- 1Shanghai Xuhui District Dental Center, Shanghai, China
- 2State Key Laboratory of High Performance Ceramics and Superfine Microstructure, Shanghai Institute of Ceramics, Chinese Academy of Sciences, Shanghai, China
- 3School of Chemistry and Materials Science, Hangzhou Institute for Advanced Study, University of Chinese Academy of Sciences, Hangzhou, China
Developing bacterial biofilm on the dental unit waterlines increases the risk of cross-infection among oral patients. Although chemical disinfectants can achieve disinfection effects in a short period of time, corrosion damage of dental unit waterlines and water contamination can also occur after continuous use of it. Herein, this study explored a one-step deposition method to prepare a durable and renewable antibacterial N-halamine polymeric coating on polyurethane waterlines. The method utilized polyelectrolyte complexes formed with polyethylenimine (PEI) and phytic acid (PA), followed by chlorination to activate the antibacterial properties. The N-halamine polymeric coating reduces the polyurethane waterline’s water contact angle, thus reducing biofouling deposits and the obstruction of the active halide site on the waterlines, thereby facilitating the maintenance of the cleanliness of the coating. In addition, benefiting both from the active chlorine release and the high density of positive charges on the coating, the polyurethane waterline antimicrobial activity is significantly enhanced. Besides, the N-halamine polymeric coating is biocompatible. This study showed that long-lasting and renewable antimicrobial requirements can be achieved by simple surface modification of N-halamine polymer coatings, which provides a practicable strategy for the production of long-term and reproducible antibacterial dental unit waterlines to reduce the incidence of hospital infection in oral department.
1 Introduction
The dental unit waterline (DUWL) is an important part of the dental chair unit, and its main function is to provide water for therapeutic devices such as mobile phones and triple guns during the courses of treatment, to play a cooling and flushing role. However, connecting parts such as dental mobile phones are difficult to disassemble and clean, and the DUWL is narrow and slender, which easily leads to the formation and continuous growth of biofilms on the surface of the waterlines. During treatment, the bacterial colonies colonized in the DUWL are often washed down by the water flow, and these bacteria can enter the patient’s mouth with the water flow, or they can be inhaled into the respiratory tract by the patient or dental staff through the aerosol generated by the ultrasonic dental cleaning machine or high-speed turbine mobile phone, resulting in hospital infection in department of stomatology. Therefore, DUWL contamination is considered to be one of the main ways to cause cross-infection among oral patients and healthcare workers (Williams et al., 1993; Pankhurst and Coulter, 2007; Coleman, O’Donnell et al., 2009).
Biofilm is an important factor of water pollution that causes water contamination in dental chair unit. It was reported that the bacteria in the waterline could reach 2 × 105 CFU/mL after a dental treatment chair was put into use for 5 days (Watanabe et al., 2016). The average thickness of mature microbial biofilm on the inner surface of DUWL is 25–35 nm, and the biofilm with loose surface structure can be washed by water and released as floating bacteria (Ramalingam et al., 2013). The microorganisms that contaminate DUWL are composed of bacteria, fungi, viruses and amoeba, and the main component is bacteria (Lizzadro et al., 2019). The most common of these bacteria are gram-negative bacilli, followed by gram-positive bacilli, gram-positive bacilli and actinomyces (O’Donnell et al., 2011). Furthermore, phytoplankton contains a large number of opportunistic pathogens, and even pathogens such as Pseudomonas aeruginosa and Legionella pneumophila (Vincent et al., 2018). If the waterline of the dental chair unit is not effectively sterilized, the biofilm fragments in it would probably be transferred to the patient’s mouth, resulting in acute and chronic infection. In addition, some biofilms can also be converted into part of aerosols, which are easily inhaled by dental clinic staff and patients, inducing asthma, rhinitis and infectious diseases (Ji et al., 2016). Therefore, the DUWL needs to be properly disinfected to minimize the microbial contamination and meet water standards for oral disease treatment.
Common disinfection methods of DUWL can be divided into two categories: non-chemical methods and chemical methods. The non-chemical methods can effectively remove bacterial films attached to the surface of the waterline through ultraviolet irradiation, ozone sterilization and mechanical erasure, etc. However, due to the narrow, complex and difficult disassembly of the waterline of dental chair unit, non-chemical methods are difficult to carry out comprehensive sterilization of the whole waterlines. Regarding chemical sterilization, the disinfectants commonly used at present mainly include chlorhexidine gluconate, sodium hypochlorite, povidone iodine, hydrogen peroxide, etc (Aprea et al., 2010; Abdouchakour et al., 2015; Ji et al., 2016). The main mechanism of such strong oxidant is to destroy the DNA or RNA structure of the pathogen and hinder the metabolic pathway of adenosine triphosphate to kill the pathogen (Dutil et al., 2007; Shajahan et al., 2017). Chemical methods can achieve excellent disinfection effect in a certain time, and it should be convenient and fast, but the long-term and frequent use of chemical disinfection method still faces many challenges. For example, it will cause corrosion to the dental chair unit and reduce the service life of the waterline, and affect the adhesion of the resin material to the dentin and tooth enamel (Ritter et al., 2007; Schönning et al., 2017). Based on this, suitable surface modification of DUWL to achieve the long-term circulation and renewable antibacterial function of the waterline is an urgent problem to be solved.
Halamine coatings show significant promise as antimicrobial materials due to several key advantages: broad-spectrum efficacy against diverse microorganisms, inherent biocompatibility, excellent long-term stability and unique regenerability. Besides, halamine coatings are less corrosive and more environmentally friendly than sodium hypochlorite (Kenawy et al., 2007; Hui and Debiemme-Chouvy, 2013; Dong et al., 2017; Debiemme-Chouvy and Cachet, 2018). N-halamine coating is an organic polymer usually formed by halogenation of amine, imine or amide groups, containing nitrogen-halogen covalent bonds (Chien and Chiu, 2020). The antimicrobial performances of N-halamines coating depends on the halide ions’ oxidation state in chloramines. Which can be bactericidal not only through direct contact with bacteria, but also through the release of chloride ions. Halogen ions in the oxidized state can directly interact with biological receptors (thiol groups or amino groups) on the surface of bacteria, resulting in bacterial inhibition or inactivation (Jain et al., 2014). What’s more, an important advantage of N-halamine coating over other fungicides is that its antibacterial properties could be regenerated by simply rinsing with bleach after the halogen ions being exhausted (Liu Y. et al., 2016; Ma et al., 2019).
In this work, an N-haloamine polymer coating based on polyelectrolyte complexes were constructed on a dental unit waterline, synthesized with PEI and PA components. And the long-lasting and renewable antimicrobial needs can be met by simple rinsing with sodium hypochlorite disinfectant. The long-lasting antibacterial properties of N-halamine polymeric coating can effectively prevent the bacterial adhesion of the inner wall of the waterline in the early stage, and the active chlorine in N-halamine coating can be recovered by regular washing with a simple sodium hypochlorite sterilized water to restore its antibacterial ability, which can prevent the establishment of bacteria biofilms, mitigate corrosion of waterlines and lengthen its service life in the long run (Figure 1).
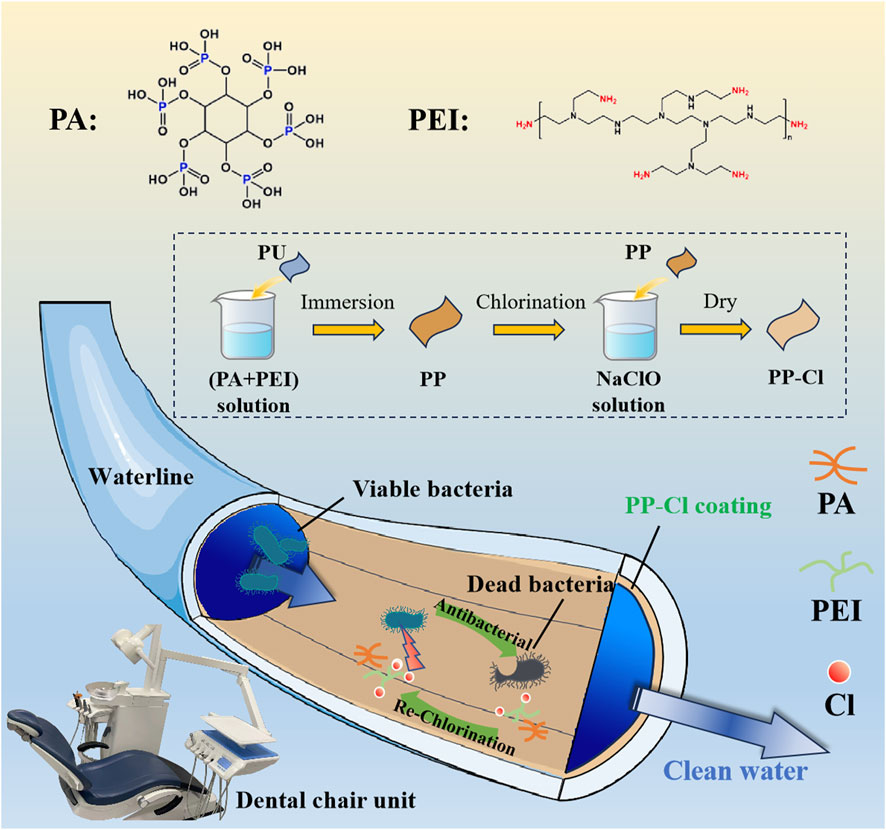
Figure 1. Design schematic of long-lasting renewable antibacterial N-halamine coating on dental unit waterlines to prevent and control oral infection.
2 Materials and methods
2.1 Materials
Polyurethane (PU) with a diameter of 12 mm and a thickness of 2 mm was purchased from LK Plastic Products Co., Ltd (Dongguan, China). Polyethylenimine (PEI), phytic acid (PA, 50% in water), Sodium iodide (NaI), hexamethyldisilazane (HMDS) and sodium thiosulfate were obtained from Aladdin Reagent Co., Ltd (Shanghai, China). Sodium hypochlorite (NaClO, 1%) was bought from Langli Biomedical Co., Ltd (Wuhan, China). Ethanol (C2H5OH) was procured from Lingfeng Chemical Reagents Co., Ltd (Shanghai, China). Starch was obtained from local markets. All the chemicals were analytical grade.
2.2 Coating preparation
A mixed solution of PA and PEI was prepared using ultrapure water (18.2 megohm-cm, Millipore) dissolution to a final concentration of 5 mg/L and 2.5 mg/L, respectively. Then the PU samples were immersed in the mixed solution for 4 h to form a PA/PEI co-deposition coating, note as PP coating. After drying the PP samples at room temperature, they were immersed in a 0.2% NaClO solution for 10 min and chlorinated to obtain the PP-Cl coating.
2.3 Surface characterization
Morphological observations of the samples were conducted using a scanning electron microscope (SEM, S-3400N, 15.0 kV, Hitachi, Japan). The chemical compositions of the samples were analyzed by an X-ray photoelectron spectroscopy (XPS, AXIS, Shimadzu, Japan). The surface contact angle of the samples was evaluated by a contact angle tester (SL200B, Solon, China).
2.4 Determination of oxidative chlorine (Cl+) content
The oxidative chlorine content of PP-Cl sample was quantified by the iodometric/thiosulfate titration procedure. In detail, the PP-Cl sample was immersed in 30 mL potassium iodide aqueous solution (1%) for 10 min to form I2. Then, 1 mL of 1% starch solution was added until the solution color changed to blue. The solution was titrated with sodium thiosulfate solution (the color of the solution changed from blue to colorless). The oxidative chlorine content was calculated according to the following formula:
Specifically, N is the concentration of the titrant sodium thiosulphate and V is its volume; S is the area of the sample (33.91 cm2).
2.5 Antibacterial properties evaluation in vitro
2.5.1 Antibacterial test
Gram-positive Staphylococcus aureus (S. aureus, ATCC 25923) and Gram-negative Escherichia coli (E. coli, ATCC 25922) were used to evaluate the antibacterial performance of the samples. The bacteria were cultured in standard Luria-Bertani (LB) for E. coli or Tryptic Soy Broth (TSB) for S. aureus at 37 °C with a constant temperature incubator. The samples were sterilized with 75% ethanol for 2 h. Bacterial suspensions with 1.0 ✕ 106 and 1.0 ✕ 107 CFU/mL were used for the antibacterial test. The samples were placed in a 24-well plate and then 500 μL bacterial suspension were introduced into each well. Subsequently, the samples were incubated at 37 °C for 6 h. Then the samples were placed in a test tube containing 4 mL sterile saline and vortexed to detach the bacteria. According to GB/T 4789.2, the diluted bacterial suspension was spread on agar plates and cultured at 37 °C for 18 h. The antibacterial rate was calculated according to the following formula:
Where, A and B are the number of bacterial colonies from control group (PU) and the experimental groups, respectively. The test was independently repeated three times.
2.5.2 Bacterial morphology observation
The bacterial morphology of S. aureus and E. coli on sample surface was observed by SEM. First, the samples were sterilized with 75% ethanol for 2 h. Then, the samples were placed in a 24-well plate and 100 μL bacterial suspension (107CFU/mL) were introduced onto each sample. Subsequently, the samples were incubated at 37°C for 6 h. The samples were then washed twice with phosphate buffer solution (PBS) and fixed in 2.5% glutaraldehyde solution for 4 h. The samples were dehydrated with graded ethanol solutions (30%, 50%, 75%, 90%, 95% and 100%, v/v) and continued to be dehydrated in a mixture of ethanol and HMDS (ethanol: HMDS = 2:1, 1:1 and 1:2, v/v) as well as 100% HMDS solution. The samples were sprayed with platinum before SEM observation.
2.6 Cytotoxicity evaluation in vitro
2.6.1 Cell culture
L929 mouse fibroblast cells (kindly provided by Cell Bank, Chinese Academy of Sciences) were cultured with α-MEM culture medium (Gibco, USA), supplemented with 10% fetal bovine serum (FBS, Gibco, USA) and 1% antimicrobial penicillin and streptomycin (Gibco, USA) in a cell incubator with 5% CO2 at 37 °C. The cell culture medium was refreshed every 2 days.
2.6.2 Cell viability assay
The extracts were prepared by immersing the samples in 3 mL α-MEM medium for 48 h, and then filtered with a bacterial membrane. Six parallel samples were set up for each group of extract. L929 cells were inoculated into 96-well cell culture plates with a density of 1 × 103 cells/well (n = 4), and incubated for 24 h. After that, the medium was changed to 100 μL fresh α-MEM with different extracts per well and incubated for 24 h and 48 h. Then the culture medium was replaced by 100 μL fresh medium with 10% alamarBlue™ (Thermo Fisher Scientific Inc., USA) in each well. The amount of reduced alamarBlue™ was detected with an excitation wavelength of 560 nm and emission wavelength of 590 nm using a microplate reader (BioTek Cytation 5, USA). The relative cell viability was calculated according to the following formula:
where,
2.6.3 Live/dead cell staining
A Live/Dead cell staining kit (Thermo Fisher Scientific Inc., USA) was used to evaluate the cell viability. L929 cells were introduced into 96-well cell culture plates with a density of 1 × 103 cells/well, and incubated for 24 h. The medium was then changed to 100 μL fresh α-MEM with different extracts and incubated for 24 and 48 h, respectively. Afterwards, samples were rinsed with PBS twice, and stained with 100 μL PBS containing calcein-AM and propidium iodide for 15 min at 37 °C. Finally, the L929 cells were observed by a fluorescence microscope (Olympus, Japan).
2.7 Statistical analysis
Data were expressed as means ± standard deviation (SD). Statistically significant differences (p) between groups were measured using the one-way analysis of the variance and Tukey’s multiple comparison tests. All the statistical analyses were determined with the GraphPad Prism statistical software. The difference was considered statistically significant at *p < 0.05, **p < 0.01, and ***p < 0.001.
3 Results and discussion
3.1 Characterization of N-halamine polymeric coating
In this work, an N-halamine polymer coating was prepared on the PU surface. PEI and PA formed a polyelectrolyte complex and deposited as a film, which was then chlorinated. Specifically, PEI has a high density of positive charge and can be used as a cationic polyelectrolyte (Lin et al., 2014; Bediako et al., 2021). PA, an eco-friendly natural compound, has strong negative charge and serves as anionic polyelectrolyte (Kong et al., 2023). The SEM morphologies of PU, PP, and PP-Cl are shown in Figure 2A. The PU surface is relatively smooth, while PP and PP-Cl surfaces have some protrusions and depressions, which may be due to the co-deposition of PA and PEI. Chlorination of the PP surface with NaClO solution did not cause significant changes in surface morphology of PP-Cl. In addition, the polar amino groups of PEI allow the coating to deposit stably on a variety of substrates, providing strong internal energy and allowing the coating to form hydrogen bonds and electrostatic interactions that anchor it to different substrates (Fan and Gong, 2021).
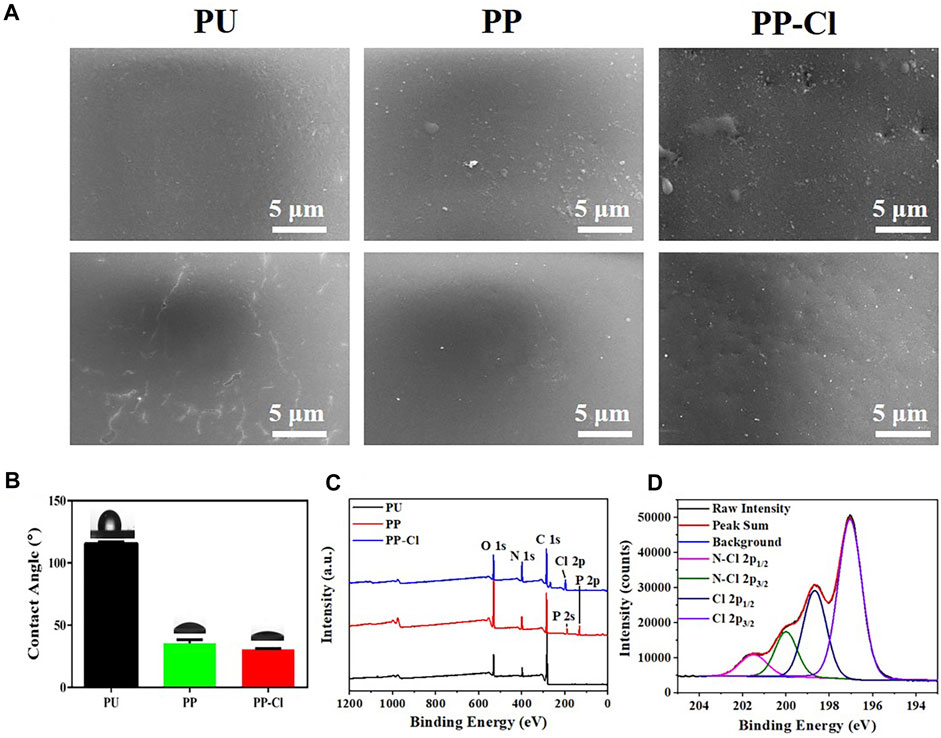
Figure 2. (A) SEM surface morphologies of PU, PP and PP-Cl; (B) Surface water contact angles of PU, PP, and PP-Cl; (C) XPS full spectra of PU, PP, and PP-Cl surfaces; (D) XPS high-resolution spectrum of Cl 2p from PP-Cl sample.
Furthermore, hydrophilic zwitterions present potential applications in preventing biofouling (Jiang and Cao, 2010). Therefore, we characterized the surface hydrophilicity of N-halamine polymeric coatings on the PU waterlines (Figure 2B; Table 1). The water contact angle of PU surface is 115.53 ± 1.44°. With the abundance of hydrophilic dihydrogen phosphate groups in PA (Bloot et al., 2023), the water contact angles of PP and PP-Cl surfaces are significantly reduced to 35.45 ± 2.84° and 30.63 ± 0.52°, respectively, rendering them hydrophilic. The incorporation of PA and PEI resulted in the formation of a hydration layer on the surface through electrostatic interaction or hydrogen bonding. As a result, the possibility of biofouling deposition on the surface and blocking of the active haloamine sites has been reduced, which advantageously contributes to the preservation of the coating’s cleanliness (Zeng et al., 2021).
To further confirm whether the N-halamine polymer coating has been successfully fabricated on the PU surface, we analyzed XPS full spectra of PU, PP, and PP-Cl as shown in Figure 2C. Due to the strong negative electricity of PA and the high positive charge density of PEI, a PA@PEI polyelectrolyte complex is formed on the PP surface. Hence, the XPS spectrum of PP shows a characteristic peak of P 2s and P 2p. After chlorination, an obvious Cl 2p characteristic peak appears in PP-Cl surface.
Studies have shown that N-halamines’ antibacterial mechanism mainly contains contact sterilization and release sterilization (Dong et al., 2017), and no matter which sterilization method, the main components of bactericidal action for N-halamines are active halogens (Wu et al., 2021). Therefore, active chlorine of PU, PP and PP-Cl was detected by XPS high-resolution spectrum and the results are presented in Figure 2D. The characteristic peaks attributed to N-Cl 2p1/2 and N-Cl 2p3/2 were observed at 201.5 eV and 200.0 eV, respectively, which indicates that active chlorine has been successfully loaded on the PP-Cl surface. In addition, the active chlorine content of the PP-Cl surface was quantified using iodometric/thiosulfate titration method. The blue starch solution gradually turns transparent after being titrated with sodium thiosulfate (Figure 3). The results of thrice measurements are shown in Table 2, and the active chlorine content is 0.200, 0.196, and 0.190 (μM/cm2), respectively, which suggests that the PP-Cl surface can release active chlorine.
3.2 Antibacterial test
Gram-negative Escherichia coli and Gram-positive Staphylococcus aureus were used to study the antibacterial performance of PP-Cl, PUand PP. Two bacterial inoculation densities (1.0 × 106 and 1.0 × 107 CFU/mL) were selected to simulate different degrees of bacterial infection scenarios. As shown in Figure 4, when the bacterial inoculation density was 1.0 × 106 CFU/mL, the PP and PP-Cl surfaces could significantly inhibit the growth of S. aureus and E. coli, with an antibacterial rate of 100% for both.
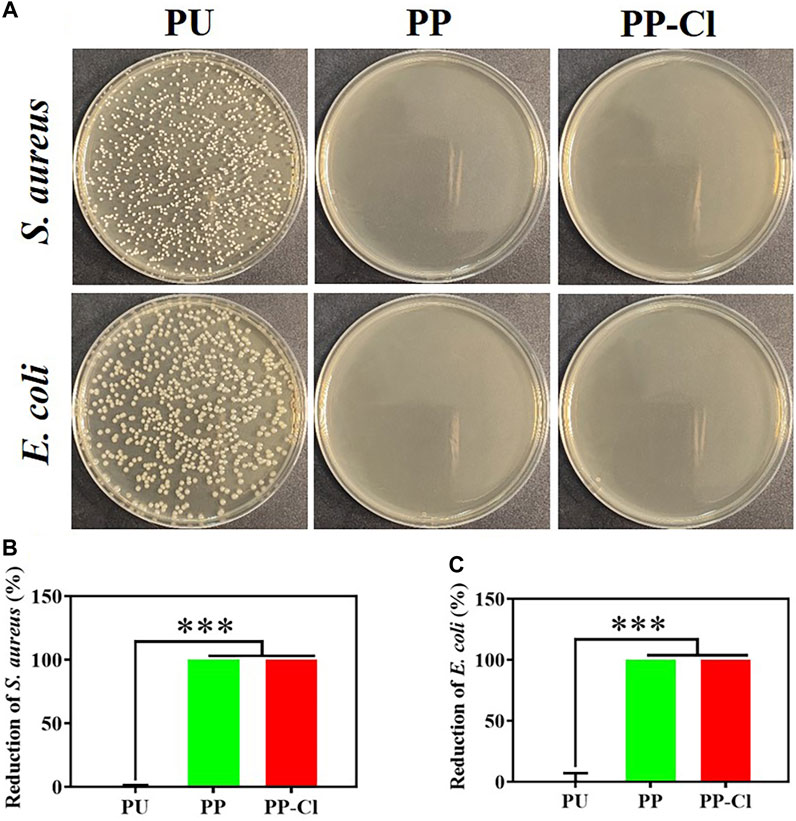
Figure 4. (A) Photographs of S. aureus and E. coli colonies (culture density of 106 CFU/mL) on agar culture plates of PU, PP and PP-Cl and the corresponding antibacterial rates of PU, PP and PP-Cl against S. aureus (B) and E. coli (C) based on the plate counting method.
As shown in Figure 5, when the bacterial inoculation density increased to 1.0 ✕ 107 CFU/mL, the antibacterial rates of PP and PP-Cl against S. aureus were 95.81 ± 0.27% and 99.99 ± 0.01%, respectively. The antibacterial rates of PP and PP-Cl against E. coli were 78.50 ± 2.01% and 100%, respectively. Furthermore, Figure 6 shows the effects of PU, PP, and PP-Cl surfaces on the morphology of S. aureus and E. coli. The S. aureus and E. coli on the PU surface maintained intact spherical and rod shapes, respectively, showing aggregated distribution. Compared to the PU surface, the number of bacteria significantly decreases on both the PP and PP-Cl surfaces, demonstrating that both PP and PP-Cl coatings are effective in preventing bacterial growth. Additionally, the PP-Cl surface showed the least amount of bacteria, along with noticeable damage to the bacterial structure, indicating a more potent antibacterial effect.
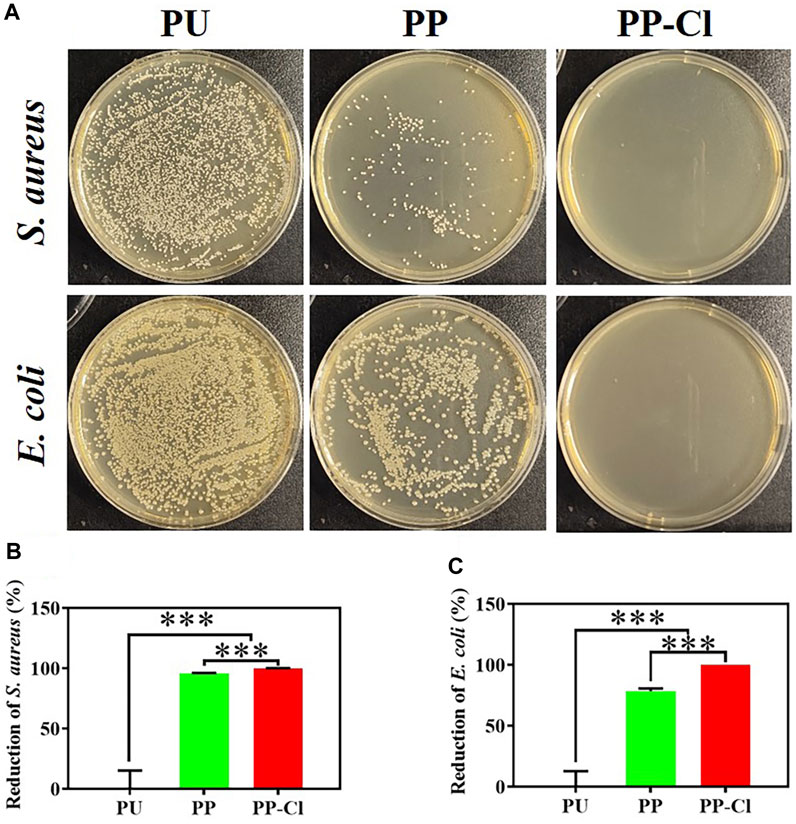
Figure 5. (A) Photographs of S. aureus and E. coli colonies (culture density of 107 CFU/mL) on agar culture plates of PU, PP and PP-Cl and the corresponding antibacterial rates of PU, PP and PP-Cl against S. aureus (B) and E. coli (C) based on the plate counting method.
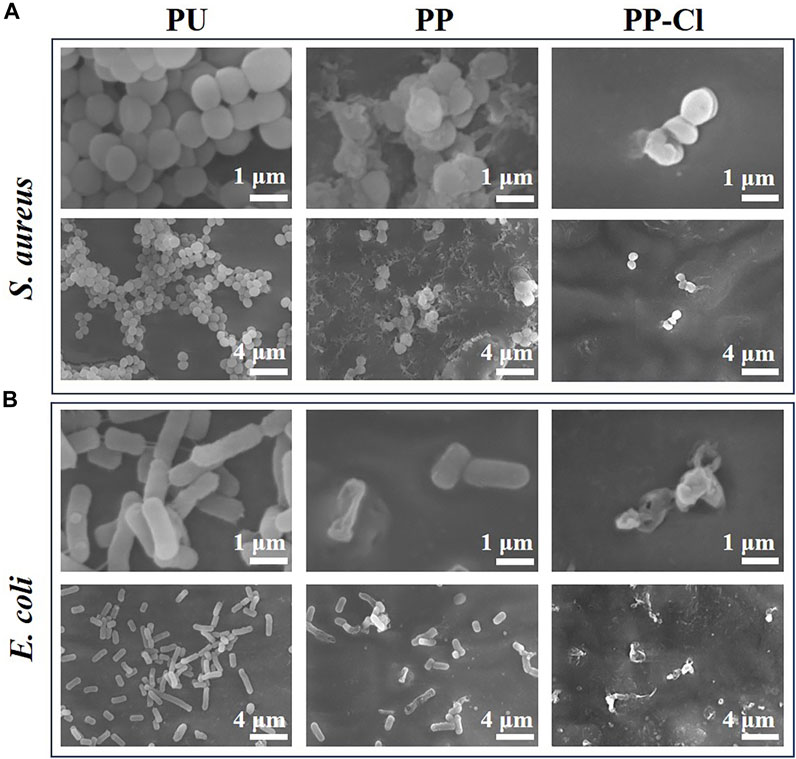
Figure 6. SEM bacteria morphologies of S. aureus (A) and E. coli (B) from PU, PP and PP-Cl at low and high magnification.
PEI has a high positive charge density, which makes it easy to contact negatively charged bacteria. Then it can destroy the bacterial cell wall and embed itself directly into the bacterial cell membrane, ultimately resulting in bacterial death (Giano et al., 2014). Therefore, PP surface without active chlorine also has a certain antibacterial effect. For PP-Cl surface, supported by active chlorine, it was more effective in severe bacterial infection scenarios, demonstrating a superior antibacterial effect. The PP-Cl surface with N atom in PEI can be repeatedly chlorinated by NaClO. To be specific, the strong oxidative ClO− attacks the electron-rich N atom in the secondary amine bond during the chlorination process. Thereafter, the shared electron is attracted to the N atom to generate positively charged Cl atoms, which ultimately creates the N-Cl bond (Wu et al., 2015). When bacterial infection occurs, the covalent bond between N and Cl is broken, leading to the release of active chlorine. Active chlorine exhibits strong oxidative properties, which enable it to oxidize and destroy bacterial structures (Yin et al., 2021). Besides, the cationic charge center may capture the bacteria with negatively charged shell and can transfer oxidative chlorine to the bacterial interior through electrostatic interaction (Li et al., 2012). This process can destroy the internal proteins and genetic materials of the bacteria (Si et al., 2017). Meanwhile, due to their non-specific interaction with bacterial proteins, N-halamines are considered sensitive to a broad range of bacteria and are unlikely to induce bacterial resistance.
3.3 Biocompatibility assessment
The therapeutic water passes through the waterlines and flows directly into the patient’s mouth. Histotoxic components can cause multiple complications such as inflammation and pain after treatment, so it is necessary to evaluate the biocompatibility of the surface coating. Mouse fibroblast cell line L929 were used to assess the cytocompatibility of PU, PP and PP-Cl extracts under immersion for 48 h. No noticeable difference in cell viability was observed among the surfaces at either time point, which means that the PP-Cl coating has good cell compatibility (Figures 7A, C). This was further confirmed by the live/dead cell staining results (Figures 7B, D), where a large numbers of live cells with green fluorescence could be observed on the PP-Cl surface, and the dead cells with red fluorescence could hardly be seen. This indicates that the PP-Cl coating prepared on the PU surface has good biocompatibility and biosafety.
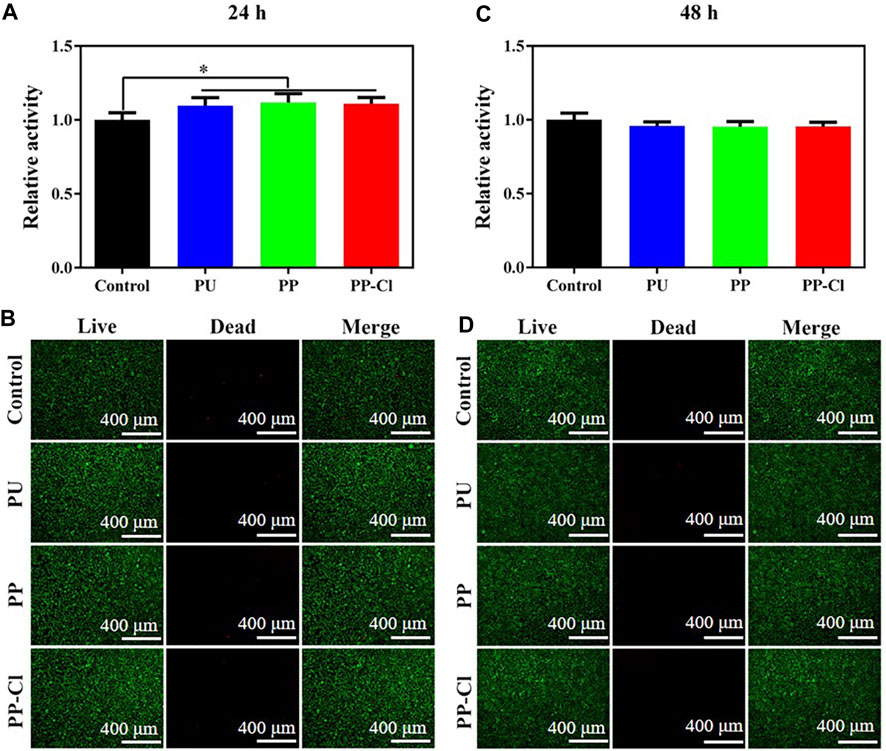
Figure 7. Cell viability (A) and Live/dead staining (B) of L929 cells co-cultured with extraction solution of PU, PP and PP-Cl for 24 h; Cell viability (C) and Live/dead staining (D) of L929 cells co-cultured with extraction solution of PU, PP and PP-Cl for 48 h.
The formation of bacterial biofilm in the waterline is a dynamic process, which generally goes through five stages: colonization stage of reversible adhesion of bacteria, agglomeration stage of irreversible adhesion, maturation stage of biofilm, and shedding and recolonization stage of bacteria (Wang et al., 2020). Bacterial adhesion is the first step to trigger surface infection of biological materials, and it is also the primary goal of targeted antimicrobial strategies. Therefore, surface coating modification is the simplest and most effective way to solve surface microbial contamination, and it can also reduce the frequency of use of chemical disinfectants, thus reducing corrosion damage to waterlines. Nowadays, many fungicides have been used for antimicrobial modification of biological materials, such as antibiotics, antimicrobial peptides, metal ions, polymeric quaternary ammonium salts and N-halamines (Pérez-Anes et al., 2015; Amin Yavari et al., 2016; Liu Z. et al., 2016). N-halamines have gained significant attention due to their potent antibacterial activity against a wide range of bacteria, long-lasting stability in water and dry conditions, renewability, biosafety and low cost (Wu et al., 2021). However, they have been rarely exploited for the antibacterial use of dental unit waterline.
Based on the above considerations, in the present work, an N-halamine coating was prepared on dental unit waterlines by forming polyelectrolyte complexes with PEI and PA, followed by chlorination. Mainly due to the bactericidal effect of active chlorine, N-halamine coating showed good antibacterial performance in both inhibiting the early bacterial adhesion and preventing the bacterial biofilms’ formation. In the process of sterilization, the active halogen of N-halamine polymeric coating will be gradually consumed. Yet, by simple rinsing with sodium hypochlorite disinfectant, it can be easily renewed with great antibacterial properties for the durable and renewable antibacterial requirements (Luo et al., 2011; Ma et al., 2019). Besides, N-halamine polymeric coatings showed good cell compatibility. Therefore, our results provide guidance for the design of long-term renewable antibacterial waterlines, and further help to save costs and lower the probability of hospital-acquired infections in the department of stomatology.
4 Conclusion
In summary, we prepared an N-halamine polymeric coating on the dental unit waterlines. This coating possesses durable and renewable antibacterial activity by repeated regeneration of active chlorine. N-halamine polymeric coating shows a hydrophilic surface due to the formation of a hydration layer through electrostatic interaction and hydrogen bonding, which can inhibit the deposition of biofouling and the obstruction of the active halide site on the waterlines, thereby helping to maintain the cleanliness of the coating. Moreover, the N-halamine polymeric coating shows significant antibacterial effects on E. coli and S. aureus, effectively inhibiting the formation of bacterial biofilms, which is mainly due to the high density of positive charge on the coating surface and the release of active chlorine. Besides, the coating showed good biocompatibility. Therefore, our work provides a new approach to designing durable and renewable antibacterial dental unit waterlines to prevent and reduce the incidence of hospital-acquired infection.
Data availability statement
The raw data supporting the conclusion of this article will be made available by the authors, without undue reservation.
Ethics statement
Ethical approval was not required for the studies on animals in accordance with the local legislation and institutional requirements because only commercially available established cell lines were used.
Author contributions
MX: Conceptualization, Data curation, Formal Analysis, Investigation, Methodology, Validation, Visualization, Writing–original draft. HZ: Investigation, Methodology, Software, Validation, Writing–review and editing. ZL: Conceptualization, Supervision, Writing–review and editing. LZ: Conceptualization, Funding acquisition, Resources, Supervision, Writing–review and editing. WQ: Conceptualization, Funding acquisition, Project administration, Resources, Supervision, Writing–review and editing.
Funding
The author(s) declare that financial support was received for the research, authorship, and/or publication of this article. This research was funded by Shanghai Municipal Health Commission (202040085) and Medical Key Subject of Xuhui District (SHXHZDXK202302).
Conflict of interest
The authors declare that the research was conducted in the absence of any commercial or financial relationships that could be construed as a potential conflict of interest.
Publisher’s note
All claims expressed in this article are solely those of the authors and do not necessarily represent those of their affiliated organizations, or those of the publisher, the editors and the reviewers. Any product that may be evaluated in this article, or claim that may be made by its manufacturer, is not guaranteed or endorsed by the publisher.
References
Abdouchakour, F., Dupont, C., Grau, D., Aujoulat, F., Mournetas, P., Marchandin, H., et al. (2015). Pseudomonas aeruginosa and achromobacter sp. clonal selection leads to successive waves of contamination of water in dental care units. Appl. Environ. Microbiol. 81 (21), 7509–7524. doi:10.1128/aem.01279-15
Amin Yavari, S., Loozen, L., Paganelli, F. L., Bakhshandeh, S., Lietaert, K., Groot, J. A., et al. (2016). Antibacterial behavior of additively manufactured porous titanium with nanotubular surfaces releasing silver ions. ACS Appl. Mater Interfaces 8 (27), 17080–17089. doi:10.1021/acsami.6b03152
Aprea, L., Cannova, L., Firenze, A., Bivona, M. S., Amodio, E., and Romano, N. (2010). Can technical, functional and structural characteristics of dental units predict Legionella pneumophila and Pseudomonas aeruginosa contamination? J. Oral Sci. 52 (4), 641–646. doi:10.2334/josnusd.52.641
Bediako, J. K., Choi, J.-W., Song, M.-H., Lim, C.-R., and Yun, Y.-S. (2021). Self-coagulating polyelectrolyte complexes for target-tunable adsorption and separation of metal ions. J. Hazard. Mater. 401, 123352. doi:10.1016/j.jhazmat.2020.123352
Bloot, A. P. M., Kalschne, D. L., Amaral, J. A. S., Baraldi, I. J., and Canan, C. (2023). A review of phytic acid sources, obtention, and applications. Food Rev. Int. 39 (1), 73–92. doi:10.1080/87559129.2021.1906697
Chien, H.-W., and Chiu, T.-H. (2020). Stable N-halamine on polydopamine coating for high antimicrobial efficiency. Eur. Polym. J. 130, 109654. doi:10.1016/j.eurpolymj.2020.109654
Coleman, D. C., O’Donnell, M. J., Shore, A. C., and Russell, R. J. (2009). Biofilm problems in dental unit water systems and its practical control. J. Appl. Microbiol. 106 (5), 1424–1437. doi:10.1111/j.1365-2672.2008.04100.x
Debiemme-Chouvy, C., and Cachet, H. (2018). Electrochemical (pre)treatments to prevent biofouling. Curr. Opin. Electrochem. 11, 48–54. doi:10.1016/j.coelec.2018.07.006
Dong, A., Wang, Y. J., Gao, Y., Gao, T., and Gao, G. (2017). Chemical insights into antibacterial N-halamines. Chem. Rev. 117 (6), 4806–4862. doi:10.1021/acs.chemrev.6b00687
Dutil, S., Veillette, M., Mériaux, A., Lazure, L., Barbeau, J., and Duchaine, C. (2007). Aerosolization of mycobacteria and legionellae during dental treatment: low exposure despite dental unit contamination. Environ. Microbiol. 9 (11), 2836–2843. doi:10.1111/j.1462-2920.2007.01395.x
Fan, H., and Gong, J. P. (2021). Bioinspired underwater adhesives. Adv. Mater. 33 (44), 2102983. doi:10.1002/adma.202102983
Giano, M. C., Ibrahim, Z., Medina, S. H., Sarhane, K. A., Christensen, J. M., Yamada, Y., et al. (2014). Injectable bioadhesive hydrogels with innate antibacterial properties. Nat. Commun. 5, 4095. doi:10.1038/ncomms5095
Hui, F., and Debiemme-Chouvy, C. (2013). Antimicrobial N-halamine polymers and coatings: a review of their synthesis, characterization, and applications. Biomacromolecules 14, 585–601. doi:10.1021/bm301980q
Jain, A., Duvvuri, L. S., Farah, S., Beyth, N., Domb, A. J., and Khan, W. (2014). Antimicrobial polymers. Adv. Healthc. Mater 3 (12), 1969–1985. doi:10.1002/adhm.201400418
Ji, X.-Y., Fei, C.-N., Zhang, Y., Zhang, W., Liu, J., and Dong, J. (2016). Evaluation of bacterial contamination of dental unit waterlines and use of a newly designed measurement device to assess retraction of a dental chair unit. Int. Dent. J. 66 (4), 208–214. doi:10.1111/idj.12225
Jiang, S., and Cao, Z. (2010). Ultralow-fouling, functionalizable, and hydrolyzable zwitterionic materials and their derivatives for biological applications. Adv. Mater. 22 (9), 920–932. doi:10.1002/adma.200901407
Kenawy, E. R., Worley, S. D., and Broughton, R. M. (2007). The chemistry and applications of antimicrobial polymers: a state-of-the-art review. Biomacromolecules 8 (5), 1359–1384. doi:10.1021/bm061150q
Kong, R., Ren, J., Mo, M., Zhang, L., and Zhu, J. (2023). Multifunctional antifogging, self-cleaning, antibacterial, and self-healing coatings based on polyelectrolyte complexes. Colloids Surfaces A Physicochem. Eng. Aspects 656, 130484. doi:10.1016/j.colsurfa.2022.130484
Li, L., Pu, T., Zhanel, G., Zhao, N., Ens, W., and Liu, S. (2012). New biocide with both N-chloramine and quaternary ammonium moieties exerts enhanced bactericidal activity. Adv. Healthc. Mater 1 (5), 609–620. doi:10.1002/adhm.201200018
Lin, Z., Chang, J., Zhang, J., Jiang, C., Wu, J., and Zhu, C. (2014). A work-function tunable polyelectrolyte complex (PEI:PSS) as a cathode interfacial layer for inverted organic solar cells. J. Mater. Chem. A 2 (21), 7788–7794. doi:10.1039/c4ta00289j
Liu, Y., He, Q., Li, R., Huang, D., Ren, X., and Huang, T.-S. (2016a). Durable antimicrobial cotton fabrics treated with a novel N-halamine compound. Fibers Polym. 17 (12), 2035–2040. doi:10.1007/s12221-016-6776-1
Liu, Z., Ma, S., Duan, S., Xuliang, D., Sun, Y., Zhang, X., et al. (2016b). Modification of titanium substrates with chimeric peptides comprising antimicrobial and titanium-binding motifs connected by linkers to inhibit biofilm formation. ACS Appl. Mater Interfaces 8 (8), 5124–5136. doi:10.1021/acsami.5b11949
Lizzadro, J., Mazzotta, M., Girolamini, L., Dormi, A., Pellati, T., and Cristino, S. (2019). Comparison between two types of dental unit waterlines: how evaluation of microbiological contamination can support risk containment. Int. J. Environ. Res. Public Health 16 (3), 328. doi:10.3390/ijerph16030328
Luo, J., Porteous, N., and Sun, Y. (2011). Rechargeable biofilm-controlling tubing materials for use in dental unit water lines. ACS Appl. Mater Interfaces 3 (8), 2895–2903. doi:10.1021/am200576q
Ma, Y., Li, J., Si, Y., Huang, K., Nitin, N., and Sun, G. (2019). Rechargeable antibacterial N-halamine films with antifouling function for food packaging applications. ACS Appl. Mater Interfaces 11 (19), 17814–17822. doi:10.1021/acsami.9b03464
O’Donnell, M. J., Boyle, M. A., Russell, R. J., and Coleman, D. C. (2011). Management of dental unit waterline biofilms in the 21st century. Future Microbiol. 6 (10), 1209–1226. doi:10.2217/fmb.11.104
Pankhurst, C. L., and Coulter, W. A. (2007). Do contaminated dental unit waterlines pose a risk of infection? J. Dent. 35 (9), 712–720. doi:10.1016/j.jdent.2007.06.002
Pérez-Anes, A., Gargouri, M., Laure, W., Van Den Berghe, H., Courcot, E., Sobocinski, J., et al. (2015). Bioinspired titanium drug eluting platforms based on a poly-β-cyclodextrin–chitosan layer-by-layer self-assembly targeting infections. ACS Appl. Mater Interfaces 7 (23), 12882–12893. doi:10.1021/acsami.5b02402
Ramalingam, K., Frohlich, N. C., and Lee, V. A. (2013). Effect of nanoemulsion on dental unit waterline biofilm. J. Dent. Sci. 8 (3), 333–336. doi:10.1016/j.jds.2013.02.035
Ritter, A. V., Ghaname, E., and Leonard, R. H. (2007). The influence of dental unit waterline cleaners on composite-to-dentin bond strengths. J. Am. Dent. Assoc. 138 (7), 985–991. doi:10.14219/jada.archive.2007.0296
Schönning, C., Jernberg, C., Klingenberg, D., Andersson, S., Pääjärvi, A., Alm, E., et al. (2017). Legionellosis acquired through a dental unit: a case study. J. Hosp. Infect. 96 (1), 89–92. doi:10.1016/j.jhin.2017.01.009
Shajahan, I., Kandaswamy, D., Lakshminarayanan, L., and Selvarajan, R. (2017). Substantivity of hypochlorous acid-based disinfectant against biofilm formation in the dental unit waterlines. J. Conservative Dent. 20 (1), 2–5. doi:10.4103/0972-0707.209076
Si, Y., Li, J., Zhao, C., Deng, Y., Ma, Y., Wang, D., et al. (2017). Biocidal and rechargeable N-halamine nanofibrous membranes for highly efficient water disinfection. ACS biomaterials Sci. Eng. 3 (5), 854–862. doi:10.1021/acsbiomaterials.7b00111
Vincent, A. T., Charette, S. J., and Barbeau, J. (2018). Unexpected diversity in the mobilome of a Pseudomonas aeruginosa strain isolated from a dental unit waterline revealed by SMRT Sequencing. Genome 61 (5), 359–365. doi:10.1139/gen-2017-0239
Wang, C., Mu, C., Lin, W., and Xiao, H. (2020). Functional-modified polyurethanes for rendering surfaces antimicrobial: an overview. Adv. Colloid Interface Sci. 283, 102235. doi:10.1016/j.cis.2020.102235
Watanabe, A., Tamaki, N., Yokota, K., Matsuyama, M., and Kokeguchi, S. (2016). Monitoring of bacterial contamination of dental unit water lines using adenosine triphosphate bioluminescence. J. Hosp. Infect. 94 (4), 393–396. doi:10.1016/j.jhin.2016.08.001
Williams, J. F., Johnston, A. M., Johnson, B., Huntington, M. K., and Mackenzie, C. D. (1993). Microbial contamination of dental unit waterlines: prevalence, intensity and microbiological characteristics. J. Am. Dent. Assoc. 124 (10), 59–65. doi:10.14219/jada.archive.1993.0205
Wu, D., Martin, J., Du, J. R., Zhang, Y., Lawless, D., and Feng, X. (2015). Effects of chlorine exposure on nanofiltration performance of polyamide membranes. J. Membr. Sci. 487, 256–270. doi:10.1016/j.memsci.2015.02.021
Wu, S., Xu, J., Zou, L., Luo, S., Yao, R., Zheng, B., et al. (2021). Long-lasting renewable antibacterial porous polymeric coatings enable titanium biomaterials to prevent and treat peri-implant infection. Nat. Commun. 12 (1), 3303. doi:10.1038/s41467-021-23069-0
Yin, X., Zhang, J., Xu, J., Tian, M., Li, L., Tan, L., et al. (2021). Fast-acting and highly rechargeable antibacterial composite nanofibrous membrane for protective applications. Compos. Sci. Technol. 202, 108574. doi:10.1016/j.compscitech.2020.108574
Zeng, X., Lin, J., Cai, W., Lu, Q., Fu, S., Li, J., et al. (2021). Fabrication of superhydrophilic PVDF membranes by one-step modification with eco-friendly phytic acid and polyethyleneimine complex for oil-in-water emulsions separation. Chemosphere 264, 128395. doi:10.1016/j.chemosphere.2020.128395
Keywords: dental unit waterlines, N-halamine coating, antibacterial, renewable, polyurethane
Citation: Xing M, Zhang H, Li Z, Zhang L and Qian W (2024) Long-lasting renewable antibacterial N-halamine coating enable dental unit waterlines to prevention and control of contamination of dental treatment water. Front. Mater. 11:1399597. doi: 10.3389/fmats.2024.1399597
Received: 12 March 2024; Accepted: 07 May 2024;
Published: 21 May 2024.
Edited by:
Luca Valentini, University of Perugia, ItalyReviewed by:
Gianluigi Cardinali, University of Perugia, ItalyGuomin Wang, City University of Hong Kong, Hong Kong SAR, China
Copyright © 2024 Xing, Zhang, Li, Zhang and Qian. This is an open-access article distributed under the terms of the Creative Commons Attribution License (CC BY). The use, distribution or reproduction in other forums is permitted, provided the original author(s) and the copyright owner(s) are credited and that the original publication in this journal is cited, in accordance with accepted academic practice. No use, distribution or reproduction is permitted which does not comply with these terms.
*Correspondence: Wenhao Qian, pingyanlaoto@163.com; Ling Zhang, tracey_ling@163.com; Zhiyao Li, sxtyyaoyao@163.com
†These authors have contributed equally to this work