- 1Department of Science, Technology and Society, University School for Advanced Studies IUSS Pavia, Pavia, Italy
- 2Department of Translational and Precision Medicine, Sapienza University of Rome, Rome, Italy
- 3Department of Public Health, College of Health Science, Woldia University, Woldia, Ethiopia
- 4Belcolle Hospital, Viterbo, Italy
The current global trend in the nutrition, epidemiologic and demographic transitions collectively alarms the need to pursue a sustainable protein diet that respects ecosystem and biodiversity from alternative sources, such as algae, fungi and edible insects. Then, changing the nutrition reality is extremely important to impede the global syndemic of obesity, undernutrition and climate change. This review aims to synthesize the published literature on the potential roles of alternative proteins and their derived bioactive peptides in preventive and clinical nutrition, identify research gaps and inform future research areas. Google Scholar and PubMed databases from their inception up to 30 June 2024 were searched using keywords to access pertinent articles published in English language for the review. Overall, proteins derived from algae, fungi, and edible insects are high-quality proteins as animal sources and demonstrate significant potential as a sustainable source of bioactive peptides, which are metabolically potent and have negligible adverse effects. They show promise to prevent and treat diseases associated with oxidative stress, obesity, diabetes, cancer, cardiovascular disease (especially hypertension), and neurodegenerative diseases. Given the abundance of algae, fungi and insect peptides performed in vitro or in vivo animals, further clinical studies are needed to fully establish their safety, efficacy and practical application in preventive and clinical nutrition. Additionally, social and behavioral change communication strategies would be important to increase health awareness of nutritional benefits and promote consumer acceptance of alternative protein sources.
1 Introduction
Nutrition is a crucial pillar of human life, health and development (1, 2). Good nutrition ensures health and wellness at each stage of the human life cycle, such as pregnancy, infancy, childhood, adolescence, adulthood, and older age (3). Unfortunately, the double burden of malnutrition, i.e., concurrent manifestation of both undernutrition and overnutrition (overweight and obesity), may become manifest within the life course (4, 5). Although the burden of malnutrition mainly affects low and middle-income countries, countries passing the economic transitions also face the problem (6). Alongside, shifting of the overall dietary structure over time, i.e., the trend of the current nutrition transition, is associated with unrelenting non-communicable diseases (NCDs). Therefore, changing the nutrition reality is extremely important because a low-quality diet ignites a double burden of malnutrition (5, 7, 8).
By the year 2050, the world population is projected to be nearly 10 billion (9), the food demand is expected to increase by 56% (10), and animal-derived protein demand will be twofold (11), requiring a sustainable food system (12). Of significant concern, the increased consumption of red and processed meat globally contributes to an abrupt increase in the attributable burden of diet-related NCDs, including colorectal cancer, diabetes, and coronary heart disease, which are markedly risen in Northern and Eastern European countries as well as island countries in the Caribbean and Oceania (13). The demand and consumption of meat is also rapidly increasing in developing countries due to urbanization and rapid income growth (14).
Furthermore, raising livestock and animal meat consumption is associated with higher greenhouse gas emissions (15, 16), higher consumption of water and land use (17), human-induced terrestrial biodiversity loss (18, 19), contracting zoonosis and antibiotic resistance (20), and raised ethical concerns about animal welfare (21). These impacts clearly illustrate the need to pursue a sustainable healthy diet from alternative protein sources, such as algae, fungi, and edible insects, to achieve global food security without expanding crop or pastureland, deprived of increasing greenhouse gas emissions and without devaluing health (11, 17, 22–26). Figure 1 summarizes the relationship between obesity, undernutrition and climate change.
Shifting towards alternative protein sources is vital to meet most of the United Nations’ sustainable development goals and to achieve a net-zero greenhouse gas emission target indicated in the Paris Agreement (27). In accordance with this, in 2022, the sixth international panel on climate change urged governments to prioritize sustainable healthy diets to feed the projected population by 2050, while mitigating the effects of climate change (28). The environmental and nutritional advantage inspired the concept of a sustainable diet, i.e., a diet that respects biodiversity and ecosystems, that is nutritionally adequate, safe, healthy and at the same time culturally acceptable, and affordable, as highlighted by the Food and Agriculture Organization of the United Nations (29, 30). The role of a sustainable diet from plant origin on metabolic syndrome in humans is extensively explored (31–34). Emerging evidence also spotlights the potential role of alternative proteins (algae, fungi and insects) and their derived bioactive peptides, i.e., a group of biological molecules activated by extraction from parental proteins, to offer therapeutic advantages by modulating metabolic pathways (35). Particularly, bioactive peptides are known for their safety, tolerability, and minimal risk of adverse effects (36). The quests of these proteins and derived peptides in the context of preventive and clinical nutrition present a promising road to uncover innovative dietary strategies and therapeutic approaches. Given no previous review in the area, this narrative review aims to (1): explore the current research on the potential roles of alternative proteins and their derived bioactive peptides in preventive and clinical nutrition (2); identify gaps in the literature; and (3) inform future research.
2 Methods
Google Scholar and PubMed databases from their inception up to 30 June 2024 were searched using keywords to access pertinent articles published in English-language for the review. The reference lists of included studies were additionally screened to locate further relevant literature. We used the following keywords for the review: “algae OR algal protein OR algae peptide + Y,” “fungi OR fungal protein OR fungi peptide + Y,” “Insect OR insect protein OR insect peptide +Y,” where Y indicates hypertension, obesity, T2DM, dementia, cancer, and sarcopenia.
2.1 The role of algae-derived protein and bioactive peptides in preventive and clinical nutrition
Algae are protein-rich marine resources that have a rapid growth rate, can adapt to extreme and competitive environments, and contain numerous health-promoting compounds (37) and proteins, such as lectins, phycobiliproteins, mycosporine-like amino acids, derived hydrolysates, and bioactive peptides (38). Commercially available microalgae, such as spirulina (Cyanobacterium Arthrospira platensis) and Chlorella vulgaris contain up to 68% protein by dry weight (39, 40), whereas red algae contain up to 47% of protein (41). Proteins extracted from spirulina and chlorella have a high degree of in vitro digestibility and contain all essential amino acids (42, 43). Further studies assessing the in vivo digestibility of these proteins are necessary. Additionally, algae-derived bioactive peptides exhibited anti-microbial, anti-mutagenic, anti-inflammatory, anti-diabetic, anti-hypertensive, anti-oxidant, anti-tumor, neurophysiological and hepatoprotective activity (44–47). Figure 2 summarizes the role of algae, fungi and insect derived bioactive peptides in preventive and clinical nutrition.
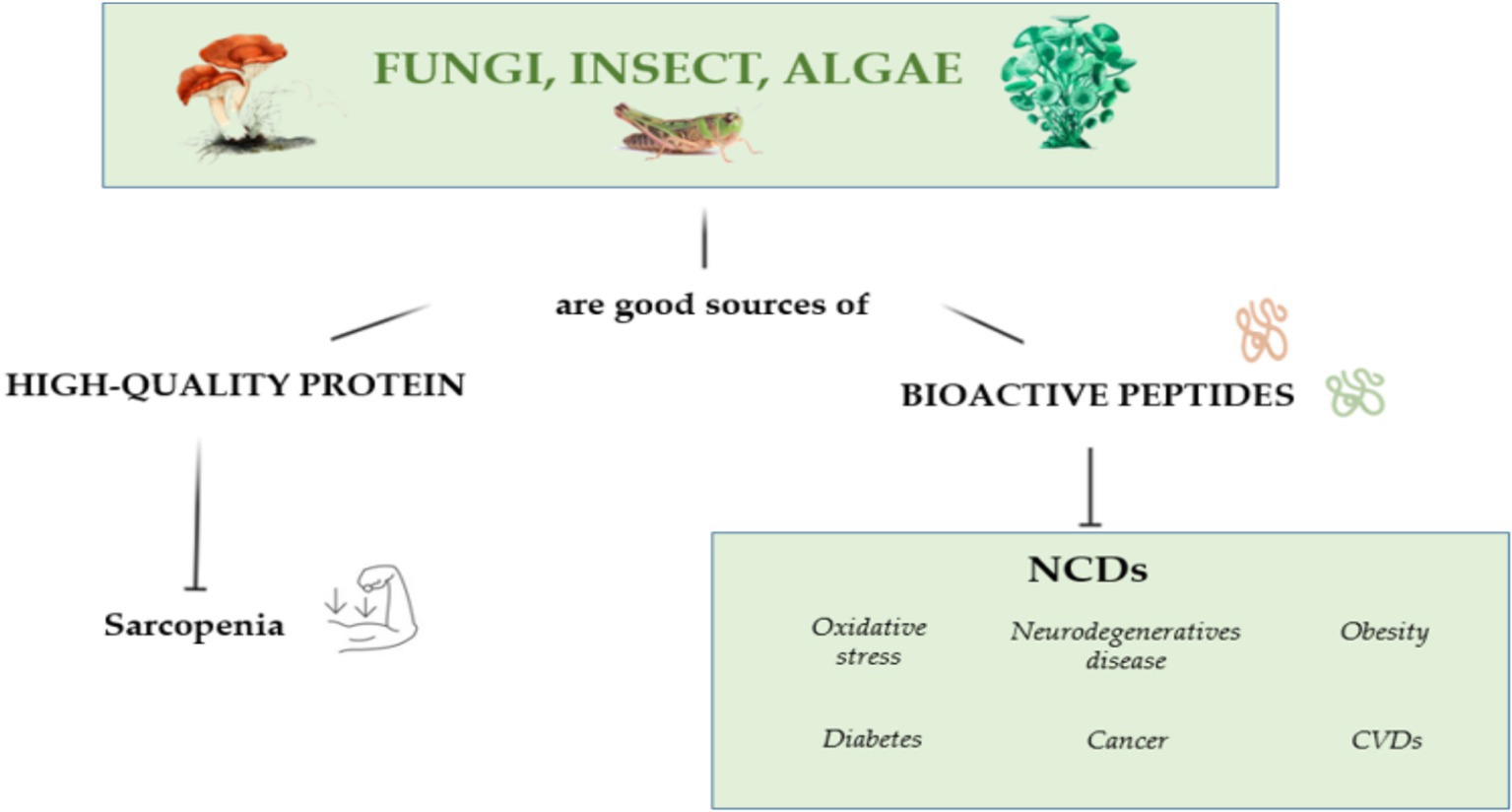
Figure 2. The possible positive effects of fungi, insects and algae in preventive and clinical nutrition.
2.1.1 Anti-sarcopenic effect of algae-derived protein and bioactive peptides
By the year 2050, the number of individuals aged 60 years and above will double (2.1 billion), particularly those over 85 years will triple (426 million) (48). Sarcopenia, the key hallmark of aging, refers to the progressive loss of muscle mass and loss of strength or performance (49). Sarcopenia negatively affects the quality of life of the individual (50, 51), burdens the public health sector (52, 53) and increases mortality rates (54, 55). Daily intake of adequate (30 g/meal) high biological value, leucine-rich proteins with physical activity is crucial to counteract sarcopenia by maximizing muscle protein synthesis (MPS) rate (56).
Food protein and their derived bioactive peptides could be a promising option in this regard (57, 58). For example, Spirulina platensis, is a potential protein supplement. A preceding study was conducted to examine the anti-sarcopenic effect of Spirulina protein hydrolysate (SPH) in dexamethasone-treated C2C12 cells. The results indicated that SPH inhibits muscle atrophy mainly by activating the Akt/FoxO3a signaling pathway, specifically by increasing MyoD1, Myf5, and myogenin and decreasing Atrogin-1, MuRF-1, and FoxO3a (59). However, further studies on algae-derived peptides using animal models is required to affirm SPH as a solution for the prevention, and treatment of sarcopenia. Recently, randomized controlled trials performed among young adults (age: 22 ± 3 years) have shown that algae-derived protein (spirulina, and chlorella) ingestion stimulates muscle protein synthesis similar to mycoprotein (60). However, further research in older adults is warranted to translate into the prevention and treatment of sarcopenia.
2.1.2 Anti-obesity effect of algae-derived protein and bioactive peptides
Globally, obesity is a major public health problem primarily associated with the consumption of high-fat diets and ultra-processed foods (7, 61). It is attributable to the global incidence of cardiovascular disease, cancer, type 2 diabetes mellitus, osteoarthritis, work disability, and sleep apnea (62). As a consequence, algae-derived proteins and bioactive peptides could serve as anti-obesity agents (63, 64). In high-fat diet-fed mice, the anti-obesity effects of Spirulina-derived protein (SPP) or peptide (SPPH) are higher to the whole Spirulina (WSP), SPP is slightly lower than SPPH, under the same dose (2 g/kg per day). Overall, SPPH showed good anti-obesity effects, such as reduction of body weight (39.8% ± 9.7%), lowering serum glucose (23.8% ± 1.6%), decreasing total cholesterol (20.8% ± 1.4%), while positive drug Simvastatin (10 mg/kg per day) had the corresponding values: 8.3 ± 4.6, 24.8 ± 1.9% and − 2.1% ± 0.2%, respectively. SPPH anti-obesity effects modulate the expressions of key genes in the brain (Acadm, Gcg) and liver (Retn, Fabp4, Ppard, and Slc27a1), which are linked with lipid metabolism and accumulation (65). A peptide, CANPHELPNK, identified from enzymatic hydrolysates of Spirulina platensis protein showed the best anti-proliferative activity on preadipocytes 3 T3-L1 (60.08%), which was close to that of Simvastatin (70.32%), at 2 mg/ml. Furthermore, the two peptides NPVWKRK and CANPHELPNK were also demonstrated to significantly reduce the accumulation of triglyceride at 600 μg/ml, with 23.7 and 19.5%, respectively compared with control (66). Moreover, in high fat diet-fed rats, supplementation with Chlorella vulgaris effectively reduced total serum lipids, liver triglycerides, and cholesterol (67).
Intriguingly, a systematic review and meta-analysis of randomized controlled trials in humans showed that spirulina supplementation significantly reduces body weight, body fat percentage, and waist circumference (68). It also decreases triglycerides and total cholesterol levels in patients with type 2 diabetes, metabolic syndrome, overweight, or obesity (69). Spirulina is “generally recognized as safe” for human consumption (70). Nevertheless, rare potential adverse effects, such as acute rhabdomyolysis (71) and anaphylaxis (72) were reported. Therefore, an allergy risk assessment before supplementation is strongly suggested.
2.1.3 Anti-hypertensive effect of algae-derived proteins and bioactive peptides
Hypertension shares a large quota as a risk factor for CVD (73). It is further leads to end-stage renal disease, stroke, disability, dementia and mortality (74–76). To lessen hypertension, angiotensin-converting enzyme (ACE) inhibition using synthetic drugs is the basic step, despite undesirable side effects, including dry cough, angioedema, disturbance, and skin rash are associated with the drug (77). Therefore, pursuing anti-hypertensive bioactive peptides from natural food sources, such as algae has paramount importance (78).
In silico and in vitro assessment showed that bioactive peptides (Alcalase, bromelain, papain, pepsin) of A. platensis demonstrated ACE inhibitory activity (80% inhibition rate at a level of 1.0 mg/ml) (79). Moreover, oral administration of protein hydrolysate from Chlorella Vulgaris (5 mg/kg of body weight) to spontaneously hypertensive rats (SHR) effectively reduced the systolic blood pressure by 50 mmHg, verifying the potent antihypertensive effects of certain peptides, equivalent to synthetic drugs (80). Interestingly, a systematic review and meta-analysis of randomized controlled trials in humans showed that chlorella supplementation (4 g/day) for 8 weeks and more, significantly reduced total cholesterol, low-density lipoprotein, systolic and diastolic blood pressure in hypertensive patients (81).
2.1.4 Anti-diabetic effect of algae-derived protein and bioactive peptides
Type 2 diabetes (T2DM) is a metabolic disorder that accounts for more than 90% of patients with diabetes, which leads to microvascular (neuropathy, retinopathy and nephropathy) and macrovascular (CVD) complications. In addition, T2DM has a significant impact on patients’ lives and puts a huge burden on healthcare systems (82, 83). The existing anti-diabetic agents are often associated with side effects (84); as a result, there is a growing interest in the use of food protein/peptides as a potential remedy for the prevention and management of T2DM (85), especially in its early forms.
Bioactive peptides derived from various algae species, such as A. platensis (79, 86, 87), Chlorella sp. (88), and P. palmata (89), have demonstrated anti-diabetic bioactivities via inhibiting Dipeptidyl peptidase IV (DPP-IV), and carbohydrate-digesting enzyme inhibition, i.e., α-amylase and α-glucosidase, thereby reduced the postprandial blood glucose absorption. Literature has shown that Spirulina platensis-derived anti-hyperglycemic peptide exhibited the best inhibition on α-amylase (62%), α-glucosidase (90%) and DPP-IV (49%) (87). Thus, the inhibitory activity of α-glucosidase was remarkably higher than α-amylase (87). This could be useful to decrease side effects due to the abnormal bacterial fermentation of undigested saccharides in the colon, which commonly occurs following excessive inhibition by α-amylase (90).
In streptozotocin-induced diabetic mice (SIDM), oral administration of P. palmata crude protein hydrolysate influenced the incretin system by directly upregulating the secretion of glucagon-like peptide 1 (GLP-1) and glucose-dependent insulinotropic polypeptide (GIP) (89).
2.1.5 Anti-dementia role of algal proteins
Among individuals diagnosed with dementia, a neurodegenerative disease, such as Alzheimer’s disease (AD) shares a large part (60%), which arises when nerve cells in the central nervous system gradually lose function due to proinflammatory stimulus that facilitates the generation of neurotoxicity substances, and eventually die (91, 92). Neurodegenerative disease affects the patient’s quality of life (93), increases the burden for caregivers (94) and poses a higher economic cost (95). Though the available treatments may relieve symptoms, no known curative treatment is found for neurodegenerative diseases. As a consequence, food-derived peptides could have the potential to mitigate neurological inflammation, thereby improving cognitive performance (91, 96). A previous study indicated that dietary administration of 1 and 2% Spirulina platensis for 16 weeks in high fat diet fed mice significantly improved spatial learning and memory performance via inhibiting Aβ accumulation, tau-hyperphosphorylation, and neuroinflammation in the hippocampus (97). This study provides further evidence for the application of Spirulina platensis derived protein as a functional supplement for the treatment of AD. In support, the effects of spirulina intake (500 mg/day spirulina powder versus placebo twice a day for 12 weeks) among 60 patients with AD significantly improved their cognitive function, as evidenced by the mini-mental state examination score (MMSE). Additionally, spirulina intake decreased high-sensitivity C-reactive protein, fasting glucose, insulin resistance, and increased insulin sensitivity compared with the placebo (98).
2.1.6 Anti-cancer role of algae-derived protein and bioactive peptides
Cancer is the leading cause of death next to CVD (99). Cancer metastasis to vital organs of the body usually results in massive tissue destruction, loss of life-sustaining functions, and death (100). The investigation of anticancer drugs derived from natural products has become a hot topic in the field of cancer research because of their lower economic cost and fewer side effects (101, 102).
Dietary algae such as Chlorella vulgaris (103), Chlorella pyrenoidosa (104), Spirulina platensis (105), and Enteromorpha prolifera (106) have shown significant anticancer bioactivities. Literature has shown that enzymatic hydrolysates of A. platensis protein at a concentration of 0.5 mg/ml exhibited comparable inhibitory effects against breast cancer cells (MCF-7), lung cancer cells (A549), gastric cancer cells (SGC-7901), colon cancer cells (HT-29), and liver cancer cells (HepG2) compared to the positive control drug 5-flurouracil (5-FU) (105, 107, 108). Moreover, the protein-derived peptide from Chlorella vulgaris stops human AGS gastric cancer cells after G1 phase (103). Papain hydrolysate purified from Chlorella pyrenoidosa showed anti-proliferation activity against HepG2 (104). Recently, heptapeptide (GPLGAGP) isolated from hydrolysates of Enteromorpha prolifera showed the most potent inhibitory activity against NCI-H460 lung cancer cells (106).
2.2 The role of fungi-derived protein and bioactive peptides in preventive and clinical nutrition
Fungi are suitable alternative sources of food for humans which have a low environmental footprint, and could be a solution for a sustainable future for our planet (109–113). Mycoprotein, which is a proteinaceous wholefood produced from the continuous fermentation of the filamentous fungus Fusarium venenatum, is the most popular example that is commercialized and sold in different countries (UK, USA, Belgium, Germany, Denmark, Ireland, France, the Netherlands, Switzerland, Sweden etc.) as an ingredient in products marketed under the brand name Quorn™ (114). Mycoprotein product contains high-quality protein (11.5%), fiber (6%) composed of glucan-chitin matrix, sugar (0.8%), fat (2.9%), and minerals, such as selenium (20%), zinc (less bioavailable than meat), iron (lower than meat), manganese, calcium, and phosphorus, and a source of vitamin B2 (115). Also, it is devoid of trans-fats and cholesterol. Due to its healthy nutritional profile, the consumption of mycoprotein is increasing worldwide (112). For example, including mycoprotein in the daily diet helps maintaining glycaemic control (116). Furthermore, edible mushrooms have tremendous health benefits including anti-inflammatory, antioxidant, anti-cancer, anti-diabetic and anti-obesity properties (117, 118).
2.2.1 The role of fungi-derived protein and bioactive peptides in weight management
Owing to its sufficient amount of fiber and high protein content, mycoprotein plays a vital role in fighting hunger, decreasing body weight, and reducing energy intake. Literature have shown that mycoprotein ingestion reduces energy intake and increases satiety effects in obese and overweight individuals (119, 120). A study with 36 overweight and obese adults showed that 132 g energy-matched mycoprotein-based preload meal compared to a chicken meal for 180 min reduced energy intake by 10% (120). This could partly be due to the thermogenic and satiety effect of mycoprotein (121). Further longer-term studies are needed to investigate the potential of mycoprotein in the prevention of obesity and T2DM.
Interestingly, Ganoderma lucidum—a medicinal mushroom—showed anti-obesity activities by modulating the gut microbiota (decreased the ratio of Firmicutes to Bacteroidetes and levels of Proteobacteria) in high-fat diet (HFD)-fed mice, suggesting as a prebiotic to reduce weight (122). The finding also showed that supplementation of water extract of Ganoderma lucidum mycelium to HFD-fed mice daily for 2 months by oral gavage results in a dose-dependently decreased weight gain and both epididymal and subcutaneous fat accumulation, and reduced inflammation and insulin resistance compared with untreated controls. Given scarce studies in the area, extensive research and clinical studies demonstrating the safety and effectiveness of fungi-derived bioactive peptides are worthy of further investigation.
2.2.2 The role of fungi-derived protein and bioactive peptides in improving cardio-metabolic health
Mycoprotein ingestion also advantageously modifies blood lipid profiles, as indicated by studies (123, 124). The greatest benefits have been observed in subjects with elevated cholesterol levels at baseline (124). A study conducted on 17 healthy participants indicated that ingestion of mycoprotein-containing products (191 g) per day for 3 weeks reduced total cholesterol, lowered low-density lipoprotein and improved high-density lipoprotein by 13, 9, and 12%, respectively (125). A randomized trial was conducted in a home setting among 72 overweight, hypercholesterolaemic adults showed that mycoprotein ingestion for 4 weeks reduces serum cholesterol, low-density lipoprotein level, blood glucose, and C-peptide concentrations by 6, 10, 13 and 27%, respectively (123). This implies that mycoprotein may improve peripheral insulin sensitivity, suggesting a role in preventive and clinical nutrition in reducing the risk of obesity and diabetes mellitus in adults. However, further investigation is warranted to consolidate this evidence. Consumption of edible mushrooms decreases plasma triglyceride, total cholesterol, and low-density lipoprotein (126). Moreover, bioactive peptides obtained from different edible mushroom species, such as Pholiota adiposa, pleurotus cornucopiae, Hypsizygus marmoreus, Agaricus bisporus, Tricholoma giganteum, Ganoderma Lucidum, and shiitake mushroom (Lentinula edodes) exhibited ACE inhibitory activities (127–133). A detailed summary of the beneficial effects of bioactive peptides has been provided in Table 1.
2.2.3 The role of fungi-derived protein and bioactive peptides in muscle anabolism
Furthermore, mycoprotein ingestion (121, 123, 134–137) increases muscle protein synthesis rate when taken as a whole food, an isolated source or in a blended form, particularly in young individuals. Mycoprotein (at a dose of 60–80 g) ingestion led to slower but more sustained hyperaminoacidemia and hyperinsulinemia when compared with 20 g protein-match milk (121). Similarly, mycoprotein ingestion (70 g) resulted in a less rapid but more sustained increase in serum insulin levels, peaking at 30 min after consumption when compared with milk protein (136). However, a large amount of mycoprotein ingestion coupled with its satiating properties (138, 139) may not be a pragmatic approach in compromised individuals (anorexic older adults, patients with sarcopenia and cachexia). In such situations, protein concentrate from mycoprotein, and BCAA-enriched mycoprotein may be a feasible option, but further investigation is warranted.
2.3 The role of insect-derived protein and bioactive peptides in preventive and clinical nutrition
Given that more than 2,100 edible insects are found worldwide, their nutritional composition is difficult to generalize (140). However, many edible insects contain high-quality protein (141), unsaturated fatty acids (linolenic and linoleic acid), micronutrients (iron, zinc, calcium, potassium, magnesium, manganese, copper, vitamin E, vitamin K, vitamin B2, and vitamin B12) and fiber (142–145). Interestingly, iron and zinc found in crickets, grasshoppers and mealworms have been shown highly bioavailable compared to sirloin beef (143). Currently, common edible insect species are house cricket (Acheta domesticus), African palm weevil (Rhynchophorus phoenicis), yellow mealworm (Tenebrio molitor), mopane worm (Gonimbrasia belina), domesticated silkworm (Bombyx mori),and honeybee (Apis mellifera) (146). Edible insects are a sustainable source of protein for food and feed given their lower water and land consumption, high feed conversion efficiency, low greenhouse gas emissions compared to livestock, short life cycle, and rapid intrinsic growth rate (147, 148).
2.3.1 The role of insect-derived protein in muscle protein synthesis: insight for sarcopenia prevention
Studies showed that ingestion of lesser mealworm-derived protein (149), and cricket protein powder (150) were capable of stimulating MPS at rest and after exercise in young men. In particular, similar postprandial amino acid availability and MPS rates occurred after ingestion of 30 g lesser mealworm-derived protein to an equivalent amount of milk protein concentrate (149). In addition, no significant difference was observed in the mammalian target of rapamycin (mTORC1) signaling between cricket, pea, and whey protein despite a higher concentration of EAA and leucine occurring after ingestion of whey (150). Similarly, the EAA, BCAA, and leucine plasma levels peaked earlier in soy, whey and beef-derived protein than for cricket and lesser-mealworm (151, 152). The observed differences may be attributed to the slowly digested properties of lesser mealworm and cricket-derived compared to animal-derived proteins.
The high muscle protein anabolic potential of insect-derived proteins provides a strong initiative for integrating insects into the diet, especially in Western countries where acceptance of insect consumption is low. The high quality of insect-derived proteins coupled with their high amounts of micronutrients and antioxidants (153–155), will be of particular relevance in populations that consume less protein and suffer from anabolic resistance (156), such as the older and more clinically compromised populations.
2.3.2 The role of insect-derived bioactive peptides in preventing obesity, diabetes and hypertension
Although human studies are still lacking, peptides and bioactive compounds derived from edible insects could provide health benefits, such as anti-oxidant (157), anti-obesity (158), anti-diabetic (159) and anti-hypertensive properties (160). A study showed that yellow mealworm larvae powder administration in obese mice reduced body weight gain by decreasing lipid accumulation and triglyceride content in adipocytes (158), thus demonstrating the potential to induce weight loss. Moreover, an ethanol extract of the Korean horn beetle Allomyrina dichotoma injection into the brain tissue of obese mice reduced both endoplasmic reticulum (ER) stress and hormone-induced change in feeding behaviour (159). Thus, it could imply in preventing and treating obesity and T2DM since reduction of ER stress enhances insulin-producing beta cells (161).
Antioxidant activity in edible insect species was reported, including free radical-scavenging activity, and iron-chelating ability (157). Moreover, inhibition of ACE, pancreatic lipase and α-glucosidase have been observed in peptides derived from yellow mealworm(Tenbrio Molitor), silkworm (Bombyx mori), cricket (Gryllodes sigillatus) and locust (Schistocerca gregaria) (160, 162–164). Besides the ACE inhibitory activity, peptides derived from Tenebrio molitor larvae showed strong antithrombotic properties (165). However, the evidence mentioned above (157–160, 162–165) requires further human studies to translate into actual health benefits.
2.4 Food safety of edible insects
Apart from the nutritional, environmental and health benefits of edible insects, food safety issues need to be considered. This includes anti-nutrient content, allergenic potential, microbial safety and chemical contamination. Anti-nutrients, such as phytic acid, tannin, saponins, oxalate and cyanogenic glycosides have been determined in edible insects (145, 166, 167). More research on the anti-nutritional properties is necessary. Allergic reactions that could be triggered by insect consumption include nausea, vomiting, diarrhea, asthma, and skin reactions (168, 169). Commonly, tropomyosin and arginine kinase pan-allergens have been identified (168–170). Cross-reactivity and co-sensitisation between (insects and house dust mites) and (insects and crustaceans) occur because of these pan-allergens (168, 169). Therefore, clear labelling and communication of the allergenic potential of insects to the consumer is required since insect protein allergenicity is not removed by thermal treatments (168).
Concerning microbial safety, different microbiota have been found on raw edible insects, including lactic acid bacteria, Enterobacteriaceae, fungi, mesophilic aerobes, spore-forming bacteria, and foodborne pathogens (171), albeit no outbreaks associated with pathogens have been reported in the scientific literature. Thus, effective decontamination, and good hygiene practices during rearing, processing and storage are essential to minimize the microbial hazards of insect-containing food products (172).
Regarding chemical contamination of edible insects, low levels of contaminants (the level below the legal maximum amount), such as dioxin compounds, pesticide residue, arsenic, cadmium, copper, nickel, mercury, lead, and tin have been identified (173, 174). Insect species, rearing conditions, feed substrate including the packaging material of the substrate, and post-harvest processing were factors for the chemical contamination of edible insects (172, 175). Regular monitoring of the rearing environment, strong supplier auditing and certification process could reduce the risk of heavy metal contamination (176). Additionally, using naturally derived biopesticides from plants, micro-organisms or beneficial insects, such as neem oil, bacillus thuringiensis, and insect pheromones could decrease the deposition of residual pesticides (177). Future research should focus on determining the best processing conditions to create insect protein isolates with good functional characteristics, cost-effectiveness, and environmental sustainability that can be used in food formulation.
2.5 Challenges and strategies to consume edible insects
Despite a surfeit of health benefits, and the potential of edible insects to represent an environmentally sustainable nutrient source, willingness to consume insects as a food is low in Western countries (178, 179); albeit they aren’t against advances and food innovations. Food disgust (the product goes beyond the internalized norm of what food is), lack of familiarity with insect consumption, neophobia (hesitance to consume unfamiliar food), lack of product information, curiosity or sensation seeking and food technology neophobia were the identified drivers for low acceptance of insect consumption (178–180). As a result, different strategies have been suggested to convince consumers, such as underscoring that insects are nutritious, harvesting properly on controlled farms, making insect products delicious (enhancing the culinary experience), familiarizing insect-based products, integrating them in unrecognizable forms in familiar products, marketing insect-based products by taste, using celebrities to promote the product, targeting specific groups (sensation seekers, adventurous consumers or children), information provision from a nutritional and environmental standpoint, devising market approaches (stylistic images, choosing supermarkets for retailing, and using promotional tools such as buy-one-get-one-free, and discounts) (176, 181).
3 Conclusion
In conclusion, proteins derived from algae, fungi, and edible insects are high-quality proteins as animal sources and demonstrate significant potential as a sustainable source of bioactive peptides, which are metabolically potent and have negligible adverse effects. They show promise to prevent and treat diseases associated with oxidative stress, obesity, diabetes, cancer, cardiovascular disease (especially hypertension), and neurodegenerative diseases. Given the abundance of algae, fungi and insect peptides performed in vitro or in vivo animals, further research, validation and clinical studies are needed to fully establish their safety, efficacy and practical application in preventive and clinical nutrition. Additionally, social and behavioral change communication strategies would be important to increase health awareness of nutritional benefits and promote consumer acceptance of alternative protein sources.
Author contributions
MY: Conceptualization, Methodology, Visualization, Writing – original draft, Writing – review & editing. MA: Conceptualization, Methodology, Visualization, Writing – original draft, Writing – review & editing. SC: Conceptualization, Methodology, Visualization, Writing – original draft, Writing – review & editing. MM: Conceptualization, Methodology, Visualization, Writing – original draft, Writing – review & editing.
Funding
The author(s) declare that no financial support was received for the research, authorship, and/or publication of this article.
Acknowledgments
This paper and related research have been conducted during and with the support of the Italian national inter-university PhD course in Sustainable Development and Climate change (link: www.phd-sdc.it).
Conflict of interest
The authors declare that the research was conducted in the absence of any commercial or financial relationships that could be construed as a potential conflict of interest.
The author(s) declared that they were an editorial board member of Frontiers, at the time of submission. This had no impact on the peer review process and the final decision.
Publisher’s note
All claims expressed in this article are solely those of the authors and do not necessarily represent those of their affiliated organizations, or those of the publisher, the editors and the reviewers. Any product that may be evaluated in this article, or claim that may be made by its manufacturer, is not guaranteed or endorsed by the publisher.
References
1. Ross, AC, Caballero, B, Cousins, RJ, and Tucker, KL. Modern nutrition in health and disease. Burlington, Massachusettes: Jones & Bartlett Learning (2020).
2. Norris, SA, Frongillo, EA, Black, MM, Dong, Y, Fall, C, Lampl, M, et al. Nutrition in adolescent growth and development. Lancet. (2022) 399:172–84. doi: 10.1016/S0140-6736(21)01590-7
3. Sharlin, J, and Edelstein, S. Essentials of life cycle nutrition. Sudbury, Massachusetts: Jones & Bartlett Publishers (2010).
4. Wells, JC, Sawaya, AL, Wibaek, R, Mwangome, M, Poullas, MS, Yajnik, CS, et al. The double burden of malnutrition: aetiological pathways and consequences for health. Lancet. (2020) 395:75–88. doi: 10.1016/S0140-6736(19)32472-9
5. Popkin, BM, Corvalan, C, and Grummer-Strawn, LM. Dynamics of the double burden of malnutrition and the changing nutrition reality. Lancet. (2020) 395:65–74. doi: 10.1016/S0140-6736(19)32497-3
6. Prentice, AM. The double burden of malnutrition in countries passing through the economic transition. Ann Nutr Metab. (2018) 72:47–54. doi: 10.1159/000487383
7. Popkin, BM. The nutrition transition: an overview of world patterns of change. Nutr Rev. (2004) 62:S140–3. doi: 10.1111/j.1753-4887.2004.tb00084.x
8. Lopez Barrera, E, and Hertel, T. Solutions to the double burden of malnutrition also generate health and environmental benefits. Nat Food. (2023) 4:616–24. doi: 10.1038/s43016-023-00798-7
9. United Nations DoEaSA (2019). Population Division. World Population Prospects 2019: ten key findings.
10. Van Dijk, M, Morley, T, Rau, ML, and Saghai, Y. A meta-analysis of projected global food demand and population at risk of hunger for the period 2010–2050. Nat Food. (2021) 2:494–501. doi: 10.1038/s43016-021-00322-9
11. Henchion, M, Hayes, M, Mullen, AM, Fenelon, M, and Tiwari, B. Future protein supply and demand: strategies and factors influencing a sustainable equilibrium. Food Secur. (2017) 6:53. doi: 10.3390/foods6070053
12. Willett, W, Rockström, J, Loken, B, Springmann, M, Lang, T, Vermeulen, S, et al. Food in the Anthropocene: the EAT–Lancet Commission on healthy diets from sustainable food systems. Lancet. (2019) 393:447–92. doi: 10.1016/S0140-6736(18)31788-4
13. Chung, MG, Li, Y, and Liu, J. Global red and processed meat trade and non-communicable diseases. BMJ. Glob Health. (2021) 6. doi: 10.1136/bmjgh-2021-006394
14. Chung, MG, Kapsar, K, Frank, KA, and Liu, J. The spatial and temporal dynamics of global meat trade networks. Sci Rep. (2020) 10:16657. doi: 10.1038/s41598-020-73591-2
15. Xu, X, Sharma, P, Shu, S, Lin, T-S, Ciais, P, Tubiello, FN, et al. Global greenhouse gas emissions from animal-based foods are twice those of plant-based foods. Nat Food. (2021) 2:724–32. doi: 10.1038/s43016-021-00358-x
16. Revell, B. (2015). Meat and milk consumption 2050: The potential for demand-side solutions to greenhouse gas emissions reduction. Wiley Online Library. p. 4–11.
17. Thavamani, A, Sferra, TJ, and Sankararaman, S. Meet the meat alternatives: The value of alternative protein sources. Curr Nut Rep. (2020) 9:346–55. doi: 10.1007/s13668-020-00341-1
18. Li, Y, Zhong, H, Shan, Y, Hang, Y, Wang, D, Zhou, Y, et al. Changes in global food consumption increase GHG emissions despite efficiency gains along global supply chains. Nat Food. (2023) 4:483–95. doi: 10.1038/s43016-023-00768-z
19. Ramankutty, N, Mehrabi, Z, Waha, K, Jarvis, L, Kremen, C, Herrero, M, et al. Trends in global agricultural land use: implications for environmental health and food security. Annu Rev Plant Biol. (2018) 69:789–815. doi: 10.1146/annurev-arplant-042817-040256
20. Van der Weele, C, Feindt, P, van der Goot, AJ, van Mierlo, B, and van Boekel, M. Meat alternatives: an integrative comparison. Trends Food Sci Technol. (2019) 88:505–12. doi: 10.1016/j.tifs.2019.04.018
21. Verbeke, WA, and Viaene, J. Ethical challenges for livestock production: Meeting consumer concerns about meat safety and animalwelfare. J Agric Environ Ethics. (2000) 12:141–51. doi: 10.1023/A:1009538613588
22. Alsaffar, AA. Sustainable diets: The interaction between food industry, nutrition, health and the environment. Food Sci Technol Int. (2016) 22:102–11. doi: 10.1177/1082013215572029
23. Van Huis, A, Van Itterbeeck, J, Klunder, H, Mertens, E, Halloran, A, Muir, G, et al. (2013). Edible insects: future prospects for food and feed security. Rome, Italy: Food and agriculture organization of the United Nations.
24. Miglietta, PP, De Leo, F, Ruberti, M, and Massari, S. Mealworms for food: A water footprint perspective. Water. (2015) 7:6190–203. doi: 10.3390/w7116190
25. Hollis, JL, Demaio, S, Yang, WY, Trijsburg, L, Brouwer, ID, Jewell, J, et al. Investing in early nutrition and food systems for human and planetary health. Lancet Child Adolesc Health. (2021) 5:772–4. doi: 10.1016/S2352-4642(21)00306-0
26. Oonincx, DG, Van Itterbeeck, J, Heetkamp, MJ, Van Den Brand, H, Van Loon, JJ, and Van Huis, A. An exploration on greenhouse gas and ammonia production by insect species suitable for animal or human consumption. PLoS One. (2010) 5:e14445. doi: 10.1371/journal.pone.0014445
28. Intergovernmental Panel on Climate Change (2022). Climate Change 2022: Mitigation of Climate Change Summary for Policymakers. Working Group III contribution to the Sixth Assessment Report of the Intergovernmental Panel on Climate Change. Available at: https://www.ipcc.ch/report/ar6/wg3/downloads/report/IPCC_AR6_WGIII_FullReport.pdf (Accessed August 21, 2024).
29. FAO. Climate smart agriculture: policies, practices and financing for food security, adaptation and mitigation. Italy: Food and Agriculture Organization Rome (2010).
31. Thomas, MS, Calle, M, and Fernandez, ML. Healthy plant-based diets improve dyslipidemias, insulin resistance, and inflammation in metabolic syndrome. A narrative review. Adv Nutr. (2023) 14:44–54. doi: 10.1016/j.advnut.2022.10.002
32. Peña-Jorquera, H, Cid-Jofré, V, Landaeta-Díaz, L, Petermann-Rocha, F, Martorell, M, Zbinden-Foncea, H, et al. Plant-based nutrition: Exploring health benefits for atherosclerosis, chronic diseases, and metabolic syndrome—A comprehensive review. Nutrients. (2023) 15:3244. doi: 10.3390/nu15143244
33. Gueugneau, M. The value of dietary plant protein in older people. Curr Opin Clin Nut Metab Care. (2023) 26:3–7. doi: 10.1097/MCO.0000000000000884
34. Ahnen, RT, Jonnalagadda, SS, and Slavin, JL. Role of plant protein in nutrition, wellness, and health. Nutr Rev. (2019) 77:735–47. doi: 10.1093/nutrit/nuz028
35. Akbarian, M, Khani, A, Eghbalpour, S, and Uversky, VN. Bioactive peptides: Synthesis, sources, applications, and proposed mechanisms of action. Int J Mol Sci. (2022) 23:1445. doi: 10.3390/ijms23031445
36. Cao, S-j, Lv, Z-q, Guo, S, Jiang, G-p, and Liu, H-l. An Update-Prolonging the action of protein and peptide drugs. J Drug Deliv Sci Technol. (2021) 61:102124. doi: 10.1016/j.jddst.2020.102124
37. Bourgougnon, N, Burlot, A-S, and Jacquin, A-G. (2021). Algae for global sustainability? Advances in botanical research. Elsevier. p. 145–212.
38. Stengel, DB, Connan, S, and Popper, ZA. Algal chemodiversity and bioactivity: sources of natural variability and implications for commercial application. Biotechnol Adv. (2011) 29:483–501. doi: 10.1016/j.biotechadv.2011.05.016
39. Safafar, H, Uldall Nørregaard, P, Ljubic, A, Møller, P, Løvstad Holdt, S, and Jacobsen, C. Enhancement of protein and pigment content in two Chlorella species cultivated on industrial process water. J Marine Sci Eng. (2016) 4:84. doi: 10.3390/jmse4040084
40. Gómez, C, Guzmán-Carrasco, A, Lafarga, T, and Acién-Fernández, FG. Optimization of a new culture medium for the large-scale production of protein-rich arthrospira platensis (oscillatoriales, cyanophyceae). J Phycol. (2021) 57:636–44. doi: 10.1111/jpy.13111
41. Sánchez-Machado, D, López-Cervantes, J, Lopez-Hernandez, J, and Paseiro-Losada, P. Fatty acids, total lipid, protein and ash contents of processed edible seaweeds. Food Chem. (2004) 85:439–44. doi: 10.1016/j.foodchem.2003.08.001
43. Spolaore, P, Joannis-Cassan, C, Duran, E, and Isambert, A. Commercial applications of microalgae. J Biosci Bioeng. (2006) 101:87–96. doi: 10.1263/jbb.101.87
44. El Gamal, AA. Biological importance of marine algae. Saudi Pharm J. (2010) 18:1–25. doi: 10.1016/j.jsps.2009.12.001
45. Sathya, R, MubarakAli, D, MohamedSaalis, J, and Kim, J-W. A systemic review on microalgal peptides: Bioprocess and sustainable applications. Sustainability. (2021) 13:3262. doi: 10.3390/su13063262
46. O’Connor, J, Garcia-Vaquero, M, Meaney, S, and Tiwari, BK. Bioactive peptides from algae: Traditional and novel generation strategies, structure-function relationships, and bioinformatics as predictive tools for bioactivity. Marine Drugs. (2022) 20:317. doi: 10.3390/md20050317
47. Cermeño, M, Stack, J, Tobin, PR, O'Keeffe, MB, Harnedy, PA, Stengel, DB, et al. Peptide identification from a Porphyra dioica protein hydrolysate with antioxidant, angiotensin converting enzyme and dipeptidyl peptidase IV inhibitory activities. Food Funct. (2019) 10:3421–9. doi: 10.1039/C9FO00680J
48. World Health Organization (2022). Ageing and Health Available at: https://www.who.int/news-room/fact-sheets/detail/ageing-and-health (Accessed April 21, 2024).
49. Fielding, RA, Vellas, B, Evans, WJ, Bhasin, S, Morley, JE, Newman, AB, et al. Sarcopenia: an undiagnosed condition in older adults. Current consensus definition: prevalence, etiology, and consequences. International working group on sarcopenia. J Am Med Dir Assoc. (2011) 12:249–56. doi: 10.1016/j.jamda.2011.01.003
50. Beaudart, C, Demonceau, C, Reginster, JY, Locquet, M, Cesari, M, Cruz Jentoft, AJ, et al. Sarcopenia and health-related quality of life: A systematic review and meta-analysis. J Cachexia Sarcopenia Muscle. (2023) 14:1228–43. doi: 10.1002/jcsm.13243
51. Rizzoli, R, Reginster, J-Y, Arnal, J-F, Bautmans, I, Beaudart, C, Bischoff-Ferrari, H, et al. Quality of life in sarcopenia and frailty. Calcif Tissue Int. (2013) 93:101–20. doi: 10.1007/s00223-013-9758-y
52. Beaudart, C, Rizzoli, R, Bruyère, O, Reginster, J-Y, and Biver, E. Sarcopenia: burden and challenges for public health. Arch Public Health. (2014) 72:1–8. doi: 10.1186/2049-3258-72-45
53. Bruyère, O, Beaudart, C, Ethgen, O, Reginster, J-Y, and Locquet, M. The health economics burden of sarcopenia: a systematic review. Maturitas. (2019) 119:61–9. doi: 10.1016/j.maturitas.2018.11.003
54. Arango-Lopera, V, Arroyo, P, Gutiérrez-Robledo, LM, Pérez-Zepeda, MU, and Cesari, M. Mortality as an adverse outcome of sarcopenia. J Nutr Health Aging. (2013) 17:259–62. doi: 10.1007/s12603-012-0434-0
55. Chang, SF, and Lin, PL. Systematic literature review and meta-analysis of the association of sarcopenia with mortality. Worldviews Evid-Based Nurs. (2016) 13:153–62. doi: 10.1111/wvn.12147
56. Bauer, J, Morley, JE, Schols, AM, Ferrucci, L, Cruz-Jentoft, AJ, Dent, E, et al. Sarcopenia: a time for action. An SCWD position paper. J Cachexia Sarcopenia Muscle. (2019) 10:956–61. doi: 10.1002/jcsm.12483
57. Zhu, X, Wang, J, Lu, Y, Zhao, Y, Zhang, N, Wu, W, et al. Potential of Food Protein-Derived Bioactive Peptides against Sarcopenia: A Comprehensive Review. J Agric Food Chem. (2023) 71:5419–37. doi: 10.1021/acs.jafc.2c09094
58. Jayawardena, TU, Kim, S-Y, and Jeon, Y-J. Sarcopenia; functional concerns, molecular mechanisms involved, and seafood as a nutritional intervention–review article. Crit Rev Food Sci Nutr. (2023) 63:1983–2003. doi: 10.1080/10408398.2021.1969889
59. Lee, C-W, Chang, YB, Park, CW, Han, SH, Suh, HJ, and Ahn, Y. Protein hydrolysate from Spirulina platensis prevents dexamethasone-induced muscle atrophy via Akt/Foxo3 signaling in C2C12 myotubes. Marine Drugs. (2022) 20:365. doi: 10.3390/md20060365
60. van der Heijden, I, West, S, Monteyne, AJ, Finnigan, TJ, Abdelrahman, DR, Murton, AJ, et al. Algae Ingestion Increases Resting and Exercised Myofibrillar Protein Synthesis Rates to a Similar Extent as Mycoprotein in Young Adults. J Nutr. (2023). doi: 10.1016/j.tjnut.2023.08.035
61. Popkin, BM, and Gordon-Larsen, P. The nutrition transition: worldwide obesity dynamics and their determinants. Int J Obes. (2004) 28:S2–9. doi: 10.1038/sj.ijo.0802804
62. Visscher, TL, and Seidell, JC. The public health impact of obesity. Annu Rev Public Health. (2001) 22:355–75. doi: 10.1146/annurev.publhealth.22.1.355
63. Wan-Loy, C, and Siew-Moi, P. Marine algae as a potential source for anti-obesity agents. Marine Drugs. (2016) 14:222. doi: 10.3390/md14120222
64. Suryaningtyas, IT, and Je, J-Y. Bioactive peptides from food proteins as potential anti-obesity agents: Mechanisms of action and future perspectives. Trends Food Sci Technol. (2023) 138:141–52. doi: 10.1016/j.tifs.2023.06.015
65. Zhao, B, Cui, Y, Fan, X, Qi, P, Liu, C, Zhou, X, et al. Anti-obesity effects of Spirulina platensis protein hydrolysate by modulating brain-liver axis in high-fat diet fed mice. PLoS One. (2019) 14:e0218543. doi: 10.1371/journal.pone.0218543
66. Fan, X, Cui, Y, Zhang, R, and Zhang, X. Purification and identification of anti-obesity peptides derived from Spirulina platensis. J Funct Foods. (2018) 47:350–60. doi: 10.1016/j.jff.2018.05.066
67. Lee, HS, Park, HJ, and Kim, MK. Effect of Chlorella vulgaris on lipid metabolism in Wistar rats fed high fat diet. Nutr Res Pract. (2008) 2:204–10. doi: 10.4162/nrp.2008.2.4.204
68. Moradi, S, Ziaei, R, Foshati, S, Mohammadi, H, Nachvak, SM, and Rouhani, MH. Effects of Spirulina supplementation on obesity: A systematic review and meta-analysis of randomized clinical trials. Complemen Therap Med. (2019) 47:102211. doi: 10.1016/j.ctim.2019.102211
69. Bohórquez-Medina, SL, Bohórquez-Medina, AL, Zapata, VAB, Ignacio-Cconchoy, FL, Toro-Huamanchumo, CJ, Bendezu-Quispe, G, et al. Impact of spirulina supplementation on obesity-related metabolic disorders: A systematic review and meta-analysis of randomized controlled trials. NFS J. (2021) 25:21–30. doi: 10.1016/j.nfs.2021.09.003
70. Marles, RJ, Barrett, ML, Barnes, J, Chavez, ML, Gardiner, P, Ko, R, et al. United States pharmacopeia safety evaluation of Spirulina. Crit Rev Food Sci Nutr. (2011) 51:593–604. doi: 10.1080/10408391003721719
71. Mazokopakis, EE, Karefilakis, CM, Tsartsalis, AN, Milkas, AN, and Ganotakis, ES. Acute rhabdomyolysis caused by Spirulina (Arthrospira platensis). Phytomedicine. (2008) 15:525–7. doi: 10.1016/j.phymed.2008.03.003
72. Le, T-M, Knulst, AC, and Röckmann, H. Anaphylaxis to Spirulina confirmed by skin prick test with ingredients of Spirulina tablets. Food Chem Toxicol. (2014) 74:309–10. doi: 10.1016/j.fct.2014.10.024
73. Mills, KT, Stefanescu, A, and He, J. The global epidemiology of hypertension. Nat Rev Nephrol. (2020) 16:223–37. doi: 10.1038/s41581-019-0244-2
74. Chen, Y, Araghi, M, Bandosz, P, Shipley, MJ, Ahmadi-Abhari, S, Lobanov-Rostovsky, S, et al. Impact of hypertension prevalence trend on mortality and burdens of dementia and disability in England and Wales to 2060: a simulation modelling study. Lancet Healthy Long. (2023) 4:e470–7. doi: 10.1016/S2666-7568(23)00129-0
75. Dubow, J, and Fink, ME. Impact of hypertension on stroke. Curr Atheroscler Rep. (2011) 13:298–305. doi: 10.1007/s11883-011-0187-y
76. Tumuklu, MM, Erkorkmaz, U, and Öcal, A. The impact of hypertension and hypertension-related left ventricle hypertrophy on right ventricle function. Echocardiography. (2007) 24:374–84. doi: 10.1111/j.1540-8175.2007.00419.x
77. Schmieder, RE, Hilgers, KF, Schlaich, MP, and Schmidt, BM. Renin-angiotensin system and cardiovascular risk. Lancet. (2007) 369:1208–19. doi: 10.1016/S0140-6736(07)60242-6
78. Harnedy, PA, and FitzGerald, RJ. Bioactive proteins, peptides, and amino acids from macroalgae 1. J Phycol. (2011) 47:218–32. doi: 10.1111/j.1529-8817.2011.00969.x
79. Liu, J, Bai, X, and Fu, P. In silico and in vitro assessment of bioactive peptides from Arthrospira platensis phycobiliproteins for DPP-IV inhibitory activity, ACE inhibitory activity, and antioxidant activity. J Appl Phycol. (2022) 34:1497–511. doi: 10.1007/s10811-022-02732-z
80. Xie, J, Chen, X, Wu, J, Zhang, Y, Zhou, Y, Zhang, L, et al. Antihypertensive effects, molecular docking study, and isothermal titration calorimetry assay of angiotensin I-converting enzyme inhibitory peptides from Chlorella vulgaris. J Agric Food Chem. (2018) 66:1359–68. doi: 10.1021/acs.jafc.7b04294
81. Fallah, AA, Sarmast, E, Dehkordi, SH, Engardeh, J, Mahmoodnia, L, Khaledifar, A, et al. Effect of Chlorella supplementation on cardiovascular risk factors: A meta-analysis of randomized controlled trials. Clin Nutr. (2018) 37:1892–901. doi: 10.1016/j.clnu.2017.09.019
82. Chatterjee, S, Khunti, K, and Davies, MJ. Type 2 diabetes. Lancet. (2017) 389:2239–51. doi: 10.1016/S0140-6736(17)30058-2
83. Fowler, MJ. Microvascular and macrovascular complications of diabetes. Clin Diabetes. (2008) 26:77–82. doi: 10.2337/diaclin.26.2.77
84. Chaudhury, A, Duvoor, C, Reddy Dendi, VS, Kraleti, S, Chada, A, Ravilla, R, et al. Clinical review of antidiabetic drugs: implications for type 2 diabetes mellitus management. Front Endocrinol. (2017) 8:6. doi: 10.3389/fendo.2017.00006
85. Kehinde, BA, and Sharma, P. Recently isolated antidiabetic hydrolysates and peptides from multiple food sources: A review. Crit Rev Food Sci Nutr. (2020) 60:322–40. doi: 10.1080/10408398.2018.1528206
86. Aiello, G, Li, Y, Boschin, G, Bollati, C, Arnoldi, A, and Lammi, C. Chemical and biological characterization of spirulina protein hydrolysates: Focus on ACE and DPP-IV activities modulation. J Funct Foods. (2019) 63:103592. doi: 10.1016/j.jff.2019.103592
87. Hu, S, Fan, X, Qi, P, and Zhang, X. Identification of anti-diabetes peptides from Spirulina platensis. J Funct Foods. (2019) 56:333–41. doi: 10.1016/j.jff.2019.03.024
88. Zhu, Q, Chen, X, Wu, J, Zhou, Y, Qian, Y, Fang, M, et al. Dipeptidyl peptidase IV inhibitory peptides from Chlorella vulgaris: In silico gastrointestinal hydrolysis and molecular mechanism. Eur Food Res Technol. (2017) 243:1739–48. doi: 10.1007/s00217-017-2879-1
89. McLaughlin, C, Harnedy-Rothwell, PA, Lafferty, R, Sharkey, S, Parthsarathy, V, Allsopp, PJ, et al. Macroalgal protein hydrolysates from Palmaria palmata influence the ‘incretin effect’in vitro via DPP-4 inhibition and upregulation of insulin, GLP-1 and GIP secretion. Eur J Nutr. (2021) 60:4439–52. doi: 10.1007/s00394-021-02583-3
90. Kumar, S, Narwal, S, Kumar, V, and Prakash, O. α-glucosidase inhibitors from plants: A natural approach to treat diabetes. Pharmacogn Rev. (2011) 5:19–29. doi: 10.4103/0973-7847.79096
91. Glass, CK, Saijo, K, Winner, B, Marchetto, MC, and Gage, FH. Mechanisms underlying inflammation in neurodegeneration. Cell. (2010) 140:918–34. doi: 10.1016/j.cell.2010.02.016
92. Thorpe, KE, Levey, AI, and Thomas, J. (2021). US burden of neurodegenerative disease. Partnership to Fight Chronic Disease (PFCD). 1-13.
93. Batista, P, and Pereira, A. Quality of life in patients with neurodegenerative diseases. Journal of Neurology and Neuroscience. (2016) 7, 1–7. doi: 10.21767/2171-6625.100074
94. Klietz, M. Caregiver Burden in Movement Disorders and Neurodegenerative Diseases. MDPI. (2022) 12:1184. doi: 10.3390/brainsci12091184
95. Zahra, W, Rai, SN, Birla, H, Singh, SS, Dilnashin, H, Rathore, AS, et al. The global economic impact of neurodegenerative diseases: Opportunities and challenges. Bioecon Sustain Dev. (2020):333–45. doi: 10.1007/978-981-13-9431-7_17
96. Kumar, S, and Malviya, R. Protein and peptide from blue food for neurological disorder: Advances and prospective. Trends Food Sci Technol. (2023) 143:104277. doi: 10.1016/j.tifs.2023.104277
97. Zhou, T, Liu, Y, Wang, Q, Dou, Q, Li, X, Pan, Y, et al. Spirulina platensis alleviates high fat diet-induced cognitive impairment in mice via the gut-brain axis. J Funct Foods. (2021) 86:104706. doi: 10.1016/j.jff.2021.104706
98. Tamtaji, OR, Heidari-soureshjani, R, Asemi, Z, and Kouchaki, E. The effects of spirulina intake on clinical and metabolic parameters in Alzheimer's disease: A randomized, double-blind, controlled trial. Phytother Res. (2023) 37:2957–64. doi: 10.1002/ptr.7791
99. Uthman, OA. Global, regional, and national life expectancy, all-cause and cause-specific mortality for 249 causes of death, 1980–2015: a systematic analysis for the Global Burden of Disease Study 2015. Lancet. (2016) 388:1459–544. doi: 10.1016/S0140-6736(16)31012-1
100. Miller, KD, Nogueira, L, Mariotto, AB, Rowland, JH, Yabroff, KR, Alfano, CM, et al. Cancer treatment and survivorship statistics, 2019. CA Cancer J Clin. (2019) 69:363–85. doi: 10.3322/caac.21565
101. Cragg, GM, Grothaus, PG, and Newman, DJ. Impact of natural products on developing new anti-cancer agents. Chem Rev. (2009) 109:3012–43. doi: 10.1021/cr900019j
102. Da Rocha, AB, Lopes, RM, and Schwartsmann, G. Natural products in anticancer therapy. Curr Opin Pharmacol. (2001) 1:364–9. doi: 10.1016/S1471-4892(01)00063-7
103. Sheih, I-C, Fang, TJ, Wu, T-K, and Lin, P-H. Anticancer and antioxidant activities of the peptide fraction from algae protein waste. J Agric Food Chem. (2010) 58:1202–7. doi: 10.1021/jf903089m
104. Wang, X, and Zhang, X. Separation, antitumor activities, and encapsulation of polypeptide from Chlorella pyrenoidosa. Biotechnol Prog. (2013) 29:681–7. doi: 10.1002/btpr.1725
105. Wang, Z, and Zhang, X. Inhibitory effects of small molecular peptides from Spirulina (Arthrospira) platensis on cancer cell growth. Food Funct. (2016) 7:781–8. doi: 10.1039/C5FO01186H
106. Lin, X, Dong, L, Yan, Q, Dong, Y, Wang, L, and Wang, F. Preparation and Characterization of an Anticancer Peptide from Oriental Tonic Food Enteromorpha prolifera. Food Secur. (2022) 11:3507. doi: 10.3390/foods11213507
107. Wang, Z, and Zhang, X. Characterization and antitumor activity of protein hydrolysates from Arthrospira platensis (Spirulina platensis) using two-step hydrolysis. J Appl Phycol. (2016) 28:3379–85. doi: 10.1007/s10811-016-0881-9
108. Wang, Z, and Zhang, X. Isolation and identification of anti-proliferative peptides from Spirulina platensis using three-step hydrolysis. J Sci Food Agric. (2017) 97:918–22. doi: 10.1002/jsfa.7815
109. Matassa, S, Boon, N, Pikaar, I, and Verstraete, W. Microbial protein: future sustainable food supply route with low environmental footprint. Microb Biotechnol. (2016) 9:568–75. doi: 10.1111/1751-7915.12369
110. Lange, L. The importance of fungi for a more sustainable future on our planet. Fungal Biol Rev. (2010) 24:90–2. doi: 10.1016/j.fbr.2010.12.002
112. Hashempour-Baltork, F, Khosravi-Darani, K, Hosseini, H, Farshi, P, and Reihani, SFS. Mycoproteins as safe meat substitutes. J Clean Prod. (2020) 253:119958. doi: 10.1016/j.jclepro.2020.119958
113. Finnigan, T, Mach, K, and Edlin, A. (2024). Mycoprotein: a healthy new protein with a low environmental impact. Sustainable protein sources : Elsevier. p. 539–66.
114. Wiebe, MG. QuornTM Myco-protein-Overview of a successful fungal product. Mycologist. (2004) 18:17–20. doi: 10.1017/S0269915X04001089
115. Derbyshire, E, and Ayoob, K-T. Mycoprotein: Nutritional and health properties. Nutr Today. (2019) 54:7–15. doi: 10.1097/NT.0000000000000316
116. Cherta-Murillo, A, Lett, AM, Frampton, J, Chambers, ES, Finnigan, TJ, and Frost, GS. Effects of mycoprotein on glycaemic control and energy intake in humans: a systematic review. Br J Nutr. (2020) 123:1321–32. doi: 10.1017/S0007114520000756
117. Bell, V, Silva, C, Guina, J, and Fernandes, T. Mushrooms as future generation healthy foods. Front Nut. (2022) 9:1050099. doi: 10.3389/fnut.2022.1050099
118. Hamza, A, Mylarapu, A, Krishna, KV, and Kumar, DS. An insight into the nutritional and medicinal value of edible mushrooms: A natural treasury for human health. J Biotechnol. (2024) 381:86–99. doi: 10.1016/j.jbiotec.2023.12.014
119. Bottin, J, Cropp, E, Finnigan, T, and Hogban, A. Mycoprotein reduces energy intake and improves insulin sensitivity compared to chicken. Obes Facts. (2012) 5:55–79.
120. Bottin, JH, Swann, JR, Cropp, E, Chambers, ES, Ford, HE, Ghatei, MA, et al. Mycoprotein reduces energy intake and postprandial insulin release without altering glucagon-like peptide-1 and peptide tyrosine-tyrosine concentrations in healthy overweight and obese adults: a randomised-controlled trial. Br J Nutr. (2016) 116:360–74. doi: 10.1017/S0007114516001872
121. Dunlop, MV, Kilroe, SP, Bowtell, JL, Finnigan, TJ, Salmon, DL, and Wall, BT. Mycoprotein represents a bioavailable and insulinotropic non-animal-derived dietary protein source: a dose–response study. Br J Nutr. (2017) 118:673–85. doi: 10.1017/S0007114517002409
122. Holmes, D. Medicinal mushroom reduces obesity by modulating microbiota. Nature Reviews. Endocrinology. (2015) 11:504. doi: 10.1038/nrendo.2015.114
123. Pavis, GF, Iniesta, RR, Roper, H, Theobald, HE, Derbyshire, EJ, Finnigan, TJ, et al. A four-week dietary intervention with mycoprotein-containing food products reduces serum cholesterol concentrations in community-dwelling, overweight adults: a randomised controlled trial. Clin Nutr. (2024) 43:649–59. doi: 10.1016/j.clnu.2024.01.023
124. Ruxton, CH, and McMillan, B. The impact of mycoprotein on blood cholesterol levels: a pilot study. Br Food J. (2010) 112:1092–101. doi: 10.1108/00070701011080221
125. Turnbull, WH, Leeds, AR, and Edwards, GD. Effect of mycoprotein on blood lipids. Am J Clin Nutr. (1990) 52:646–50. doi: 10.1093/ajcn/52.4.646
126. Mustafa, F, Chopra, H, Baig, AA, Avula, SK, Kumari, S, Mohanta, TK, et al. Edible mushrooms as novel myco-therapeutics: effects on lipid level, obesity, and BMI. J Fungi. (2022) 8:211. doi: 10.3390/jof8020211
127. Kyo-Chul, K, and Hyung-Eun, Y. Production and characterization of antihypertensive angiotensin I-converting enzyme inhibitor from Pholiota adiposa. J Microbiol Biotechnol. (2006) 16:757–63.
128. Jang, J-H, Jeong, S-C, Kim, J-H, Lee, Y-H, Ju, Y-C, and Lee, J-S. Characterisation of a new antihypertensive angiotensin I-converting enzyme inhibitory peptide from Pleurotus cornucopiae. Food Chem. (2011) 127:412–8. doi: 10.1016/j.foodchem.2011.01.010
129. Kang, M-G, Kim, Y-H, Bolormaa, Z, Kim, M-K, Seo, G-S, and Lee, J-S. Characterization of an antihypertensive angiotensin I-converting enzyme inhibitory peptide from the edible mushroom Hypsizygus marmoreus. Biomed Res Int. (2013) 2013:283964. doi: 10.1155/2013/283964
130. Lau, CC, Abdullah, N, Shuib, AS, and Aminudin, N. Novel angiotensin I-converting enzyme inhibitory peptides derived from edible mushroom Agaricus bisporus (JE Lange) Imbach identified by LC–MS/MS. Food Chem. (2014) 148:396–401. doi: 10.1016/j.foodchem.2013.10.053
131. Lee, DH, Kim, JH, Park, JS, Choi, YJ, and Lee, JS. Isolation and characterization of a novel angiotensin I-converting enzyme inhibitory peptide derived from the edible mushroom Tricholoma giganteum. Peptides. (2004) 25:621–7. doi: 10.1016/j.peptides.2004.01.015
132. Wu, Q, Li, Y, Peng, K, Wang, X-L, Ding, Z, Liu, L, et al. Isolation and characterization of three antihypertension peptides from the mycelia of Ganoderma lucidum (Agaricomycetes). J Agric Food Chem. (2019) 67:8149–59. doi: 10.1021/acs.jafc.9b02276
133. Paisansak, S, Sangtanoo, P, Srimongkol, P, Saisavoey, T, Reamtong, O, Choowongkomon, K, et al. Angiotensin-I converting enzyme inhibitory peptide derived from the shiitake mushroom (Lentinula edodes). J Food Sci Technol. (2021) 58:85–97. doi: 10.1007/s13197-020-04517-z
134. West, S, Monteyne, AJ, Whelehan, G, van der Heijden, I, Abdelrahman, DR, Murton, AJ, et al. Ingestion of mycoprotein, pea protein, and their blend support comparable postexercise myofibrillar protein synthesis rates in resistance-trained individuals. Am J Physiol Endocrinol Metabol. (2023) 325:E267–79. doi: 10.1152/ajpendo.00166.2023
135. Monteyne, AJ, Coelho, MOC, Porter, C, Abdelrahman, DR, Jameson, TSO, Finnigan, TJA, et al. Branched-Chain Amino Acid Fortification Does Not Restore Muscle Protein Synthesis Rates following Ingestion of Lower- Compared with Higher-Dose Mycoprotein. J Nutr. (2020) 150:2931–41. doi: 10.1093/jn/nxaa251
136. Monteyne, AJ, Coelho, MO, Porter, C, Abdelrahman, DR, Jameson, TS, Jackman, SR, et al. Mycoprotein ingestion stimulates protein synthesis rates to a greater extent than milk protein in rested and exercised skeletal muscle of healthy young men: a randomized controlled trial. Am J Clin Nutr. (2020) 112:318–33. doi: 10.1093/ajcn/nqaa092
137. West, S, Monteyne, AJ, Whelehan, G, Abdelrahman, DR, Murton, AJ, Finnigan, TJA, et al. Mycoprotein ingestion within or without its wholefood matrix results in equivalent stimulation of myofibrillar protein synthesis rates in resting and exercised muscle of young men. Br J Nutr. (2023) 130:20–32. doi: 10.1017/S0007114522003087
138. Williamson, DA, Geiselman, PJ, Lovejoy, J, Greenway, F, Volaufova, J, Martin, CK, et al. Effects of consuming mycoprotein, tofu or chicken upon subsequent eating behaviour, hunger and safety. Appetite. (2006) 46:41–8. doi: 10.1016/j.appet.2005.10.007
139. Burley, V, Paul, A, and Blundell, J. Influence of a high-fibre food (myco-protein^*) on appetite: effects on satiation (within meals) and satiety (following meals). Eur J Clin Nutr. (1993) 47:409–18.
140. Jongema, Y. List of edible insects of the world. Wageningen, the Netherlands: Wageningen University & Research (2017).
141. Malla, N, Nørgaard, JV, Lærke, HN, Heckmann, L-HL, and Roos, N. Some insect species are good-quality protein sources for children and adults: digestible indispensable amino acid score (DIAAS) determined in growing pigs. J Nutr. (2022) 152:1042–51. doi: 10.1093/jn/nxac019
142. Rumpold, BA, and Schlüter, OK. Nutritional composition and safety aspects of edible insects. Mol Nutr Food Res. (2013) 57:802–23. doi: 10.1002/mnfr.201200735
143. Latunde-Dada, GO, Yang, W, and Vera, AM. In vitro iron availability from insects and sirloin beef. J Agric Food Chem. (2016) 64:8420–4. doi: 10.1021/acs.jafc.6b03286
144. Schmidt, A, Call, L-M, Macheiner, L, and Mayer, HK. Determination of vitamin B12 in four edible insect species by immunoaffinity and ultra-high performance liquid chromatography. Food Chem. (2019) 281:124–9. doi: 10.1016/j.foodchem.2018.12.039
145. Oibiokpa, FI, Akanya, H, Jigam, A, and Saidu, A. Nutrient and antinutrient compositions of some edible insect species in Northern Nigeria. Fount J Nat Appl Sci. (2017) 6, 9–24. doi: 10.53704/fujnas.v6i1.159
146. Tang, C, Yang, D, Liao, H, Sun, H, Liu, C, Wei, L, et al. Edible insects as a food source: a review. Food Prod Process Nutr. (2019) 1:1–13. doi: 10.1186/s43014-019-0008-1
147. Oonincx, DG, and De Boer, IJ. Environmental impact of the production of mealworms as a protein source for humans–a life cycle assessment. PLoS One. (2012) 7:e51145. doi: 10.1371/journal.pone.0051145
148. Veldkamp, T, Van Duinkerken, G, van Huis, A, Lakemond, C, Ottevanger, E, Bosch, G, et al. (2012). Insects as a sustainable feed ingredient in pig and poultry diets: a feasibility study= Insecten als duurzame diervoedergrondstof in varkens-en pluimveevoeders: een haalbaarheidsstudie. Wageningen UR Livestock Research. Report No.: 1570-8616.
149. Hermans, WJ, Senden, JM, Churchward-Venne, TA, Paulussen, KJ, Fuchs, CJ, Smeets, JS, et al. Insects are a viable protein source for human consumption: from insect protein digestion to postprandial muscle protein synthesis in vivo in humans: a double-blind randomized trial. Am J Clin Nutr. (2021) 114:934–44. doi: 10.1093/ajcn/nqab115
150. Lanng, SK, Oxfeldt, M, Pedersen, SS, Johansen, FT, Risikesan, J, Lejel, T, et al. Influence of protein source (cricket, pea, whey) on amino acid bioavailability and activation of the mTORC1 signaling pathway after resistance exercise in healthy young males. Eur J Nutr. (2023) 62:1295–308. doi: 10.1007/s00394-022-03071-y
151. Dai, J, Lov, J, Martin-Arrowsmith, PW, Gritsas, A, and Churchward-Venne, TA. The acute effects of insect vs. beef-derived protein on postprandial plasma aminoacidemia, appetite hormones, appetite sensations, and energy intake in healthy young men. Eur J Clin Nutr. (2022) 76:1548–56. doi: 10.1038/s41430-022-01157-8
152. Vangsoe, MT, Thogersen, R, Bertram, HC, Heckmann, LL, and Hansen, M. Ingestion of insect protein isolate enhances blood amino acid concentrations similar to soy protein in a human trial. Nutrients. (2018) 10:1357. doi: 10.3390/nu10101357
153. Rumpold, BA, and Schlüter, O. Insect-based protein sources and their potential for human consumption: Nutritional composition and processing. Anim Front. (2015) 5:20–4.
154. Van Huis, A. Nutrition and health of edible insects. Curr Opin Clin Nut Metabol Care. (2020) 23:228–31. doi: 10.1097/MCO.0000000000000641
155. Churchward-Venne, TA, Pinckaers, PJ, van Loon, JJ, and van Loon, LJ. Consideration of insects as a source of dietary protein for human consumption. Nutr Rev. (2017) 75:1035–45. doi: 10.1093/nutrit/nux057
156. Wall, BT, Gorissen, SH, Pennings, B, Koopman, R, Groen, BB, Verdijk, LB, et al. Aging is accompanied by a blunted muscle protein synthetic response to protein ingestion. PLoS One. (2015) 10:e0140903. doi: 10.1371/journal.pone.0140903
157. Zielińska, E, Karaś, M, and Jakubczyk, A. Antioxidant activity of predigested protein obtained from a range of farmed edible insects. Int J Food Sci Technol. (2017) 52:306–12. doi: 10.1111/ijfs.13282
158. Seo, M, Goo, T-W, Chung, MY, Baek, M, Hwang, J-S, Kim, M-A, et al. Tenebrio molitor larvae inhibit adipogenesis through AMPK and MAPKs signaling in 3T3-L1 adipocytes and obesity in high-fat diet-induced obese mice. Int J Mol Sci. (2017) 18:518. doi: 10.3390/ijms18030518
159. Kim, J, Yun, E-Y, Park, S-W, Goo, T-W, and Seo, M. Allomyrina dichotoma larvae regulate food intake and body weight in high fat diet-induced obese mice through mTOR and Mapk signaling pathways. Nutrients. (2016) 8:100. doi: 10.3390/nu8020100
160. Cito, A, Botta, M, Francardi, V, and Dreassi, E. Insects as source of angiotensin converting enzyme inhibitory peptides. J Insects Food Feed. (2017) 3:231–40. doi: 10.3920/JIFF2017.0017
161. Laybutt, D, Preston, A, Åkerfeldt, M, Kench, J, Busch, A, Biankin, A, et al. Endoplasmic reticulum stress contributes to beta cell apoptosis in type 2 diabetes. Diabetologia. (2007) 50:752–63. doi: 10.1007/s00125-006-0590-z
162. Zielińska, E, Karaś, M, Baraniak, B, and Jakubczyk, A. Evaluation of ACE, α-glucosidase, and lipase inhibitory activities of peptides obtained by in vitro digestion of selected species of edible insects. Eur Food Res Technol. (2020) 246:1361–9. doi: 10.1007/s00217-020-03495-y
163. Lee, HJ, Lee, H-S, Choi, JW, Ra, KS, Kim, J-M, and Suh, HJ. Novel tripeptides with α-glucosidase inhibitory activity isolated from silk cocoon hydrolysate. J Agric Food Chem. (2011) 59:11522–5. doi: 10.1021/jf202686m
164. Wu, Q, Jia, J, Yan, H, Du, J, and Gui, Z. A novel angiotensin-І converting enzyme (ACE) inhibitory peptide from gastrointestinal protease hydrolysate of silkworm pupa (Bombyx mori) protein: biochemical characterization and molecular docking study. Peptides. (2015) 68:17–24. doi: 10.1016/j.peptides.2014.07.026
165. Chen, F, Jiang, H, Lu, Y, Chen, W, and Huang, G. Identification and in silico analysis of antithrombotic peptides from the enzymatic hydrolysates of Tenebrio molitor larvae. Eur Food Res Technol. (2019) 245:2687–95. doi: 10.1007/s00217-019-03381-2
166. Ekop, E, Udoh, A, and Akpan, P. Proximate and anti-nutrient composition of four edible insects in Akwa Ibom State, Nigeria. World J Appl Sci Technol. (2010) 2:224–31.
167. Sailo, S, Bhagawati, S, Sarmah, S, and Pathak, K. Nutritional and antinutritional properties of few common edible insect species of Assam. J Entomol Zool Stud. (2020) 8:1785–91.
168. de Gier, S, and Verhoeckx, K. Insect (food) allergy and allergens. Mol Immunol. (2018) 100:82–106. doi: 10.1016/j.molimm.2018.03.015
169. Ribeiro, J, Sousa-Pinto, B, Fonseca, J, Fonseca, SC, and Cunha, L. Edible insects and food safety: allergy. J Insects Food Feed. (2021) 7:833–47. doi: 10.3920/JIFF2020.0065
170. Wu, X, He, K, Velickovic, TC, and Liu, Z. Nutritional, functional, and allergenic properties of silkworm pupae. Food Sci Nut. (2021) 9:4655–65. doi: 10.1002/fsn3.2428
171. Garofalo, C, Milanović, V, Cardinali, F, Aquilanti, L, Clementi, F, and Osimani, A. Current knowledge on the microbiota of edible insects intended for human consumption: A state-of-the-art review. Food Res Int. (2019) 125:108527. doi: 10.1016/j.foodres.2019.108527
172. Van der Fels-Klerx, H, Camenzuli, L, Belluco, S, Meijer, N, and Ricci, A. Food safety issues related to uses of insects for feeds and foods. Compr Rev Food Sci Food Saf. (2018) 17:1172–83. doi: 10.1111/1541-4337.12385
173. Meyer, A, Meijer, N, Hoek-Van den Hil, E, and Van der Fels-Klerx, H. Chemical food safety hazards of insects reared for food and feed. J Insects Food Feed. (2021) 7:823–31. doi: 10.3920/JIFF2020.0085
174. Poma, G, Cuykx, M, Amato, E, Calaprice, C, Focant, JF, and Covaci, A. Evaluation of hazardous chemicals in edible insects and insect-based food intended for human consumption. Food Chem Toxicol. (2017) 100:70–9. doi: 10.1016/j.fct.2016.12.006
175. Poma, G, Yin, S, Tang, B, Fujii, Y, Cuykx, M, and Covaci, A. Occurrence of selected organic contaminants in edible insects and assessment of their chemical safety. Environ Health Perspect. (2019) 127:127009. doi: 10.1289/EHP5782
176. Okaiyeto, SA, Yu, SH, Deng, LZ, Wang, QH, Sutar, PP, Wang, H, et al. How to enhance the acceptability of insects food—A review. Food Front. (2024) 5, 311–328. doi: 10.1002/fft2.349
177. Kumar, J, Ramlal, A, Mallick, D, and Mishra, V. An overview of some biopesticides and their importance in plant protection for commercial acceptance. Plants. (2021) 10:1185. doi: 10.3390/plants10061185
178. Hartmann, C, and Siegrist, M. Insects as food: Perception and acceptance. Findings from current research. Ernahrungs-Umschau. (2017) 64:44–50. doi: 10.4455/eu.2017.010
179. Lammers, P, Ullmann, LM, and Fiebelkorn, F. Acceptance of insects as food in Germany: Is it about sensation seeking, sustainability consciousness, or food disgust? Food Qual Prefer. (2019) 77:78–88. doi: 10.1016/j.foodqual.2019.05.010
180. Onwezen, MC, Bouwman, EP, Reinders, MJ, and Dagevos, H. A systematic review on consumer acceptance of alternative proteins: Pulses, algae, insects, plant-based meat alternatives, and cultured meat. Appetite. (2021) 159:105058. doi: 10.1016/j.appet.2020.105058
Keywords: algae, fungi, insect, protein, peptides, cancer, cardiovascular disease, dementia
Citation: Yimam MA, Andreini M, Carnevale S and Muscaritoli M (2024) The role of algae, fungi, and insect-derived proteins and bioactive peptides in preventive and clinical nutrition. Front. Nutr. 11:1461621. doi: 10.3389/fnut.2024.1461621
Edited by:
Johannes le Coutre, University of New South Wales, AustraliaReviewed by:
Barbara Korousic Seljak, Institut Jožef Stefan (IJS), SloveniaAhmed A. Zaky, National Research Centre, Egypt
Copyright © 2024 Yimam, Andreini, Carnevale and Muscaritoli. This is an open-access article distributed under the terms of the Creative Commons Attribution License (CC BY). The use, distribution or reproduction in other forums is permitted, provided the original author(s) and the copyright owner(s) are credited and that the original publication in this journal is cited, in accordance with accepted academic practice. No use, distribution or reproduction is permitted which does not comply with these terms.
*Correspondence: Mohammed Ahmed Yimam, bW9oYW1tZWQueWltYW1AaXVzc3BhdmlhLml0; Maurizio Muscaritoli, bWF1cml6aW8ubXVzY2FyaXRvbGlAdW5pcm9tYTEuaXQ=