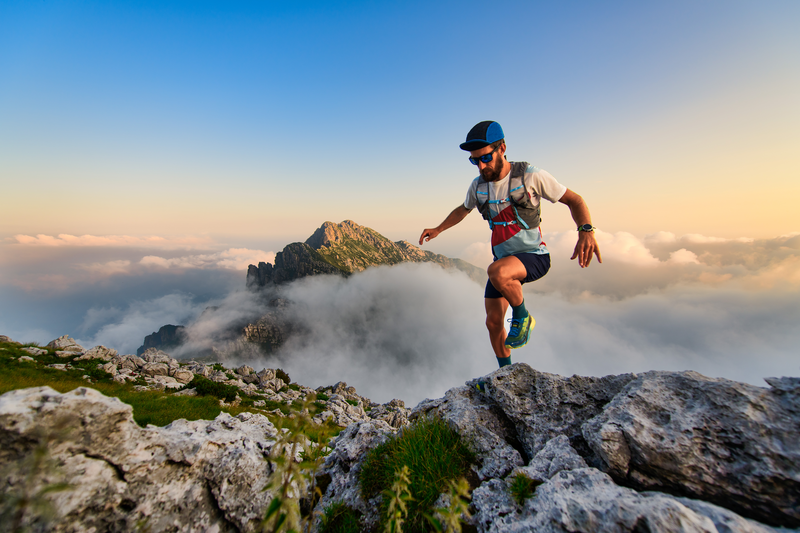
94% of researchers rate our articles as excellent or good
Learn more about the work of our research integrity team to safeguard the quality of each article we publish.
Find out more
ORIGINAL RESEARCH article
Front. Nutr. , 02 February 2023
Sec. Nutrition and Food Science Technology
Volume 10 - 2023 | https://doi.org/10.3389/fnut.2023.1080487
This article is part of the Research Topic Dietary Phytochemicals: Identification, Bioactivities, and Delivery Strategy View all 28 articles
Introduction: Oxidative stress is closely related to the development of many diseases. Essential oils (EOs) show potent antioxidant activity from natural sources. Kaempferia galanga L. is an important medicine rich in high-value essential oil (KGEO). However, the antioxidant activity of KGEO remains to be fully studied.
Methods: Chemical composition of KGEO was analyzed using gas chromatography-mass spectrometry (GC-MS). The antioxidant activity was determined using the DPPH, ABTS, hydroxyl radical scavenging assays and reducing power assay in vitro. A zebrafish model was used to evaluate the protective effect of KGEO against H2O2-induced oxidative stress damage in vivo.
Results: The major components of KGEO were found to be trans ethyl p-methoxycinnamate (32.01%), n-pentadecane (29.14%) and trans ethyl cinnamate (19.50%). In vitro pharmacological results showed that KGEO had good free radical scavenging capacity in DPPH, ABTS, and hydroxyl radical scavenging assays (IC50 values: 19.77 ± 1.28, 1.41 ± 0.01, and 3.09 ± 0.34 mg/mL, respectively) and weak reducing capacity in the reducing power assay (EC50 value: 389.38 ± 4.07 mg/mL). In vivo zebrafish experiments results indicated that the survival rate and heart rate increased, and ROS generation, cell death, and lipid peroxidation were attenuated after KGEO treatment. In addition, a decrease in malondialdehyde (MDA) levels and increases in superoxide dismutase (SOD), catalase (CAT) and glutathione peroxidase (GSH-Px) activities were observed in the KGEO-treated groups.
Discussion: This study validated the in vitro and in vivo antioxidant activities of KGEO, which provides a theoretical basis for a profound study of KGEO and its application in the pharmaceutical, food and cosmetic industries.
Graphical Abstract. The chemical composition of KGEO was determined by using GC-MS. The antioxidant activities of KGEO were evaluated through various experiments in vitro and in vivo.
Oxidative stress is associated with the development of many diseases, such as cancer, diabetes, aging, inflammation, and Parkinson’s disease (1). Oxidative stress is induced due to an imbalance between reactive oxygen species (ROS) and antioxidants in the human body (2–4). Normally, the production and clearance of ROS such as hydrogen peroxide (H2O2), hydroxyl radicals (OH⋅), and superoxide anion radicals (O2⋅–) are balanced in the processes of normal cellular metabolism and the antioxidant system (2). However, abnormal ROS production caused by excessive environmental stimulation results in oxidative stress and related diseases (3), and the accumulation of excessive ROS causes irreversible oxidative damage (4). Synthetic antioxidants have been used to inhibit the oxidative damage caused by the generation of excessive ROS. However, they are suspected to have side effects, such as digestive problems. Thus, new natural antioxidants without toxicity have become the focus of numerous studies.
Essential oils (EOs) are an important class of compounds with diverse pharmacological activities, including antioxidant, antimicrobial, and anti-inflammatory activities. They are extracted from plant materials and are mainly composed of monoterpenes and sesquiterpenes (5–6). Kaempferia galanga is an important herbal medicine and is widely used in India, China, Myanmar, Bangladesh, and Thailand (7). It has been used in folk medicine to treat indigestion, rheumatism, toothaches, asthma, piles, and wounds (8). Pharmacological studies have shown that K. galanga possesses antimicrobial (9), antioxidant (10), anti-inflammatory and analgesic (11), vasorelaxant (12), and antitumour (13) activities. As the main component of K. galanga, K. galanga essential oil (KGEO) also plays an important medicinal role and is used to treat indigestion, rheumatism, asthma, coughs, and skin issues (8).
Excessive free radicals induce oxidative stress, which is harmful to the human body (14). Novel antioxidants from plants and their underlying mechanisms have attracted much attention because of the side effects of synthetic drugs (15). As a free radical scavenger, EO can prevent various types of damage to the body caused by free radicals.
However, the antioxidant activity of KGEO remains to be fully studied. The aim of the study was to investigate the chemical composition characteristics of KGEO by using gas chromatography-mass spectrometry (GC-MS). Furthermore, the antioxidant activity of KGEO were systematically evaluated in vitro and in vivo. The findings of this study indicated its potential to develop as novel antioxidant in the pharmaceutical, food, and cosmetic industries.
Kaempferia galanga rhizomes were purchased from Qingping Chinese medicinal herbs market (Guangdong Province, China), and were identified by deputy chief pharmacist Junbiao Wu (The Second Clinical Medical College, Guangzhou University of Chinese Medicine, Guangzhou, China). Prior to use, the rhizomes were ground into powder.
The reference standard of n-alkanes (C8−C20) was received from ANPEL Laboratory Technologies (Shanghai) Inc. (Shanghai, China). 2,2-diphenyl-1-picrylhydrazyl (DPPH, 98%), 2,2′-amino-di(2-ethyl-benzothiazoline sulfonic acid-6) ammonium salt (ABTS, 98%), ascorbic acid (≥ 99%), 2′,7′-dichlorodihydrofluorescein diacetate (DCF-DA), and acridine orange were acquired from Sigma-Aldrich (Shanghai, China). Malondialdehyde (MDA), superoxide dismutase (SOD), catalase (CAT), and glutathione peroxidase (GSH-Px) were obtained from Nanjing Jiancheng Biotechnology Co., Ltd (Nanjing, China). Dimethyl sulfoxide (DMSO) was obtained from MP Biomedicals (CA, USA). Diphenyl-1-pyrenylphosphine (DPPP, catalog no. DJ799) was obtained from Macklin (Shanghai, China). Other chemicals used in this study were of analytical grade.
A Clevenger-type apparatus with ethanol was used to extract the EO of the rhizome powder (500 g) by distillation for 6 h, and then water was removed with anhydrous sodium sulfate. KGEO was stored in a well-closed container at 4°C.
The volatile compounds were identified by GC-MS (Agilent 7890A-5975C, Agilent Technologies, USA) using an HP−5MS capillary column (30 m × 0.25 mm, film thickness 0.25 μm). Helium was the carrier gas at 1.0 ml/min. One microlitre was injected with a split ratio of 1/10, and the injector temperature was 250°C. The oven temperature was increased from 60 to 122°C at 3°C/min, subsequently raised to 126, 148, 152, and 250°C at 0.5, 2, 0.5, and 5°C/min, respectively, and held at 250°C for 2 min. The mass spectrometry (MS) parameters were as follows: ion source temperature, 230°C; electron impact, 70 eV; scan mass range, 50−550 m/z (1.0 scan/s). The retention indices (RIs) of all components were calculated by using n-alkanes (C8−C20) under the same conditions as those of the normal sample to identify the constituents of the EO by comparing the RI of the n-alkanes and the spectra with the MS Library (NIST 14) under identical experimental conditions.
The DPPH assay was used to determine the DPPH radical scavenging activity of KGEO according to the described method (16–17) after making the changes. One milligram of DPPH was dissolved in ethanol (5 ml) to prepare a fresh DPPH working solution. Fifty microlitres of different concentrations of KGEO sample solutions (1, 5, 10, 20, 30, and 40 mg/ml) were mixed well with 50 μL of 0.5 mM DPPH solution to react in the dark at room temperature for 30 min as a sample group. For the blank group, the sample was replaced with an equal volume (50 μL) of ethanol, and ascorbic acid was used as a positive control. A microplate reader (M1000pro, Tecan, Männedorf, Switzerland) was used to determine the absorbance at 517 nm. Three replicates of the experiment were carried out. The IC50 (mg/ml) value was determined by the following Eq. 1:
where A0 represents the absorbance values of the control and A1 represents the absorbance values of KGEO. The scavenging rate and IC50 of ascorbic acid were calculated under the same conditions.
The ABTS assay was carried out based on a previously reported method (16) with some modifications. To prepare the ABTS radical stock solution, ABTS (7.4 mM) solution and K2S2O8 (2.45 mM) solution were mixed with an equal volume and reacted for 12−16 h in the dark. Before use, the ABTS radical stock solution was diluted withphosphate buffered saline (PBS) until the absorbance was 0.70 ± 0.02 at 734 nm. Fifty microlitres of different concentrations of sample solutions (0.4, 0.6, 0.8, 1.0, 1.2, 1.4, 1.6, 1.8, and 2.0 mg/ml) were mixed with 150 μL of ABTS radical working solution to react for 6 min in the dark at room temperature, which was used as the experimental group. For the blank group, the sample solutions were replaced by the same volume of ethanol (50 μL). The absorbance of each group was measured at 734 nm using a microplate reader. The results are presented as the IC50 value (mg/ml), and the scavenging rate was determined according to Eq. 1. The inhibition percentage and IC50 of ascorbic acid were calculated under the same conditions.
The ability of KGEO to inhibit hydroxyl radicals was evaluated according to a reported method (18) with some modifications. KGEO samples (1 ml) with a range of concentrations (1, 5, 10, 20, and 30 mg/ml) were combined with 1 ml of 1 mM salicylic acid ethanol solution, 1 ml of FeSO4 (1 mM), and 1 ml of 0.01% H2O2 (v/v), which were mixed in order. The mixture was maintained at 37°C for 30 min, and then the absorbance (Ai) was measured at 510 nm. Ascorbic acid was used for the positive control group. An equal volume of distilled water replaced the 0.01% H2O2 to prepare Aj. A0 was prepared with an ethanol solution instead of KGEO samples. The ability of KGEO to inhibit hydroxyl radicals was determined using Eq. 2:
The total reduction ability of KGEO was evaluated by using the method reported in the literature (19–20) with some modifications. KGEO samples (0.5 ml) of a range of concentrations (0.2, 0.3, 0.4, 0.5, 0.6, and 0.7 g/ml) were mixed with 0.2 M phosphate buffer (pH 6.6) solution (2.5 ml) and 1% potassium ferricyanide (2.5 ml) in order. Then, the mixture was maintained at 50°C for 20 min. Afterward, 10% trichloroacetic acid (2.5 ml) was added, followed by incubation for 10 min at room temperature. After incubation, 0.10% ferric chloride (0.5 ml) and distilled water (2.5 ml) were added to the mixture (2.5 ml), and then the absorbance was measured at 700 nm. The EC50 value was the value at which the absorbance was 0.5. Ascorbic acid was used as the positive control.
Wild-type AB zebrafish were provided by Guangdong Laboratory Animals Monitoring Institute. The zebrafish were kept at 28.5 ± 0.5°C with a 14:10 h light/dark cycle and fed flake food three times daily. Embryos were obtained from natural spawning, collected after 45 min of light, and then incubated in E3 medium (21) (CaCl2 0.33 mM, NaCl 5 mM, KCl 0.18 mM, MgSO4 0.34 mM).
We evaluated the effect of KGEO against H2O2-induced embryotoxicity. KGEO was dissolved in DMSO. The 48 h post-fertilization (hpf) embryos were treated with different concentrations of KGEO (0.4, 0.8, and 1.6 μg/ml) for 1 h. After 1 h, H2O2 (2 mM) was added as a stimulant. DMSO (0.32%) was used as the solvent control. The embryos were rinsed in fresh medium, and the survival rate was examined after 24 h.
The heartbeat rates of zebrafish embryos were measured after 24 h of H2O2 (2 mM) stimulation at 72 hpf. The average heart rate of zebrafish embryos was measured by manual counting with a 10 s period for 15 live zebrafish embryos under a microscope (Nikon Ci-E, Japan). The heartbeat rates were expressed as a percentage.
Intracellular ROS, cell death, and lipid peroxidation were measured by DCF-DA, acridine orange, and DPPP assays to assess the protective effect of KGEO against H2O2-induced oxidative stress (22). The zebrafish embryos were treated with phenylthiourea (2 mg/ml) to inhibit pigment pattern formation in zebrafish for better observation of fluorescence images starting at 5 hpf. The 48 hpf zebrafish embryos were treated with or without KGEO (0.4, 0.8, and 1.6 μg/ml) for 1 h. Then, H2O2 (2 mM) was added, followed by incubation for 24 h. At 72 hpf, the embryos were washed with E3 medium and transferred into 24-well plates. DCF-DA is an oxidation-sensitive fluorescent probe dye used to detect ROS levels. The zebrafish embryos were incubated with DCF-DA solution (2.5 μM) for 50 min at 28°C in the dark. Apoptotic cells were detected in embryos by using acridine orange (7 μg/ml), a nucleic acid-selective fluorescent dye, and the embryos were incubated for 30 min in the dark at 28°C. When DPPP is oxidized, it becomes fluorescent. DPPP is a fluorescent probe used to measure lipid peroxidation for the detection of membrane damage. The embryos were treated with DPPP solution (20 μg/ml) and incubated for 40 min in the dark at 28.5°C. After incubation, the embryos were washed with E3 medium at least three times and anesthetized with MS222 (150 μg/ml) before visualization. Fluorescence images of embryos were captured using a fluorescence microscope (Nikon Ci-E, Japan), and the fluorescence intensity of individual embryos was quantified using ImageJ software (Fiji version) (National Institutes of Health, Bethesda, USA).
The 48 hpf zebrafish embryos were treated with or without KGEO (0.4, 0.8, and 1.6 μg/ml) for 1 h and then incubated with H2O2 (2 mM) for 24 h. At 72 hpf, the embryos from each group were collected and homogenized with buffer (pH 7.2) containing EDTA-2Na (0.0001 M), Tris−HCl (0.01 M), and NaCl (0.65%) using a tissue homogenizer (1,700 r/min, 30 s × 3). After centrifugation (3,000 r/min, 15 min), the supernatants were collected immediately to determine the activity of SOD, CAT and GSH-Px and MDA levels according to the manufacturer’s directions (A003−1, A001−1, A007−1, and A005, Jiancheng, Nanjing, China).
Data were analyzed using SPSS 26.0 (SPSS Software, Chicago, IL, USA). One-way ANOVA followed by least significant difference (LSD) or Dunnett’s multiple comparisons test was employed to analyze the statistical significance between the control group and other treatment groups. The non-parametric data were analyzed by a Kruskal−Wallis test. Data are presented as the means ± SDs of three independent experiments. P < 0.05 was considered statistically significant (###P < 0.001 compared with the H2O2 group; *P < 0.05, **P < 0.01, and ***P < 0.001 compared with the control group).
The KGEO chemical components that were determined are shown in Table 1, which are arranged in the order of elution from the HP−5MS column. Twenty-eight constituents were found, which comprised 95.18% of the KGEO. Phenylpropanoids (53.60%) were the dominant class in the EO, followed by hydrocarbons (33.18%), monoterpenoids (4.07%), and sesquiterpenoids (2.20%). The most abundant chemical compounds were determined to be trans ethyl p-methoxycinnamate (32.01%), n-pentadecane (29.14%), and trans ethyl cinnamate (19.50%), which together represented 80.65% of the EO.
Because of the complex reactive facets of EO, at least two antioxidant systems should be employed to ensure the reliability of its antioxidant activity. Therefore, four complementary spectrophotometric methods, DPPH, ABTS, hydroxyl radical scavenging and reducing power assays, were used to carry out the following experiments. The results are presented in Table 2.
DPPH is often used in antioxidant experiments as a stable radical (23). The ability of KGEO to scavenge DPPH radicals was studied. In the oxidation reaction, KGEO showed a potential DPPH radical scavenging effect with an IC50 value of 19.77 ± 1.28 mg/ml (Table 2), with an increase in DPPH radical scavenging with increasing KGEO concentration. The scavenging effect of KGEO at a concentration of 10 mg/ml was 52.70%, and the activity appeared to be dose-dependent (24). This may be related to the plant source and processing procedure based on the content of its main components. DPPH radicals can be scavenged in the form of reduced DPPH-H, when a hydrogen radical combines with a DPPH radical (25). An EO with DPPH radical scavenging activity is beneficial for inhibiting damage to biomolecules (protein, sugars, PUFA, DNA) caused by reactive radical species in susceptible food and biological systems (24).
The ABTS radical scavenging assay is also usually employed to determine the in vitro antioxidant activity of different substrates. The scavenging activity of KGEO at different concentrations for ABTS radicals was determined. The IC50 of the EO was determined to be 1.41 ± 0.01 mg/ml (Table 2). The EO exhibited higher activity toward the ABTS radical compared to the DPPH radical. After proton dissociation, the ABTS radical (ABTS+) accepts a single electron and combines with hydrogen (H+) to form stable ABTS, which plays a significant role in free radical scavenging. The main mechanisms in the process of free radical scavenging are proton priority loss and single electron transfer (26). The DPPH radical scavenging assay can only be used in hydrophobic antioxidant systems, while the ABTS radical scavenging assay can be used in hydrophobic and lipophilic systems, which are more common (27).
Hydroxyl radicals are powerful oxidants that can react with many redox-sensitive elements and organics non-selectively in the environment and damage various biomolecules (28). As a main ROS, hydroxyl radicals can lead to lipid peroxidation or other oxidative damage (29). Different concentrations of KGEO were evaluated. KGEO had hydroxyl radical scavenging activity, with an IC50 of 3.09 ± 0.34 mg/ml, as shown in Table 2. KGEO exhibited higher activity toward the DPPH radical and lower activity toward the ABTS radical. Similar to DPPH and ABTS radicals, hydroxyl radical species are neutralized by a hydrogen atom.
The ferricyanide reduction method was used to determine the reducing power. The electron-donating activity is a significant mechanism of antioxidant activity, which can be indicated by Fe3+ reduction (19). The reducing power was assessed on KGEO. As presented in Table 2, the EC50 value for Fe3+ reduction of KGEO was 389.38 ± 4.07 mg/ml. This result suggested that KGEO has weak potency to donate electrons to reactive free radicals. During the ferric reducing assay, reducing agents reduced the Fe3+−ferricyanide complex to the ferrous form. Thus, Fe2+ can be assessed by the density of blue color in the reaction medium at 700 nm (30).
The protective effect of KGEO on oxidative stress in vivo induced by H2O2 was established using a zebrafish model. The survival rate of zebrafish embryos treated with different concentrations of KGEO is shown in Figure 1A. After exposure to H2O2, the survival rate dropped to 66.67% compared to 100.00% of the control group. However, the survival rate increased after preadministration of 0.4, 0.8, and 1.6 μg/ml KGEO, and the rate rose to 73.33, 86.67, and 93.33%, respectively. DMSO (0.32%) as a solvent control had no effect on the survival rate. The heartbeat rate of embryos treated with H2O2 (2 mM) was decreased to 80.56%, while pretreatment with different concentrations of KGEO (0.4, 0.8, and 1.6 μg/ml) recovered the heart rate to 91.16, 93.94, and 98.48%, respectively (Figure 1B). These results suggested that KGEO alleviated oxidative damage caused by H2O2.
Figure 1. Survival rate and heartbeat rate of H2O2-induced zebrafish treated with Kaempferia galanga essential oil (KGEO). The survival rate (A) and the heartbeat (B) of zebrafish. The results are expressed as mean ± SD (n = 15). ##P < 0.01, ###P < 0.001 as compared to the control group. *P < 0.05, **P < 0.01, ***P < 0.001 as compared to the H2O2-treated group.
The fluorescence images of intracellular ROS generation are shown in Figure 2A. After treatment with H2O2, the DCF fluorescence intensity was enhanced to 170.63%, with brighter fluorescence compared to the control group, while the fluorescence intensity was reduced up to 103.31% by cotreatment with KGEO.
Figure 2. The effects of Kaempferia galanga essential oil (KGEO) on H2O2-induced ROS Production, cell death, and lipid peroxidation in zebrafish. The protective effect of KGEO against H2O2-induced ROS generation (A), Cell death (B), and lipid peroxidation (C). The relative fluorescence intensities of zebrafish were analyzed using ImageJ software. The data were expressed as the mean ± SD (n = 15). ###P < 0.001 as compared to the control group. *P < 0.05, ***P < 0.001 as compared to the H2O2-treated group.
Acridine orange preferentially stains necrotic and late apoptotic cells, as it is able to permeate cells with disrupted plasma membranes. Fluorescence images showing cell death in zebrafish embryos as assessed by acridine orange staining are shown in Figure 2B. In the H2O2-treated group, the fluorescence intensity was significantly enhanced to 157.01% compared with the control group. After KGEO was administered, the fluorescence expression was significantly decreased, and the fluorescence value decreased to 105.04% with increasing KGEO concentration.
The fluorescence images for DPPP are shown in Figure 2C, and the fluorescence intensity indicated the degree of lipid peroxidation. H2O2 significantly induced lipid peroxidation, with the fluorescence intensity increasing to 170.55%, while lipid peroxidation was reduced to 108.00% after KGEO treatment.
The fluorescence intensities of images of ROS generation, cell death, and lipid peroxidation were measured using ImageJ software. The results suggested that KGEO showed protective activity in zebrafish embryos damaged by H2O2-induced oxidative damage via a decrease in ROS and lipid peroxidation and by alleviating cell death.
The antioxidant enzyme activities (SOD, CAT, and GSH-Px) and MDA levels were evaluated in H2O2-induced zebrafish embryos. The results are shown in Figure 3. In the H2O2-treated group compared to the control group, the activities of CAT, GSH-Px, and SOD in zebrafish embryos were decreased by 3.62−, 2.26−, and 1.56−fold, respectively, and MDA levels were increased by 2.27−fold. However, after treatment with different concentrations of KGEO (0.4, 0.8, and 1.6 μg/ml), the oxidative damage was alleviated in a dose-dependent manner. When treated with KGEO (1.6 μg/ml), the activities of CAT, GSH-Px, and SOD were increased by 3.78−, 2.5−, and 1.80−fold, respectively, and the MDA level showed a decrease of 63.00% compared to the H2O2-treated group. The results indicated that H2O2 caused oxidative damage and lipid peroxidation, which were due to the decrease in antioxidant enzyme activities and the increase in the formation of MDA, while KGEO alleviated this damage.
Figure 3. The effects of Kaempferia galanga essential oil (KGEO) on levels of malondialdehyde (MDA) and antioxidant enzymes activity in H2O2-induced zebrafish. Superoxide dismutase (SOD) activity (A), catalase (CAT) activity (B), GSH-Px activity (C), and MDA level (D) were determined after incubation. The data are expressed as mean ± SD (n = 15). ###P < 0.001 as compared to the control group. **P < 0.01, ***P < 0.001 as compared to the H2O2-treated group.
According to the literature, for K. galanga, the study of volatile oils has been the focus, especially in China, India, and Malaysia. The results of this study are consistent with those of previous studies, showing that trans ethyl p-methoxycinnamate, n-pentadecane, and trans ethyl cinnamate were the main constituents in the EO (8). However, after oils were obtained by hydrodistillation (MHD) and traditional hydrodistillation (HD), the main constituents of both oils were similar, but their n-pentadecane (32.80 and 21.60%), trans ethyl cinnamate (17.10 and 16.10%) and cyperene (3.40 and 2.00%) contents differed (31). The volatile oil extracted with n-hexane by steam distillation was identified as having trans ethyl p-methoxycinnamate (38.60%), trans ethyl cinnamate (23.20%), 1,8-cineole (11.50%), trans-cinnamaldehyde (5.30%), and borneol (5.20%) as the major components, which represented a high content of 1,8−cineole (32). In another study, 39 components were reported from KGEO; among them, trans ethyl p-methoxycinnamate, trans ethyl cinnamate and trans cinnamaldehyde were the main compounds, with contents of 30.60, 26.80, and 11.50%, respectively, which represented a high content of trans cinnamaldehyde (33). 1,8-cineole and trans cinnamaldehyde were not detected in this oil, which may be related to geography, climate conditions and postharvest processing, and the applied extraction methods also influenced the chemical profile. In addition, the relative percentages of major compounds were also affected by these factors.
Generally, the results of the in vitro antioxidant tests described above indicated that KGEO’s scavenging ability for DPPH, ABTS, hydroxyl radicals and reducing power exhibited a concentration-dependent manner comparable to the reference standard, ascorbic acid. Antioxidants can prevent or reduce damaging effects from free radicals in the human body by reacting with free radicals and neutralizing them (34). Moreover, the antioxidant activity of KGEO may be related to different mechanisms because of the chemical complexity of the oil.
Herbs and spices have a long history of being used to improve sensory and flavor characteristics. Many studies have explored the antioxidant activity of EOs and many herbs for addition to various foods. The vapor phase of EOs has bioactivity, which makes them useful for protecting commodities (35).
The zebrafish is a popular in vivo model that is widely used for modern bioactivity research and is characterized by easy handling, transparent embryos, and a short generation time. In previous studies, zebrafish stimulated with H2O2 have been used successfully to estimate antioxidant activity (36–37). As a result, H2O2-stimulated zebrafish were selected to evaluate the in vivo antioxidant effect of KGEO as an in vivo model. In vivo zebrafish studies indicated that KGEO alleviated oxidative stress damage and reversed the ROS generation, cell death and lipid peroxidation induced by H2O2.
The uncontrolled generation of free radicals and their damage to antioxidant defensive mechanisms cause superfluous ROS and induce oxidative damage (38). SOD, CAT, and GSH-Px are regarded as important defense systems against oxidative stress. O2– is converted to H2O2 by SOD and then is detoxified to H2O and O2 either by CAT or GSH-Px. SOD also inhibits lipid peroxidation by terminating the chain reaction. MDA is an indicator of lipid peroxidation, which is caused by redundant ROS (39). This study showed that KGEO could alleviate this damage caused by H2O2 by increasing the activities of antioxidant enzymes and downregulating the formation of MDA in zebrafish.
The chemical study of KGEO showed that it contains monoterpenoids, sesquiterpenoids, phenylpropanoids, hydrocarbons, and aliphatic acid esters. Among them, trans ethyl p-methoxycinnamate, trans ethyl cinnamate, and n-pentadecane were determined to be the major components. The antioxidant activity tests in vitro and in vivo showed that KGEO could effectively eliminate different free radicals and protect zebrafish embryos against H2O2-induced oxidative stress. These findings suggested that KGEO had good antioxidant properties. It laid a solid foundation for further research to explain the underlying mechanisms and signaling pathways for the protective effects of KGEO, and is promising for development as a functional ingredient in the pharmaceutical, food and cosmetic industries.
The original contributions presented in this study are included in this article/supplementary material, further inquiries can be directed to the corresponding author.
The animal study was reviewed and approved by the Guangdong Laboratory Animals Monitoring Institute IACUC.
S-YW carried out the main experiments, analyzed the data, and wrote the manuscript. LC contributed to the zebrafish experiments. NY performed some of the in vitro antioxidant activity tests. F-FX and Y-SW contributed to the revision of the manuscript. BL designed the research plan and conceived the project. All authors contributed to the article and approved the submitted version.
This work was supported by the Key-Area Research and Development Program of Guangdong Province (Nos. 2020B1111110007 and 2008A08003), the Science and Technology Project of Guangzhou (Nos. 202201020488 and 202201020491), the Natural Science Foundation of Guangdong Province (Nos. 2017B030314166 and 2022A1515010103), the Specific Research Fund for TCM Science and Technology of Guangdong Provincial Hospital of Chinese Medicine (No. YN2020QN02), the China Postdoctoral Science Foundation (Nos. 2021M690756 and 2022M710908), Research Fund for Bajian Talents of Guangdong Provincial Hospital of Chinese Medicine (No. BJ2022KY08), the Collaborative Innovation Project of Guangzhou University of Chinese Medicine (Nos. 2021xk69 and 2021xk08), and the Special Funds for State Key Laboratory of Dampness Syndrome of Chinese Medicine (No. SZ2021ZZ33).
The authors declare that the research was conducted in the absence of any commercial or financial relationships that could be construed as a potential conflict of interest.
All claims expressed in this article are solely those of the authors and do not necessarily represent those of their affiliated organizations, or those of the publisher, the editors and the reviewers. Any product that may be evaluated in this article, or claim that may be made by its manufacturer, is not guaranteed or endorsed by the publisher.
1. Fernando IPS, Sanjeewa KKA, Samarakoon KW, Lee WW, Kim H, Kim E, et al. FTIR characterization and antioxidant activity of water soluble crude polysaccharides of Sri Lankan marine algae. Algae. (2017) 32:75–86. doi: 10.4490/algae.2017.32.12.1
2. Wang L, Jo MJ, Katagiri R, Harata K, Ohta M, Ogawa A, et al. Antioxidant effects of citrus pomace extracts processed by super-heated steam. LWT Food Sci Technol. (2018) 90:331–8. doi: 10.1016/j.lwt.2017.12.024
3. Kim YS, Hwang JW, Sung SH, Jeon YJ, Jeong JH, Jeon BT, et al. Antioxidant activity and protective effect of extract of Celosia cristata L. Flower on tert-butyl hydroperoxide-induced oxidative hepatotoxicity. Food Chem. (2015) 168:572–9. doi: 10.1016/j.foodchem.2014.07.106
4. Zheng F, Gonçalves FM, Abiko Y, Li H, Kumagai Y, Aschner M. Redox toxicology of environmental chemicals causing oxidative stress. Redox Biol. (2020) 34:101475. doi: 10.1016/j.redox.2020.101475
5. Balcerzak L, Gibka J, Sikora M, Kula J, Strub DJ. Minor constituents of essential oils and aromatic extracts. Oximes derived from natural flavor and fragranceraw materials – sensory evaluation, spectral and gas chromatographic characteristics. Food Chem. (2019) 301:125283. doi: 10.1016/j.foodchem.2005.07.042
6. Sarikurkcu C, Ceylan O, Targan S, Æavar Zeljkoviæ S. Chemical compositionand biological activities of the essential oils of two endemic Nepeta species. Ind Crop Prod. (2018) 125:5–8. doi: 10.1016/j.indcrop.2018.09.001
7. Wang SY, Zhao H, Xu HT, Han XD, Wu YS, Xu FF, et al. Kaempferia galanga L.: progresses in phytochemistry, pharmacology, toxicology and ethnomedicinal uses. Front Pharmacol. (2021) 12:675350. doi: 10.3389/fphar.2021.675350
8. Munda S, Saikia P, Lal M. Chemical composition and biological activity of essential oil of Kaempferia galanga: a review. J Essent Oil Res. (2018) 30:303–8. doi: 10.1080/10412905.2018.1486240
9. Jantan IB, Yassin MSM, Chin CB, Chen LL, Sim NL. Antifungal activity of the essential oils of nine zingiberaceae species. Pharmaceut Biol. (2003) 41:392–7. doi: 10.1076/phbi.41.5.392.15941
10. Mustafa RA, Hamid AA, Mohamed S, Bakar FA. Total phenolic compounds, flavonoids, and radical scavenging activity of 21 selected tropical plants. J Food Sci. (2010) 75:28–35. doi: 10.1111/j.1750-3841.2009.01401.x
11. Sulaiman MR, Zakaria ZA, Daud IA, Ng FN, Ng YC, Hidayat MT. Antinociceptive and anti-inflammatory activities of the aqueous extract of Kaempferia galanga leaves in animal models. J Nat Med. (2008) 62:221–7. doi: 10.1007/s11418-007-0210-3
12. Othman R, Ibrahim H, Mohd MA, Mustafa MR, Awang K. Bioassay-guided isolation of a vasorelaxant active compound from Kaempferia galanga L. Phytomedicine. (2006) 13:61–6. doi: 10.1016/j.phymed.2004.07.004
13. Ali H, Yesmin R, Satter A, Habib R, Yeasmin T. Antioxidant and anti-neoplastic activities of methanolic extract of Kaempferia galanga linn. Rhizome against Ehrlich ascites carcinoma cells. J King Saud Univ Sci. (2018) 30:386–92. doi: 10.1016/j.jksus.2017.05.009
14. Durmaz L, Kiziltas H, Guven L, Karagecili H, Alwasel S, Gulcin Ý. Antioxidant, antidiabetic, anticholinergic, and antiglaucoma effects of magnofluorine. Molecules. (2022) 18:5902. doi: 10.3390/molecules27185902
15. Rashid S, Rather MA, Shah WA, Bhat BA. Chemical composition, anti-microbial, cytotoxic and antioxidant activities of the essential oil of Artemisia indica willd. Food Chem. (2013) 138:693–700. doi: 10.1016/j.foodchem.2012.10.102
16. Nie JY, Li R, Jiang ZT, Wang Y, Tan J, Tang SH, et al. Screening and evaluation of radical scavenging active compounds in the essential oil from Magnolia biondii pamp by electronic nose coupled with chemical methodology. Ind Crops Prod. (2020) 144:112060. doi: 10.1016/j.indcrop.2019.112060
17. Durmaz L, Erturk A, Akyüz M, Polat Kose L, Uc EM, Bingol Z, et al. Screening of carbonic anhydrase, acetylcholinesterase, butyrylcholinesterase and α-glycosidase enzymes inhibition effects and antioxidant activity of coumestrol. Molecules. (2022) 10:3091. doi: 10.3390/molecules27103091
18. Wu WL, Zhu YT, Zhang L, Yang RW, Zhou YT. Extraction, preliminary structural characterization, and antioxidant activities of polysaccharides from Salvia miltiorrhiza bunge. Carbohyd Polym. (2012) 87:1348–53. doi: 10.1016/j.carbpol.2011.09.024
19. Zhang YY, Zhang F, Thakur K, Ci AT, Wang H, Zhang JG, et al. Effect of natural polyphenol on the oxidative stability of pecan oil. Food Chem Toxicol. (2017) 119:489–95. doi: 10.1016/j.fct.2017.10.001
20. Kiziltaş H, Bingol Z, Goren AC, Pınar S, Alwasel S, Gülçin Ý. LC-HRMS profiling of phytochemicals, antidiabetic, anticholinergic and antioxidant activities of evaporated ethanol extract of Astragalus brachycalyx Fischer. J Chem Metrol. (2021) 10:3091. doi: 10.25135/jcm.62.2107.2155
21. Sun Y, Zhang G, He Z, Wang Y, Cui J, Li Y. Effects of copper oxide nanoparticles on developing zebrafish embryos and larvae. Int J Nanomed. (2016) 11:905–18. doi: 10.2147/IJN.S100350
22. Kang MC, Kim KN, Kang SM, Yang X, Kim EA, Song CB, et al. Protective effect of dieckol isolated from Ecklonia cava against ethanol caused damage in vitro and in zebrafish model. Environ Toxicol Pharmacol. (2013) 36:1217–26. doi: 10.1016/j.etap.2013.09.018
23. Ma YL, Zhu DY, Thakur K, Wang CH, Wang H, Ren YF, et al. Antioxidant and antibacterial evaluation of polysaccharides sequentially extracted from onion (Allium cepa L.). Int J Biol Macromol. (2018) 111:92–101. doi: 10.1016/j.ijbiomac.2017.12.154
24. Amma KPP, Rani MP, Sasidharan I, Sreekumar MM. Comparative chemical composition and in vitro antioxidant activities of essential oil isolated from the leaves of Cinnamomum tamala and Pimenta dioica. Nat Prod Res. (2013) 27:290–4. doi: 10.1080/14786419.2012.668691
25. Ray A, Jena S, Dash B, Kar B, Halder T, Chatterjee T, et al. Chemical diversity, antioxidant and antimicrobial activities of the essential oils from Indian populations of Hedychium coronarium koen. Ind Crops Prod. (2018) 112:353–62. doi: 10.1016/j.indcrop.2017.12.033
26. Pei L, Li F. Theoretical investigation on the antioxidative property of imine resveratrol. Chem Res Appl. (2019) 31:612–8.
27. Kim DO, Lee KW, Lee HJ, Lee CY. Vitamin C equivalent antioxidant capacity (VCEAC) of phenolic phytochemicals. J Agric Food Chem. (2002) 50:3713–7. doi: 10.1021/jf020071c
28. Zhang P, Yuan S. Production of hydroxyl radicals from abiotic oxidation of pyrite by oxygen under circumneutral conditions in the presence of low-molecular-weight organic acids. Geochim Cosmochim Acta. (2017) 218:153–66. doi: 10.1016/j.gca.2017.08.032
29. Hazra B, Sarkar R, Biswas S, Mandal N. Comparative study of the antioxidant and reactive oxygen species scavenging properties in the extracts of the fruits of Terminalia chebula, Terminalia belerica and Emblica officinalis. BMC Complementary Altern Med. (2010) 10:20. doi: 10.1186/1472-6882-10-20
30. Zhang Y, Chen J, Wang L, Cao J, Xu L. Chemical composition and biological activities of the essential oil from Rubus pungens var. oldhamii. Nat Prod Res. (2017) 31:1454–8. doi: 10.1080/14786419.2016.1253076
31. Wang Y, Jiang ZT, Li R, Guan WQ. Composition comparison of essential oils extracted by microwave assisted hydrodistillation and hydrodistillation from Kaempferia galanga l. Grown in china. J Essent Oil Bear Plants. (2009) 12:415–21. doi: 10.1080/0972060X.2009.10643739
32. Liu XC, Liang Y, Shi WP, Liu QZ, Zhou L, Liu ZL. Repellent and insecticidal effects of the essential oil of Kaempferia galanga rhizomes to Liposcelis bostrychophila (psocoptera: liposcelidae). J Econ Entomol. (2014) 4:1706–12. doi: 10.1603/EC13491
33. Alsalhi MS, Elumalai K, Devanesan S, Govindarajan M, Krishnappa K, Maggi F. The aromatic ginger Kaempferia galanga l. (zingiberaceae) essential oil and its main compounds are effective larvicidal agents against Aedes vittatus and anopheles maculatus without toxicity on the non-target aquatic fauna. Ind Crops Prod. (2020) 158:113012. doi: 10.1016/j.indcrop.2020.113012
34. Cherrat L, Espina L, Bakkali M, García-Gonzalo D, Pagán R, Laglaoui A. Chemical composition and antioxidant properties of Laurus nobilis l. And Myrtus communis l. Essential oils from morocco and evaluation of their antimicrobial activity acting alone or in combined processes for food preservation. J Sci Food Agric. (2014) 94:1197–204. doi: 10.1002/jsfa.6397
35. Rashid S, Rather MA, Shah WA, Bhat BA. Chemical composition, antimicrobial, cytotoxic and antioxidant activities of the essential oil of Artemisia indica willd. Food Chem. (2013) 138:693–700. doi: 10.1016/j.foodchem.2012.10.102
36. Wang L, Cui YR, Lee HG, Fu X, Wang K, Xu J, et al. Fucoidan isolated from fermented Sargassum fusiforme suppresses oxidative stress through stimulating the expression of superoxidase dismutase and catalase by regulating Nrf2 signaling pathway. Int J Biol Macromol. (2022) 209:935–41. doi: 10.1016/j.ijbiomac.2022.04.083
37. Lee HG, Jayawardena TU, Liyanage NM, Song KM, Choi YS, Jeon YJ, et al. Antioxidant potential of low molecular weight fucoidans from Sargassum autumnale against H2O2-induced oxidative stress in vitro and in zebrafish models based on molecular weight changes. Food Chem. (2022) 384:132591. doi: 10.1016/j.foodchem.2022.132591
38. Willcox JK, Ash SL, Catignani GL. Antioxidants and prevention of chronic disease. Crit Rev Food Sci Nutr. (2004) 44:275–95. doi: 10.1080/10408690490468489
Keywords: Kaempferia galanga L., essential oil, antioxidant activity, zebrafish, oxidative stress
Citation: Wang S-Y, Cai L, Yang N, Xu F-F, Wu Y-S and Liu B (2023) Chemical composition of the Kaempferia galanga L. essential oil and its in vitro and in vivo antioxidant activities. Front. Nutr. 10:1080487. doi: 10.3389/fnut.2023.1080487
Received: 26 October 2022; Accepted: 16 January 2023;
Published: 02 February 2023.
Edited by:
Lin Zhou, Guangdong Pharmaceutical University, ChinaCopyright © 2023 Wang, Cai, Yang, Xu, Wu and Liu. This is an open-access article distributed under the terms of the Creative Commons Attribution License (CC BY). The use, distribution or reproduction in other forums is permitted, provided the original author(s) and the copyright owner(s) are credited and that the original publication in this journal is cited, in accordance with accepted academic practice. No use, distribution or reproduction is permitted which does not comply with these terms.
*Correspondence: Bo Liu, ZG9jdGxpdUAyNjMubmV0
Disclaimer: All claims expressed in this article are solely those of the authors and do not necessarily represent those of their affiliated organizations, or those of the publisher, the editors and the reviewers. Any product that may be evaluated in this article or claim that may be made by its manufacturer is not guaranteed or endorsed by the publisher.
Research integrity at Frontiers
Learn more about the work of our research integrity team to safeguard the quality of each article we publish.