Corrigendum: Fabrication and surface characterization of titanium dioxide nanotubes on titanium implants
Corrigendum: Fabrication and surface characterization of titanium dioxide nanotubes on titanium implants
- 1Department of Orthopedic Surgery, The People’s Hospital of Danyang, Danyang, China
- 2Department of Stomatology, The Seventh Medical Center of PLA General Hospital, Beijing, China
- 3Department of Orthopedics, Guizhou Provincial People’s Hospital, Guiyang, Guizhou, China
- 4Department of Orthopedics, Dafeng People’s Hospital, Yancheng, Jiangsu, China
Titanium has been widely used in orthopedics and dental implants due to its excellent biocompatibility and mechanical properties. However, the surface of titanium is biologically inert and lacks biological activity, resulting in poor integration between titanium-based implants and surrounding natural bone tissue, which is a common challenge in its clinical application. Surface modification is currently an effective means to improve the biocompatibility and bioactivity of titanium implants. The natural tissues of the human body are assembled from nanomodules, so from a biomimetic perspective, nanostructures should have better biological activity. TiO2 nanotubes have unique physical and chemical properties due to their elastic modulus, large specific surface area, and regular hollow structure similar to those of bone tissue. This study used anodic oxidation technology to prepare TiO2 nanotubes on the surface of titanium. The surface properties of the nanotubes were evaluated using field emission scanning electron microscopy (FE-SEM), X-ray diffraction (XRD), atomic force microscopy profilometry, contact angle measurements, etc. The corrosion resistance was tested using an electrochemical workstation. The results indicate that anodic oxidation can be used to successfully prepare titanium dioxide nanotube arrays on the surface of titanium. The nanotubes not only exhibit a good structure but also improve the surface hydrophilicity and corrosion resistance of titanium, thereby demonstrating potential for clinical application.
1 Introduction
Titanium and titanium alloys have excellent mechanical properties, biocompatibility, and corrosion resistance and have been widely used in materials for medical implants such as artificial joints and oral implants. However, because titanium is biologically inert, it cannot directly bind to bone and cannot stimulate bone formation on the surface of the material during the early period after implantation. This leads to issues such as poor bone bonding and prolonged healing in the clinical application of titanium implants. At the same time, due to the weak chemical bonds between titanium and bone, when titanium implants are placed in the body, a fibrous tissue envelope may form around them, separating the implanted material from the surrounding bone, which can lead to dislocation and premature loosening of the implant (Kaur and Singh, 2019). Good osseointegration of titanium implants with bone tissue is the foundation for implant function. To achieve better and faster implant osseointegration, domestic and foreign scholars have given increasing attention to titanium surface modification methods. Among the related topics, how the titanium surface affects the biological reaction of the surrounding tissue to the implant and how bone tissue adapts to and ideally bonds with the titanium surface have become research hotspots in the field of implantation.
Various surface treatment methods have been used to optimize the surface of titanium implants, including electrochemical anodization, template methods, and hydrothermal methods (Jemat et al., 2015; Han et al., 2023). Anodic oxidation is an electrochemical treatment method that can produce nanotube morphologies on the surface of materials such as Ti, Zr, and C (Minagar et al., 2012). The morphology of TiO2 nanotubes formed on a Ti surface shows unique characteristics compared to those formed on other surfaces; that is, the nanotubes are perpendicular to the material surface and arranged in an orderly manner. Nanomaterials are an important research direction in the field of biomaterials due to their ability to better simulate the natural surface characteristics of tissues in vivo, making it possible to achieve ideal tissue binding (Kulkarni et al., 2015).
The surface characteristics of titanium implants are important factors closely related to bone integration. Surface morphology and surface chemical properties are key factors affecting the interaction of an implant with cells or extracellular matrix and are crucial for the attachment and formation of bone around an implant. An increasing number of studies have shown that material surface morphology plays a very important role in regulating tissue and cellular biological reactions (Le Guéhennec et al., 2007), and compared to optimization of material chemical composition, optimization of surface morphology offers better durability, stability, and controllability and can be used to simultaneously optimize parameters such as material surface roughness, wettability, and surface energy (Albrektsson and Wennerberg, 2019). As a prerequisite for successful clinical implantation, titanium and its alloys must exhibit excellent bone bonding ability at the bone-implant interface.
In recent years, the research and application of biomedical materials have shifted their focus from microscopic morphology to nanoscale morphology. A nanoscale surface morphology on titanium implants can increase the free energy, enhance the adhesion of osteoblasts, and promote subsequent bone maturation and bone formation processes (Souza et al., 2019). On titanium nanostructures, the high surface energy of the nanoscale surface can increase the initial level of protein adsorption, which is crucial for regulating cellular interactions on the implant surface (Khang et al., 2012). Hollow and highly ordered TiO2 nanotubes form an ideal surface, promoting the release of functional substances, especially nanoparticles. On the other hand, research has shown that the presence of titanium dioxide nanostructures has advantages in implant materials. At the same time, this nanoscale surface exhibits properties similar to those of physiological bone, and the nanoscale topological morphology can effectively promote multiple cellular responses, including improved osseointegration and increased differentiation of mesenchymal stem cells. Additionally, nanotube structures offer certain advantages in improving the biocompatibility of titanium dioxide materials (Minagar et al., 2013), and the diameter of TiO2 nanotubes can vary in the range of tens to hundreds of nanometers, which is beneficial for selecting sizes suitable for different tissue combinations. TiO2 nanotubes can simulate the size and arrangement of collagen fibrils in bone tissue, and their elastic modulus is similar to that of bone tissue, which may result in better biological activity (Das et al., 2009a).
From a biomimetic perspective, natural tissues in the human body are assembled from nanomodules, so nanostructures should have better biocompatibility and biological activity. In view of this, we used anodic oxidation technology to prepare biomimetic TiO2 nanotubes on the surface of titanium. The surface properties of the nanotubes were evaluated using field emission scanning electron microscopy (FE-SEM), X-ray diffraction (XRD), atomic force microscopy (AFM), profilometry, contact angle measurements, and other methods. The corrosion resistance of the TiO2 nanotubes on the titanium surface was tested using an electrochemical workstation. At the same time, protein adsorption experiments were used to evaluate the protein adsorption ability of TiO2 nanotubes with the aim of laying a theoretical foundation for the clinical application of biomimetic TiO2 nanotubes on titanium surfaces.
2 Materials and methods
2.1 Preparation of experimental samples
Pure titanium (99.9%) was cut into titanium discs with a diameter of 10 mm and a thickness of 1 mm and then polished using a gradient of SiC sandpaper (200 #, 600 #, 800 #, 1500 #, and 2000 #). The titanium discs were ultrasonically cleaned with acetone, alcohol, and distilled water and then allowed to dry in air naturally.
Using a titanium disc as the anode and a platinum disc as the cathode, a diameter of approximately 80–110 nm nanotube array was formed on the surface of the titanium sheet when subjected to a voltage of 20 V in a 0.5 wt% HF reaction solution for 30 min. After the reaction was completed, the sample was washed with distilled water and dried for later use.
2.2 Surface characterization analysis
FE-SEM and AFM were used to observe the surface morphology of the experimental samples, XRD was used to analyze the surface composition of the samples, a profilometer was used to evaluate the roughness of the samples, and a contact angle measuring instrument was used to evaluate the hydrophilicity of the samples.
2.3 Hydrophilicity testing
A static water contact angle tester was used to test the surface hydrophilicity of the samples. First, 5 µL of deionized water was added dropwise onto the surface of each sample, and the contact angle instrument’s built-in imaging system was used to take photos of the droplet and analyze the contact angle. The test was conducted separately in three different areas on the surface of each sample.
2.4 Corrosion resistance
The Nyquist curve and potentiodynamic polarization curve of the samples in simulated body fluid were determined using an electrochemical workstation (model: Shanghai Chenhua CHI760E) with a three-electrode system, including a saturated calomel electrode (SCE) as the reference electrode (RE), a platinum electrode as the auxiliary electrode (CE), and the sample as the working electrode. The frequency testing range was 90 kHz–10 mHz, the potential scanning range for the polarization curve measurement was −1,000–600 mV, and the scanning speed was 1 mV/s.
2.5 Protein adsorption capacity
The protein adsorption ability of the sample surface represents the biological characteristics of the sample. In this experiment, each group of samples was immersed in a solution containing 10% FBS α-incubated in MEM culture medium for 2 h and rinsed with PBS to remove unadsorbed protein. Then, 1% SDS was added to dissolve the protein adsorbed on the surface of the sample, and a Micro BCA protein kit was used to detect the amount of protein adsorbed on the surface of each sample.
2.6 Statistical analysis
The experimental data results are all expressed as the mean ± SD, and statistical analysis was conducted using SPSS 14.0 statistical software. p < 0.05 was considered to indicate a significant difference.
3 Results
Figure 1 shows the morphology of TNTs formed by anodization of titanium at different magnifications under SEM. The surface of the TNTs consists of an array of vertical tubular structures; the nanotubes are consistent in diameter, arranged neatly, and evenly distributed. The tube diameter is approximately 80–110 nm, with clear spacing between adjacent nanotubes.
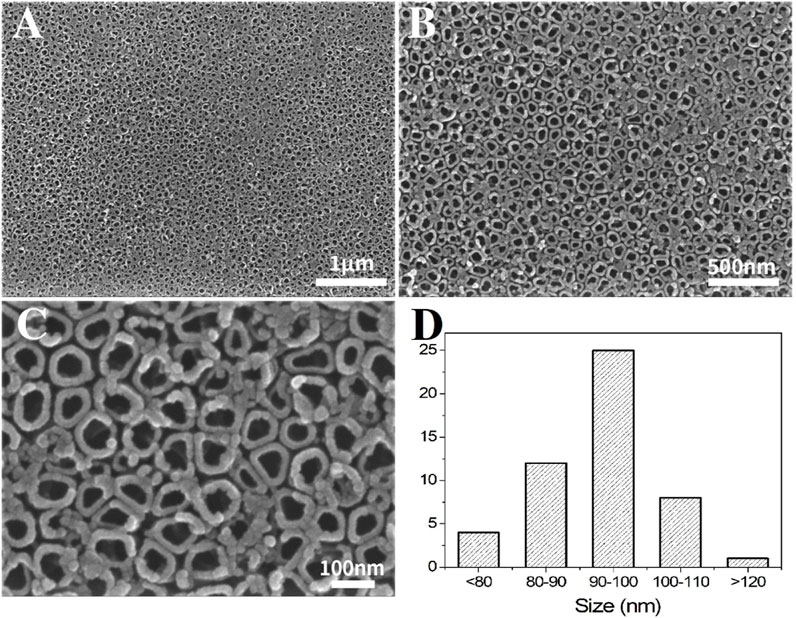
Figure 1. SEM morphology of TNTs at different magnifications. ((A–C): Different magnifications; (D): Distribution plot of the tube diameter).
Figure 2 shows the XRD analysis results of the Ti and TNT samples. The Ti sample was mainly composed of the titanium phase, and after anodization, the TNT sample did not show TiO2. It is considered that it mainly consists of amorphous TiO2.
Figure 3 shows the surface morphology of the Ti and TNT samples under atomic force microscopy. The surfaces of the two groups of samples are not the same. Compared to the Ti sample, the TNT sample surface has more small particle-like protrusions.
Figure 4 shows the profilometer surface morphology of each group of samples. Similar to AFM, profilometry showed that the surface of the Ti sample was relatively flat, while the surface of the TNT sample was rougher. Quantitative analysis showed that the roughness of the TNT sample was significantly greater than that of the Ti sample.
Figure 5 shows the contact angle test results of each group of samples. The hydrophilicity of a material surface is usually measured by the contact angle or free energy of the material surface. The smaller the contact angle is, the better the hydrophilicity and biocompatibility of the sample. It is obvious that compared with the Ti sample, the contact angle of the TNT sample was significantly reduced, indicating that the hydrophilicity of titanium was significantly improved after anodic oxidation.
Figure 6 shows the results of the corrosion resistance tests of each group of samples. Figure 6A shows the Nyquist curves of the two sets of samples; both samples show similar impedance curves and a capacitive arc. The diameter of the capacitive arc of the TNT samples was larger than that of the Ti samples, demonstrating good corrosion resistance. Figure 6B shows the polarization curves of the Ti and TNT samples. According to the Tafel curve extrapolation method, the corrosion current Icorr was fitted. The corrosion current Icorr (5.13*10–9) of the TNT samples was smaller than that of the Ti samples (1.73*10–7 A). According to the mixed potential theory, under similar cathode plans, the corrosion rate is generally proportional to the magnitude of the corrosion current Icorr. Therefore, in simulated body fluid, the TNTs would show a slower corrosion rate, indicating stronger corrosion resistance.
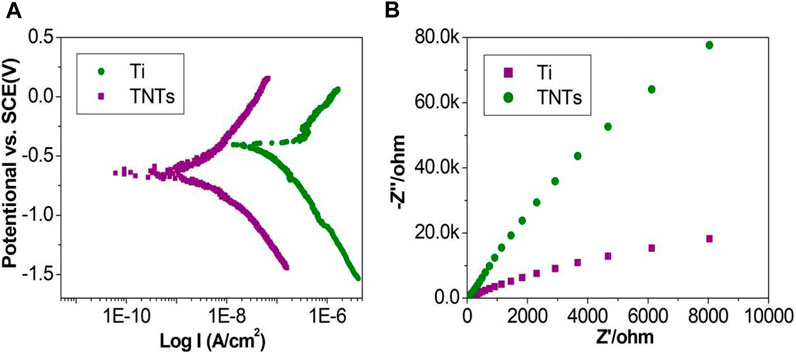
Figure 6. Nyquist curves and polarization curves of different samples ((A): Nyquist curves; (B): Polarization curves).
Figure 7 shows the protein adsorption capacity of each group of samples. The protein adsorption ability of a sample surface represents the biological characteristics of the sample. In this study, compared with the Ti samples, the amount of protein adsorbed by the TNT samples was significantly increased, indicating that the TiO2 nanotube structure is conducive to protein adsorption.
4 Discussion
Titanium (Ti) and its alloys have been widely used in orthopedic implants due to their superior mechanical properties. However, the surface of titanium is inert, which is not conducive to implant osseointegration. Therefore, the modification of titanium-based surfaces is necessary to improve the early osseointegration of titanium implants. Surface modification methods mainly enhance the osseointegration potential of titanium materials by changing the surface chemical composition, wettability, roughness, surface energy, microstructure, and nanomorphology (Kreve et al., 2022).
Among the numerous nanostructures used on implant surfaces, TiO2 nanotubes are currently a research hotspot. The equipment and method for the preparation of TiO2 nanotubes are simple, and the diameter and length of the nanotubes can be accurately controlled. At present, there is a clear understanding of the mechanism of TiO2 nanotube formation. After the start of anodic oxidation, a dense oxide film barrier layer is rapidly formed on the surface of the material. As the electric field intensity increases sharply, random breakdown dissolution occurs under the combined action of F- ions and the electric field, gradually forming evenly distributed small pores. There are small pits at the junction of the pores, and both the pits and pores continuously dissolve downwards. As a result, the undissolved oxide film gradually forms the walls of the nanotubes. The growth and elongation of nanotubes are actually the result of the continuous dissolution of the oxide layer toward the titanium matrix (Cipriano et al., 2014).
In this study, we used anodic oxidation technology to prepare TiO2 nanotubes on the surface of titanium. The nanotubes were uniformly sized and had a diameter of approximately 100 nm. The array consisted of vertically arranged nanotubes, and the pore size could be precisely controlled by adjusting the anodic oxidation process parameters. The influence of nanotube structures with different diameters on cell proliferation and differentiation varies. The main factors affecting the nanotube diameter include the type of electrolyte, electrolyte concentration, and anodizing voltage (Wang et al., 2014). In this experiment, we placed titanium sheets in HF electrolyte and prepared titanium dioxide nanotube structures with a diameter of approximately 100 nm by anodic oxidation at a voltage of 20 V. Experiments have shown that nanotube structures with a regular morphology and stable chemical structure can be prepared under this voltage.
Improving the biological activity and osseointegration of titanium through surface treatment, as well as improving the bone induction capability of titanium implants, can shorten the treatment time and enhance the initial stability of implants. Research has shown that TiO2 nanotubes may be more capable of promoting specific protein interactions than traditional materials, thereby promoting the function of osteoblast-related cells and vascular endothelial cells, indicating that TiO2 nanotubes have good tissue compatibility (Khudhair et al., 2016). In addition, TiO2 nanotubes can also be loaded with bioactive factors or antibacterial ions to promote their binding to tissues and cells (Wu et al., 2021) while exerting certain antibacterial effects.
The physical and chemical properties of materials may greatly affect their biological activity and function. Research has shown that compared to pure titanium surfaces, surfaces altered to have a nanomorphology and different surface chemical properties have been shown to induce new bone tissue formation, thereby promoting implant osseointegration (Zhu et al., 2021). Further research has shown that compared to Ti surfaces without nanostructures or surfaces with nanostructures with an average diameter of 30 nm, surfaces with nanostructures with an average diameter of 100 nm have higher hydrophilicity (Faghihi and Eslaminejad, 2014). There is currently no consensus on the optimal nanostructure morphology for osteogenesis, but the optimal size is generally believed to be 50–100 nm, which is suitable in terms of cell diameter (Ocampo and Echeverría, 2021). According to research, the mechanism by which the nanoscale surface morphology of this titanium implant promotes osteogenesis is as follows. First, from a cellular dynamics perspective, nanostructures induce osteoblasts to extend filamentous and plate-like pseudopodia, resulting in the recombination of extracellular matrix proteins and cytoskeletal proteins, which is beneficial for osteoblast adhesion and spreading. Second, nanostructures act as mechanical stimulation signals, which are mediated by calcium channel proteins to regularly aggregate and elongate the cytoskeleton, thereby altering the morphology of the cell nucleus and chromosome sequence, which is beneficial for osteoblast proliferation. In addition, by regulating cell signaling pathways, nanostructures can induce cell differentiation toward the osteogenic lineage (Gulati et al., 2016).
The contact angle can reflect the hydrophilicity or hydrophobicity of a surface. The surface characteristics of titanium materials, such as hydrophilicity and surface energy, are also important factors affecting their bioactivity and osseointegration capability. A decrease in the contact angle between a material surface and water indicates an improvement in the hydrophilicity of the material, which is beneficial for cell adhesion (Menzies and Jones, 2010). The results of this experiment indicate that the contact angle of the TNT samples was significantly reduced compared to that of the Ti samples, indicating that TiO2 nanotubes have good hydrophilicity. The reason why TiO2 nanotubes increase hydrophilicity may be related to the following two aspects. First, due to the nanomorphology, TiO2 nanotubes can better promote cell adhesion than flat Ti. Second, the characteristics of the nanotubes themselves can enhance the hydrophilic surface properties of TiO2 (Das et al., 2009b).
After a material is implanted into an organism, various proteins first adsorb onto the surface of the material in stages, forming a layer of biological protein molecules. Mediated by this process, cells within the tissue adhere to the surface of the material, with greater protein adsorption to the surface being more conducive to cell adhesion (Ngandu Mpoyi et al., 2016). Research has shown that the protein adsorption capacity of the surface of biomaterials reflects the biological effects of the biomaterial, and the number, type, and configuration of adsorbed proteins on the material surface largely determine the subsequent tissue reactions (Barberi and Spriano, 2021). In this study, compared to the control titanium samples, the TiO2 nanotube samples adsorbed more proteins, indicating that the nanotube structure could promote protein adsorption.
Through surface modification technology, TiO2 nanotube arrays with nanometer morphology characteristics were prepared on the surface of titanium to combine the titanium surface structure and biomedical function. Nanostructured biomaterials are closer to the natural bone tissue morphology and have chemical properties, which may provide a more ideal growth support environment for bone tissue regeneration (Hill et al., 2019). Nanomorphologies such as nanocrystals (Wang et al., 2021), nanohydroxyapatite (Bezerra et al., 2017) and electrospun nanofibers (Dumitriu et al., 2014) have been prepared on the surface of titanium implants, and biological experiments have found that cells are very sensitive to these nanomorphologies and that nanostructures can better induce multiple biological functions, such as alkaline phosphatase activity and extracellular bone matrix mineralization ability of bone marrow mesenchymal stem cells (BMSCs). Based on the perspective of biomimetics, TiO2 nanotube arrays were prepared on the surface of titanium implants by anodic oxidation in this study. By studying the preparation and properties of TiO2 nanotube arrays on the surface of titanium, a new method for improving the biological activity of titanium implants was provided, which has potential application prospects.
In summary, this study used anodic oxidation technology to prepare biomimetic TiO2 nanotubes on the surface of titanium. The nanotubes formed an array of vertical tubular structures with a uniform diameter, which not only improved the roughness and hydrophilicity of titanium but also increased its corrosion resistance. More importantly, the TiO2 nanotube structure was conducive to protein adsorption, thereby exhibiting good biocompatibility and potential for clinical application.
Data availability statement
The original contributions presented in the study are included in the article/supplementary material, further inquiries can be directed to the corresponding authors.
Author contributions
HZ: Resources, Writing–Original Draft, Writing–Review and Editing. LX: Resources, Writing–Original Draft. YJ: Investigation, Validation. YX: Investigation, Formal analysis. XW: Investigation, Methodology. KL: Investigation, Methodology. PZ: Investigation, Data Curation. QZ: Conceptualization, Writing–Review and Editing. LZ: Conceptualization, Data Curation, Visualization, Supervision. XN: Conceptualization, Visualization, Project administration, Supervision.
Funding
The author(s) declare that financial support was received for the research, authorship, and/or publication of this article. This work was supported by the Key Research and Development (Key R & D) plan of Jiangsu Province - social development (BE2021683), Guiyang Science and Technology Plan Project (grant number [2023] 48-23), National Science Foundation of China (82160415), Guizhou Province Excellent Young Science and Technology Talents Project (YQK[2023] 022), Guizhou Provincial Science and Technology Projects (grant number ZK [2024] general 475) and Guizhou Provincial People’s Hospital fund (grant numbers GZSYBS [2022] 01, GZSYQN[2021] 05 and GZSYQN [2022] 03).
Conflict of interest
The authors declare that the research was conducted in the absence of any commercial or financial relationships that could be construed as a potential conflict of interest.
Publisher’s note
All claims expressed in this article are solely those of the authors and do not necessarily represent those of their affiliated organizations, or those of the publisher, the editors and the reviewers. Any product that may be evaluated in this article, or claim that may be made by its manufacturer, is not guaranteed or endorsed by the publisher.
References
Albrektsson, T., and Wennerberg, A. (2019). On osseointegration in relation to implant surfaces. Clin. Implant Dent. Relat. Res. 21 (S1), 4–7. doi:10.1111/cid.12742
Barberi, J., and Spriano, S. (2021). Titanium and protein adsorption: an overview of mechanisms and effects of surface features. Materials 14 (7), 1590. doi:10.3390/ma14071590
Bezerra, F., Ferreira, M. R., Fontes, G. N., da Costa Fernandes, C. J., Andia, D. C., Cruz, N. C., et al. (2017). Nano hydroxyapatite-blasted titanium surface affects pre-osteoblast morphology by modulating critical intracellular pathways. Biotechnol. Bioeng. 114 (8), 1888–1898. doi:10.1002/bit.26310
Cipriano, A. F., Miller, C., and Liu, H. (2014). Anodic growth and biomedical applications of TiO2 nanotubes. J. Biomed. Nanotechnol. 10 (10), 2977–3003. doi:10.1166/jbn.2014.1927
Das, K., Bose, S., and Bandyopadhyay, A. (2009). TiO2 nanotubes on Ti: influence of nanoscale morphology on bone cell–materials interaction. J. Biomed. Mat. Res. Part A 90 (1), 225–237. doi:10.1002/jbm.a.32088
Das, K., Bose, S., and Bandyopadhyay, A. (2009). TiO2 nanotubes on Ti: influence of nanoscale morphology on bone cell–materials interaction. J. Biomed. Mater. Res. - Part A 90A (1), 225–237. doi:10.1002/jbm.a.32088
Dumitriu, C., Stoian, A. B., Titorencu, I., Pruna, V., Jinga, V. V., Latonen, R. M., et al. (2014). Electrospun TiO2 nanofibers decorated Ti substrate for biomedical application. Mat. Sci. Eng. C Mat. Biol. Appl. 45, 56–63. doi:10.1016/j.msec.2014.08.055
Faghihi, F., and Eslaminejad, M. B. (2014). The effect of nano-scale topography on osteogenic differentiation of mesenchymal stem cells. Biomed. Pap. 158 (1), 5–16. doi:10.5507/bp.2013.013
Gulati, K., Maher, S., Findlay, D. M., and Losic, D. (2016). Titania nanotubes for orchestrating osteogenesis at the bone–implant interface. Nanomedicine 11 (14), 1847–1864. doi:10.2217/nnm-2016-0169
Han, X., Ma, J., Tian, A., Wang, Y., Li, Y., Dong, B., et al. (2023). Surface modification techniques of titanium and titanium alloys for biomedical orthopaedics applications: a review. Colloids Surfaces B Biointerfaces 227, 113339. doi:10.1016/j.colsurfb.2023.113339
Hill, M. J., Qi, B., Bayaniahangar, R., Araban, V., Bakhtiary, Z., Doschak, M. R., et al. (2019). Nanomaterials for bone tissue regeneration: updates and future perspectives. Nanomedicine 14 (22), 2987–3006. doi:10.2217/nnm-2018-0445
Jemat, A., Ghazali, M. J., Razali, M., and Otsuka, Y. (2015). Surface modifications and their effects on titanium dental implants. Biomed. Res. Int. 2015, 1–11. doi:10.1155/2015/791725
Kaur, M., and Singh, K. (2019). Review on titanium and titanium based alloys as biomaterials for orthopaedic applications. Mat. Sci. Eng. C 102, 844–862. doi:10.1016/J.MSEC.2019.04.064
Khang, D., Choi, J., Im, Y.-M., Kim, Y.-J., Jang, J.-H., Kang, S. S., et al. (2012). Role of subnano-nano- and submicron-surface features on osteoblast differentiation of bone marrow mesenchymal stem cells. Biomaterials 33 (26), 5997–6007. doi:10.1016/j.biomaterials.2012.05.005
Khudhair, D., Bhatti, A., Li, Y., Hamedani, H. A., Garmestani, H., Hodgson, P., et al. (2016). Anodization parameters influencing the morphology and electrical properties of TiO2 nanotubes for living cell interfacing and investigations. Mat. Sci. Eng. C 59, 1125–1142. doi:10.1016/j.msec.2015.10.042
Kreve, S., Ferreira, I., da Costa Valente, M. L., and Dos Reis, A. C. (2022). Relationship between dental implant macro-design and osseointegration: a systematic review. Oral Maxillofac. Surg. 26, 1–14. doi:10.1007/s10006-022-01116-4
Kulkarni, M., Mazare, A., Gongadze, E., Perutkova, S., Kralj-Iglic, V., Milošev, I., et al. (2015). Titanium nanostructures for biomedical applications. Nanotechnology 26 (6), 062002. doi:10.1088/0957-4484/26/6/062002
Le Guéhennec, L., Soueidan, A., Layrolle, P., and Amouriq, Y. (2007). Surface treatments of titanium dental implants for rapid osseointegration. Dent. Mat. 23 (7), 844–854. doi:10.1016/j.dental.2006.06.025
Menzies, K. L., and Jones, L. (2010). The impact of contact angle on the biocompatibility of biomaterials. Optom. Vis. Sci. 87 (6), 387–399. doi:10.1097/OPX.0b013e3181da863e
Minagar, S., Berndt, C. C., Wang, J., Ivanova, E., and Wen, C. (2012). A review of the application of anodization for the fabrication of nanotubes on metal implant surfaces. Acta Biomater. 8 (8), 2875–2888. doi:10.1016/j.actbio.2012.04.005
Minagar, S., Wang, J., Berndt, C. C., Ivanova, E. P., and Wen, C. (2013). Cell response of anodized nanotubes on titanium and titanium alloys. J. Biomed. Mat. Res. Part A 101 (9), 2726–2739. doi:10.1002/jbm.a.34575
Ngandu Mpoyi, E., Cantini, M., Reynolds, P. M., Gadegaard, N., Dalby, M. J., and Salmerón-Sánchez, M. (2016). Protein adsorption as a key mediator in the nanotopographical control of cell behavior. ACS Nano 10 (7), 6638–6647. doi:10.1021/acsnano.6b01649
Ocampo, R. A., and Echeverría, F. E. (2021). Antibacterial and biological behavior of TiO2 nanotubes produced by anodizing technique. Crit. Rev. Biomed. Eng. 49 (1), 51–65. doi:10.1615/CritRevBiomedEng.2021037758
Souza, J. C. M., Sordi, M. B., Kanazawa, M., Ravindran, S., Henriques, B., Silva, F. S., et al. (2019). Nano-scale modification of titanium implant surfaces to enhance osseointegration. Acta Biomater. 94, 112–131. doi:10.1016/j.actbio.2019.05.045
Wang, Y., Wen, C., Hodgson, P., and Li, Y. (2014). Biocompatibility of TiO2 nanotubes with different topographies. J. Biomed. Mat. Res. Part A 102 (3), 743–751. doi:10.1002/jbm.a.34738
Wang, Z., Wang, X., Wang, Y., Zhu, Y., Liu, X., and Zhou, Q. (2021). NanoZnO-modified titanium implants for enhanced anti-bacterial activity, osteogenesis and corrosion resistance. J. Nanobiotechnology 19 (1), 353. doi:10.1186/s12951-021-01099-6
Wu, Y., Li, Q., Xu, B., Fu, H., and Li, Y. (2021). Nano-hydroxyapatite coated TiO2 nanotubes on Ti-19Zr-10Nb-1Fe alloy promotes osteogenesis in vitro. Colloids Surfaces B Biointerfaces 207, 112019. doi:10.1016/j.colsurfb.2021.112019
Keywords: anodic oxidation, TiO2 nanotubes, bioactive coating, corrosion resistance, titanium implants
Citation: Zheng H, Xu L, Jiao Y, Xia Y, Wu X, Lu K, Zhang P, Zhao Q, Zhang L and Ni X (2023) Fabrication and surface characterization of titanium dioxide nanotubes on titanium implants. Front. Mater. 10:1325997. doi: 10.3389/fmats.2023.1325997
Received: 22 October 2023; Accepted: 04 December 2023;
Published: 14 December 2023.
Edited by:
Marco Alfano, University of Waterloo, CanadaReviewed by:
Kailu Xiao, Texas A and M University, United StatesMojgan Heydari, Materials and Energy Research Center, Iran
Copyright © 2023 Zheng, Xu, Jiao, Xia, Wu, Lu, Zhang, Zhao, Zhang and Ni. This is an open-access article distributed under the terms of the Creative Commons Attribution License (CC BY). The use, distribution or reproduction in other forums is permitted, provided the original author(s) and the copyright owner(s) are credited and that the original publication in this journal is cited, in accordance with accepted academic practice. No use, distribution or reproduction is permitted which does not comply with these terms.
*Correspondence: Quanming Zhao, cHJvZmVzc29yZ2tAMTYzLmNvbQ==; Lu Zhang, MjI3MjI4Mzk5NEBxcS5jb20=; Xiaohui Ni, bml4aWFvaHVpQGpzbWMuZWR1LmNu
†These authors have contributed equally to this work and share first authorship
‡These authors have contributed equally to this work