3,4-dihydroxycinnamic acid; 4-hydroxy-3-methoxycinnamic acid; 3,4,5-trihydroxybenzoic acid; 4-hydroxyhippuric acid; dihydroresveratrol; kaempferol; catechin; epicatechin; gallocatechin; epigallocatechin; resveratrol 3-O-sulfate; 4-O-caffeoylquinic acid; 3-O-caffeoylquinic acid; 5-O-caffeoylquinic acid; epigallocatechin gallate; urolithin A; taxifolin; 3,4-dihydroxybenzaldehyde; 3-(4-hydroxy-3-methoxyphenyl)propionic acid; myricetin; isourolithin A; 3-(3,4-dihydroxyphenyl)propionic acid; dihydrocaffeic acid 3-O-sulfate; naringenin; ferulic acid 4-O-sulfate; benzoic acid 4-O-glucuronide; caffeic acid 4-O-glucuronide; caffeic acid 3-O-glucuronide; ferulic acid 4-O-glucuronide; dihydroferulic acid 4-O-glucuronide; isourolithin A 9-glucuronide; 3,4-dihydroxyphenylacetic acid; hesperetin 3-O-glucuronide; and ethyl gallate.
2.2 Study design and participants
The present study was a secondary analysis of breastfeeding women that were enrolled in a within-subject intervention pilot study (NCT03744429). This study was designed with the intent of leveraging results to design a more comprehensive intervention for evaluation of relationships between maternal dietary and non-dietary factors, HM bioactive composition, and infant developmental outcomes. Healthy women with obesity (BMI = 30–50 kg/m2) at 4 to 5 months postpartum who were exclusively breastfeeding were recruited from the Little Rock, Arkansas community between April 2019 and February 2020, as described previously (26). All procedures within the study were approved by the University of Arkansas for Medical Sciences Institutional Review Board (Protocol #228407). Participants underwent a written, informed consent process at enrollment. Enrollment criteria have been described previously (26). Ninety women were screened, 28 were eligible, and 16 (BMI = 30–50 kg/m2) were enrolled. Due to three participants not completing all study visits, 13 participants completed all study visits and were included for analysis. Maternal race and ethnicity, age, and infant sex were self-reported at screening. The study consisted of three visits: enrollment, 2 weeks, and 4 weeks following the introduction of the dietary intervention. The enrollment visit is considered pre-intervention and the 4-week time point is considered post-intervention.
2.3 3-day food records
Prior to initiation of the dietary intervention, participants recorded all food, beverages, supplements, and medications consumed over 3 days (two weekdays and one weekend day). For the entirety of the intervention, participants recorded all food, beverages, dietary supplements, medications, and any substitutions made to the meal plan. Dietary intake information was analyzed by trained staff with the Nutrition Data System for Research (NDSR, Nutrition Coordinating Center, University of Minnesota, MN, USA) software. Average daily intake at pre-intervention and during the intervention (average of wk. 1–wk. 4) for carotenoids, polyphenols and phytochemical-rich food groups, were estimated from NDSR diet records. Intake of food groups and the carotenoids β-cryptoxanthin, lutein+zeaxanthin, lycopene, α-carotene, and β-carotene were provided from NDSR. Polyphenol intake was estimated using a database developed from Phenol-Explorer (37) and polyphenol concentrations reported in published literature.
2.4 Dietary intervention
The intervention consisted of a Mediterranean-style diet pattern with macronutrient distribution (20–35% of calories from fat, 45–65% carbohydrates, 10–35% protein) and caloric intake that met recommendations from the Dietary Guidelines for Americans (DGA). Participants were provided with all lunches and dinners (fresh-packaged meals) weekly throughout the 4-week intervention by Trifecta Nutrition (Sacramento, CA, USA). Breakfast and snacks consisting of breakfast sandwiches, oatmeal, walnuts, granola bars, Greek yogurt, and fruits were provided by the research team. Additionally, participants were provided with Palermo extra virgin olive oil to add to meals and were instructed to buy 1% low fat milk to drink or add to fruit smoothies. Adherence to the dietary intervention was greater than 80%, as reported previously (26).
2.5 Human milk collection
Prior to each visit, mothers collected HM over a 24-h time period. Mothers were asked to collect milk from each feed with the option to feed their infant expressed milk or feed from one breast and pump from the other. If the latter option was selected, the pumped breast would be alternated at every feed time. Following collection, expressed milk was gently inverted and 4 ml was aliquoted into provided polypropylene tubes. Human milk was stored at 4°C until the full 24-h was completed. Pooled milk samples were aliquoted and stored at −80°C until further analysis.
2.6 Extraction and analysis of carotenoids from human milk by HPLC
Concentrations of carotenoids in human milk were determined by HPLC, as described previously (6, 38). Carotenoids were separated on a YMC C30 (2.0 × 150 mm) column (YMC Kyoto, Japan) with diode array detection. Quantification of carotenoids was accomplished with authentic carotenoid standards for β-carotene, lutein, and lycopene via calibration curves extracted at 450 nm. Identification of β-cryptoxanthin, α-carotene, and zeaxanthin was based on comparison of absorption spectra and elution profiles of previous results using similar C30 chromatography (5). Concentrations of β-cryptoxanthin and α-carotene were estimated using the calibration curve for β-carotene. Zeaxanthin content was estimated based on the calibration curve for lutein.
2.7 Extraction and analysis of polyphenol metabolites from human milk by UPLC-MS/MS
Extraction methodologies for polyphenol metabolites were adapted from Henning et al. (17). Aliquots of HM (250 μl) were extracted with 1 ml of acetonitrile:formic acid (98:2) three times. Dried extracts were reconstituted in 400 μl of water:formic acid (99.9:0.1), filtered on AcroPrep™ 96-well filter plates (1 ml, 0.45 μm Supor membrane, Cytiva, Marlborough, MA, USA), and analyzed by UPLC-MS/MS. Final concentrations were adjusted by recovery of the internal standard taxifolin, which was added to all milk samples as internal standard at the beginning of analysis. Phenolic compounds and metabolites were resolved with an Acquity UPLC BEH C18 column using a Waters Acquity Premier UPLC system equipped with a XEVO TQS-micro mass spectrometer (Waters, Milford, MA, USA) as previously described (39). Multiple reaction monitoring (MRM) was used to identify and quantify 35 individual phenolic and metabolite compounds (Supplementary Table S1). Validation parameters are reported in Supplementary Table S2.
2.8 Human milk oligosaccharide composition and maternal FUT2 phenotype
Concentrations of the 19 most abundant HMOs (3) were determined by HPLC, as previously described (27). Individual HMOs were separated on an amide-80 column (2 μm particle size, 2 mm ID, 15 cm length) with fluorescent detection. Final concentrations were adjusted by recovery of the internal standard raffinose, which was added to all milk samples as internal standard at the beginning of analysis. Maternal FUT2 secretor phenotype was determined by presence (secretor) or near-absence (non-secretor) of 2′-fucosyllactose (2’FL) in HM (<100 nmol/ml).
2.9 Statistical analysis
Using macronutrient (fat, protein, carbohydrates) content of 24-h pooled samples measured with a Miris HM Analyzer (Miris, Uppsala, Sweden), HM carotenoid concentrations were expressed on a lipid basis (nmol/g fat). Polyphenol metabolites (nmol/l) and HMOs (nmol/ml) were expressed on a volume basis. For statistical analyses, concentrations that were not detected (ND) and below the limit of detection (<LOD) were imputed as 1/10 and 1/5 of the LOD, respectively. Data in tables represents raw data, while data in figures represents data used for statistical analyses (i.e., with imputed values). Repeated measures ANOVA was used to determine the main effects of time of the dietary intervention (Pre vs. Wk4) and the time-by-secretor status (secretor vs. non-secretor) interaction for diet and HM bioactive composition. Due to skewness and non-normality of HM bioactives (carotenoids, HMOs, polyphenol metabolites), ANOVA models and post-hoc analyses were evaluated after rank-based transformation was carried out using the nparLD R package (version 2.2) (40). ANOVA models and post-hoc analyses (Tukey HSD) of diet composition were evaluated using the lmerTest (version 3.1.3) (41) and emmeans (version 1.10.0) (42) R packages. An exploratory analysis of associations between HM bioactives for secretors and non-secretors was carried out using repeated measures correlations using the rmcorr (version 0.6.0) (43) R package. p-values for the correlation analysis were FDR-adjusted. All statistical analyses were carried out using R statistical software (version 4.3.2) (44). Sensitivity analyses were carried out for measurements identified as extreme outliers (3 times above the upper quartile or 3 times below the lower quartile).
3 Results
3.1 Maternal baseline characteristics
Participants were on average 33 ± 4 years of age (secretors: 35 ± 4; non-secretors: 31 ± 3) and 77% of participants were of non-Hispanic, Caucasian descent (secretors: 67%; non-secretors: 86%; Table 1). All participants were with obesity at baseline (all participants mean body mass index: 36 ± 5 kg/m2; secretors: 35 ± 5 kg/m2; non-secretors: 37 ± 5 kg/m2).
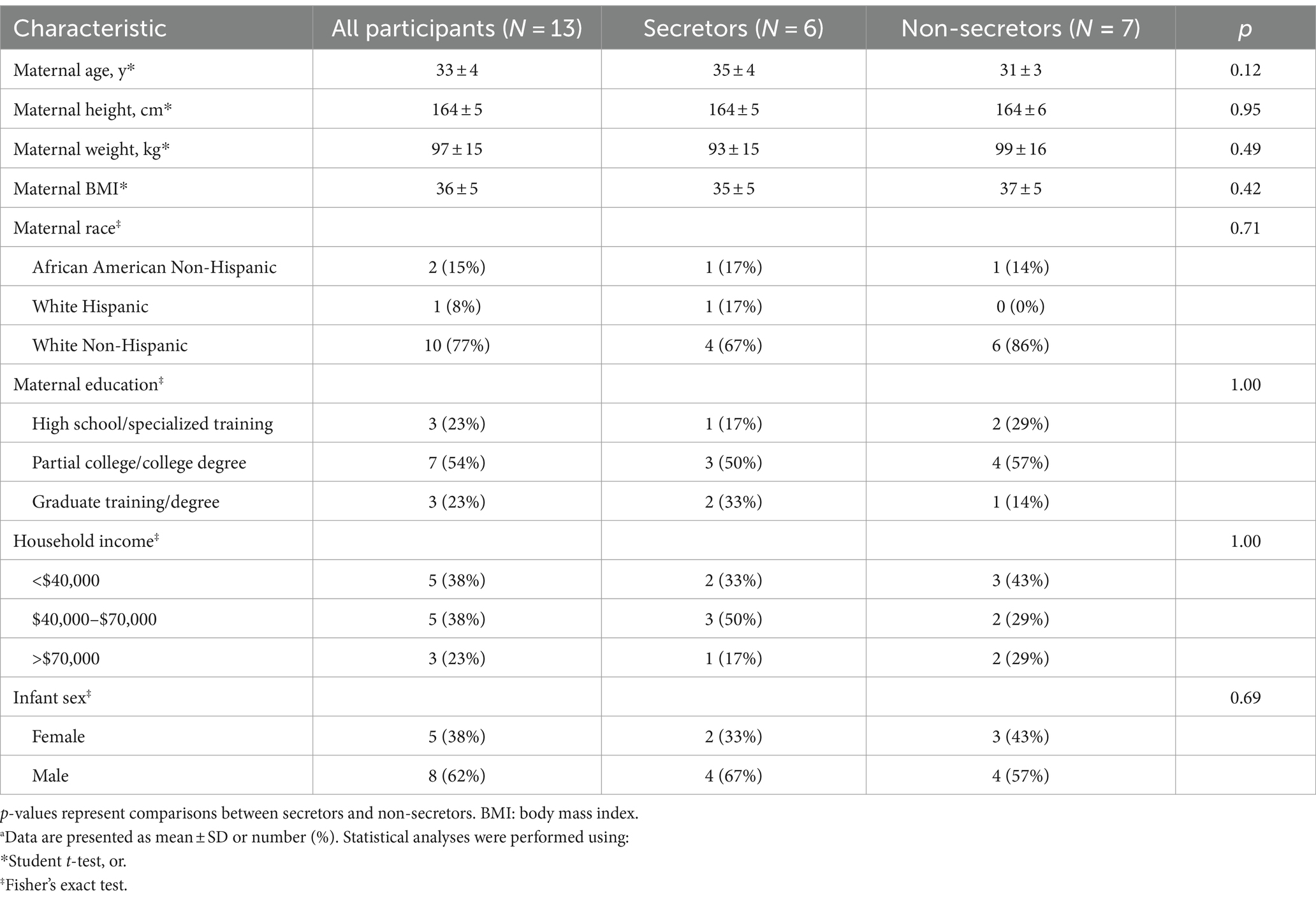
Table 1. Baseline characteristics of lactating women with obesity upon enrollment.a
3.2 Maternal dietary intake before and during the intervention
During the intervention (wk 1 to wk. 4), intake of whole grain (+75%, p < 0.001), fruit (+225%, p < 0.001), and vegetable (+136%, p < 0.001) intake significantly increased for all participants, regardless of secretor status (Table 2). With the exception of lycopene, intake of all other phytochemicals increased, including β-carotene (+841%, p < 0.001), β-cryptoxanthin (+232%, p < 0.001), and lutein+zeaxanthin (+290%, p < 0.001), and total polyphenols (+114%, p < 0.001). The observed decrease in lycopene intake (−58%, p < 0.001) was due to three participants that consumed greater amounts of lycopene derived from intake of tomato products at baseline. After exclusion of these participants, there was no significant change in lycopene intake (Pre-intervention: 3,097 ± 2,194 μg/d; Intervention: 2,431 ± 1,840 μg/d; p = 0.34).
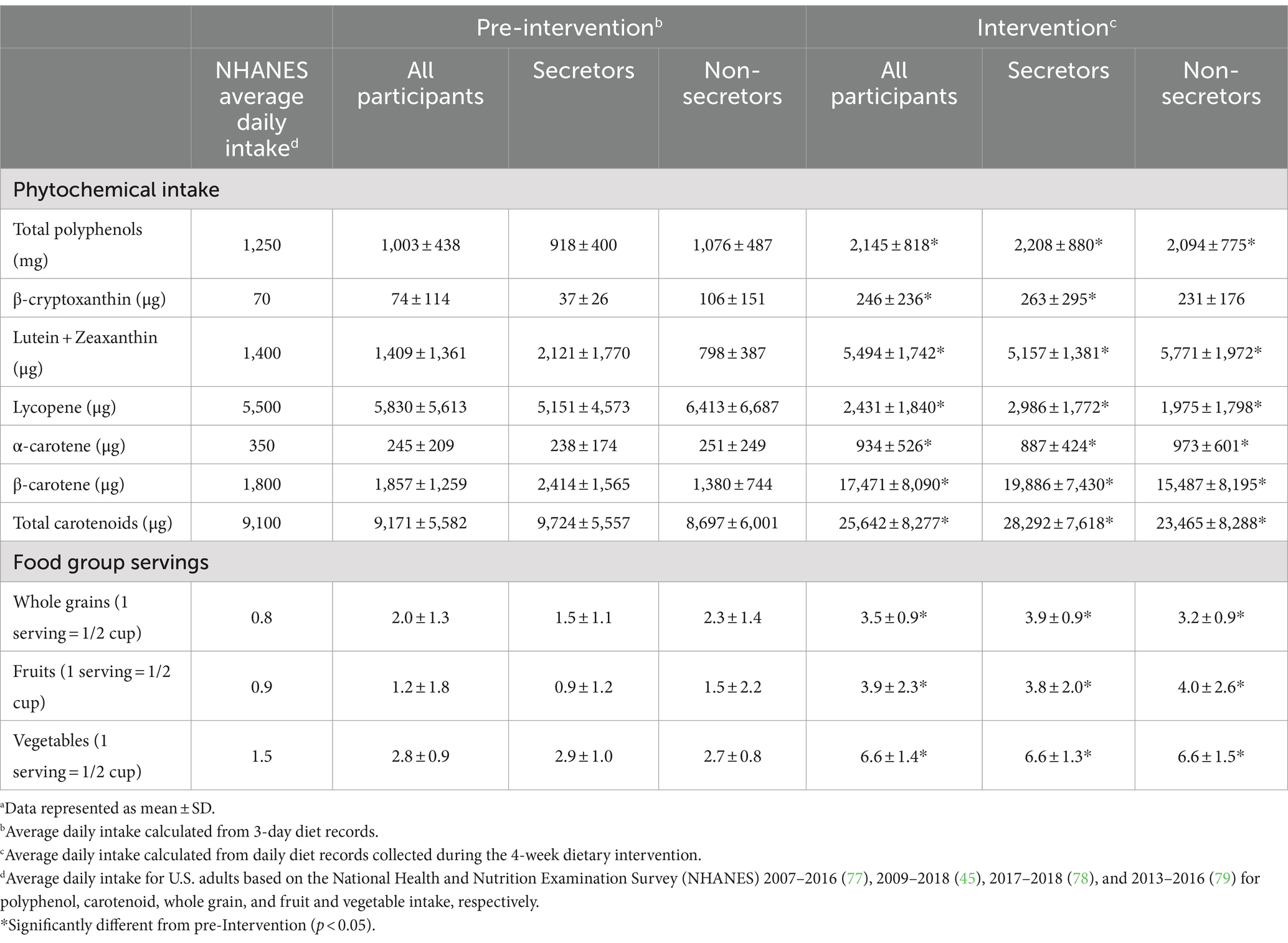
Table 2. Daily intake of polyphenols, carotenoids, and phytochemical-rich food groups before and during Mediterranean dietary intervention.a
3.3 Association of maternal diet and secretor status with human milk bioactive composition
3.3.1 Carotenoids
Concentrations of major carotenoid species in HM before and after the dietary intervention are summarized in Table 3. Because significant time-by-secretor status interactions were not observed, only main effects of time were analyzed. Despite significant increases in dietary carotenoid intake, significant changes in concentrations of carotenoids in HM were not observed. Analysis of dietary intake revealed wide variation in carotenoid intake during the intervention (Supplementary Table S3), despite consuming amounts greater than NHANES average daily intakes for U.S. adults (45) on at least 60% of intake days during the intervention. The largest fluctuations in daily intakes were observed for β-carotene (IQR: 2,767–30,342 μg/d) as a result of daily participant food choices.
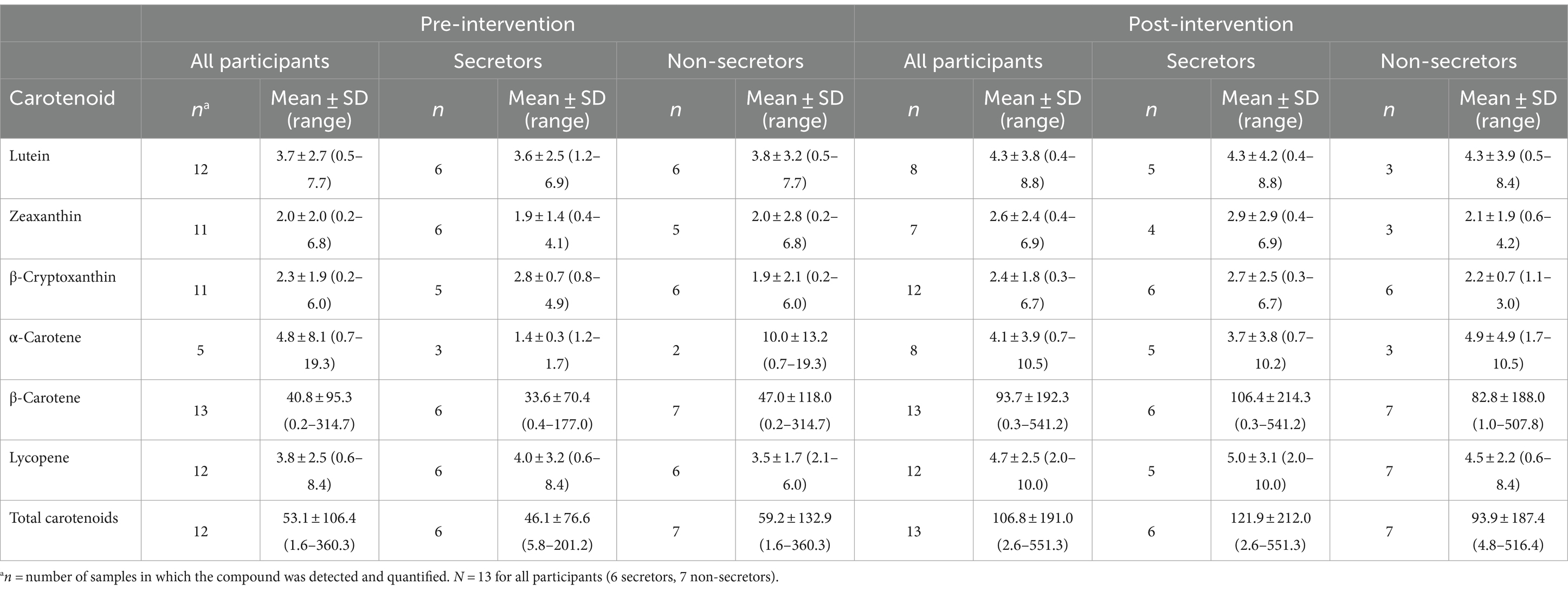
Table 3. Concentrations of carotenoids (nmol/g fat) in human milk samples of lactating women before and after 1 month Mediterranean-style dietary intervention.
Concentrations of α-carotene and β-carotene concentrations in HM have rarely been reported to exceed ~10 nmol/g fat, even with large supplementation doses of α-carotene and β-carotene (6, 19–21). Because extreme outliers were observed in α-carotene and β-carotene concentrations, a sensitivity analysis was carried out (Figure 1). No significant changes in the concentrations of α-carotene and β-carotene were observed when all data was included nor after exclusion of extreme outliers. A summary of ANOVA model p-values for analyses of carotenoids and sensitivity analysis for α-carotene and β-carotene are shown in Supplementary Table S4. The complete dataset for individual carotenoid concentrations in all HM samples is in Table A in Supplementary File S1.
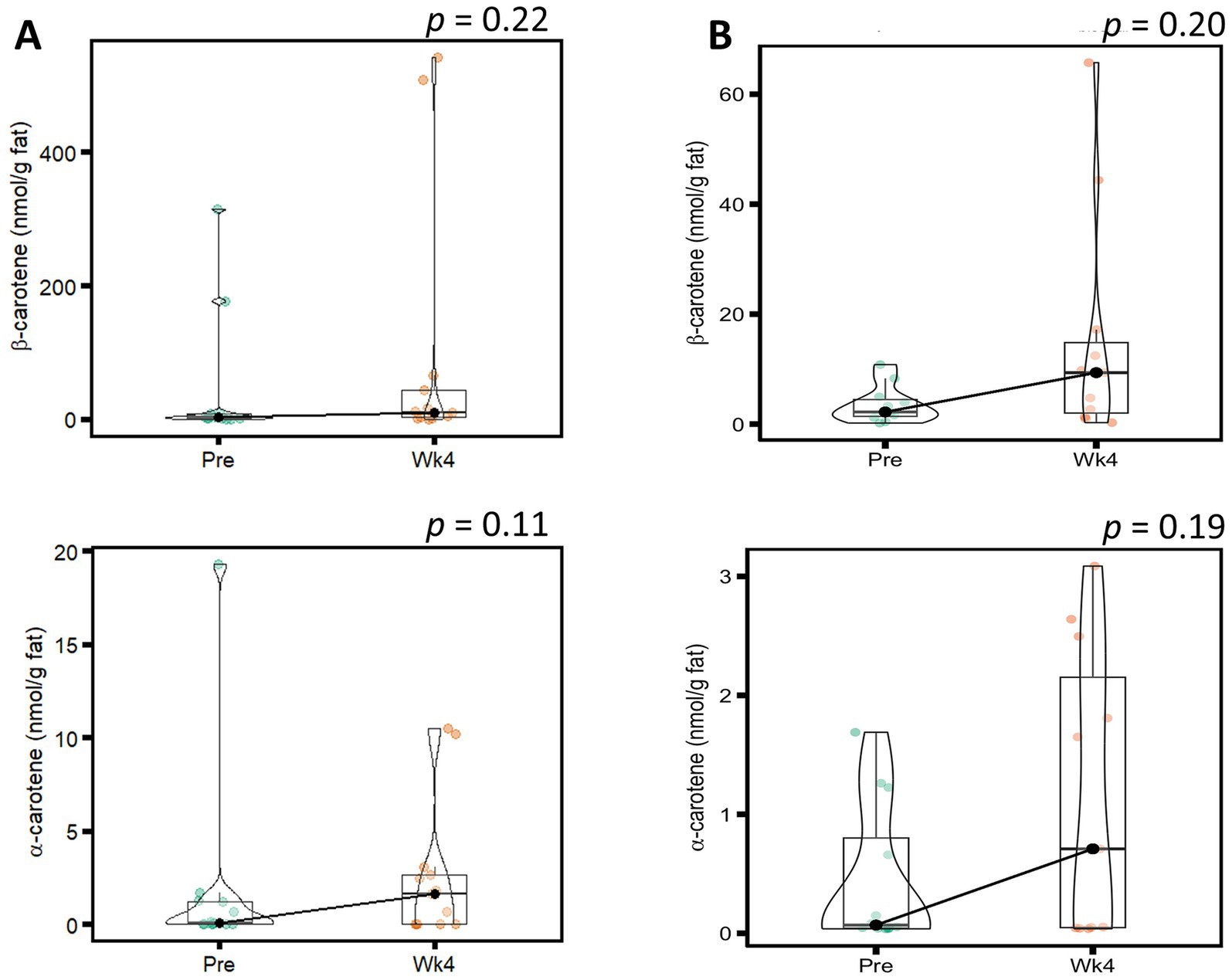
Figure 1. Violin plot with boxplot overlay of sensitivity analysis for ɑ-carotene and β-carotene (nmol/g fat) before (Pre) and after (Wk4) the intervention for (A) all participants and (B) after exclusion of extreme outliers. Statistics determined using 2-way repeated measures ANOVA models of time by secretor status following rank-based transformation of data. p-values shown represent the main effects of time in the ANOVA models since the interaction term was not significant.
3.3.2 Polyphenols
Concentrations of polyphenol metabolites in HM before and after the dietary intervention are summarized in Table 4. Unlike carotenoid intake, less variability in day-to-day polyphenol intake was observed across all intervention intake days (IQR: 1,249–2,642 mg/d, Supplementary Table S3). Time-by-secretor status interactions were observed for 4-HBA (p = 0.08) and ferulic acid (p = 0.02). Post-hoc analyses revealed that 4-HBA and ferulic acid significantly increased over the intervention time frame for secretors (4-HBA: +227%, p = 0.02; ferulic acid: +488%, p = 0.006), but not for non-secretors (4-HBA: +17%; ferulic acid: −7%). P-coumaric acid significantly increased over time (median at Pre-Intervention: 0 nmoL/L; median at Post-Intervention: 2.58 nmoL/L; p = 0.02) with no time-by-secretor interaction. A summary of ANOVA model p-values for analyses of polyphenol metabolites are shown in Supplementary Table S4. The complete dataset for individual polyphenol metabolite concentrations in all HM samples is in Table B in Supplementary File S1.
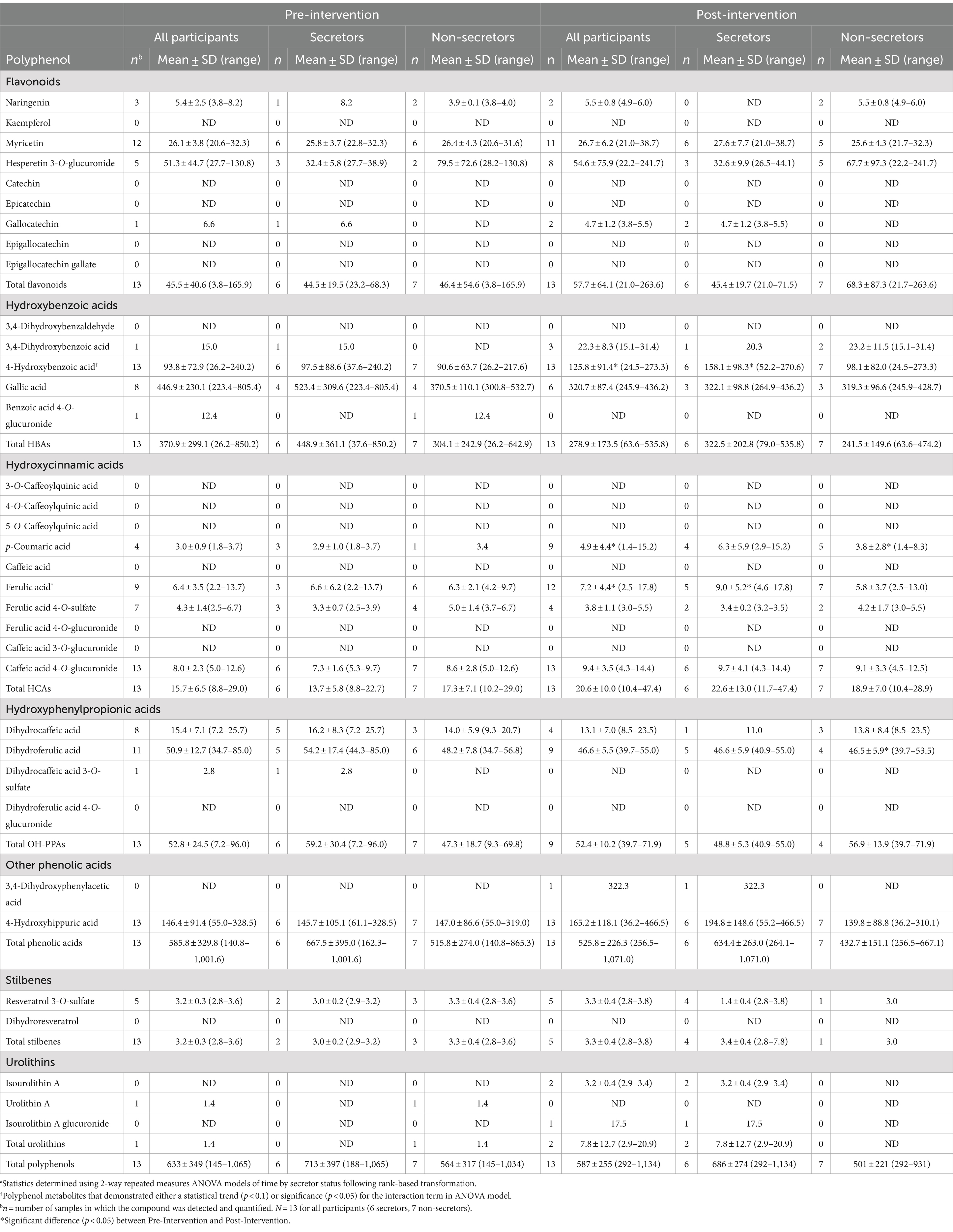
Table 4. Concentrations of polyphenol metabolites (nmol/l) in human milk samples of lactating women before and after 1 month Mediterranean-style dietary intervention.a
3.3.3 Human milk oligosaccharides
Concentrations of HMOs before and after the dietary intervention are summarized in Table 5 and were reported previously without stratification by secretor status (26). A significant time-by-secretor status interaction was observed for lacto-N-tetraose (LNT), lacto-N-fucopentaose II (LNFP II), difucosyllacto-N-tetraose (DFLNT), and disialyllacto-N-tetraose (DSLNT). Post-hoc analyses demonstrated a significant increase in concentrations of DSLNT over the intervention timeframe for secretors (+38%, p = 0.0008) and decreases in concentrations of LNT (−15%, p < 0.001), LNFP II (−20%, p = 0.008), and DFLNT (−96%, p < 0.001) for non-secretors. A summary of ANOVA model p-values for analysis of HMOs are shown in Supplementary Table S4.
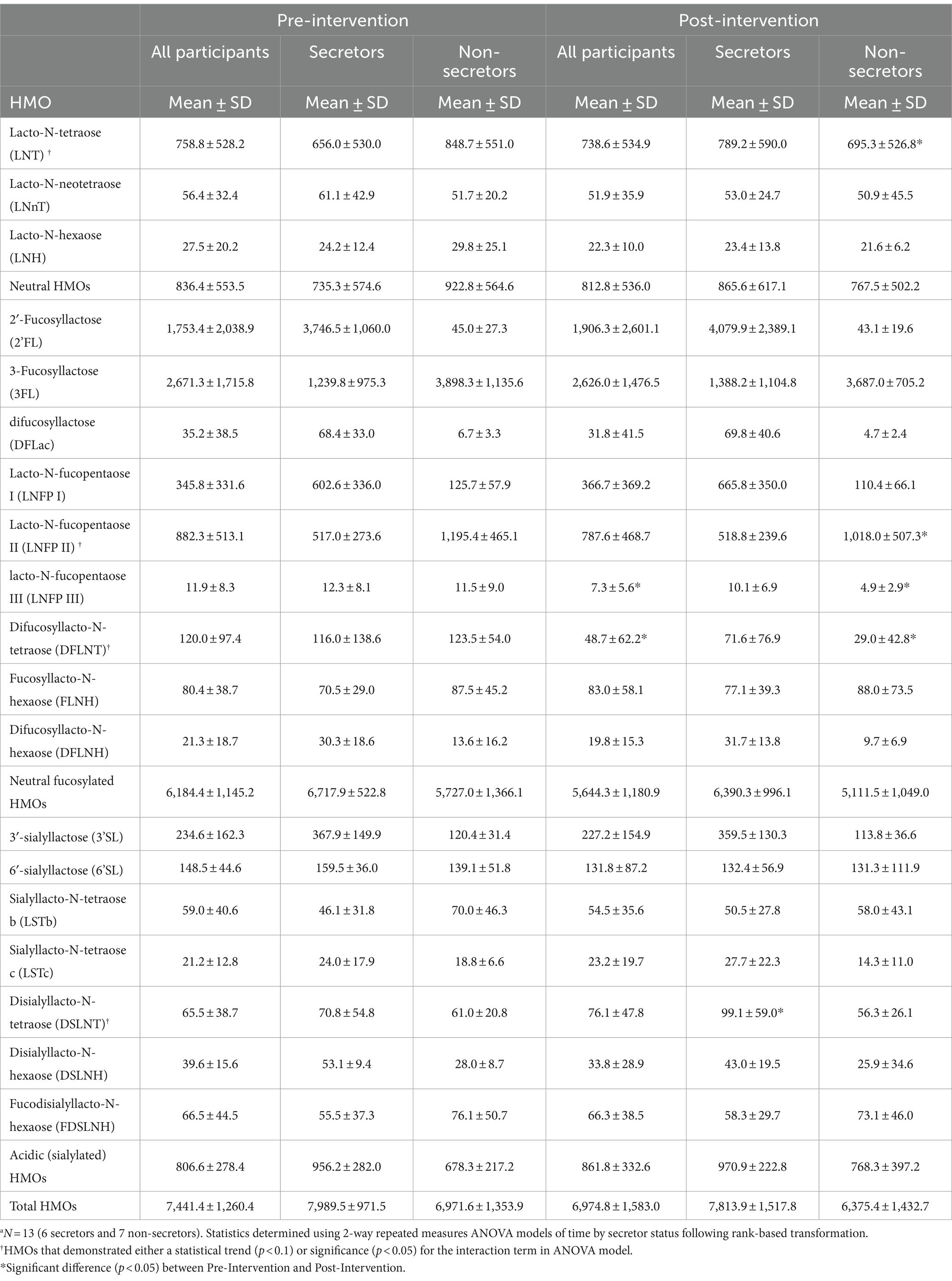
Table 5. Concentrations of oligosaccharides (HMOs, nmol/ml) in human milk samples of lactating women before and after 1 month Mediterranean-style dietary intervention.a
3.4 Correlations between human milk bioactives depend on maternal diet and secretor status, an exploratory analysis
An exploratory correlation analysis was carried out between 4-HBA and ferulic acid with LNT, LNFP II, DFLNT, and DSLNT (Figure 2) due to the overlap in maternal factors associated with profiles of these bioactives. For secretors, 4-HBA positively correlated with LNT (rrm = 0.82, p = 0.06) and DSLNT (rrm = 0.90, p = 0.03), and ferulic acid with LNT (rrm = 0.87, p = 0.04) and DSLNT (rrm = 0.90, p = 0.03). The only negative correlation for secretors was between 4-HBA and DFLNT (rrm = −0.94, p = 0.02). 4-HBA and ferulic acid were not significantly correlated with LNT, LNFP II, DFLNT, and DSLNT for non-secretors.
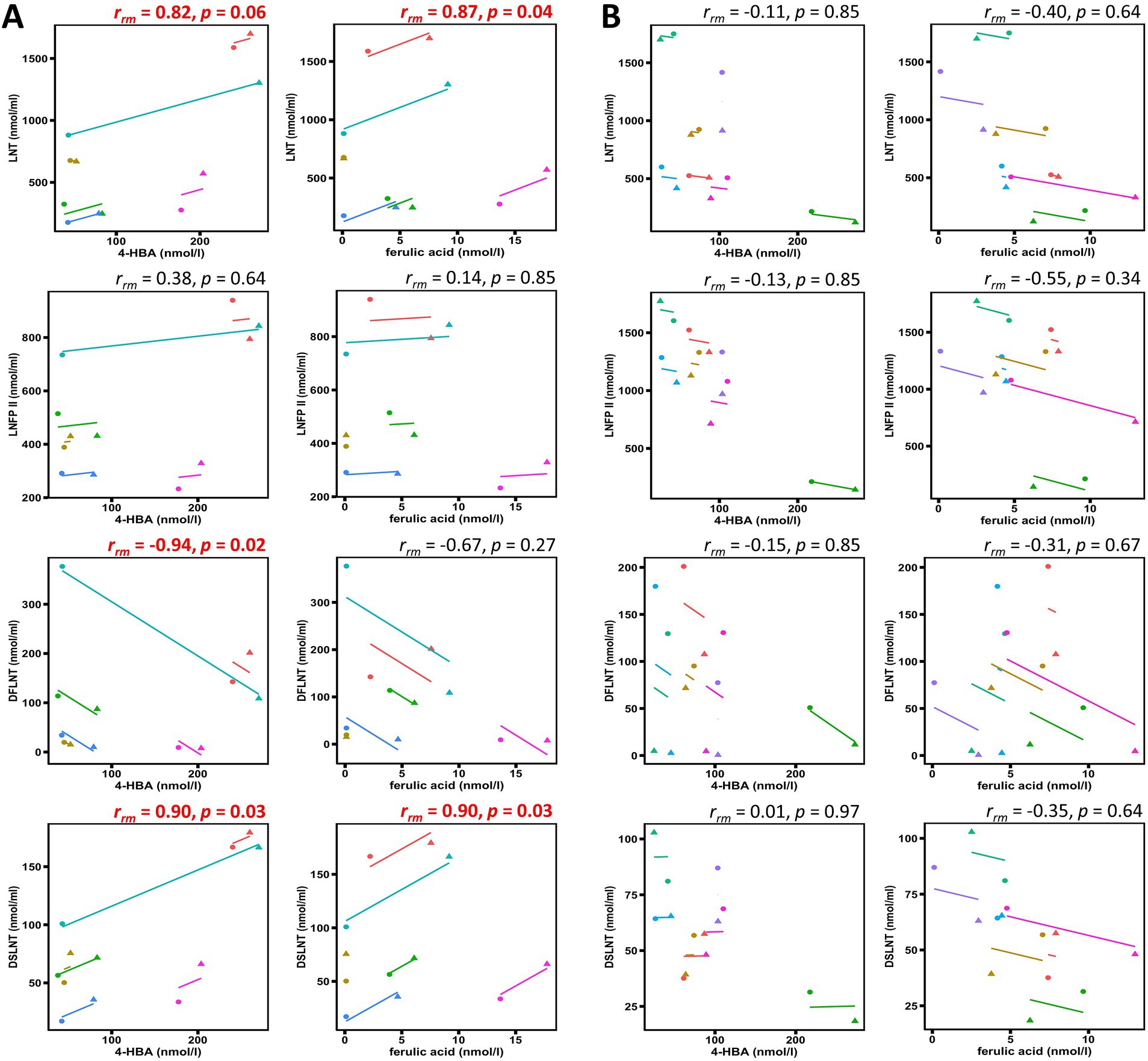
Figure 2. Scatterplots showing repeated measures correlations between human milk oligosaccharides and polyphenol metabolites. Correlation coefficients and p-values shown demonstrate within-participant associations amongst human milk bioactive components for (A) secretors and (B) non-secretors. Each color represents pre-intervention (●) and post-intervention (▲) measurements of different participants. The p-values shown are FDR-adjusted and correlations demonstrating a statistical trend (p < 0.1) or significance (p < 0.05) are highlighted in red. 4-HBA, 4-hydroxybenzoic acid; LNT, lacto-N-tetraose; LNFP II, lacto-N-fucopentaose II; DFLNT, difucosyllacto-N-tetraose; DSLNT, disialyllacto-N-tetraose.
4 Discussion
There is growing evidence that exposures to bioactives such as carotenoids, polyphenols, and oligosaccharides from HM are associated with gut development and/or neurodevelopment in breastfed infants (9–11, 13, 17, 18, 46). Polyphenols are unique amongst these bioactives since broad exposures occur only through HM during the first 6 months of life due to low levels in infant formula (8). Substantial variability in composition of HM carotenoids, polyphenol metabolites, and oligosaccharides exists between women (5, 7, 17, 18, 25, 28), highlighting the need to understand the impact of maternal dietary and non-dietary factors on profiles of these HM bioactives. In this study, we explored relationships between maternal diet and secretor status on composition of HM carotenoids, polyphenol metabolites, and oligosaccharides. We report for the first time evidence to suggest that dietary modulation of select oligosaccharides and polyphenol metabolites in HM may be associated with maternal secretor status. We also observed that correlations between select polyphenol metabolites (4-HBA, ferulic acid) and HMOs (LNT, DFLNT, DSLNT) differed depending on maternal secretor status. These findings are suggestive of a link between oligosaccharide and polyphenol metabolite profiles in HM that is driven by both dietary and non-dietary factors.
4.1 Maternal diet and carotenoid profiles of human milk
Increasing maternal carotenoid intake through supplementation and single dietary components has been demonstrated to increase carotenoid concentrations in HM. (19–22) The present study expands on these findings by evaluating HM carotenoid concentrations within the context of a broad change in maternal dietary pattern consistent with the 2020 DGA (47) executed in a manner reflective of normal daily dietary intake compared to highly controlled dietary interventions commonly used. Although average daily intakes of β-cryptoxanthin, lutein+zeaxanthin, α-carotene, and β-carotene were consistently greater than average intakes for U.S. adults (45), we did not observe significant changes in HM carotenoid concentrations over the intervention timeframe. Previous reports have demonstrated that β-carotene supplementation (30 mg/d) (19) and dietary sources of carotenoids, such as orange sweet potatoes (12 mg β-carotene/d) and tangerines (5.3 mg β-cryptoxanthin/d) (22) increase concentrations of these carotenoids in HM after 3–4 weeks. In the present study, carotenoid intake was lower than these previous reports, with β-carotene intake reaching 12 mg on less than 50% of intake days across all participants during the intervention. Therefore, it is likely that daily fluctuations in carotenoid intake resulted in dietary intakes of these experimental patterns not being sufficient to elicit a more significant response in HM carotenoid concentrations. Establishing a direct link between maternal dietary patterns and HM carotenoid concentrations will require that future studies utilize a more targeted approach in designing meal plans with more consistent carotenoid levels within the DGA dietary patterns. Additionally, future studies utilizing these dietary patterns should take into consideration dietary factors, such as co-consumption of lipids, that can modify absorption of carotenoids (22, 48). It should also be noted that carotenoid concentrations decrease over time during lactation (6, 49), highlighting the need for randomized controlled trials (RCTs) with multiple longitudinal measurements to capture any modulation of these temporal changes.
4.2 Maternal diet-secretor status interaction and human milk bioactive composition
Appearance of polyphenol metabolites in HM occurs as a result of metabolism and absorption of dietary polyphenols. Various host-related factors are known to modify polyphenol bioavailability, including health status (50), gut microbiome composition (51), and genetics (52). Despite this, the only factor that has been identified to increase polyphenol concentrations in HM is maternal dietary polyphenol intake (7, 17, 18, 23). Previous reports have linked maternal dietary polyphenol intake derived from single dietary components to HM through pharmacokinetic studies (7, 23, 33) and short duration interventions (≤14 days) (17, 53). Maternal dietary polyphenol intake is associated with increased HM polyphenol concentrations, including isoflavones (53), urolithins (17), epicatechin metabolites (7), and quercetin (23). Our study expands on these findings by evaluating changes in HM polyphenol metabolite concentrations within the context of a broad change in maternal dietary patterns (i.e., multiple and varying sources of dietary polyphenols) that resulted in a 2X increase of polyphenol intake. In the present study, we demonstrated that this dietary pattern increases concentrations of the polyphenol metabolites 4-HBA, ferulic acid, and p-coumaric acid in HM by ~1.5X. In previous interventions, intake of 470–480 mg/d of polyphenols resulted in greater HM polyphenol concentrations (17, 18). Unlike dietary carotenoid intake, participants’ daily polyphenol intake was greater than 480 mg on >95% of intervention days. To our knowledge, this study is the first to show that a broad dietary change consistent with the 2020 DGA can modify polyphenol metabolite profiles of HM.
Previous reports demonstrate substantial variability in concentrations of polyphenols in HM, even in women consuming similar sources and amounts of dietary polyphenols (17, 18, 33). This study is the first to explore whether dietary modulation of HM polyphenol concentrations depends on maternal secretor status during a dietary intervention. Although HMO profiles depend on maternal secretor status (4, 54), there are no previous reports describing associations between secretor status and HM polyphenol metabolite profiles. In the present study, we demonstrate, for the first time, that higher dietary intake of polyphenols was differentially associated with 4-HBA and ferulic acid concentrations in HM depending on maternal secretor status. This suggests that secretor status may explain, at least in part, the variability in HM polyphenol profiles in women with similar polyphenol intake. Increased concentrations of 4-HBA and ferulic acid were observed for secretors, but not non-secretors, over the intervention time frame. The major dietary source of ferulic acid is whole grain intake (55), with >90% bound to non-starch polysaccharides (56), allowing release and absorption after microbial fermentation in the lower gastrointestinal tract (57). Appearance of 4-HBA in circulation, and subsequently in HM, occurs from microbial catabolism of anthocyanins (58, 59), other flavonoids (60), and phenolic acids including ferulic acid (61) and p-coumaric acid (58). Therefore, the diet-by-secretor status interaction that we observed for ferulic acid and 4-HBA suggests that secretor status may influence microbial polyphenol metabolism. Although maternal gut and milk microbiome composition was not evaluated in the present study, others have reported differences in both gut (62) and milk microbiome (34) composition between secretors and non-secretors. However, future studies will be needed to elucidate whether differences in gut or milk microbiome composition in secretors and non-secretors acts as a mediator between maternal dietary polyphenol intake and differential responses in HM polyphenol metabolite profiles.
Maternal secretor status is a well-established factor that drives differences in HMO profiles, with secretors having greater concentrations of α1-2 fucosylated HMOs (4, 63–65). There is also emerging clinical evidence demonstrating a direct link between maternal diet and HMO profiles (26, 27). In this study, we are demonstrating that the response of several HMOs (LNT, LNFP II, DFLNT, and DSLNT) to dietary modulation is differentially associated with maternal secretor status. Interestingly, the decrease in LNFP II and DFLNT, which our group identified in the original analysis (26), specifically occurred in non-secretors. We also observed a decrease in LNT and an increase in DSLNT in non-secretors and secretors, respectively. Our findings are consistent with a recent cross-sectional study suggesting that the correlation of maternal polyphenol intake with HMO profiles depends on maternal secretor status (25). Due to the focus on women with obesity and short duration of this pilot study, it will be important to include women of all weight statuses and longitudinal measurements over the course of lactation to elucidate factors driving the observed changes in HMO profiles.
To our knowledge, this pilot study represents the first example of a dataset that allows an integrated evaluation of interactions between maternal diet, secretor status, HMOs, and polyphenol metabolites in HM. The results of our correlation analysis suggest that relationships between oligosaccharides and polyphenols in HM may depend on maternal secretor status. We have shown that correlations between specific polyphenol metabolites (4-HBA, ferulic acid) and oligosaccharides (LNT, DFLNT, DSLNT) in HM were associated with maternal secretor status during a dietary intervention that increases polyphenol intake. Specifically, we observed that polyphenol metabolites and HMOs are significantly correlated in secretors, but not in non-secretors. This is suggestive of relationships between maternal secretor status and the dietary modulation of oligosaccharides and polyphenols in HM and is consistent with a previous report demonstrating that maternal polyphenol intake is a significant predictor of HMO profiles in secretors (25). Because a primary source of polyphenol metabolites in circulation, and subsequently in HM, is microbial metabolism of dietary polyphenols (35, 36), it is possible that the relationships between maternal diet, secretor status, and oligosaccharides and polyphenol metabolites in HM are mediated by the gut microbiota. Although we did not evaluate maternal gut microbiota in the present study, it has been demonstrated that intake of probiotics (66) modifies HMO profiles, suggesting a link between modulation of gut microbiome composition and HMO profiles. Additionally, dietary polyphenols have been associated with “prebiotic”-like effects leading to modulation of gut microbiome composition (67, 68). Therefore, future studies are warranted to explore whether polyphenol intake may modify HMO profiles via modulation of the gut microbiota as well as a combination of microbial and host metabolism. It is also possible that the interactions observed in the present study between maternal diet, secretor status, and HM bioactives may be mediated by HM microbiota. Interestingly, maternal secretor status (34) and diet (24) are associated with modulation of Bifidobacterium spp. in HM, with increases reported in secretors and with greater polyphenol intake. Certain species and strains of Bifidobacterium, some of which may be present in HM (69, 70), can assimilate complex fucosylated HMOs (71–73). It has been suggested that utilization of fucosylated HMOs is dependent on ATP-binding cassette (ABC) transporters (72), which can also transport polyphenols (74). The strategy for utilization of sialylated HMOs such as DSLNT by Bifidobacterium likely involves extracellular release of sialic acid (75), which may subsequently become available to other HM microbiota or for utilization to produce sialylated HMOs. Thus, the relationship between maternal secretor status, diet, and oligosaccharides and polyphenol metabolites in HM may reflect the interplay between available substrates and Bifidobacterium composition and abundance. However, future studies are needed to evaluate whether factors that modify Bifidobacterium composition and abundance (e.g., diet, secretor status) in HM influence the interplay between substrates such as HMOs, polyphenols, and monosaccharides derived from HMO breakdown.
4.3 Future research directions
The results of this study suggest that maternal FUT2 secretor status may be associated with differential responses in HM bioactive composition (HMOs, polyphenols) within the context of a healthy dietary pattern rich in polyphenols. Growing evidence suggests that these same dietary substrates may modulate the gut-brain axis in breastfed infants (9–12, 17, 76). However, several critical gaps exist limiting the translation of these results to developmental impacts for breastfed infants. Due to the complexity of factors likely driving profiles of HMOs and polyphenols, both longitudinal cohort and dietary intervention studies are required, and these studies should include a deeper phenotypic characterization of the mother-infant dyad throughout pregnancy and lactation. To understand potential interactions between maternal dietary and non-dietary factors on composition of HMOs and polyphenols, the following should be considered through application of systematic and integrated methodologies: (1) broader characterization of maternal diet composition, especially during critical stages of development such as lactation, (2) genetics (e.g., secretor status, phase II host metabolism enzymes), (3) health status (e.g., weight status, cardiometabolic biomarkers), and (4) gut microbiome composition. To evaluate the extent to which these HM bioactives may influence the gut-brain axis of breastfed infants, similar considerations are needed across infancy, childhood, and beyond, with the addition of the following: (1) quantification of breastfed infant intakes of both HMOs and individual polyphenols or metabolites through human milk and complimentary feeding, (2) omics data relevant to gut-brain axis development (e.g., short-chain fatty acids, gut microbiome, tryptophan metabolites), and (3) clinical outcomes relevant to cognitive development and function (e.g., cognitive assessment, EEG, MRI). Working to address these specific knowledge gaps will advance our understanding of human milk bioactives and their potential role in development of the gut-brain axis in breastfed infants.
4.4 Strengths and limitations
The results of this pilot study provide foundational information needed to design and conduct future studies exploring factors that influence HMO and polyphenol metabolite composition of HM and subsequent impacts on infant development. However, the study is limited by sample size highlighting a clear need to confirm the findings of our pilot study in a RCT, which will also be critical in identifying how dietary interventions can be leveraged for modifying composition of HM bioactives in a manner that promotes optimal infant development. A second limitation of the present study is the storage of HM samples during the 24-h collection period. The effect of participants storing HM samples at 4°C during this time period on stability of polyphenol metabolites was not investigated. A third limitation is the lack of a normal weight group since obesity is associated with modified HMO profiles (3) and polyphenol metabolism and absorption (50). There were several significant strengths to this study: (1) dietary modulation of multiple HM bioactives within the context of a dietary pattern consistent with the 2020 DGA recommendations (47); (2) simultaneous analyses of HMOs and polyphenol metabolites in HM, allowing for evaluation of associations between these two classes of HM bioactives for the first time; (3) inclusion of diet and a non-dietary factor (maternal secretor status) in an evaluation of polyphenol metabolites in HM; and (4) significant interactions between maternal diet and secretor status associated with differential profiles of HMOs and microbial-derived polyphenol metabolites in HM. Future studies need to incorporate a more integrated approach in RCTs involving a larger sample size that can encompass multiple non-dietary factors (e.g., weight status/BMI, secretor status, microbiome composition), longer intervention time frame, and ability to link maternal diet to changes in HM bioactive composition and infant developmental outcomes.
5 Conclusion
This study is the first to demonstrate that the impact of maternal diet on composition of HMOs and polyphenol metabolites in HM may depend on maternal secretor status. It is also the first to suggest a link between maternal diet, secretor status, HMOs, and microbial metabolites of dietary polyphenols. This investigation allows for a better understanding of factors driving HM bioactive composition and establishes a need for further studies, which could be leveraged for optimizing infant health and development.
Data availability statement
The original contributions presented in the study are included in the article/Supplementary material, further inquiries can be directed to the corresponding authors.
Ethics statement
The studies involving humans were approved by University of Arkansas for Medical Sciences Institutional Review Board. The studies were conducted in accordance with the local legislation and institutional requirements. The participants provided their written informed consent to participate in this study.
Author contributions
CF: Data curation, Formal analysis, Investigation, Writing – original draft, Writing – review & editing. MC: Data curation, Investigation, Writing – original draft, Writing – review & editing. AH: Data curation, Investigation, Writing – review & editing. CS: Conceptualization, Funding acquisition, Investigation, Project administration, Writing – review & editing. DW: Formal analysis, Writing – review & editing. LB: Conceptualization, Data curation, Investigation, Writing – review & editing. AM: Investigation, Writing – review & editing. AA: Conceptualization, Funding acquisition, Investigation, Project administration, Writing – review & editing. MF: Conceptualization, Funding acquisition, Investigation, Project administration, Resources, Supervision, Writing – review & editing.
Funding
The author(s) declare that financial support was received for the research, authorship, and/or publication of this article. The United States Department of Agriculture Agricultural Research Service (USDA-ARS) funded the Arkansas Children’s Nutrition Center through project plans #6026-51000-010-05S and #6026-51000-012-06S. Arkansas Children’s Research Institute/Arkansas Biosciences Institute provided funding through grant GR037121. The National Institutes of Health/National Institute of Diabetes and Digestive Kidney Diseases partially supported CS and AA through grant R01DK107516.
Acknowledgments
We thank the participants and the clinical research team at ACNC for their dedication and hard work in producing and collecting the samples and data presented in this manuscript.
Conflict of interest
The authors declare that the research was conducted in the absence of any commercial or financial relationships that could be construed as a potential conflict of interest.
Publisher’s note
All claims expressed in this article are solely those of the authors and do not necessarily represent those of their affiliated organizations, or those of the publisher, the editors and the reviewers. Any product that may be evaluated in this article, or claim that may be made by its manufacturer, is not guaranteed or endorsed by the publisher.
Supplementary material
The Supplementary material for this article can be found online at: https://www.frontiersin.org/articles/10.3389/fnut.2024.1463969/full#supplementary-material
References
1. World Health Organization (2024). Breastfeeding. Available at: https://www.who.int/health-topics/breastfeeding#tab=tab_2 (Accessed March 01, 2024).
2. Meek, JY, and Noble, L. Section on breastfeeding. Policy statement: breastfeeding and the use of human milk. Pediatrics. (2022) 150:e2022057988. doi: 10.1542/peds.2022-057988
3. Saben, JL, Sims, CR, Abraham, A, Bode, L, and Andres, A. Human milk oligosaccharide concentrations and infant intakes are associated with maternal overweight and obesity and predict infant growth. Nutrients. (2021) 13:446. doi: 10.3390/nu13020446
4. Kunz, C, Meyer, C, Collado, MC, Geiger, L, García-Mantrana, I, Bertua-Ríos, B, et al. Influence of gestational age, secretor, and Lewis blood group status on the oligosaccharide content of human Milk. J Pediatr Gastroenterol Nutr. (2017) 64:789–98. doi: 10.1097/MPG.0000000000001402
5. Lipkie, TE, Morrow, AL, Jouni, ZE, McMahon, RJ, and Ferruzzi, MG. Longitudinal survey of carotenoids in human milk from urban cohorts in China, Mexico, and the USA. PLoS One. (2015) 10:e0127729. doi: 10.1371/journal.pone.0127729
6. Song, BJ, Jouni, ZE, and Ferruzzi, MG. Assessment of phytochemical content in human milk during different stages of lactation. Nutrition. (2013) 29:195–202. doi: 10.1016/j.nut.2012.07.015
7. Khymenets, O, Rabassa, M, Rodríguez-Palmero, M, Rivero-Urgell, M, Urpi-Sarda, M, Tulipani, S, et al. Dietary epicatechin is available to breastfed infants through human breast milk in the form of host and microbial metabolites. J Agric Food Chem. (2016) 64:5354–60. doi: 10.1021/acs.jafc.6b01947
8. Sánchez-Hernández, S, Esteban-Muñoz, A, Samaniego-Sánchez, C, Giménez-Martínez, R, Miralles, B, and Olalla-Herrera, M. Study of the phenolic compound profile and antioxidant activity of human milk from Spanish women at different stages of lactation: a comparison with infant formulas. Food Res Int. (2021) 141:110149. doi: 10.1016/j.foodres.2021.110149
9. Bosheva, M, Tokodi, I, Krasnow, A, Pedersen, HK, Lukjancenko, O, Eklund, AC, et al. Infant formula with a specific blend of five human milk oligosaccharides drives the gut microbiota development and improves gut maturation markers: a randomized controlled trial. Front Nutr. (2022) 9:920362. doi: 10.3389/fnut.2022.920362
10. Berger, PK, Plows, JF, Jones, RB, Alderete, TL, Yonemitsu, C, Poulsen, M, et al. Human milk oligosaccharide 2'-Fucosyllactose links feedings at 1 month to cognitive development at 24 months in infants of normal and overweight mothers. PLoS One. (2020) 15:e0228323. doi: 10.1371/journal.pone.0228323
11. Oliveros, E, Martín, M, Torres-Espínola, F, Segura-Moreno, M, Ramírez, M, Santos, A, et al. Human milk levels of 2-fucosyllactose and 6-sialyllactose are positively associated with infant neurodevelopment and are not impacted by maternal BMI or diabetic status. J Nutr Food Sci. (2021) 4:100024
12. Ding, J, Ouyang, R, Zheng, S, Wang, Y, Huang, Y, Ma, X, et al. Effect of breastmilk microbiota and sialylated oligosaccharides on the colonization of infant gut microbial community and fecal metabolome. Meta. (2022) 12:1136. doi: 10.3390/metabo12111136
13. Zielinska, MA, Hamulka, J, Grabowicz-Chądrzyńska, I, Bryś, J, and Wesolowska, A. Association between breastmilk Lc Pufa, carotenoids and psychomotor development of exclusively breastfed infants. Int J Environ Res Public Health. (2019) 16:1144. doi: 10.3390/ijerph16071144
14. Cheatham, CL, and Sheppard, KW. Synergistic effects of human milk nutrients in the support of infant recognition memory: an observational study. Nutrients. (2015) 7:9079–95. doi: 10.3390/nu7115452
15. Bone, RA, Landrum, JT, Fernandez, L, and Tarsis, SL. Analysis of the macular pigment by HPLC: retinal distribution and age study. Invest Ophthalmol Vis Sci. (1988) 29:843–9.
16. Vishwanathan, R, Kuchan, MJ, Sen, S, and Johnson, EJ. Lutein and preterm infants with decreased concentrations of brain carotenoids. J Pediatr Gastroenterol Nutr. (2014) 59:659–65. doi: 10.1097/MPG.0000000000000389
17. Henning, SM, Yang, J, Lee, RP, Huang, J, Thames, G, Korn, M, et al. Pomegranate juice alters the microbiota in breast milk and infant stool: a pilot study. Food Funct. (2022) 13:5680–9. doi: 10.1039/D2FO00280A
18. Cortés-Martín, A, García-Villalba, R, García-Mantrana, I, Rodríguez-Varela, A, Romo-Vaquero, M, Collado, MC, et al. Urolithins in human breast milk after walnut intake and kinetics of gordonibacter colonization in newly born: the role of mothers' urolithin metabotypes. J Agric Food Chem. (2020) 68:12606–16. doi: 10.1021/acs.jafc.0c04821
19. Canfield, LM, Giuliano, AR, Neilson, EM, Blashil, BM, Graver, EJ, and Yap, HH. Kinetics of the response of milk and serum beta-carotene to daily beta-carotene supplementation in healthy. Lactating Women Am J Clin Nutr. (1998) 67:276–83. doi: 10.1093/ajcn/67.2.276
20. Canfield, LM, Giuliano, AR, Neilson, EM, Yap, HH, Graver, EJ, Cui, HA, et al. Beta-carotene in breast milk and serum is increased after a single beta-carotene dose. Am J Clin Nutr. (1997) 66:52–61. doi: 10.1093/ajcn/66.1.52
21. Canfield, LM, Kaminsky, RG, Taren, DL, Shaw, E, and Sander, JK. Red palm oil in the maternal diet increases provitamin a carotenoids in breastmilk and serum of the mother-infant dyad. Eur J Nutr. (2001) 40:30–8. doi: 10.1007/PL00007383
22. Turner, T, Burri, BJ, Jamil, KM, and Jamil, M. The effects of daily consumption of Β-cryptoxanthin-rich tangerines and Β-carotene-rich sweet potatoes on vitamin a and carotenoid concentrations in plasma and breast milk of Bangladeshi women with low vitamin a status in a randomized controlled trial. Am J Clin Nutr. (2013) 98:1200–8. doi: 10.3945/ajcn.113.058180
23. Romaszko, E, Wiczkowski, W, Romaszko, J, Honke, J, and Piskula, MK. Exposure of breastfed infants to quercetin after consumption of a single meal rich in quercetin by their mothers. Mol Nutr Food Res. (2014) 58:221–8. doi: 10.1002/mnfr.201200773
24. Cortes-Macías, E, Selma-Royo, M, García-Mantrana, I, Calatayud, M, González, S, Martínez-Costa, C, et al. Maternal diet shapes the breast milk microbiota composition and diversity: impact of mode of delivery and antibiotic exposure. J Nutr. (2021) 151:330–40. doi: 10.1093/jn/nxaa310
25. Selma-Royo, M, González, S, Gueimonde, M, Chang, M, Fürst, A, Martínez-Costa, C, et al. Maternal diet is associated with human milk oligosaccharide profile. Mol Nutr Food Res. (2022) 66:e2200058. doi: 10.1002/mnfr.202200058
26. Sims, CR, Saben, JL, Martinez, A, Sobik, SR, Crimmins, MR, Bulmanski, JE, et al. A Mediterranean diet plan in lactating women with obesity reduces maternal energy intake and modulates human Milk composition – a feasibility study. Front Nutr. (2024) 11:1303822. doi: 10.3389/fnut.2024.1303822
27. Seferovic, MD, Mohammad, M, Pace, RM, Engevik, M, Versalovic, J, Bode, L, et al. Maternal diet alters human milk oligosaccharide composition with implications for the milk metagenome. Sci Rep. (2020) 10:22092. doi: 10.1038/s41598-020-79022-6
28. Azad, MB, Robertson, B, Atakora, F, Becker, AB, Subbarao, P, Moraes, TJ, et al. Human milk oligosaccharide concentrations are associated with multiple fixed and modifiable maternal characteristics, environmental factors, and feeding practices. J Nutr. (2018) 148:1733–42. doi: 10.1093/jn/nxy175
29. Totten, SM, Zivkovic, AM, Wu, S, Ngyuen, U, Freeman, SL, Ruhaak, LR, et al. Comprehensive profiles of human milk oligosaccharides yield highly sensitive and specific markers for determining secretor status in lactating mothers. J Proteome Res. (2012) 11:6124–33. doi: 10.1021/pr300769g
30. Lefebvre, G, Shevlyakova, M, Charpagne, A, Marquis, J, Vogel, M, Kirsten, T, et al. Time of lactation and maternal fucosyltransferase genetic polymorphisms determine the variability in human milk oligosaccharides. Front Nutr. (2020) 7:574459. doi: 10.3389/fnut.2020.574459
31. Austin, S, De Castro, CA, Benet, T, Hou, Y, Sun, H, Thakkar, SK, et al. Temporal change of the content of 10 oligosaccharides in the milk of Chinese urban mothers. Nutrients. (2016) 8:346. doi: 10.3390/nu8060346
32. Sprenger, N, Lee, LY, De Castro, CA, Steenhout, P, and Thakkar, SK. Longitudinal change of selected human milk oligosaccharides and association to Infants' growth, an observatory, single center, longitudinal cohort study. PLoS One. (2017) 12:e0171814. doi: 10.1371/journal.pone.0171814
33. Romaszko, E, Marzec-Wróblewska, U, Badura, A, and Buciński, A. Does consumption of red grapefruit juice Alter Naringenin concentrations in milk produced by breastfeeding mothers? PLoS One. (2017) 12:e0185954. doi: 10.1371/journal.pone.0185954
34. Cabrera-Rubio, R, Kunz, C, Rudloff, S, García-Mantrana, I, Crehuá-Gaudiza, E, Martínez-Costa, C, et al. Association of Maternal Secretor Status and Human Milk Oligosaccharides with milk microbiota. J Pediatr Gastroenterol Nutr. (2019) 68:256–63. doi: 10.1097/MPG.0000000000002216
35. Castello, F, Costabile, G, Bresciani, L, Tassotti, M, Naviglio, D, Luongo, D, et al. Bioavailability and pharmacokinetic profile of grape pomace phenolic compounds in humans. Arch Biochem Biophys. (2018) 646:1–9. doi: 10.1016/j.abb.2018.03.021
36. Del Rio, D, Calani, L, Cordero, C, Salvatore, S, Pellegrini, N, and Brighenti, F. Bioavailability and catabolism of green tea flavan-3-ols in humans. Nutrition. (2010) 26:1110–6. doi: 10.1016/j.nut.2009.09.021
37. Neveu, V, Perez-Jiménez, J, Vos, F, Crespy, V, du Chaffaut, L, Mennen, L, et al. Phenol-explorer: an online comprehensive database on polyphenol contents in foods. Database. (2010) 2010:bap024. doi: 10.1093/database/bap024
38. Kean, EG, Hamaker, BR, and Ferruzzi, MG. Carotenoid bioaccessibility from whole grain and degermed maize meal products. J Agric Food Chem. (2008) 56:9918–26. doi: 10.1021/jf8018613
39. Mohamedshah, Z, Hayes, M, Chadwick-Corbin, S, Neilson, AP, and Ferruzzi, MG. Bioaccessibility, gut microbial metabolism and intestinal transport of phenolics from 100% Concord grape juice and whole grapes are similar in a simulated digestion and fecal fermentation model. Food Funct. (2022) 13:4315–30. doi: 10.1039/D1FO04226B
40. Noguchi, K, Gel, YR, Brunner, E, and Konietschke, F. Nparld: an R software package for the nonparametric analysis of longitudinal data in factorial experiments. J Stat Softw. (2012) 50:1–23. doi: 10.18637/jss.v050.i12
41. Kuznetsova, A, Brockhoff, PB, and Christensen, RHB. Lmertest package: tests in linear mixed effects models. J Stat Softw. (2017) 82:1–26. doi: 10.18637/jss.v082.i13
42. Lenth, RV. (2023). Emmeans: estimated marginal means, Aka least-squares means. R Package Version 1.8.9. Available at: https://cran.r-project.org/package=emmeans (Accessed March 01, 2024).
43. Bakdash, JZ, and Marusich, LR. Repeated measures correlation. Front Psychol. (2017) 8:252904. doi: 10.3389/fpsyg.2017.00456
44. R Core Team (2023). R: a language and environment for statistical computing. R Foundation for Statistical Computing, Vienna, Austria. Available at: https://www.r-project.org/. (Accessed March 01, 2024).
45. Kim, K, Madore, MP, and Chun, OK. Changes in intake and major food sources of carotenoids among U.S. adults between 2009-2018. Meta. (2023) 14:13. doi: 10.3390/metabo14010013
46. Chichlowski, M, van Diepen, JA, Prodan, A, Olga, L, Ong, KK, Kortman, GAM, et al. Early development of infant gut microbiota in relation to breastfeeding and human milk oligosaccharides. Front Nutr. (2023) 10:1003032. doi: 10.3389/fnut.2023.1003032
47. U.S. Department of Agriculture and U.S. Department of Health and Human Services. Dietary guidelines for Americans, 2020–2025. 9th ed (2020).
48. van het Hof, KH, Weststrate, JA, West, CE, and Hautvast, JGAJ. Dietary factors that affect the bioavailability of carotenoids. J Nutr. (2000) 130:503–6. doi: 10.1093/jn/130.3.503
49. Gossage, CP, Deyhim, M, Yamini, S, Douglass, LW, and Moser-Veillon, PB. Carotenoid composition of human milk during the first month postpartum and the response to Β-carotene Supplementation1,2,3. Am J Clin Nutr. (2002) 76:193–7. doi: 10.1093/ajcn/76.1.193
50. Novotny, JA, Chen, TY, Terekhov, AI, Gebauer, SK, Baer, DJ, Ho, L, et al. The effect of obesity and repeated exposure on pharmacokinetic response to grape polyphenols in humans. Mol Nutr Food Res. (2017) 61:43. doi: 10.1002/mnfr.201700043
51. Ho, L, Zhao, D, Ono, K, Ruan, K, Mogno, I, Tsuji, M, et al. Heterogeneity in gut microbiota drive polyphenol metabolism that influences Α-synuclein misfolding and toxicity. J Nutr Biochem. (2019) 64:170–81. doi: 10.1016/j.jnutbio.2018.10.019
52. Inoue-Choi, M, Yuan, JM, Yang, CS, Van Den Berg, DJ, Lee, MJ, Gao, YT, et al. Genetic association between the COMT genotype and urinary levels of tea polyphenols and their metabolites among daily green tea drinkers. Int J Mol Epidemiol Genet. (2010) 1:114–23.
53. Jochum, F, Alteheld, B, Meinardus, P, Dahlinger, N, Nomayo, A, and Stehle, P. Mothers' consumption of soy drink but not black tea increases the flavonoid content of term breast milk: a pilot randomized, controlled intervention study. Ann Nutr Metab. (2017) 70:147–53. doi: 10.1159/000471857
54. Durham, SD, Robinson, RC, Olga, L, Ong, KK, Chichlowski, M, Dunger, DB, et al. A one-year study of human milk oligosaccharide profiles in the milk of healthy Uk mothers and their relationship to maternal Fut2 genotype. Glycobiology. (2021) 31:1254–67. doi: 10.1093/glycob/cwab057
55. Zhao, Z, and Moghadasian, MH. Chemistry, natural sources, dietary intake and pharmacokinetic properties of ferulic acid: a review. Food Chem. (2008) 109:691–702. doi: 10.1016/j.foodchem.2008.02.039
56. Chateigner-Boutin, A-L, and Saulnier, L. Chapter five - ferulic and coumaric acids in the cereal grain: occurrence, biosynthesis, biological and technological functions In: R Sibout, editor. Advances in botanical research. 104. San Diego, London, Boston, New York, Sydney, Tokyo, and Toronto: Academic Press (2022). 169–213.
57. Zhao, Z, Egashira, Y, and Sanada, H. Digestion and absorption of ferulic acid sugar esters in rat gastrointestinal tract. J Agric Food Chem. (2003) 51:5534–9. doi: 10.1021/jf034455u
58. Boto-Ordóñez, M, Urpi-Sarda, M, Queipo-Ortuño, MI, Tulipani, S, Tinahones, FJ, and Andres-Lacueva, C. High levels of bifidobacteria are associated with increased levels of anthocyanin microbial metabolites: a randomized clinical trial. Food Funct. (2014) 5:1932–8. doi: 10.1039/C4FO00029C
59. Zhu, Y, Sun, H, He, S, Lou, Q, Yu, M, Tang, M, et al. Metabolism and prebiotics activity of anthocyanins from black Rice (Oryza Sativa L.) in vitro. PLoS One. (2018) 13:e0195754. doi: 10.1371/journal.pone.0195754
60. Zeng, X, Su, W, Zheng, Y, He, Y, He, Y, Rao, H, et al. Pharmacokinetics, tissue distribution, metabolism, and excretion of Naringin in aged rats. Front Pharmacol. (2019) 10:34. doi: 10.3389/fphar.2019.00034
61. Clifford Michael, N, Jaganath, IB, Ludwig, IA, and Crozier, A. Chlorogenic acids and the acyl-Quinic acids: discovery, biosynthesis, bioavailability and bioactivity. Nat Prod Rep. (2017) 34:1391–421. doi: 10.1039/C7NP00030H
62. Wacklin, P, Mäkivuokko, H, Alakulppi, N, Nikkilä, J, Tenkanen, H, Räbinä, J, et al. Secretor genotype (Fut2 gene) is strongly associated with the composition of bifidobacteria in the human intestine. PLoS One. (2011) 6:e20113. doi: 10.1371/journal.pone.0020113
63. Tonon, KM, de Morais, MB, Abrão, ACFV, Miranda, A, and Morais, TB. Maternal and infant factors associated with human milk oligosaccharides concentrations according to secretor and Lewis phenotypes. Nutrients. (2019) 11:1358. doi: 10.3390/nu11061358
64. Austin, S, De Castro, CA, Sprenger, N, Binia, A, Affolter, M, Garcia-Rodenas, CL, et al. Human milk oligosaccharides in the milk of mothers delivering term versus preterm infants. Nutrients. (2019) 11:1282. doi: 10.3390/nu11061282
65. Wang, M, Zhao, Z, Zhao, A, Zhang, J, Wu, W, Ren, Z, et al. Neutral human milk oligosaccharides are associated with multiple fixed and modifiable maternal and infant characteristics. Nutrients. (2020) 12:826. doi: 10.3390/nu12030826
66. Seppo, AE, Kukkonen, AK, Kuitunen, M, Savilahti, E, Yonemitsu, C, Bode, L, et al. Association of maternal probiotic supplementation with human milk oligosaccharide composition. JAMA Pediatr. (2019) 173:286–8. doi: 10.1001/jamapediatrics.2018.4835
67. Le Sayec, M, Xu, Y, Laiola, M, Gallego, FA, Katsikioti, D, Durbidge, C, et al. The effects of aronia berry (poly)phenol supplementation on arterial function and the gut microbiome in middle aged men and women: results from a randomized controlled trial. Clin Nutr. (2022) 41:2549–61. doi: 10.1016/j.clnu.2022.08.024
68. Moreno-Indias, I, Sánchez-Alcoholado, L, Pérez-Martínez, P, Andrés-Lacueva, C, Cardona, F, Tinahones, F, et al. Red wine polyphenols modulate fecal microbiota and reduce markers of the metabolic syndrome in obese patients. Food Funct. (2016) 7:1775–87. doi: 10.1039/C5FO00886G
69. Martín, R, Jiménez, E, Heilig, H, Fernández, L, Marín, ML, Zoetendal, EG, et al. Isolation of bifidobacteria from breast milk and assessment of the bifidobacterial population by PCR-denaturing gradient gel electrophoresis and quantitative real-time PCR. Appl Environ Microbiol. (2009) 75:965–9. doi: 10.1128/AEM.02063-08
70. Soto, A, Martín, V, Jiménez, E, Mader, I, Rodríguez, JM, and Fernández, L. Lactobacilli and bifidobacteria in human breast milk: influence of antibiotherapy and other host and clinical factors. J Pediatr Gastroenterol Nutr. (2014) 59:78–88. doi: 10.1097/MPG.0000000000000347
71. Ojima, MN, Jiang, L, Arzamasov, AA, Yoshida, K, Odamaki, T, Xiao, J, et al. Priority effects shape the structure of infant-type bifidobacterium communities on human milk oligosaccharides. ISME J. (2022) 16:2265–79. doi: 10.1038/s41396-022-01270-3
72. Garrido, D, Ruiz-Moyano, S, Kirmiz, N, Davis, JC, Totten, SM, Lemay, DG, et al. A novel gene cluster allows preferential utilization of fucosylated milk oligosaccharides in Bifidobacterium longum Subsp. Longum Sc596. Sci Rep. (2016) 6:35045. doi: 10.1038/srep35045
73. Ruiz-Moyano, S, Totten, SM, Garrido, DA, Smilowitz, JT, German, JB, Lebrilla, CB, et al. Variation in consumption of human milk oligosaccharides by infant gut-associated strains of Bifidobacterium breve. Appl Environ Microbiol. (2013) 79:6040–9. doi: 10.1128/AEM.01843-13
74. Yazaki, K. ABC transporters involved in the transport of plant secondary metabolites. FEBS Lett. (2006) 580:1183–91. doi: 10.1016/j.febslet.2005.12.009
75. Egan, M, O'Connell Motherway, M, Ventura, M, and van Sinderen, D. Metabolism of sialic acid by Bifidobacterium breve UCC2003. Appl Environ Microbiol. (2014) 80:4414–26. doi: 10.1128/AEM.01114-14
76. Ehrlich, AM, Pacheco, AR, Henrick, BM, Taft, D, Xu, G, Huda, MN, et al. Indole-3-lactic acid associated with bifidobacterium-dominated microbiota significantly decreases inflammation in intestinal epithelial cells. BMC Microbiol. (2020) 20:357. doi: 10.1186/s12866-020-02023-y
77. Huang, Q, Braffett, BH, Simmens, SJ, Young, HA, and Ogden, CL. Dietary polyphenol intake in us adults and 10-year trends: 2007-2016. J Acad Nutr Diet. (2020) 120:1821–33. doi: 10.1016/j.jand.2020.06.016
78. Du, M, Mozaffarian, D, Wong, JB, Pomeranz, JL, Wilde, P, and Zhang, FF. Whole-grain food intake among us adults, based on different definitions of whole-grain foods, Nhanes 2003-2018. Am J Clin Nutr. (2022) 116:1704–14. doi: 10.1093/ajcn/nqac267
Keywords: human milk, human milk oligosaccharides, carotenoids, polyphenols, obesity, Mediterranean meal plan
Citation: Fiecke C, Crimmins M, Hameed A, Sims C, Williams DK, Bode L, Martinez A, Andres A and Ferruzzi MG (2024) Dietary modulation of human milk bioactives is associated with maternal FUT2 secretor phenotype: an exploratory analysis of carotenoids and polyphenol metabolites. Front. Nutr. 11:1463969. doi: 10.3389/fnut.2024.1463969
Edited by:
Abdelhafid Nani, Université Ahmed Draia Adrar, AlgeriaReviewed by:
Patrick Solverson, Washington State University, United StatesAdélie Dumont, Université de Bourgogne, France
Copyright © 2024 Fiecke, Crimmins, Hameed, Sims, Williams, Bode, Martinez, Andres and Ferruzzi. This is an open-access article distributed under the terms of the Creative Commons Attribution License (CC BY). The use, distribution or reproduction in other forums is permitted, provided the original author(s) and the copyright owner(s) are credited and that the original publication in this journal is cited, in accordance with accepted academic practice. No use, distribution or reproduction is permitted which does not comply with these terms.
*Correspondence: Mario G. Ferruzzi, bWZlcnJ1enppQHVhbXMuZWR1; Aline Andres, YW5kcmVzYWxpbmVAdWFtcy5lZHU=
†These authors have contributed equally to this work and share first authorship