- State Key Laboratory of Natural Medicines, School of Life Sciences and Technology, China Pharmaceutical University, Nanjing, China
The aim of this study was to investigate the antioxidative and neuroprotective activities of total flavonoids from sea buckthorn (Hippophae rhamnoides L.) (TFH). Results indicated that TFH possessed DPPH radicals, hydroxyl radicals and superoxide anions scavenging activities. The neuroprotective potential was assessed with acetylcholinesterase (AChE) and monoamine oxidase A (MAO-A). The inhibition rates of AChE and MAO-A by 50 μg/ml TFH were 75.85 and 51.22%, respectively. The in vivo antioxidative and neuroprotective potential of TFH were explored in Caenorhabditis elegans. In the longevity assay, TFH (50 μg/ml) significantly increased the lifespan of wild-type C. elegans (29.40%). In the hydrogen peroxide-induced oxidative stress challenge, the antioxidant capacity of TFH-treated wild-type C. elegans was significantly enhanced. The C. elegans mutant strain CL4176 was used to study the neuroprotective effect of TFH in vivo. Results showed that TFH significantly delayed paralysis in C. elegans CL4176. Our study suggested total flavonoids from sea buckthorn (Hippophae rhamnoides L.) had the potential as an antioxidative and neuroprotective agent to extend aging and treat neurodegenerative diseases.
Introduction
With the lifestyle changes and the aging of the population in modern society, diseases such as neurodegenerative disorders pose a serious threat to human health. Aging is a major risk factor for most neurodegenerative diseases, including Alzheimer’s disease (AD) and Parkinson’s disease (PD) (1). Biochemical changes in brain are responsible of age-related cognitive impairment and the development of these neurodegenerative diseases. Alzheimer’s disease is currently treated primarily with acetylcholinesterase (AChE) inhibitors, which can increase the concentration of acetylcholine in the brain, thus ameliorating the cognitive and mental decline associated with this neurodegenerative disease (2). Recent studies have also shown that Monoamine oxidase A (MAO-A) has the potential to play a role in the disease-modifying treatment of neurodegenerative diseases (3). In addition, one of the main causes of aging and age-related diseases is the production of reactive oxygen species (ROS) during the metabolic process (4, 5). Therefore, there is an increasing need to find compounds with antioxidant and neuroprotective effects to delay aging and prevent or treat aging-related diseases.
In the recent years, there has been a dramatic increase in usage of plant-derived foods in preventive diseases area. Polyphenolic compounds, especially flavonoids, are known as most common phytochemicals which possess a multiple range of biological effects. Sea buckthorn (Hippophae rhamnoides L.) is a medicinal and food plant which belongs to the Elaeagnaceae family and which has attracted much research attention because of its, rich in nutrients and bioactive substances (6). The chemical composition of sea buckthorn is mainly flavonoids and saponins, and it is also rich in vitamins, amino acids, trace elements and other nutrients which possess a variety of biological activities and health effects such as antioxidant, anti-tumor, hypoglycemic, improve blood lipid metabolism and other physiological effects (7).
The aim of this study was to investigate the antioxidative activity of total flavonoids from sea buckthorn (Hippophae rhamnoides L.) (TFH), and we also further evaluate its neuroprotective activity using Caenorhabditis elegans (C. elegans) as an in vivo model. These findings highlighted those total flavonoids from sea buckthorn (Hippophae rhamnoides L.) had the potential to delay aging and prevent or treat neurodegenerative diseases associated with aging.
Materials and Methods
Reagents and Chemicals
Total flavonoids from sea buckthorn (Hippophae rhamnoides L.) (TFH) was purchased from Nanjing Yuanzhi Biotechnology Co., Ltd. (Nanjing, China) which was extracted from the fruits. The main components of TFH is isorhamnetin, and the purity is more than 95%. The concentration of TFH solution [dissolved in Dimethyl sulfoxide (DMSO)] used in this study was 10, 20, and 50 μg/ml (8, 9). Other chemical regents used in this study were obtained from Sigma-Aldrich Merck (Sigma-Aldrich, St. Louis, MO, United States).
Caenorhabditis elegans Strains and Maintenance
The C. elegans strains used in this study were N2 Bristol (wild-type) and CL4176 [smg-1(CC546) I; dv] s27X. The wild-type strain was obtained from Caenorhabditis Genetics Center (University of Minnesota, United States). The mutant strain was kindly provided by Prof. Hongbing Wang (School of Life Sciences and Technology, Tongji University, Shanghai, China). The wild-type C. elegans strain was grown at 20°C (CL4176 at 16°C) on nematode growth medium (NGM) agar plates seeded with the E. coli strain OP50 under standard laboratory conditions (10).
In vitro Antioxidant Activity
DPPH Assay
DPPH-free radicals scavenging activity of the different concentrations of TFH was determined as described by Shi et al. (11). First, TFH solution (different concentrations) was filter-sterilized (0.22 μm). Then, 0.4 ml of TFH solution was mixed with 1.6 ml freshly prepared DPPH solution (0.2 mM in ethanol) and allowed to react for 25 min in dark. The control group contained the corresponding volume of DMSO and DPPH of TFH. Then, the absorbance was measured at 517 nm. The value of OD517 of experimental group and control group was recorded, respectively, as A1 and A0. The scavenging rate is expressed as follows:
Hydroxyl Radical Assay
The hydroxyl radical scavenging ability was determined according to the method by Yu et al. with some modifications (12). First, 1.4 ml of sodium salicylate (5 mM), FeSO4 (5 mM), and H2O2 (3 mM) were mixed with 100 μl of TFH solution or DMSO (control group contained the corresponding volume of DMSO of TFH). Then, the mixture was incubated at room temperature for 50 min. The absorbance of the solution was measured at 510 nm. The value of OD510 of experimental group and control group was recorded, respectively, as A1 and A0. The scavenging rate is expressed as follows:
Superoxide Anion Assay
The ability to scavenge superoxide anion was tested by the method as described by Shivangi et al. (13) with some modifications. The reaction mixture contained 1 ml TFH solution or DMSO (control group), 2.8 ml Tris–HCl buffer (0.05 M, pH 8.2) and 0.1 ml pyrogallic acid (0.05 M). Then the mixture was reacted for 4 min at room temperature in dark. 80 μl of 0.1 M HCl was added to stop the reaction and absorbance was measured at 325 nm. The value of OD325 of experimental group and control group was recorded, respectively, as A1 and A0. The scavenging rate is expressed as follows:
Neuroprotective Potential
Acetylcholinesterase Enzyme Inhibitory Activity
Inhibitory AChE activity was detected using commercial acetylcholinesterase assay kit (Nanjing Jiancheng Bioengineering Institute, Nanjing, China) according to the manufacturer’s instruction (14). All experiments were performed in triplicate.
Monoamine Oxidase A Enzyme Inhibitory Activity
The inhibition of MAO-A activity was assessed using commercial MAO monoamine oxidase assay kit (Nanjing Jiancheng Bioengineering Institute, Nanjing, China) according to the manufacturer’s instruction (15). All experiments were performed in triplicate.
Caenorhabditis elegans Assays
Lifespan Assay
This assay has been carried out following the method by Sugawara et al. (16) with a slight modification. First, 100 μl of fresh overnight culture of E. coli OP50 was, respectively, spread on NGM plates containing different concentrations of isorhamnetin (treatment group) or DMSO (control group). 5-fluorodeoxyuridine (final concentration of 70 mmol/l) was added to prevent nematodes from hatching. Synchronized wild-type C. elegans worms (L4 stage) were transferred to above NGM plates and cultured at 20°C. The number of live worms was counted every 24 h. A worm was considered dead when it failed to respond to gentle touch with a worm picker. The experiment was done in triplicate. The mean lifespan was estimated as follows:
Where j is the age (day), dj is the number of worms that died in the age interval (xj, xj+1), and N is the total number of worms.
Assessment of Resistance to Oxidative Stress
The antioxidant activity of TFH on C. elegans (N2) was assessed based on previous method with slight modifications (17). First, 100 μl of fresh overnight culture of E. coli OP50 was, respectively, spread on NGM plates containing different concentrations of TFH (treatment group) or DMSO (control group). 5-fluorodeoxyuridine (final concentration of 70 mmol/l) was added to prevent nematodes from hatching. The synchronized worms (L4 stage) were transferred to above plates and cultured at 20°C for 3 days. After that, each group of 20 worms were transferred to an NGM plate containing 50 mmol/l hydrogen peroxide. The dead worms were counted after 16 h. Experiments were done in triplicate.
Paralysis Assay
The C. elegans strain CL4176 is a mutant strain that contains a temperature-sensitive mutation. The mutant expresses human amyloid β (1-42). The Aβ (β-Amyloid) peptide is expressed and aggregates in muscle cells, resulting in paralysis in the mutants. CL4176 worms were egg-synchronized onto fresh NGM plates with different concentrations of TFH (treatment group) or DMSO (control group) at 16°C (18). When the worms grew to L3 larvae, the temperature was raised to 25°C to induce Aβ transgene expression. After 36 h (recorded as 0 h), paralysis was scored at 16 h. Each assay was done in triplicate.
Statistical Analysis
The experiments were repeated three times. Data analysis and mapping using GraphPad 8.3 software. Statistical differences between groups were determined using one-way ANOVA. The level of p < 0.05 was considered to be statistically significant, and the level of p < 0.01 was considered to be statistically extremely significant.
Results
Antioxidant Activities of Total Flavonoids From Sea Buckthorn (Hippophae rhamnoides L.)
DPPH-Free Radicals
Elimination of DPPH-free radicals is the base of the commonly used antioxidant assays (19). As shown in Figure 1A, all three concentrations of TFH had a certain scavenging activity against DPPH-free radicals. Moreover, the scavenging activity increased with TFH concentration. Among them, the scavenging activity was highest at a concentration of 50 μg/ml, with a scavenging rate of 83.59%.
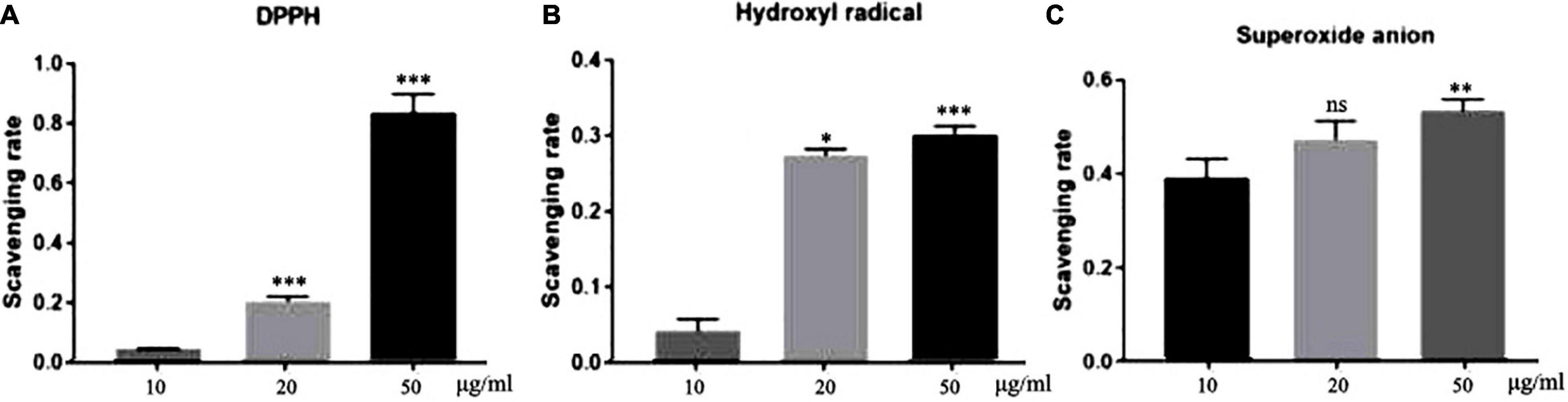
Figure 1. In vitro antioxidant potential of TFH. Results are represented as mean ± SEM of three independent replicates. ns, no significance; ***p < 0.001, **p < 0.01, *p < 0.05 vs. the 10 μg/ml group.
Hydroxyl Radicals
Hydroxyl radicals play an important role in the endogenous damage of DNA (20). As shown in Figure 1B, the scavenging rate of TFH was 30.07 and 27.45% at the concentrations of 50 μg/ml and 20 μg/ml, respectively. The scavenging rate was 4.27% at 10 μg/ml.
Superoxide Anions
The superoxide anion can induce oxidative stress and thus cause damage to biological molecules (21). As shown in Figure 1C, the highest clearance rate was 54.15% at a concentration of 50 μg/ml. The difference was that as the concentration decreased, the clearance rate appeared to decrease.
Potential Neuroprotective Effect of Total Flavonoids From Sea Buckthorn (Hippophae rhamnoides L.)
The in vitro neuroprotective potential of TFH was determined through the AChE and MAO-A inhibitory activity, which are associated with neurodegenerative disorders (22). Results are summarized in Figure 3. All concentrations of TFH showed inhibitory activity against both AChE (Figure 3A) and MAO-A (Figure 3B), among them, the strongest inhibitory activity of TFH was observed at 50 μg/ml. When the concentration of TFH was 50 μg/ml, the AChE and MAO-A inhibitory rate were 75.85 and 51.22%, respectively. Results showed that TFH had potential neuroprotective effect.
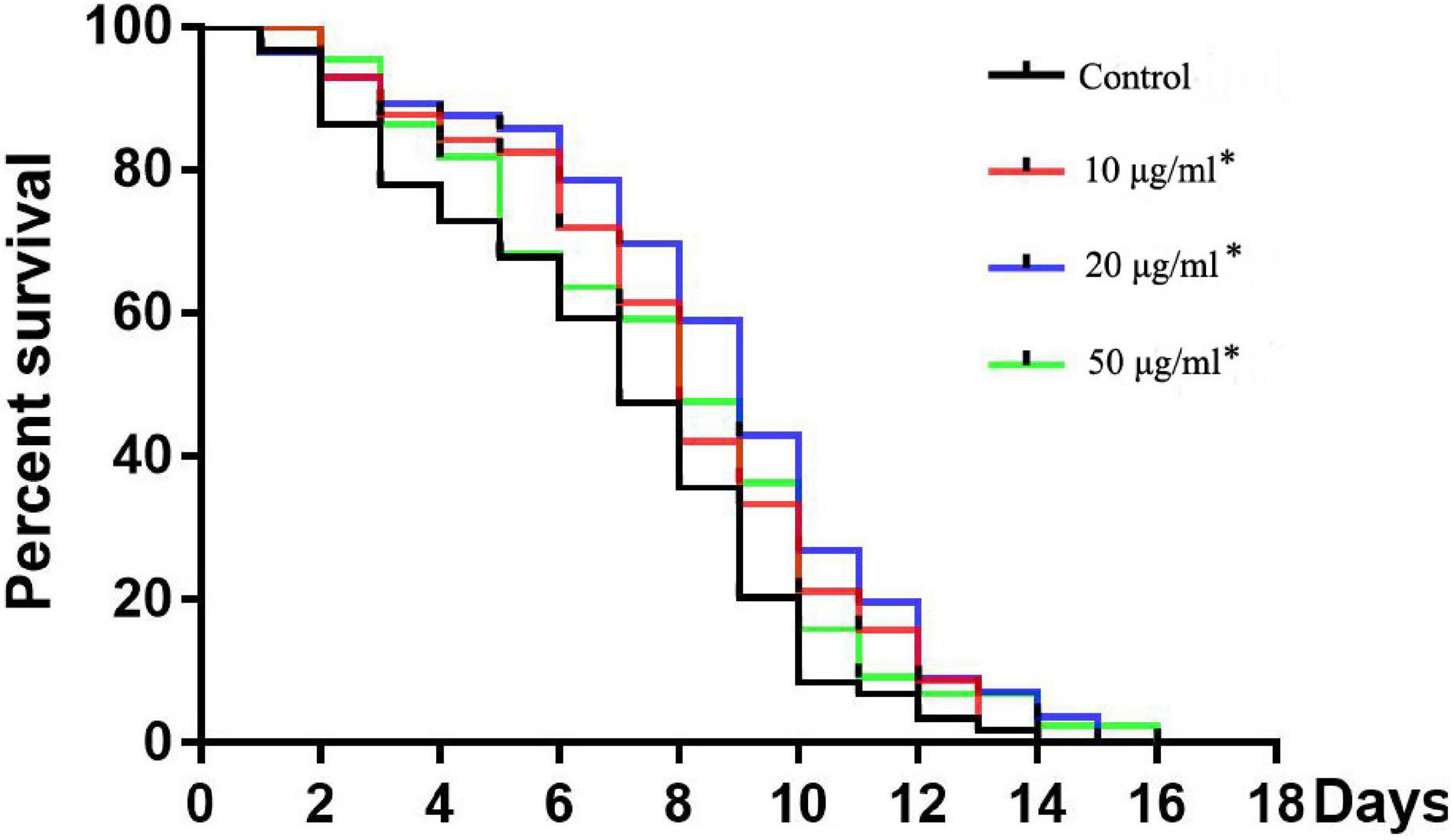
Figure 2. Effects of TFH on lifespan of C. elegans. Three replicates were used per treatment. Differences in survival curves between treatment and control groups were found in all TFH treated groups. Differences compared to control group were considered significant at *p ≤ 0.05.
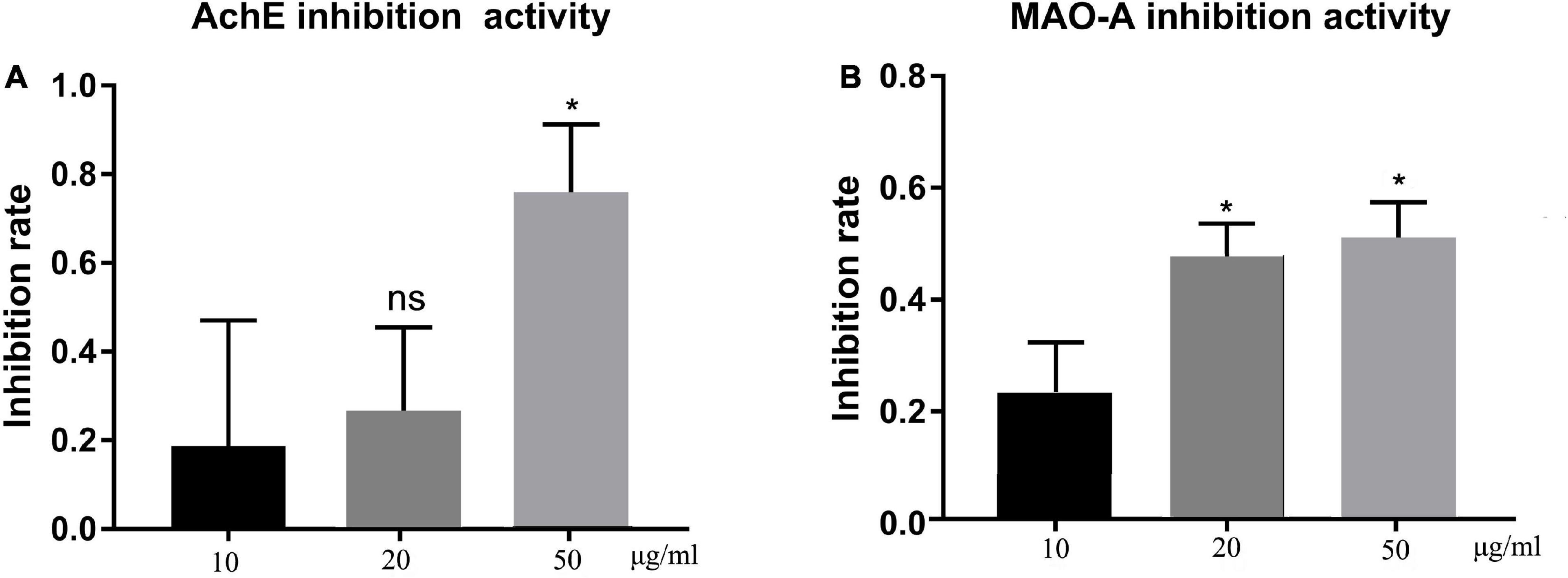
Figure 3. In vitro AChE and MAO-A inhibitory activity of TFH. Results are represented as mean ± SEM of three independent replicates. ns, no significance; *p < 0.05 vs. the 10 μg/ml group.
Total Flavonoids From Sea Buckthorn (Hippophae rhamnoides L.) Enhances the Lifespan of C. elegans
All concentrations of TFH significantly (p < 0.05) increased the mean lifespan (MLS) of C. elegans (N2) compared with the control group (shown in Figure 2 and Table 1). In addition, the survival rate of all TFH-treated groups was higher than that of the control group during the whole experiment. Among the three concentrations, TFH at a concentration of 50 μg/ml is the most effective, increasing the MLS by 29.40%, followed by TFH at a concentration of 20 μg/ml by 21.86%, and TFH at a concentration of 10 μg/ml by 18.55%. Therefore, TFH had a life-prolonging effect on C. elegans.
Total Flavonoids From Sea Buckthorn (Hippophae rhamnoides L.) Enhances the Oxidative Stress Tolerance of C. elegans
Oxidative stress is harmful to life processes and especially responsible for aging and age-related diseases (23). After exposure to hydrogen peroxide, which is a known source of active oxygen, the survival rates of TFH-treated worms were significantly (p < 0.05) different with control group (shown in Figure 4). Among them, TFH had a maximum effect at a concentration of 50 μg/ml, and the survival rate of worms can achieve 66.67%. Hence, TFH improved tolerance to oxidative stress in C. elegans.
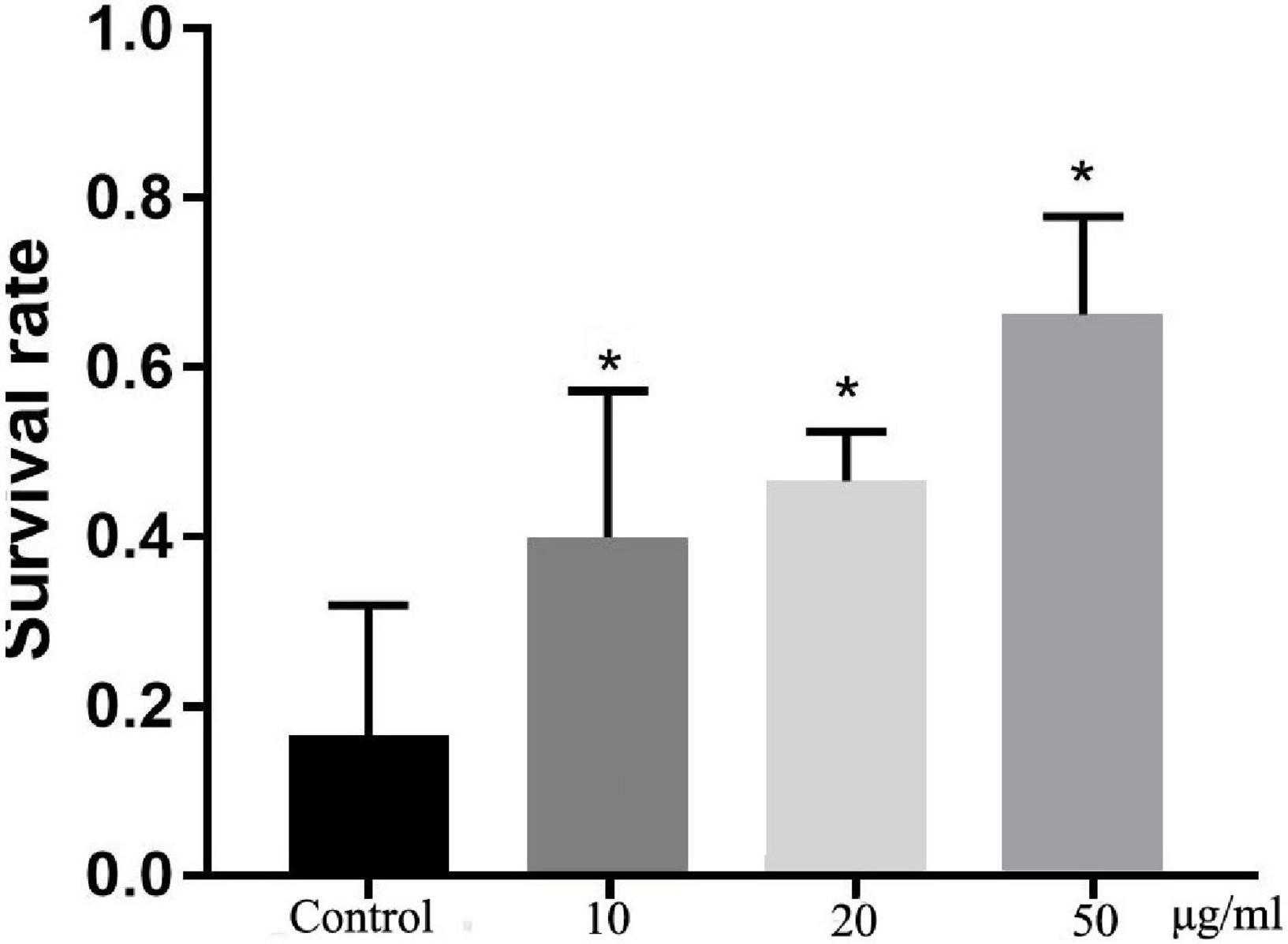
Figure 4. Effects of TFH on oxidative stress tolerance of C. elegans. Three replicates were used per treatment. Differences compared to control group were considered significant at *p ≤ 0.05.
Total Flavonoids From Sea Buckthorn (Hippophae rhamnoides L.) Delays the Paralysis of β-Amyloid Transgenic C. elegans
To detect the neuroprotective effect of TFH in vivo, we fed different concentrations of TFH to transgenic C. elegans CL4176 strain and compared the paralysis rate at 16 h. Compared with the control group, the paralysis rate of worms in TFH-treated group was significantly reduced (shown in Figure 5). Among them, TFH had a maximum effect at a concentration of 50 μg/ml, and the paralysis rate was 40.00%. Our results showed that T FH exhibited significant delay of Aβ-induced paralysis.
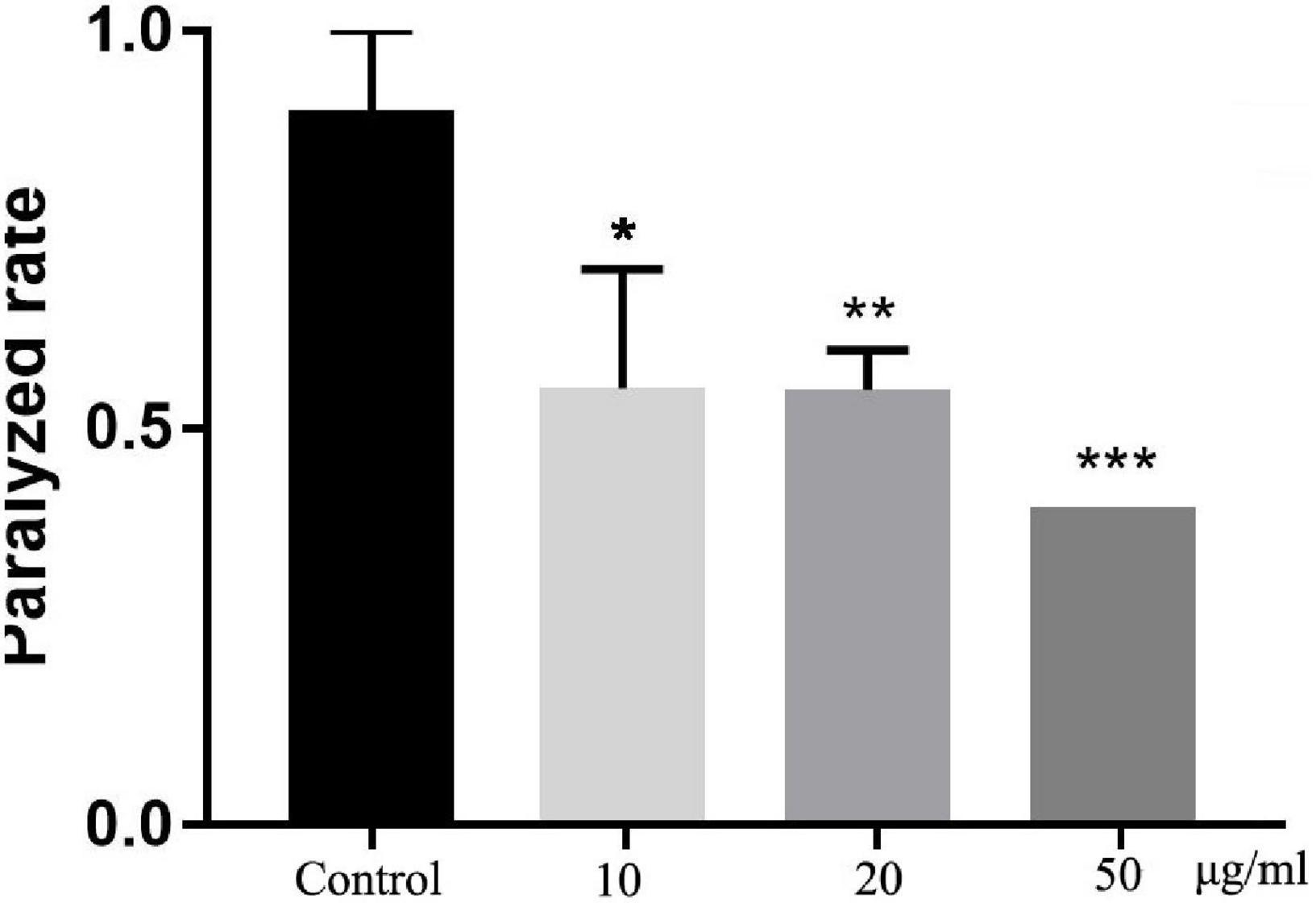
Figure 5. Effect of TFH on Aβ-induced paralysis in transgenic C. elegans CL4176. Three replicates were examined per treatment. Differences compared to control group were considered significant at *p ≤ 0.05, **p ≤ 0.01, and ***p ≤ 0.001.
Discussion
In recent years, more and more plant-derived drugs have been used to prevent and treat diseases. There are approximately 4,000 flavonoids in plants, many of which have a wide range of biological activities (24). Sea buckthorn has a high content of flavonoids, which are widely present in the fruits, seeds and leaves, mainly including isorhamnetin, quercetin, kaempferol, prunetin, rutin, catechin and other flavonoid glycosides and their glycosides (such as isorhamnetin-3-O-β-D-glucoside, quercetin-3-O-β-D-glucoside, and kaempferol-3-O-rutinoside-7-O-rhamnoside, etc.) (25). In the current study, we investigated the antioxidant activity and neuroprotective effects of total flavonoids from sea buckthorn (Hippophae rhamnoides L.) (TFH). In the in vitro antioxidant assay, three concentrations (10, 20, and 50 μg/ml) of TFH were shown to scavenge DPPH-free radicals, hydroxyl radicals and superoxide anions. The scavenging rate of DPPH radicals, hydroxyl radicals and superoxide anions tended to increase as the concentration of TFH increased. According to previous report, flavonoids exhibited antioxidant activity. Isorhamnetin, one of the main compounds from Sea buckthorn could scavenge DPPH-free radicals and ABTS radicals in vitro and exhibited antioxidant activity (26, 27). Quercetin, as a flavonoid, has also been reported to have free radical scavenging properties (28). Polyphenolic compounds extracted from three Romanian Lamiaceae plants, sage (Salvia officinalis), rosemary (Rosmarinus officinalis) and lavender (Lavandula angustifolia) showed antioxidant activity (29). Further, in our study, all concentrations of TFH inhibited acetylcholinesterase and monoamine oxidase A in vitro. The strongest inhibitory activity of TFH was observed at 50 μg/ml. Similarly, isorhamnetin has previously been reported to inhibit AChE (30). Other flavonoids have also been reported to possess this activity, for example, 15 flavonoids isolated from Eupatorium adenophorum have been reported to have inhibitory activity against AChE (31).
This study also used C. elegans as a model to investigate the activity of TFH in vivo. The results showed that TFH prolonged the lifespan of wild-type C. elegans. In particular, TFH at 50 μg/ml increased the lifespan by 29.40%. It had been reported that the flavonoids in peony petals had anti-aging and anti-oxidative stress properties, including prolonging the lifespan and providing antioxidant activity (32). Similarly, the rutin had been reported to increase lifespan in C. elegans (33). In addition, our results showed that TFH enhanced the tolerance of C. elegans to oxidative stress induced by hydrogen peroxide. It had been reported that flavonoids kaempferol and fisetin can increase the survival of C. elegans, diminish the extent of induced oxidative stress in C. elegans (34). It is also found that isorhamnetin enhances cellular antioxidant defense against H2O2-induced cytotoxicity in C2C12 cells (35). Isorhamnetin also had a potential protective effect against oxidative stress in human RPE cells (36). So, it is suggested that the antioxidant activity of TFH is based on isorhamnetin.
The present study showed that TFH significantly reduced the paralysis rate in transgenic C. elegans CL4176 strain. At the 16 h after induction of paralysis, the paralysis rate was 90% in the control group, whereas the paralysis rate in the TFH-treated group (50 μg/ml) were 40.00%. These results suggested that TFH had neuroprotective effects. Flavonoids are well-documented for neuroprotective and neurorestorative effects against various neurodegenerative diseases (37, 38). It has been reported that flavonoids from Good King Henry (Chenopodium bonus-henricus L.) showed statistically significant neuroprotective activities on isolated rat brain synaptosomes using 6-hydroxydopamine in vitro model (39). Likewise, Agathisflavone, a flavonoid derived from Poincianella pyramidalis (Tul.), enhances the neuroprotective properties of microglia and astrocytes to significantly ameliorate glutamate-mediated neurotoxicity (40). However, the molecular mechanism and which compounds in TFH exerts the antioxidant and neuroprotective effects need to be further explored.
Conclusion
In conclusion, the present study demonstrated that total flavonoids from sea buckthorn (Hippophae rhamnoides L.) had antioxidant activity and potential neuroprotective effects in vitro and in vivo. Furthermore, C. elegans can be used in aging and aging-related neurodegenerative disease studies. This study supported the potential application of total flavonoids from sea buckthorn (Hippophae rhamnoides L.) as an antioxidative and neuroprotective agent to delay aging and treat aging-related diseases in humans.
Data Availability Statement
The original contributions presented in the study are included in the article/supplementary material, further inquiries can be directed to the corresponding authors.
Author Contributions
WW: investigation, visualization, and writing – original draft. ZW and CZ: investigation, visualization, and methodology. WC and XG: supervision and writing – review and editing. All authors contributed to the article and approved the submitted version.
Funding
The study presented in the manuscript was funded by the Priority Academic Program Development of Jiangsu Higher Education Institutions (PAPD) and Innovation and Entrepreneurship Training Program for Undergraduates of China Pharmaceutical University (202010316191).
Conflict of Interest
The authors declare that the research was conducted in the absence of any commercial or financial relationships that could be construed as a potential conflict of interest.
Publisher’s Note
All claims expressed in this article are solely those of the authors and do not necessarily represent those of their affiliated organizations, or those of the publisher, the editors and the reviewers. Any product that may be evaluated in this article, or claim that may be made by its manufacturer, is not guaranteed or endorsed by the publisher.
References
1. Hou Y, Dan X, Babbar M, Wei Y, Hasselbalch SG, Croteau DL, et al. Ageing as a risk factor for neurodegenerative disease. Nat Rev Neurol. (2019) 15:565–81. doi: 10.1038/s41582-019-0244-7
2. Moss DE. Improving anti-neurodegenerative benefits of acetylcholinesterase inhibitors in Alzheimer’s disease: are irreversible inhibitors the future? Int J Mol Sci. (2020) 21:3438. doi: 10.3390/ijms21103438
3. Naoi M, Maruyama W, Inaba-Hasegawa K. Type A and B monoamine oxidase in age-related neurodegenerative disorders: their distinct roles in neuronal death and survival. Curr Top Med Chem. (2012) 12:2177–88. doi: 10.2174/156802612805219950
5. Johannesson T, Kristinsson J, Snadal J. Neurodegenerative diseases, antioxidative enzymes and copper. A review of experimental research. Laeknabladid. (2003) 89:659–71.
6. Raudonis R, Raudone L, Janulis V, Viskelis P. Flavonoids in cultivated berries of sea buckthorn (Hippophaë rhamnoides L.). Planta Med. (2014) 80:L24.
7. Lü Z, Yuan W, Bolin Z, Xing G. A review on mass distribution of active components from Hippophae rhamnoides fruits. J Beijing For Univ. (2021) 43:144–52.
8. Choi HI, Lee DH, Park SH, Jang YJ, Ahn J, Ha TY, et al. Antiobesity effects of the combination of Patrinia scabiosaefolia root and Hippophae rhamnoides leaf extracts. J Food Biochem. (2020) 44:e13214. doi: 10.1111/jfbc.13214
9. Stochmal A, Skalski B, Pietukhov R, Sadowska B, Rywaniak J, Wójcik-Bojek U, et al. Evaluation of antimicrobial, antioxidant, and cytotoxic activity of phenolic preparations of diverse composition, obtained from Elaeagnus rhamnoides (L.) A. Nelson leaf and twig extracts. Molecules. (2021) 26:2835. doi: 10.3390/molecules26102835
10. Stiernagle T. Maintenance of C. elegans. In: The C. elegans Research Community editor. WormBook. Pasadena, CA: WormBook (2006). p. 1–11. doi: 10.1895/wormbook.1.101.1
11. Shi Y, Cui X, Gu S, Yan X, Li R, Xia S, et al. Antioxidative and probiotic activities of lactic acid bacteria isolated from traditional artisanal milk cheese from northeast China. Probiotics Antimicrob Proteins. (2019) 11:1086–99. doi: 10.1007/s12602-018-9452-5
12. Yu HS, Jang HJ, Lee NK, Paik HD. Evaluation of the probiotic characteristics and prophylactic potential of Weissella cibaria strains isolated from Kimchi. LWT Food Sci Technol. (2019) 112:108229. doi: 10.1016/j.lwt.2019.05.127
13. Shivangi S, Devi PB, Ragul K, Shetty PH. Probiotic potential of Bacillus strains isolated from an acidic fermented food Idli. Probiotics Antimicrob Proteins. (2020) 12:1502–13. doi: 10.1007/s12602-020-09650-x
14. Moliner C, Barros L, Dias MI, Lopez V, Langa E, Ferreira ICFR, et al. Edible flowers of Tagetes erecta L. as functional ingredients: phenolic composition, antioxidant and protective effects on Caenorhabditis elegans. Nutrients. (2018) 10:2002. doi: 10.3390/nu10122002
15. Stafford GI, Pedersen PD, Jager AK, Van Staden J. Monoamine oxidase inhibition by Southern African traditional medicinal plants. S Afr J Bot. (2007) 73:384–90. doi: 10.1016/j.sajb.2007.03.001
16. Sugawara T, Sakamoto K. Killed Bifidobacterium longum enhanced stress tolerance and prolonged life span of Caenorhabditis elegans via DAF-16. Br J Nutr. (2018) 120:872–80. doi: 10.1017/s0007114518001563
17. Martorell P, Llopis S, Gonzalez N, Chenoll E, Lopez-Carreras N, Aleixandre A, et al. Probiotic strain Bifidobacterium animalis subsp lactis CECT 8145 reduces fat content and modulates lipid metabolism and antioxidant response in Caenorhabditis elegans. J Agric Food Chem. (2016) 64:3462–72. doi: 10.1021/acs.jafc.5b05934
18. Yang ZZ, Yu YT, Lin HR, Liao DC, Cui XH, Wang HB. Lonicera japonica extends lifespan and healthspan in Caenorhabditis elegans. Free Radic Biol Med. (2018) 129:310–22. doi: 10.1016/j.freeradbiomed.2018.09.035
19. Sharma OP, Bhat TK. DPPH antioxidant assay revisited. Food Chem. (2009) 113:1202–5. doi: 10.1016/j.foodchem.2008.08.008
20. Fleming AM, Burrows CJ. On the irrelevancy of hydroxyl radical to DNA damage from oxidative stress and implications for epigenetics. Chem Soc Rev. (2020) 49:6524–8. doi: 10.1039/d0cs00579g
21. Liu HL, Zhang DL, Zhao BL, Zhao JY. Superoxide anion, the main species of ROS in the development of ARDS induced by oleic acid. Free Radic Res. (2004) 38:1281–7. doi: 10.1080/10715760400006940
22. Oboh G, Ogunruku OO, Oyeleye SI, Olasehinde TA, Ademosun AO, Boligon AA. Phenolic extracts from Clerodendrum volubile leaves inhibit cholinergic and monoaminergic enzymes relevant to the management of some neurodegenerative diseases. J Diet Suppl. (2017) 14:358–71. doi: 10.1080/19390211.2016.1237401
23. Kim SY, Park SC. Physiological antioxidative network of the blirubin system in aging and age-related diseases. Front Pharmacol. (2012) 3:45. doi: 10.3389/fphar.2012.00045
24. Marcarini JC, Ferreira Tsuboy MS, Luiz RC, Ribeiro LR, Hoffmann-Campo CB, Mantovani MS. Investigation of cytotoxic, apoptosis-inducing, genotoxic and protective effects of the flavonoid rutin in HTC hepatic cells. Exp Toxicol Pathol. (2011) 63:459–65. doi: 10.1016/j.etp.2010.03.005
25. Ciesarová Z, Murkovic M, Cejpek K, Kreps F, Tobolková B, Koplík R, et al. Why is sea buckthorn (Hippophae rhamnoides L.) so exceptional? A review. Food Res Int. (2020) 133:109170. doi: 10.1016/j.foodres.2020.109170
26. Jin NZ, Qi YJ, Xiang CJ, Zhe JW, Yang BC. Study on antioxidant activity of isorhamnetin and isorhamnetin-3-O-glucoside. Chin J Bioinform. (2017) 15:112–9.
27. Bakır T, Sönmezoğlu I, Imer F, Apak R. Antioxidant/prooxidant effects of α-tocopherol, quercetin and isorhamnetin on linoleic acid peroxidation induced by Cu(II) and H2O2. Int J Food Sci Nutr. (2013) 65:226–34. doi: 10.3109/09637486.2013.845654
28. Sestili P, Guidarelli A, Dacha M, Cantoni O. Quercetin prevents DNA single strand breakage and cytotoxicity caused by tert-butylhydroperoxide: free radical scavenging versus iron chelating mechanism. Free Radic Biol Med. (1998) 25:196–200. doi: 10.1016/s0891-5849(98)00040-9
29. Gaspar-Pintiliescu A, Mihai E, Ciucan T, Popescu AF, Luntraru C, Tomescu J, et al. Antioxidant and acetylcholinesterase inhibition capacity of hyrosols from lamiaceae plants for biopesticide use: role of phenolics. Int J Food Prop. (2022) 25:996–1008.
30. Olennikov DN, Kashchenko NI, Chirikova NK, Akobirshoeva A, Zilfikarov IN, Vennos C. Isorhamnetin and quercetin derivatives as anti-acetylcholinesterase principles of marigold (Calendula officinalis) flowers and preparations. Int J Mol Sci. (2017) 18:1685. doi: 10.3390/ijms18081685
31. Li M, Gao X, Lan M, Liao X, Su F, Fan L, et al. Inhibitory activities of flavonoids from Eupatorium adenophorum against acetylcholinesterase. Pestic Biochem Physiol. (2020) 170:104701. doi: 10.1016/j.pestbp.2020.104701
32. Wang S, Xue J, Zhang S, Zheng S, Xue Y, Xu D, et al. Composition of peony petal fatty acids and flavonoids and their effect on Caenorhabditis elegans lifespan. Plant Physiol Biochem. (2020) 155:1–12. doi: 10.1016/j.plaphy.2020.06.029
33. Cordeiro LM, Machado ML, da Silva AF, Obetine Baptista FB, da Silveira TL, Antunes Soares FA, et al. Rutin protects Huntington’s disease through the insulin/IGF1 (IIS) signaling pathway and autophagy activity: study in Caenorhabditis elegans model. Food Chem Toxicol. (2020) 141:111323. doi: 10.1016/j.fct.2020.111323
34. Kampktter A, Nkwonkam CG, Zurawski RF, Timpel C, Chovolou Y, Wätjen W, et al. Effects of the flavonoids kaempferol and fisetin on thermotolerance, oxidative stress and FoxO transcription factor DAF-16 in the model organism Caenorhabditis elegans. Arch Toxicol. (2007) 81:849–58. doi: 10.1007/s00204-007-0215-4
35. Choi YH. The cytoprotective effect of isorhamnetin against oxidative stress is mediated by the upregulation of the Nrf2-dependent HO-1 expression in C2C12 myoblasts through scavenging reactive oxygen species and ERK inactivation. Gen Physiol Biophys. (2016) 35:145–54. doi: 10.4149/gpb_2015034
36. Wang J, Gong HM, Zou HH, Liang L, Wu XY. Isorhamnetin prevents H2O2-induced oxidative stress in human retinal pigment epithelial cells. Mol Med Rep. (2017) 17:648–52. doi: 10.3892/mmr.2017.7916
37. Zhao J, Zhu M, Kumar M, Fung NY, Li Y, Lao L, et al. A pharmacological appraisal of neuroprotective and neurorestorative flavonoids against neurodegenerative diseases. CNS Neurol Disord Drug Targets. (2019) 18:103–14. doi: 10.2174/1871527317666181105093834
38. Hwang SL, Shih PH, Yen GC. Neuroprotective effects of citrus flavonoids. J Agric Food Chem. (2012) 60:877–85.
39. Kokanova-Nedialkova Z, Kondeva-Burdina M, Nedialkov PT. Neuroprotective, anti- α -glucosidase and prolipase active flavonoids from Good King Henry (Chenopodium bonus-henricus L.). Nat Prod Res. (2020) 35:5484–8. doi: 10.1080/14786419.2020.1784172
40. Dos Santos Souza C, Grangeiro MS, Lima Pereira EP, Dos Santos CC, da Silva AB, Sampaio GP, et al. Agathisflavone, a flavonoid derived from Poincianella pyramidalis (Tul.), enhances neuronal population and protects against glutamate excitotoxicity. Neurotoxicology. (2018) 65:85–97. doi: 10.1016/j.neuro.2018.02.001
Keywords: Hippophae rhamnoides L., flavonoids, antioxidative activity, neuroprotective activity, C. elegans
Citation: Wang Z, Wang W, Zhu C, Gao X and Chu W (2022) Evaluation of Antioxidative and Neuroprotective Activities of Total Flavonoids From Sea Buckthorn (Hippophae rhamnoides L.). Front. Nutr. 9:861097. doi: 10.3389/fnut.2022.861097
Received: 24 January 2022; Accepted: 06 June 2022;
Published: 21 June 2022.
Edited by:
Ali Imran, Government College University Faisalabad, PakistanReviewed by:
Carlos L. Cespedes-Acuña, University of Bío-Bío, ChileShuwen Guan, Jilin University, China
Copyright © 2022 Wang, Wang, Zhu, Gao and Chu. This is an open-access article distributed under the terms of the Creative Commons Attribution License (CC BY). The use, distribution or reproduction in other forums is permitted, provided the original author(s) and the copyright owner(s) are credited and that the original publication in this journal is cited, in accordance with accepted academic practice. No use, distribution or reproduction is permitted which does not comply with these terms.
*Correspondence: Xiangdong Gao, xdgao@cpu.edu.cn; Weihua Chu, chuweihua@cpu.edu.cn
†These authors have contributed equally to this work and share first authorship