- 1State Key Laboratory of Animal Nutrition, Institute of Animal Sciences, Chinese Academy of Agricultural Sciences, Beijing, China
- 2Precision Livestock and Nutrition Unit, Gembloux Agro-Bio Tech, University of Liège, Gembloux, Belgium
- 3YangXiang Joint Stock Company, Guigang, China
- 4Hangzhou Viablife Biotech Co., Ltd., Hangzhou, China
Semen quality is one of the most important factors for the success of artificial insemination which has been widely applied in swine industry to take the advantages of the superior genetic background and higher fertility capability of boars. Hydroxytyrosol (HT), a polyphenol, has attracted broad interest due to its strong antioxidant, anti-inflammatory, and antibacterial activities. Sperm plasma membrane contains a large proportion of polyunsaturated fatty acids which is easily impaired by oxidative stress and thus to diminish semen quality. In current investigation, we aimed to explore the effects of dietary supplementation of HT on boar semen quality and the underlying mechanisms. Dietary supplementation of HT tended to increase sperm motility and semen volume/ejaculation. And the follow-up 2 months (without HT, just basal diet), the semen volume was significantly more while the abnormal sperm was less in HT group than that in control group. HT increased the “beneficial microbes” Bifidobacterium, Lactobacillus, Eubacterium, Intestinimonas, Coprococcus, and Butyricicoccus, however, decreased the relative abundance of “harmful microbes” Streptococcus, Oscillibacter, Clostridium_sensu_stricto, Escherichia, Phascolarctobacterium, and Barnesiella. Furthermore, HT increased plamsa steroid hormones such as testosterone and its derivatives, and antioxidant molecules while decreased bile acids and the derivatives. All the data suggest that HT improves gut microbiota to benefit plasma metabolites then to enhance spermatogenesis and semen quality. HT may be used as dietary additive to enhance boar semen quality in swine industry.
Introduction
Artificial insemination (AI) has been extensively used in swine industry in order to take the advantages of the superior genetic background and higher fertility capability of boars and sows (1–4). The application of AI is restricted to the semen quality, which can be influenced by multiple factors including the boar itself (e.g., age) and the environment factors (e.g., temperature and light) (1–4). Sperm plasma membrane contains a large proportion of polyunsaturated fatty acids (PUFA), which is easily impaired by oxidative stress and thus to diminish semen quality (1, 2). Increasing evidence suggests the potential to use nutritional interventions to enhance antioxidant capacity for improving boar semen quality (3, 4). In vitro studies found that supplementation with exogenous antioxidants, such as rosmarinic acid, polysaccharide, and skim milk could improve boar sperm quality by alleviating oxidative stress during the cryopreservation (5–7). Moreover, a lot of evidence from in vivo studies also illustrated that dietary supplementation with antioxidants improved the boar semen quality. For example, dietary supplementation of L-arginine and lysine improved boar semen quality by increasing antioxidant capacity (3, 4).
Hydroxytyrosol (HT), a polyphenol, is extracted from olive leaves and oil. HT has attracted broad interest due to its strong antioxidant, anti-inflammatory, and antibacterial activities (8, 9). HT exhibited antioxidant capacity by scavenging oxidant chemical species and promoting the expressions of antioxidant enzymes via activating nuclear factor E2-related factor 2 (Nrf2) signaling (8). Furthermore, Wei et al. reported that HT increased bacterial membrane permeabilization and then interacted with DNA, which collectively inhibited bacterial growth (10). Our recent study also showed that olive fruit extracts enriching HT exhibited strong antioxidant effects by benefiting gut microbiota in mice (11). Moreover, our previous study found that improved gut microbiota was able to ameliorate sperm quality by modulating the plasma metabolomes and small intestinal function in mice (12, 13). To search the effective strategies to improve sperm quality and enhance the production of swine production, current investigation was designed to explore the effects of dietary supplementation of HT on the boar semen quality and the underlying mechanisms.
Materials and Methods
Boars and Experimental Design
All animal procedures were approved by the Animal Care and Use Committee of the Institute of Animal Sciences of Chinese Academy of Agricultural Sciences (IAS2021-67). A total of 20 healthy boars (Duroc) aged from 31 to 33 months were selected in this study at the artificial insemination center of Yangxiang Joint Stock Company (Guangxi, China) (1). Boar feeding conditions have been previously reported (2). All boars were randomly divided into 2 groups (n = 10 per group). Boars in the control group (Con) were fed with a commercially prepared corn and soybean meal-based diet (Table 1), and boars in the HT supplement group (HT) were fed with a basal diet supplemented with 20 mg/kg body weight of HT. The dose of HT was adopted according to our previous mice experiment (11). The boars received two meals at 11:00 and 17:00 and the total feed intake amounts were 2.5 kg (1.25 kg per meal) every day. HT was mixed with the diet when the boars received the first meal and HT was taken almost completely by the boars each day. HT (purity > 99%) was kindly donated by Viablife Biotech Co., Ltd. (Hangzhou, China). The boars were housed in individual crates and HT was supplied for 60 days (Figure 1A).
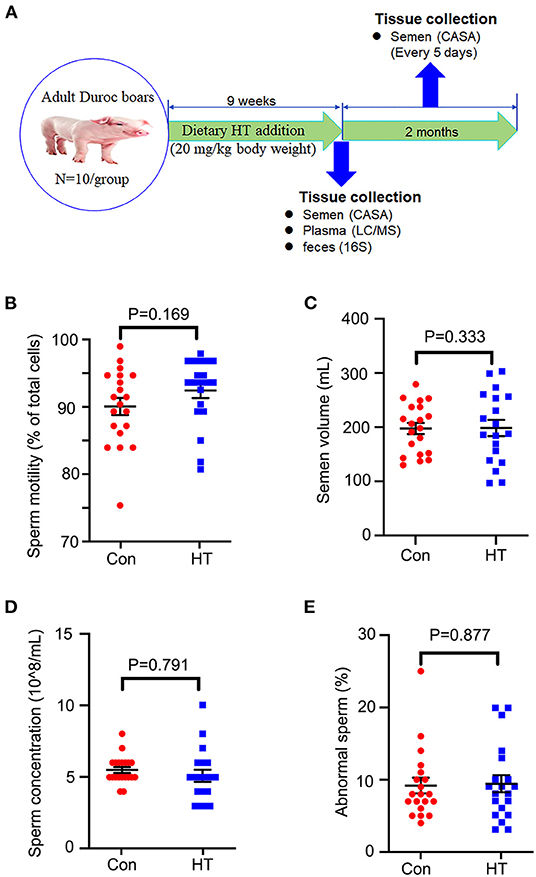
Figure 1. Effects of HT on the semen quality. (A) Study design. (B) Sperm motility. (C) Semen volume. (D) Sperm concentration. (E) Abnormal sperm. Data were expressed as the mean ± SEM.
Semen samples were collected by gloved-hand techniques. After collection, four semen parameters were assessed: semen volume, sperm concentration, sperm motility, and abnormal sperm rate, according to the reported methods (1, 14). Blood samples were collected into ethylenediamine tetraacetic acid (EDTA) plasma tubes by venipuncture from the hindlimb vein of boars during ejaculations. Each blood sample was then centrifuged at 3,000 × g for 10 min at 4°C to obtain a plasma sample and subsequently stored at −80°C until analysis. Each boar's rectum was massaged to stimulate defecation, and then fresh feces were collected and stored at −80°C for subsequent microbiota analysis (1).
The follow-up long term beneficial effects of HT on boar semen quality were determined. After HT supplementation, all the boars were fed with basal diet (without HT supplementation). The semen was collected every 5 days and the semen quality was analyzed. The long-term analysis was for 2 months (Figure 1A).
Evaluation of Spermatozoa Motility Using a Computer-Assisted Sperm Analysis System
Spermatozoa motility and concentration, and abnormal sperm rate were determined by the computer-assisted sperm assay (CASA) method according to World Health Organization guidelines and our previous reports (ML-210JZ, Nanning SongJing TianLun Biological Technology Co., LTD, Nanning, China) (15–18).
Morphological Observations of Spermatozoa
Boar sperm was stained with Eosin Y (1%) (15–17). Spermatozoa abnormalities were then viewed using an optical microscope and were classified into head or tail morphological abnormalities: two heads, two tails, blunt hooks, and short tails. The examinations were repeated three times, and 500 spermatozoa per animal were scored.
Boar Feces Microbiota Sequencing
Total genome DNA from feces was extracted using E.Z.N.A.R Stool DNA Kit (Omega Biotek Inc, USA). The V3-V4 region of the bacterial 16S rRNA gene was amplified using a specific primer (338F, 5'-ACTCCTACGGGAGGCAGCAG-3'; 806R, 5'-GGACTACHVGGGTWTCTAAT-3'). Then the library was sequenced on the Illumina HiSeq 2500 platform and 300 bp paired-end reads were generated at the Novo gene. The sequences were analyzed and assigned to operational taxonomic units (OTUs; 97% identity). OUT abundance information was normalized using a standard of sequence number corresponding to the sample with the least sequences (12, 13).
Plasma Metabolites Determined by LC-MS/MS
The metabolites were detected as reported in our early study (19). Briefly, boar plasma was collected and maintained at −80°C. LC-MS/MS analysis with ACQUITY UPLC and AB Sciex Triple TOF 5600 (LC/MS) was applied (19). Before LC-MS/MS analysis, the serum samples (100 μl) were precipitated with 10 μl methanol (0.3 mg/ml L-2-Chlorophe) on ice and processed to remove proteins. All samples were then centrifuged at 13,000 g for 10 min at 4°C and the supernatants were injected onto a ACQUITY UPLC HSS T3 column, which was at a flow rate of 0.35 ml/min with 2 μl mobile phase. MS analyses were conducted using electrospray ionization in the positive and negative ion models. Using full scan analysis. Progenesis QI v. 2.3 (Nonlinear Dynamics, Newcastle, UK) was applied to normalize the peaks. Human Metabolome Database (HMDB), Lipidmaps (v. 2.3), and METLIN software were applied to qualify the data. Furthermore, the data were analyzed with SIMCA software (v. 14.0, Umetrics, Umeå, Sweden) and KEGG database (https://www.kegg.jp/) was applied for the pathway enrichment analysis (19).
Detection of Protein Levels and Location in Spermatozoa Using Immunofluorescence Staining
Microscope slides loaded with boar sperm cells were incubated with 2% Triton X-100 for 1 h at room temperature. Then the samples were blocked for non-specific binding by incubating in 1% BSA with 1% goat serum for 30 min at room temperature. After washing, the cells were incubated with appropriate diluted primary antibodies (anti-Catsper, 1:100; anti-p-ERK, 1:100; anti-PKA, 1:100; anti-ZAG, 1:100) at 4°C overnight and then stained with secondary antibodies (Alexa Flour 546 goat-anti-rabbit IgG) at 1:200 dilution in PBS for 30 min at room temperature. Cell nuclei were counterstained with DAPI. All the samples were observed at room temperature using Leica Laser Scanning Confocal Microscope (LEICA TCS SP% II, Germany) (17).
Determination of Protein Levels by Western Blotting
Proteins was isolated from sperm cells using RIPA buffer containing protease and phosphate inhibitors (Sangong Biotech, Ltd, Shanghai, China). Total protein concentration was measured using BCA kit (Beyotime Institute of Biotechnology, Shanghai, Chain) according to the manufacture's instruction. Fifty microgram protein was loaded onto 10% polyacrylamide gels and transferred to polyvinylidene fluoride (PVDF) PVDF membrane. The membranes were blocked for 1 h at room temperature in TBST buffer containing 5% non-fat milk. Then the membranes were incubated overnight at 4°C with the primary antibodies (Novex® by life technologies, USA) at a dilution of 1:500 prepares in blocking solution. The control samples were incubated with actin antibody. After washed 3 times, the membranes were incubated with secondary donkey anti-goat IgG-HRP (Beyotime Institute of Biotechnology, Shanghai, P.R. China) or goat anti-rabbit IgG-HRP (Novex® by life technologies, USA) for 1 h at room temperature. finally, the blots were imaged following three washes (12, 13, 17).
Statistical Analysis
All statistical analyses were performed by using the student's t-test (SPSS 21 software). Spearman correlation analysis between the relative abundance of gut microbiota and plasma metabolites using GraphPad Prism 7.0. Data are expressed as the mean ± SEM. P-value < 0.05 was considered significant.
Data Availability
The microbiota raw sequencing data generated in this study has been uploaded to the NCBI SRA database with the accession number PRJNA779574.
Results
Effects of HT on Semen Quality
The semen quality was analyzed by using the semen obtained from the last two collections before the end of the experiment. Compared to control group, dietary supplementation of HT tended to increase sperm motility and semen volume/ejaculation even though not significantly (Figures 1B,C). However, HT did not alter sperm concentration or abnormal rate (Figures 1D,E).
Effects of HT on the Protein Expression of Important Genes Related to Sperm Quality
Compared to control group, dietary supplementation of HT increased the protein levels of important genes related to sperm quality such as Catsper, p-ERK, PKA, p-AKT, and ZAG by IHF staining analysis (Figures 2A,B; P < 0.05). The data were confirmed by the WB analysis of Catsper, Gelsolin, and PKA (Figures 2C,D; P < 0.05) which suggested that HT improved sperm quality by enhancing the protein expression of important genes related to sperm quality.
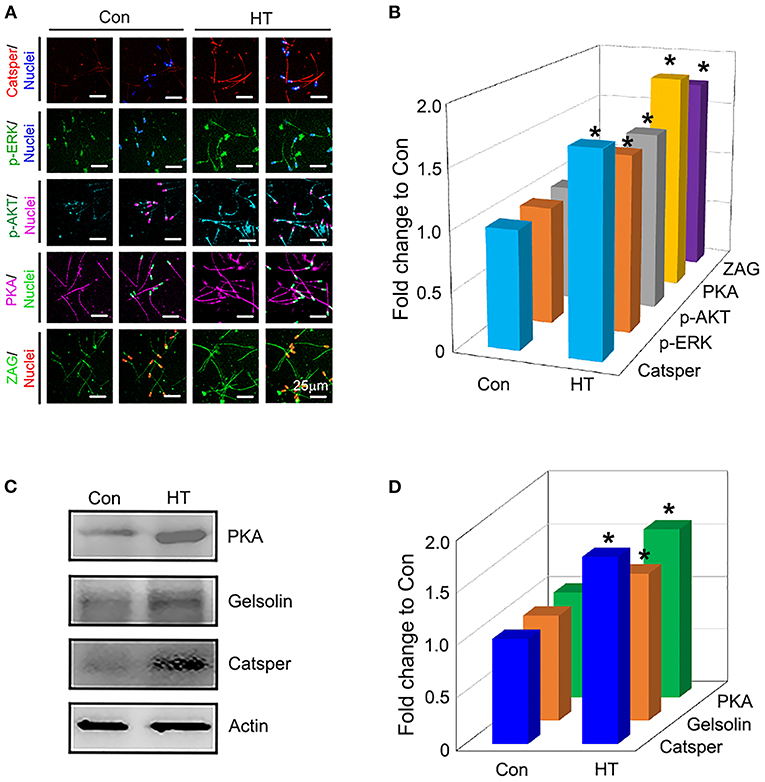
Figure 2. Effects of HT on the protein expression of important genes related to sperm quality. (A) Immunofluorescence staining (IHF) of Catsper, p-ERK, p-ERK, PKA, and ZAG. (B) Quantitative data for IHF staining (Fold change to Con). (C) Western blotting (WB) of PKA, Gelsolin, and Catsper. (D) Quantitative data for WB staining (Fold change to Con). *P < 0.05.
Improvement of HT on Fecal Microbiota
The results showed that dietary supplementation of HT had no significant effects on the α-diversity of fecal microbiota, which was characterized by ACE, Chao1, Simpson, and Shannon indexes (Supplementary Figure 1A). There were 1,109 common OTUs for both Con and HT groups (Supplementary Figure 1B). Moreover, the Con group and HT group contained specific 93 and 77 OTUs, respectively (Supplementary Figure 1B).
The microbial structure was different between HT group and Con group by PCoA analysis (Supplementary Figure 1C). At the phylum level, HT tended to reduce the relative abundance of Bacteroidetes and to increase the relative abundance of Actinobacteria (Figure 3A; Supplementary Figure 1E). At the order level, HT tended to decrease the level of Bacteroidales and to increase the relative abundance of Bifidobacteriales (Figure 3B; Supplementary Figure 1F). Moreover, at the family level, HT had the tendency to decrease the relative abundance of Prevotellaceae (Figure 3C; Supplementary Figure 1G). At the genus level, HT tended to increase the relative abundance of “beneficial microbes” Bifidobacterium, Lactobacillus, Eubacterium, Intestinimonas, Coprococcus, and Butyricicoccus (Figures 3D,E; Supplementary Figure 1H), however, to decrease the relative abundance of “harmful microbes” Streptococcus, Oscillibacter, Clostridium_sensu_stricto, Escherichia, Phascolarctobacterium, and Barnesiella (Figures 3D,F; Supplementary Figure 1H) (12). LDA effect size (LEfSe) analysis of the taxonomic alterations revealed that the content of some microbiota was different between HT group and control group (Supplementary Figure 1D).
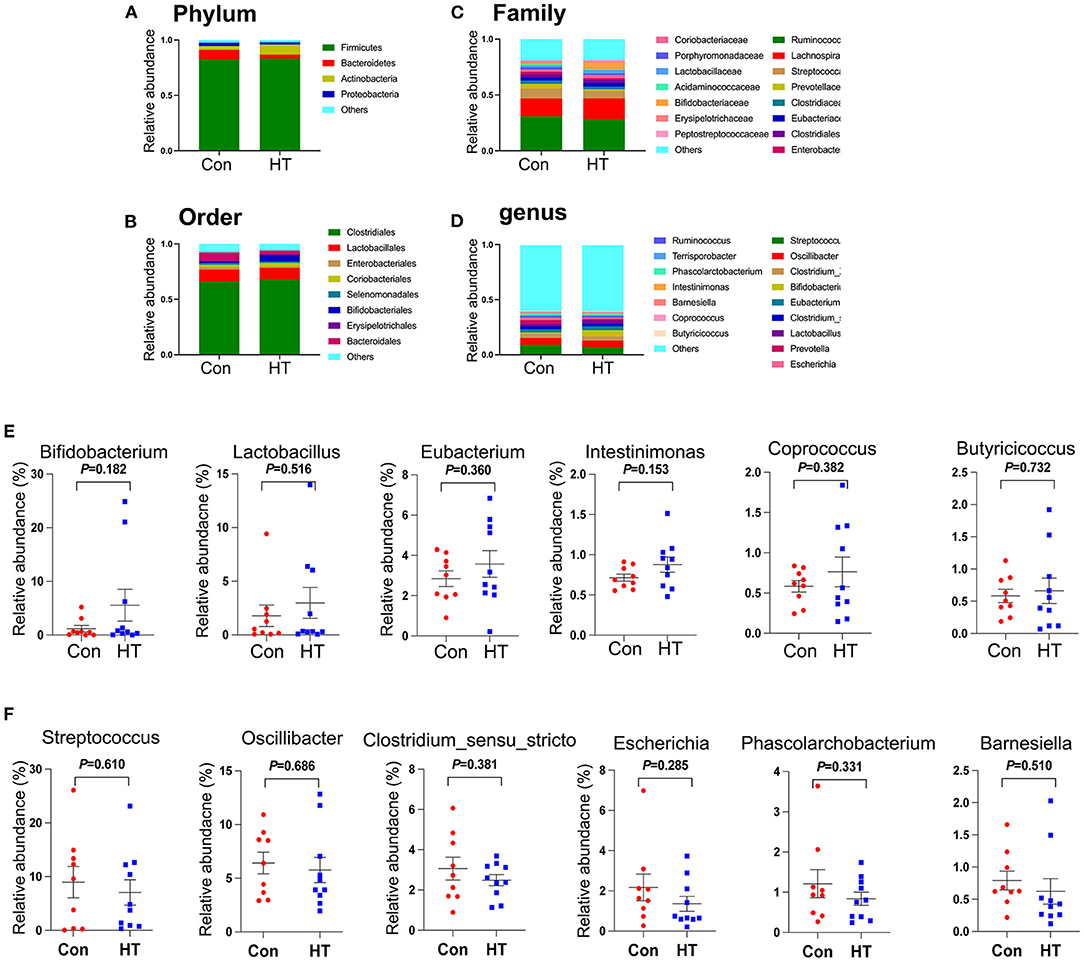
Figure 3. Effects of HT on the fecal microbial composition. (A) The relative amount of microbiota in feces at Phylum level. (B) The relative amount of microbiota in feces at Order level. (C) The relative amount of microbiota in feces at Family level. The relative amount of microbiota in feces at Genus level (D–F). Data were expressed as the mean ± SEM.
Effects of HT Supplement on the Plasma Metabolites
Compared to control group, HT significantly changed boar plasma metabolites indicated by the plot from the partial least squares discriminant analysis (PLS-DA) (Supplementary Figure 2A). There were 16 significantly changed metabolites between the HT and Con groups (Supplementary Figure 2B). The potential metabolic pathways of the changed metabolites were determined by KEGG pathway analysis. The results showed that the changed metabolites were involved in sphingolipid metabolism, Fc gamma R-mediated phagocytosis, apelin signaling pathway, choline metabolism in cancer, calcium signaling pathway, phospholipase D signaling pathway, tuberculosis, sphingolipid signaling pathway, and insulin resistance signaling pathways (Supplementary Figure 2C). It is very interesting to notice that HT increased plasma level of steroid hormones and their derivatives (Figures 4A–F), especially testosterone glucuronide (Figure 4A). And HT tended to increase the plasma levels of flavonoids: Naringenin 7-O-glucuronide, and 3,4-Dihydroxyphenylglycol (Figures 4G,H). Moreover, it is very interesting that melatonin metabolite 6-hydroxymelatonin, L-Acetylcarnitine and Propionylcarnitine were also elevated by HT in boar plasma although not significantly (Figures 4I–K). However, HT decreased plasma levels of bile acids: taurocholic, taurodeoxycholic acid, taurochenodesoxycholic acid, chenodeoxycholic acid glycine conjugate, 3a,7a,12a-Trihydroxy-5b-cholestan-26-al, isoursodeoxycholic acid, and deoxycholic acid glycine conjugate (Figures 4L–R). Furthermore, HT tended to decrease plasma LysoPC (16:0), 4-Hydroxy-3-polyprenylbenzoate, Phenethylamine glucuronide and Sphingosine 1-phosphate (Figures 4S–W).
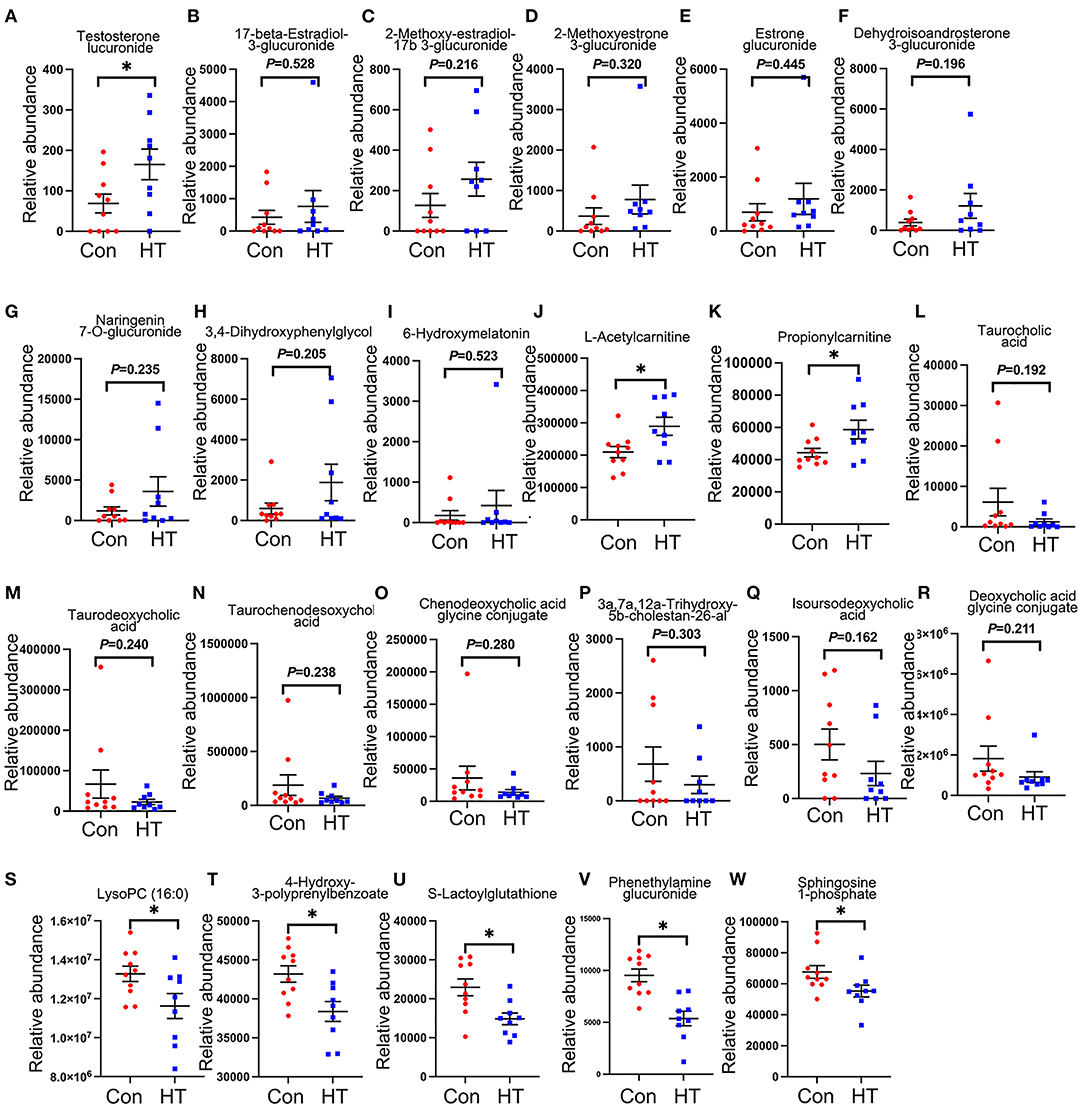
Figure 4. HT-induced alterations in plasma metabolites. (A–W) The relative quantification of plasma metabolites. Data were expressed as the mean ± SEM. *P < 0.05.
Correlation of Fecal Microbiota, Plasma Metabolites, and/or Sperm Quality
There was very good correlation between fecal microbiota and plasma metabolites, between plasma metabolites and semen parameters, and between fecal microbiota and semen parameters (the average of semen quality of each boar obtained from the last two collections before the end of the experiment) by the spearman correlation analysis (Figure 5). As shown in Figure 5A, the fecal Lactobacillus and Bifidobacterium were positively correlated with the plasma naringenin 7-O-glucuronide; while Streptococcus and Escherichia were negatively correlated with the plasma 3,4-dihydroxyphenylglycol and naringenin 7-O-glucuronide; Coprococcus was negatively correlated with plasma taurocholic, taurodeoxycholic acid, taurochenodesoxycholic acid, chenodeoxycholic acid glycine conjugate. Plasma LysoPC (16:0), S-lactoylglutathione, phenethylamine glucuronide, 4-hydroxy-3-polyprenylbenzoate, and ethyl glucuronide were negatively correlated with sperm motility (Figure 5B); while plasma gamma-glutamyl leucine, lithocholate 3-O-glucuronide, estrone glucuronide, and gamma-glutamylleucine were positively correlated with sperm motility (Figure 5B). Moreover, there was a significantly positive association between the sperm motility and the fecal Ruminococcus and a significantly negative association between the sperm volume and the fecal Streptococcus and Escherichia (Figure 5C).
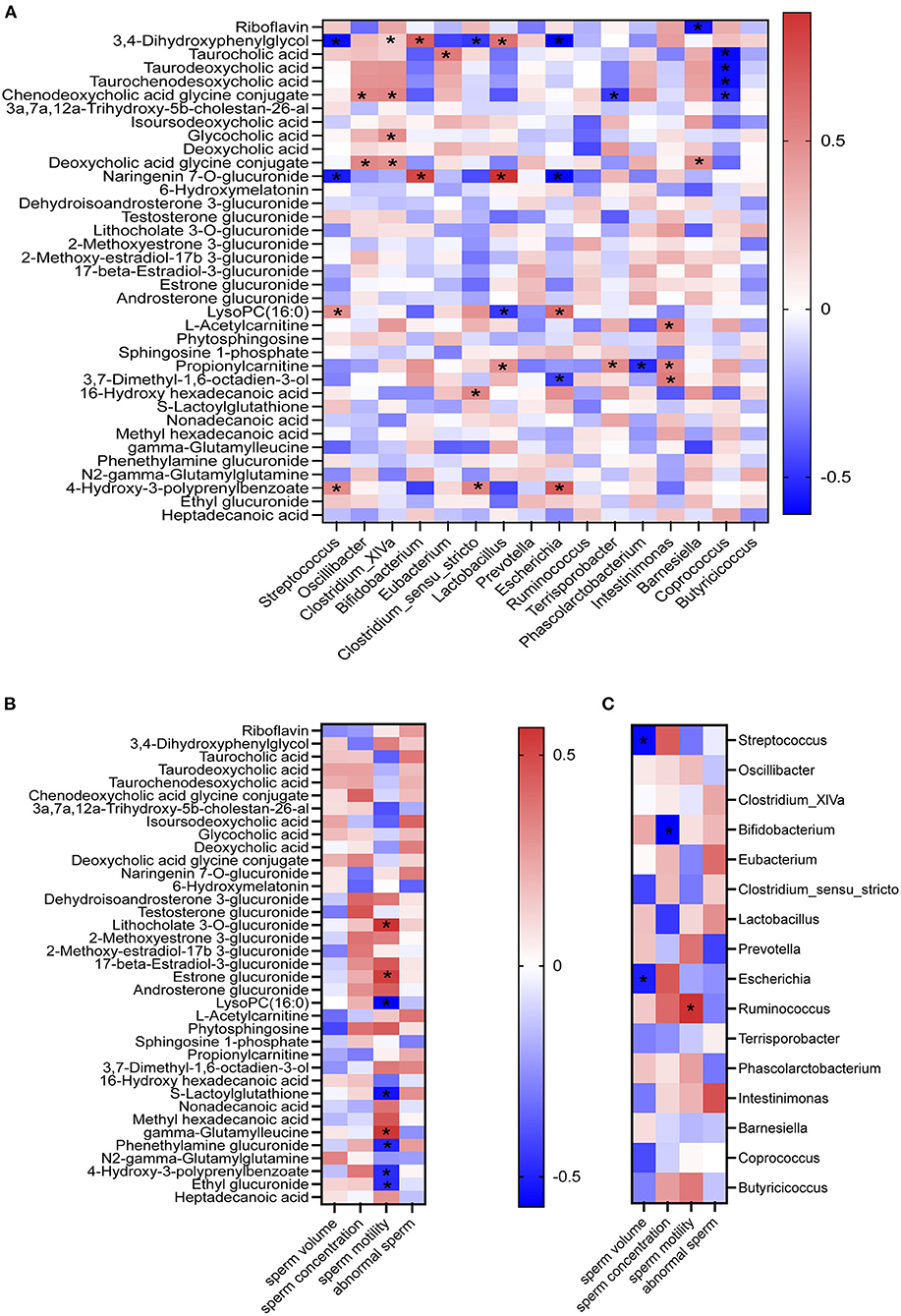
Figure 5. Correlations. Correlations between fecal microbiota and plasma metabolites (A); between plasma metabolites and semen quality parameters (B); and between fecal microbiota and semen quality parameters (C) by Pearson correlation analyses. *P < 0.05.
Long-Term Beneficial Effects of HT on Semen Quality
HT had a long-term beneficial effect on boar semen quality by the increase in the semen volume and the decrease in the percentage of abnormal sperm in the follow-up 2 months analysis (without HT supplementation) (Figures 6A,B). However, HT did not change sperm concentration and motility in the follow-up determination (Figures 6C,D).
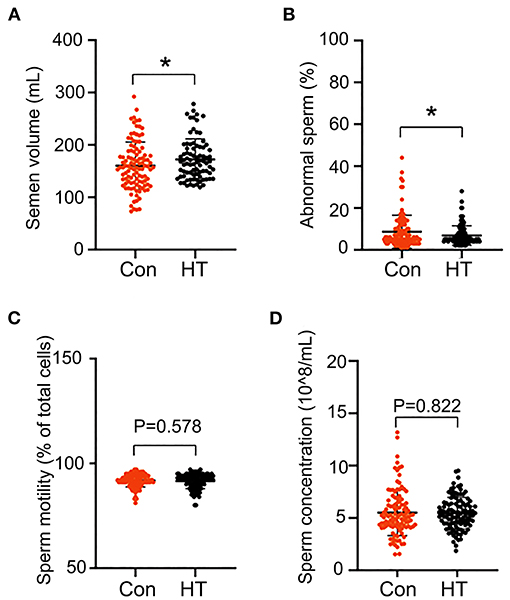
Figure 6. Long-term effects of HT on semen quality. After HT supplementation, semen quality was determined every 5 days for 2 months (no HT supplementation). (A) Semen volume. (B) Abnormal sperm. (C) Sperm motility. (D) Sperm concentration. Data were expressed as the mean ± SEM. *P < 0.05.
Discussion
Previous studies have reported that dietary supplementation of antioxidants, such as lysine and L-arginine could improve sperm quality in boars (3, 4). Our recent study found that HT had antioxidant effects via modulating gut microbiota and enhancing the expressions of antioxidant enzymes in mice (11). In current investigation, we found that dietary supplementation of HT tended to increase the semen quality, which may be associated with the alterations in gut microbiota and plasma metabolites.
Accumulating evidence suggests that gut microbiota involves in various perspectives of host health (20, 21). Moreover, previous studies have well-established the causal relationship between the gut microbiota and sperm quality in both animal models and humans (1, 12, 13, 22, 23). Polyphenols, including HT, can be metabolized by gut microbiota in the colon and on the other hand HT can modify gut microbiota (24, 25). HT enhanced the relative abundance of Actinobacteria, Prevotellaceae_UCG-001, and Lactobacillus johnsoniiin in mice (26, 27). Similarly, in current study, we found that HT tended to increase the relative abundance of Lactobacillus, which has been shown to have the ability to improve sperm motility in zebrafish (28, 29). Moreover, it has been shown that the increase in the relative abundance of Bacteroides and Prevotella was associated with higher circulating endotoxin and decreased spermatogenesis (22). HT decreased the relative amount of Prevotella and Bacteroides in current study. We previously found that alginate oligosaccharides (AOS) could enhance the sperm motility and concentration by the increase in the relative abundance of Bifidobacteriales in mice (12). In current study, we found that HT tended to increase the relative abundance of Bifidobacteriales. Collectively, our data suggested that HT had the potential to improve spermatogenesis and sperm motility by benefiting gut microbiota.
Our previous study has shown that improved gut microbiota can enhance sperm quality by altering circulating metabolome (12). In current investigation, dietary supplementation of HT altered plasma metabolites by the increase in steroid hormones, antioxidant molecules, and many others. Testosterone and its derivatives play vital roles in spermatogenesis which were increased by HT in boar plasma. L-carnitine plays an important role in improving sperm motility and has been used to treat infertility due to its strong anti-oxidant and anti-inflammatory effects in men and animal models (30–33). It has been shown that epididymis has high level of L-carnitine (32). Interestingly, in current investigation, HT enhanced the plasma level of propionyl-L-carnitine, which is a derivate of L-carnitine and can enhance cellular content of L-carnitine (34). Additionally, in this study, we found that HT enhanced the plasma levels of flavonoids and riboflavin. It has been shown that flavonoids and flavanone metabolites, such as naringenin 7-O-glucuronide and 3,4-dihydroxyphenylglycol, had powerful antioxidant ability, thus they may contribute to improved sperm quality and function (35–38). 6-Hydroxymelatonin (increased by HT) is one of the metabolites of melatonin and has been shown to have strong antioxidant activity (39). Thus, we speculate that HT might improve sperm quality by enhancing the antioxidant capacity of boars. Furthermore, various studies have reported that bile acids can cause oxidative stress by promoting the production of oxygen free radicals from mitochondria (40). Moreover, bile acids contribute to infertility by activating farnesoid X receptor and G-protein-coupled bile acid receptor expressed in sperm, which then influence glucose and lipid metabolism and lead to abnormal sperm (41, 42). In current study, plasma levels of several bile acids and their derivates were decreased by HT. Furthermore, there was a positive correlation between the plasma level of propionyl-L-carnitine and the relative abundance of Lactobacillus. Gut microbiota, including Lactobacillus, regulates the L-carnitine metabolism (43, 44). Collectively, these data demonstrated that HT might have the potential to improve plasma metabolome to benefit spermatogenesis.
Indeed, HT improved spermatogenesis by the increase in the protein levels of important genes (PKA, p-AKT, p-ERK, Catsper, ZAG, and Gelsolin) for spermatogenesis and sperm quality. Cation channel of sperm (Catsper) is the primary spermatozoan calcium ion channel and plays an essential role in male fertility via modulating sperm tail calcium entry and sperm hyperactivated motility (45). It has been reported that the cyclic adenosine monophosphate (cAMP) dependent protein kinase (protein kinase A, PKA), AKT, and ERK signaling are involved in modulating the sperm maturation, capacitation, and motility (46, 47). Zn-alpha2-glycoprotein (ZAG) can promote sperm motility via cAMP/PKA signaling pathway (48). Notably, in current study, HT increased the protein levels of these genes which suggested HT enhancing spermatogenesis.
The underlying mechanisms of HT improving semen quality is that it benefits gut microbiota to improve plamsa metabolites then to enhance spermatogenesis and semen quality. The data were confirmed by the follow-up long-term analysis. After the feeding period, all the boars were fed a basal diet (without HT supplementation) and semen samples were collected and analyzed every 5 days for 2 months. HT increased semen volume while decreased the percentage of abnormal sperm in the follow-up 2 months. In summary, HT improves boar semen quality via the benefiting on gut microbiota and plamsa metabolome. HT may be applied as dietary additive to improve boar semen quality in swine industry.
Data Availability Statement
The datasets presented in this study can be found in online repositories. The names of the repository/repositories and accession number(s) can be found in the article/Supplementary Material.
Ethics Statement
The animal study was reviewed and approved by the Animal Care and Use Committee of the Institute of Animal Sciences of Chinese Academy of Agricultural Sciences (IAS2021-67).
Author Contributions
YZha and HZ designed the experiment. HH, RZ, YZho, BX, LC, LL, HS, JT, and FT conducted the experiment and analyzed the data. HZ and YZha wrote and edited the manuscript. All authors contributed to the article and approved the submitted version.
Funding
This research was supported by the Agricultural Science and Technology Innovation Program (CAAS-ZDRW202006-02, ASTIPIAS07) and the State Key Laboratory of Animal Nutrition (2004DA125184G2102).
Conflict of Interest
HS and JT were employed by company YangXiang Joint Stock Company. FT was employed by company Hangzhou Viablife Biotech Co., Ltd.
The remaining authors declare that the research was conducted in the absence of any commercial or financial relationships that could be construed as a potential conflict of interest.
The handling editor declared a shared affiliation with the authors HH, RZ, YZ, BX, LC, YJ, and LL at the time of review.
Publisher's Note
All claims expressed in this article are solely those of the authors and do not necessarily represent those of their affiliated organizations, or those of the publisher, the editors and the reviewers. Any product that may be evaluated in this article, or claim that may be made by its manufacturer, is not guaranteed or endorsed by the publisher.
Acknowledgments
We thank the investigators and staff of The Beijing Genomics Institute (BGI) and Shanghai LUMING Biotechnology CO., LCD for technical support.
Supplementary Material
The Supplementary Material for this article can be found online at: https://www.frontiersin.org/articles/10.3389/fnut.2021.815922/full#supplementary-material
Supplementary Figure 1. Effects of HT on the fecal microbial diversity. (A) α-diversity with chao1, ACE, Shannon, and Simpson indexes. (B) Venn diagram of OUT. (C) PCoA of OUT. (D) Phylum level. (E) Order level. (F) Family level; (G) Genus level.
Supplementary Figure 2. Effects of HT on plasma metabolites. (A) OPLS-DA. (B) Heatmap of altered serum metabolites. (C) KEGG enriched pathways of altered plasma metabolites.
Supplementary Table 1. Primary antibody information.
Supplementary Data Set 1. Plasma metabolites.
References
1. Guo L, Wu Y, Wang C, Wei H, Tan J, Sun H, et al. Gut microbiological disorders reduce semen utilization rate in duroc boars. Front Microbiol. (2020) 11:581926. doi: 10.3389/fmicb.2020.581926
2. Wu YH, Lai W, Liu ZH, Wei HK, Zhou YF, Tan JJ, et al. Serum and seminal plasma element concentrations in relation to semen quality in Duroc boars. Biol Trace Elem Res. (2019) 189:85–94. doi: 10.1007/s12011-018-1459-y
3. Chen JQ, Li YS, Li ZJ, Lu HX, Zhu PQ, Li CM. Dietary l-arginine supplementation improves semen quality and libido of boars under high ambient temperature. Animal. (2018) 12:1611–20. doi: 10.1017/S1751731117003147
4. Dong HJ, Wu D, Xu SY, Li Q, Fang ZF, Che LQ, et al. Effect of dietary supplementation with amino acids on boar sperm quality and fertility. Anim Reprod Sci. (2016) 172:182–9. doi: 10.1016/j.anireprosci.2016.08.003
5. He Y, Li D, Zhang W, Tian X, Pang W, Du R, et al. Boar sperm quality and oxidative status as affected by rosmarinic acid at 17 °C. Trop Anim Health Prod. (2020) 52:2169–77. doi: 10.1007/s11250-020-02246-1
6. Ren Z, Shaoyong W, Li Q, Ma L, Xiao J, Jiao J, et al. Effects of Isatis root polysaccharide on boar sperm quality during liquid storage and in vitro fertilization. Anim Reprod Sci. (2019) 210:106178. doi: 10.1016/j.anireprosci.2019.106178
7. Namula Z, Sato Y, Kodama R, Morinaga K, Luu VV, Taniguchi M, et al. Motility and fertility of boar semen after liquid preservation at 5°C for more than 2 weeks. Anim Sci J. (2013) 84:600–6. doi: 10.1111/asj.12049
8. Bertelli M, Kiani AK, Paolacci S, Manara E, Kurti D, Dhuli K, et al. Hydroxytyrosol: a natural compound with promising pharmacological activities. J Biotechnol. (2020) 309:29–33. doi: 10.1016/j.jbiotec.2019.12.016
9. Karković Marković A, Torić J, Barbarić M, Jakobušić Brala C. Hydroxytyrosol, tyrosol and derivatives and their potential effects on human health. Molecules. (2019) 24:2001. doi: 10.3390/molecules24102001
10. Wei J, Wang S, Pei D, Qu L, Li Y, Chen J, et al. Antibacterial activity of hydroxytyrosol acetate from olive leaves (Olea Europaea L.). Nat Prod Res. (2018) 32:1967–70. doi: 10.1080/14786419.2017.1356830
11. Wang M, Zhang S, Zhong R, Wan F, Chen L, Liu L, et al. Olive fruit extracts supplement improve antioxidant capacity via altering colonic microbiota composition in mice. Front Nutr. (2021) 8:645099. doi: 10.3389/fnut.2021.645099
12. Zhang P, Feng Y, Li L, Ge W, Yu S, Hao Y, et al. Improvement in sperm quality and spermatogenesis following faecal microbiota transplantation from alginate oligosaccharide dosed mice. Gut. (2021) 70:222–5. doi: 10.1136/gutjnl-2020-320992
13. Zhao Y, Zhang P, Ge W, Feng Y, Li L, Sun Z, et al. Alginate oligosaccharides improve germ cell development and testicular microenvironment to rescue busulfan disrupted spermatogenesis. Theranostics. (2020) 10:3308–24. doi: 10.7150/thno.43189
14. Wu YH, Guo LL, Liu ZH, Wei HK, Zhou YF, Tan JJ, et al. Microelements in seminal and serum plasma are associated with fresh semen quality in Yorkshire boars. Theriogenology. (2019) 132:88–94. doi: 10.1016/j.theriogenology.2019.04.002
15. Zhang W, Zhao Y, Zhang P, Hao Y, Yu S, Min L, et al. Decrease in male mouse fertility by hydrogen sulfide and/or ammonia can be inheritable. Chemosphere. (2018) 194:147–57. doi: 10.1016/j.chemosphere.2017.11.164
16. Zhang P, Zhao Y, Zhang H, Liu J, Feng Y, Yin S, et al. Low dose chlorothalonil impairs mouse spermatogenesis through the intertwining of estrogen receptor pathways with histone and DNA methylation. Chemosphere. (2019) 230:384–95. doi: 10.1016/j.chemosphere.2019.05.029
17. Zhao Y, Zhang W, Liu X, Zhang P, Hao Y, Li L, et al. Hydrogen sulfide and/or ammonia reduces spermatozoa motility through AMPK/AKT related pathways. Sci Rep. (2016) 6:37884. doi: 10.1038/srep37884
18. WHO. WHO Laboratory Manual for the Examination and Processing of Human Semen. 5th ed. Cambridge: Cambridge University Press (2010).
19. Zhang P, Liu J, Xiong B, Zhang C, Kang B, Gao Y, et al. Microbiota from alginate oligosaccharide dosed mice successfully mitigated small intestinal mucositis. Microbiome. (2020) 8:112. doi: 10.1186/s40168-020-00886-x
20. Han H, Jiang Y, Wang M, Melaku M, Liu L, Zhao Y, et al. Intestinal dysbiosis in nonalcoholic fatty liver disease (NAFLD): focusing on the gut-liver axis. Crit Rev Food Sci Nutr. (2021) 1–18. doi: 10.1080/10408398.2021.1966738
21. Han H, Yi B, Zhong R, Wang M, Zhang S, Ma J, et al. From gut microbiota to host appetite: gut microbiota-derived metabolites as key regulators. Microbiome. (2021) 9:162. doi: 10.1186/s40168-021-01093-y
22. Ding N, Zhang X, Zhang XD, Jing J, Liu SS, Mu YP, et al. Impairment of spermatogenesis and sperm motility by the high-fat diet-induced dysbiosis of gut microbes. Gut. (2020) 69:1608–19. doi: 10.1136/gutjnl-2019-319127
23. Lundy SD, Sangwan N, Parekh NV, Selvam MKP, Gupta S, McCaffrey P, et al. Functional and taxonomic dysbiosis of the gut, urine, and semen microbiomes in male infertility. Eur Urol. (2021) 79:826–36. doi: 10.1016/j.eururo.2021.01.014
24. Tuck KL, Freeman MP, Hayball PJ, Stretch GL, Stupans I. The in vivo fate of hydroxytyrosol and tyrosol, antioxidant phenolic constituents of olive oil, after intravenous and oral dosing of labeled compounds to rats. J Nutr. (2001) 131:1993–6. doi: 10.1093/jn/131.7.1993
25. Visioli F, Galli C, Bornet F, Mattei A, Patelli R, Galli G, et al. Olive oil phenolics are dose-dependently absorbed in humans. FEBS Lett. (2000) 468:159–60. doi: 10.1016/S0014-5793(00)01216-3
26. Liu Z, Wang N, Ma Y, Wen D. Hydroxytyrosol improves obesity and insulin resistance by modulating gut microbiota in high-fat diet-induced obese mice. Front Microbiol. (2019) 10:390. doi: 10.3389/fmicb.2019.00390
27. Wang N, Ma Y, Liu Z, Liu L, Yang K, Wei Y, et al. Hydroxytyrosol prevents PM(2.5)-induced adiposity and insulin resistance by restraining oxidative stress related NF-κB pathway and modulation of gut microbiota in a murine model. Free Radic Biol Med. (2019) 141:393–407. doi: 10.1016/j.freeradbiomed.2019.07.002
28. Valcarce DG, Riesco MF, Martínez-Vázquez JM, Robles V. Diet supplemented with antioxidant and anti-inflammatory probiotics improves sperm quality after only one spermatogenic cycle in zebrafish model. Nutrients. (2019) 11:843. doi: 10.3390/nu11040843
29. Valcarce DG, Riesco MF, Martínez-Vázquez JM, Robles V. Long exposure to a diet supplemented with antioxidant and anti-inflammatory probiotics improves sperm quality and progeny survival in the zebrafish model. Biomolecules. (2019) 9:338. doi: 10.3390/biom9080338
30. Abd-Elrazek AM, Ahmed-Farid OAH. Protective effect of L-carnitine and L-arginine against busulfan-induced oligospermia in adult rat. Andrologia. (2018) 50:1–8. doi: 10.1111/and.12806
31. Micic S, Lalic N, Djordjevic D, Bojanic N, Bogavac-Stanojevic N, Busetto GM, et al. Double-blind, randomised, placebo-controlled trial on the effect of L-carnitine and L-acetylcarnitine on sperm parameters in men with idiopathic oligoasthenozoospermia. Andrologia. (2019) 51:e13267. doi: 10.1111/and.13267
32. Mongioi L, Calogero AE, Vicari E, Condorelli RA, Russo GI, Privitera S, et al. The role of carnitine in male infertility. Andrology. (2016) 4:800–7. doi: 10.1111/andr.12191
33. Reuter SE, Evans AM. Carnitine and acylcarnitines: pharmacokinetic, pharmacological and clinical aspects. Clin Pharmacokinet. (2012) 51:553–72. doi: 10.1007/BF03261931
34. Ferrari R, Merli E, Cicchitelli G, Mele D, Fucili A, Ceconi C. Therapeutic effects of L-carnitine and propionyl-L-carnitine on cardiovascular diseases: a review. Ann N Y Acad Sci. (2004) 1033:79–91. doi: 10.1196/annals.1320.007
35. Anacleto SL, Milenkovic D, Kroon PA, Needs PW, Lajolo FM, Hassimotto NMA. Citrus flavanone metabolites protect pancreatic-β cells under oxidative stress induced by cholesterol. Food Funct. (2020) 11:8612–24. doi: 10.1039/D0FO01839B
36. Kuang W, Zhang J, Lan Z, Deepak R, Liu C, Ma Z, et al. SLC22A14 is a mitochondrial riboflavin transporter required for sperm oxidative phosphorylation and male fertility. Cell Rep. (2021) 35:109025. doi: 10.1016/j.celrep.2021.109025
37. Saedisomeolia A, Ashoori M. Riboflavin in human health: a review of current evidences. Adv Food Nutr Res. (2018) 83:57–81. doi: 10.1016/bs.afnr.2017.11.002
38. Tvrdá E, Debacker M, Duračka M, Kováč J, Bučko O. Quercetin and naringenin provide functional and antioxidant protection to stored boar semen. Animals. (2020) 10:1930. doi: 10.3390/ani10101930
39. Maharaj DS, Walker RB, Glass BD, Daya S. 6-Hydroxymelatonin protects against cyanide induced oxidative stress in rat brain homogenates. J Chem Neuroanat. (2003) 26:103–7. doi: 10.1016/S0891-0618(03)00034-6
40. Bomzon A, Holt S, Moore K. Bile acids, oxidative stress, and renal function in biliary obstruction. Semin Nephrol. (1997) 17:549–62.
41. Baptissart M, Vega A, Martinot E, Pommier AJ, Houten SM, Marceau G, et al. Bile acids alter male fertility through G-protein-coupled bile acid receptor 1 signaling pathways in mice. Hepatology. (2014) 60:1054–65. doi: 10.1002/hep.27204
42. Malivindi R, Santoro M, De Rose D, Panza S, Gervasi S, Rago V, et al. Activated-farnesoid X receptor (FXR) expressed in human sperm alters its fertilising ability. Reproduction. (2018) 156:249–59. doi: 10.1530/REP-18-0203
43. Kim M, Kim M, Kang M, Yoo HJ, Kim MS, Ahn YT, et al. Effects of weight loss using supplementation with Lactobacillus strains on body fat and medium-chain acylcarnitines in overweight individuals. Food Funct. (2017) 8:250–61. doi: 10.1039/C6FO00993J
44. Ussher JR, Lopaschuk GD, Arduini A. Gut microbiota metabolism of L-carnitine and cardiovascular risk. Atherosclerosis. (2013) 231:456–61. doi: 10.1016/j.atherosclerosis.2013.10.013
45. Lishko PV, Kirichok Y, Ren D, Navarro B, Chung JJ, Clapham DE. The control of male fertility by spermatozoan ion channels. Annu Rev Physiol. (2012) 74:453–75. doi: 10.1146/annurev-physiol-020911-153258
46. Baro Graf C, Ritagliati C, Stival C, Luque GM, Gentile I, Buffone MG, et al. Everything you ever wanted to know about PKA regulation and its involvement in mammalian sperm capacitation. Mol Cell Endocrinol. (2020) 518:110992. doi: 10.1016/j.mce.2020.110992
47. Li X, Luo T, Li H, Yan N. Sphingomyelin synthase 2 participate in the regulation of sperm motility and apoptosis. Molecules. (2020) 25:4231. doi: 10.3390/molecules25184231
Keywords: hydroxytyrosol, boar, semen quality, gut microbiota, blood metabolome
Citation: Han H, Zhong R, Zhou Y, Xiong B, Chen L, Jiang Y, Liu L, Sun H, Tan J, Tao F, Zhao Y and Zhang H (2022) Hydroxytyrosol Benefits Boar Semen Quality via Improving Gut Microbiota and Blood Metabolome. Front. Nutr. 8:815922. doi: 10.3389/fnut.2021.815922
Received: 16 November 2021; Accepted: 23 December 2021;
Published: 17 January 2022.
Edited by:
Fengjiao Xin, Laboratory of Biomanufacturing and Food Engineering, Institute of Food Science and Technology, Chinese Academy of Agricultural Science (CAAS), ChinaReviewed by:
Jetty Chung-Yung Lee, The University of Hong Kong, Hong Kong SAR, ChinaYan Lin, Sichuan Agricultural University, China
Fengjie Huang, Shanghai Jiao Tong University, China
Copyright © 2022 Han, Zhong, Zhou, Xiong, Chen, Jiang, Liu, Sun, Tan, Tao, Zhao and Zhang. This is an open-access article distributed under the terms of the Creative Commons Attribution License (CC BY). The use, distribution or reproduction in other forums is permitted, provided the original author(s) and the copyright owner(s) are credited and that the original publication in this journal is cited, in accordance with accepted academic practice. No use, distribution or reproduction is permitted which does not comply with these terms.
*Correspondence: Yong Zhao, eXpoYW84MThAaG90bWFpbC5jb20=; eW9uZy56aGFvQG11cmRvY2guZWR1LmF1; Hongfu Zhang, emhhbmdob25nZnVAY2Fhcy5jbg==
†These authors have contributed equally to this work