- 1Department of Forest Sciences, University of Helsinki, Helsinki, Finland
- 2Finnish Environment Institute, Helsinki, Finland
- 3Helsinki Institute of Life Science (HiLIFE), University of Helsinki, Helsinki, Finland
- 4AFRY Management Consulting Oy, Vantaa, Finland
- 5Natural Resources Institute Finland (Luke), Helsinki, Finland
This study investigates the carbon offset potential in Espoo, Finland, by comparing a construction-impacted deforestation site with a larger conserved forest area. Addressing a knowledge gap in localized forest conservation as a CO2 offset method, our research quantifies the carbon stock and sequestration impacts under both baseline and alternative scenarios for the two study sites. The baseline scenario for offset site reflects standard forest management practices, while the alternative scenario involves complete forest conservation without active management. Our findings reveal that the conserved forest (79 ha), dominated by Norway spruce (Picea abies) and Scots pine (Pinus sylvestris), increased its carbon stock by 26 Mg C ha−1 in soil and 65 Mg C ha−1 in biomass. This enhancement is sufficient to compensate for the smaller deforestation site’s (19 ha), also containing a mix of Norway spruce and Scots pine, stock loss of 186 Mg C ha−1 in soil and 43 Mg C ha−1 in biomass. Furthermore, this study illuminates the complexities of CO2 compensation regulation and emphasizes the necessity for robust, transparent carbon accounting practices. The insights offer a valuable perspective on integrating nature-based solutions in urban planning to achieve broader ecological and climate goals.
1 Introduction
Urbanization profoundly influences the global carbon cycle, often escalating CO2 emissions. This surge primarily stems from vegetation clearing and soil translocation during urban development, releasing stored carbon, and accelerating climate change. Additionally, urbanization threatens biodiversity through the destruction of natural habitats, contributing to habitat fragmentation, decreasing species diversity, and potential ecosystem service losses. As per recent population projections made by the United Nations, the proportion of the global population residing in urban environments is anticipated to increase from the present 55 percent to 68 percent by 2050 (United Nations, 2022). This surge in urban dwellers translates to an additional 2.5 billion individuals, necessitating extensive urban development. Careful city planning can mitigate the land-use effects of urbanization, but in addition there is a need for new offset and compensation strategies.
IPCC attributes 10%–15% of global greenhouse gas emissions to land-use changes (IPCC, 2022), and urban expansion has been estimated to account for approximately 5% of these emissions (Seto et al., 2012; Churkina, 2016). The Intergovernmental Panel on Climate Change (IPCC) said in its April 2022 report, focusing on mitigating climate change, that limiting warming to 1.5 C requires, in addition to emission reductions, around 6 GtCO2 of carbon dioxide removals per year by 2050 (IPCC, 2022). Finland presents a unique scenario, with about 55% of total forest loss between 2000 and 2021 attributed to urbanization, contrasting starkly with global averages (Statistics Finland, 2022; UNFCCC, 2023).
Finland’s recent commitment to climate neutrality by 2035 (Finland’s Climate Change Act, 2022) and similar objectives by Finnish municipalities (Karhinen et al., 2021) highlight the pressing need to examine urban development’s environmental impact. Municipalities are increasingly considering carbon compensation measures like forest conservation as a strategy to offset urbanization-caused emissions (Hildén et al., 2023). Forestry related initiatives have been proposed as a potential compensation measure for environmental damage from urban development also in the literature (Skärbäck, 2007; Liu and Li, 2012; Strohbach and Haase, 2012; Russo et al., 2015; Jo et al., 2019; Pascoe et al., 2019). Safeguarding existing forests or undertaking afforestation initiatives are considered potential strategies for increasing carbon sequestration and stock, thereby serving as CO2 offsets for greenhouse gas emissions resulting from urban development.
In spite of the broad acknowledgment of urbanization’s impacts and preliminary countermeasures like forest conservation, there remain significant knowledge gaps. While prior research has explored the climate impacts of deforestation and forests’ carbon sequestration role (see, e.g., Dixon et al., 1994; IPCC, 1995; IPCC, 2007; McDonald et al., 2008; Griscom et al., 2017), uncertainties persist regarding the feasibility and quantification of using localized forest conservation as a CO2 offset method for urbanization-induced carbon losses. Global case studies demonstrating implemented conservation projects are limited, and research on the practicalities of municipalities or cities leveraging forest conservation for quantifiable carbon offsets is sparse. The domain remains in development phase, with established international agreements, such as Kyoto Protocol and Paris Agreement, underscoring emission reduction and carbon markets (Falkner, 2016; Rogelj et al., 2021). However, complexities arise due to regulatory framework, like potential CO2 offset double-counting, and the precise activities that public bodies can claim as CO2 compensation remain ambiguous (Pauw et al., 2018; Dubash et al., 2018; Haya et al., 2020; Rogelj et al., 2021).
In this paper, we incorporate a critical dimension of environmental economics: discounting environmental benefits, or, namely, carbon values. Discounting in environmental compensation and offset studies has been a focal point of discussion when addressing the time value of ecosystem services. The principle behind discounting carbon values is rooted in the immediacy of climate action. Given the heightened risks associated with nature-based solutions, such as the potential for premature carbon release due to natural disturbances, and considering the global urgency to reach net zero emissions by 2050, near-term sequestration is greatly more desired than delayed climate actions (Johnston and van Kooten, 2015). While deforestation results in immediate carbon losses, the conservation benefits expected from the offset site may span a century, especially when adhering to CO2 offset credit requirements for permanence (Allen et al., 2020).
The overall aim of this study was to examine the carbon dynamics of forest biomass and soil associated with an urban development initiative and a proposed forest conservation in Espoo, Finland. This study offers insights on the potential effectiveness of forest conservation as an offset tool against the repercussions of urbanization, while acknowledging the complexities in the prevailing CO2 compensation regulation and carbon accounting frameworks. Objectives of the study are threefold. First, we quantify the impacts of the anticipated construction project on carbon stock and sequestration. Following that, we aim to project the possible enhancements in carbon stock and sequestration that could be achieved by conservation of a mature forest ecosystem in another location. Conclusively, the research evaluates the practicality and inherent uncertainties of utilizing the modelled conservation area as a carbon offset based on the current regulatory landscape.
Following the introduction section, the remaining sections of the paper are arranged as follows. Second part synthesizes existing scholarship on CO2 compensation regulation, with an emphasis on the Finnish context, setting the stage for a deeper exploration of the subject matter and employed case study in Finland. The third section contains methodology and details the research design, tools, and analytical approaches utilized. The fourth section presents the empirical results derived from the research. Fifth section critically examines these findings in the discussion segment, where their wider implications are explored. The paper culminates in the conclusion section.
2 Methodology
2.1 Case study sites
The empirical investigation in this study revolves around two distinct forest areas located in Espoo, Finland, propelled into focus by a collaborative data center and energy project between Microsoft and Fortum announced in March 2022. The proposed project initiated a plan to compensate impacts of deforestation by a new forest conservation area. The planned deforestation and conservation site are situated within approximately 5.5–6 km of each other (Figures 1, 2).
The deforestation site (Hepokorvenkallio) designated for data center construction, encompassing roughly 19 ha, includes a diverse mix of sub-areas, ranging from drained peatland containing Norway spruce (Picea abies (L.) H. Karst.) to Scots pine (Pinus sylvestris L.) dominated less fertile peatland and upland forest (Ympäristösuunnittelu, 2017). The forest primarily comprises middle-aged and mature stands, as detailed in Supplementary Table S1 showing current standing forest stock.
The offset site (Hynkänlampi), sprawling over 79 ha, is under the ownership of city of Espoo. The area is officially designated as a recreation area in the current general zoning plan of the city. The Espoo environmental conservation program (LuonTo) underscores its value in maintaining ecological networks, which means that it has not been identified to have immediate nature conservation value, but nevertheless, it is an important area in terms of wider ecological connectivity and natural diversity. Supplementary Table S2 displays the current standing forest stock, which is particularly characterized by a predominance of mature trees, illustrated by the fact that approx. 65% of the stock is over 80 years old, with the highest volumes in the 81–120-year bracket. The offset site forest is partly naturally regenerated stands of managed and commercially exploited forests.
2.2 Data collection
Stand-level forest inventory data, containing also detailed information on stand ages, used in forest simulations was obtained from the Environmental Protection Services department of the City of Espoo. The data was downloaded and saved into XML data format for processing.
Information concerning the future construction and development of the deforestation site are based on initial high level zoning plans made by the city, as definitive building constructions plans will be known only after detailed building design has been done. It is worth noting that the detailed construction plans could significantly influence the assumptions and analysis of soil carbon stocks (i.e., the extent of the impact on soil, the depth of digging during construction, etc.). As of this writing, the forest at the deforestation site remains untouched and the land has not been cleared.
2.3 Data analysis and forest simulations
The study was conducted as a computer-based forest simulation over a 100-year period by using software IPTIM (AFRY Management Consulting Oy, Finland).
The applied IPTIM version uses Motti-model (Hynynen et al., 2002) for forest growth, Yasso15-model (Tuomi et al., 2011) for soil carbon dynamics, and a variety of biomass models for the carbon stock in the growing stock biomass and undergrowth. These models allow detailed description of development of growing stock structure, and, for example, accounting of diverse impacts from different stand thinning types. Carbon stock in the growing stock biomass and understored vegetation is calculated with biomass models (Muukkonen and Mäkipää, 2006; Helmisaari et al., 2007; Repola et al., 2007) within the IPTIM-software. Biomass models result in mass of dry matter, from which carbon is assumed to represent 47% (IPCC, 2019).
The Motti-models for forest growth are calibrated using data from the National Forest Inventory (VMI) provided by the Natural Resources Institute Finland (Luke). This calibration ensures that the models accurately reflect the current growth conditions of the forests. The Yasso15-model for soil carbon dynamics utilizes 13 aggregated areas within Finland to derive initial conditions based on forest stand and location information (Tuomi et al., 2011).
The forest carbon accounting methodology applied in this study follows the good practices, current industry standard, in estimating forest carbon emissions and removals which currently rely heavily on the IPCC Guidelines for National GHG Accounting (2006), and Refinement from 2019) (IPCC, 2019). Modelling of the biomass and soil carbon stock growth meets the general requirements of the highest level of accuracy (Tier 3).
With respect to the simulated scenarios, two (baseline and alternative) were formed for both sites:
• Deforestation site baseline: no construction, normal forest management following the ‘Best practices for sustainable forest management in Finland’ -guidelines (Ministry of Agriculture and Forestry of Finland, 2023).
• Deforestation site alternative scenario: land-use change and building construction as planned.
• Offset site baseline: normal forest management following the ‘Best practices for sustainable forest management in Finland’ -guidelines.
• Offset site alternative scenario: forest conservation, no management.
The best practices for sustainable forest management are offered as a service by the Ministry of Agriculture and Forestry to Finnish forest owners and professionals and are part of the national forest strategy implementation (Ministry of Agriculture and Forestry of Finland, 2023). The best practice guidelines are published and made available for general public by Tapio Ltd. (Tapio, 2023). These can be considered as a so-called regional baseline setting, as defined by Oldfield et al. (2021).
The establishment of baseline and alternative scenarios is an indispensable step within carbon offset initiatives, especially those relating to forestry (Michaelowa, 2009). These scenarios function as a quantitative benchmark, enabling the determination of the net carbon sequestration or emission resulting from the project, as compared to ‘business-as-usual’ situation. Without the comparison of baseline and alternative scenarios, it would be impossible to validate that the CO2 emission reductions a project is achieving are additional, a key component of CO2 offsetting (Allen et al., 2020; Laine et al., 2023).
The impact of construction at deforestation site on soil organic carbon was separately simulated using carbon balance calculator developed through the Climate Resilient City (ILKKA) project. The tool enables assessment of land use impacts on vegetation and soil carbon stocks (Rasinmäki and Känkänen, 2014). The carbon balance calculator, an Excel-based model, is freely accessible online (Ilmastonkestävän kaupungin suunnitteluopas, 2023).
In our offset and compensation calculations, we employ average values rather than singular data points, such as those from the end of the simulation period. This approach offers a more comprehensive picture by accounting for fluctuations over time, thereby providing a truer representation of the forest’s carbon stock performance (Pan et al., 2011). These averages are derived from the annual carbon stock values, both for undiscounted and discounted figures.
2.4 Discounting carbon values
Suitable discount rate has been a topic of extensive debate among scholars. An illuminating survey involving 197 expert climate economists found that the mean preferred long-term discount rate was 2.25% (Howard and Sylvan, 2015). Notably, the consensus among most respondents revolved around a range between 1% and 3%, with only a marginal group endorsing higher rates. In alignment with this, the IPCC has cautioned against the application of steep rates, asserting that there’s no empirical backing to justify rates as high as 5% for climate-centric analyses (Kolstad et al., 2014). The U.S. Federal Interagency Working Group on the Social Cost of Greenhouse Gases (IWG) has employed three discount rates (2.5%, 3%, and 5%) in their assessments of future climatic damages (National Academies of Sciences and Medicine, 2017). Historically, in significant deforestation instances like the Australia-based Strongman mine offset case, a 1% rate was the choice of preference in their offset assessment (von Hase, 2014).
Considering the academic debate and practical implications, this paper adopts a multi-faceted approach. We present our findings using three distinct discount rates: 1%, 3%, and 5%. These values were derived using the subsequent formula:
Where i denotes discount rate and n the number of periods between the present time and future time point of the carbon stock value in question.
To address the economic implications of carbon stocks, we also calculated the net present value (NPV) of these elements using the Social Cost of Carbon (SCC) and discount rate assumptions (1.5%, 2.0% and 2.5%) developed by the U.S. Environmental Protection Agency (EPA) (U.S. Environmental Protection Agency (EPA), 2023). This approach considers the increasing SCC over time due to escalating climate damages, providing a comprehensive economic evaluation. By integrating the EPA’s latest SCC estimates, we ensure our analysis reflects both current and future economic impacts of carbon dynamics in the study systems (U.S. Environmental Protection Agency (EPA), 2023).
3 Results
3.1 Deforestation site
At the onset of the simulation in 2022, the estimated biomass carbon stock of the planned deforestation site stands at 1,253 Mg C (approx. 67 Mg C ha-1), while the soil carbon stock is notably higher at 4,419 Mg C (approx. 235 Mg C ha-1) (Figure 3). In the baseline scenario, where the forest is managed according to the ‘best practices for sustainable forest management’ guidelines, biomass carbon fluctuates between 650-1,150 Mg C over the simulation period of 100 years, averaging 813 Mg C (approx. 43 Mg C ha-1). These dynamics reflect gradual biomass accumulation, mortality and harvested yields as forest stands mature and undergo thinning. Soil carbon stock sees a slight decline, decreasing from 4,419 Mg C (approx. 235 Mg C ha-1) in 2022 to 3,972 Mg C (approx. 210 Mg C ha-1) in 2122. This minor decrease is attributable to modeled thinning operations and related disturbance impacts. Soil carbon stock averages 4,149 Mg C (approx. 220 Mg C ha-1) over the entire 100-year simulation horizon.
Results from the deforestation scenario reveal that, over the simulation period of 100 years, the total carbon stock averages at 647 Mg C (approx. 35 Mg C ha-1). The biomass carbon stock would be completely lost due to deforestation, causing the immediate release of the entire 1,253 Mg C (approx. 67 Mg C ha-1) initially stored in the biomass (Figure 3). Consequently, soil carbon stock represents the entire carbon stock at the deforestation site within the alternative scenario. The soil carbon stock remains constant over the century, premised on the simulation’s assumption that soil carbon remains unchanged beneath constructed buildings. This simplification is due to a lack of detailed analysis regarding specific construction plans and building types at this stage. Over the 100-year simulation period, the deforestation scenario projects a carbon stock loss of 4,315 Mg C (approx. 230 Mg C ha-1) when compared against preserving the managed forest ecosystem (baseline) (Table 1).
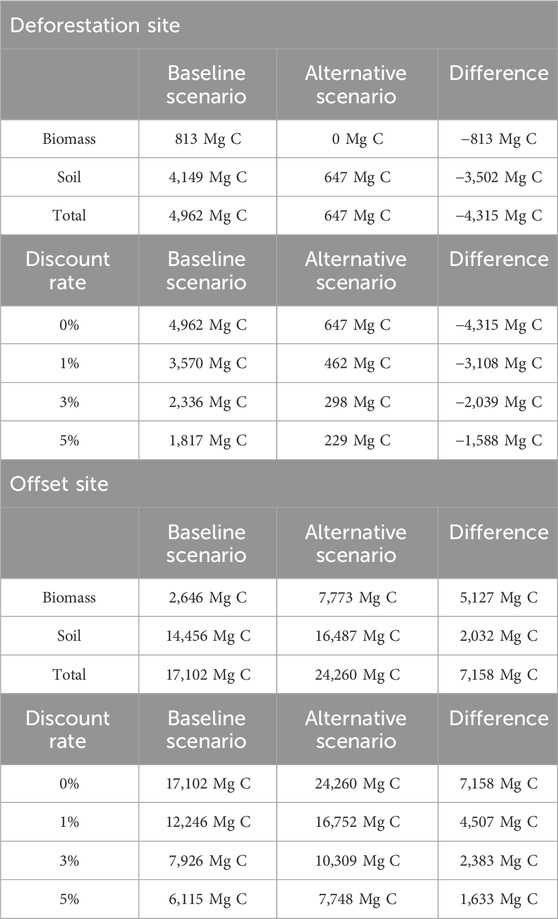
Table 1. Differences between simulated carbon stocks under the baseline and the alternative scenarios at deforestation site and offset site. Values for the two scenarios are averages for the simulation horizon of 100 years. Difference column values are calculated as alternative–baseline.
Alongside the non-discounted carbon stock projections, values adjusted for time preference regarding carbon sequestration and stock (discounted values) were computed (Table 1). Four discount rates were applied, ranging from 0% to 5%, where 0% rate reflects non-discounted values. With a 1% discount rate, the net carbon stock differential between the alternative scenario and the baseline narrows to 3,108 Mg C over the century-long modeling period. At a 3% discount rate this difference diminishes further to 2,039 Mg C, and with a 5% rate it drops to 1,588 Mg C.
Simulations also charted the trajectories of net carbon sinks for biomass. Figure 4 presents results under the baseline scenario, which assumes that deforestation and construction activities do not take place. The onset of the analysis period in 2023 sees a substantial decrease of carbon stocks with values plummeting to −530 Mg C (approx. −28 Mg C ha-1), resulting from large-scale thinning projections at the beginning of the simulation period. These early harvests are indicative of the currently relatively old stands in deforestation site (refer to Table 1 in Supplementary Materials for details). In the medium term, the rate of carbon stock change is anticipated to fluctuate between approximately −30 and +31 Mg C annually. This fluctuation illustrates the effects of periodic thinning and harvesting interventions, which intermittently reduce net sequestration as stands are managed.
During the full simulation period for the baseline scenario, the cumulative sum of annual biomass carbon stock changes is estimated to yield −596 Mg C (approx. −32 Mg C ha-1) by 2122, indicating carbon loss (mathematical sum of all the annual carbon sequestrations, natural mortality and management activities between 2023 and 2122). These results provide a reference point for evaluating the compensation required to offset the lost stock and sequestration opportunities due to deforesting activities.
3.2 Offset site
At the commencement of the simulation period, combined biomass and soil carbon stock is estimated to be 20,629 Mg C (approx. 260 Mg C ha-1) (Figure 5). Soil carbon stock constitutes the majority at 15,289 Mg C (approx. 195 Mg C ha-1), whereas aboveground biomass carbon stock amount to 5,340 Mg C (approx. 70 Mg C ha-1).
The baseline scenario, where forest management was continued, presents an initial downturn in biomass carbon, a direct consequence of the removal of mature timber (refer to Table 2 in Supplementary Materials). However, by the 2070s, notable biomass recovery is anticipated. Throughout the simulation, biomass carbon stock fluctuates between 1,900–3,500 Mg C due to recurring harvests. Soil organic carbon exhibits a gradual decline, from 15,289 Mg C (approx. 195 Mg C ha-1) in 2022 to 13,312 Mg C (approx. 170 Mg C ha-1) by 2122. By the end of 2122, projections based on the baseline harvesting strategy indicate a total carbon stock of 16,279 Mg C (approx. 210 Mg C ha-1). On average, the carbon stock combining both biomass and soil carbon under the baseline scenario amounts to 17,102 Mg C (approximately 215 Mg C ha-1).
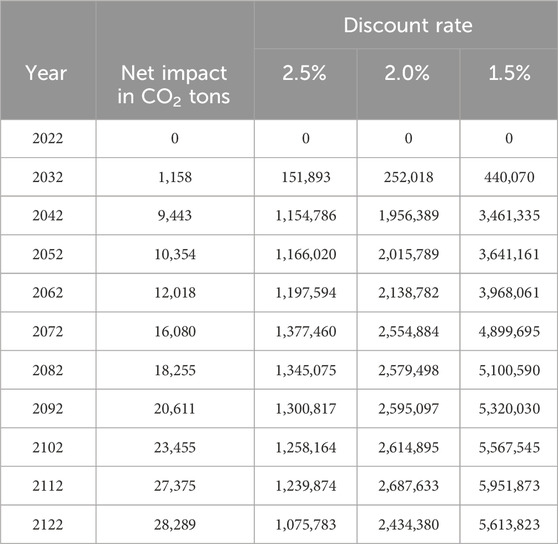
Table 2. Discounted USD values of the offset project in 10-year intervals. Present value year and dollar value year in calculations is 2022. Net impact in CO2 tons column values are calculated as the offset site difference (alternative–baseline) minus the deforestation site difference (alternative–baseline). Refer to Table 1 or Section 2.3 for further explanation on how the net difference has been calculated based on the baseline and alternative scenarios. 2022 is the year of forest inventory and the initial starting point of the forest simulations, thus the values are zero.
The alternative scenario, focusing on forest conservation, paints a contrasting picture compared to the baseline scenario (Figure 5). In the absence of timber harvests, aboveground biomass carbon accumulates, reaching 9,946 Mg C (approx. 130 Mg C ha-1) by 2122, from the initial 5,340 Mg C (approx. 70 Mg C ha-1). The uninterrupted forest cover allows soil organic carbon to rise, culminating at 18,030 Mg C (approx. 230 Mg C ha-1) by 2122. Consequently, forest conservation is projected to result in 27,976 Mg C (approx. 350 Mg C ha-1) of carbon stock by 2122, contrasting significantly with the 16,279 Mg C (approx. 210 Mg C ha-1) under the baseline scenario. Average carbon stock for the combined biomass and soil carbon equals 24,260 Mg C (approx. 310 Mg C ha-1), also greatly differing from the baseline scenario.
Table 1 offers a comparative view of the carbon stock outcomes spanning both scenarios throughout the simulation. As elaborated in the methodology, the figures presented in the table represent the mean values over the entire simulation stretch. Under the baseline scenario, biomass displays a carbon stock of 2,646 Mg C (approx. 35 Mg C ha-1), whereas the soil stock reveals a notably higher value of 14,456 Mg C (approx. 180 Mg C ha-1). When compared with the alternative scenario, there’s a marked increase in both the biomass and soil stocks, registering values of 7,773 (approx. 100 Mg C ha-1) Mg C and 16,487 Mg C (approx. 210 Mg C ha-1), respectively. This results in a difference of 7,158 Mg C (approx. 90 Mg C ha-1) in overall carbon stock between the two scenarios at the offset site.
When incorporating varying discount rates for offset site results, there’s a noticeable decline in carbon stock as higher discount rates are applied. As illustrated in Table 1, without any discounting, the net carbon stock advantage of the alternative scenario over the baseline is the previously mentioned 7,158 Mg C (approx. 90 Mg C ha-1). This extra buffer, however, narrows with increasing discount rates: it shrinks to 4,507 Mg C (approx. 60 Mg C ha-1) with a 1% rate, to 2,383 Mg C (approx. 30 Mg C ha-1) with a 3% rate, and diminishes further to 1,633 Mg C (approx. 20 Mg C ha-1) when a 5% rate is imposed. Importantly, even with a 5% discount rate, the projected net carbon stock gains from forest conservation over a 100-year duration remain significant. Furthermore, these gains surpass the carbon stock deficits predicted for the deforestation site, as can be compared in Table 1.
As for the trajectory of the CO2 flux, Figure 6 depicts the outcomes under the baseline scenario. The year 2023 sees a significant release of carbon, amounting to −3,084 Mg C (approx. 40 Mg C ha-1). Following this sharp release, there’s a marked recovery in carbon sequestration. By 2027, the CO2 flux is positive, with 35 Mg C (approx. 0.5 Mg C ha-1) being stored. This positive trend continues, peaking at 105 Mg C (approx. 1 Mg C ha-1) in 2048. Towards the latter part of the century, there’s a consistent carbon release, culminating in an emission of −84 Mg C (approx. −1 Mg C ha-1) by 2102. This trend illustrates the increasing harvesting efforts under the simulated active forest management practices. The simulated cumulative biomass carbon sequestration under the baseline plan from 2023 to 2122 amounts to −2,374 Mg C (approx. −30 Mg C ha-1), indicating carbon loss rather than a sink.
Finally, Figure 7 displays carbon stock change projections for the conservation scenario. In the initial years, sequestration is projected to be at the higher end of this spectrum, with 100 Mg C (approx. 1 Mg C ha-1) in 2023. This reflects the continued maturation and growth of the current mature standing timber stocks when protected from harvesting disturbances. Sequestration then gradually declines over the timeframe as forest stands reach later successional stages characterized by slower growth rates and increasing natural mortality. By the final simulated year 2122, annual net sink is forecasted to diminish to 7 Mg C (approx. 0.1 Mg C ha-1). The cumulative biomass net sink from 2023 to 2122 under the alternative scenario is 4,606 Mg C (approx. 60 Mg C ha-1), differing greatly from the −2,374 Mg C (approx. −30 Mg C ha-1) which was the respective result for the net biomass carbon stock change under the baseline scenario.
4 Discussion
Our results suggest that mathematically the additional carbon stock at the offset site is enough to compensate carbon stock losses of the deforestation site. The proposed offset is positive from CO2 perspective, and potentially besides the climate impact, the forest conservation at offset site also benefits ecological values. REDD+ programs and other forest conservation carbon offset schemes, which have grown substantially in volume in the past decade, provide interesting comparison point for the case study of this paper. When contrasted with REDD+ programs and other voluntary carbon offset schemes, our study echoes some of the complexities observed in these initiatives, yet with distinct outcomes and implications. West et al. (2023) highlighted a concern for carbon credit ‘profiteers’, a concern not present in our case study, as the city of Espoo is not seeking to commercialize the potential CO2 offset created at the offset site.
4.1 Impact on carbon stocks
Over the 100-year simulation period, the simulated construction activity and deforestation scenario projects a carbon stock loss of 229 Mg C ha-1 when compared against preserving the managed forest ecosystem (baseline). Simulations showed that biomass carbon loss (43 Mg C ha-1) represent 19% of overall loss and major carbon loss (81%) was due to decreased soil carbon stock (186 Mg C ha-1), which is particularly result of the construction as the simulations assume that significant amount of soil will be excavated during building construction activities. However, the soil carbon estimate comes with very high uncertainty since the lifetime of sealed soil carbon under urban construction is not known. Our results are in line with a significant body of literature underscoring urbanization’s substantial impacts on the global carbon cycle (Seto et al., 2012; Churkina, 2016). The immediate biomass carbon loss of 1,253 Mg C (approx. 67 Mg C ha-1) represents the emissions released from the clearance of forest vegetation for construction activities. These findings are consistent with earlier research highlighting deforestation as a substantial source of carbon emissions, as vegetation and soil carbon stocks are destroyed (Foley et al., 2005; IPCC, 2022). Recent research by Moisa et al. (2023) documented significant carbon stock reductions due to deforestation in Africa, with a decline of 588 Mg C ha-1. In the boreal forest context, Thuille et al. (2000) reported a deforestation impact on soil carbon of 65 Mg C ha-1, including carbon losses from both the organic layer and the upper mineral horizon. Research has consistently flagged deforestation and the conversion to impervious surfaces as significant contributors to the carbon footprint of urbanization (Foley et al., 2005; Seto et al., 2012). Our study reinforces these dynamics within the Finnish context, emphasizing the environmental consequences, if proper offset strategies are not adopted.
Results of the baseline scenario for the deforestation site yielded contrastingly different results, when compared to the alternative scenario (Figure 3) (Table 1). A key observation of the baseline scenario is the persistence of substantial carbon stocks over the 100-year simulation period, particularly when contrasted with the markedly lower carbon stocks projected under the deforestation for construction scenario. If forest is maintained as managed forest, the living biomass carbon stock, commencing at 1,253 Mg C in 2022 (approx. 67 Mg C ha-1), exhibits a range of fluctuations between 650–1,150 Mg C across the modeled timeframe, maintaining an average of 813 Mg C (approx. 40 Mg C ha-1).
Biomass simulations for the offset site vary drastically also between the two scenarios. In the normal forest management scenario, the modelled biomass stocks amount 2,646 Mg C (approx. 30 Mg C ha-1) on average throughout the 100-year period. As was the case with deforestation site in the baseline scenario, the offset site simulations show similar dynamics with biomass accumulation, natural mortality, and occasional harvests taking place as the forest matures (Figure 5). In contrast, forest conservation is simulated to yield significantly higher biomass carbon stock, totaling 7,773 Mg C (approx. 100 Mg C ha-1) on average through the simulation period.
Soil organic carbon reductions represent a more insidious and less visible aspect of carbon loss. The results indicate a substantial decrease of 3,771 Mg C (approx. 200 Mg C ha-1) in soil organic carbon stock at the deforestation site from the construction activities (Figure 3). This demonstrates the significant impact of deforestation when it happens in connection with urban construction, as the soil will be heavily impacted by various construction activities. Furthermore, it underscores the long-term effects of deforestation, which removes the forest vegetation that could otherwise replenish soil carbon through litter input and decomposition (Lal, 2004; Ontl and Schulte, 2012). Within the baseline scenario at deforestation site, soil organic carbon shows steady development, registering a minor decline from 4,419 Mg C (approx. 235 Mg C ha-1) in 2022 to 3,972 Mg C (approx. 210 Mg C ha-1), which represents the average value for the century long simulation period. This trend exemplifies the inherent dynamics in normal production forests under active management regimes, with biomass accumulation, natural mortality, and harvests intertwining as the forest matures and undergoes thinning.
In the case of the offset site, soil carbon stock shows predictable development (Figure 5). The baseline scenario shows soil carbon decreasing slowly during the simulation period, as a consequence from the active forest management operations. Meanwhile, forest conservation case shows a steady increase of soil carbon stock as the forest remains unmanaged. Forest conservation elevated soil organic carbon stock by 2,032 Mg C (approx. 30 Mg C ha-1) compared to the baseline (Table 1).
Our results highlight the risks of underestimating actual carbon impacts when focusing solely on biomass stock losses (McNicol et al., 2018; Li et al., 2022). Overlooking soil carbon dynamics could potentially skew the understanding of perceived magnitude of the disruption. Hence, comprehensively accounting for belowground soil dynamics is imperative for grasping land-use change’s full emissions impact and highlights the interest to simulate both biomass and soil carbon stocks. Our findings reinforce the need to evaluate all relevant carbon stocks when appraising carbon footprints from urban expansion.
Given the substantial soil carbon loss witnessed at the deforestation site under the construction scenario, an interesting parallel discourse emerges around sustainable construction waste management, particularly soil reuse. Scholars have advocated the climate benefits of recycling, repurposing, and reusing construction waste (Simion et al., 2013; Hale et al., 2021). Notably, repurposing soil materials such as clay could indirectly reduce emissions elsewhere, decreasing the need for virgin materials. This would be a particularly relevant consideration in comprehensive carbon accounting from a city-wide perspective. Growing body of research demonstrates the climate benefits of reusing construction carbon stocks in this manner rather than incurring permanent emissions through landfilling (Magnusson et al., 2015). Traditionally, surplus soil material from construction has been sent to landfill sites, which are quickly reaching capacity, particularly in Finland’s larger urban regions (Vierikko et al., 2020). While the potential for soil carbon reuse presents an interesting solution to mitigate the impacts of construction, quantifying climate impacts would necessitate in-depth soil surveys and potentially further soil assessments. Consequently, this study does not incorporate the potential for soil carbon reuse in its results and conclusions. It does, however, highlight soil carbon reuse as an intriguing area warranting further research.
Finally, looking at the combined biomass and soil carbon results, comparison of deforestation site scenarios highlights the substantial carbon stock loss of 4,315 Mg C (approx. 230 Mg C ha-1) (Table 1), resulting from the repurposing of approx. 19 ha from forest to a building site. This significant net difference of 4,315 Mg C underscores the need for proportionately scaled mitigation activities to counterbalance the simulated carbon stock losses. The magnitude of this carbon stock loss warrants particular attention. Sitra (2019) estimates that carbon footprint of an average individual residing in Finland to be roughly 10.3 MgCO2eq. (equivalent to released carbon stock of 2.8 Mg C). This comparison brings to light a crucial perspective: the carbon stock loss from planned land clearing at the deforestation area of 19 ha equates to the annual carbon footprint of over 1,500 Finnish residents.
Additionally, it's important to highlight the translation of C values to CO2 equivalents in public discourse, especially in the context of CO2 offsetting. Deforestation impact at the deforestation site, amounting to 4,315 Mg C (approx. 230 Mg C ha-1), equates to 15,820 tCO2. This conversion highlights the potential CO2 offsetting need if the offset site’s conservation does not occur. Purchasing equivalent CO2 credits from the carbon market could be an alternative for the city of Espoo, should conservation at the offset site not be implemented.
The offset site and the conservation scenario showed a potential of ‘gain’ of 7,158 Mg C (approx. 90 Mg C ha-1) (calculated as alternative scenario - baseline scenario) in total carbon stock of planned 79 ha conservation area, emphasizing sequestration capacity of maturing forests over time when protected from harvesting disturbances (Table 1). In context of previous literature, the projected trajectories align clearly with forest carbon balance studies. In the absence of thinning and harvesting disturbances, aboveground biomass carbon exhibits sustained accumulation, mirroring the ability of older natural forests to accumulate and store carbon (Harmon et al., 1990; Carey et al., 2001; Luyssaert et al., 2008; Pan et al., 2011; Birdsey et al., 2023; Mo et al., 2023).
These findings theoretically validate the potential for conservation of localized forest areas, as proposed at Espoo, Finland, to potentially offset emissions from urban land-use changes like those modelled at the deforestation site. Our case study lends quantitative support to the perspective advocated by some scholars that strategically enhancing urban green infrastructure and nature-based solutions can help counterbalance development-driven ecological harm (Silaydin Aydin and Çukur, 2012; Yang et al., 2022).
Looking through the lenses of qualitative assessment, voluntary REDD+ projects in the Brazilian Amazon have been found to overstate impacts on avoided deforestation and climate change mitigation, as observed by West et al. (2020). In contrast, our study presents a robust modeling for additional carbon stock and sequestration calculated for the offset site, which in business-as-usual situation would be under normal active management practices. This approach, aimed at reflecting genuine climate benefits, stands in stark contrast to certain carbon offset market practices, such as over-crediting occurring in case offset developers select forest that are ecologically distinct from regional baseline averages (Badgley et al., 2022). Notably, even if we conservatively assume soil carbon stock at the deforestation site to be zero post-construction—thereby increasing the total carbon stock loss to over 4,962 Mg C (approx. 260 Mg C ha-1)—the offset site’s projected compensatory capacity of 7,158 Mg C (approx. 90 Mg C ha-1) remains more than sufficient to offset this loss. This indicates not only the robustness of the offset site’s carbon sequestration potential but also the importance of credible, transparent baseline setting in carbon offset initiatives.
4.2 Discounted CO2 values
The importance of discounting carbon impacts in the context of CO2 compensation and offsetting cannot be understated. Given the global urgency to achieve net-zero emissions by 2050, sequestration in the near term is notably more valued than its delayed counterpart (Moilanen et al., 2009; Johnston and van Kooten, 2015). In the context of our study, it is crucial to acknowledge that the long-term fate of biomass carbon stocks remains subject to risks from various disturbances.
In our study, the practice of discounting carbon impacts was central to understanding the long-term value of carbon stocks. The choice of which attribute to discount in ecosystem services varies, as shown in research. Michaelowa et al. (2019) provide a detailed exposition on the approach for discounting carbon and CO2 credits. Hean et al. (2003) delve into the intricacies of discounting both carbon redemption and the net carbon benefit of a forest, encompassing sequestration and stock dynamics. Parisa et al. (2022) introduce a dual perspective, suggesting that discounting can be applied either to carbon capture and release or carbon stock. Furthermore, Baumgärtner et al. (2015) advocate for a universal discounting approach, arguing that any ecosystem service should be subject to an appropriate discount rate.
Discounting the nominal carbon stock values substantially alters the conclusions drawn from the deforestation site. Table 1 provides a clear illustration of how different discount rates can significantly alter the perceived value of carbon stock over time. Employing a 1% discount rate would already reduce the impact of forest clearing at deforestation site to a loss of 3,108 Mg C instead of 4,315 Mg C. This effect from discounting underscores the diminished value assigned to carbon stocks 50 or even 100 years in the future and impact of this size is similar to other studies (see, e.g., Howard and Sylvan, 2015; National Academies of Sciences and Medicine, 2017).
In the case of the offset site, key observation is the extent of the sensitivity of offset site’s buffer capacity for carbon stock to varying discount rates. As visible from the values presented in Table 1, without any discounting, the offset site could yield a buffer of 2,843 Mg C (calculated as offset site difference–deforestation site difference, i.e., 7,158 Mg C – 4,315 Mg C). When contrasting the outcomes to discounted values, this surplus of C stock at the offset site would diminish to 1,399 Mg C with a 1% discount rate, and further shrink to 344 Mg C with a 3% discount rate. Utilizing the highest discount rate of 5% would reduce the offset site’s offsetting impact to a minimal surplus of 44 Mg C (calculated as 1,633 Mg C – 1,588 Mg C), when compared with the simulated carbon stock losses at the deforestation site. These results and level of the impact from discounting are in line with the earlier academic publications, such as the study by Pihlainen et al. (2014), which included discounting of additional carbon sequestration resulting from changes in forest stand management.
This paper deposits that this diminishing offset viability suggests secured near term CO2 reductions should hold primacy in urban development planning. Reliance purely on projected offsets in distant future, vulnerable also to natural disasters, provides limited guarantee of cushioning land use impacts. Integrating nature-based solutions for CO2 offsetting with discounting of prospective stocks offers greater assurance of counterbalancing initial carbon losses.
To further enhance our analysis, we incorporated the Social Cost of Carbon (SCC) and discount rate assumptions developed by the U.S. Environmental Protection Agency (EPA). This approach considers the increasing SCC over time due to escalating climate damages, providing a comprehensive economic evaluation. By integrating the EPA’s latest SCC estimates, we ensure our analysis reflects both current and future economic impacts of carbon dynamics in the study systems. Table 2 illustrates the discounted USD values of the offset project at 10-year intervals. Our results indicate that the present value of carbon stock gains from forest conservation at the offset site is more than enough to offset the carbon stock losses at the deforestation site, even when considering different discount rates (2.5%, 2.0%, and 1.5%). These results and level of the impact from discounting are in line with the earlier academic publications, such as the study by Pihlainen et al. (2014), which reported the present monetary value of the change in carbon pool that resulted from changes in forest stand management. This approach not only highlights the financial viability of forest conservation as a CO2 offset strategy but also underscores the importance of incorporating future SCC projections into carbon offset assessments to capture the long-term economic benefits.
4.3 Complexities of the CO2 offset regulation
The performed scenario analyses suggest that planned forest conservation of 79 ha could produce adequate carbon stock increase to offset urban construction impacts of the deforestation of 19 ha area. However, translating these results into defined CO2 compensation or offset claims involves navigating the complex technical and policy dimensions at two distinctive levels: the policy landscape of greenhouse gas inventories related to forestry and land-use change, and the criteria applicable to CO2 offset projects. This complexity is mirrored in voluntary forest conservation and REDD+ initiatives, where the validity and effectiveness of conservation efforts are under increased scrutiny (West et al., 2020; Badgley et al., 2022; West et al., 2023).
There are particularly two influencing aspects to consider in the policy landscape. First, geographical boundaries and municipal borders play an integral role in the carbon budgeting related to forestry and land-use change. The fact that the suggested carbon stock compensation takes place within the same municipality as the urban expansion emissions (Figure 2) implies that it is essentially an internal carbon budgeting process for the city of Espoo.
Second, the distinction between public and private sector entities is relevant. The city of Espoo, like any Finnish municipality, is part of the public sector. Emission reduction measures undertaken by a municipality are simultaneously contributions to Finland’s overall reduction measures (Lounasheimo et al., 2020). The emissions reductions and potential enhancements of carbon sinks of all municipality owned land are already included in Finland’s national greenhouse gas inventory. In practical terms, both the effects of land clearing at deforestation site and the forest conservation at offset site, would be accounted for in Finland’s greenhouse gas inventory.
Complexities surrounding the formalization of CO2 compensation, echoed in our study, have been reported by previous scholarly papers. Nolte et al. (2019) expressed concerns regarding selection bias, meaning protection of land which would have been conserved in the absence of formal protection, and local spillover effects, meaning effectively leakage, in voluntary forest conservation. Our study emphasizes the Finnish policy landscape and brings to light the internal carbon budgeting approach for the city of Espoo. This sets our case study apart from the conventional external market-driven methods commonly seen in REDD+ projects.
New guidelines in Finland differentiate between so-called ‘offset claims’ and ‘contribution claims’ regarding forest carbon sinks (Laine et al., 2023). The former addresses mitigation uncounted towards national climate targets, while the latter denotes supporting domestic goals. Consequently, any additional carbon sequestration or reduced emissions by Finnish public entities automatically feed into national reporting. This implies that, from a procedural standpoint, the proposed conservation efforts at offset cannot constitute conventional offsetting. Rather, the city could view their conservation action as a contribution claim facilitating Finland’s climate objectives.
Transitioning from the overarching policy considerations, attention must also be brought to the individual CO2 offset project criteria. These criteria can be seen as a useful guideline, even if the city of Espoo does not have explicit plans to apply for certified CO2 offset credits, and most likely would not qualify due to the details explained above, from organizations such as Verra or Gold Standard.
The first criterion, additionality, ensures that the emission reductions generated by a project exceed those that would occur without it. In this context, given that no formal conservation program has previously been considered for the offset site, an official conservation effort would represent a novel, additional action by the city. However, it should be noted that the offset site is not currently under active forest management. This status is evidenced by the existing forest stock per age class, as detailed in Supplementary Table S1. Therefore, the baseline scenario for the offset site, which applies the ‘best practices for sustainable forest management in Finland’ guidelines, may potentially overestimate the severity of thinning and harvesting activities. Should this be the case, the additional carbon impact resulting from forest conservation (additionality compared to the baseline scenario) might be lower than what the results currently indicate.
Secondly, the baseline setting criterion involves creating a plausible scenario that illustrates the carbon impact absent the proposed project activity. For both the deforestation site, as well as for the offset site, a detailed baseline scenario has been simulated and used as a reference to calculate the carbon impact. However, the preceding reflection regarding the potential overestimation of thinning and harvesting activities remains relevant.
The third criterion, permanence, guarantees that the carbon sequestered by a project remains stored and is not subsequently re-emitted into the atmosphere. A commitment of 100 years to land-use changes is typically required to classify an activity as permanent (Allen et al., 2020; Regan et al., 2020). The forest simulations performed in this study adhere to this requirement, with a 100-year projection period. To achieve the simulated outcomes, the City of Espoo must commit to protecting the offset site for the next century. Studies like Guizar-Coutiño et al. (2022) have found that REDD+ projects did not always deliver reductions in deforestation. The offset site of our study is on the path to formal conservation. In 2024, the conservation proposal is scheduled for review by the city’s environment and building board, followed by deliberation and decision-making by the city council. Subsequently, the council will submit the proposal to the ELY Centre, a regulatory authority within the Finnish Ministry of Economic Affairs and Employment, which is responsible for issuing the final conservation ruling. Our perspective is that such a conservation guarantees the permanence, with the official conservation being also naturally prone to any potential disturbances such as forest fires or storm damages.
Lastly, the leakage criterion relates to the inadvertent displacement of emissions as a consequence of a project’s activities (Vinca et al., 2018). For example, increased logging elsewhere as a result of a forest protection scheme would be considered leakage. In the case of our offset site, the small scale and localized nature of the conservation effort limit the displacement risks often associated with offset projects. Given that offset site’s total wood stock is approximately 15,000 cubic meters, a figure negligible in comparison to the Finnish wood market’s 75 million cubic meters of removals in 2022 (Natural Resources Institute Finland, 2023), it's unlikely that conserving the offset site’s stocks would stimulate additional harvests elsewhere.
5 Conclusion
Our findings illustrate that under the simulated scenarios, the additional carbon stock at the offset site is indeed sufficient to compensate for the loss of carbon stock at the deforestation site. Thus, a targeted forest conservation could serve as a viable strategy for cities to manage their carbon budget while dealing with the urban growth. The results affirm that safeguarding existing forests can yield significant carbon sequestration benefits, particularly when compared to the carbon losses from deforestation for urban development.
Our approach to carbon stock evaluation and the application of discounting of future carbon values offer a methodological framework that contrasts with some criticized practices within the voluntary carbon offset market. In doing so, our study contributes to a more transparent and accountable discourse on carbon offsetting, emphasizing the need for credible and verifiable carbon accounting practices. The qualitative comparison to REDD+ project cases further contextualize our findings within the broader landscape of forest conservation initiatives. While our study presents a localized analysis, the insights garnered here resonate with global discussions on the integrity and credibility of forest-based carbon offset projects.
Moreover, the discussion of the complexities surrounding CO2 offset regulation brings to light the challenges inherent in translating theoretical carbon stock into recognized CO2 compensation claims. The evolving regulatory landscape in Finland, particularly concerning public sector entities and their contributions to national climate targets, serves as a prime example of these challenges.
Ultimately, while this localized case study demonstrates the potential effectiveness of forest conservation from a climate mitigation standpoint, its designation as formal carbon offsetting faces constraints under prevailing carbon accounting regulation. Nevertheless, the numerical viability shown here lays the groundwork for future initiatives, supporting an environmentally responsible approach to urban development of cities. As municipalities globally strive towards climate neutrality, the insights from this study could inform more sustainable urban planning and the integration of nature-based solutions to achieve broader ecological and climate goals.
Data availability statement
The data analyzed in this study is subject to the following licenses/restrictions: Stand-level forest inventory data used in our forest simulations was obtained from the Environmental Protection Services department of the city of Espoo. The forest inventory data is confidential property of the city, and not publicly available without a prior approval. Requests to access these datasets should be directed to mikko.jarvelainen@alumni.helsinki.fi.
Author contributions
MJ: Conceptualization, Data curation, Formal Analysis, Investigation, Methodology, Project administration, Validation, Visualization, Writing–original draft, Writing–review and editing. SP: Supervision, Writing–original draft, Writing–review and editing. KK: Supervision, Writing–original draft, Writing–review and editing. NÖ: Formal Analysis, Software, Writing–original draft, Writing–review and editing. RM: Supervision, Writing–original draft, Writing–review and editing.
Funding
The author(s) declare that financial support was received for the research, authorship, and/or publication of this article. This study is part of a Finnish Ministry of Agriculture and Forestry funded research project KUNTANIELU, which investigates the possibilities of municipalities to use offsetting based on net carbon sinks in the land use sector and evaluates challenges of the compensation scheme. This study received funding from the Ministry of Agriculture and Forestry (KUNTANIELU-project) and it was done with affiliation to the UNITE Flagship funded by the Research Council of Finland (decision no 337655).
Acknowledgments
The content of the manuscript has previously partially appeared online in a master’s thesis published at the Department of Forest Sciences, University of Helsinki. The thesis has been published in September 2023. This project would not have been possible without the generosity and cooperation from the city of Espoo. In addition, we thank AFRY AB Smart Forestry (AFRY Management Consulting Oy) for the forest simulation and optimization software provided to conduct the forest simulations.
Conflict of interest
Author NÖ was employed by AFRY Management Consulting Oy.
The remaining authors declare that the research was conducted in the absence of any commercial or financial relationships that could be construed as a potential conflict of interest.
The author(s) declared that they were an editorial board member of Frontiers, at the time of submission. This had no impact on the peer review process and the final decision.
Publisher’s note
All claims expressed in this article are solely those of the authors and do not necessarily represent those of their affiliated organizations, or those of the publisher, the editors and the reviewers. Any product that may be evaluated in this article, or claim that may be made by its manufacturer, is not guaranteed or endorsed by the publisher.
Supplementary material
The Supplementary Material for this article can be found online at: https://www.frontiersin.org/articles/10.3389/fenvs.2024.1379630/full#supplementary-material
SUPPLEMENTARY TABLE S1 | Deforestation site (Hepokorvenkallio) - current standing forest stock per age class.
SUPPLEMENTARY TABLE S2 | Offset site (Hynkänlampi) – current standing forest stock per age class.
References
Allen, M., Axelsson, K., Caldecott, B., Hale, T., Cameron, H., Hickey, C., et al. (2020). The oxford principles for net zero aligned carbon offsetting 2020. Oxford, United Kingdom: University of Oxford.
Badgley, G., Freeman, J., Hamman, J. J., Haya, B., Trugman, A. T., Anderegg, W. R. L., et al. (2022). Systematic over-crediting in California's forest carbon offsets program. Glob. Change Biol. 28, 1433–1445. doi:10.1111/gcb.15943
Baumgärtner, S., Klein, A. M., Thiel, D., and Winkler, K. (2015). Ramsey discounting of ecosystem services. Environ. Resour. Econ. (Dordr) 61, 273–296. doi:10.1007/s10640-014-9792-x
Birdsey, R. A., DellaSala, D. A., Walker, W. S., Gorelik, S. R., Rose, G., and Ramírez, C. E. (2023). Assessing carbon stocks and accumulation potential of mature forests and larger trees in U.S. federal lands. Front. For. Glob. Change 5. doi:10.3389/ffgc.2022.1074508
Carey, E. V., Sala, A., Keane, R., and Callaway, R. M. (2001). Are old forests underestimated as global carbon sinks? Glob. Change Biol. 7, 339–344. doi:10.1046/j.1365-2486.2001.00418.x
Churkina, G. (2016). The role of urbanization in the global carbon cycle. Front. Ecol. Evol. 3, 144. doi:10.3389/FEVO.2015.00144
Dixon, R. K., Solomon, A. M., Brown, S., Houghton, R. A., Trexier, M. C., and Wisniewski, J. (1994). Carbon pools and flux of global forest ecosystems. Science 263, 185–190. doi:10.1126/science.263.5144.185
Dubash, N., Khosla, R., Kelkar, U., and Lele, S. (2018). India and climate change: evolving ideas and increasing policy engagement. Annu. Rev. Environ. Resour. 43, 395–424. doi:10.1146/annurev-environ-102017-025809
Falkner, R. (2016). The Paris Agreement and the new logic of international climate politics. Int. Aff. 92, 1107–1125. doi:10.1111/1468-2346.12708
Finland’s Climate Change Act 423/2022 (2022) Naantali and helsinki, 10.6. Available at: https://www.finlex.fi/fi/laki/alkup/2022/20220423 (Accessed July 8, 2023).
Foley, J., Defries, R., Asner, G., Barford, C., Bonan, G., Carpenter, S., et al. (2005). Global consequences of land use. Science 309, 570–574. doi:10.1126/science.1111772
Griscom, B. W., Adams, J., Ellis, P. W., Houghton, R. A., Lomax, G., Miteva, D. A., et al. (2017). Natural climate solutions. Proc. Natl. Acad. Sci. 114, 11645–11650. doi:10.1073/pnas.1710465114
Guizar-Coutiño, A., Jones, J. P. G., Balmford, A., Carmenta, R., and Coomes, D. A. (2022). A global evaluation of the effectiveness of voluntary REDD+ projects at reducing deforestation and degradation in the moist tropics. Conserv. Biol. 36, e13970. doi:10.1111/COBI.13970
Hale, S. E., Roque, A. J., Okkenhaug, G., Sørmo, E., Lenoir, T., Carlsson, C., et al. (2021). The reuse of excavated soils from construction and demolition projects: limitations and possibilities. Sustain. Switz. 13, 6083. doi:10.3390/su13116083
Harmon, M. E., Ferrell, W. K., and Franklin, J. F. (1990). Effects on carbon storage of conversion of old-growth forests to young forests. Science 247, 699–702. doi:10.1126/SCIENCE.247.4943.699
Haya, B., Cullenward, D., Strong, A. L., Grubert, E., Heilmayr, R., Sivas, D. A., et al. (2020). Managing uncertainty in carbon offsets: insights from California’s standardized approach. Clim. Policy 20, 1112–1126. doi:10.1080/14693062.2020.1781035
Hean, R. L., Cacho, O., Menz, K. M., Hean, R. L., Cacho, O., and Menz, K. M. (2003). “Temporary carbon storage and discount rates,” in Australian agricultural and resource economics society. doi:10.22004/AG.ECON.57888
Helmisaari, H.-S., Derome, J., Nöjd, P., and Kukkola, M. (2007). Fine root biomass in relation to site and stand characteristics in Norway spruce and Scots pine stands. Tree Physiol. 27, 1493–1504. doi:10.1093/treephys/27.10.1493
Hildén, M., Pihlainen, S., Saikku, L., Axelsson, K., Aamaas, B., Pedersen, A. B., et al. (2023). Municipalities can contribute to the strengthening of carbon sinks. Available at: https://pub.norden.org/nord2023-035/Nord2023-035.pdf (Accessed January 3, 2024).
Howard, P., and Sylvan, D. (2015). Expert consensus on the economics of climate change. New York: Institute for Policy Integrity, New York University School of Law. Available at: https://policyintegrity.org/publications/detail/expert-climate-consensus (Accessed November 15, 2023).
Hynynen, J., Ojansuu, R., Hökkä, H., Siipilehto, J., Salminen, H., and Haapala, P. (2002). Models for predicting stand development in MELA system. Metsäntutkimuslaitoksen Tied. 835.
Ilmastonkestävän kaupungin suunnitteluopas (2023). “Raportit ja työkalut,” in Ilmastonkestävän kaupungin suunnitteluopas. Available at: https://ilmastotyokalut.fi/raportit-ja-tyokalut/index.htm (Accessed October 14, 2023).
IPCC (1995). “Climate change 1995: a report of the intergovernmental Panel on climate change,” in Second assessment report of the intergovernmental Panel on climate change. Available at: https://archive.ipcc.ch/pdf/climate-changes-1995/ipcc-2nd-assessment/2nd-assessment-en.pdf (Accessed April 19, 2023).
IPCC (2019). “2019 refinement to the 2006 IPCC guidelines for national greenhouse gas inventories - the national greenhouse gas inventories programme,”. Geneva: IPCC. Available at: https://www.ipcc.ch/report/2019-refinement-to-the-2006-ipcc-guidelines-for-national-greenhouse-gas-inventories (Accessed April 20, 2023).
IPCC (2022). in Climate change 2022: mitigation of climate change. Contribution of working group III to the sixth assessment report of the intergovernmental Panel on climate change. Editors P. R. Shukla, J. Skea, R. Slade, A. Al Khourdajie, R. van Diemen, D. McCollumet al. (Cambridge, UK and New York, NY, USA: Cambridge University Press). doi:10.1017/9781009157926
IP CC (2007). in Climate change 2007: mitigation. Contribution of working group III to the fourth assessment report of the intergovernmental Panel on climate change. Editors B. Metz, O. R. Davidson, P. R. Bosch, R. Dave, and L. A. Meyer (Cambridge, United Kingdom and New York, NY, USA: Cambridge University Press).
Järveläinen, M. (2023). Evaluating the effectiveness of forest conservation as a CO2 offset measure for urban development: a case study in Espoo, Finland. Helsinki, Finland: University of Helsinki. [dissertation/master’s thesis.
Jo, H. K., Kim, J. Y., and Park, H. M. (2019). Carbon reduction and planning strategies for urban parks in Seoul. Urban Urban Green 41, 48–54. doi:10.1016/J.UFUG.2019.03.009
Johnston, C., and van Kooten, C. (2015). Back to the past: burning wood to save the globe. Ecol. Econ. 120, 185–193. doi:10.1016/j.ecolecon.2015.10.008
Karhinen, S., Peltomaa, J., Riekkinen, V., and Saikku, L. (2021). Impact of a climate network: the role of intermediaries in local level climate action. Glob. Environ. Change 67, 102225. doi:10.1016/J.GLOENVCHA.2021.102225
Kolstad, C., Urama, K., Broome, J., Bruvoll, A., Cariño Olvera, M., Fullerton, D., et al. (2014). “Social, economic and ethical concepts and methods,” in Climate change 2014: mitigation of climate change. Contribution of working group III to the fifth assessment report of the intergovernmental Panel on climate change. Editors O. Edenhofer, R. Pichs-Madruga, Y. Sokona, E. Farahani, S. Kadner, K. Seybothet al. (Cambridge, United Kingdom and New York, NY, USA: Cambridge University Press).
Laine, A., Ahonen, H.-M., Pakkala, A., Laininen, J., Kulovesi, K., and Mäntylä, I. (2023). Guide to good practices for supporting voluntary carbon markets. Helsinki: Government of Finland. Available at: https://julkaisut.valtioneuvosto.fi/handle/10024/164732 (Accessed June 12, 2023).
Lal, R. (2004). Soil carbon sequestration to mitigate climate change. Geoderma 123, 1–22. doi:10.1016/J.GEODERMA.2004.01.032
Li, Y., Brando, P. M., Morton, D. C., Lawrence, D. M., Yang, H., and Randerson, J. T. (2022). Deforestation-induced climate change reduces carbon storage in remaining tropical forests. Nat. Commun. 13, 1964. doi:10.1038/s41467-022-29601-0
Liu, C., and Li, X. (2012). Carbon storage and sequestration by urban forests in Shenyang, China. Urban Urban Green 11, 121–128. doi:10.1016/J.UFUG.2011.03.002
Lounasheimo, J., Karhinen, S., Grönroos, J., Savolainen, H., Forsberg, T., Munther, J., et al. (2020). Suomen kuntien kasvihuonekaasupäästöjen laskenta, Suomen ympäristökeskuksen raportteja 25/2020. Helsinki: Suomen ympäristökeskus. Available at: https://helda.helsinki.fi/server/api/core/bitstreams/c6654d08-a1f3-4ffb-8244-e1f56c5839ee/content (Accessed August 20, 2023).
Luyssaert, S., Schulze, E. D., Börner, A., Knohl, A., Hessenmöller, D., Law, B. E., et al. (2008). Old-growth forests as global carbon sinks. Nature 455 (7210 455), 213–215. doi:10.1038/nature07276
Magnusson, S., Lundberg, K., Svedberg, B., and Knutsson, S. (2015). Sustainable management of excavated soil and rock in urban areas – a literature review. J. Clean. Prod. 93, 18–25. doi:10.1016/J.JCLEPRO.2015.01.010
McDonald, R., Kareiva, P., and Forman, R. (2008). The implications of current and future urbanization for global protected areas and biodiversity conservation. Biol. Conserv. 141, 1695–1703. doi:10.1016/j.biocon.2008.04.025
McNicol, I. M., Ryan, C. M., and Mitchard, E. T. A. (2018). Carbon losses from deforestation and widespread degradation offset by extensive growth in African woodlands. Nat. Commun. 9, 3045. doi:10.1038/s41467-018-05386-z
Michaelowa, A. (2009). “Interpreting the additionality of CDM projects: changes in additionality definitions and regulatory practices over time,” in Legal aspects of carbon trading: Kyoto, Copenhagen, and beyond (Oxford University Press), 248–271.
Michaelowa, A., Shishlov, I., and Brescia, D. (2019). Evolution of international carbon markets: lessons for the Paris Agreement. Wiley Interdiscip. Rev. Clim. Change 10, e613. doi:10.1002/WCC.613
Ministry of Agriculture and Forestry of Finland (2023). Forest management practices. Available at: https://mmm.fi/en/forests/forestry/sustainable-forest-management/best-practices-for-forest-management (Accessed October 25, 2023).
Mo, L., Zohner, C. M., Reich, P. B., Liang, J., de Miguel, S., Nabuurs, G. J., et al. (2023). Integrated global assessment of the natural forest carbon potential. Nature 624, 92–101. doi:10.1038/s41586-023-06723-z
Moilanen, A., Van Teeffelen, A. J. A., Ben-Haim, Y., and Ferrier, S. (2009). How much compensation is enough? A framework for incorporating uncertainty and time discounting when calculating offset ratios for impacted habitat. Restor. Ecol. 17, 470–478. doi:10.1111/j.1526-100x.2008.00382.x
Moisa, M. B., Dejene, I. N., Deribew, K. T., Gurmessa, M. M., and Gebeda, D. B. (2023). Impacts of forest cover change on carbon stock, carbon emission and land surface temperature in Sor watershed, Baro Akobo Basin, Western Ethiopia. J. Water Clim. Change 14, 2842–2860. doi:10.2166/wcc.2023.208
Muukkonen, P., and Mäkipää, R. (2006). Empirical biomass models of understorey vegetation in boreal forests according to stand and site attributes. Boreal Environ. Res. 11, 355–369.
National Academies of Sciences Medicine, E. (2017). Valuing climate damages: updating estimation of the social Cost of carbon dioxide. Washington, DC: The National Academies Press. doi:10.17226/24651
Natural Resources Institute Finland (2023). Press release: removals decreased to 75 million cubic metres in 2022. Available at: https://www.luke.fi/en/news/removals-decreased-to-75-million-cubic-metres-in-2022 (Accessed October 23, 2023).
Nolte, C., Meyer, S. R., Sims, K. R. E., and Thompson, J. R. (2019). Voluntary, permanent land protection reduces forest loss and development in a rural-urban landscape. Conserv. Lett. 12. doi:10.1111/CONL.12649
Oldfield, E. E., Eagle, A. J., Rubin, R. L., Rudek, J., Sanderman, J., and Gordon, D. R. (2021). Agricultural Soil Carbon Credits: making sense of protocols for carbon sequestration and net greenhouse gas removals. Available at: https://www.edf.org/sites/default/files/content/agricultural-soil-carbon-credits-protocol-synthesis.pdf (Accessed October 30, 2023).
Pan, Y., Birdsey, R. A., Fang, J., Houghton, R., Kauppi, P. E., Kurz, W. A., et al. (2011). A large and persistent carbon sink in the world’s forests. Science 333, 988–993. doi:10.1126/SCIENCE.1201609
Parisa, Z., Marland, E., Sohngen, B., Marland, G., and Jenkins, J. (2022). The time value of carbon storage. Policy Econ 144, 102840. doi:10.1016/J.FORPOL.2022.102840
Pascoe, S., Cannard, T., and Steven, A. (2019). Offset payments can reduce environmental impacts of urban development. Environ. Sci. Policy 100, 205–210. doi:10.1016/J.ENVSCI.2019.06.009
Pauw, W. P., Klein, R. J. T., Mbeva, K., Dzebo, A., Cassanmagnago, D., and Rudloff, A. (2018). Beyond headline mitigation numbers: we need more transparent and comparable NDCs to achieve the Paris Agreement on climate change. Clim. Change 147, 23–29. doi:10.1007/s10584-017-2122-x
Pihlainen, S., Tahvonen, O., and Niinimäki, S. (2014). The economics of timber and bioenergy production and carbon storage in Scots pine stands. Can. J. For. Res. 44 (9), 1091–1102. doi:10.1139/cjfr-2013-0475
Rasinmäki, J., and Känkänen, R. (2014). Helsingin kaupungin ympäristökeskuksen julkaisuja 10/2014: kuntien hiilitasekartoitus osa 2 - hiilitaselaskuri ja toimenpidevalikoima. Available at: https://www.hel.fi/static/ymk/julkaisut/julkaisu-10-14.pdf (Accessed June 21, 2023).
Regan, C. M., Connor, J. D., Summers, D. M., Settre, C., O’Connor, P. J., and Cavagnaro, T. R. (2020). The influence of crediting and permanence periods on Australian forest-based carbon offset supply. Land use policy 97, 104800. doi:10.1016/j.landusepol.2020.104800
Repola, J., Ojansuu, R., and Kukkola, M. (2007). “Biomass functions for Scots pine, Norway spruce and birch in Finland,” in Working papers of the Finnish forest research Institute 53.
Rogelj, J., Geden, O., Cowie, A., and Reisinger, A. (2021). Net-zero emissions targets are vague: three ways to fix. Nature 591, 365–368. doi:10.1038/d41586-021-00662-3
Russo, A., Escobedo, F. J., Timilsina, N., and Zerbe, S. (2015). Transportation carbon dioxide emission offsets by public urban trees: a case study in Bolzano, Italy. Urban Urban Green 14, 398–403. doi:10.1016/J.UFUG.2015.04.002
Seto, K. C., Güneralp, B., and Hutyra, L. R. (2012). Global forecasts of urban expansion to 2030 and direct impacts on biodiversity and carbon pools. Proc. Natl. Acad. Sci. U. S. A. 109, 16083–16088. doi:10.1073/PNAS.1211658109
Silaydin Aydin, M. B., and Çukur, D. (2012). Maintaining the carbon–oxygen balance in residential areas: a method proposal for land use planning. Urban Urban Green 11, 87–94. doi:10.1016/J.UFUG.2011.09.008
Simion, I. M., Fortuna, M. E., Bonoli, A., and Gavrilescu, M. (2013). Comparing environmental impacts of natural inert and recycled construction and demolition waste processing using LCA. J. Environ. Eng. Landsc. Manag. 21, 273–287. doi:10.3846/16486897.2013.852558
Sitra (2019). Is cutting our personal carbon footprint by 20 per cent enough? Available at: https://www.sitra.fi/en/articles/is-cutting-our-personal-carbon-footprint-by-20-per-cent-enough (Accessed August 1, 2023).
Skärbäck, E. (2007). Urban forests as compensation measures for infrastructure development. Urban Urban Green 6, 279–285. doi:10.1016/J.UFUG.2007.05.006
Statistics Finland (2022). “Greenhouse gas emissions in Finland 1990 to 2021,” in National inventory report under the UNFCCC and the Kyoto Protocol. Helsinki, Finland: Submission to the European Union. Available at: https://www.stat.fi/tup/khkinv/khkaasut_raportointi_en.html (Accessed June 10, 2023).
Strohbach, M. W., and Haase, D. (2012). Above-ground carbon storage by urban trees in Leipzig, Germany: analysis of patterns in a European city. Landsc. Urban Plan. 104, 95–104. doi:10.1016/J.LANDURBPLAN.2011.10.001
Tapio (2023). Best practive guidelines for sustainable forest management. Available at: https://metsanhoidonsuositukset.fi/en (Accessed August 10, 2023).
Thuille, A., Buchmann, N., and Schulze, E.-D. (2000). Carbon stocks and soil respiration rates during deforestation, grassland use and subsequent Norway spruce afforestation in the Southern Alps, Italy. Tree Physiol. 20, 849–857. doi:10.1093/treephys/20.13.849
Tuomi, M., Laiho, R., Repo, A., and Liski, J. (2011). Wood decomposition model for boreal forests. Ecol. Model. 222, 709–718. doi:10.1016/j.ecolmodel.2010.10.025
UNFCCC (2023). Finland - 2023 common reporting format (CRF) table. Available at: https://unfccc.int/documents/627719 (Accessed July 15, 2023).
United Nations (2022). World cities report 2022. Nairobi, Kenya. Available at: https://unhabitat.org/sites/default/files/2022/06/wcr_2022.pdfs (Accessed April 10, 2023).
U.S. Environmental Protection Agency (EPA) (2023). EPA Report on the Social Cost of Greenhouse Gases: Estimates Incorporating Recent Scientific Advances. Available at: https://www.epa.gov/environmental-economics/scghg (Accessed June 4, 2024).
Vierikko, K., Nieminen, H., Salomaa, V., Häkkinen, J., Salminen, J., and Sorvari, J. (2020). Kiertotalous maankäytön suunnittelussa. Kaavoitus kestävän ja luonnonvaroja säästävän kaupunkiympäristön edistäjänä. Suom. ympäristökeskuksen Rap. 45/2020. Available at: https://helda.helsinki.fi/items/b18dcbcc-2857-4232-b384-b547c6a9f4c6 (Accessed August 21, 2023).
Vinca, A., Emmerling, J., and Tavoni, M. (2018). Bearing the cost of stored carbon leakage. Front. Energy Res. 6, 40. doi:10.3389/FENRG.2018.00040
von Hase, A. (2014). Working towards nnl of biodiversity and beyond: strongman mine – a case study. Available at: https://www.forest-trends.org/wp-content/uploads/imported/strongman_2014.pdf (Accessed August 10, 2023).
West, T. A. P., Börner, J., Sills, E. O., and Kontoleon, A. (2020). Overstated carbon emission reductions from voluntary REDD+ projects in the Brazilian Amazon. Proc. Natl. Acad. Sci. U. S. A. 117, 24188–24194. doi:10.1073/pnas.2004334117
West, T. A. P., Wunder, S., Sills, E. O., Börner, J., Rifai, S. W., Neidermeier, A. N., et al. (2023). Action needed to make carbon offsets from forest conservation work for climate change mitigation. Science 381, 873–877. doi:10.1126/SCIENCE.ADE3535
Yang, S., Fu, W., Hu, S., and Ran, P. (2022). Watershed carbon compensation based on land use change: evidence from the Yangtze River Economic Belt. Habitat Int. 126, 102613. doi:10.1016/J.HABITATINT.2022.102613
Keywords: CO2 compensation, CO2 offset, urban development, land-use change, forest conservation, climate change mitigation, public sector net-zero
Citation: Järveläinen M, Pihlainen S, Karhu K, Österberg N and Mäkipää R (2024) Forest conservation as a CO2 offset measure: a case of an urban development project in Finland. Front. Environ. Sci. 12:1379630. doi: 10.3389/fenvs.2024.1379630
Received: 31 January 2024; Accepted: 30 July 2024;
Published: 29 August 2024.
Edited by:
Martin Siegert, University of Exeter, United KingdomReviewed by:
Richard Howarth, Dartmouth College, United StatesSamrat Ghosh, Emergent Ventures India, India
Copyright © 2024 Järveläinen, Pihlainen, Karhu, Österberg and Mäkipää. This is an open-access article distributed under the terms of the Creative Commons Attribution License (CC BY). The use, distribution or reproduction in other forums is permitted, provided the original author(s) and the copyright owner(s) are credited and that the original publication in this journal is cited, in accordance with accepted academic practice. No use, distribution or reproduction is permitted which does not comply with these terms.
*Correspondence: Mikko Järveläinen, mikko.jarvelainen@alumni.helsinki.fi