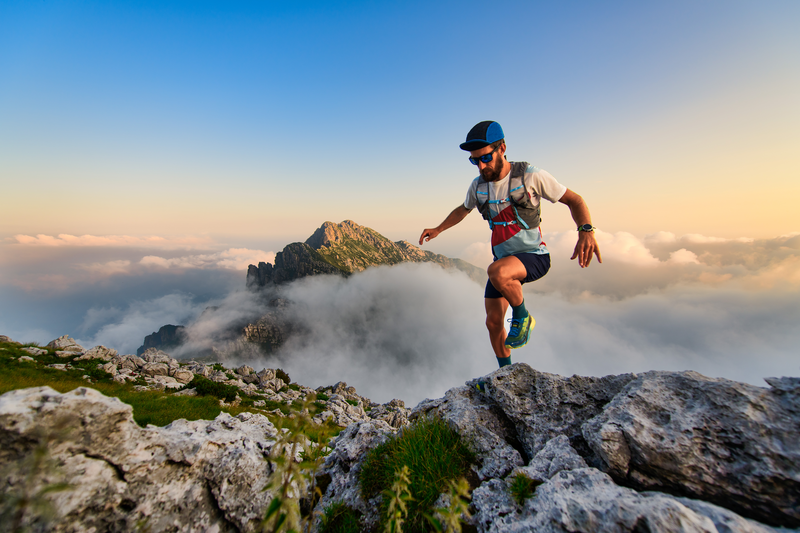
94% of researchers rate our articles as excellent or good
Learn more about the work of our research integrity team to safeguard the quality of each article we publish.
Find out more
REVIEW article
Front. Nutr. , 12 July 2024
Sec. Food Chemistry
Volume 11 - 2024 | https://doi.org/10.3389/fnut.2024.1388155
Krill oil (KO), extracted from the Antarctic marine crustacean Euphausia superba, is a nutrient-dense substance that includes rich profiles of n-3 polyunsaturated fatty acids (n-3 PUFAs), phospholipids (PLs), astaxanthin (ASX), as well as vitamins A and E, minerals, and flavonoids. As a high-quality lipid resource, KO has been widely used as a dietary supplement for its health-protective properties in recent years. KO has various benefits, including antioxidative, anti-inflammatory, metabolic regulatory, neuroprotective, and gut microbiome modulatory effects. Especially, the antioxidant and anti-inflammatory effects make KO have potential in skin care applications. With increasing demands for natural skin anti-aging solutions, KO has emerged as a valuable nutraceutical in dermatology, showing potential for mitigating the effects of skin aging and enhancing overall skin health and vitality. This review provides an overview of existing studies on the beneficial impact of KO on the skin, exploring its functional roles and underlying mechanisms through which it contributes to dermatological health and disease management.
Antarctic krill (E. superba) is a small, marine crustacean from the Antarctic Ocean. It is one of the most abundant organisms in the world, with a total biomass of up to 1 billion tons. Krill is the cornerstone of Antarctic ecology, serving as food for most fish. More importantly, despite its small size, with adults reaching only lengths of 4.5–6 cm, krill is remarkably valuable. Its unique nutritional composition has attracted intense research interest, not only in metabolic regulation (1), cardiovascular health (2), brain development (3) but also in skin health (4).
The skin is the largest organ of the human body, which consists of three layers: epidermis, dermis, and subcutaneous. It protects against ultraviolet (UV) rays, physical trauma, and external microbial environment and prevents moisture loss (5). Problems with skin health are related to many factors, including individual phenotype, lifestyle choices (e.g., alcohol consumption and lack of sleep), environmental factors (e.g., UV rays and pollutants), and various diseases (6). Skin aging is categorized into two types: intrinsic aging, which refers to the natural cellular aging process leading to wrinkles and pigmentation linked to oxidative stress, and external aging, predominantly resulting from environmental factors, most notably UV-radiation-induced photoaging (7, 8). The cutaneous immune system exerts a protective effect by responding to inflammatory stimuli (9). Exposure to multiple environmental factors, such as UV rays and microorganisms, activates the skin's inflammatory response (10). The cutaneous immune system is regulated by mediators such as cytokines and bioactive lipids. However, when immune responses are inadequate or mounted against non-infectious agents, it leads to chronic inflammation, which is a feature of many pathological skin conditions (11). Skin trauma includes a range of damages, including wounds, burns, scars, etc., which are caused by various factors such as physical, chemical, microbial, and pathological (e.g., diabetic foot). The skin condition during the wound-healing process is critical for chronic wound management. Thus, discovering new therapeutic approaches for skin problems remains a significant challenge (12).
Skin health is dependent on adequate nutrition. Vitamins, minerals, and essential fatty acids are thought to improve skin problems caused by both internal and external causes (13). With increasing demands for solutions to skin problems, research focusing on natural ingredients that enhance skin beauty and health is advancing the fields of dermatology and nutrition. Compared with chemically synthesized cosmetics, natural active substances extracted from natural plants, herbs, marine organisms, etc., have the advantages of being more natural, pure, and hypoallergenic. In particular, they have strong beneficial effects on the skin, such as UV radiation protection, antioxidant activity (14–16), regulating epidermal protection barrier (17–19) and maintaining water–ion balance (20, 21). Natural active substances, including aloin (22, 23), ginsenosides (24, 25), hydroxycinnamic acids (26, 27), and astaxanthin (ASX) (28, 29) have been proven to effectively solve a series of skin problems. Increasing research demonstrated that oral administration of some active substances also enhances skin performance, while traditional beauty and skin care products are generally used topically. Collagen is orally taken to improve skin elasticity and reduce wrinkles (30). Dietary supplements rich in antioxidants and anti-inflammatory ingredients are expanding their use for skin health. Using phospholipids (PLs) as a carrier can better penetrate cell membranes, promote human body absorption, and increase bioavailability (31, 32). Therefore, it is necessary to explore more natural substances with efficacious, safe, natural, and sustainable features, and develop novel products for skincare to meet market concerns and demands (33).
Krill oil (KO), extracted from Antarctic krill, is showing its potential in skin care applications due to its unique characteristics. KO is a nutrient-dense substance, including n-3 polyunsaturated fatty acids (n-3 PUFAs), PLs, ASX, as well as vitamins A and E, minerals, and flavonoids (34). In the past decade, KO has been approved as a safe food by the US Food and Drug Administration (FDA), European EFSA, and China, and has been approved for safe use in pregnant and lactating women. KO has been widely used as a dietary supplement for its health-protective properties in recent years. Especially the active ingredients of n-3 PUFA and ASX have anti-inflammatory and antioxidant properties, and PLs, as an important component of the cell membrane, can enhance the bioavailability of other effective ingredients. These three components have been widely used in the field of skin health (35–37). Recently, research on cellular, animal, and clinical trials of the benefits of KO on skin health has gradually emerged. This paper reviews for the first time the effects and potential mechanisms of KO and its ingredients on skin health, including anti-aging, anti-inflammation, and wound-promoting, which aim to provide new directions for skin nutrition research and skin health and disease management.
KO has been widely used as a nutritional supplement to improve human health in recent years. Increasing research shows that KO has various benefits, including antioxidant (4, 38, 39), anti-inflammatory (40–42), metabolic regulation (1, 43, 44), neuroprotective (3, 45, 46), and gut microbiota balance (47–49) (Figure 1). Especially, the antioxidant and anti-inflammatory effects make KO have potential in skin care applications.
Figure 1. Krill oil has a variety of benefits for human health. KO has positive effects on numerous aspects of human health, including antioxidant, anti-inflammation, anti-obesity, anti-diabetic, anti-atherosclerosis, and anti-hypertension. Additionally, it provides neuroprotection, improves skin health and joint health, benefits women's health, and maintains gut microbiome balance.
Compared with other oils from marine organisms, KO has a unique composition and proportion (Table 1). Lipids account for 0.4%−3.6% of the content of fresh krill (34). Depending on the sample type and analysis method, the phospholipid content of the extracted KO ranges from 39.29% to 80.69% (50), with 30%−65% fatty acids incorporated into PLs (54). Eicosapentaenoic acid (EPA) (ranging from 14.3% to 28%) and docosahexaenoic acid (DHA) (ranging from 7.1% to 15.7%) are the most abundant fatty acids found in KO (34). ASX content is about 40–5000 mg/kg (50, 52, 55).
The efficient extraction method of KO is still being actively explored. Some studies have reported that the lipid yield increased to 4.18% after two-step chitinase/protease hydrolysis. Each gram of KO contains 246.05 mg of phospholipids, 80.96 mg of free EPA and DHA, and 0.82 mg of ASX (56). Unsaturated fatty acids in KO are very easy to oxidize (57, 58). Further research on the extraction process and storage stability of KO will help improve the content, proportion, and activity of the active ingredients in KO.
DHA and EPA are the most important n-3 PUFA in KO. DHA is a 22-carbon fatty acid with six double bonds. EPA refers to a 20-carbon fatty acid with five double bonds. KO contains both PLs and triglycerides (TAG), and the content of PLs is as high as 65% (59). In KO, the majority of EPA (31.13%) and DHA (14.87%) bind to PLs, while a small portion of DHA (1.5%) and EPA (3.17%) bind to TAG (60). Since the cell membrane is constructed of PLs, DHA is usually esterified to phosphatidylcholine (PC) or phosphatidylethanolamine (PE) at the sn-2 position, which significantly increases the absorption of DHA and EPA in KO (52, 61, 62). The ratio of DHA and EPA in KO is about 1:2 (59). It has been found in cellular, animal, and clinical trials that EPA can resist skin aging, be anti-inflammatory, and promote wound healing, not only used as a dietary supplement but also topically used on the skin (63–65), which inferred that the effect of KO on improving skin health may be related to the high ratio of EPA. Compared with fish oil (FO), KO has higher efficiency, showing similar benefits with smaller doses. FO is primarily composed of TAG, DHA, and EPA; and FO mainly binds to TAG; and TAG-bound DHA/EPA cannot accumulate in membrane phospholipids. In addition, the ratio of DHA and EPA is about 1:1 in FO, with similar total fatty acid content to KO (52, 59).
Research has shown that n-3 PUFA can affect the neurological development of fetuses and infants (66), improve retina (67), provide cardiovascular protection (68), enhance memory function (69), improve anxiety, and reduce inflammatory markers (70). Increasing research has shown that n-3 PUFA, primarily EPA and DHA, are known to affect the structure and function of the skin (71). It can provide photoprotection and reduce UV-induced skin problems, including skin hydration, erythema, occlusion, and inflammatory problems, and even reduce the risk of skin cancer (72). n-3 PUFAs, to a certain extent, have many aspects of anti-inflammation, including leukocyte chemotaxis, production of inflammatory cytokines, and lymphocyte reactivity (73). Clinical trials have confirmed that dietary supplementation of PUFA can significantly improve inflammatory skin diseases such as acne (74) and psoriasis (75). DHA and EPA intake is associated with an 80% reduction in the risk of melanoma (76). DHA is superior to the effect of EPA in promoting wound healing (77). In addition, studies have proven that EPA can not only be used as a dietary supplement but also as a topical application, which expands the application scope and effectiveness of PUFA. EPA can increase the efficacy of cannabidiol (CBD). After 56 days of EPA and CBD application on subjects' faces, the area and number of erythema were significantly reduced, and skin hydration and elasticity increased by 31.2% and 25.6%, respectively (78). This may be related to the ability of PUFA to improve aging by oxidizing the telomere-reverse transcriptional gene axis (79). Topical application of EPA has a significant impact on UV-induced skin damage and intrinsic aging (80).
ASX (3,30-dihydroxy-b, b-carotene-4,40-dione) is a type of carotenoid and a red pigment that is responsible for the color of KO. Because the structure of ASX contains both hydroxyl and ketone groups, it shows extremely high resistance to peroxyl radicals (81). ASX has strong antioxidant activity, ten times more than other carotenoids (82). The exact content is mainly related to the krill raw materials, extraction process, and analysis technology. The content of ASX in KO will decrease with increasing storage time. Changing the storage environment conditions or microencapsulation treatment of KO might delay this phenomenon (83, 84).
ASX was proven to prevent cardiovascular diseases (85), protect the nervous system (86), strengthen the immune system to improve aging (87), and prevent cataracts (88). Also, ASX has been extensively studied to improve skin health and combat skin aging, including photoprotection, antioxidation, and anti-inflammation. The production of reactive oxygen species (ROS) is one of the key processes of skin aging (28). ASX pretreatment can reduce the ROS induced by UVB. ASX can also effectively inhibit skin cancer and reduce skin dehydration and wrinkles (89). It is worth noting that animal experiments have shown that ASX could reach the skin layers, including the dermis and epidermis, after oral consumption of ASX (35). At present, beauty products using ASX as raw materials have occupied a certain market and are sought after, including capsules or oral liquids for internal use and skin care products for external use. Several clinical studies have shown that ASX improves skin condition and delays skin aging by exerting its antioxidant and anti-inflammatory effects, suppressing hyperpigmentation and photoaging, reducing transepidermal water loss (TEWL), increasing skin elasticity, and reducing skin wrinkles (42, 90–92). The research showed that ASX has stronger antioxidant and antipollution effects than other natural crocin and other synthetic antioxidants such as alpha-tocopherol (AT), butylhydroxytoluene, butylhydroxyanisole and gallic acid, and is more effective in protecting human skin (93).
Phospholipids (PLs) are an important component of cell membranes (94). KO contains a large amount of PLs, and its content can be as high as 80.69%, depending on the sample type and analysis method. The most abundant PLs in KO include phosphatidylcholine (PC) (44.58% to 99.80%), phosphatidylethanolamine (PE) (0.20% to 24.74%), and lysophosphatidylcholine (LPC) (0.51% to 44.39%) (Table 1) (34).
As mentioned above, increasing evidence showed that PLs might be a more effective delivery form of n-3 PUFAs to body tissues than TAGs (95, 96). The presence of PLs in KO greatly improved the bioavailability of KO and the absorption of fatty acids, especially DHA and EPA (52). It is confirmed that EPA and DHA in PL form have better biological activity than triacylglycerol (TAG) or ethyl ester (EE) forms, and can play a role in brain function, antitumor activity, lipid metabolism, and glucose metabolism (97). Lysophosphatidic acid (LPA) is the simplest phospholipid found in nature, not only it can adjust skin function, hair follicle development, wound healing, and skin itching, but also it can play an important role in scleroderma and skin cancer (94).
PLs are commonly used as carrier substances in the field of skin health. PLs can enhance solubility, increase skin permeability, and assist in enhancing photoprotection (31, 32, 98). Luteolin is a natural antioxidant that can absorb a wide range of UV rays, but its water solubility and skin permeability are insufficient. Adding a certain proportion of phospholipids can improve the solubility, permeability, and photoprotective activity of luteolin (98). Vismodegib (VSD) is approved for the prevention and treatment of skin cancer, but its bioavailability is poor. The VSD gel containing phospholipids, ethanol, and terpenes enhanced the skin permeability of VSD, which resulted in a 3.59-fold increase in bioavailability compared to oral VSD, and exhibited excellent antitumor effect (32). Phospholipids and anti-skin cancer substances have synergistic effects to a certain extent, enhancing their bioavailability and anti-skin cancer effect (31).
In summary, the n-3 PUFA, ASX, and PL present in KO each contribute distinctive benefits to skin health. Nonetheless, when combined within KO, they work synergistically to produce superior effects that surpass the benefits of any single component alone.
Skin aging is an inevitable process, which means the skin function gradually weakens and the regeneration ability of skin cells gradually disappears. It manifests as wrinkles, reduced skin moisture, reduced skin elasticity and pigmentation (99, 100). Skin aging can be categorized as chronological aging and photoaging (or internal aging and external aging), and is affected by intrinsic factors and extrinsic factors. Intrinsic factors refer to the aging of skin cells, which are mainly related to time, genetics, hormones, etc. Extrinsic factors include UV exposure, air pollution, nutritional factors, etc. (101). Photoaging, caused primarily by long-term exposure to UV radiation from sunlight, is clinically characterized by deep wrinkling, dryness, pigmentation, and laxity. UVA (320–400 nm) can penetrate the dermis, resulting in damage to dermal collagen and elastin, while UVB (280–320 nm) mainly affects the epidermis and causes DNA damage. UV radiation can cause cell damage by generating ROS and inflammatory mediators, which directly attack the DNA of skin cells (102). ROS are a major factor of skin aging, involving damage to DNA, the inflammatory response, reduced production of antioxidants, and the generation of matrix metalloproteinases (MMPs) that degrade collagen and elastin in the dermal skin layer, leading to skin wrinkles and reduced elasticity (103). Therefore, dietary supplementation with antioxidants and anti-inflammatory agents, especially those that can accumulate in the dermis, could protect the skin from UV-induced damage. Research has demonstrated that KO effectively combats skin aging, as evidenced by its ability to reduce wrinkles, prevent skin water loss, slow down collagen degradation, and alleviate skin edema (Figure 2).
Figure 2. Krill oil has protective effects on skin health, including skin anti-aging, anti-inflammation, and wound healing enhancement. KO combats skin aging, manifesting in the reduction of wrinkles, skin water loss, collagen degradation, and skin edema. KO also relieves inflammatory skin diseases, including atopic dermatitis and psoriasis, by strengthening the stratum corneum lipid barrier and maintaining the correct intercellular lipid balance. Additionally, KO promotes wound healing by improving fibroblast function and repairing endothelial angiogenesis damage.
Photoaging results in skin histological changes, collagen fiber injuries, and uneven pigmentation, normally known as wrinkled and coarse skin (102). UV causes an increase in matrix metalloproteinases (MMPs), promotes extracellular matrix (ECM) protein degradation, and forms wrinkles.
Human skin cells exposed to UV irradiation were used to study photoaging in vitro. KO was evaluated for potential effect on wrinkle improvement and antioxidant activity in human dermal fibroblasts (HDF) cells. HDF exposed to UVB irradiation at 50 mJ/cm2 for 2 min induced a significant increase in MMP-1 activity. However, HDF cells treated with KO (0.5–8 mg/mL) at the same time resulted in a significant decrease in MMP-1 activity. Additionally, KO showed a concentration-dependent (from 0.25 to 8 mg/ml) increase in elastase inhibitory activity and procollagen synthesis in HDF. Moreover, KO was found to significantly increase DPPH radical scavenging activity (4). KO showed the protective effects on wrinkle formation in photoaging mice induced by UV radiation. Hairless female mice exposed to UVB radiation thrice a week for 15 weeks induce photoaging symptoms. However, after oral administration of KO (100, 200, or 400 mg/kg) every day for 105 days, the skin wrinkles were significantly inhibited, with the length and depth of wrinkles alleviated. Moreover, skin collagen I (COL1) contents were significantly increased, and the gene expression of MMP-1, MMP-9, and MMP13 in UV-radiated skin tissue was significantly decreased by KO treatments (4).
Wang et al. performed whole-genome proteomics and lipidomics analyses using a photoaging mouse model with n-3 PUFA or n-6 PUFA supplementation. Significant alleviation of photoaging was observed. They found that n-3 PUFA may alleviate photoaging by upregulating Hmmr (hyaluronic acid receptor) expression, which can decrease Mmp9 expression, reducing collagen degradation (36). Clinical studies have confirmed that topical application of EPA can significantly improve the characteristics of photoaging. Kim et al. applied 2% EPA or solvent to the buttock skin of young men for topical application twice, 24 h apart each time, and used 2 MEDs UV irradiation 24 h after the second application (MED, minimum erythema dose: 70–90 mJ/cm2). Skin biopsy results showed that EPA significantly improved UV-induced skin epidermal thickening. Immunohistochemistry and western blot analysis showed that UV reduced the procollagen expression of fibroblasts in the upper dermis and dermoepidermal junction. EPA restored the expression of procollagen after UV irradiation. Procollagen levels were similar to control levels. EPA significantly inhibited the expression of MMP-1 and MMP-9 after UV treatment, inhibited c-Jun phosphorylation, inhibited the activation of JNK and p38, and inhibited the expression of COX-2. In addition, 2% EPA was applied to the naturally aging buttock skin of elderly men thrice a week for 2 weeks, and a skin biopsy was performed 24 h later. The results showed that topical application of EPA to elderly skin increased the procollagen of the entire dermal fibroblasts. Increased expression of tropoelastin and fibrillin-1 and increased expression of TGF-β1, -β2, and -β3 in aged skin proved that EPA has preventive and therapeutic effects on intrinsic skin aging (80).
ASX has investigated the protection effect on UVB-induced oxidative stress and apoptosis in normal human epidermal keratinocytes (NHEKs). NHEKs were pre-treated with ASX for 24 h and then exposed to UVB (280–360 nm) irradiation at UV20–60 mJ/cm2. ASX (20 μM) could significantly inhibit UVB-induced NHEK cytotoxicity and ROS production. ROS can activate apoptosis signaling pathways. ASX was demonstrated to effectively reduce the number of early apoptotic cells. The molecular mechanisms could be the inhibition of the expression of apoptosis-related proteins BCL2-Associated X (BAX), Caspase-3 (CASP3), and poly ADP-ribose polymerase (PARP). Results indicated that ASX acted as a ROS scavenger to protect skin from UVB-induced damage (89). ASX is a superior antioxidant, having greater antioxidant capacity than canthaxanthin and β-carotene in human dermal fibroblasts. It was reported that dietary ASX could accumulate in the skin and could reduce UVA-induced wrinkle formation in hairless mice (35). Mice were fed ASX supplements for 70 days, HPLC-PDA analysis showed that ASX could accumulate in both the dermis and epidermis in a dose-dependent manner, and the concentration of ASX in the dermis is ~20–30 times that of the epidermis per square millimeter of skin area. A total of 65 healthy women (aged 35–60 years) were given oral supplements containing 6 mg or 12 mg dose of ASX or a placebo daily for 16 weeks. The study found that wrinkle parameters and skin moisture content significantly worsened in the placebo control group at 16 weeks, while the ASX group maintained the skin level as week 0. The viscoelastic fraction and bioelasticity of the ASX low-dose group were significantly improved at week 16. It shows that long-term supplementation of ASX can preventively inhibit skin deterioration caused by environmental damage, thereby delaying the skin aging process (91). To assess the protective role of astaxanthin for UV-induced skin deterioration, 23 healthy Japanese participants were recruited and assigned to the astaxanthin group supplemented with 4 mg of astaxanthin every day for 9 weeks or the placebo group. The back skin was irradiated with a solar simulator. Compared with the control group, the minimal erythema dose (MED) after ASX supplementation was significantly increased from baseline levels, and the “rough skin improvement” and “texture” of the skin in the non-irradiated areas of the ASX group were also significantly improved compared with baseline (104). These results suggest that KO and its ingredients can be developed as a dietary supplement or for topical use to prevent skin photoaging.
PLs may also have the potential to prevent and treat skin aging. Cell experiments confirmed that PS, lysophosphatidylserine (LPS) and lysophosphatidic acid (LPA) can inhibit the UV-induced decrease in type I procollagen and the increase in MMP-1 in human skin fibroblasts, and PS can block UV-induced IL-6 and COX-2 gene expression dose-dependently (105). The clinical experiment was performed using PS with topical application on human skin in vivo, just as Kim's study (80). The young and aged Korean adults were treated with 2% PS on the skin for 24 h. It was found that topical application of PS could prevent the reduction of procollagen and the upregulation of MMP-1 expression in UV-irradiated skin, and can stimulate protein synthesis in young human skin. Notably, PS-treated skin also showed increased procollagen expression in skin without UV exposure. However, PS did not prevent UV-induced expression of TNF-a and IL-1a in young human skin. Results were similar in experiments in the elderly, with PS increasing the expression of type I procollagen and decreasing the expression of MMP-1 in intrinsically aged human skin (105). Clinical trials of dietary supplementation with PUFA found that severe photoaging was inversely associated with a higher intake of ALA in men and with a higher intake of EPA in women. Dietary supplementation and topical use of PUFA have shown good anti-aging effects and can improve both natural aging and UV-induced photoaging (65).
Skin moisture is the most important factor in maintaining skin health and controlling aging. The stratum corneum, which affects skin moisturizing, forms a lipid layer composed of ceramides, cholesterol, and free fatty acids between keratinocytes (106). ROS produced by UV exposure causes skin barrier dysfunction, which leads to dry skin. Supplementing with ceramide, free fatty acids, and hyaluronic acid, either applied to the skin or ingested orally, can improve skin hydration.
Ceramide plays a role in maintaining the moisture in the epidermis and skin barrier, and supplementation with dietary sphingomyelin (precursors of ceramides) increases ceramide levels in the body (107). It was demonstrated that oral administration with sphingomyelin-containing milk phospholipid improved skin hydration in a UVB-induced photoaging mice model (106). It was found that milk phospholipids suppressed UV-induced increase in erythema and skin thickness, decreased transepidermal water loss, and increased skin moisture in hairless mice. Milk phospholipids increased the expression of skin moisture-related factors, including filaggrin, involucrin, and aquaporin3 (AQP3). Additionally, hyaluronic acid (HA) content in the skin tissue was increased by regulating the expression of HA synthesis- and degradation-related enzymes. Milk phospholipids suppressed UV-induced decrease in ROS levels and upregulated the expression of the antioxidant enzymes superoxidase dismutase 1 and 2 (SOD1, SOD2), catalase (CAT), and glutathione peroxidase1 (Gpx-1). Moreover, milk phospholipids demonstrated ROS scavenging effects by regulated heme oxygenase-1 (HO-1), an ROS regulator, through activation of nuclear factor erythroid-2-related factor 2 (Nrf2). In summary, sphingomyelin-containing milk phospholipids inhibit loss of skin moisture and reduce damage to skin barriers caused by photoaging by maintaining HA content and reducing ROS levels.
Skin aging often leads to decreased hyaluronic acid, which is a critical component in maintaining skin moisture (99). Fatty acids are essential for skin hydration, and polyunsaturated fatty acid deficiencies increase water loss through the skin barrier (71, 108). Evidence showed that UVB exposure and aging downregulated the expression of genes responsible for hyaluronic acid synthesis (HAS) in the dermis (109). However, oral administration of KO reversed the downregulation of the HAS genes, restored hyaluronic acid content in the skin, prevented skin water loss, and enhanced the moisturizing effect of the skin in UVB-induced skin photoaging mice (4). In HaCaT cells, the moisturizing activity of KO was evaluated by hyaluronic acid synthesis. Compared with the control, KO increased the synthesis of hyaluronic acid, showing a concentration-dependent effect (4). For treating inflammatory skin diseases, such as atopic dermatitis (AD), it was found that feeding AD mice with krill-derived alkyl phospholipids (Alk) for 3–5 weeks significantly reduced the transepidermal water loss (TEWL) of the dorsal skin (37).
The commonality of KO and its ingredients in resisting skin aging is mainly to reduce the production of ROS. Whether these active ingredients can exert synergistic effects in KO and promote anti-aging effects deserves further in-depth research. An experimental study of KO and ingredients on skin anti-aging is summarized in Table 2.
Inflammation is a protective physiological response to internal or external damage to the body. The skin is a protective organ, and the cutaneous immune system responds to inflammatory stimuli. The skin immune system includes innate immunity and adaptive immunity. The skin contains a variety of innate immune cells and mediators as well as phagocytes, such as antimicrobial peptides, proteins of the complement system, neutrophils, and macrophages. Pathological inflammatory skin diseases may develop into chronic inflammation when the skin's adaptive immune system is dysfunctional. KO has demonstrated the ability to relieve inflammation-related skin disorders, including UV-induced skin inflammation, atopic dermatitis, and psoriasis. This beneficial effect is attributed to its ability to strengthen the stratum corneum lipid barrier and maintain the correct intercellular lipid balance (Figure 2).
KO has been proven to have anti-inflammatory properties. A combined dietary supplement containing multiple natural antioxidants and/or anti-inflammatory drugs, including KO, coenzyme Q10, lipoic acid, resveratrol, grape seed oil, α-tocopherol, and selenium, was investigated in vitro study on the prevention of skin disorders by Fasano et al. Each ingredient was administered to human keratinocytes alone or in combination. It was found that these compounds, including KO, whether used in combination or alone, including KO, can produce anti-inflammatory effects on HaCaT keratinocytes treated with TNF-α. The compound modulates the nuclear factor-κB (NF-κB) pathway, which is closely related to pro-inflammatory cytokine synthesis, and significantly reduces the production of IL-6 and monocyte chemoattractant protein-1 (MCP-1)—one of the most expressed cytokines by keratinocytes during inflammation. This study further demonstrates the potential of KO to be applied in topical formulations as it can overcome issues related to oral formulations, which is biodistribution and metabolic transformation, and directly target keratinocytes (110).
UV radiation can cause skin inflammation. When human skin is acutely exposed to sunlight, UV radiation results in a sunburn reaction characterized by erythema, edema, itching and pain. Histopathological changes include thickening of the stratum corneum and the formation of apoptotic epidermal keratinocytes. Changes in immune cells include dermal neutrophil, lymphocyte, and monocyte infiltration, leading to changes in inflammatory cytokines, such as increasing the amount of tumor necrosis factor-α (TNF-α) and the pro-inflammatory cytokine IL-1 in the entire human skin tissue, and decreasing the expression of the anti-inflammatory cytokine IL-10 (9, 111). This is mediated, in part, by lipids such as pro-inflammatory eicosanoids. Lipids actively participate in or mediate multifaceted effects on skin and immune cells residence and infiltration in inflammatory settings.
When UV rays irradiate the skin, polymorphic neutrophils (PMNs) and neutrophils are recruited into the damaged tissue. Myeloperoxidase (MPO), a cytotoxic enzyme that activates inflammation, is related to both PMNs and neutrophils and can be released by PMNs (112–115). Studies have found that oral administration of KO can reduce UV-induced skin inflammation. Evaluate the effect of KO on skin edema by measuring the weight of 6 mm diameter skin samples. It was found that the average skin weight of mice in the KO group was significantly decreased in a dose-dependent manner. Moreover, KO directly inhibits MPO activity, resulting in a reduction in the number of neutrophils recruited to the inflammatory site. KO increased the expression of anti-inflammatory cytokine IL-10 and decreased pro-inflammatory cytokine IL-1β. Therefore, KO treatments balance pro- and anti-inflammatory cytokines and modulate the inflammatory response induced by UVB radiation effects (4).
Clinical studies have shown that intake of n-3 PUFA can reduce the pro-inflammatory and immunosuppressive prostaglandin E2 (PGE2) production in human skin exposed to UV radiation, reduce erythema development, and protect the skin from immunosuppression. It was supposed that supplementation ω- 3 fatty acids could reduce the occurrence of non-melanoma skin cancer in high-risk populations (116). In clinical trials, ASX plays a photoprotective role by inhibiting UVB-induced skin inflammation (117). These anti-inflammatory or anti-cancer active ingredients are main components of KO, and their beneficial mechanisms of skin health can be used as a reference for further research on KO's skin protection.
Psoriasis is also a chronic inflammatory skin disease that generally manifests as skin thickening, scales, and erythema. It can occur in various parts of the body. Currently, there is no complete cure. It can only be managed through the use of topical or systemic drugs, which affect the patient's life quality.
Alterations in fatty acid composition are observed in psoriasis, and dietary supplementation may have the potential to improve fatty acid metabolism (11). Vijayapoopathi et al. evaluated the antipsoriasis effects of KO in combination with cannabidiol and myo-inositol using a mouse model of IMQ-induced psoriasis. Mice were orally treated with nutraceutical combination twice a day for 7 consecutive days. The clinical psoriasis area and severity index (PASI) was used to score the severity of skin inflammation on the back of mice. The study found that the treatment delayed the onset of erythema and skin scaling when compared with the model group. Mice treated with the KO combination displayed reduced scales, erythema, skin thickness, ear thickness, and vascularity, along with mild-to-moderate PASI scores, indicating that KO combined with myo-inositol and cannabidiol can significantly improve IMQ-induced psoriasis in mice (118). In Skroza et al.'s research, 40 patients with psoriasis and metabolic syndrome were treated with nutritional supplements containing coenzyme Q10 and KO. Results showed that nutraceuticals restored both psoriasis and normal lipid profile (119).
Atopic dermatitis (AD) is an inflammatory skin lesion accompanied by symptoms such as itching, skin buckling, erythema, papules, skin vesicles, and skin thickening. AD is a type of allergy caused by the dysfunction of the barrier function of the skin. It affects more than 15% of children in developed countries. AD is associated with various factors, including immunological abnormalities and exposure to allergens.
The occurrence of atopic dermatitis is associated with reduced n-3 PUFA intake and increased n-6 PUFA intake. Koch et al. conducted a randomized double-blind controlled trial to explore whether n-3 PUFA can improve atopic dermatitis. The experimental group was supplemented with 5.4 grams of DHA per day, and the control group was supplemented with saturated fatty acids of the same energy. The results found that DHA significantly improved the SCORAD (Severity Score of Atopic Dermatitis) index. After intervention treatment, although the activation status of peripheral blood mononuclear cells (PBMCs) was adjusted in both groups, anti-CD40/interleukin 4-mediated IgE synthesis by PBMCs was significantly reduced only in the DHA group. In addition, among plasma fatty acids, n-3 PUFA increased and n-6/n-3 PUFA ratio decreased in the DHA group (120). Oral EPA and DHA can also promote wound healing by improving chronic inflammation. Chronic venous lower extremity ulcers (CVLUs) patients consumed 2.5 grams of EPA and 0.5 grams of DHA every day. After 56 days, the levels of matrix metalloproteinase-8 and human neutrophil elastase in the CVLU fluid of the treatment group were significantly reduced, as well as the proportion of CD66b+ cells and the wound area was significantly reduced. The reduction was significantly inversely correlated with CD15+ cells and CD66b+ cells in wound fluid. These cytokines, associated with activated polymorphonuclear leukocytes (PMNs) and their derived proteases, destroy newly developing tissue and degrade key growth factors. This study confirmed that oral treatment with EPA and DHA can improve chronic inflammation and promote the healing of CVLU wounds by regulating PMN activity (121).
Oxidative stress has been reported to play an important role in the pathophysiology and exacerbation of AD symptoms, and the use of antioxidants has been shown to be beneficial in protecting against the harmful effects of increased oxidative stress. ASX has strong antioxidant activity and anti-inflammatory effects to improve dermatitis. NC/Nga mice were used to induce an AD model by mite treatment. ASX (100 mg/kg) was administered orally once daily and thrice weekly for 26 days. Results showed that ASX significantly reduced clinical skin severity scores and reduced spontaneous scratching in AD mice. Skin histological analysis showed that the number of eosinophils, total mast cells, and degranulated mast cells in the skin of mice was significantly reduced by ASX treatment. Serum IgE levels were significantly reduced, and the mRNA and protein levels of eotaxin, migration inhibitory factor (MIF), IL-4, IL-5, and L-histidine decarboxylase were significantly reduced in ASX-treated mice. These results indicated that ASX improved dermatitis and pruritus by regulating inflammation and the expression of inflammatory cytokines (122). ASX is one of the main components of KO. The mechanism of ASX improving dermatitis may be one of the pathways through which KO works.
With the occurrence of AD, the total amount of phospholipids and the proportion of plasmalogen (PLs) in plasma decreased. PLs are an endogenous defense factor that protects the skin from oxidative stress and are in a high concentration in the skin. Krill contains a relatively high concentration of alkyl phospholipids (Alk), which is the precursor of PLs. Watanabe et al. supplemented AD-infected NC/Nga mice with phospholipid-enriched Alk extracted from krill for 3–5 weeks and found that supplementation of Alk can strengthen the skin intercellular lipid barrier and alleviate AD. After feeding Alk to AD mice, the contents of 20:5 (EPA) and 22:6 (DHA) at sn-2 position of PLs in serum were increased, while 20:4 (arachidonic acid) was decreased at this position. This showed that Alk alleviated inflammation by reducing arachidonic acid binding to PLs and increasing DHA and EPA binding to PLs (37). Ceramide is the main lipid component of the lamellar sheets present in the intercellular spaces of the stratum corneum and plays an important role in building and maintaining the skin's water-permeable barrier function (123). Pro-inflammatory cytokines were supposed to alter the activity of ceramide-metabolizing enzymes, thereby changing the ceramide composition of the skin and further leading to skin barrier damage (124). The composition of ceramides in the skin is changed in AD, which can be reversed by Alk. It was found that the ratio of non-hydroxy ceramides and hydroxy ceramides in AD mice treated with Alk was similar to that in normal mice, while it was not observed in AD mice treated with fish oil (37). The experimental study of KO and ingredients for alleviating skin inflammation is summarized in Table 3.
As a barrier organ that protects the body, the skin has a complex self-repair process, which can quickly and effectively promote wound healing. Wound healing is divided into four phases, including hemostasis (blood clot formation), inflammation (infiltration of immune cells), proliferation (angiogenesis, granulation, epithelialization, and ECM remodeling through proliferation and migration of fibroblasts and keratinocytes), as well as skin layer maturation (wound contraction and inflammation resolution). The initial inflammatory phase is characterized by platelet activation, the recruitment of immune cells, the release of growth factors and cytokine, followed by the secretion of growth factors and enhanced cell proliferation, and finally, in a remodeling phase, collagen production and histogenesis leads to mature scars (125). While the skin is susceptible to a variety of internal and external factors, when the skin is irritated by exposure to the environment, it increases inflammation, resulting in ulcerative chronic wounds, hypertrophic scars, or keloids. Cracked and dry skin is often accompanied by changes in skin lipids and loss of moisture. Physiological factors such as stress influence the wound healing process by affecting blood flow, skin metabolic and immune status, as well as hair follicle function through neurohormonal and steroid hormone levels. KO has been shown to promote wound healing by improving the function of fibroblasts and repairing endothelial angiogenesis injury. n-3 fatty acids and other active ingredients in KO help reduce inflammation, and promote cell proliferation and angiogenesis, thereby accelerating the wound healing process (Figure 2).
Some cases such as diabetes and aging can damage the wound-healing process and result in chronic, non-healing wounds (126). Diabetes mellitus (DM) can lead to excessive and prolonged inflammation, which, in turn, impairs wound repair and affects wound healing. At present, there are still few effective methods to treat diabetic wound healing (127), but KO has been shown to improve skin conditions and promote wound healing. Studies have found that topical application of KO could significantly accelerate wound healing in type 2 diabetes mellitus mice by reversing the symptoms of excessive inflammation, impaired collagen deposition, and inhibited neovascularization in diabetic mice (128). Diabetic mice induced by streptozotocin and a high-fat diet received dorsal skin incision to establish a diabetic wound model. It was found that KO treatment reduced the width and area of the wound, and promoted skin thickening and hair follicle formation at the wound (128). Furthermore, KO increased CD31, a marker of endothelial cells (ECs), and also significantly activated the expression of Angpt2, Angpt4, Vegf-a, and E-Cadherin in the skin, indicating that KO enhanced neovascularization in diabetic wounds (128). Moreover, collagen deposition is important for wound healing. TGF-β1 and α-SMA are sensitive factors for fibroblast differentiation, and their expression is inhibited under DM, thereby affecting collagen formation. KO can reactivate the expression of Tgf-β1 and Acta2 in the skin and accelerate the accumulation of collagen in diabetic wounds (128). Other studies have found that oral administration of KO significantly promoted wound healing of colonic anastomosis after colectomy in rats under the influence of inflammatory cell infiltration, fibroblast activity, angiogenesis, and collagen deposition (129).
Fatty acids are key components for cell membrane structure and function and play an active role in skin health. Peng et al. used mixed oil SMOF (soybean oil, medium-chain triglyceride, olive oil, fish oil), which was rich in n-3 PUFA, for wound healing in rats. SMOF was administered intravenously to rats with wound excision on the back. It was found that SMOF decreased the wound area significantly from 72 h. The level of IL-10 in the SMOF group increased after 48 h of treatment. And the distribution rate of fibroblasts and collagen fibers in the SMOF group is higher than the control group. It shows that SMOF rich in n-3 PUFA can promote wound healing. And this may be related to the anti-inflammatory effect of n-3 PUFA (130). In addition, local treatment with DHA combined with pigment epithelium-derived factor (PEDF) can selectively recruit type 2 macrophages and prevent neutrophil infiltration from diabetic corneal injury, thereby promoting corneal nerve regeneration and wound healing in diabetic mice (131). EPA can promote neovascularization mediated by endothelial progenitor cell migration (132).
ROS are involved in all phases of wound healing. ASX, a powerful antioxidant, has proved to be an effective compound for accelerating wound healing. In lipopolysaccharide-stimulated fibroblasts, ASX eliminated ROS and inflammatory effects and improved the cell viability and proliferative capacity (133). It was reported that ASX could enhance gingival wound healing after hyperglycemia-induced oxidative stress through its antioxidant properties (134). Healthy female mice who received full-thickness dermal wounds were topically treated with ASX twice daily for 15 days. ASX-treated wounds showed contraction by day 3 and complete wound closure by day 9. The expression of wound healing biological markers such as collagen type I alpha 1 (Col1A1) and basic fibroblast growth factor (bFGF) was increased, but the expression of oxidative stress marker inducible nitric oxide synthase (iNOS) was decreased in ASX-treated mice (135).
It has been reported that proteolytic enzymes can cause the debridement of wounds and the removal of necrotic tissue. A multienzyme preparation isolated from Antarctic krill has intense proteolytic activity on protein substrates. It is more active than other commonly used proteolytic agents for wound debridement and has sound effects on ulcerative wounds. The effect of debridement was confirmed by cell experiments, animal experiments, and clinical experiment (136–139). Overall, KO may control excessive inflammation and oxidative stress in the skin, promote new blood vessels and collagen production, and promote wound healing. The experimental study of KO and ingredients on wound healing enhancement is summarized in Table 4.
Increasing studies support the beneficial effects of KO on skin health, including anti-aging, anti-inflammatory properties, and wound healing promotion, which have been preliminarily evaluated in clinical, animal, and cellular experiments (Tables 2–4). The potential mechanisms of action are summarized in Figure 3. This indicates that KO has great potential as a skin protectant. However, further research is needed to understand the specific effects of KO on the skin under environmental stress and its potential to improve skin condition, as well as to elucidate the molecular mechanisms underlying the multiple benefits of KO on skin health. In addition, the improvement of technological processes for green extraction and transportation systems will support consumer's growing interest and demand for efficacious, safe, natural, and sustainable natural alternative skincare solutions (140). In summary, the application of KO as a therapeutic measure represents an innovative and efficient approach that can be incorporated into future skin health treatments and improvements. Further illumination of its biological function and the underlying mechanisms, and translation of the basic research findings into novel skin health interventions, dietary supplements, and therapeutic approaches for preventing chronic diseases will certainly be the focus of research for years to come.
Figure 3. Mechanisms of krill oil's role on skin health and problems. Skin aging is categorized into two types: (1) intrinsic aging, which is influenced by hormonal changes and cellular aging, and (2) extrinsic aging, which results from external factors like UV exposure, air pollution, and smoking. KO and its functional ingredients decelerate skin aging processes through antioxidation, anti-inflammation, thereby protecting skin barrier, fostering wound healing, maintaining hydration, and promoting youthful and healthy skin. KO, krill oil; n-3 PUFA, n-3 polyunsaturated fatty acids; DHA, docosahexaenoic acid; EPA, eicosapentaenoic acid; ASX, astaxanthin; PLs, phospholipids; HAS, hyaluronic acid synthesis; MMP, matrix metalloproteinases; COL1, type I collagen; GSH, glutathione; MDA, malondialdehyde; DPPH, 2,2-diphenyl-1-picrylhydrazyl; MCP-1, monocyte chemoattractant protein-1; IL-1, interleukin-1; IL-6, interleukin-6; IL-10, interleukin-10; MPO, myeloperoxidase; NF-κB, nuclear factor kappa-B; Nos2, nitric oxide synthase 2; IL-1β, interleukin-1beta; P65, NF-κB p65 protein; p-P65, phospho-NF-κB p65 protein; Tgf-β1, transforming growth factor beta 1; Acta2, actin alpha 2; CD31, platelet endothelial cell adhesion molecule-1; Angpy, angiopoietin; VEGF, vascular endothelial growth factor; TEWL, trans-epidermal water loss; PASI, psoriasis area severity index. ↑, stands for enhancement or activation; ↓, stands for weakening or inhibiting.
LD: Writing – original draft, Visualization, Investigation. JY: Writing – review & editing, Project administration, Conceptualization. XW: Writing – original draft, Visualization. GZ: Writing – original draft, Visualization. JZ: Writing – review & editing, Project administration, Conceptualization. HZ: Writing – review & editing, Writing – original draft, Visualization, Methodology, Conceptualization. ZW: Writing – review & editing, Supervision, Conceptualization. YL: Funding acquisition, Writing – review & editing, Supervision, Conceptualization.
The author(s) declare financial support was received for the research, authorship, and/or publication of this article. This work was supported by the grants from National Key R&D Program of China (2019YFA0802502), National Natural Science Foundation of China (81925008 and 32130047), Project supported by Shanghai Municipal Science and Technology Major Project, and Open Project Program of Metabolic Vascular Diseases Key Laboratory of Sichuan Province (2022MVDKL-K2) to YL. This work was also supported by Sunline Deep Sea Fishery fund.
We are grateful to Tingting Zhang, Qiuli Yang, Aoyuan Cui and Yuyin Wu (CAS Engineering Laboratory for Nutrition, SINH) for the insightful discussion and technical assistance. We are grateful to Xiaoyi Wei, Zhan Liu, Lianjia Li, and Keyu Qian (University of Chinese Academy of Sciences, SINH, CAS) for the literature search support.
JY and ZW were employed by Jiangsu Sunline Deep Sea Fishery Co., Ltd.
The remaining authors declare that the research was conducted in the absence of any commercial or financial relationships that could be construed as a potential conflict of interest.
All claims expressed in this article are solely those of the authors and do not necessarily represent those of their affiliated organizations, or those of the publisher, the editors and the reviewers. Any product that may be evaluated in this article, or claim that may be made by its manufacturer, is not guaranteed or endorsed by the publisher.
1. Hwang SM, Kim YU, Kim JK, Chun YS, Kwon YS, Ku SK, et al. Preventive and therapeutic effects of krill oil on obesity and obesity-induced metabolic syndromes in high-fat diet-fed mice. Mar Drugs. (2022) 20:483. doi: 10.3390/md20080483
2. Hals PA, Wang X, Xiao YF. Effects of a purified krill oil phospholipid rich in long-chain omega-3 fatty acids on cardiovascular disease risk factors in non-human primates with naturally occurring diabetes type-2 and dyslipidemia. Lipids Health Dis. (2017) 16:11. doi: 10.1186/s12944-017-0411-z
3. Decandia D, Gelfo F, Landolfo E, Balsamo F, Petrosini L, Cutuli D. Dietary protection against cognitive impairment, neuroinflammation and oxidative stress in Alzheimer's disease animal models of lipopolysaccharide-induced inflammation. Int J Mol Sci. (2023) 24:921. doi: 10.3390/ijms24065921
4. Kim J, Lee N, Chun YS, Lee SH, Ku SK. Krill oil's protective benefits against ultraviolet b-induced skin photoaging in hairless mice and in vitro experiments. Mar Drugs. (2023) 21:479. doi: 10.3390/md21090479
6. Tan KW, Tiddeman B, Stephen ID. Skin texture and colour predict perceived health in Asian faces. Evol Hum Behav. (2018) 39:320–35. doi: 10.1016/j.evolhumbehav.2018.02.003
7. Jeong JH, Kim MB, Kim C, Hwang JK. Inhibitory effect of vitamin C on intrinsic aging in human dermal fibroblasts and hairless mice. Food Sci Biotechnol. (2018) 27:555–64. doi: 10.1007/s10068-017-0252-6
8. Xiong ZM, O'Donovan M, Sun L, Choi JY, Ren M, Cao K. Anti-aging potentials of methylene blue for human skin longevity. Sci Rep. (2017) 7:2475. doi: 10.1038/s41598-017-02419-3
9. Nicolaou A, Pilkington SM, Rhodes LE. Ultraviolet-radiation induced skin inflammation: dissecting the role of bioactive lipids. Chem Phys Lipids. (2011) 164:535–43. doi: 10.1016/j.chemphyslip.2011.04.005
10. Dainichi T, Kitoh A, Otsuka A, Nakajima S, Nomura T, Kaplan DH, et al. The epithelial immune microenvironment (EIME) in atopic dermatitis and psoriasis. Nat Immunol. (2018) 19:1286–98. doi: 10.1038/s41590-018-0256-2
11. Kendall AC, Nicolaou A. Bioactive lipid mediators in skin inflammation and immunity. Prog Lipid Res. (2013) 52:141–64. doi: 10.1016/j.plipres.2012.10.003
12. Moore EM, Wagner C, Komarnytsky S. The enigma of bioactivity and toxicity of botanical oils for skin care. Front Pharmacol. (2020) 11:785. doi: 10.3389/fphar.2020.00785
13. Boelsma E, Hendriks HF, Roza L. Nutritional skin care: health effects of micronutrients and fatty acids. Am J Clin Nutr. (2001) 73:853–64. doi: 10.1093/ajcn/73.5.853
14. Hwang YP, Oh KN, Yun HJ, Jeong HG. The flavonoids apigenin and luteolin suppress ultraviolet A-induced matrix metalloproteinase-1 expression via MAPKs and AP-1-dependent signaling in HaCaT cells. J Dermatol Sci. (2011) 61:23–31. doi: 10.1016/j.jdermsci.2010.10.016
15. Lorencini M, Brohem CA, Dieamant GC, Zanchin NI, Maibach HI. Active ingredients against human epidermal aging. Ageing Res Rev. (2014) 15:100–15. doi: 10.1016/j.arr.2014.03.002
16. Tanaka YT, Tanaka K, Kojima H, Hamada T, Masutani T, Tsuboi M, et al. Cynaropicrin from Cynara scolymus L. suppresses photoaging of skin by inhibiting the transcription activity of nuclear factor-kappa B. Bioorg Med Chem Lett. (2013) 23:518–23. doi: 10.1016/j.bmcl.2012.11.034
17. Babilas P, Knie U, Abels C. Cosmetic and dermatologic use of alpha hydroxy acids. J Dtsch Dermatol Ges. (2012) 10:488–91. doi: 10.1111/j.1610-0387.2012.07939.x
18. Hou M, Man M, Man W, Zhu W, Hupe M, Park K, et al. Topical hesperidin improves epidermal permeability barrier function and epidermal differentiation in normal murine skin. Exp Dermatol. (2012) 21:337–40. doi: 10.1111/j.1600-0625.2012.01455.x
19. Pain S, Altobelli C, Boher A, Cittadini L, Favre-Mercuret M, Gaillard C, et al. Surface rejuvenating effect of Achillea millefolium extract. Int J Cosmet Sci. (2011) 33:535–42. doi: 10.1111/j.1468-2494.2011.00667.x
20. Buono S, Langellotti AL, Martello A, Bimonte M, Tito A, Carola A, et al. Biological activities of dermatological interest by the water extract of the microalga Botryococcus braunii. Arch Dermatol Res. (2012) 304:755–64. doi: 10.1007/s00403-012-1250-4
21. Ishikawa J, Shimotoyodome Y, Chen S, Ohkubo K, Takagi Y, Fujimura T, et al. Eucalyptus increases ceramide levels in keratinocytes and improves stratum corneum function. Int J Cosmet Sci. (2012) 34:17–22. doi: 10.1111/j.1468-2494.2011.00675.x
22. Liu FW, Liu FC, Wang YR, Tsai HI, Yu HP. Aloin protects skin fibroblasts from heat stress-induced oxidative stress damage by regulating the oxidative defense system. PLoS ONE. (2015) 10:e0143528. doi: 10.1145/2818302
23. Sanchez M, Gonzalez-Burgos E, Iglesias I, Gomez-Serranillos MP. Pharmacological update properties of aloe vera and its major active constituents. Molecules. (2020) 25:324. doi: 10.3390/molecules25061324
24. Li Z, Jiang R, Wang M, Zhai L, Liu J, Xu X, et al. Ginsenosides repair UVB-induced skin barrier damage in BALB/c hairless mice and HaCaT keratinocytes. J Ginseng Res. (2022) 46:115–25. doi: 10.1016/j.jgr.2021.05.001
25. Lim TG, Lee CC, Dong Z, Lee KW. Ginsenosides and their metabolites: a review of their pharmacological activities in the skin. Arch Dermatol Res. (2015) 307:397–403. doi: 10.1007/s00403-015-1569-8
26. Contardi M, Lenzuni M, Fiorentini F, Summa M, Bertorelli R, Suarato G, et al. Hydroxycinnamic acids and derivatives formulations for skin damages and disorders: a review. Pharmaceutics. (2021) 13:999. doi: 10.3390/pharmaceutics13070999
27. Taofiq O, Gonzalez-Paramas AM, Barreiro MF, Ferreira IC. Hydroxycinnamic acids and their derivatives: cosmeceutical significance, challenges and future perspectives, a review. Molecules. (2017) 22:281. doi: 10.3390/molecules22020281
28. Davinelli S, Nielsen ME, Scapagnini G. Astaxanthin in skin health, repair, and disease: a comprehensive review. Nutrients. (2018) 10:522. doi: 10.3390/nu10040522
29. Zhou X, Cao Q, Orfila C, Zhao J, Zhang L. Systematic review and meta-analysis on the effects of astaxanthin on human skin ageing. Nutrients. (2021) 13:917. doi: 10.3390/nu13092917
30. Choi FD, Sung CT, Juhasz ML, Mesinkovsk NA. Oral collagen supplementation: a systematic review of dermatological applications. J Drugs Dermatol. (2019) 18:9–16.
31. Abu-Baker S, Chu Z, Stevens AM, Li J, Qi X. Cytotoxicity and selectivity in skin cancer by SapC-DOPS nanovesicles. J Cancer Ther. (2012) 3:321–6. doi: 10.4236/jct.2012.34041
32. Salem HF, Gamal A, Saeed H, Kamal M, Tulbah AS. Enhancing the bioavailability and efficacy of vismodegib for the control of skin cancer: in vitro and in vivo studies. Pharmaceuticals. (2022) 15:126. doi: 10.3390/ph15020126
33. Sriram R, Gopal V. Aging skin and natural bioactives that impede cutaneous aging: a narrative review. Indian J Dermatol. (2023) 68:414–24. doi: 10.4103/ijd.ijd_932_22
34. Xie D, Gong M, Wei W, Jin J, Wang X, Wang X, et al. Antarctic krill (Euphausia superba) oil: a comprehensive review of chemical composition, extraction technologies, health benefits, and current applications. Compr Rev Food Sci Food Saf. (2019) 18:514–34. doi: 10.1111/1541-4337.12427
35. Komatsu T, Sasaki S, Manabe Y, Hirata T, Sugawara T. Preventive effect of dietary astaxanthin on UVA-induced skin photoaging in hairless mice. PLoS ONE. (2017) 12:e0171178. doi: 10.1371/journal.pone.0171178
36. Wang P, Yan G, Xue H, Shen S, Cao Y, Zhang G, et al. Proteomics and lipidomics reveal the protective mechanism of dietary n-3 PUFA supplementation for photoaging. Food Funct. (2021) 12:7883–96. doi: 10.1039/D0FO03228J
37. Watanabe N, Suzuki T, Yamazaki Y, Sugiyama K, Koike S, Nishimukai M. Supplemental feeding of phospholipid-enriched alkyl phospholipid from krill relieves spontaneous atopic dermatitis and strengthens skin intercellular lipid barriers in NC/Nga mice. Biosci Biotechnol Biochem. (2019) 83:717–27. doi: 10.1080/09168451.2018.1559024
38. Huang L, Wu W, Huang L, Zhong J, Chen L, Wang M, et al. Antarctic krill (Euphausia superba) oil modulatory effects on ethanol-induced acute injury of the gastric mucosa in rats. Front Nutr. (2022) 9:1003627. doi: 10.3389/fnut.2022.1003627
39. Qiang X, Guo C, Gu W, Song Y, Zhang Y, Gong X, et al. The Complex of phycobiliproteins, fucoxanthin, and krill oil ameliorates obesity through modulation of lipid metabolism and antioxidants in obese rats. Nutrients. (2022) 14:815. doi: 10.3390/nu14224815
40. Ku SK, Kim JK, Chun YS, Song CH. Anti-osteoarthritic effects of antarctic krill oil in primary chondrocytes and a surgical rat model of knee osteoarthritis. Mar Drugs. (2023) 21:513. doi: 10.3390/md21100513
41. SenGupta T, Lefol Y, Lirussi L, Suaste V, Luders T, Gupta S, et al. Krill oil protects dopaminergic neurons from age-related degeneration through temporal transcriptome rewiring and suppression of several hallmarks of aging. Aging. (2022) 14:8661–87. doi: 10.18632/aging.204375
42. Zhou L, Wu X, Yang F, Zhang M, Huang R, Liu J. Characterization of molecular species and anti-inflammatory activity of purified phospholipids from antarctic krill oil. Mar Drugs. (2021) 19:124. doi: 10.3390/md19030124
43. Panchal D, Pandya T, Kevlani V, Shah S, Acharya S. Development and evaluation of novel krill oil-based clomiphene microemulsion as a therapeutic strategy for PCOS treatment. Drug Deliv Transl Res. (2023) 13:2254–71. doi: 10.1007/s13346-023-01304-z
44. Shi J, Wang Y, Lei Y, Chen X, Liu Y, Xu YJ. Lipidome reveals the alleviation of acrylamide-induced impairment by krill oil. Food Funct. (2022) 13:8012–21. doi: 10.1039/D2FO00781A
45. Du Y, Song L, Dong X, Li H, Xie W, Wang Y, et al. Long-term krill oil administration alleviated early mild cognitive impairment in APP/PS1 mice. Mol Nutr Food Res. (2024) 68:e2200652. doi: 10.1002/mnfr.202200652
46. Singlar Z, Szentesi P, Fodor J, Angyal A, Csernoch L, Sztretye M. Assessing the potential of nutraceuticals as geroprotectors on muscle performance and cognition in aging mice. Antioxidants. (2021) 10:415. doi: 10.3390/antiox10091415
47. Guo P, Xue M, Teng X, Wang Y, Ren R, Han J, et al. Antarctic krill oil ameliorates liver injury in rats exposed to alcohol by regulating bile acids metabolism and gut microbiota. J Nutr Biochem. (2022) 107:109061. doi: 10.1016/j.jnutbio.2022.109061
48. Liu F, Smith AD, Solano-Aguilar G, Wang TTY, Pham Q, Beshah E, et al. Mechanistic insights into the attenuation of intestinal inflammation and modulation of the gut microbiome by krill oil using in vitro and in vivo models. Microbiome. (2020) 8:83. doi: 10.1186/s40168-020-00843-8
49. Liu F, Smith AD, Wang TTY, Pham Q, Hou P, Cheung L, et al. Phospholipid-rich krill oil promotes intestinal health by strengthening beneficial gut microbial interactions in an infectious colitis model. Food Funct. (2024) 15:2604–15. doi: 10.1039/D3FO04980A
50. Colletti A, Cravotto G, Citi V, Martelli A, Testai L, Cicero AFG. Advances in technologies for highly active omega-3 fatty acids from krill oil: clinical applications. Mar Drugs. (2021) 19:306. doi: 10.3390/md19060306
51. Araujo P, Zhu H, Breivik JF, Hjelle JI, Zeng Y. Determination and structural elucidation of triacylglycerols in krill oil by chromatographic techniques. Lipids. (2014) 49:163–72. doi: 10.1007/s11745-013-3855-6
52. Burri L, Johnsen L. Krill products: an overview of animal studies. Nutrients. (2015) 7:3300–21. doi: 10.3390/nu7053300
53. Sun W, Shi B, Xue C, Jiang X. The comparison of krill oil extracted through ethanol-hexane method and subcritical method. Food Sci Nutr. (2019) 7:700–10. doi: 10.1002/fsn3.914
54. Tou JC, Jaczynski J, Chen YC. Krill for human consumption: nutritional value and potential health benefits. Nutr Rev. (2007) 65:63–77. doi: 10.1111/j.1753-4887.2007.tb00283.x
55. Barros MP, Poppe SC, Bondan EF. Neuroprotective properties of the marine carotenoid astaxanthin and omega-3 fatty acids, and perspectives for the natural combination of both in krill oil. Nutrients. (2014) 6:1293–317. doi: 10.3390/nu6031293
56. Teng XN, Wang SC, Zeb L, Dong YS, Xiu ZL. Two-step enzymolysis of antarctic krill for simultaneous preparation of value-added oil and enzymolysate. Mar Drugs. (2023) 21:047. doi: 10.3390/md21010047
57. Lu FS, Bruheim I, Haugsgjerd BO, Jacobsen C. Effect of temperature towards lipid oxidation and non-enzymatic browning reactions in krill oil upon storage. Food Chem. (2014) 157:398–407. doi: 10.1016/j.foodchem.2014.02.059
58. Song G, Wang H, Zhang M, Zhang Y, Wang H, Yu X, et al. Real-time monitoring of the oxidation characteristics of antarctic krill oil (Euphausia superba) during storage by electric soldering iron ionization mass spectrometry-based lipidomics. J Agric Food Chem. (2020) 68:1457–67. doi: 10.1021/acs.jafc.9b07370
59. Ulven SM, Kirkhus B, Lamglait A, Basu S, Elind E, Haider T, et al. Metabolic effects of krill oil are essentially similar to those of fish oil but at lower dose of EPA and DHA, in healthy volunteers. Lipids. (2011) 46:37–46. doi: 10.1007/s11745-010-3490-4
60. Ramprasath VR, Eyal I, Zchut S, Jones PJ. Enhanced increase of omega-3 index in healthy individuals with response to 4-week n-3 fatty acid supplementation from krill oil versus fish oil. Lipids Health Dis. (2013) 12:178. doi: 10.1186/1476-511X-12-178
61. Batetta B, Griinari M, Carta G, Murru E, Ligresti A, Cordeddu L, et al. Endocannabinoids may mediate the ability of (n-3) fatty acids to reduce ectopic fat and inflammatory mediators in obese Zucker rats. J Nutr. (2009) 139:1495–501. doi: 10.3945/jn.109.104844
62. Stillwell W, Wassall SR. Docosahexaenoic acid: membrane properties of a unique fatty acid. Chem Phys Lipids. (2003) 126:1–27. doi: 10.1016/S0009-3084(03)00101-4
63. Burger B, Sagiorato RN, Silva JR, Candreva T, Pacheco MR, White D, et al. Eicosapentaenoic acid-rich oil supplementation activates PPAR-gamma and delays skin wound healing in type 1 diabetic mice. Front Immunol. (2023) 14:1141731. doi: 10.3389/fimmu.2023.1141731
64. Kim HH, Shin CM, Park CH, Kim KH, Cho KH, Eun HC, et al. Eicosapentaenoic acid inhibits UV-induced MMP-1 expression in human dermal fibroblasts. J Lipid Res. (2005) 46:1712–20. doi: 10.1194/jlr.M500105-JLR200
65. Latreille J, Kesse-Guyot E, Malvy D, Andreeva V, Galan P, Tschachler E, et al. Association between dietary intake of n-3 polyunsaturated fatty acids and severity of skin photoaging in a middle-aged Caucasian population. J Dermatol Sci. (2013) 72:233–9. doi: 10.1016/j.jdermsci.2013.07.006
66. Devarshi PP, Grant RW, Ikonte CJ, Hazels Mitmesser S. Maternal omega-3 nutrition, placental transfer and fetal brain development in gestational diabetes and preeclampsia. Nutrients. (2019) 11:107. doi: 10.3390/nu11051107
67. James G, Bohannan W, Adewunmi E, Schmidt K, Park HG, Shchepinov MS, et al. Pharmacokinetics and metabolism in mouse retina of bis-allylic deuterated docosahexaenoic acid (D-DHA), a new dry AMD drug candidate. Exp Eye Res. (2022) 222:109193. doi: 10.1016/j.exer.2022.109193
68. Mori TA. Dietary n-3 PUFA and CVD: a review of the evidence. Proc Nutr Soc. (2014) 73:57–64. doi: 10.1017/S0029665113003583
69. Kulzow N, Witte AV, Kerti L, Grittner U, Schuchardt JP, Hahn A, et al. Impact of omega-3 fatty acid supplementation on memory functions in healthy older adults. J Alzheimers Dis. (2016) 51:713–25. doi: 10.3233/JAD-150886
70. Kiecolt-Glaser JK, Belury MA, Andridge R, Malarkey WB, Glaser R. Omega-3 supplementation lowers inflammation and anxiety in medical students: a randomized controlled trial. Brain Behav Immun. (2011) 25:1725–34. doi: 10.1016/j.bbi.2011.07.229
71. Michalak M, Pierzak M, Krecisz B, Suliga E. Bioactive compounds for skin health: a review. Nutrients. (2021) 13:203. doi: 10.3390/nu13010203
72. Yum HW, Park J, Park HJ, Shin JW, Cho YY, Kim SJ, et al. Endogenous omega-3 fatty acid production by fat-1 transgene and topically applied docosahexaenoic acid protect against UVB-induced mouse skin carcinogenesis. Sci Rep. (2017) 7:11658. doi: 10.1038/s41598-017-11443-2
73. Calder PC. Marine omega-3 fatty acids and inflammatory processes: effects, mechanisms and clinical relevance. Biochim Biophys Acta. (2015) 1851:469–84. doi: 10.1016/j.bbalip.2014.08.010
74. Jung JY, Kwon HH, Hong JS, Yoon JY, Park MS, Jang MY, et al. Effect of dietary supplementation with omega-3 fatty acid and gamma-linolenic acid on acne vulgaris: a randomised, double-blind, controlled trial. Acta Derm Venereol. (2014) 94:521–5. doi: 10.2340/00015555-1802
75. Guida B, Napoleone A, Trio R, Nastasi A, Balato N, Laccetti R, et al. Energy-restricted, n-3 polyunsaturated fatty acids-rich diet improves the clinical response to immuno-modulating drugs in obese patients with plaque-type psoriasis: a randomized control clinical trial. Clin Nutr. (2014) 33:399–405. doi: 10.1016/j.clnu.2013.09.010
76. Donat-Vargas C, Berglund M, Glynn A, Wolk A, Akesson A. Dietary polychlorinated biphenyls, long-chain n-3 polyunsaturated fatty acids and incidence of malignant melanoma. Eur J Cancer. (2017) 72:137–43. doi: 10.1016/j.ejca.2016.11.016
77. Komprda T. Effect of n-3 long-chain polyunsaturated fatty acids on wound healing using animal models – a review. Acta Veterinaria Brno. (2018) 80:309–20. doi: 10.2754/avb201887040309
78. Cohen G, Jakus J, Portillo M, Gvirtz R, Ogen-Shtern N, Silberstein E, et al. In vitro, ex vivo, and clinical evaluation of anti-aging gel containing EPA and CBD. J Cosmet Dermatol. (2023) 22:3047–57. doi: 10.1111/jocd.15815
79. Chen J, Wei Y, Chen X, Jiao J, Zhang Y. Polyunsaturated fatty acids ameliorate aging via redox-telomere-antioncogene axis. Oncotarget. (2017) 8:7301–14. doi: 10.18632/oncotarget.14236
80. Kim HH, Cho S, Lee S, Kim KH, Cho KH, Eun HC, et al. Photoprotective and anti-skin-aging effects of eicosapentaenoic acid in human skin in vivo. J Lipid Res. (2006) 47:921–30. doi: 10.1194/jlr.M500420-JLR200
81. Stachowiak B, Szulc P. Astaxanthin for the Food Industry. Molecules. (2021) 26:666. doi: 10.3390/molecules26092666
82. Naguib YM. Antioxidant activities of astaxanthin and related carotenoids. J Agric Food Chem. (2000) 48:1150–4. doi: 10.1021/jf991106k
83. Ortiz Sánchez CA, Zavaleta EB, Urrea García GR, Solano GL, Rascón Díaz MP. Krill oil microencapsulation: antioxidant activity, astaxanthin retention, encapsulation efficiency, fatty acids profile, in vitro bioaccessibility and storage stability. Food Sci Technol. (2021) 147:111476. doi: 10.1016/j.lwt.2021.111476
84. Zeng XB, Yin FW, Zhao GH, Guo C, Li DY, Liu HL, et al. Mechanism of color change in Antarctic krill oil during storage. Food Chem. (2024) 444:138583. doi: 10.1016/j.foodchem.2024.138583
85. Shatoor AS, Al Humayed S. Astaxanthin Ameliorates high-fat diet-induced cardiac damage and fibrosis by upregulating and activating SIRT1. Saudi J Biol Sci. (2021) 28:7012–21. doi: 10.1016/j.sjbs.2021.07.079
86. Ito N, Saito H, Seki S, Ueda F, Asada T. Effects of composite supplement containing astaxanthin and sesamin on cognitive functions in people with mild cognitive impairment: a randomized, double-blind, placebo-controlled trial. J Alzheimers Dis. (2018) 62:1767–75. doi: 10.3233/JAD-170969
87. Balcerczyk A, Gajewska A, Macierzynska-Piotrowska E, Pawelczyk T, Bartosz G, Szemraj J. Enhanced antioxidant capacity and anti-ageing biomarkers after diet micronutrient supplementation. Molecules. (2014) 19:14794–808. doi: 10.3390/molecules190914794
88. Higuera-Ciapara I, Felix-Valenzuela L, Goycoolea FM. Astaxanthin: a review of its chemistry and applications. Crit Rev Food Sci Nutr. (2006) 46:185–96. doi: 10.1080/10408690590957188
89. Chung BY, Park SH, Yun SY, Yu DS, Lee YB. Astaxanthin protects ultraviolet B-induced oxidative stress and apoptosis in human keratinocytes via intrinsic apoptotic pathway. Ann Dermatol. (2022) 34:125–31. doi: 10.5021/ad.2022.34.2.125
90. Chalyk NE, Klochkov VA, Bandaletova TY, Kyle NH, Petyaev IM. Continuous astaxanthin intake reduces oxidative stress and reverses age-related morphological changes of residual skin surface components in middle-aged volunteers. Nutr Res. (2017) 48:40–8. doi: 10.1016/j.nutres.2017.10.006
91. Tominaga K, Hongo N, Fujishita M, Takahashi Y, Adachi Y. Protective effects of astaxanthin on skin deterioration. J Clin Biochem Nutr. (2017) 61:33–9. doi: 10.3164/jcbn.17-35
92. Tominaga K, Hongo N, Karato M, Yamashita E. Cosmetic benefits of astaxanthin on humans subjects. Acta Biochim Pol. (2012) 59:43–7. doi: 10.18388/abp.2012_2168
93. Ácsová A, Hojerová J, Hergesell K, Hideg É, Csepregi K, Bauerová K, et al. Antioxidant and anti-pollution effect of naturally occurring carotenoids astaxanthin and crocin for human skin protection. Chem Eur. (2022) 7:e202201595. doi: 10.1002/slct.202201595
94. Lei L, Su J, Chen J, Chen W, Chen X, Peng C. The role of lysophosphatidic acid in the physiology and pathology of the skin. Life Sci. (2019) 220:194–200. doi: 10.1016/j.lfs.2018.12.040
95. Liu L, Bartke N, Van Daele H, Lawrence P, Qin X, Park HG, et al. Higher efficacy of dietary DHA provided as a phospholipid than as a triglyceride for brain DHA accretion in neonatal piglets. J Lipid Res. (2014) 55:531–9. doi: 10.1194/jlr.M045930
96. Wijendran V, Huang MC, Diau GY, Boehm G, Nathanielsz PW, Brenna JT. Efficacy of dietary arachidonic acid provided as triglyceride or phospholipid as substrates for brain arachidonic acid accretion in baboon neonates. Pediatr Res. (2002) 51:265–72. doi: 10.1203/00006450-200203000-00002
97. Zhang TT, Xu J, Wang YM, Xue CH. Health benefits of dietary marine DHA/EPA-enriched glycerophospholipids. Prog Lipid Res. (2019) 75:100997. doi: 10.1016/j.plipres.2019.100997
98. Hsieh YS, Chen YF, Cheng YY, Liu WY, Wu YT. Self-Emulsifying Phospholipid Preconcentrates for the Enhanced Photoprotection of Luteolin. Pharmaceutics. (2022) 14:896. doi: 10.3390/pharmaceutics14091896
99. Papakonstantinou E, Roth M, Karakiulakis G. Hyaluronic acid: A key molecule in skin aging. Dermatoendocrinol. (2012) 4:253–8. doi: 10.4161/derm.21923
100. Robert L, Labat-Robert J, Robert AM. Physiology of skin aging. Clin Plast Surg. (2012) 39:1–8. doi: 10.1016/j.cps.2011.09.006
101. Farage MA, Miller KW, Elsner P, Maibach HI. Intrinsic and extrinsic factors in skin ageing: a review. Int J Cosmet Sci. (2008) 30:87–95. doi: 10.1111/j.1468-2494.2007.00415.x
102. Salminen A, Kaarniranta K, Kauppinen A. Photoaging: UV radiation-induced inflammation and immunosuppression accelerate the aging process in the skin. Inflamm Res. (2022) 71:817–31. doi: 10.1007/s00011-022-01598-8
103. Ansary TM, Hossain MR, Kamiya K, Komine M, Ohtsuki M. Inflammatory molecules associated with ultraviolet radiation-mediated skin aging. Int J Mol Sci. (2021) 22:974. doi: 10.3390/ijms22083974
104. Ito N, Seki S, Ueda F. The protective role of astaxanthin for UV-induced skin deterioration in healthy people-a randomized, double-blind, placebo-controlled trial. Nutrients. (2018) 10:817. doi: 10.3390/nu10070817
105. Cho S, Kim HH, Lee MJ, Lee S, Park CS, Nam SJ, et al. Phosphatidylserine prevents UV-induced decrease of type I procollagen and increase of MMP-1 in dermal fibroblasts and human skin in vivo. J Lipid Res. (2008) 49:1235–45. doi: 10.1194/jlr.M700581-JLR200
106. Ahn Y, Kim MG, Jo K, Hong KB, Suh HJ. Effects of sphingomyelin-containing milk phospholipids on skin hydration in UVB-exposed hairless mice. Molecules. (2022) 27:545. doi: 10.3390/molecules27082545
107. Lee K, Kim S, Kim A, Suh HJ, Hong KB. Sphingolipid identification and skin barrier recovery capacity of a milk sphingolipid-enriched fraction (MSEF) from buttermilk powder. Int J Cosmet Sci. (2020) 42:270–6. doi: 10.1111/ics.12612
108. Meguro S, Arai Y, Masukawa Y, Uie K, Tokimitsu I. Relationship between covalently bound ceramides and transepidermal water loss (TEWL). Arch Dermatol Res. (2000) 292:463–8. doi: 10.1007/s004030000160
109. Dai G, Freudenberger T, Zipper P, Melchior A, Grether-Beck S, Rabausch B, et al. Chronic ultraviolet B irradiation causes loss of hyaluronic acid from mouse dermis because of down-regulation of hyaluronic acid synthases. Am J Pathol. (2007) 171:1451–61. doi: 10.2353/ajpath.2007.070136
110. Fasano E, Serini S, Mondella N, Trombino S, Celleno L, Lanza P, et al. Antioxidant and anti-inflammatory effects of selected natural compounds contained in a dietary supplement on two human immortalized keratinocyte lines. Biomed Res Int. (2014) 2014:327452. doi: 10.1155/2014/327452
111. Serrano G, Almudever P, Serrano JM, Milara J, Torrens A, Exposito I, et al. Phosphatidylcholine liposomes as carriers to improve topical ascorbic acid treatment of skin disorders. Clin Cosmet Investig Dermatol. (2015) 8:591–9. doi: 10.2147/CCID.S90781
112. Botelho MA, Rao VS, Carvalho CB, Bezerra-Filho JG, Fonseca SG, Vale ML, et al. Lippia sidoides and Myracrodruon urundeuva gel prevents alveolar bone resorption in experimental periodontitis in rats. J Ethnopharmacol. (2007) 113:471–8. doi: 10.1016/j.jep.2007.07.010
113. Campanini MZ, Pinho-Ribeiro FA, Ivan AL, Ferreira VS, Vilela FM, Vicentini FT, et al. Efficacy of topical formulations containing Pimenta pseudocaryophyllus extract against UVB-induced oxidative stress and inflammation in hairless mice. J Photochem Photobiol B. (2013) 127:153–60. doi: 10.1016/j.jphotobiol.2013.08.007
114. Iseri SO, Sener G, Yuksel M, Contuk G, Cetinel S, Gedik N, et al. Ghrelin against alendronate-induced gastric damage in rats. J Endocrinol. (2005) 187:399–406. doi: 10.1677/joe.1.06432
115. Sullivan GW, Sarembock IJ, Linden J. The role of inflammation in vascular diseases. J Leukoc Biol. (2000) 67:591–602. doi: 10.1002/jlb.67.5.591
116. Black HS, Rhodes LE. Potential benefits of omega-3 fatty acids in non-melanoma skin cancer. J Clin Med. (2016) 5:23. doi: 10.3390/jcm5020023
117. Chang MX, Xiong F. Astaxanthin and its effects in inflammatory responses and inflammation-associated diseases: recent advances and future directions. Molecules. (2020) 25:342. doi: 10.3390/molecules25225342
118. Vijayapoopathi S, Ramamoorthy R, Meganathan J, Kalaiyazhagan A, Bhuvarahamurthy S, Venugopal B. Nutraceutical combination ameliorates imiquimod-induced psoriasis in mice. Chem Biol Drug Design. (2023) 102:1578–87.doi: 10.1111/cbdd.14350
119. Skroza N, Proietti I, Bernardini N, La Viola G, Nicolucci F, Pampena R, et al. Efficacy of food supplement to improve metabolic syndrome parameters in patients affected by moderate to severe psoriasis during anti-TNFalpha treatment. G Ital Dermatol Venereol. (2013) 148:661–5. doi: 10.1155/2013/983902
120. Koch C, Dolle S, Metzger M, Rasche C, Jungclas H, Ruhl R, et al. Docosahexaenoic acid (DHA) supplementation in atopic eczema: a randomized, double-blind, controlled trial. Br J Dermatol. (2008) 158:786–92. doi: 10.1111/j.1365-2133.2007.08430.x
121. McDaniel JC, Szalacha L, Sales M, Roy S, Chafee S, Parinandi N. EPA + DHA supplementation reduces PMN activation in microenvironment of chronic venous leg ulcers: a randomized, double-blind, controlled study. Wound Repair Regen. (2017) 25:680–90. doi: 10.1111/wrr.12558
122. Yoshihisa Y, Andoh T, Matsunaga K, Rehman MU, Maoka T, Shimizu T. Efficacy of astaxanthin for the treatment of atopic dermatitis in a murine model. PLoS ONE. (2016) 11:e0152288. doi: 10.1371/journal.pone.0152288
123. Coderch L, Lopez O, de la Maza A, Parra JL. Ceramides and skin function. Am J Clin Dermatol. (2003) 4:107–29. doi: 10.2165/00128071-200304020-00004
124. Berth-Jones J, Damstra RJ, Golsch S, Livden JK, Van Hooteghem O, Allegra F, et al. Twice weekly fluticasone propionate added to emollient maintenance treatment to reduce risk of relapse in atopic dermatitis: randomised, double blind, parallel group study. BMJ. (2003) 326:1367. doi: 10.1136/bmj.326.7403.1367
125. Baum CL, Arpey CJ. Normal cutaneous wound healing: clinical correlation with cellular and molecular events. Dermatol Surg. (2005) 31:674–86. doi: 10.1097/00042728-200506000-00011
126. Wilkinson HN, Hardman MJ. Wound healing: cellular mechanisms and pathological outcomes. Open Biol. (2020) 10:200223. doi: 10.1098/rsob.200223
127. Li M, Hou Q, Zhong L, Zhao Y, Fu X. Macrophage related chronic inflammation in non-healing wounds. Front Immunol. (2021) 12:681710. doi: 10.3389/fimmu.2021.681710
128. Hao W, Meng H, Li H, Zheng Y, Song C, Jiang Z, et al. Local application of krill oil accelerates the healing of artificially created wounds in diabetic mice. Nutrients. (2022) 14:139. doi: 10.3390/nu14194139
129. Ferhatoglu MF, Kivilcim T, Vural G, Kartal A, Filiz AI, Kebudi A. Comparison of the effects of two different marine-derived omega-3 fatty acid sources, krill oil, and fish oil, on the healing of primary colonic anastomoses after colectomy applied Wistar albino rat model. Ulus Travma Acil Cerrahi Derg. (2019) 25:324–30. doi: 10.14744/tjtes.2019.03051
130. Peng YC, Yang FL, Subeq YM, Tien CC, Chao YC, Lee RP. Lipid emulsion enriched in omega-3 PUFA accelerates wound healing: a placebo-controlled animal study. World J Surg. (2018) 42:1714–20. doi: 10.1007/s00268-017-4404-x
131. He J, Pham TL, Kakazu A, Bazan HEP. Recovery of corneal sensitivity and increase in nerve density and wound healing in diabetic mice after PEDF plus DHA treatment. Diabetes. (2017) 66:2511–20. doi: 10.2337/db17-0249
132. Kaur G, Cameron-Smith D, Garg M, Sinclair AJ. Docosapentaenoic acid (22:5n-3): a review of its biological effects. Prog Lipid Res. (2011) 50:28–34. doi: 10.1016/j.plipres.2010.07.004
133. Chae SY, Park R, Hong SW. Surface-mediated high antioxidant and anti-inflammatory effects of astaxanthin-loaded ultrathin graphene oxide film that inhibits the overproduction of intracellular reactive oxygen species. Biomater Res. (2022) 26:30. doi: 10.1186/s40824-022-00276-4
134. Aras-Tosun D, Onder C, Akdogan N, Kurgan S, Aktay I, Tuncay E, et al. Astaxanthin enhances gingival wound healing following high glucose-induced oxidative stress. Biomed Res Int. (2022) 2022:4043105. doi: 10.1155/2022/4043105
135. Meephansan J, Rungjang A, Yingmema W, Deenonpoe R, Ponnikorn S. Effect of astaxanthin on cutaneous wound healing. Clin Cosmet Investig Dermatol. (2017) 10:259–65. doi: 10.2147/CCID.S142795
136. Anheller JE, Hellgren L, Karlstam B, Vincent J. Biochemical and biological profile of a new enzyme preparation from Antarctic krill (E. superba) suitable for debridement of ulcerative lesions. Arch Dermatol Res. (1989) 281:105–10. doi: 10.1007/BF00426587
137. Mekkes JR, Le Poole IC, Das PK, Bos JD, Westerhof W. Efficient debridement of necrotic wounds using proteolytic enzymes derived from Antarctic krill: a double-blind, placebo-controlled study in a standardized animal wound model. Wound Repair Regen. (1998) 6:50–7. doi: 10.1046/j.1524-475X.1998.60108.x
138. Mekkes JR, Le Poole IC, Das PK, Kammeyer A, Westerhof W. In vitro tissue-digesting properties of krill enzymes compared with fibrinolysin/DNAse, papain and placebo. Int J Biochem Cell Biol. (1997) 29:703–06. doi: 10.1016/S1357-2725(96)00168-9
139. Westerhof W., Van Ginkel, C. J. W., Cohen, E. B., and Mekkes, J. R. (1990). Prospective randomized study comparing the debriding effect of krill enzymes and a non-enzymatic treatment in venous leg ulcers. Dermatologica 181(4):293–297. doi: 10.1159/000247828
Keywords: krill oil, skin health, anti-aging, anti-inflammatory, wound healing, antioxidant, ultraviolet protection
Citation: Duo L, Yang J, Wang X, Zhang G, Zhao J, Zou H, Wang Z and Li Y (2024) Krill oil: nutraceutical potential in skin health and disease. Front. Nutr. 11:1388155. doi: 10.3389/fnut.2024.1388155
Received: 19 February 2024; Accepted: 14 June 2024;
Published: 12 July 2024.
Edited by:
Tomás García-Cayuela, Tecnologico de Monterrey, MexicoReviewed by:
Lei Wang, Ocean University of China, ChinaCopyright © 2024 Duo, Yang, Wang, Zhang, Zhao, Zou, Wang and Li. This is an open-access article distributed under the terms of the Creative Commons Attribution License (CC BY). The use, distribution or reproduction in other forums is permitted, provided the original author(s) and the copyright owner(s) are credited and that the original publication in this journal is cited, in accordance with accepted academic practice. No use, distribution or reproduction is permitted which does not comply with these terms.
*Correspondence: Yu Li, bGl5dUBzaW5oLmFjLmNu; Zhi Wang, bGF1cmVudC53YW5nQGNtaWdyb3VwLmNvbS5jbg==; Hong Zou, aHpob3UwMUBzaW5oLmFjLmNu
†These authors have contributed equally to this work and share first authorship
Disclaimer: All claims expressed in this article are solely those of the authors and do not necessarily represent those of their affiliated organizations, or those of the publisher, the editors and the reviewers. Any product that may be evaluated in this article or claim that may be made by its manufacturer is not guaranteed or endorsed by the publisher.
Research integrity at Frontiers
Learn more about the work of our research integrity team to safeguard the quality of each article we publish.