- 1Centro de Investigación Genética y Genómica, Facultad de Ciencias de la Salud Eugenio Espejo, Universidad UTE, Quito, Ecuador
- 2Universidad Espíritu Santo, Samborondón, Ecuador
- 3Centro de Investigación de Salud Pública y Epidemiología Clínica (CISPEC), Universidad UTE, Quito, Ecuador
Obesity, a chronic global health problem, is associated with an increase in various comorbidities, such as cardiovascular disease, type 2 diabetes mellitus, hypertension, and certain types of cancer. The increasing global prevalence of obesity requires research into new therapeutic strategies. Glucagon-like peptide-1 receptor agonists, specifically semaglutide and liraglutide, designed for type 2 diabetes mellitus treatment, have been explored as drugs for the treatment of obesity. This minireview describes the molecular mechanisms of semaglutide and liraglutide in different metabolic pathways, and its mechanism of action in processes such as appetite regulation, insulin secretion, glucose homeostasis, energy expenditure, and lipid metabolism. Finally, several clinical trial outcomes are described to show the safety and efficacy of these drugs in obesity management.
Introduction
Obesity is a chronic disease, and its prevalence has increased worldwide (1). According to the World Health Organization (WHO), there were approximately 1.9 billion overweight adults in 2016, of which 650 million were obese (2, 3). Genetic, environmental, and behavioral interactions have been linked to obesity; thus, the consumption of high-calorie foods associated with sedentary lifestyles increases the number of obese people in the world (4). Therefore, obesity can lead to a high risk of cardiovascular diseases, type 2 diabetes mellitus (DM2), hypertension, and some types of cancer. In addition, other diseases, such as dyslipidemia and even depression, are associated with obesity (5, 6).
Despite the increase in weight management approaches, the pandemic of obesity is still rising. However, strategies to control this phenomenon have primarily focused on physical exercise, behavioral factors, surgery (including bariatric surgery), and pharmaceutical interventions (7).
Pharmacological strategies have been fundamental for reducing the obesity pandemic (8). In this context, the group of glucagon-like peptide 1 receptor agonists (GLP-1RA), which mimic the natural hormone glucagon-like peptide 1 (GLP-1), has been mainly used for the treatment of DM2 (9). In addition, studies have shown that these drugs can have pleiotropic effects such as blood pressure lowering, weight reduction, endothelial protection, and insulin sensitivity (Figure 1). For instance, semaglutide and liraglutide, two GLP-1RA agonist drugs, have been associated with weight loss and body weight maintenance (10–12).
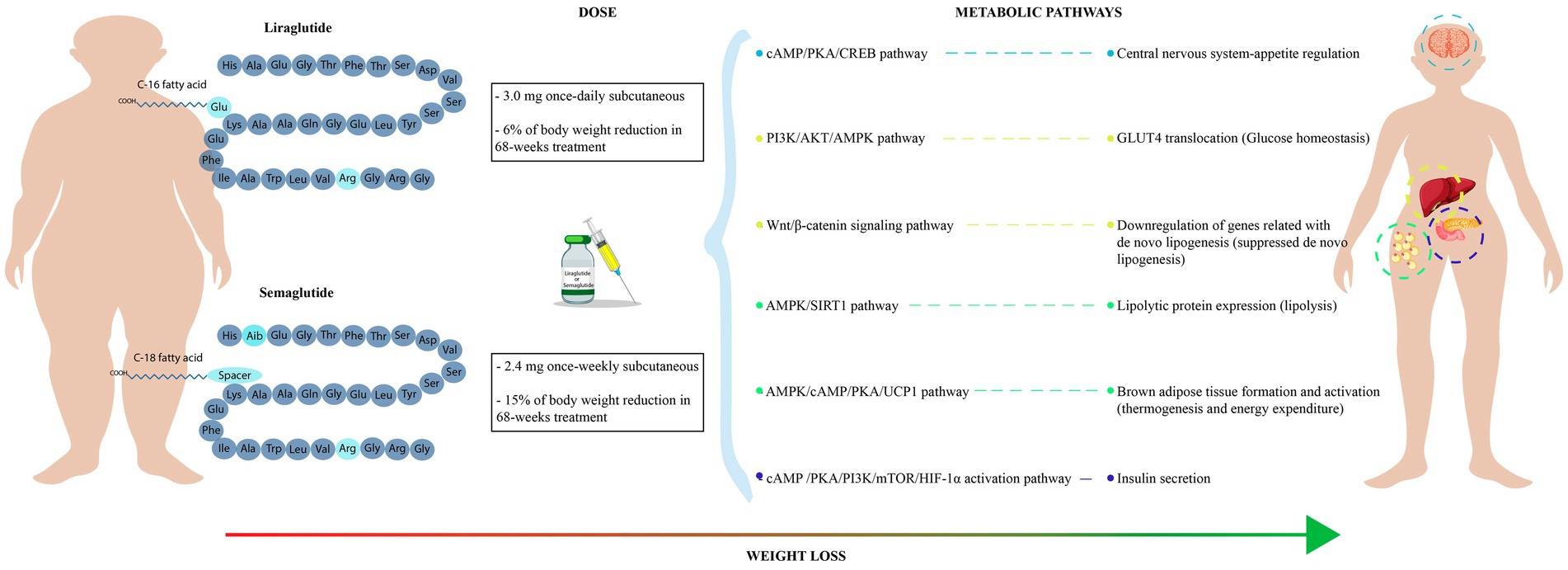
Figure 1. Metabolic outcomes of semaglutide and liraglutide treatment. It shows the molecular structure and recommended dose of GLP-1RA (semaglutide and liraglutide). Mechanisms of action of semaglutide and liraglutide in several metabolic pathways related with body weight loss.
The present review aims to elucidate and describe the molecular mechanisms of the GLP-1RA drugs, semaglutide and liraglutide, and their effectiveness and potential as a therapeutic option against obesity.
Methodology
The scientific papers search was performed in Google Scholar and PubMed databases. The following individual and combined search terms were used: “GLP-1,” “Semaglutide and Liraglutide,” “molecular mechanism,” “lipid metabolism,” “insulin metabolism,” “glucose homeostasis,” “energy expenditure” “obesity treatment,” “type 2 diabetes mellitus,” “side effects,” “clinical trials,” “treatment option,” “fatty liver disease,” “hypertension,” “neurodegenerative diseases,” “cardiovascular diseases,” “therapeutic options.” Only articles published in the last 10 years are included, although older pivotal studies were added.
Molecular mechanisms of semaglutide and liraglutide
GLP-1 receptor activation
GLP-1 is an incretin hormone secreted by enteroendocrine L-cells and α-cells in the pancreas and central nervous system. GLP-1 activates insulin secretion in response to elevated plasma glucose levels (13). In addition, GLP-1 activation increases neogenesis and proliferation while decreasing apoptosis of pancreatic β-cells (14). This GLP-1 hormone binds to the G protein-coupled GLP-1 receptor (GLP-1R) and activates key intracellular metabolic pathways. For instance, the GLP-1 hormone can trigger the adenylate cyclase (AC) metabolic pathway, leading to elevated levels of intracellular cyclic AMP (cAMP), subsequently activating the protein kinase A (PKA). PKA promotes exocytosis of insulin-containing vesicles from pancreatic β-cells and increases glucose-dependent insulin secretion (15). Conversely, GLP-1 can inhibit glucagon release from pancreatic α-cells, decreasing liver production of glucose (16).
Furthermore, cAMP-dependent mechanisms acting through PKA, and the exchange protein directly activated by cAMP (EPAC) inhibit ATP-dependent potassium channels and increase the activity of calcium channels. The influx of more calcium ions into the cell activates glucose-induced membrane depolarization and elevated cell sensitivity to glucose (17).
In addition, GLP-1 can stimulate the epidermal growth factor (EGF), triggering the phosphatidylinositol-3 kinase (PI3K), which activates transcription factors associated with β-cell growth while inhibiting those linked to β-cell apoptosis (18).
Moreover, these mechanisms are a consequence of Gβγ signaling, facilitated by the interaction of GLP-1R with distinct G proteins, which can activate various metabolic pathways using the identical ligand. Consequently, the metabolic pathways initiated by Gα proteins within GLP-1R involve cAMP activation, extracellular signal-regulated kinases 1 and 2 (ERK 1/2) and increased intracellular calcium levels (17, 19).
Chemical structure of semaglutide and liraglutide and its interaction with GLP-1 receptor
One of the pharmacological approaches against diseases like diabetes and obesity involves prolonging the time of action of GLP-1. For example, some GLP-1RA drugs, such as semaglutide and liraglutide, have already been approved by the United States Food and Drug Administration (FDA) for DM2 and chronic weight control (20).
Semaglutide and liraglutide were derived from the native structure of GLP-1. Semaglutide is a drug with 94% homology with human GLP-1. Its chemical structure consists of 31 amino acids with two amino acid substitutions (Aib8 and Arg34). Notably, the substitution at position 8 reduces susceptibility to degradation by dipeptidyl peptidase-4 (DPP-4) (21, 22). Conversely, liraglutide, another GLP-1RA, has 97% homology with human GLP-1. Its chemical structure, unlike semaglutide, has an Arginine-Lysine substitution at position 34 and a fatty acid residue is attached to Lysine 26. This modification enhances albumin binding, contributing to an increase in the half-life of the drug to 11 to 13 h (14).
Both, semaglutide and liraglutide, by binding to GLP-1R, can activate cAMP and PKA signaling cascades. As a result, there is an increased insulin secretion in the pancreatic β-cells and suppression of glucagon release. Furthermore, these pathways have also been linked to appetite regulation, modulation of gastric emptying, and cardiovascular effects (23, 24).
Semaglutide and liraglutide effects on central nervous system regulation
GLP-1 and GLP-1RA are involved in the activation of various central nervous system (CNS) processes, including satiety, thermogenesis, blood pressure, neurogenesis, and inflammation reduction (15). GLP-1 increases the expression of the cAMP, PKA, and CREB metabolic pathways. Additionally, GLP-1 modulates the phosphorylation of AKT, ERK, GSK-3B, and mTOR to increase cell viability and growth (25, 26).
Obesity has been associated with chronic inflammation, and GLP-1RA can modulate these inflammatory processes (27). GLP-1R is present in macrophages, lymphocytes, and monocytes which regulate immune cell signaling by suppressing proinflammatory cytokines such as TNF-α, IL-6, and IL-1β (28). In addition, studies have shown that GLP-1RAs decrease oxidative stress and improve endothelial function contributing to a reduction of inflammation in obesity (29).
Moreover, GLP-1 promotes neurogenesis by reducing inflammation and decreasing the expression of proinflammatory cytokines IL-6, IL-10, and microglial activation (25). GLP-1R is present in different brain regions, such as the cerebral cortex, thalamus, hypothalamus, substantia nigra, and cerebellum, having crossed the blood–brain barrier, influencing processes like appetite control and satiety (15). The GLP-1RA drugs semaglutide and liraglutide, due to their longer half-lives, may have more prolonged effects in the CNS and regulate appetite metabolic processes (30).
Studies have observed that GLP-1RA decreases food intake, slows gastric emptying, and promotes the release of hormones such as leptin and peptide YY, involved in satiety (7). The hormone leptin is fundamental in the regulation of body weight since it suppresses the orexigenic (appetite-inducing) pathway and activates the anorexigenic (satiety-inducing) pathway (8). Therefore, GLP-1RA may target different pathways to control appetite and inflammatory processes which would contribute to the treatment of obesity.
Metabolic effects
Semaglutide and liraglutide on insulin secretion
The effect of GLP-1 and GLP-1RA in pancreatic β-cells promotes glucose catabolism and insulin secretion (15, 31). This process relies on the mTOR-dependent HIF-1α activation pathway, which starts with the binding of the GLP-1 to the GLP-1R, activating the AC. Subsequently, AC increases the cAMP expression, which promotes the activation of both, PKA and EPAC. Activated PKA promotes the PI3K/mTOR pathway in β-cells, leading to the activation of the Hypoxia-Inducible Factor 1 (HIF-1). The transcriptional factor HIF-1 induces glycolytic genes activation in response to hypoxia and growth factors. Enhanced glycolysis facilitates citric acid cycle activation, elevating intracellular ATP concentration. High ATP amounts induce the closure of potassium channels, depolarizing the cell membrane, leading to calcium influx and insulin vesicle release. This release can be rapid, with a peak at 10 min post-initial glucose stimulus, followed by a sustained release from major insulin granules (approximately 90–95%) lasting up to 60 min under normal conditions (15, 31). However, the low half-life of GLP-1 incretin could affect this process in individuals with DM2 or obesity (32, 33). Therefore, GLP-1RA drugs like liraglutide or semaglutide can counteract DPP-4 degradation, prolonging the GLP-1R signal and maintaining insulin secretion via the mTOR-dependent HIF-1α pathway (34).
Semaglutide, liraglutide and glucose homeostasis
Liraglutide and semaglutide are mainly prescribed for the treatment of DM2 (34). These GLP-1RA drugs can improve blood glucose levels and reduce body weight (14, 35). There are various mechanisms involved in glucose homeostasis, like glucose-dependent insulin secretion, insulin biosynthesis, and glucagon regulation (34). Moreover, studies have shown that GLP-1RA could regulate glucose homeostasis in an insulin-independent mechanism via the 5′-AMP-activated protein kinase (AMPK) pathway (36, 37). The AMPK pathway is activated by the PI3K/AKT pathway, which promotes the translocation of the glucose transporter 4 (GLUT4) from intracellular vesicles to the plasma membrane. The increased levels of GLUT4 in the plasma membrane stimulate glucose uptake, promoting glucose homeostasis (36–38).
Lipid metabolism
Semaglutide and Liraglutide effects on lipid metabolism
GLP-1RA drugs have been associated with reduced food intake and body weight loss (39). The molecular mechanisms involve the stimulation of the Wnt/β-catenin signaling pathway by GLP-1R. Activation of the Wnt/β-catenin pathway has negative effects on adipogenesis by downregulating the expression of genes related to de novo lipogenesis. These downregulated genes include DGAT1, SCD1, ApoB, FABP1, and FOXA1, which are involved in fatty acid and triglycerides synthesis. Moreover, a decreased expression of the FABP1 and FOXA1 genes leads to decreased uptake of free fatty acids. The AMPK pathway also plays a role in inhibiting lipogenesis by regulating lipogenic genes like acetyl-CoA carboxylase. Inhibition of the acetyl-CoA carboxylase suppresses the de novo lipogenesis and improves fatty acids oxidation (37, 40).
The effect of GLP-1RA drugs in adipose tissue involves the lipolytic process. These agonists stimulate the AMPK pathway, leading to the activation of Sirtuin 1 (SIRT1), a NAD+-dependent deacetylase that upregulates the expression of lipolytic proteins like triacylglycerol lipase. Consequently, triglyceride depletion occurs in white adipose tissue, resulting in reduced fat accumulation and improved energy expenditure. Moreover, GLP-1RA negatively influences the expression of peroxisome proliferator-activated receptors (PPARs), leading to a downregulation of proteins associated with lipid metabolism (37, 41, 42).
Semaglutide and liraglutide in thermogenesis and energy expenditure
GLP-1 and GLP-1RA stimulate the GLP-1R in the central nervous system to modulate lipid metabolism in white and brown adipose tissues leading to a reduction in body weight. This process involves AMPK activation in the hypothalamic ventromedial nucleus by GLP-1 or GLP-1RA, promoting brown adipose tissue thermogenesis and white adipose tissue browning. The activated AMPK pathway enhances brown tissue activation via transcriptional regulators of genes involved in brown tissue development (PR domain containing 16, peroxisome proliferator-activated receptor γ, peroxisome proliferator-activated receptor γ coactivator 1α). Moreover, AMPK stimulates the activation of the cAMP/PKA pathway in brown adipocytes, triggering lipolysis through the release of free fatty acids and upregulation of uncoupling protein 1 (UCP1). Additionally, the cGMP second messenger is involved in mitochondrial biogenesis and UCP1 activation in brown adipose via the nitric oxide-sensitive soluble guanylyl cyclase pathway. UCP1 is essential for releasing electrons during oxidative phosphorylation in the inner mitochondrial membrane, generating heat (thermogenesis). Increased expression of UPC1 and a higher number of mitochondria are characteristics of activated brown adipose tissue, facilitating the lipolytic cycle (43–45).
Another effect of GLP-1RA is the increase of sympathetic nervous system (SNS) activity. Activated SNS promotes brown adipose tissue thermogenesis and white adipose tissue browning via hypothalamic AMPK activity. Furthermore, the thyroid hormone (TH) is an essential regulator of brown adipose tissue activity. In this context, GLP-1RA drugs could activate the thermogenic proteins in brown adipocytes, increasing intracellular type 2 deiodinase (D2) expression and subsequent TH activation. Therefore, TH activates brown adipocytes and promotes oxygen consumption and thermogenesis (43, 46, 47).
Discussion
The metabolic effect of semaglutide and liraglutide in obese people is remarkable. The main mechanism of action of these GLP-1RA is the stimulation of the GLP-1R that triggers the activation of several metabolic pathways involved in insulin secretion, lipid metabolism, energy expenditure, pro-survival and anti-apoptotic cellular signaling, and oxidative stress prevention, in several tissues like the pancreas, central nervous system, heart, muscle, kidneys, gut, among others (15). The wide distribution of the GLP-1R has allowed the study of semaglutide and liraglutide pharmacological protocols to improve metabolic diseases in several experimental models (26, 40, 48–50). The assessment of these GLP-1RA could elucidate the role of these drugs in the cellular signaling process of obese-related diseases, which could support the development of new pharmacological approaches. For instance, the combination of GLP-1RA and enzyme inhibitors of proinflammatory, lipogenesis, pro-apoptotic, and pro-oxidative stress processes could be evaluated in metabolic diseases. Although, rigorous safety and efficacy-controlled trials must be carried out, like those performed to assess the effect of semaglutide and liraglutide in obese or DM2 individuals.
Clinical trials have evaluated the effectiveness of GLP-1RA drugs, semaglutide, and liraglutide, in treating obesity. The Semaglutide Treatment Effect in People with Obesity (STEP) program evaluated the once-weekly administration of 2.4 mg subcutaneous semaglutide in people with obesity or overweight. In the STEP 1 trial, 1961 participants underwent dietary and exercise interventions. In this trial, 69 to 79% of the participants lost weight with an average weight reduction of ≥10% after 68 weeks of treatment compared with placebo group (12–17%) (51). Additionally, the STEP trials evaluated patients with obesity and DM2; the results supported the recommended dose of 2.4 mg of semaglutide per week for weight loss in individuals with obesity, with or without DM2 (52).
Similarly, in the Peptide Innovation for Early Diabetes Treatment (PIONEER) clinical trial, oral semaglutide was tested in patients with DM2. Results showed that oral semaglutide has a significantly higher efficacy compared to placebo and other DPP4 inhibitor drugs (53). Moreover, the results from the PIONEER clinical trials suggested that oral semaglutide effectively reduced HbA1c and body weight, showing responsiveness in diverse age groups with a diagnosis of DM2 (54).
The efficiency of liraglutide was evaluated in the clinical trial called SCALE (Satiety and Clinical Adiposity: Evidence for liraglutide), where it was determined that a dosage of 3.0 mg induced an 8% weight loss equivalent to 8.4 kg of the initial weight in over 56 weeks. In comparison, the placebo group experienced a decrease of 2.6% equivalent to 2.8 kg. Participants also improved weight-related comorbidities through lifestyle and diet adjustments (55).
Azuri et al. showed that semaglutide had a better performance than liraglutide in terms of weight loss (12.4% [95% CI: 11.5–13.4%] vs. 5.4% [95% CI: 5–5.8%]). Moreover, this study reported that semaglutide had a lower economic spend than liraglutide for obesity management (56). This highlights the potential use of semaglutide due to its low cost and superior performance.
The posology of semaglutide and liraglutide also showed differences in body weight reduction. In obese individuals without diabetes mellitus, a once-weekly subcutaneous semaglutide (2.4 mg) dose had a significant body weight change compared with a once-daily subcutaneous liraglutide (3.0 mg) dose after 68 weeks. Participants under the semaglutide treatment showed a 15% weight loss than baseline, while liraglutide recipients experienced a 6% weight loss (57). Therefore, semaglutide also has an advantage due to the smaller number of doses and greater weight loss.
A meta-analysis revealed that a 2.4 mg dose of semaglutide led to a reduction of 12.4 kg of body weight, while doses of 3.0 mg of liraglutide, 1.0 mg of semaglutide, and 1.8 mg of liraglutide led to reductions in body weight of 5.2 kg, 3.7 kg, and 1.8 kg, respectively. The evaluation of these doses was performed in various periods, ranging from 20 weeks to 68 weeks. The most effective treatment involved a weekly dose of 2.4 mg semaglutide for 68 weeks. Moreover, for the dose of 2.4 mg semaglutide, the decrease in glycated hemoglobin was the highest (1.48% reduction with 2.4 mg semaglutide dose) compared with the other doses (58). Once again, semaglutide demonstrates a higher performance compared with liraglutide, although individual medical status must be assessed before the prescription of this treatment to prevent side effects.
Another important component of the treatment with GLP-1RA is the inclusion of lifestyle interventions, including exercise and a low-calorie diet (1, 59). This factor is significant for weight loss sustaining, given that after subcutaneous semaglutide (2.4 mg) treatment suspension, studies have shown that there may be a weight regain worsening cardiometabolic parameters (60). This highlights that GLP-1RA treatment must be continuous, along with a healthy lifestyle. Therefore, monitoring after several years of semaglutide or liraglutide withdrawal should be performed to verify metabolic status and weight. For instance, the outcomes of semaglutide and liraglutide in patients with DM2 have shown a cardiovascular risk factors reduction and an improvement in glucose levels and nutritional status (22, 58, 61–63).
The safety profile of GLP-1RA drugs generally indicates a low incidence of side effects, although it depends on the dose and the administration mode. For instance, oral semaglutide (14 mg) exhibited more side effects (such as vomiting or gastrointestinal issues) than subcutaneous liraglutide (1.2 mg); however, these side effects were no different from those observed with subcutaneous semaglutide treatment (64). Moreover, the use of semaglutide has been related to the increased risk of cholelithiasis (65).
The effectiveness of these GLP-1RA drugs, especially semaglutide, is associated with an improvement in other diseases like cardiovascular diseases (22, 50). Studies suggest that DM2 accompanied by insulin resistance may contribute to memory impairment and the development of neurodegenerative diseases such as Alzheimer’s and Parkinson’s disease (66). Furthermore, these GLP-1RA medications have also been related to a reduction of neuroinflammation, probably due to its possible role in neuronal insulin signaling pathway restoration (49, 67, 68). Hence, semaglutide and liraglutide could also have the potential to ameliorate neurodegeneration processes observed in pathologies like Alzheimer’s and Parkinson’s diseases, although further research is needed (48, 69). Moreover, clinical trials have reported a reduction of the inflammatory C-reactive protein (70), which could indicate that this drug may also regulate the immune system, although this approach requires further assessment. Therefore, given the association with improved nutritional status under semaglutide treatment, this GLP-1RA could be explored in other obesity-related conditions, including fatty liver disease, dyslipidemia, hypertension, and potentially even cancer.
Conclusion
Obesity is a current public health issue that must be addressed to avoid and prevent several underlying pathologies like cardiovascular diseases, fatty liver disease, DM2, hypertension, and cancer. Therefore, lifestyle and pharmacological options must be evaluated to improve or prevent those obesity underlying pathologies. Between the pharmacological options, the GLP-1RA, originally approved for the treatment of DM2, have been demonstrated to be useful for body weight reduction in obese individuals. The main molecular mechanisms of GLP-1RA in obesity reduction include the increased production of insulin by the cAMP/PKA pathway in pancreatic β-cells, along with an augmented translocation of GLUT-4 in the cellular membrane for improved glucose homeostasis, and energy expenditure by lipolytic cycle stimulation. The GLP-1RA with better outcomes in clinical trials is semaglutide since this drug can reduce up to 15% of the baseline weight after a 2.4 mg subcutaneous weekly dose. Moreover, semaglutide has shown a reduction of cardiovascular risk factors and inflammatory proteins observed in obese individuals. Therefore, semaglutide could improve other obesity-related pathologies like cardiovascular, hypertension, or fatty liver diseases.
Author contributions
RT-T: Conceptualization, Writing – original draft, Writing – review & editing. VR-P: Conceptualization, Writing – original draft, Writing – review & editing. SC-U: Writing – original draft, Writing – review & editing. PG-R: Writing – original draft, Writing – review & editing, Writing – original draft. EP-C: Writing – original draft, Writing – review & editing. RZ-V: Writing – original draft, Writing – review & editing. DS-R: Conceptualization, Resources, Writing – original draft, Writing – review & editing. AZ: Conceptualization, Funding acquisition, Project administration, Resources, Supervision, Writing – original draft, Writing – review & editing.
Funding
The author(s) declare financial support was received for the research, authorship, and/or publication of this article. The publication fee of this article will be funded by Universidad UTE.
Acknowledgments
The authors are grateful to Universidad UTE for their support.
Conflict of interest
The authors declare that the research was conducted in the absence of any commercial or financial relationships that could be construed as a potential conflict of interest.
Publisher’s note
All claims expressed in this article are solely those of the authors and do not necessarily represent those of their affiliated organizations, or those of the publisher, the editors and the reviewers. Any product that may be evaluated in this article, or claim that may be made by its manufacturer, is not guaranteed or endorsed by the publisher.
References
1. Wilding, JPH, Batterham, RL, Calanna, S, Davies, M, Van Gaal, LF, Lingvay, I, et al. Once-weekly Semaglutide in adults with overweight or obesity. N Engl J Med. (2021) 384:989–1002. doi: 10.1056/NEJMoa2032183
2. World Health Organization (WHO). Controlling the global obesity epidemic. [cited 2024 Mar 5]. (2021). Available from: https://www.who.int/activities/controlling-the-global-obesity-epidemic
3. Goh, GBB, and Tham, KW. Combating obesity: a change in perspectives. Singapore Med J. (2023) 64:153–4. doi: 10.4103/singaporemedj.SMJ-2023-043
4. Hruby, A, and Hu, FB. The epidemiology of obesity: a big picture. PharmacoEconomics. (2015) 33:673–89. doi: 10.1007/s40273-014-0243-x
5. Wong, MCS, Huang, J, Wang, J, Chan, PSF, Lok, V, Chen, X, et al. Global, regional and time-trend prevalence of central obesity: a systematic review and meta-analysis of 13.2 million subjects. Eur J Epidemiol. (2020) 35:673–83. doi: 10.1007/s10654-020-00650-3
6. Ansari, S, Haboubi, H, and Haboubi, N. Adult obesity complications: challenges and clinical impact. Ther Adv Endocrinol Metab. (2020) 11:204201882093495–14. doi: 10.1177/2042018820934955
7. Temple, NJ. The origins of the obesity epidemic in the USA–lessons for today. Nutrients. (2022) 14:4253. doi: 10.3390/nu14204253
8. Kloock, S, Ziegler, CG, and Dischinger, U. Obesity and its comorbidities, current treatment options and future perspectives: challenging bariatric surgery? Pharmacol Ther. (2023) 251:108549–17. doi: 10.1016/j.pharmthera.2023.108549
9. Zhao, X, Wang, M, Wen, Z, Lu, Z, Cui, L, Fu, C, et al. GLP-1 receptor agonists: beyond their pancreatic effects. Front Endocrinol (Lausanne). (2021) 12:1–19. doi: 10.3389/fendo.2021.721135
10. Ma, X, Liu, Z, Ilyas, I, Little, PJ, Kamato, D, Sahebka, A, et al. GLP-1 receptor agonists (GLP-1RAs): cardiovascular actions and therapeutic potential. Int J Biol Sci. (2021) 17:2050–68. doi: 10.7150/ijbs.59965
11. Iorga, RA, Bacalbasa, N, Carsote, M, Bratu, OG, Stanescu, AMA, Bungau, S, et al. Metabolic and cardiovascular benefits of GLP-1 agonists, besides the hypoglycemic effect (review). Exp Ther Med. (2020) 20:2396–400. doi: 10.3892/etm.2020.8714
12. Yazlcl, D, Yaplcl Eser, H, Klylcl, S, Sancak, S, Sezer, H, Uygur, M, et al. Clinical impact of glucagon-like Peptide-1 receptor analogs on the complications of obesity. Obes Facts. (2023) 16:149–63. doi: 10.1159/000526808
13. de Graaf, C, Donnelly, D, Wootten, D, Lau, J, Sexton, PM, Miller, LJ, et al. Glucagon-like Peptide-1 and its class B G protein–coupled receptors: a long march to therapeutic successes. Pharmacol Rev. (2016) 68:954–1013. doi: 10.1124/pr.115.011395
14. Smith, NK, Hackett, TA, Galli, A, and Flynn, CR. GLP-1: molecular mechanisms and outcomes of a complex signaling system. Neurochem Int. (2019) 128:94–105. doi: 10.1016/j.neuint.2019.04.010
15. Rowlands, J, Heng, J, Newsholme, P, and Carlessi, R. Pleiotropic effects of GLP-1 and analogs on cell signaling, metabolism, and function. Front Endocrinol. (2018) 9:1–23. doi: 10.3389/fendo.2018.00672
16. Andraos, J, Muhar, H, and Smith, SR. Beyond glycemia: comparing tirzepatide to GLP-1 analogues. Rev Endocr Metab Disord. (2023) 24:1089–101. doi: 10.1007/s11154-023-09825-1
17. Marzook, A, Tomas, A, and Jones, B. The interplay of glucagon-like Peptide-1 receptor trafficking and Signalling in pancreatic Beta cells. Front Endocrinol (Lausanne). (2021) 12:1–12. doi: 10.3389/fendo.2021.678055
18. Buteau, J, Roduit, R, Susini, S, and Prentki, M. Glucagon-like peptide-1 promotes DNA synthesis, activates phosphatidylinositol 3-kinase and increases transcription factor pancreatic and duodenal homeobox gene 1 (PDX-1) DNA binding activity in beta (INS-1)- cells. Diabetologia. (1999) 42:856–64. doi: 10.1007/s001250051238
19. Wootten, D, Simms, J, Miller, LJ, Christopoulos, A, and Sexton, PM. Polar transmembrane interactions drive formation of ligand-specific and signal pathway-biased family B G protein-coupled receptor conformations. Proc Natl Acad Sci USA. (2013) 110:5211–6. doi: 10.1073/pnas.1221585110
20. Chao, AM, Tronieri, JS, Amaro, A, and Wadden, TA. Clinical insight on Semaglutide for chronic weight Management in Adults: patient selection and special considerations. Drug Des Devel Ther. (2022) 16:4449–61. doi: 10.2147/DDDT.S365416
21. Andersen, A, Knop, FK, and Vilsbøll, T. A pharmacological and clinical overview of Oral Semaglutide for the treatment of type 2 diabetes. Drugs. (2021) 81:1003–30. doi: 10.1007/s40265-021-01499-w
22. Mahapatra, MK, Karuppasamy, M, and Sahoo, BM. Semaglutide, a glucagon like peptide-1 receptor agonist with cardiovascular benefits for management of type 2 diabetes. Rev Endocr Metab Disord. (2022) 23:521–39. doi: 10.1007/s11154-021-09699-1
23. Del Olmo-Garcia, MI, and Merino-Torres, JF. GLP-1 receptor agonists and cardiovascular disease in patients with type 2 diabetes. J Diabetes Res. (2018) 2018:1–12. doi: 10.1155/2018/4020492
24. Shah, M, and Vella, A. Effects of GLP-1 on appetite and weight. Rev Endocr Metab Disord. (2014) 15:181–7. doi: 10.1007/s11154-014-9289-5
25. Eren-Yazicioglu, CY, Yigit, A, Dogruoz, RE, and Yapici-Eser, H. Can GLP-1 be a target for reward system related disorders? A qualitative synthesis and systematic review analysis of studies on palatable food, drugs of abuse, and alcohol. Front Behav Neurosci. (2021) 14:1–15. doi: 10.3389/fnbeh.2020.614884
26. Yang, X, Qiang, Q, Li, N, Feng, P, Wei, W, and Hölscher, C. Neuroprotective mechanisms of glucagon-like Peptide-1-based therapies in ischemic stroke: an update based on preclinical research. Front Neurol. (2022) 13:1–20. doi: 10.3389/fneur.2022.844697
27. Mehdi, SF, Pusapati, S, Anwar, MS, Lohana, D, Kumar, P, Nandula, SA, et al. Glucagon-like peptide-1: a multi-faceted anti-inflammatory agent. Front Immunol. (2023) 14:1–20. doi: 10.3389/fimmu.2023.1148209
28. Alharbi, SH. Anti-inflammatory role of glucagon-like peptide 1 receptor agonists and its clinical implications. Ther Adv Endocrinol Metab. (2024) 15:1–18. doi: 10.1177/20420188231222367
29. Li, Q, Tuo, X, Li, B, Deng, Z, Qiu, Y, and Xie, H. Semaglutide attenuates excessive exercise-induced myocardial injury through inhibiting oxidative stress and inflammation in rats. Life Sci. (2020) 250:117531–9. doi: 10.1016/j.lfs.2020.117531
30. Reddy, IA, Stanwood, GD, and Galli, A. Moving beyond energy homeostasis: new roles for glucagon-like Peptide-1 in food and drug reward. Neurochem Int. (2014) 73:49–55. doi: 10.1016/j.neuint.2013.10.003
31. Carlessi, R, Chen, Y, Rowlands, J, Cruzat, VF, Keane, KN, Egan, L, et al. GLP-1 receptor signalling promotes β-cell glucose metabolism via mTOR-dependent HIF-1α activation. Sci Rep. (2017) 7:1–13. doi: 10.1038/s41598-017-02838-2
32. Wang, JY, Wang, QW, Yang, XY, Yang, W, Li, DR, Jin, JY, et al. GLP−1 receptor agonists for the treatment of obesity: role as a promising approach. Front Endocrinol. (2023) 14:1–11. doi: 10.3389/fendo.2023.1085799
33. Galstyan, GR, Karataeva, EA, and Yudovich, EA. Evolution of glucagon-like peptide-1 receptor agonists for the treatment of type 2 diabetes. Diabetes Mellitus. (2017) 20:286–98. doi: 10.14341/DM8804
34. Knudsen, LB, and Lau, J. The discovery and development of liraglutide and semaglutide. Front Endocrinol. (2019) 10:1–32. doi: 10.3389/fendo.2019.00155
35. Goldenberg, RM, and Steen, O. Semaglutide: review and place in therapy for adults with type 2 diabetes. Can J Diabetes. (2019) 43:136–45. doi: 10.1016/j.jcjd.2018.05.008
36. Hardie, DG, Ross, FA, and Hawley, SA. AMPK: a nutrient and energy sensor that maintains energy homeostasis. Nat Rev Mol Cell Biol. (2012) 13:251–62. doi: 10.1038/nrm3311
37. Wen, X, Zhang, B, Wu, B, Xiao, H, Li, Z, Li, R, et al. Signaling pathways in obesity: mechanisms and therapeutic interventions. Signal Transduct Target Ther. (2022) 7:1–31. doi: 10.1038/s41392-022-01149-x
38. Andreozzi, F, Raciti, GA, Nigro, C, Mannino, GC, Procopio, T, Davalli, AM, et al. The GLP-1 receptor agonists exenatide and liraglutide activate glucose transport by an AMPK-dependent mechanism. J Transl Med. (2016) 14:1–13. doi: 10.1186/s12967-016-0985-7
39. Hansen, G, Jelsing, J, and Vrang, N. Effects of liraglutide and sibutramine on food intake, palatability, body weight and glucose tolerance in the gubra DIO-rats. Acta Pharmacol Sin. (2012) 33:194–200. doi: 10.1038/aps.2011.168
40. Petrovic, A, Igrec, D, Rozac, K, Bojanic, K, and Kuna, L. The role of GLP1-RAs in direct modulation of lipid metabolism in hepatic tissue as determined using in vitro models of NAFLD. Curr Issues Mol Biol. (2023) 45:4544–56. doi: 10.3390/cimb45060288
41. Xu, F, Lin, B, Zheng, X, Chen, Z, Cao, H, Xu, H, et al. GLP-1 receptor agonist promotes brown remodelling in mouse white adipose tissue through SIRT1. Diabetologia. (2016) 59:1059–69. doi: 10.1007/s00125-016-3896-5
42. Zhu, R, and Chen, S. Proteomic analysis reveals semaglutide impacts lipogenic protein expression in epididymal adipose tissue of obese mice. Front Endocrinol. (2023) 14:1–19. doi: 10.3389/fendo.2023.1095432
43. Beiroa, D, Imbernon, M, Gallego, R, Senra, A, Herranz, D, Villarroya, F, et al. GLP-1 agonism stimulates brown adipose tissue thermogenesis and browning through hypothalamic AMPK. Diabetes. (2014) 63:3346–58. doi: 10.2337/db14-0302
44. Zhu, E, Yang, Y, Zhang, J, Li, Y, Li, C, Chen, L, et al. Liraglutide suppresses obesity and induces brown fat-like phenotype via soluble guanylyl cyclase mediated pathway in vivo and in vitro. Oncotarget. (2016) 7:81077–89. doi: 10.18632/oncotarget.13189
45. Lo, KA, and Sun, L. Turning WAT into BAT: a review on regulators controlling the browning of white adipocytes. Biosci Rep. (2013) 33:711–9. doi: 10.1042/BSR20130046
46. Oliveira, FCB, Bauer, EJ, Ribeiro, CM, Pereira, SA, Beserra, BTS, Wajner, SM, et al. Liraglutide activates type 2 deiodinase and enhances β3-adrenergic-induced thermogenesis in mouse adipose tissue. Front Endocrinol. (2022) 12:1–11. doi: 10.3389/fendo.2021.803363
47. Ribeiro, MO, Carvalho, SD, Schultz, JJ, Chiellini, G, Scanlan, TS, Bianco, AC, et al. Thyroid hormone-sympathetic interaction and adaptive thermogenesis are thyroid hormone receptor isoform-specific. J Clin Invest. (2001) 108:97–105. doi: 10.1172/JCI200112584
48. Poupon-Bejuit, L, Hughes, MP, Liu, W, Geard, A, Faour-Slika, N, Whaler, S, et al. A GLP1 receptor agonist diabetes drug ameliorates neurodegeneration in a mouse model of infantile neurometabolic disease. Sci Rep. (2022) 12:1–17. doi: 10.1038/s41598-022-17338-1
49. Mahapatra, MK, Karuppasamy, M, and Sahoo, BM. Therapeutic potential of Semaglutide, a newer GLP-1 receptor agonist, in abating obesity, non-alcoholic steatohepatitis and neurodegenerative diseases: a narrative review. Pharm Res. (2022) 39:1233–48. doi: 10.1007/s11095-022-03302-1
50. García-Vega, D, Sánchez-López, D, Rodríguez-Carnero, G, Villar-Taibo, R, Viñuela, JE, Lestegás-Soto, A, et al. Semaglutide modulates prothrombotic and atherosclerotic mechanisms, associated with epicardial fat, neutrophils and endothelial cells network. Cardiovasc Diabetol. (2024) 23:1–18. doi: 10.1186/s12933-023-02096-9
51. Bergmann, NC, Davies, MJ, Lingvay, I, and Knop, FK. Semaglutide for the treatment of overweight and obesity: a review. Diabetes Obes Metab. (2023) 25:18–35. doi: 10.1111/dom.14863
52. Alabduljabbar, K, Al-Najim, W, and Le Roux, CW. The impact once-weekly Semaglutide 2.4 mg will have on clinical practice: a focus on the STEP trials. Nutrients. (2022) 14. 1–14. doi: 10.3390/nu14112217
53. Thethi, TK, Pratley, R, and Meier, JJ. Efficacy, safety and cardiovascular outcomes of once-daily oral semaglutide in patients with type 2 diabetes: the PIONEER programme. Diabetes Obes Metab. (2020) 22:1263–77. doi: 10.1111/dom.14054
54. Aroda, VR, Bauer, R, Christiansen, E, Haluzík, M, Kallenbach, K, Montanya, E, et al. Efficacy and safety of oral semaglutide by subgroups of patient characteristics in the PIONEER phase 3 programme. Diabetes Obes Metab. (2022) 24:1338–50. doi: 10.1111/dom.14710
55. Kolotkin, RL, Fujioka, K, Wolden, ML, Brett, JH, and Bjorner, JB. Improvements in health-related quality of life with liraglutide 3.0 mg compared with placebo in weight management. Clin Obes. (2016) 6:233–42. doi: 10.1111/cob.12146
56. Azuri, J, Hammerman, A, Aboalhasan, E, Sluckis, B, and Arbel, R. Liraglutide versus semaglutide for weight reduction—a cost needed to treat analysis. Obesity. (2023) 31:1510–3. doi: 10.1002/oby.23752
57. Rubino, DM, Greenway, FL, Khalid, U, O’Neil, PM, Rosenstock, J, Sørrig, R, et al. Effect of weekly subcutaneous Semaglutide vs daily Liraglutide on body weight in adults with overweight or obesity without diabetes: the STEP 8 randomized clinical trial. JAMA. (2022) 327:138–50. doi: 10.1001/jama.2021.23619
58. Xie, Z, Yang, S, Deng, W, Li, J, and Chen, J. Efficacy and safety of Liraglutide and Semaglutide on weight loss in people with obesity or overweight: a systematic review. Clin Epidemiol. (2022) 14:1463–76. doi: 10.2147/CLEP.S391819
59. O’Neil, PM, Birkenfeld, AL, McGowan, B, Mosenzon, O, Pedersen, SD, Wharton, S, et al. Efficacy and safety of semaglutide compared with liraglutide and placebo for weight loss in patients with obesity: a randomised, double-blind, placebo and active controlled, dose-ranging, phase 2 trial. Lancet. (2018) 392:637–49. doi: 10.1016/S0140-6736(18)31773-2
60. Wilding, JPH, Batterham, RL, Davies, M, Van Gaal, LF, Kandler, K, Konakli, K, et al. Weight regain and cardiometabolic effects after withdrawal of semaglutide: the STEP 1 trial extension. Diabetes Obes Metab. (2022) 24:1553–64. doi: 10.1111/dom.14725
61. Aroda, VR, Blonde, L, and Pratley, RE. A new era for oral peptides: SNAC and the development of oral semaglutide for the treatment of type 2 diabetes. Rev Endocr Metab Disord. (2022) 23:979–94. doi: 10.1007/s11154-022-09735-8
62. Vilsbøll, T, Zdravkovic, M, Le-Thi, T, Krarup, T, Schmitz, O, Courrèges, JP, et al. Liraglutide, a long-acting human glucagon-like Peptide-1 analog, given as monotherapy significantly improves glycemic control and lowers body weight without risk of hypoglycemia in patients with type 2 diabetes. Diabetes Care. (2007) 30:1608–10. doi: 10.2337/dc06-2593
63. Volpe, S, Lisco, G, Fanelli, M, Racaniello, D, Colaianni, V, Lavarra, V, et al. Oral semaglutide improves body composition and preserves lean mass in patients with type 2 diabetes: a 26-week prospective real-life study. Front Endocrinol. (2023) 14:1–11. doi: 10.3389/fendo.2023.1240263
64. Alhindi, Y, and Avery, A. The efficacy and safety of oral semaglutide for glycaemic management in adults with type 2 diabetes compared to subcutaneous semaglutide, placebo, and other GLP-1 RA comparators: a systematic review and network meta-analysis. Contemp Clin Trials Commun. (2022) 28:100944–13. doi: 10.1016/j.conctc.2022.100944
65. Smits, MM, and Van Raalte, DH. Safety of Semaglutide. Front Endocrinol. (2021) 12:1–19. doi: 10.2337/DC06-2593
66. Ruiz-Pozo, VA, Tamayo-Trujillo, R, Cadena-Ullauri, S, Frias-Toral, E, Guevara-Ramírez, P, Paz-Cruz, E, et al. The molecular mechanisms of the relationship between insulin resistance and Parkinson’s disease pathogenesis. Nutrients. (2023) 15:1–18. doi: 10.3390/nu15163585
67. Nowell, J, Blunt, E, and Edison, P. Incretin and insulin signaling as novel therapeutic targets for Alzheimer’s and Parkinson’s disease. Mol Psychiatry. (2023) 28:217–29. doi: 10.1038/s41380-022-01792-4
68. Hölscher, C. Protective properties of GLP-1 and associated peptide hormones in neurodegenerative disorders. Br J Pharmacol. (2022) 179:695–714. doi: 10.1111/bph.15508
69. Nowell, J, Blunt, E, Gupta, D, and Edison, P. Antidiabetic agents as a novel treatment for Alzheimer’s and Parkinson’s disease. Ageing Res Rev. (2023) 89:101979–22. doi: 10.1016/j.arr.2023.101979
Keywords: obesity, molecular mechanism, semaglutide, liraglutide, weight loss
Citation: Tamayo-Trujillo R, Ruiz-Pozo VA, Cadena-Ullauri S, Guevara-Ramírez P, Paz-Cruz E, Zambrano-Villacres R, Simancas-Racines D and Zambrano AK (2024) Molecular mechanisms of semaglutide and liraglutide as a therapeutic option for obesity. Front. Nutr. 11:1398059. doi: 10.3389/fnut.2024.1398059
Edited by:
Jorge Carriel Mancilla, Catholic University of Santiago de Guayaquil, EcuadorReviewed by:
Ludwig Roberto Álvarez Córdova, Catholic University of Santiago de Guayaquil, EcuadorJaneth Castano Jimenez, Indiana University Bloomington, United States
Raffaele Carraro, La Princesa University Hospital, Spain
Copyright © 2024 Tamayo-Trujillo, Ruiz-Pozo, Cadena-Ullauri, Guevara-Ramírez, Paz-Cruz, Zambrano-Villacres, Simancas-Racines and Zambrano. This is an open-access article distributed under the terms of the Creative Commons Attribution License (CC BY). The use, distribution or reproduction in other forums is permitted, provided the original author(s) and the copyright owner(s) are credited and that the original publication in this journal is cited, in accordance with accepted academic practice. No use, distribution or reproduction is permitted which does not comply with these terms.
*Correspondence: Ana Karina Zambrano, YW5hemFtYnJhbm8xN0Bob3RtYWlsLmNvbQ==
†These authors have contributed equally to this work and share first authorship