- 1Key Laboratory of Animal Disease-Resistant Nutrition, Ministry of Education, Ministry of Agriculture and Rural Affairs, Key Laboratory of Sichuan Province, Animal Nutrition Institute, Sichuan Agricultural University, Chengdu, China
- 2Adisseo France SAS, Commentry, France
- 3Sichuan Province Key Laboratory of Animal Disease and Human Health, Key Laboratory of Environmental Hazard and Human Health of Sichuan Province, College of Veterinary Medicine, Sichuan Agricultural University, Chengdu, China
Selenium (Se) is assumed to promote the follicle development by attenuating oxidative stress. The current study was developed to evaluate the effects of dietary 2-hydroxy-4-methylselenobutanoic acid (HMSeBA) supplementation on the follicle development in vivo and on the function of ovarian granulosa cells (GCs) in vitro. Thirty-six gilts were randomly assigned to fed control diet (CON), Na2SeO3 diet (0.3 mg Se/kg) or HMSeBA diet (0.3 mg Se/kg). The results showed that HMSeBA and Na2SeO3 supplementation both increased the total selenium content in liver and serum compared with control, while HMSeBA increased the total selenium content in liver compared with Na2SeO3 group. HMSeBA tended to increase the total selenium content in ovary compared with control. HMSeBA and Na2SeO3 supplementation both increased the weight of uteri in gilts at the third estrus. Moreover, HMSeBA supplementation down-regulated the gene expression of growth differentiation factor-9 (GDF-9) and bone morpho-genetic protein-15 (BMP-15) in cumulus-oocyte complexes (COCs). HMSeBA supplementation decreased malondialdehyde (MDA) content in serum, liver and ovary, increased activity of T-AOC in liver, TXNRD in ovary and GPX in serum, liver and ovary, while up-regulated the liver GPX2, SOD1 and TXNRD1, ovarian GPX1 gene expression. In vitro, HMSeBA treatment promoted GCs' proliferation and secretion of estradiol (E2). HMSeBA treatment increased the activity of T-AOC, T-SOD, GPX, TXNRD and decreased MDA content in GCs in vitro. Meanwhile, HMSeBA treatment up-regulated SOD2 and GPX1 gene expression in GCs in vitro. In conclusion, HMSeBA supplementation is more conducive to promoting follicle development by antioxidant pathway.
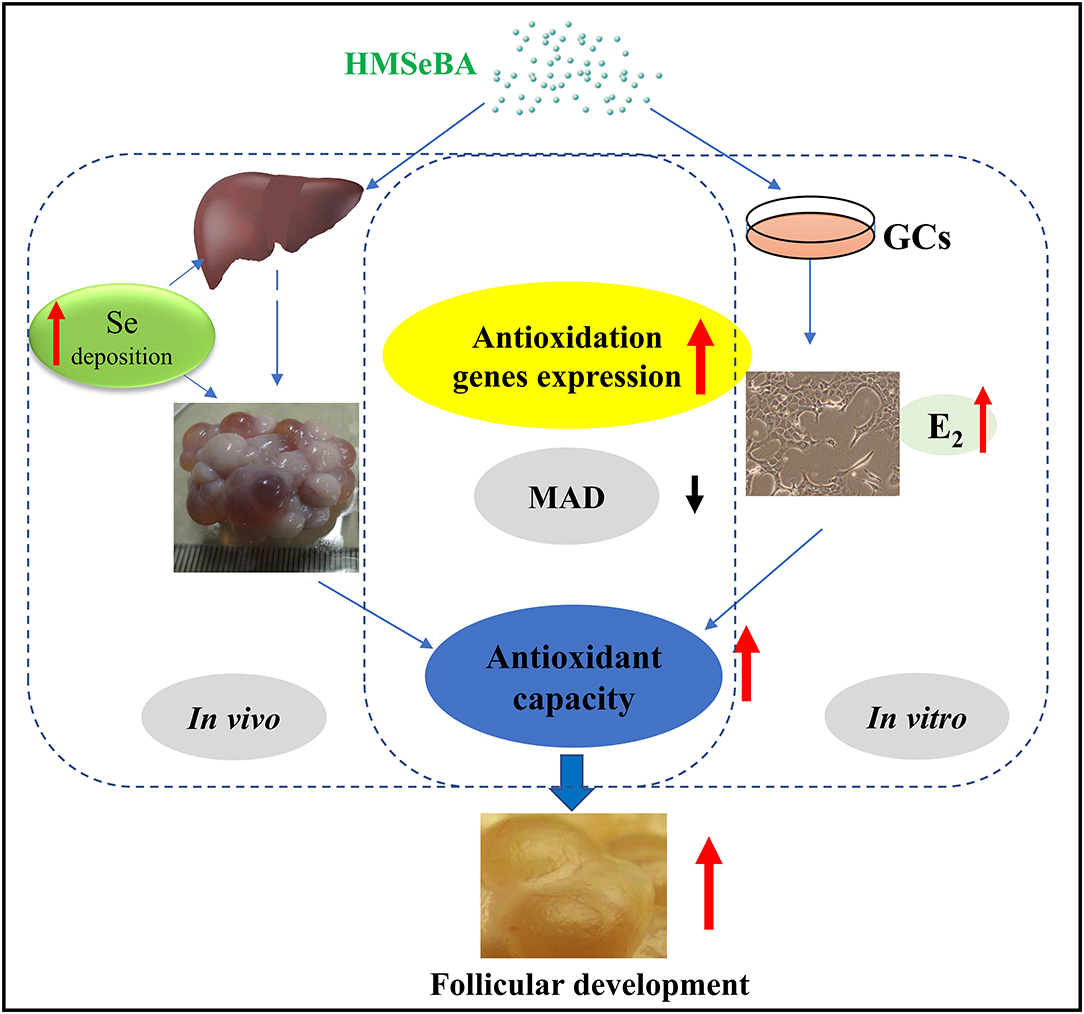
Graphical Abstract. Model of proposed mechanism of 2-Hydroxy-4-methylselenobutanoic acid (HMSeBA) regulated follicle development by antioxidant pathway.
Introduction
Ovarian follicular development is the most important event in reproductive performance in the gilt. The proliferation and differentiation of granulosa cells (GCs) determine ovarian follicular development (1). 17β-estradiol (E2) is synthesized by androgen in granulose cell via the aromatization by cytochrome P450 aromatase. E2 is one of the key ovarian hormones produced by the developing ovulatory follicle. E2 content reflect the differentiation of ovarian GCs. E2 is essential for female reproduction, as evidenced by severe fertility defects when its synthesis or action are suppressed (2, 3). With the metabolism of ovary, the reactive oxygen species (ROS) and free radicals would be generated (4). Those metabolites must be locally neutralized to maintain tissue integrity and function. The antioxidants such as vitamins C and the Se-dependent glutathione peroxidase (GPX) system would protect against ROS and free radicals.
Selenium (Se) is one of the essential trace elements for animals, which can effectively improve its reproductive performances (5–7). Types of selenium in nature include inorganic and organic selenium compounds (8, 9). Se may has contribution to the basal high ovulation rate, such as the high basal Se level (0.3 mg/kg) increased the average number of corpora lutea (19.4 vs. 17.4, number of corpus luteum represented the number of ovulations of last estrus) than lower Se concentration (Se level 0.2 mg/kg) (10, 11). Fortier et al. (12) studied the effects of organic selenium and inorganic selenium on the antioxidant capacity and reproductive performance of sow. The results showed that the GPX activity in the blood of control group sows decreased by 3.2%, while the inorganic (sodium selenite) and organic selenium (Se-enriched yeast) groups increased by 19.6 and 13.7%, respectively (12). The above studies showed that sow diet supplementation with selenium can improve the antioxidant capacity and then protect itself from oxidative stress.
Recently, one new organic selenium product named Selisseo® (SO) has been developed. Selenium in this product is present as 2-hydroxy-4-methylselenobutyric acid (HMSeBA). Studies have shown that HMSeBA is more bioavailable than selenium yeast or sodium selenite in poultry, growing pigs and weaned pigs (9, 13–15). Our laboratory also found HMSeBA supplementation during sow pregnancy increased the number of total born piglets, decreased piglet birth interval, improved concentrations of total selenium, and also improved activity of antioxidant enzymes compare with the control and sodium selenite treatment (16). However, the available data concerning HMSeBA action on the gilts' reproduction and function on the granulose cells remain insufficient. Since the outbreak of African swine fever in China in 2018, to restore production capacity as soon as possible, many pig farms in China have begun to use Duroc × Landrace × Yorkshire (DLY) commercial gilts for breeding. However, before the outbreak of African swine fever, there were fewer reports on the use of DLY gilt as breeding gilt. Therefore, the effect of HMSeBA supplementation on the onset of puberty and follicle development in DLY gilts, as well as its potential mechanisms on the granulose cell in vitro were examined.
Materials and Methods
Animals and Diet
All experimental procedures used in present study were approved by the Animal Care and Use Committee of Sichuan Agricultural University (Approval Number 20200722). Thirty-six female piglets (Duroc × Landrace × Yorkshire, 5.50 ± 0.09 kg) were randomly assigned to three dietary treatment groups: (1) control diet (CON, negative control, basal diet, 0.09, 0.06 and 0.04 mg Se/kg at three phases), (2) sodium selenite (Na2SeO3) supplementation diet (Na2SeO3, positive control, basal diet + Na2SeO3 at 0.3 mg Se/kg), (4) 2-hydroxy-4-methylselenobutyric acid supplementation diet (HMSeBA, Selisseo® 2% Se provided by Adisseo France, basal diet + HMSeBA at 0.3 mg Se/kg). Each treatment had 12 gilts, six repetitions in each treatment and two gilts per repetition. CON diet was corn-soybean meal-based (Supplementary Table S1). The experiment was terminated at the 19th day after the second estrus. Gilts were fed four times (08:00; 12:00; 16:00; 20:00) a day from beginning to 90 days of age, and from 90 days to slaughter, were fed twice (08: 00; 16:00) a day. Gilts were feed freely before 176 days of age, the feed limited 2.5 kg/d/gilt from 176 days of age to slaughter. After 176 days of age, gilts were exposed to a rotation of mature boars twice (before feeding at 8:00 and 16:00) per day. Estrous identification was performed based on the behavioral and vulvar characteristics by an experienced stockman. Recorded the estrus interval and backfat thickness of gilts.
Sample Collection
One gilt from each repeatment (n = 5/treatment, one repeatment was excluded, because of diarrhea and sick when younger) of each treatment were randomly selected and slaughtered on the morning of the 19th day after the second estrus after fasting for 12 h. Before slaughter, 10 ml blood sample was collected from jugular vein. To obtaining the serum, blood samples were centrifuged at 3,000 × g, 4°C for 15 min, and then serum was stored at −20°C.
After the gilt was slaughtered, the liver and reproductive tract were dissected. The liver sample from left side were collected from each gilt and frozen in liquid nitrogen until transferring them to store at −80°C. Ovaries were separated from the uterine horns and weighed. The uterus was subsequently trimmed and weighed. The antral follicles which > 1 mm in diameter were measured and recorded within different size categories (small: 1–5 mm in diameter, large: >5 mm in diameter). The number of corpora lutea was recorded, too. Then, the follicle contents of left ovary were aspirated from the follicles with > 5 mm in diameter by a 10-ml syringe. Cumulus-oocyte complexes (COCs) were recovered from the aspirate using a dissecting microscope (40 × magnification). Then the rest follicle contents were centrifuged at 2,000 rpm for 5 min. The follicular fluid (supernatant) was collected and stored at −20°C until analysis was conducted.
Granulosa Cell Culture and Cell Proliferation Assay
Mouse ovarian granulosa cells (GC, Shanghai Sixin Biological Technology Co., Ltd. Due to the strict control of slaughterhouses since the outbreak of African swine fever in China, there was no way to enter the slaughterhouse to obtain porcine ovaries. Then mouse granule cell line was chosen as the model in the in vitro experiments based on the high homology of swine and mice) at passage 3 or 4 at 2 × 105 cells (viable cell, determined by trypan blue) per well were seeded in 6-well tissue culture-treated plate in 2 ml DMEM supplemented with extra 1X antibiotic/antimicotic and 10% FBS. Added sodium selenite or HMSeBA working solution at 1 ng Se/ul according to the final concentration of 0, 2.5, 5, 10 ng Se /ml, respectively. Then the plate incubated in a humidified atmosphere of 5% CO2 at 37°C for 24 h. The cells should be around 40–50 % confluent, aspirated culture medium and washed attached cells once with 1* PBS. Added new culture medium with the final treatment concentration of selenium and incubated for 24 h (about 90 % confluent) (17). Then the supernatant and cells were collected and stored at −80°C.
The MTT [3-(4, 5-dimethylthiazol-2-yl)-2, 5-diphenyltetrazolium bromide] assay was applied to analysis the GCs' proliferation according to the manufacturer's protocol (Nanjing Jiancheng Institute, Jiangsu, China). Briefly, GCs were cultured with different concentration of sodium selenite or HMSeBA at 96-well tissue culture plates. After cultured for 44 h, the supernatant was removed and replaced by a solution composed of complete medium and MTT salt solution at ratio of 4:1. After 4 h of incubation, supernatant was removed, then added DMSO to each well and shake for 10 min to fully melt the crystals. Finally, the measurements were performed by a microplate reader (Model 680 Microplate Reader, Bio-Rad Laboratories) with 570 nm interference filters. Each experiment was carried out in triplicate.
Selenium Content Analysis
The concentrations of selenium in serum, tissue and feed samples were detected as previously described (18). Briefly, potassium borohydride and hydrochloric acid were used to reduce the hexavalent selenium in samples into hydrogen selenide in a hydrochloric acid medium. Then, selenium content in samples were detected by hydride atomic fluorescence spectrometry (AFS-9230, Beijing Auspicious Day Instrument Co., LTD., Beijing, China).
Hormone Levels Analysis
Serum and follicular fluid follicle stimulating hormone (FSH), estradiol (E2) and luteinizing hormone (LH) were detected by porcine enzyme-linked immunosorbent assay (ELISA) Kits (R&D Systems Inc., Minneapolis, MN, USA). Optical density (OD) values were detected at 450 nm by a MuLtisKan MK3-Thermo Labsystems microplate reader (Thermo Labsystems, CA, USA). Serum three iodine thyroid (T3) and thyroxine (T4) were detected by porcine ELISA Kits (Nanjing Jiancheng Institute of Biological Technology, Jiangsu, China) according to manufacturer's protocol.
GCs were cultured with different concentration of Na2SeO3 or HMSeBA. After cultured 48 h with once media change, the media was collected. The spent media were assayed for the presence of E2 by ELISA kit (R&D Systems Inc., Minneapolis, MN, USA) based on the manufacturer's protocol. Each experiment was carried out in triplicate for in vitro experiment.
Antioxidant Analysis
The activities of glutathione peroxidase (GPX), glutathione reductase (GR), thioredoxin reductase (TXNRD), total antioxidative capability (T-AOC), total superoxide dismutase (T-SOD) and malondialdehyde (MDA) of gilts' serum, liver, ovary, and supernatant and GCs of in vitro experiment were quantified by the respective assay kits (Nanjing Jiancheng Institute, Jiangsu, China). Briefly, the activities of GPX, GR and SOD were assayed as described by Mou et al. (19). MDA content was measured based on the thiobarbituric acid (TBA) method.
Assessment of Gene Expression
Total RNA was isolated from gilt samples and GCs of in vitro experiment using the Trizol reagent (TaKaRa Biotechnology, Dalian, China). Agarose gel electrophoresis was applied to determine the integrity of RNA. By evaluating the OD260/OD280 ratio to determine RNA purity. Genomic DNA removal and reverse transcription (RT) were performed by PrimeScript RT reagent kit with gDNA eraser (TaKaRa Biotechnology, Dalian, China) based on the manufacturer's protocol. SYBR® Premix Ex TaqTM Kits (TaKaRa Biotechnology, Dalian, China) was appiled for real-time PCR analysis of mRNA expression. The primer sequences of the target genes were showed in Supplementary Table S2. The PCR protocol was 95°C for 30 s, then 40 cycles at 95°C for 5 s, followed 60°C for 35 s. The PCR products were electrophoresed on agarose gel to determine the product size. The data were analyzed by 2 -delta delta CT method with β-actin as housekeeping gene (20).
Statistical Analysis
The repeatment was regarded as the experimental units for the animal growth performance. One gilt of a repeatment of three treatments had diarrhea or sick when younger, therefore, that repeatment was excluded from the present study. The gilt was regarded as the experimental units for the animal study except for the animal growth performance. Before using parametric analyses, all data were checked for normality and homogeneity of variance with Shapio-Wilk W test and Levene's test, respectively. All data were analyzed by one-way ANOVA using SPSS Statistics 22 (IBM® SPSS® Statistics, New York, NY, USA) and GraphPad Prism 6.0 (GraphPad Inc., La Jolla, CA, USA; figures). Then multiple comparison by DUNCAN analysis (Tukey's multiple range test was used in all figures) were used to determine statistical differences between treatment groups. The results were presented as mean with SE. Statistically significant difference was considered at P < 0.05. A tendency was assumed at P < 0.1.
Results
Effects of Selenium on the Selenium Content in Gilts
Dietary supplementation with HMSeBA and Na2SeO3 increased the total selenium content in serum and liver (P < 0.05, Figure 1) compared with control treatment in gilts, while HMSeBA treatment increased the total selenium content in liver compared with Na2SeO3 treatment in gilts (P < 0.05). Dietary supplementation with HMSeBA tended to increase the total selenium content in ovary compared with control treatment in gilts (P = 0.08).
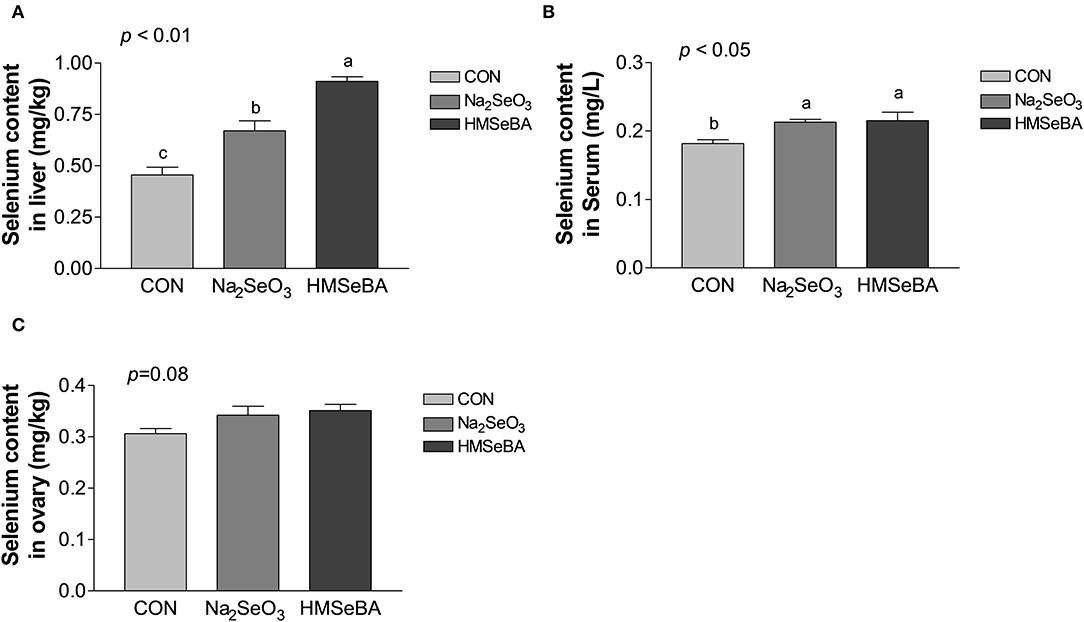
Figure 1. Effects of dietary selenium supplementation on the selenium content in liver (A), serum (B) and ovary (C) of gilts. Data are expressed as means values and standard error, n = 5. Mean values with different superscript letters were significantly different (P < 0.05). CON, basal diet; Na2SeO3, 0.3 mg Se/kg Na2SeO3; HMSeBA, 0.3 mg Se/kg HMSeBA.
HMSeBA Changed the Growth and Reproduction Performance in Gilts
Dietary supplementation with HMSeBA increased the average daily body weight gain (ADG) (P < 0.05, Supplementary Table S3) and reduce the ratio of feed: gain from day 120 to 176 in gilts.
Age at puberty, body weight at puberty, backfat at puberty and duration of estrus cycle were not affected by selenium (Supplementary Figure S1). HMSeBA and Na2SeO3 both increased the weight of uteri and relative weight of uteri (P < 0.05, Figure 2). The bodyweight at slaughter, ovaries weight, length of uterine horn and internal organs was not affected by HMSeBA and Na2SeO3 (Supplementary Table S4). The number of small follicles (diameter, 1–5 mm), large follicles (>5 mm; these follicles will ovulate in the estrus) and corpora lutea (No. of corpus luteum represents the number of ovulations at last estrus.) were not affected by HMSeBA and Na2SeO3 (Figures 3A–C). Despite no morphological changes we have also tested molecular markers of oocyte development included porcine growth differentiation factor-9 (GDF-9) and bone morphogenetic protein-15 (BMP-15). The relative gene expression of GDF-9 and BMP-15 in COCs was lower in HMSeBA treatment than that in control treatment (P < 0.05, Figures 3D,E).
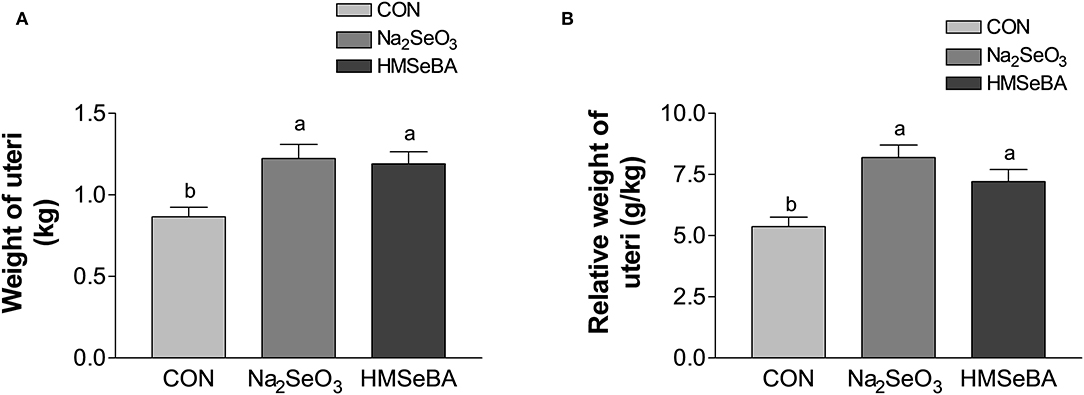
Figure 2. Effects of dietary selenium supplementation on the weight of uteri (A) and relative weight of uteri (B) of gilts. Data are expressed as means values and standard error, n = 5. Mean values with different superscript letters were significantly different (P < 0.05). CON, basal diet; Na2SeO3, 0.3 mg Se/kg Na2SeO3; HMSeBA, 0.3 mg Se/kg HMSeBA.
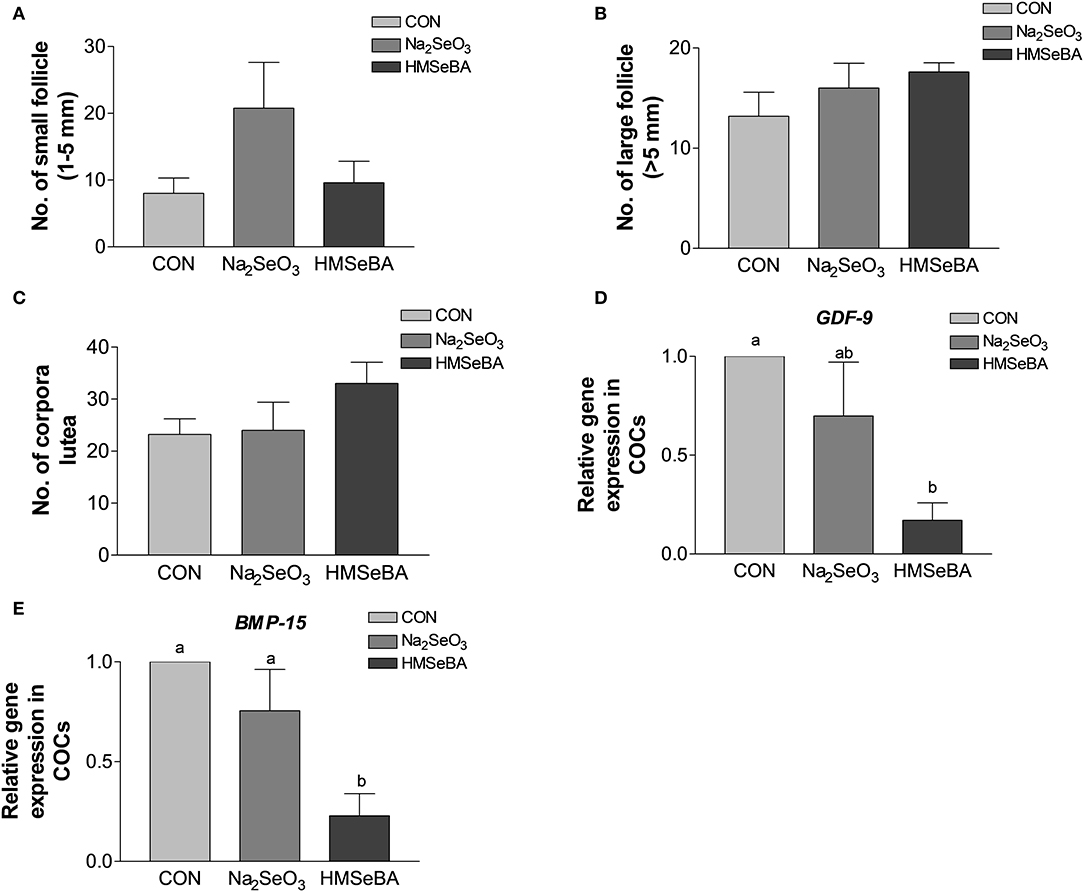
Figure 3. Effects of dietary selenium supplementation on the development of follicles and relative gene expression of COCs in gilts. (A) Number of small follicle (diameter 1–5 mm). (B) Number of large follicle (diameter > 5 mm). (C) Number of corpora lutea (represented the number of ovulations of last estrus). (D) Relative gene expression of GDF-9 in cumulus-oocyte complexes (COCs). (E) Relative gene expression of BMP15 in COCs. Mean values with different superscript letters were significantly different (P < 0.05). CON, basal diet; Na2SeO3, 0.3 mg Se/kg Na2SeO3; HMSeBA, 0.3 mg Se/kg HMSeBA; GDF-9, growth differentiation factor-9; BMP-15, bone morpho-genetic protein-15.
Dietary supplementation with selenium (HMSeBA and Na2SeO3) did not change the FSH, LH and estradiol concentration in serum and follicular fluid of gilts compared with control treatment (Supplementary Table S5).
Oxidative Status Indicators in the Serum and Tissues in Gilts
HMSeBA supplementation decreased the concentration of MDA (P < 0.05, Figure 4A) as compared with control treatment in serum, liver and ovary of gilts, and increased the GPX activity in serum, liver and ovary compared with control treatment (P < 0.05, Figure 4B). The concentration of T-AOC in liver increased in HMSeBA and Na2SeO3 treatments compared with control (P < 0.05, Figure 4C). The activity of T-SOD increased in Na2SeO3 treatment compared with HMSeBA and control in the ovary of gilts (P < 0.05, Figure 4D). There was no difference in GR concentration among treatments (P > 0.05, Figure 4E). The concentration of TXNRD increased in HMSeBA and Na2SeO3 treatments compared with control in ovary of gilts (P < 0.05, Figure 4F).
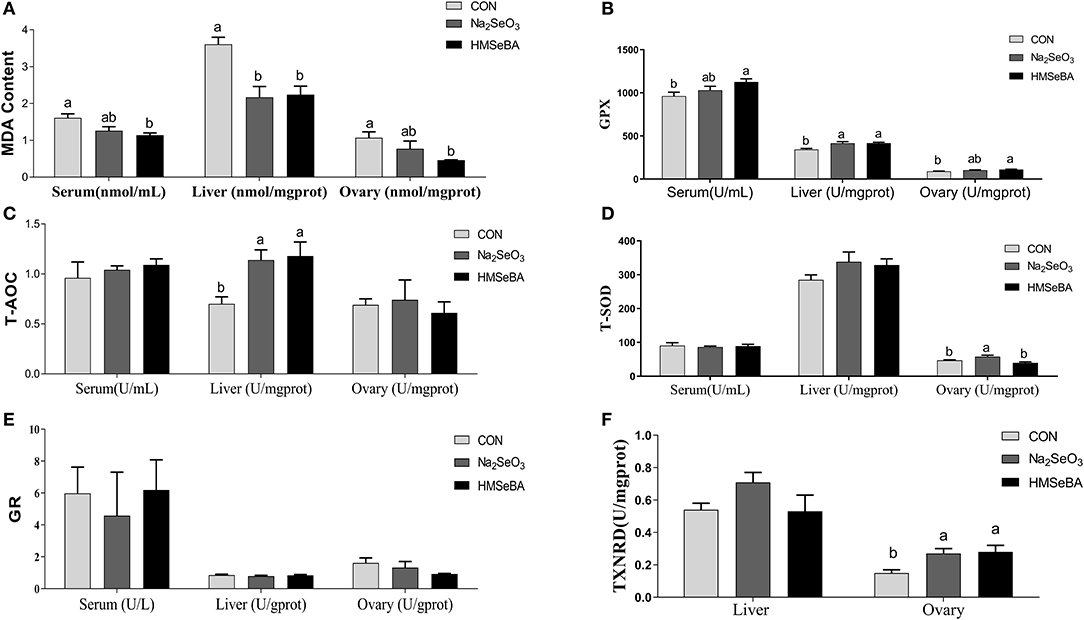
Figure 4. Effects of dietary selenium supplementation on the oxidative status indicators in gilts. (A) MDA, (B) GPX activity, (C) T-AOC, (D) SOD activity, (E) GR activity, and (F) TXNRD in serum, liver and ovary of gilt. Data are expressed as means values and standard error, n = 5. Mean values with different superscript letters were significantly different (P < 0.05). CON, basal diet; Na2SeO3, 0.3 mg Se/kg Na2SeO3; HMSeBA, 0.3 mg Se/kg HMSeBA; MDA, malondialdehyde; GPX, glutathione peroxidase; T-AOC, total antioxidant capacity; T-SOD, total superoxide dismutase; GR, gluathione reductase; TXNRD, thioredoxin reductase.
HMSeBA Changed the Expression of Selenoproteins and Antioxidant Capacity Related Genes in Gilts
The relative gene expression of GPX2, GPX3 and TXNRD1 increased (P < 0.05) in HMSeBA treatment compared with Na2SeO3 treatment in the liver of gilts, while the relative gene expression of GPX1 increased in HMSeBA treatment compared with Na2SeO3 treatment in the ovary of gilts (Figure 5). The expression of SELENOW and SELENOO decreased (P < 0.05) in Na2SeO3 supplementation treatment compared with control in the ovary. Besides, HMSeBA supplementation significantly increased (P < 0.05) the expression of SOD1 in the liver compared with control and Na2SeO3 groups. The expression of TXN1 increased (P < 0.05) in HMSeBA treatment compared with Na2SeO3 group in the liver of gilts. In addition to the above genes, although the difference was not significant, it was observed a numerical improvement in gene expression with HMSeBA compared to Na2SeO3 for all of the selenoproteines in liver.
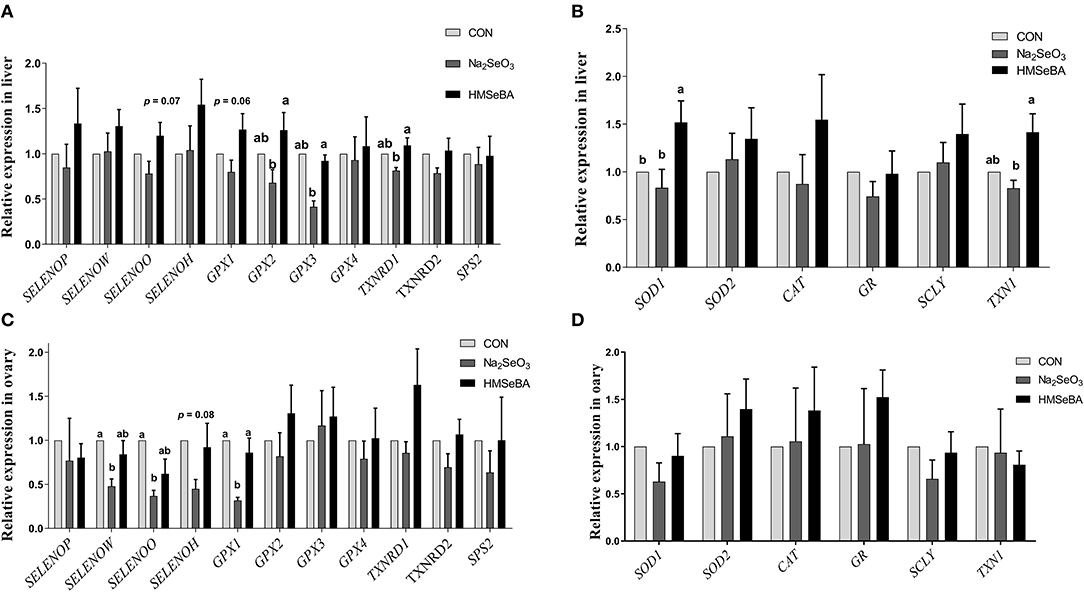
Figure 5. Effects of dietary selenium supplementation on the relative expression of genes related to selenoprotein and antioxidant capacity in the liver and ovary of gilts. (A) Expression of selenoprotein genes in liver of gilt. (B) Expression of antioxidant capacity system related genes in liver of gilt. (C) Expression of selenoprotein genes in ovary of gilt. (D) Expression of antioxidant capacity system related genes in ovary of gilt. Data are expressed as means values and standard error, n = 5. Mean values with different superscript letters were significantly different (P < 0.05). CON, basal diet; Na2SeO3, 0.3 mg Se/kg Na2SeO3; HMSeBA, 0.3 mg Se/kg HMSeBA; SELENOP: selenoprotein P; SELENOW: selenoprotein W; SELENOO: selenoprotein O; SELENOH: selenoprotein H; GPX: glutathione peroxidase; TXNRD: thioredoxin reductase; SPS2: Selenophosphate synthetase 2; SOD: superoxide dismutase; CAT: catalase; GR: Glutathione reductase; SCLY: Selenocysteine lyase; TXN1: Thioredoxin l.
Effect of Selenium on the Granulosa Cell Proliferation and Estradiol Secretion in vitro
HMSeBA significantly increased the cell proliferation at 5 ng/ml (P < 0.05, Figure 6A), Na2SeO3 had tendency to increased cell proliferation at 2.5 ng/ml (P = 0.097). Estradiol secretion is an important manifestation of the physiological function of granular cells. HMSeBA significantly increased the E2 concentration compared with control treatment at 5 and 10 ng/ml (P < 0.05, Figure 6B). However, E2 concentration was not affected by different concentrations of Na2SeO3 in granulosa cells.
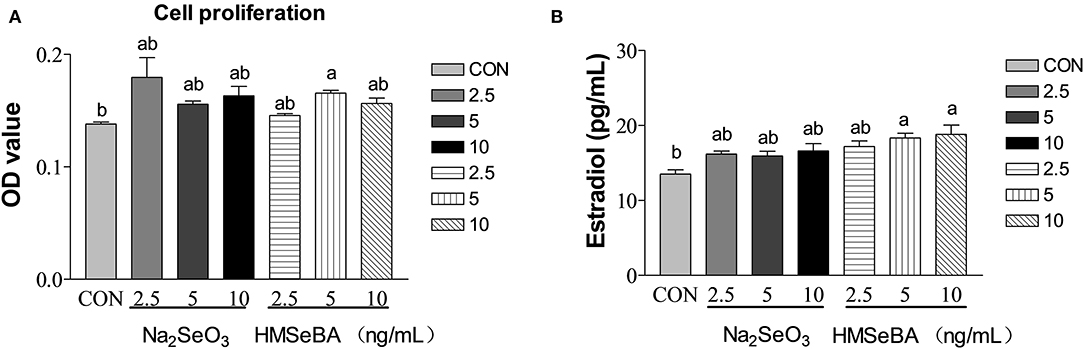
Figure 6. Effects of selenium from different sources and concentrations on the granulosa cells' proliferation (A) and estradiol (B) secretion in granulosa cells in vitro. Data are expressed as means values and standard error. a,b Mean values with different superscript letters were significantly different (P < 0.05). CON: basal media, Na2SeO3 2.5, 5 and 10 ng Se/ml, HMSeBA 2.5, 5, and 10 ng Se/ml.
Effect of Selenium on the Oxidative Stress Status in Granulosa Cells in vitro
Compared with control, the total antioxidant capacity of GCs in vitro was improved by HMSeBA and Na2SeO3 (P < 0.05, Figure 7A). The intracellular T-SOD content increased (P < 0.05, Figure 7B) at 2.5 ng/ml of HMSeBA. The intracellular GPX level increased (P < 0.05, Figure 7C) at a concentration of 5 ng/ml of Na2SeO3 and HMSeBA. The GR concentration increased (P < 0.05, Figure 7D) at 2.5 ng/ml of HMSeBA compared with control. The content of TXNRD increased at 2.5 ng/ml of HMSeBA in the GCs (P < 0.05, Figure 7E). Compared with control, Na2SeO3 and HMSeBA both decreased the concentration of MDA (P < 0.05, Figure 7F) at the concentration of 5 ng/ml of Na2SeO3 and 5, 10 ng/ml of HMSeBA.
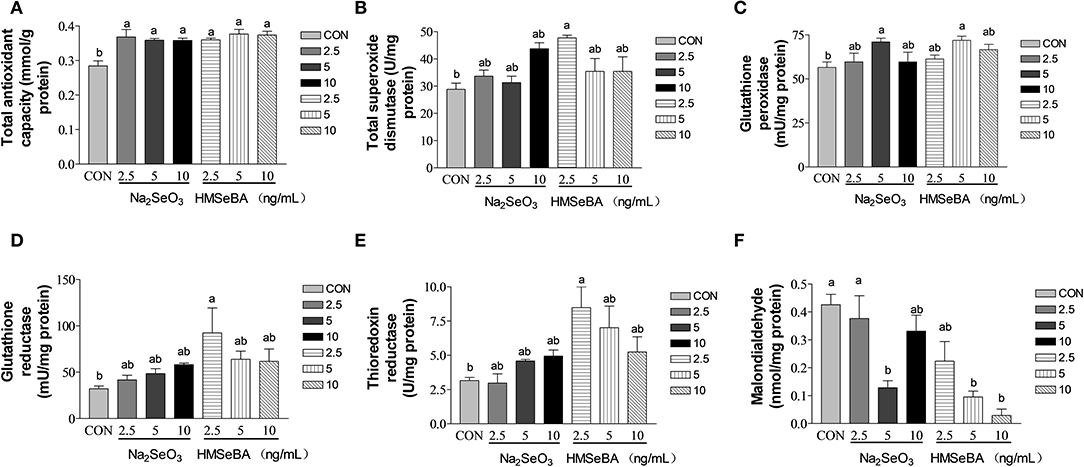
Figure 7. Effects of selenium from different sources and concentrations on the oxidative stress status in the granulosa cells in vitro. (A) T-AOC activity, (B) T-SOD activity, (C) GPX activity, (D) GR activity, (E) TXNRD, and (F) MDA in the granulosa cells in vitro. Data are expressed as means values and standard error. a,b Mean values with different superscript letters were significantly different (P < 0.05). CON: basal media, Na2SeO3 2.5, 5 and 10 ng Se/ml, HMSeBA 2.5, 5, and 10 ng Se/ml.
Effect of Selenium on the Oxidative Stress Relative Gene Expression in Granulosa Cells in vitro
HMSeBA increased the expression of SOD2 and GR gene in granulosa cells at 10 ng/ml and 2.5 ng/ml (P < 0.05, Figures 8B,C). HMSeBA and inorganic selenium Na2SeO3 both up-regulated (P < 0.05, Figure 8E) antioxidant-related GPX1 gene expression in GCs at the concentration of 5, 10 ng/ml of Na2SeO3 and 5 ng/ml of HMSeBA. However, there was no significant difference at transcription level of SOD1 and TXNRD1 when granulosa cells treated with different concentrations of Na2SeO3 or HMSeBA (Figures 8A,D).
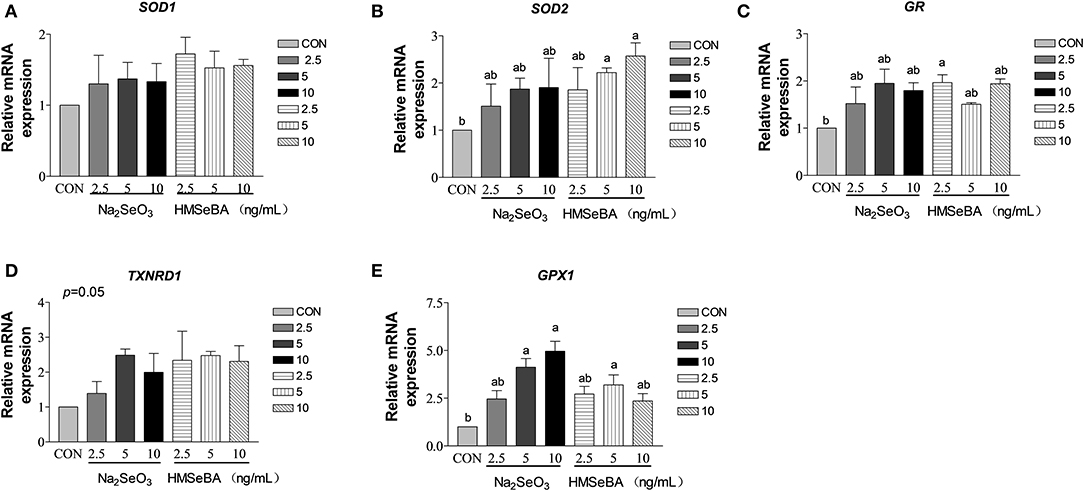
Figure 8. Effects of selenium from different sources and concentrations on the oxidative stress relative gene expression in granulosa cells in vitro. Relative gene expression of (A) SOD1, (B) SOD2, (C) GR, (D) TXNRD, and (E) GPX1 in the granulosa cells in vitro. Data are expressed as means values and standard error. a,b Mean values with different superscript letters were significantly different (P < 0.05). CON: basal media, Na2SeO3 2.5, 5 and 10 ng Se/ml, HMSeBA 2.5, 5, and 10 ng Se/ml; SOD1, superoxide dismutase 1; SOD2, superoxide dismutase 2; GR, glutathione reductase; TXNRD, thioredoxin reductase; GPX1, glutathione peroxidase.
Discussion
Studies show that selenium is necessary for fertility (5–7, 9). HMSeBA as an organic Se source has been studied in poultry, weaned piglets, growing pigs and sows (9, 13, 16, 21). This study focused on the effect of dietary supplementation with HMSeBA on the follicle development in gilts. The elemental distribution in the bovine ovary showed that Se is localized at the granulosa cell layer of large follicles (410 mm) which was identified by X-ray fluorescence (XRF) imaging (22). As far as we know, folliculogenesis is characterized by a rapid growth of small primary follicles with few GCs to mature preovulatory follicles with several layers of GCs (23). Granulosa cell growth is therefore an important feature in this developmental process. So, further we investigated the effect of HMSeBA on the proliferation and function in GCs in vitro. The results of present study will provide a reference for the application of HMSeBA in gilt diet.
In this study, the gilts were applied the same treatments with their maternal generation during gestation (16). Selenium concentrations in serum and liver was increased by both HMSeBA and Na2SeO3 diet in comparison with gilts receiving the unsupplemented control diet. These results agreed with previous studies on pigs (9, 15, 24) and even on other species such as poultry (13, 21), sheep (25) and cattle (26). The total selenium content increased in liver in HMSeBA treatment compared with Na2SeO3 treatment, similarity results were obtained by Hu et al. (27) and Zhan et al. (28), which found that Se concentration elevation was greater when the organic Se source was provided. We also found that dietary supplementation with HMSeBA tended to increase the total selenium content in ovary. The average daily gain (ADG) increased and the feed/gain decreased with the supplementation of HMSeBA during day 120 to 176 in gilts. However, Jlali et al. (15) and Chao et al. (9) did not find the dietary HMSeBA supplementation affect growth performance in growing pigs (a 32-d experiment) and weaned piglets (a 28-d experiment). This could be attributed to the long-term effect of HMSeBA on the gilt.
It also found that the long-term HMSeBA treatment increased the weight of uteri at the third estrus in gilts in this study. In addition, although the statistical difference was not significant, dietary supplementation with HMSeBA increased the average number of large follicles (≥ 5 mm, 17.6 vs. 13.2) and corpora lutea (33.0 vs. 23.2). Bone morphogenetic protein-15 (BMP-15) and growth differentiation factor-9 (GDF-9) are important oocyte-secreted factors which stimulate GCs proliferation and suppress progesterone production in the early stages of follicular development (29, 30). Porcine GDF-9 and BMP-15 genes were reported to be highly expressed in immature oocytes and declined with the oocyte maturation process (31, 32). In present study, dietary supplementation with HMSeBA decreased GDF-9 and BMP-15 gene expression compared with control group which might reflected the maturity of oocytes were much better than control group. Zhou et al. (33) also found that the down-regulated of GDF-9 and BMP-15 expression conducive to the improvement of oocyte quality by increased energy feeding in gilt (33).
In present study, consistent with previous study (12), we found that selenium did not increase FSH and LH concentration in serum at the third estrus of gilts. Fortier et al. (12) found that there's no difference of FSH concentration among the selenium treatments on the third estrus but the FSH concentration decreased with the estrus progress when dietary supplementation with 0.3 mg/kg Na2SeO3 and Se-enriched yeast (12). There is little known about the interaction between FSH concentration and Se status. The correlation between FSH and GPX has been observed in rats, and FSH was found to prevent oxidative stress-induced apoptosis of preovulatory follicles by stimulating follicular glutathione synthesis and inhibiting ROS production (34). In this study, HMSeBA supplementation increased the GPX activity in serum, liver and ovary, increased the T-AOC in liver and the TXNRD in ovary, decreased the MDA in serum, liver and ovary. For E2, there was no treatment effect in the serum and follicular fluid among treatments. However, there was a numerical improvement in follicular fluid in HMSeBA treatment (45.8 pg/ml in HMSeBA vs. 39.8 pg/ml in Na2SeO3 and 43.7 pg/ml in control). Fortier et al. (12) found that plasma E2 concentration peaked on d −1 of peri-ovulatory in gilts and rapid decline with the ovulation progress. They also found that the E2 concentration was less in sodium selenite treatment than in Se-enriched yeast and control (without supplemental Se) treatment on d −1 of peri-ovulatory and no difference among the treatments with other time of the peri-ovulatory. This response might be related to the different sampling time in vivo or the complexity of the in vivo test did not reflect the treatment effect. In our in vitro experiment, HMSeBA significantly increased the E2 concentration compared with control.
It is well known that maintaining homeostatis of redox system plays an important role female reproductive function. However, if oxygen free radicals are not eliminated in time, the redox state in the body will be out of balance, leading the body in a state of oxidative stress (35). Once oxidative stress occurs in granulose cell, it impairs its function. In turn, it affects ovulation of the ovary and the reproductive performance of gilt (9). Our in vitro experiment suggested that HMSeBA, a new organic selenium, played a regulatory role in GC proliferation and E2 synthesis. These findings are consistent with previous studies reporting that sodium selenite supplementation acts a regulatory role on E2 synthesis and the proliferation of bovine GCs (36) and goat luteinized granulosa cells (LGCs) (37) in vitro. However, the mechanism by which selenium regulates the proliferation of granulosa cells is not yet clear.
Cell proliferation is closely related to oxidative stress (38). Free radicals are continuously produced in aerobic cells. Therefore, we investigated the antioxidant status of GCs. Administration of HMSeBA to GCs increased total antioxidant capacity and activity of GPX in GCs, it was also accompanied by an increase in cell proliferation rate. The enzyme GR recycles oxidized glutathione (GSSG) by converting it to the reduced form (GSH) in an NADPH-dependent manner. In rat estrous cycle, the strongest expression of GR was observed in oocytes, followed by granulosa cells (39). GSH is known to increase gamete motility and reproductive efficiency, so it is speculated that GR plays a key role in reproduction as a source of GSH (39). In our in vitro study, HMSeBA (2.5 ng/ml) treatment increased the GR concentration, which was consistent with the results of gene expression. However, no difference in GR in the ovary at the transcription level and translation level in in vivo experiment. This may be due to HMSeBA regulates the expression of GR at the post-transcriptional level or too many cell types in the ovary mask the effect of HMSeBA on granulosa cells in vivo. However, this hypothesis requires more research to verify. The increasing of GR with the supplementation of HMSeBA indicated that the HMSeBA might be beneficial with GCs to increase oocyte viability and the fertility efficiency. In addition, this study found that HMSeBA (10 ng/ml) increased SOD2 mRNA expression in vitro, HMSeBA (5 ng/ml) and Na2SeO3 (5, 10 ng/ml) increased mRNA expression of GPX1 in vitro and HMSeBA group increased mRNA expression of GPX1 in the ovary in vivo. These results were agreed with previous researches, which found that Se might affect cell proliferation by regulating the activity of antioxidant enzymes in testis (40, 41).
SOD2 (Mn-SOD) is exclusively localized in the mitochondrial spaces, which been demonstrated that high expression in rodent steroidogenic (42, 43). Oxidative stress induced by SOD2-deficiency inhibits steroidogenesis in ovarian granulosa cell in mice, mainly by interfering with cholesterol transport to mitochondria and attenuating the expression of Star protein genes and key steroidogenic enzyme genes (44). Similarity to the results of this study, in our in vitro study, the SOD2 mRNA expression and E2 level both increased in the HMSeBA treatment at 10 ng/ml. Ceko et al. (22) found that in large healthy follicles only GPX1 was up-regulated in bovine and human granulosa cells (22). GPX1 is one of the most sensitive selenoproteins to change with selenium status (45). Mammals have highest levels of selenium and GPX1 in granulosa cells of large follicles (22, 46), which express higher levels of cytochrome P450s (cholesterol side-chain cleavage and aromatase) (47) to allow the large follicles to synthesize progesterone and estradiol. In these studies GPX1 expression was strongly associated with large follicles, suggesting that antioxidant may be involved in the signaling process that leading to dominance, or it may simply scavenge ROS, which would otherwise accelerate the atresia of competing follicles, or it may protect oocytes in maturing follicles from increasing levels of ROS associated with increasing steroidogenesis. In present study, GPX1 expression both increased in Na2SeO3 and HMSeBA in vitro, and in HMSeBA in the ovary in vivo, which further proof of the important role of selenium in reproduction.
Thioredoxin reductase (TXNRD) in mammalian is a member of the selenium-containing pyridine nucleotide-disulphide oxidoreductases family. TXNRD, Thioredoxin (TXN) and NADPH are the components of the TXN/TXNRD system, which have been considered key antioxidant system against oxidative stress (48, 49). Using 75Se, the Se incorporated into TXNRD has been reported to increase with increasing selenium concentration in cancer cell culture medium (50). The mRNA of TXNRD increased 2-5-fold at 1 microM selenium compared with that in the absence of selenium (51). The studies in rats also found that fed selenium deficiency diet decreased TXNRD activity in the liver, kidney and lung for several weeks (51, 52). In broiler chicks, it had been found that the activity of TXNRD in pectoral and thigh muscles (20–37%) was increased with the diet supplementation of HMSeBA compared with selenium-deficient treatment (53). Similar to previous study, in this study compared with control, although the difference was not significant at the transcription level, HMSeBA rather than Na2SeO3 significantly increases the activity of TXNRD at the protein level in the GCs in vitro and in the ovary in vivo. These results indicated that HMSeBA might have much more effects on the TXN/TXNRD system to help cells to defense against oxidative stress.
MDA is an oxidized lipid metabolite that can be used to reflect lipid peroxidation levels (54). In this study, Na2SeO3 and HMSeBA both decreased the concentration of MDA thus beneficial to the redox balance in vitro in granulosa cells and in the liver in vivo in gilts. At same time, HMSeBA decreased the concentration of MAD in the serum and ovary in vivo in gilts. Maintenance of redox balance is critical for effective immunity and health, and biomarkers of oxidative stress are linked to cellular function (55). Therefore, Na2SeO3 and HMSeBA both have the positive impact on the redox balance.
Conclusions
Dietary HMSeBA supplementation increased the selenium deposition, antioxidant capacities and promoted the development of COCs in gilts. HMSeBA promoted follicle development by stimulated GCs' proliferation, estradiol (E2) secretion and enhanced antioxidant capacities in vitro. Therefore, this study proved that HMSeBA promoted follicle development by antioxidant pathway from the in vivo and in vitro data. In future, long-term effect of HMSeBA supplementation on farrowing performance in gilt and exact mechanism of HMSeBA will be further explored.
Data Availability Statement
The original contributions presented in the study are included in the article/Supplementary Material, further inquiries can be directed to the corresponding authors.
Ethics Statement
The animal study was reviewed and approved by Guide for the Care and Use of Laboratory Animals prepared by the Animal Care and Use Committee of Sichuan Agricultural University.
Author Contributions
SX and DW designed and supervised the experiments. YD, ZL, SC, YLiu, and XJia conducted the experiments. SX, YD, ZL, XJiang, JL, YLin, LC, ZF, BF, YZ, JW, and ZR performed the data measurements, statistical data analysis, and supervision. SX, SC, and MB wrote and revised the manuscript. All authors have reviewed and approved the manuscript.
Funding
This study was supported by Adisseo France S.A.S, Sichuan Province 145 Breeding Tackle Project (Project No. 2021YFYZ0008).
Conflict of Interest
MK was employed by the company Adisseo France SAS.
The remaining authors declare that the research was conducted in the absence of any commercial or financial relationships that could be construed as a potential conflict of interest.
Publisher's Note
All claims expressed in this article are solely those of the authors and do not necessarily represent those of their affiliated organizations, or those of the publisher, the editors and the reviewers. Any product that may be evaluated in this article, or claim that may be made by its manufacturer, is not guaranteed or endorsed by the publisher.
Acknowledgments
We thank the 111 Project (D17015).
Supplementary Material
The Supplementary Material for this article can be found online at: https://www.frontiersin.org/articles/10.3389/fnut.2022.900789/full#supplementary-material
Supplementary Figure 1. Effects of dietary selenium supplementation on pubertal onset of gilt. (A) Age at puberty, (B) body weight at puberty, (C) backfat at puberty, (D) duration of estrus cycle. Data are expressed as means values and standard error, n = 5. CON, basal diet; Na2SeO3, 0.3 mg Se/kg Na2SeO3; HMSeBA, 0.3 mg Se/kg HMSeBA.
Supplementary Table 1. Composition and nutrient level of the basal diet (as-fed basis). 1Per kilogram of diet provided: 125 mg Fe; 14 mg Cu; 30 mg Mn; 110 mg Zn; 0.3 mg I. 2Per kilogram of diet provided: 120 mg Fe; 12 mg Cu; 30 mg Mn; 100 mg Zn; 0.28 mg I. 3Per kilogram of diet provided: 12000 IU VA; 2400 IU VD3; 100 IU VE; 4.8 mg VK3; 2 mg VB1; 7.2 mg VB2; 3.6 mg VB6; 0.025 mg VB12; 0.48 mg biotin; 25 mg pantothenic acid; 4 mg folic acid; 40 mg niacin. 4Per kilogram of diet provided: 7200 IU VA; 1440 IU VD3; 60 IU VE; 2.88 mg VK3; 1.2 mg VB1; 4.32 mg VB2; 2.16 mg VB6; 0.015 mg VB12; 0.288 mg biotin; 15 mg pantothenic acid; 2.4 mg folic acid; 30 mg niacin. 5Excepted the calculated values of digestible energy, the rest were all measured values. For the control, the three phases of the measured selenium concentration were 0.09, 0.06, and 0.04 mg/kg, the Na2SeO3 treatment of the three phases of the measured selenium concentration were 0.35, 0.33, and 0.31 mg/kg, the HMSeBA treatment of the three phases of the measured selenium concentration were 0.37, 0.41, and 0.41 mg/kg, respectively.
Supplementary Table 2. Primer sequences of the target genes. SELENOP: selenoprotein P; SELENOW: selenoprotein W; SELENOO: selenoprotein O; SELENOH: selenoprotein H; GPX: glutathione peroxidase; TXNRD: thioredoxin reductase; TXN1: Thioredoxin l; GR: Glutathione reductase; SOD: superoxide dismutase; CAT: catalase; SCLY: Selenocysteine lyase; SPS2: Selenophosphate synthetase 2; GDP-9: growth differentiation factor-9; BMP-15: bone morphogenetic protein-15; β-actin, beta-actin.
Supplementary Table 3. Effects of dietary selenium supplementation on growth performance in gilts. Data are expressed as means values and standard error, n = 5. Mean values with different superscript letters were significantly different (p < 0.05). Control, basal diet; Na2SeO3, 0.3 mg Se/kg Na2SeO3; HMSeBA, 0.3 mg Se/kg HMSeBA; ADG, average daily bodyweight gain; ADFI, average daily feed intake.
Supplementary Table 4. Effects of dietary selenium supplementation on the development of reproductive tracts and internal organs of gilts. Data are expressed as means values and standard error, n = 5. Control, basal diet; Na2SeO3, 0.3 mg Se/kg Na2SeO3; HMSeBA, 0.3 mg Se/kg HMSeBA.
Supplementary Table 5. Effects of dietary selenium supplementation on the hormone concentration in gilts. Data are expressed as means values and standard error, n = 5. Control, basal diet; Na2SeO3, 0.3 mg Se/kg Na2SeO3; HMSeBA, 0.3 mg Se/kg HMSeBA; LH, luteinizing hormone; FSH, follicle stimulating hormones; E2, Estradiol.
References
1. Craig J, Orisaka M, Wang H, Orisaka S, Thompson W, Zhu C, et al. Gonadotropin and intra-ovarian signals regulating follicle development and atresia: the delicate balance between life and death. Front Biosci. (2007) 12:3628–39. doi: 10.2741/2339
2. Schomberg DW, Couse JF, Mukherjee A, Lubahn DB, Sar M, Mayo KE, et al. Targeted disruption of the estrogen receptor-alpha gene in female mice: characterization of ovarian responses and phenotype in the adult. Endocrinology. (1999) 140:2733–44. doi: 10.1210/endo.140.6.6823
3. Simpson ER. Models of aromatase insufficiency. Semin Reprod Med. (2004) 22:25–30. doi: 10.1055/s-2004-823024
4. Agarwal A, Gupta S, Sharma RK. Role of oxidative stress in female reproduction. Reprod Biol Endocrinol. (2005) 3:28. doi: 10.1186/1477-7827-3-28
5. Dumont E, Vanhaecke F, Cornelis R. Selenium speciation from food source to metabolites: a critical review. Anal Bioanal Chem. (2006) 385:1304–23. doi: 10.1007/s00216-006-0529-8
6. Mistry HD, Pipkin FB, Redman CW, Poston L. Selenium in reproductive health. Am J Obstet Gynecol. (2012) 206:21–30. doi: 10.1016/j.ajog.2011.07.034
7. Shaniko S, Asad S, Wayne B. Selenium biochemistry and bioavailability: implications for animal agriculture. Agriculture. (2015) 5:1277–88. doi: 10.3390/agriculture5041277
8. Lyons MP, Papazyan TT, Surai PF. Selenium in food chain and animal nutrition: lessons from nature -review-. Asian-Australas J Anim Sci. (2007) 20:1135–55. doi: 10.5713/ajas.2007.1135
9. Chao Y, Yu B, He J, Huang Z, Mao X, Luo J, et al. Effects of different levels of dietary hydroxy-analogue of selenomethionine on growth performance, selenium deposition and antioxidant status of weaned piglets. Arch Anim Nutr. (2019) 73:374–83. doi: 10.1080/1745039X.2019.1641368
10. Dalto DB, Audet I, Lapointe J, Matte JJ. The importance of pyridoxine for the impact of the dietary selenium sources on redox balance, embryo development, and reproductive performance in gilts. J Trace Elem Med Biol. (2016) 34:79–89. doi: 10.1016/j.jtemb.2016.01.001
11. Dalto DB, Roy M, Audet I, Palin MF, Guay F, Lapointe J, et al. Interaction between vitamin B6 and source of selenium on the response of the selenium-dependent glutathione peroxidase system to oxidative stress induced by oestrus in pubertal pig. J Trace Elem Med Biol. (2015) 32:21–9. doi: 10.1016/j.jtemb.2015.05.002
12. Fortier ME, Audet I, Giguère A, Laforest JP, Bilodeau JF, Quesnel H, et al. Effect of dietary organic and inorganic selenium on antioxidant status, embryo development, and reproductive performance in hyperovulatory first-parity gilts. J Anim Sci. (2012) 90:231–40. doi: 10.2527/jas.2010-3340
13. Couloigner F, Jlali M, Briens M, Rouffineau F, Geraert PA, Mercier Y. Selenium deposition kinetics of different selenium sources in muscle and feathers of broilers. Poult Sci. (2015) 94:2708–14. doi: 10.3382/ps/pev282
14. Tufarelli V, Ceci E, Laudadio V. 2-Hydroxy-4-Methylselenobutanoic acid as new organic selenium dietary supplement to produce selenium-enriched eggs. Biol Trace Elem Res. (2016) 171:453–8. doi: 10.1007/s12011-015-0548-4
15. Jlali M, Briens M, Rouffineau F, Geraert PA, Mercier Y. Evaluation of the efficacy of 2-hydroxy-4-methylselenobutanoic acid on growth performance and tissue selenium retention in growing pigs. J Anim Sci. (2014) 92:182–8. doi: 10.2527/jas.2013-6783
16. Mou D, Ding D, Li S, Yan H, Qin B, Li Z, et al. Effect of maternal organic selenium supplementation during pregnancy on sow reproductive performance and long-term effect on their progeny. J Anim Sci. (2020) 98:skaa366. doi: 10.1093/jas/skaa366
17. Xu S, Linher-Melville K, Yang BB, Wu D, Li J. Micro-RNA378 (miR-378) regulates ovarian estradiol production by targeting aromatase. Endocrinology. (2011) 152:3941–51. doi: 10.1210/en.2011-1147
18. Li Z, Dong Y, Chen S, Jia X, Jiang X, Che L, et al. Organic selenium increased gilts antioxidant capacity, immune function, and changed intestinal microbiota. Front Microbiol. (2021) 12:723190. doi: 10.3389/fmicb.2021.723190
19. Mou D, Ding D, Yang M, Jiang X, Zhao L, Che L, et al. Maternal organic selenium supplementation during gestation improves the antioxidant capacity and reduces the inflammation level in the intestine of offspring through the NF-kappaB and ERK/Beclin-1 pathways. Food Funct. (2021) 12:315–27. doi: 10.1039/D0FO02274H
20. Livak KJ, Schmittgen TD. Analysis of relative gene expression data using real-time quantitative PCR and the 2 (-Delta Delta C (T)) method. Methods. (2001) 25:402–8. doi: 10.1006/meth.2001.1262
21. Briens M, Mercier Y, Rouffineau F, Vacchina V, Geraert PA. Comparative study of a new organic selenium source v. seleno-yeast and mineral selenium sources on muscle selenium enrichment and selenium digestibility in broiler chickens. Br J Nutr. (2013) 110:617–24. doi: 10.1017/S0007114512005545
22. Ceko MJ, Hummitzsch K, Hatzirodos N, Bonner WM, Aitken JB, Russell DL, et al. X-Ray fluorescence imaging and other analyses identify selenium and GPX1 as important in female reproductive function. Metallomics. (2015) 7:71–82. doi: 10.1039/C4MT00228H
23. Xu SY, Wu XL, Dong YP, Li ZM, Lin Y, Che LQ, et al. Glucose activates the primordial follicle through the AMPK/mTOR signaling pathway. Clin Transl Med. (2020) 10:122. doi: 10.1002/ctm2.122
24. Speight SM, Estienne MJ, Harper AF, Barb CR, Pringle TD. Effects of organic selenium supplementation on growth performance, carcass measurements, tissue selenium concentrations, characteristics of reproductive organs, and testis gene expression profiles in boars. J Anim Sci. (2012) 90:533–42. doi: 10.2527/jas.2010-3747
25. Davis PA, McDowell LR, Wilkinson NS, Buergelt CD, Van Alstyne R, Weldon RN, et al. Comparative effects of various dietary levels of Se as sodium selenite or Se yeast on blood, wool, and tissue Se concentrations of wether sheep. Small Rumin Res. (2008) 74:149–58. doi: 10.1016/j.smallrumres.2007.05.003
26. Juniper DT, Phipps RH, Ramos-Morales E, Bertin G. Effect of dietary supplementation with selenium-enriched yeast or sodium selenite on selenium tissue distribution and meat quality in beef cattle. J Anim Sci. (2008) 86:3100–9. doi: 10.2527/jas.2007-0595
27. Hu H, Wang M, Zhan X, Li X, Zhao R. Effect of different selenium sources on productive performance, serum and milk Se concentrations, and antioxidant status of sows. Biol Trace Elem Res. (2011) 142:471–80. doi: 10.1007/s12011-010-8803-1
28. Zhan X, Qie Y, Wang M, Li X, Zhao R. Selenomethionine: an Effective selenium source for sow to improve Se distribution, antioxidant status, and growth performance of pig offspring. Biol Trace Elem Res. (2011) 142:481–91. doi: 10.1007/s12011-010-8817-8
29. Shimizu T, Miyahayashi Y, Yokoo M, Hoshino Y, Sasada H, Sato E. Molecular cloning of porcine growth differentiation factor 9 (GDF-9) cDNA and its role in early folliculogenesis: direct ovarian injection of GDF-9 gene fragments promotes early folliculogenesis. Reproduction. (2004) 128:537–43. doi: 10.1530/rep.1.00224
30. Otsuka F, Yao ZX, Lee TH, Yamamoto S, Shimasaki S. Bone morphogenetic protein-15-Identification of target cells and biological functions. J Biol Chem. (2001) 275:39523–8. doi: 10.1074/jbc.M007428200
31. Li HK, Kuo TY, Yang HS, Chen LR, Li SL, Huang HW. Differential gene expression of bone morphogenetic protein 15 and growth differentiation factor 9 during in vitro maturation of porcine oocytes and early embryos. Anim Reprod Sci. (2008) 103:312–22. doi: 10.1016/j.anireprosci.2006.12.017
32. Prochazka R, Nemcova L, Nagyova E, Kanka J. Expression of growth differentiation factor 9 messenger RNA in porcine growing and preovulatory ovarian follicles. Biol Reprod. (2004) 71:1290–5. doi: 10.1095/biolreprod.104.027912
33. Zhou DS, Fang ZF, Wu D, Zhuo Y, Xu SY, Wang YZ, et al. Dietary energy source and feeding levels during the rearing period affect ovarian follicular development and oocyte maturation in gilts. Theriogenology. (2010) 74:202–11. doi: 10.1016/j.theriogenology.2010.02.002
34. Tsai-Turton M, Luderer U. Opposing effects of glutathione depletion and follicle-stimulating hormone on reactive oxygen species and apoptosis in cultured preovulatory rat follicles. Endocrinology. (2006) 147:1224–36. doi: 10.1210/en.2005-1281
35. Sohal RS, Allen RG. Oxidative stress as a causal factor in differentiation and aging: a unifying hypothesis. Exp Gerontol. (1990) 25:499–522. doi: 10.1016/0531-5565(90)90017-V
36. Basini G, Tamanini C. Selenium stimulates estradiol production in bovine granulosa cells: possible involvement of nitric oxide. Domest Anim Endocrin. (2000) 18:1–17. doi: 10.1016/S0739-7240(99)00059-4
37. Yao X, Ei-Samahy MA, Fan L, Zheng L, Jin Y, Pang J, et al. In vitro influence of selenium on the proliferation of and steroidogenesis in goat luteinized granulosa cells. Theriogenology. (2018) 114:70–80. doi: 10.1016/j.theriogenology.2018.03.014
38. Wang K, Fu XT, Li Y, Hou YJ, Yang MF, Sun JY, et al. Induction of S-Phase arrest in human glioma cells by selenocysteine, a natural selenium-containing agent via triggering reactive oxygen species-mediated DNA damage and modulating MAPKs and AKT pathways. Neurochem Res. (2016) 41:1439–47. doi: 10.1007/s11064-016-1854-8
39. Kaneko T, Iuchi Y, Kawachiya S, Fujii T, Saito H, Kurachi H, et al. Alteration of glutathione reductase expression in the female reproductive organs during the estrous cycle. Biol Reprod. (2001) 65:1410–6. doi: 10.1095/biolreprod65.5.1410
40. Shi L, Zhao H, Ren Y, Yao X, Song R, Yue W. Effects of different levels of dietary selenium on the proliferation of spermatogonial stem cells and antioxidant status in testis of roosters. Anim Reprod Sci. (2014) 149:266–72. doi: 10.1016/j.anireprosci.2014.07.011
41. Shi L, Song R, Yao X, Ren Y. Effects of selenium on the proliferation, apoptosis and testosterone production of sheep Leydig cells in vitro. Theriogenology. (2017) 93:24–32. doi: 10.1016/j.theriogenology.2017.01.022
42. Cao L, Leers-Sucheta S, Azhar S. Aging alters the functional expression of enzymatic and non-enzymatic anti-oxidant defense systems in testicular rat Leydig cells. J Steroid Biochem Mol Biol. (2004) 88:61–7. doi: 10.1016/j.jsbmb.2003.10.007
43. Matzuk MM, Dionne L, Guo Q, Kumar TR, Lebovitz RM. Ovarian function in superoxide dismutase 1 and 2 knockout mice. Endocrinology. (1998) 139:4008–11. doi: 10.1210/endo.139.9.6289
44. Zaidi SK, Shen WJ, Cortez Y, Bittner S, Bittner A, Arshad S, et al. SOD2 deficiency-induced oxidative stress attenuates steroidogenesis in mouse ovarian granulosa cells. Mol Cell Endocrinol. (2021) 519:110888. doi: 10.1016/j.mce.2020.110888
45. Sunde RA, Raines AM, Barnes KM, Evenson JK. Selenium status highly regulates selenoprotein mRNA levels for only a subset of the selenoproteins in the selenoproteome. Biosci Rep. (2009) 29:329–38. doi: 10.1042/BSR20080146
46. Mihm M, Baker PJ, Fleming LM, Monteiro AM, O'Shaughnessy PJ. Differentiation of the bovine dominant follicle from the cohort upregulates mRNA expression for new tissue development genes. Reproduction. (2008) 135:253–65. doi: 10.1530/REP-06-0193
47. Irving-Rodgers HF, Harland ML, Sullivan TR, Rodgers RJ. Studies of granulosa cell maturation in dominant and subordinate bovine follicles: novel extracellular matrix focimatrix is co-ordinately regulated with cholesterol side-chain cleavage CYP11A1. Reproduction. (2009) 137:825–34. doi: 10.1530/REP-08-0485
49. Lillig CH, Holmgren A. Thioredoxin and related molecules–from biology to health and disease. Antioxid Redox Signal. (2007) 9:25–47. doi: 10.1089/ars.2007.9.25
50. Gallegos A, Berggren M, Gasdaska JR, Powis G. Mechanisms of the regulation of thioredoxin reductase activity in cancer cells by the chemopreventive agent selenium. Cancer Res. (1997) 57:4965–70.
51. Hill KE, McCollum GW, Boeglin ME, Burk RF. Thioredoxin reductase activity is decreased by selenium deficiency. Biochem Biophys Res Commun. (1997) 234:293–5. doi: 10.1006/bbrc.1997.6618
52. Berggren MM, Mangin JF, Gasdaka JR, Powis G. Effect of selenium on rat thioredoxin reductase activity: increase by supranutritional selenium and decrease by selenium deficiency. Biochem Pharmacol. (1999) 57:187–93. doi: 10.1016/S0006-2952(98)00283-4
53. Zhao L, Sun LH, Huang JQ, Briens M, Qi DS, Xu SW, et al. A novel organic selenium compound exerts unique regulation of selenium speciation, selenogenome, and selenoproteins in broiler chicks. J Nutr. (2017) 147:789–97. doi: 10.3945/jn.116.247338
54. Del Rio D, Stewart AJ, Pellegrini N. A review of recent studies on malondialdehyde as toxic molecule and biological marker of oxidative stress. Nutr Metab Cardiovasc Dis. (2005) 15:316–28. doi: 10.1016/j.numecd.2005.05.003
Keywords: 2-hydroxy-4-methylselenobutanoic acid (HMSeBA), gilt, antioxidant capacity, follicle development, granulose cell
Citation: Xu S, Dong Y, Chen S, Liu Y, Li Z, Jia X, Briens M, Jiang X, Lin Y, Che L, Zhuo Y, Li J, Feng B, Fang Z, Wang J, Ren Z and Wu D (2022) 2-Hydroxy-4-Methylselenobutanoic Acid Promotes Follicle Development by Antioxidant Pathway. Front. Nutr. 9:900789. doi: 10.3389/fnut.2022.900789
Received: 21 March 2022; Accepted: 11 April 2022;
Published: 10 May 2022.
Edited by:
Jiaqiang Huang, China Agricultural University, ChinaReviewed by:
Baoming Shi, Northeast Agricultural University, ChinaXiudong Liao, Institute of Animal Sciences (CAAS), China
Copyright © 2022 Xu, Dong, Chen, Liu, Li, Jia, Briens, Jiang, Lin, Che, Zhuo, Li, Feng, Fang, Wang, Ren and Wu. This is an open-access article distributed under the terms of the Creative Commons Attribution License (CC BY). The use, distribution or reproduction in other forums is permitted, provided the original author(s) and the copyright owner(s) are credited and that the original publication in this journal is cited, in accordance with accepted academic practice. No use, distribution or reproduction is permitted which does not comply with these terms.
*Correspondence: Shengyu Xu, shengyuxu@sicau.edu.cn; De Wu, wude@sicau.edu.cn
†These authors have contributed equally to this work