- 1Laboratory of Neuroimaging (LNI), National Institute on Alcohol Abuse and Alcoholism, Bethesda, MD, United States
- 2Center for Studies of Addiction, Department of Psychiatry, Perelman School of Medicine, University of Pennsylvania, Philadelphia, PA, United States
Background and aims: Increasing evidence suggests that a ketogenic (high-fat, low-carbohydrate) diet (KD) intervention reduces alcohol withdrawal severity and alcohol craving in individuals with alcohol use disorder (AUD) by shifting brain energetics from glucose to ketones. We hypothesized that the KD would reduce a neurobiological craving signature when individuals undergoing alcohol detoxification treatment were exposed to alcohol cues.
Methods: We performed a secondary analysis of functional magnetic resonance data of 33 adults with an AUD who were randomized to a KD (n = 19) or a standard American diet (SA; n = 14) and underwent 3 weeks of inpatient alcohol detoxification treatment. Once per week, participants performed an alcohol cue-reactivity paradigm with functional magnetic resonance imaging. We extracted brain responses to food and alcohol cues and quantified the degree to which each set of brain images shared a pattern of activation with a recently established ‘Neurobiological Craving Signature’ (NCS). We then performed a group-by-time repeated measures ANOVA to test for differences in craving signature expression between the dietary groups over the three-week treatment period. We also correlated these expression patterns with self-reported wanting ratings for alcohol cues.
Results: For alcohol relative to food cues, there was a main effect of group, such that the KD group showed lower NCS expression across all 3 weeks of treatment. The main effect of time and the group-by-time interaction were not significant. Self-reported wanting for alcohol cues reduced with KD compared to SA but did not correlate with the NCS score.
Conclusion: A ketogenic diet reduces self-reported alcohol wanting, and induced lower NCS to alcohol cues during inpatient treatment for AUD. However, in the KD group alcohol wanting continued to decrease across the 3 weeks of abstinence while the NCS scores remained stable, suggesting that this cue-induced NCS may not fully capture ongoing, non-cue-induced alcohol desire.
Introduction
Alcohol use disorder (AUD) is a chronic relapsing condition that accounts for 5% of deaths globally (1) and is characterized by high levels of craving and drinking despite negative consequences. Fewer than 4% of patients with AUD receive an FDA-approved medication to treat the disorder (2) and available treatments are not efficacious for all patients, and have clinical limitations due to side effects, which can reduce adherence (3, 4). Thus, there is a critical need to identify treatments that can reduce alcohol craving and consumption.
Improving brain energetics by means of a ketogenic diet (KD; high-fat, low-carbohydrate diet) intervention may be a novel avenue for treatment of AUD (5, 6). Acute alcohol intake shifts brain energetics from glucose to acetate, an alcohol metabolite (7, 8). In heavy alcohol drinkers and individuals with AUD, low brain glucose and high acetate metabolism persist beyond acute intoxication (8–10), which we hypothesize contributes to alcohol withdrawal signs and symptoms, alcohol craving, and relapse due to low acetate availability (6). Ketone bodies (β-hydroxybutyrate [BHB], acetoacetate and acetone) structurally resemble acetate and provide an alternative to glucose as an energy source in the brain (11). We recently found that a KD intervention reduced alcohol withdrawal severity and alcohol craving in individuals with an AUD undergoing inpatient detoxification, by elevating blood and brain ketone bodies (12). Preclinical models have also demonstrated that a KD reduced both alcohol self-administration (12, 13) and the signs of alcohol withdrawal (14–16).
Koban et al. (17) recently established a Neurobiological Craving Signature (NCS) based on brain reactivity to drug cues using functional Magnetic Resonance Imaging (fMRI). The NCS predicted drug and food craving, and distinguished drug users (alcohol, cigarettes, and cocaine) from non-users with 82% accuracy across imaging studies using machine learning techniques. The NCS is a whole-brain pattern of responses to cues, with prominent regions including ventromedial prefrontal and cingulate cortices, ventral striatum, temporal/parietal association areas, mediodorsal thalamus and cerebellum (17). Here, we performed a secondary analysis of fMRI alcohol cue reactivity data of the individuals with AUD who were randomized to receive a 3-week KD intervention versus a Standard American control diet (SA) intervention during an inpatient alcohol detoxification program, as previously reported in Wiers et al. (12). The previous study reported both clinical outcomes and dorsal anterior cingulate cortical activation outcomes for alcohol > neutral cues, and food > neutral cues (12), but not for alcohol > food contrasts. Here we computed the novel NCS expression levels for the alcohol > food cues, as per Koban et al. (17). We hypothesized lower NCS with KD compared to SA, which would associate with low alcohol wanting ratings and elevated blood BHB levels.
Methods
Participants and screening
This report involves a secondary analysis of the fMRI alcohol cue reactivity paradigm of a dataset previously reported (12). Data on patient demographics, blood ketone levels, and behavioral craving ratings have been reported previously and were shown again here for context. Details of the clinical trial methodology can be found in (12). Briefly, individuals with AUD were admitted to the National Institute on Alcohol Abuse and Alcoholism (NIAAA) inpatient unit while receiving treatment as usual, i.e., detoxification treatment for up to 4 weeks and treatment with benzodiazepines (Oxazepam or Diazepam) in the first week to treat acute withdrawal signs and symptoms based on Clinical Institute Withdrawal Assessment – Alcohol revised (CIWA-Ar) assessment (18). Within 2 days of admission, patients were randomized to receive either a KD (n = 19 randomized within 0.95 ± 0.23SD days) or a SA (n = 14 randomized within 0.78 ± 0.42SD days) for 3 weeks. There were no significant differences in demographic or clinical characteristics between groups, including no group differences in benzodiazepine use at admission (16 out of 19 patients who would be subsequently randomized to KD received benzodiazepines; and 10 out of 14 patients who would be randomized to SA received Oxazepam; 0 Diazepam, 4 none), or on the MRI day (4 out of 19 in the KD group and 2 out of 14 in the SA group received benzodiazepines on the MRI day; Table 1). Patients provided written informed consent to participate in the study, which was approved by the Institutional Review Board at the National Institutes of Health (Combined Neurosciences White Panel). Participants were MRI scanned between October 2017 and February 2020. The study was registered at ClinicalTrials.gov (NCT03255031).
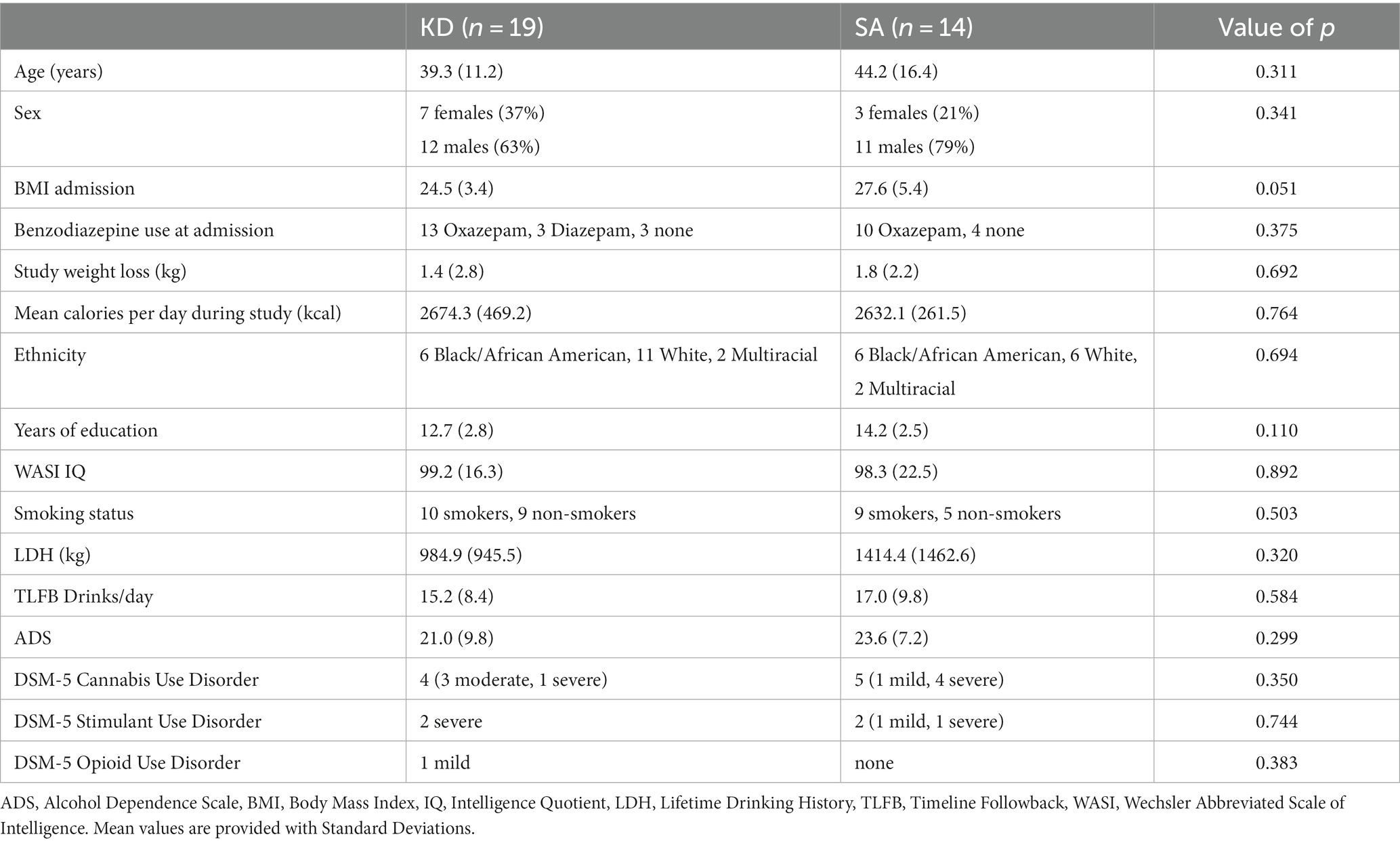
Table 1. Demographics and clinical characteristics of the ketogenic diet (KD) and standard American (SA) groups.
Patients were screened to exclude individuals who had contraindications for MRI, major medical problems including metabolic diseases (e.g., diabetes), chronic use of psychoactive medications, liver disease, head trauma, or milk or soy allergy; and current DSM-IV or DSM 5 diagnosis of a major psychiatric disorder (other than alcohol and nicotine use disorders, or substance use disorders that are mild/moderate) that required hospitalization, or that required daily medications for over 4 weeks in the past year, as assessed by the Structured Clinical Interview for the Diagnostic and Statistical Manual of Mental Disorders (DSM-IV or 5) (19, 20). Participants had at least 5 years’ history of heavy drinking (5+ drinks/day or 4+ drinks/day on at least 5 days per month for men or women, respectively). Alcohol had to be specified as the preferred drug of choice. All participants were free of psychoactive medications within 24 h of study procedures (except benzodiazepines as needed for detoxification) and had a negative urine drug screen [and, if needed, a negative saliva screen to rule out 11-Nor-9-carboxy-Δ9-tetrahydrocannabinol (THC-COOH) use, Draeger DrugTest5000; Draeger Safety Diagnostics, Lübeck, Germany] on days of testing. All patients demonstrated severe AUD (DSM criteria range 7–11).
Diet intervention
The KD and SA meals were equicaloric. The KD provided a classic 4: 1 ratio of grams of fat: grams of carbohydrate and protein (i.e., 80% fat, 15% protein, 5% carbohydrates). The SA corresponded to 50% calories from carbohydrates, 15% protein and 35% fat. For each meal at breakfast, lunch and dinner, the diets consisted of a shake (either KD or SA) that provided 75% of meal calories, and a ketogenic solid snack (e.g., scrambled egg, yogurt with nuts, salad, chicken salad, broth) that provided 25% of meal calories, which ensured double blinding (12). Patients were allowed to drink water ad libitum and were given the option to drink tea or coffee with or without stevia, and/or diet ginger-ale with their meals. Meals were provided from the Nutrition Department Metabolic Kitchen with all foods and beverages weighed on a gram scale. There was no effect of group on diet expectation, indicating that the blinding of the diet was successful (12).
Blood ketone measures
BHB ketone levels were assessed after informed consent was obtained and prior to diet randomization (baseline), and before breakfast on the morning of each MRI scan in week 1, 2, and 3, using a finger stick and Precision Xtra Blood Ketone Monitoring System strips (Abbott; Alameda, CA).
Questionnaires and ratings
Participants completed the Wechsler Abbreviated Scale of Intelligence (WASI-II) subtests Matrix Reasoning and Vocabulary as a proxy for general intelligence (21), the Timeline Followback (TLFB) to assess daily alcohol consumption in the 90 days prior to the study (22), the Lifetime Drinking History (LDH) to assess lifetime alcohol consumption (23), the Alcohol Dependence Scale (ADS) to assess severity of dependence (24).
On a weekly basis, participants rated their alcohol craving on the Desire for Alcohol Questionnaire (DAQ) (25).
MRI acquisition and preprocessing
Participants underwent three MRI scans, in the first, second and third week of diet initiation. Because patients were in acute withdrawal and due to scheduling, the MRI in week 1 was performed 4.0 ± 1.7SD days after inpatient admission and after diet initiation (no KD/SA group differences). MRIs were performed on a 3.0 T Magnetom Prisma scanner (Siemens Medical Solutions USA, Inc., Malvern, PA) equipped with a 32-channel head coil. T1-weighted 3D magnetization-prepared gradient-echo (MP-RAGE, TR/TE = 2200/4.25 ms; FA = 9°, 1 mm isotropic resolution) and T2-weighted multi-slice spin-echo (TR/TE = 8000/72 ms; 1.1 mm in-plane resolution; 94 slices, 1.7-mm slice thickness; matrix = 192) pulse sequences were used to acquire high-resolution anatomical brain images. One participant did not complete session 3 due to scheduling problems. For functional MRI, a 32-channel head coil and a standard echo planar imaging (EPI) sequence were used: sequential interleaved acquisition, repetition time 1.5 s, echo time 30 ms, flip angle α = 70°, 64 × 64 pixels in-plane resolution, 36 slices, slice thickness 4 mm, voxel dimensions 3 × 3 × 4 mm3, field of view 192 × 192 mm2. Stimuli were presented on a black background under dimmed room lighting using a liquid-crystal display screen (BOLDscreen 32, Cambridge Research Systems; United Kingdom).
fMRI cue reactivity
A total of 40 alcohol, 40 appetitive food, 40 neutral images were randomly presented in an event-related design using E-prime software (see Wiers et al. (26), for details). There were 3 runs showing 40 cues that included all 3 categories of cues (alcohol, food, neutral). Cues were presented at 750 ms. Pictures were selected from the International Affective Picture System (IAPS) and from in-house pictures libraries. To ensure that participants were paying attention they were instructed to press a button if they saw a bicycle. Total task duration was 13 min and 30 s. After completion of the MRI sessions, participants were asked to rate the cues for their subjective valence (“How negative/positive do you find the picture?,” −3 to 3 [very negative – very positive]) and wanting (“How much do you want to consume this right now?” from 0 to 6 [not at all – extremely]), on a 7-point Likert scale.
Preprocessing
Functional data analysis was performed with SPM8 (Welcome Department of Cognitive Neurology, London, United Kingdom). During preprocessing, scans were slice-time corrected, spatially realigned, co-registered to the T1 structural images and normalized to the Montreal Neurological Institute (MNI) template. Smoothing was performed with a 6 mm full-width at half-maximum Gaussian kernel.
Neurobiological craving score
For fMRI, 3 fMRI regressors—food, alcohol, and neutral events were created and then convolved with the hemodynamic response function with default temporal filtering of 128 s. Six realignment parameters were included as regressors of no interest. The following contrasts were calculated at the single-subject level: alcohol>baseline (average), food>baseline, for which dorsal anterior cingulate activation has been reported previously for KD versus SA (12). In a secondary analysis, we took the first-level whole brain maps for each individual, and performed dot-product multiplication between the NCS map from Koban et al. (17) and the contrast alcohol > food cues. If the resulting NCS ‘expression level’ is high, it indicates a brain response pattern to alcohol > food cues that is similar to the NCS map which predicted alcohol and drug craving.
Statistical analysis
For all measures, we performed mixed ANOVAs with group (KD/SA) as between-group factor and time as within-subjects factor using SPSS. Post-hoc t-tests were performed with significance threshold of α < 0.05.
Results
Serum beta-hydroxybutyrate levels
The 4:1 KD intervention significantly increased serum BHB levels compared to SA (group × time interaction: F3,93 = 67.0, p < 0.0001, η2 = 0.68). Blood ketones did not differ between groups at baseline before diet initiation (t31 = 1.4, p = 0.2), and became significantly elevated in the KD group the morning of the MRI day in week 1 (mean BHB in KD group = 1.6 ± 1.5 mM), week 2 (mean KD = 4.6 ± 1.0 mM), and week 3 (mean KD = 4.4 ± 1.5 mM; all p < 0.001; Figure 1), indicative of nutritional ketosis. The slight decrease in week 3 compared to week 2 for blood measures reflected two participants who were not compliant with the KD in week 3, one for 1 day and the other for 2 days.
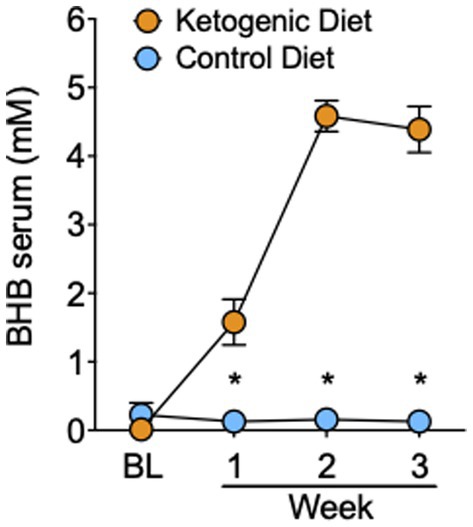
Figure 1. Serum levels of BHB in the ketogenic and standard American control diet group. *p < 0.001, ketogenic diet different from standard American control diet.
All participants on the SA demonstrated blood BHB levels of <0.2 mM, indicating compliance to the SA, and no presence of nutritional ketosis.
Self-reported alcohol craving
Self-reported DAQ alcohol craving showed a trend for a main effect of time (F2,58 = 2.5, p = 0.09, η2 = 0.08), which was largely due to reductions in DAQ scores in the KD group (F2,32 = 3.2, p = 0.056, η2 = 0.17), but not the SA group (F2,26 = 0.45, p = 0.64, η2 = 0.03). The effect of group or group × time for DAQ did not reach statistical significance.
Alcohol cue reactivity
Mean ratings of “wanting” alcohol cues relative to the neutral cues used in the fMRI cue reactivity task, decreased over time in the KD (F2,32 = 6.6, p = 0.004, η2 = 0.29) but not in the SA group (F2,24 = 0.5, p = 0.62, η2 = 0.04), and the group × time interaction effect was significant (F2,56 = 4.9, p = 0.048, η2 = 0.20; Figure 2A). There were no significant group effects for “wanting” ratings when exposed to food cues versus neutral cues (Figure 2A), and no effects for valence ratings of alcohol cues or food cues, relative to neutral cues.
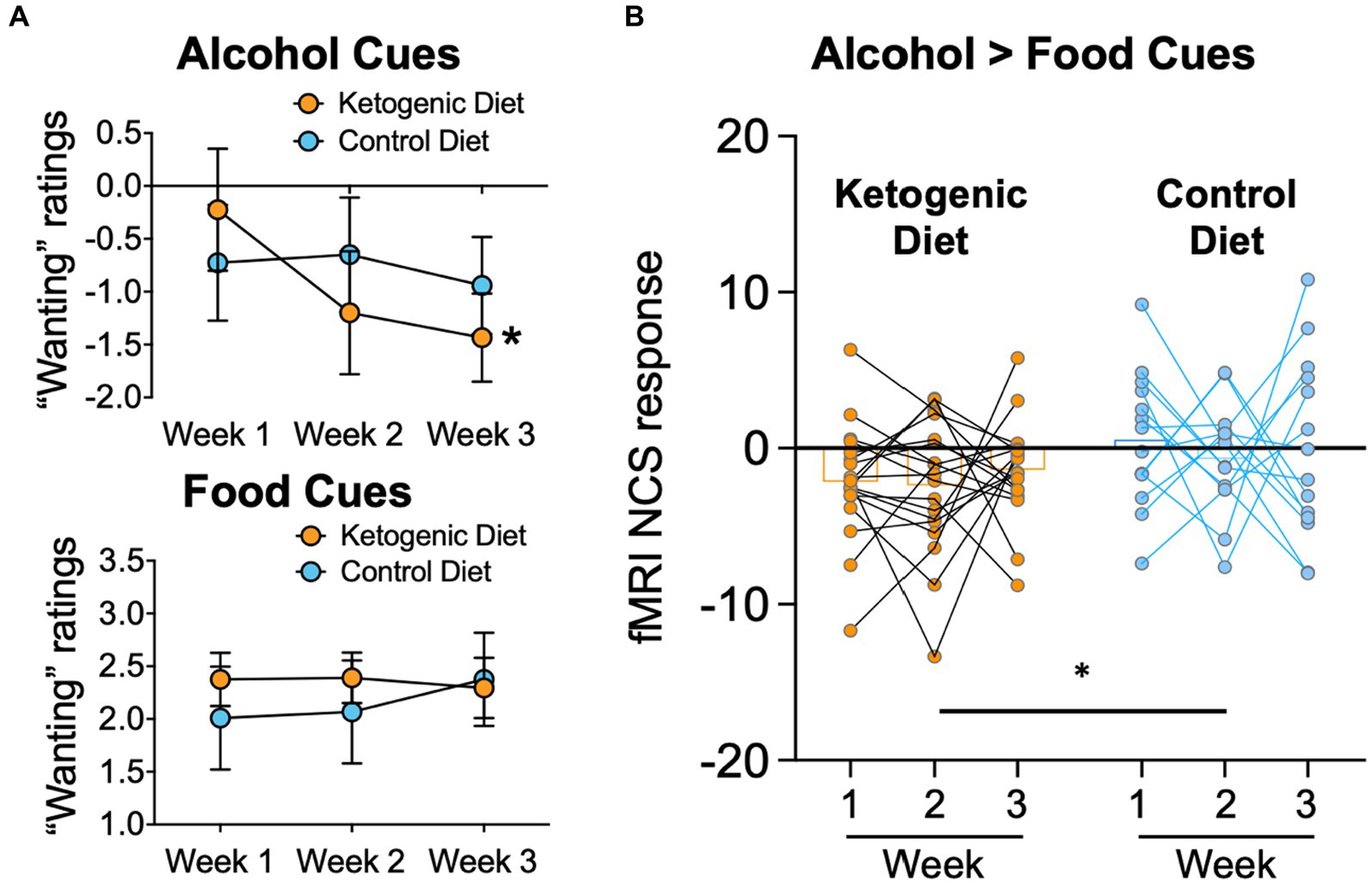
Figure 2. (A) Alcohol “wanting” ratings of alcohol cues were lower in ketogenic than standard American control diet after diet initiation. There were no group differences for “wanting” ratings caused by food-cues. (B)The fMRI NCS response was lower in the KD group than the SA Control diet group throughout the 3 weeks of dietary intervention.
Neurobiological craving score
There was a main effect of group on the alcohol > food NCS (F1,30 = 9.9, p = 0.004, η2 = 0.248), with lower overall NCS in the KD group than the SA group (Figure 2B). The effect of group × time or main effect of time for NCS did not reach statistical significance (F2,60 = 5.6, p = 0.77, η2 = 0.009). Average 3-week NCS scores correlated with average 3-week serum BHB levels in both diet groups pooled together (r = −0.365, p = 0.037), corroborating that higher ketone levels covaried with lower neurobiological craving for alcohol in AUD patients. There were no other significant associations between BHB levels and alcohol craving or wanting or between the alcohol NCS with alcohol craving or wanting.
Discussion
We applied a newly identified fMRI Neurobiological Craving Signature (NCS) for drug craving (17) on a previously reported fMRI alcohol cue reactivity dataset acquired from individuals with AUD who were randomized into a 3-week KD or SA control diet interventions during inpatient alcohol detoxification (12). The KD group demonstrated lower NCS responses compared to the SA throughout the 3-week intervention, which covaried with the average blood BHB levels on the days of the MRI scans. Moreover, the KD compared to SA demonstrated lower “wanting” ratings of the alcohol cues presented in the alcohol cue reactivity task, which did not correlate with NCS. These findings provide novel insight into the effects of KD on neural signatures of alcohol craving, and add to the previously reported effects of KD increasing dorsal cingulate cortical activation for both alcohol and food cues. Overall, these findings provide evidence for the potential therapeutic benefit of a KD in reducing alcohol wanting and neurobiological craving for alcohol.
These findings are in line with recent preclinical models of alcohol dependence that showed that a 1-week KD reduced alcohol self-administration (but not the motivation to consume alcohol) in mice (13) and that a history of a 9-week KD reduced alcohol self-administration in alcohol-dependent rats (12). Moreover a KD reduced the signs of alcohol withdrawal in rats (14–16). The reductions in alcohol NCS in the KD group may be the consequence of the reduced withdrawal symptoms and need for benzodiazepines in the KD group as reported previously (12), or may reflect a potentially beneficial effect of KD in disrupting conditioning to alcohol through its provision of ketone bodies that can serve as energy substrates. Although most of the research studying conditioned effects of alcohol associate it to its reinforcing effects mediated by its enhancement of GABAergic, opioid and dopaminergic systems, it is possible that the KD-induced bioenergetic switch from glucose to ketones is also relevant to its reinforcing effects, particularly given that energy-rich stimuli engender strong conditioned responses (8, 27, 28). In line with this, the NCS scores were negatively correlated with serum BHB levels of participants. We thus speculate that a KD would satisfy the altered energy requirements associated with chronic alcohol consumption through its metabolism of BHB. Alternatively, ketone supplementation may have reduced levels of neuroinflammation, or restored imbalances in glutamate and GABA neurotransmission (6), or in homeostatic hormones including ghrelin and leptin (29). Future studies are needed to further investigate mechanisms in which KD may decrease alcohol craving.
Our findings confirm our overall hypothesis that providing ketone bodies via a KD intervention during detoxification would attenuate the emergence of alcohol craving. We reasoned that the abrupt transition from the brain’s consumption of acetate (which occurs in AUD as an adaptation to repeated alcohol intake) to the use of glucose as energy source, and reemerges with detoxification, may contribute to the alcohol withdrawal syndrome (7). Notably, BHB, which is a major ketone body is thought to be a more efficient ‘fuel’ source than glucose (30). Therefore, inducing ketosis with a KD during detoxification would bolster or halt such an abrupt transition (11), thereby reducing alcohol withdrawal signs and symptoms, alcohol craving, and conditioned brain reactivity to alcohol cues. Disorders of brain glucose metabolism are associated with disrupted neuronal excitability, including epilepsy (31), and are ameliorated by KD. Thus, the reduction of withdrawal symptoms by KD may reflect an attenuation of neuronal excitability following alcohol discontinuation (32).
This study examined the effect of inpatient substance use disorder treatment on the NCS. Establishing a biomarker of craving has been a highly sought after target in neuroscience and psychiatry (33). Addiction is a chronic condition, and even in recovery, an individual will experience strong fluctuations in vulnerability to relapse (34). However, abstinence and drug use themselves are often not the best outcome measures to predict subsequent relapse; therefore, a neuroimaging biomarker that could better track recovery would be a highly useful tool for identifying when to intervene and prevent future drug use (35). The NCS, along with other recent machine learning-based neural signatures of craving (36) represent an exciting new approach for tracking risk of relapse during recovery. The current findings suggest that the NCS may indeed be sensitive to substance use disorder intervention and the recovery process, complementing recent studies using decision-making behavior to predict craving fluctuations in an opioid use disorder sample (37). Although Koban et al. (17) was published in a high impact journal, the Koban NCS has not been replicated or validated by other studies. Thus, justification for using the Koban NCS vs. other fMRI derived measures of craving using machine learning (e.g., Garrison (36)) is not provided. Future markers that combine imaging and behavioral markers may improve prediction further.
The main limitation of our clinical study was the relatively small sample size, which was constrained by the complexity of the study that required 3 weeks of hospitalization and compliance with the diet protocol. Another important limitation is that the first MRI was performed 4.0 ± 1.7SD days after inpatient admission (no group differences) at a time when the KD participants were already in mild ketosis (blood BHB = 1.6 ± 1.5 mM). It was not possible to perform MRI scans at an earlier time point before diet initiation, due to clinical constraints of participants being in acute alcohol withdrawal (that is, participants were too ill to be MRI scanned before diet initiation). Our design therefore did not have an absolute MRI baseline that allowed for comparison of NCS before and after diet initiations. This may be why our study found a main effect of KD on the alcohol NCS, but no group x diet interaction effect, as expected. Additionally, group differences in fMRI response, BHB levels, and withdrawal severity emerged at week 1, whereas craving differences did not emerge until week 3. It is possible that these brain changes are broadly related to, but not 100% explanatory of, self-reported tonic craving levels. This might be expected as the cue-reactivity fMRI task elicits cue-induced craving, which is not the same as tonic craving levels assessed via the DAQ self-report survey. Thus, there may be some additional variability in the DAQ that the NCS cannot account for. Future studies are needed that test the effects of ketone supplementation in participants with AUD that would include a baseline measure. Moreover, groups differed in BMI at baseline at a trending level. However, exploratory analyses demonstrated that BMI did not significantly associate with NSC (F1,29 = 0.69, p = 0.41) and the effects of diet on NCS remained while controlling for baseline BMI (F1,29 = 6.9, p = 0.014). Last, nicotine use was not assessed repeatedly in this study, and future studies should include repeated assessments for smoking in their design. Despite these limitations, we demonstrated the first clinical trial on a KD intervention in inpatients with AUD undergoing detoxification, with a complex and successful randomized and blinded design.
In sum, this study documents an effect of KD in reducing a neurobiological signature associated with alcohol craving in individuals with AUD. These findings are in line with recent preclinical data that show that a KD (13) and a history of KD (12) reduce acute self-administration in alcohol-dependent rodents, and the intake of an oral ketone BHB supplement reduces alcohol liking and wanting in human volunteers (38). It remains to be studied whether a KD or a history of KD decreases alcohol craving and alcohol consumption in a human population with AUD.
Data availability statement
The raw data supporting the conclusions of this article will be made available by the authors, without undue reservation.
Ethics statement
The studies involving humans were approved by Institutional Review Board at the National Institutes of Health (Combined Neurosciences White Panel). The studies were conducted in accordance with the local legislation and institutional requirements. The participants provided their written informed consent to participate in this study.
Author contributions
CW: Conceptualization, Data curation, Formal analysis, Funding acquisition, Investigation, Methodology, Project administration, Resources, Software, Supervision, Validation, Visualization, Writing – original draft, Writing – review & editing. PM: Conceptualization, Data curation, Formal analysis, Funding acquisition, Investigation, Methodology, Resources, Software, Supervision, Validation, Visualization, Writing – original draft, Writing – review & editing. G-JW: Conceptualization, Data curation, Formal analysis, Funding acquisition, Investigation, Methodology, Project administration, Resources, Software, Supervision, Validation, Visualization, Writing – original draft, Writing – review & editing. NV: Conceptualization, Data curation, Formal analysis, Funding acquisition, Investigation, Methodology, Project administration, Resources, Software, Supervision, Validation, Visualization, Writing – original draft, Writing – review & editing.
Funding
The author(s) declare financial support was received for the research, authorship, and/or publication of this article. This work was accomplished with support from the National Institute on Alcohol Abuse and Alcoholism, Intramural Research Program (Y1AA-3009 to NV). CW was supported by a NARSAD Young Investigator Grant (28778), and a K99/R00 grant AA026892.
Acknowledgments
We thank Nancy Diazgranados, Leandro Vendruscolo, Karen Torres, Minoo McFarland, Lori Talagala, Michele-Vera Yonga, Yvonne Horneffer, David George, Tonette Vinson, Ehsan Shokri-Kojori, Dardo Tomasi, Rui Zhang, Sukru Baris Demiral, Mackenzie Cervenka, Sara Turner, Shanna Yang, Todd King, and Richard Veech for their contributions and discussions. All data needed to evaluate the conclusions in the paper are present in the paper and/or the Supplementary materials.
Conflict of interest
The authors declare that the research was conducted in the absence of any commercial or financial relationships that could be construed as a potential conflict of interest.
Publisher’s note
All claims expressed in this article are solely those of the authors and do not necessarily represent those of their affiliated organizations, or those of the publisher, the editors and the reviewers. Any product that may be evaluated in this article, or claim that may be made by its manufacturer, is not guaranteed or endorsed by the publisher.
References
1. Rehm, J, and Imtiaz, S. A narrative review of alcohol consumption as a risk factor for global burden of disease. Subst Abuse Treat Prev Policy. (2016) 11:37. doi: 10.1186/s13011-016-0081-2
2. Mark, TL, Kassed, CA, Vandivort-Warren, R, Levit, KR, and Kranzler, HR. Alcohol and opioid dependence medications: prescription trends, overall and by physician specialty. Drug Alcohol Depend. (2009) 99:345–9. doi: 10.1016/j.drugalcdep.2008.07.018
3. Campbell, EJ, Lawrence, AJ, and Perry, CJ. New steps for treating alcohol use disorder. Psychopharmacology (Berl). (2018) 235:1759–73. doi: 10.1007/s00213-018-4887-7
4. Kranzler, HR, and Soyka, M. Diagnosis and pharmacotherapy of alcohol use disorder: a review. JAMA. (2018) 320:815–24. doi: 10.1001/jama.2018.11406
5. Li, X, Shi, Z, Byanyima, J, Morgan, PT, Van Der Veen, JW, Zhang, R, et al. Brain glutamate and sleep efficiency associations following a ketogenic diet intervention in individuals with alcohol use disorder. Drug Alcohol Depend Rep. (2022) 5:100092. doi: 10.1016/j.dadr.2022.100092
6. Mahajan, VR, Elvig, SK, Vendruscolo, LF, Koob, GF, Darcey, VL, King, MT, et al. Nutritional ketosis as a potential treatment for alcohol use disorder. Front Psych. (2021) 12:781668. doi: 10.3389/fpsyt.2021.781668
7. Volkow, ND, Kim, SW, Wang, GJ, Alexoff, D, Logan, J, Muench, L, et al. Acute alcohol intoxication decreases glucose metabolism but increases acetate uptake in the human brain. Neuroimage. (2013) 64:277–83. doi: 10.1016/j.neuroimage.2012.08.057
8. Volkow, ND, Wiers, CE, Shokri-Kojori, E, Tomasi, D, Wang, GJ, and Baler, R. Neurochemical and metabolic effects of acute and chronic alcohol in the human brain: studies with positron emission tomography. Neuropharmacology. (2017) 122:175–88. doi: 10.1016/j.neuropharm.2017.01.012
9. Jiang, L, Gulanski, BI, De Feyter, HM, Weinzimer, SA, Pittman, B, Guidone, E, et al. Increased brain uptake and oxidation of acetate in heavy drinkers. J Clin Invest. (2013) 123:1605–14. doi: 10.1172/JCI65153
10. Wang, J, Du, H, Jiang, L, Ma, X, De Graaf, RA, Behar, KL, et al. Oxidation of ethanol in the rat brain and effects associated with chronic ethanol exposure. Proc Natl Acad Sci U S A. (2013) 110:14444–9. doi: 10.1073/pnas.1306011110
11. Courchesne-Loyer, A, Croteau, E, Castellano, CA, St-Pierre, V, Hennebelle, M, and Cunnane, SC. Inverse relationship between brain glucose and ketone metabolism in adults during short-term moderate dietary ketosis: a dual tracer quantitative positron emission tomography study. J Cereb Blood Flow Metab. (2017) 37:2485–93. doi: 10.1177/0271678X16669366
12. Wiers, CE, Vendruscolo, LF, Van Der Veen, JW, Manza, P, Shokri-Kojori, E, Kroll, DS, et al. Ketogenic diet reduces alcohol withdrawal symptoms in humans and alcohol intake in rodents. Sci Adv. (2021) 7:1–13. doi: 10.1126/sciadv.abf6780
13. Blanco-Gandía, MDC, Ródenas-González, F, Pascual, M, Reguilón, MD, Guerri, C, Miñarro, J, et al. Ketogenic diet decreases alcohol intake in adult male mice. Nutrients. (2021) 13:1–16. doi: 10.3390/nu13072167
14. Bornebusch, AB, Mason, GF, Tonetto, S, Damsgaard, J, Gjedde, A, Fink-Jensen, A, et al. Effects of ketogenic diet and ketone monoester supplement on acute alcohol withdrawal symptoms in male mice. Psychopharmacology (Berl). (2021) 238:833–44. doi: 10.1007/s00213-020-05735-1
15. Dencker, D, Molander, A, Thomsen, M, Schlumberger, C, Wortwein, G, Weikop, P, et al. Ketogenic diet suppresses alcohol withdrawal syndrome in rats. Alcohol Clin Exp Res. (2018) 42:270–7. doi: 10.1111/acer.13560
16. Derr, RF, Draves, K, and Derr, M. Abatement by acetate of an ethanol withdrawal syndrome. Life Sci. (1981) 29:1787–90. doi: 10.1016/0024-3205(81)90189-2
17. Koban, L, Wager, TD, and Kober, H. A neuromarker for drug and food craving distinguishes drug users from non-users. Nat Neurosci. (2023) 26:316–25. doi: 10.1038/s41593-022-01228-w
18. Sullivan, JT, Sykora, K, Schneiderman, J, Naranjo, CA, and Sellers, EM. Assessment of alcohol withdrawal: the revised clinical institute withdrawal assessment for alcohol scale (CIWA-Ar). Br J Addict. (1989) 84:1353–7. doi: 10.1111/j.1360-0443.1989.tb00737.x
19. American Psychiatric Association. Diagnostic and statistical manual of mental disorders: DSM-IV-TR. 4th ed. Washington, DC: (2000).
20. American Psychiatric Association. Diagnostic and statistical manual of mental disorders. 5th ed. Washington DC: (2013).
21. Wechsler, D. Wechsler abbreviated scale of intelligence. San Antonio, TX: The Psychological Corporation (1999).
22. Sobell, LC, and Sobell, MB. Timeline Followback: User’s guide - a calendar method for assessing alcohol and drug use. Toronto: Addiction Research Foundation (1996).
23. Skinner, HA, and Sheu, WJ. Reliability of alcohol use indices. The lifetime drinking history and the MAST. J Stud Alcohol. (1982) 43:1157–70. doi: 10.15288/jsa.1982.43.1157
24. Skinner, HA, and Allen, BA. Alcohol dependence syndrome: measurement and validation. J Abnorm Psychol. (1982) 91:199–209. doi: 10.1037/0021-843X.91.3.199
25. Love, A, James, D, and Willner, P. A comparison of two alcohol craving questionnaires. Addiction. (1998) 93:1091–102. doi: 10.1046/j.1360-0443.1998.937109113.x
26. Wiers, CE, Zhao, J, Manza, P, Murani, K, Ramirez, V, Zehra, A, et al. Conscious and unconscious brain responses to food and cocaine cues. Brain Imaging Behav. (2021) 15:311–9. doi: 10.1007/s11682-020-00258-x
27. De Araujo, IE, Oliveira-Maia, AJ, Sotnikova, TD, Gainetdinov, RR, Caron, MG, Nicolelis, MA, et al. Food reward in the absence of taste receptor signaling. Neuron. (2008) 57:930–41. doi: 10.1016/j.neuron.2008.01.032
28. Rejeski, WJ, Blumenthal, TD, Miller, GD, Lobe, M, Davis, C, and Brown, L. State craving, food availability, and reactivity to preferred snack foods. Appetite. (2010) 54:77–83. doi: 10.1016/j.appet.2009.09.009
29. Farokhnia, M, Grodin, EN, Lee, MR, Oot, EN, Blackburn, AN, Stangl, BL, et al. Exogenous ghrelin administration increases alcohol self-administration and modulates brain functional activity in heavy-drinking alcohol-dependent individuals. Mol Psychiatry. (2018) 23:2029–38. doi: 10.1038/mp.2017.226
30. Li, X, Young, AJ, Pereira-Rufino, LS, Shi, Z, Byanyima, J, Vesslee, S, et al. Pharmacokinetic effects of a single-dose nutritional ketone ester supplement on brain ketone and glucose metabolism in alcohol use disorder – a pilot study. medRxiv. (2023) 2023:23296090. doi: 10.1101/2023.09.25.23296090
31. Pascual, JM, Campistol, J, and Gil-Nagel, A. Epilepsy in inherited metabolic disorders. Neurologist. (2008) 14:S2–s14. doi: 10.1097/01.nrl.0000340787.30542.41
32. Long, D, Long, B, and Koyfman, A. The emergency medicine management of severe alcohol withdrawal. Am J Emerg Med. (2017) 35:1005–11. doi: 10.1016/j.ajem.2017.02.002
33. Kronberg, G, and Goldstein, RZ. An fMRI marker of drug and food craving. Nat Neurosci. (2023) 26:178–80. doi: 10.1038/s41593-022-01246-8
34. Yip, SW, and Konova, AB. Densely sampled neuroimaging for maximizing clinical insight in psychiatric and addiction disorders. Neuropsychopharmacology. (2022) 47:395–6. doi: 10.1038/s41386-021-01124-0
35. Goldstein, RZ. Neuropsychoimaging measures as alternatives to drug use outcomes in clinical trials for addiction. JAMA Psychiatry. (2022) 79:843–4. doi: 10.1001/jamapsychiatry.2022.1970
36. Garrison, KA, Sinha, R, Potenza, MN, Gao, S, Liang, Q, Lacadie, C, et al. Transdiagnostic connectome-based prediction of craving. Am J Psychiatry. (2023) 180:445–53. doi: 10.1176/appi.ajp.21121207
37. Biernacki, K, Lopez-Guzman, S, Messinger, JC, Banavar, NV, Rotrosen, J, Glimcher, PW, et al. A neuroeconomic signature of opioid craving: how fluctuations in craving bias drug-related and nondrug-related value. Neuropsychopharmacology. (2022) 47:1440–8. doi: 10.1038/s41386-021-01248-3
Keywords: brain energetics, ketone, ketosis, neuroimaging, withdrawal
Citation: Wiers CE, Manza P, Wang G-J and Volkow ND (2024) Ketogenic diet reduces a neurobiological craving signature in inpatients with alcohol use disorder. Front. Nutr. 11:1254341. doi: 10.3389/fnut.2024.1254341
Edited by:
Dieter J. Meyerhoff, University of California, United StatesReviewed by:
Octavian Vasiliu, Dr. Carol Davila University Emergency Military Central Hospital, RomaniaElisabet Jerlhag, University of Gothenburg, Sweden
Graeme F. Mason, Yale University, United States
Copyright © 2024 Wiers, Manza, Wang and Volkow. This is an open-access article distributed under the terms of the Creative Commons Attribution License (CC BY). The use, distribution or reproduction in other forums is permitted, provided the original author(s) and the copyright owner(s) are credited and that the original publication in this journal is cited, in accordance with accepted academic practice. No use, distribution or reproduction is permitted which does not comply with these terms.
*Correspondence: Corinde E. Wiers, corinde.wiers@pennmedicine.upenn.edu; Peter Manza, peter.manza@nih.gov; Nora D. Volkow, nvolkow@nida.nih.gov
†These authors have contributed equally to this work