- 1Australian Rivers Institute, School of Environment and Science, Griffith University, Nathan, QLD, Australia
- 2Coastal and Marine Research Centre, School of Environment and Science, Griffith University, Gold Coast, QLD, Australia
- 3Australian Rivers Institute, School of Environment and Science, Griffith University, Gold Coast, QLD, Australia
- 4The Nature Conservancy Australia, Carlton, VIC, Australia
Oysters are ecosystem engineers that form biogenic reef habitats in shallow coastal and estuarine waters and provide important ecosystem services. Widespread global declines have triggered a world-wide restoration movement, however a paucity of information on tropical oyster reefs has resulted in their exclusion from existing global assessments and, consequently, restoration. In this review we quantified the known global diversity of native reef-building oysters to compare diversity between temperate and tropical regions and assessed historic oyster reef presence and declines using two tropical case studies. We then summarised the biology, ecology, and benefits of tropical oyster reefs, which have four functional differences to temperate reefs: 1) the diversity of reef-building oysters is over four times higher in tropical than in temperate regions; 2) tropical reef-building oysters can have continuous spatfall throughout the year whereas temperate species have a defined season; 3) tropical reef-building oysters are generally faster growing than temperate reef-building oysters; and 4) tropical oysters commonly create mixed-species oyster reefs whereas temperate oyster reefs are generally formed by a single oyster species. There is evidence of unsustainable and destructive harvesting that has resulted in the decline of tropical oyster reefs, and these reefs should therefore be included in restoration efforts. We highlight knowledge gaps that can guide future research to develop important foundational information that will remove barriers to tropical oyster reef restoration.
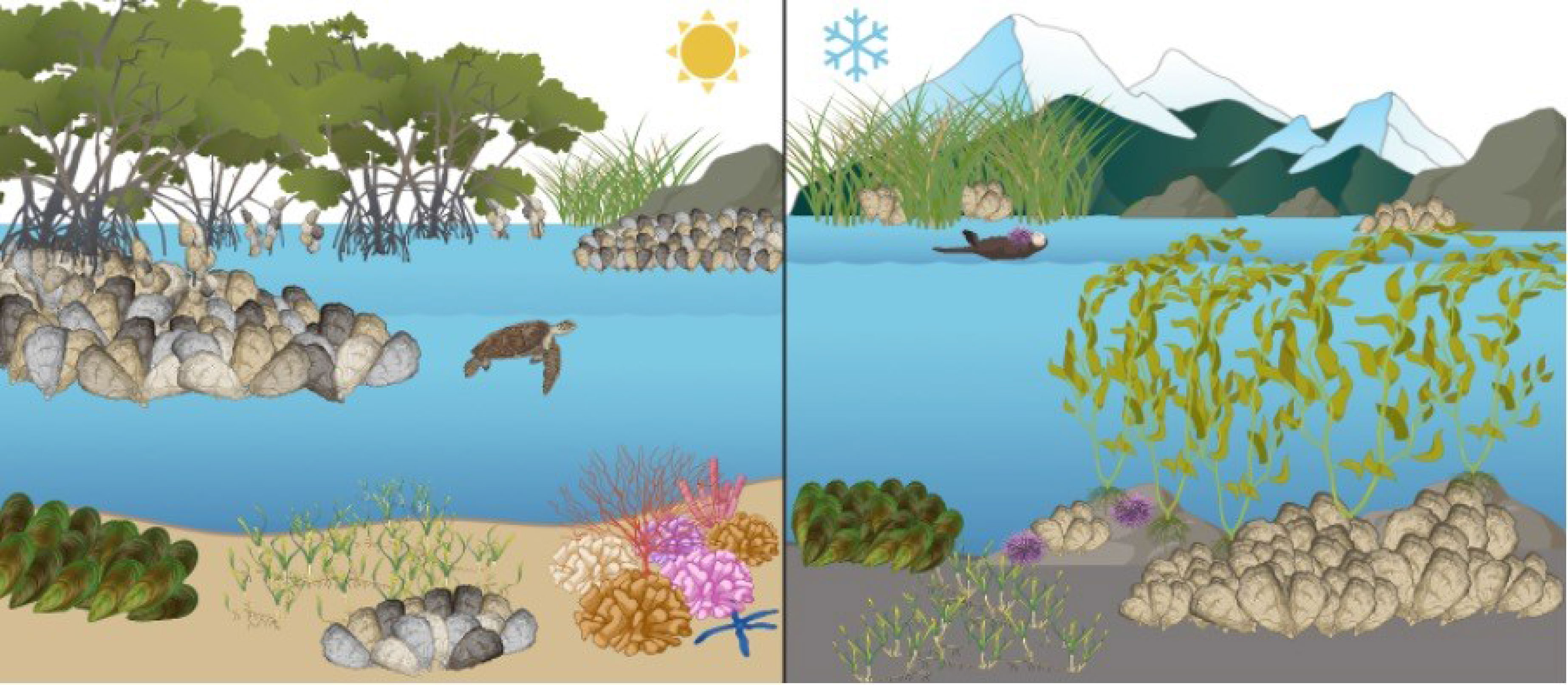
Graphical Abstract Graphical depiction of tropical oyster reefs (left) and temperate oyster reefs (right).
Introduction
Cumulative pressures of pollution, overfishing and climate change have resulted in the destruction of marine ecosystems around the world. The loss of these ecosystems, for example, coral reefs (Palandro et al., 2008; De’ath et al., 2012), mangroves (Goldberg et al., 2020), saltmarshes (Silliman et al., 2009), and kelp (Filbee-Dexter and Wernberg, 2018), has subsequently resulted in the loss of marine biodiversity as well as the ecosystem services that these habitats provide (Sala and Knowlton, 2006; Barbier, 2017). Oysters are ecosystem engineers that are found globally in shallow coastal and estuarine waters, and can grow in dense aggregations to form three-dimensional biogenic structures (Beck et al., 2011). These structures are often called ‘reefs’, ‘banks’, or ‘beds’, depending on the physical shape of oyster aggregations, i.e., either high or low profile structures, clumped aggregations in soft sediment, or encrusting veneers on rocks and other hard substrates (Alleway et al., 2015; Mcleod et al., 2020). Here we will refer to all of these structures as ‘reefs’. Oyster reefs provide important ecosystem services including shoreline stabilization, water filtration, nutrient assimilation, and the provision of habitat for marine life including commercially important fishes and crustaceans (Coen et al., 2007; Grabowski and Peterson, 2007; Hancock and Zu Ermgassen, 2019). Destructive and unsustainable harvesting coupled with pollution, disease, and coastal development, has destroyed 85% of oyster reefs globally, making them one of the most imperilled marine ecosystems (Beck et al., 2011).
Despite the magnitude of oyster reef loss, restoration efforts, including over 5000 hectares of Crassostrea virginica reefs restored on the east coast of the USA, show that restoration is possible (Hernández et al., 2018). Restoration has now expanded to other species including Ostrea lurida on the west coast of the USA and Canada (Pritchard et al., 2015), Ostrea edulis in Europe (Pogoda et al., 2019; Preston et al., 2020), and Ostrea angasi and Saccostrea glomerata in Australia (Alleway et al., 2015; Gillies et al., 2018). More research has been conducted on C. virginica than all other species combined, and there is now a need to broaden research to a wider range of reef-building bivalves to provide ecological evidence to inform restoration beyond the United States (Toone et al., 2021). A review of the benefits of other bivalve species that are targets of shellfish reef restoration demonstrated that they provide similar ecosystem services to those provided by C. virginica (Zu Ermgassen et al. (2020), however most of these species reviewed are subtropical and temperate. Very little is known about tropical oyster reefs. Tropical marine systems generally harbour greater biodiversity than temperate systems (Gray, 1997; Roberts et al., 2002) and this also holds true for oysters where the diversity of species is higher in the tropics (Guo et al., 2018). Despite this, tropical oysters remain underrepresented in global reviews and oyster reef restoration manuals (Beck et al., 2011; Fitzsimons et al., 2019; Fitzsimons et al., 2020; Zu Ermgassen et al., 2020).
Restoration of tropical marine systems such as coral reefs (Meesters et al., 2015), seagrass (Paling et al., 2009) and mangroves (Hashim et al., 2010; Su et al., 2021) is occurring, but to date there has been no documentation of tropical oyster reef restoration in the scientific literature. Oyster reefs occur throughout tropical inshore coastal and estuarine systems (Naik and Gowda, 2013; Lau et al., 2020) but knowledge of these assemblages, including the extent of occurrence, the identity of the dominant ecosystem engineers, their biology and ecology, and the benefits they provide, remains poorly understood. Oysters exhibit high levels of morphological plasticity and molecular techniques are required for accurate identification (Sekino and Yamashita, 2016). These techniques have only recently been applied in the tropics, revealing an unexpectedly high diversity of oysters and a number of new and undescribed species (Guo et al., 2018; Mcdougall et al., 2020; Al-Kandari et al., 2021; Cui et al., 2021). Obtaining foundational information for tropical reef-building oysters is an important precursor for assessing their conservation status, ecological significance, and potential for restoration.
The aim of this review is to elucidate the global diversity of reef-building oysters, summarise the known biology and ecology of tropical oyster reefs and the ecosystem services that they provide, and identify functional differences between tropical and temperate oyster reefs to guide future oyster reef restoration in the tropics. We cover five key topics: 1) global diversity and distribution of reef-building oysters; 2) the decline of tropical oyster reefs; 3) biology and ecology of tropical reef-building oysters; 4) ecosystem benefits of tropical oyster reefs; and 5) addressing tropical oyster reef knowledge gaps to guide restoration.
Assessing the Global Diversity and Distribution of Reef-Building Oysters
To date, no assessments have estimated the global diversity of reef-building oysters in shallow coastal and estuarine waters. Previous studies focus on the most common and widely distributed species or those important to aquaculture, meaning some species are yet to be reported definitively as reef-building or non-reef-building (Gillies et al., 2018). Our limited understanding of which species of oysters are reef-building is a problem when planning and setting objectives for oyster reef restoration. In this review, we considered “reef-building” to be oysters that created biogenic habitat by settling gregariously (when oysters attach and grow on each other to form clumps) on soft sediment and/or hard substrates, and excluded endobenthic species that reside in sediments (Gillies et al., 2018).
Compiling Reef-Building Oyster Species
To assess the global diversity of reef-building oysters, we compiled a list of oyster genera known to contain reef-building species from reef restoration reviews and reports (Alleway et al., 2015; Fitzsimons et al., 2019; Pogoda et al., 2019; Zu Ermgassen et al., 2020). From this search five genera were identified: Crassostrea, Isognomon, Ostrea, Pinctada and Saccostrea. Oyster species have undergone extensive taxonomic reclassifications (Salvi and Mariottini, 2016; Guo et al., 2018). To account for reclassified species, we searched for reassigned and alternative representations of identified genera in the World Register of Marine Species (WoRMS), an authoritative and comprehensive list of names of marine organisms, including information on synonymy (https://www.marinespecies.org). From this search we identified the additional genera Hyotissa, Lopha, Magallana, Planostrea, Pteria, Striostrea, and Talonostrea. Reassigned genera from Ostrea were excluded from this search as all oysters were originally described as this genus (Linnaeus, 1758).
To create a species list, we searched each genus using an advanced search for “Status = accepted” and “Fossil = extant, not fossil-only” in WoRMS. This search returned a total of 156 species. All species discussed in this review are referred to by their accepted name as they appear in WoRMS which includes the accepted reclassification of some Crassostrea species as Magallana (Salvi and Mariottini, 2016). Once species were identified, we searched for reviews in Google Scholar using the search terms “species name” + “review”. Where reviews were found, evidence of reef-building (reference to reef-building, habitat-forming, restoration, existing reefs, or gregarious settling) was recorded. For each species, we then searched the terms “species name” + “beds” OR “reefs” OR “banks” OR “restoration” to document existing reefs or evidence of reef-building where reviews did not exist. These search terms were repeated using alternative representations and past species names when accepted names did not yield reviews or reef-building evidence. If these search terms did not yield papers, we searched “species name” to scan additional papers for evidence of reef-building (as described above) in understudied species.
Assessing Reef-Building Oyster Distributions and Ecological Interactions
Once reef-building oysters were identified, we recorded their native distributions from reviews and original papers published in English. Almost all species had some subtropical distributions. To delineate which species were tropical or temperate, we classed species with subtropical and temperate distributions as temperate and species with subtropical and tropical distributions as tropical. Species found in tropical, subtropical, and temperate regions were classed as eurythermal. Species that only had subtropical distributions in the Arabian Gulf have been considered tropical as these regions are separated from temperate zones and are latitudinally closer to the tropics. We also recorded any reported instances of oysters co-habiting to form mixed-species assemblages within native distributions.
To explore the ecology of oysters in tropical waters, we searched literature for interactions between tropical and eurythermal oyster species and co-occurring habitats. Interactions were garnered from identified reviews and, where a review did not exist, we searched Google Scholar using the terms “species name” + “coral” OR “seagrass” OR “mangrove” OR “saltmarsh”. These search terms were repeated using alternative representations and past species names when accepted names did not yield results. For a full analysis, species list and references see Supplementary Material. For a justification of species inclusions and exclusions see Supplementary Methods.
Global Diversity of Reef-Building Oysters
We found a total of 38 species of reef-building oysters from eight genera: Crassostrea, Isognomon, Magallana, Ostrea, Pinctada, Saccostrea, Striostrea and Talonostrea. Evidence of gregarious settling (indicating reef-building) was found for three species (Crassostrea columbiensis, Pinctada margaritifera and Talonostrea zhanjiangensis); these have been included in our diversity count. Tropical regions harbour over four times greater diversity of reef-building oysters than temperate regions with a total of 25 tropical species, six temperate species, and seven eurythermal species (Figure 1). A paucity of literature from tropical regions (Beck et al., 2011; Toone et al., 2021) coupled with ongoing taxonomic ambiguities has likely resulted in some species of reef-building oysters being undocumented or not yet described. For example, Saccostrea cucullata has been used as a catch-all name for what is likely multiple species comprised of at least nine genetically distinct lineages (Lam and Morton, 2006; Guo et al., 2018), while the reef-building species Talonostrea salpinx was only described in 2021 (Al-Kandari et al., 2021). Our diversity count is therefore conservative and will likely increase as the taxonomy of these groups is refined.
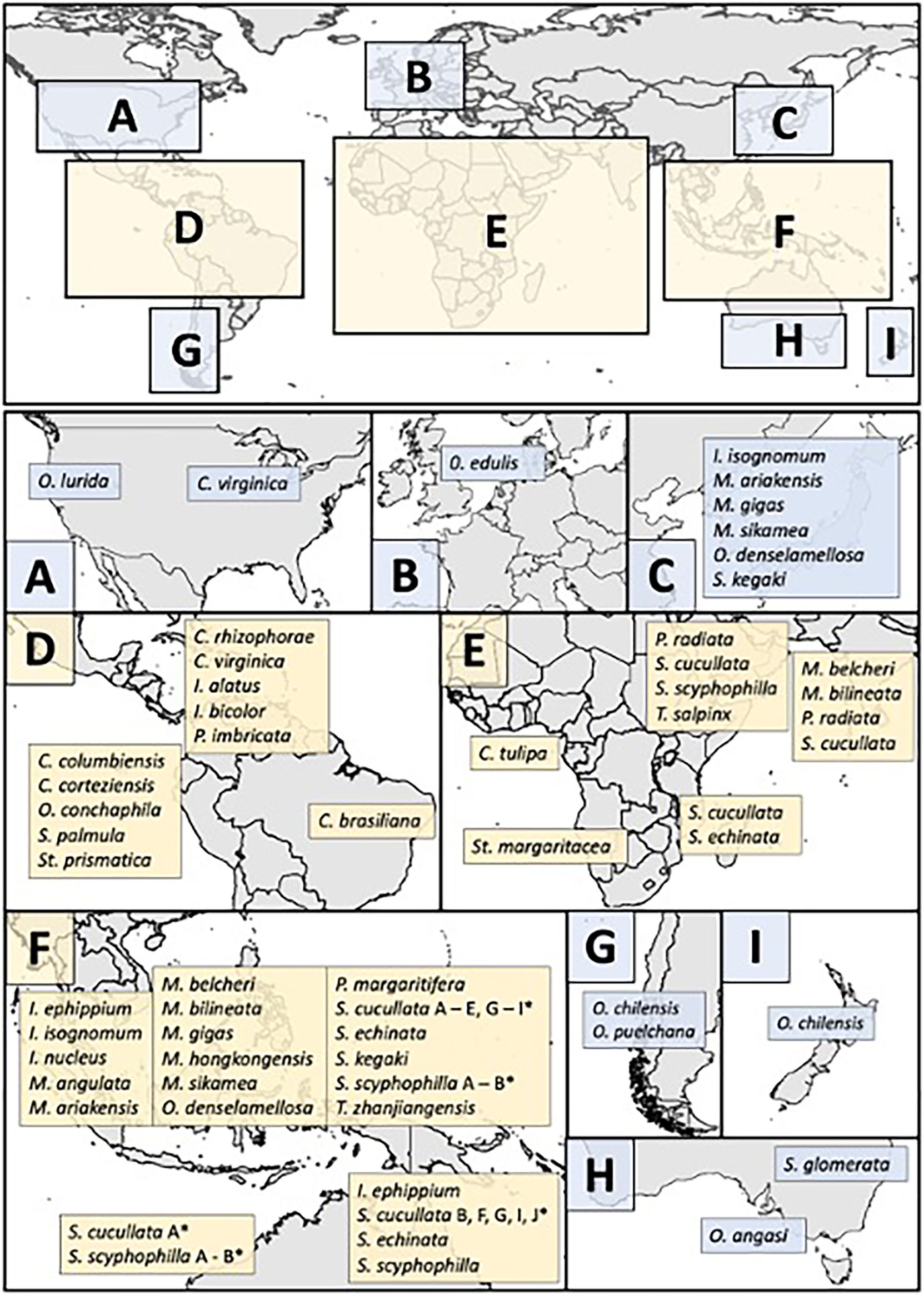
Figure 1 Global distribution of native reef-building oysters (tropical, eurythermal and temperate). Blue boxes (A–C) and (G–I) are temperate regions while yellow boxes (D–F) are tropical regions. C, Crassostrea; I, Isognomon; M, Magallana; O, Ostrea; P, Pinctada; S, Saccostrea; St, Striostrea; and T, Talonostrea. An asterisk* denotes multiple lineages which are likely to be multiple species as per Lam and Morton (2006) and Sekino and Yamashita (2016). For further details see Supplementary Methods.
The Decline of Tropical Oyster Reefs
Oyster reefs have declined in tropical regions, primarily as a result of over-exploitation and unsustainable harvesting. Reef conditions have been assessed in tropical South America and the Western Atlantic, and range from “Good” to “Functionally extinct” (Beck et al., 2009; Beck et al., 2011). Historical declines have also been documented in literature for Striostrea prismatica reefs in Costa Rica (Campos and Fournier, 1989; Rios Gonzalez et al., 2018), Crassostrea brasiliana in the Western Atlantic (Carranza et al., 2009; Westphal and Ostrensky, 2016), Pinctada imbricata in Venezuela (Romero, 2003), and Crassostrea rhizophorae in Venezuela (Carranza et al., 2009). Detailed assessments of reef conditions and extent are still lacking, however, possibly due to a lack of economic support and management interest in these systems in South American countries (Beck et al., 2009). This is also likely the case for tropical regions outside of South America and could be a contributing factor to their relative absence in existing global assessments and reviews.
There is very little information documenting the decline of tropical oyster reefs outside South America. Some local declines have been documented for pearl oyster reefs in Sri Lanka and Qatar (Smyth et al., 2016; Ostroff, 2020), mixed Magallana belcheri and Magallana bilineata reefs in India (Alagarswami and Narasimham, 1973; Nagi et al., 2011), and Magallana bilineata and Magallana hongkongensis reefs in Hong Kong (Lau et al., 2020). Talonostrea salpinx reefs in Kuwait have also declined, with historical photographs showing how extensive and well developed the reefs once were (Al-Kandari et al., 2021). In the absence of historical records in the scientific literature, evidence for the presence and destruction of reefs can be obtained using indirect methods (i.e., proxies). Previous studies have inferred the historical presence of significant oyster populations from the location of place names including the word ‘oyster’, or from the presence of historical lime kilns as oysters were often the major raw ingredient for lime prior to the use of limestone (Gillies et al., 2018). Historical reef presence can also be garnered from grey literature such as archived newspaper articles (Gillies et al., 2018; Cook et al., 2021). Whist a complete analysis of the historical presence of tropical oyster reefs in all data poor regions was outside the scope of this study, we tested our hypothesis using two case studies to assess the historical presence and decline of tropical oyster reefs. These case studies outline two easily replicable methods using alternate sources of information that can be applied to other data poor regions in the tropics.
Case Study 1: Pearl River Delta, China
We chose the Pearl River Delta, China (which includes Hong Kong and Macao) for our first case study. This region was selected due to the presence of existing, degraded oyster reefs (Beck et al., 2011; Lau et al., 2020) and the world’s longest recorded history of oyster cultivation dating back 1000 years (Cheung, 2019). Oyster reef restoration projects in this region are also underway, however monitoring and results have not yet been reported on. In Shenzen Bay, naturally high abundances of oysters once supported cultivated reefs that covered 5123 hectares, however these reefs ceased to operate by the 1980s due to a series of pollution crises (Liu, 1995; Watson, 2022). Lime-burning was one of Hong Kong’s oldest and most prosperous industries where shell was burned to create lime for many purposes including construction, plastering, brickmaking and curing leather (Tak-Yan, 1984; Watson, 2022; Weng et al., 2021). The process of lime-burning involved burning live oysters as well as dredged shell, which removed hard substrates essential for new oyster growth. Lime kilns were once prevalent across Hong Kong, with the island of Ping Chau described as “having lime kilns thick along the sheltered western and southern shores” (Schofield, 1983).
To search for evidence of undocumented historic oyster reefs and their decline, we searched Google Maps for place names containing ‘oyster’ (‘蚝’ and ‘蠔’ means oyster), historic lime kilns, and the term ‘fui yiu ha’ which means ‘near a lime kiln’ (Schofield, 1983). We identified 10 historical lime kiln sites (which contained multiple kilns), and 14 oyster place names including Macao which was previously called “Hao Jing Ao” which translates to “oyster mirror bay” (Figure 2). For a full list of place names and co-ordinates, see Supplementary Material. These results indicate that oysters, and likely reefs, once had a much wider distribution across the lower basin of the Pearl River Delta than they do now.
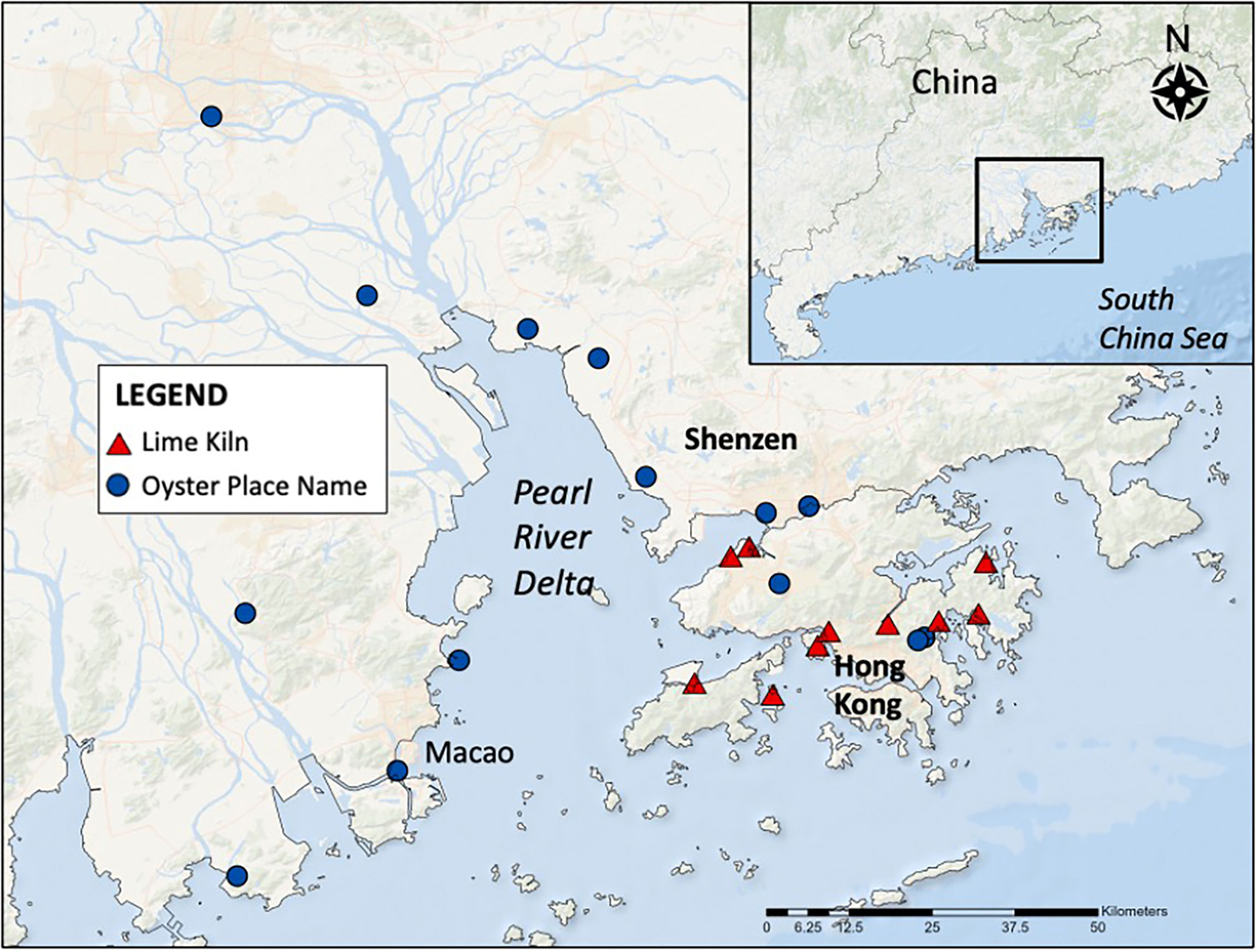
Figure 2 A map of the lower basin of the Pearl River Delta displaying oyster place names and historic lime kilns.
Case Study 2: Central and Far North Queensland, Australia
For our second case study, we chose tropical Queensland, Australia, where the historical presence of oyster reefs remains unknown. We searched newspaper articles (National Library of Australia Trove database; http://trove.nla.gov.au/) for evidence of oyster reefs by searching the terms “oyster beds” and “oyster reefs” in 24 newspapers from coastal towns north of Seventeen Seventy between the earliest available year and 1939 (after which harvest years in northern Queensland had peaked, (Alleway et al., 2015). Reference to plural “beds” or “reefs” were recorded as two reefs unless a higher number was explicitly stated. From this search we conservatively identified 94 historic reefs across 58 sites (Figure 3). For a full list of Trove newspaper results, see Supplementary Material. Reef size and structure was rarely mentioned, however one reef in Cape Upstart (Figure 3B) was reportedly “three quarters of a mile long” while in The Narrows (Figure 3C), layers of old oyster shells were up to 1.2 m thick, and calcareous rocky reefs rose 1.5 m above the water “carrying millions of oysters and extending along the banks for hundreds of yards”. Although the current extent of oyster reefs in the region is unclear, declines over the last 110 years including smothering induced die-offs, overharvesting and destructive practices such as the use of dynamite in the Keppel Islands suggests that oyster reefs have been severely reduced (Mcleod et al., 2015). We found similar evidence for oyster reef declines, and overharvesting, pollution and dredging was reported in 16 sites from as early as 1902 (see Supplementary Material). Currently, Isognomon ephippium is the only species with documented reefs in tropical Queensland (Mcleod et al., 2015), however it is likely that most historic reefs we identified were Saccostrea sp as most references were made to oysters being eaten or commercially leased, and I. ephippium is generally not consumed (Benthotage et al., 2020).
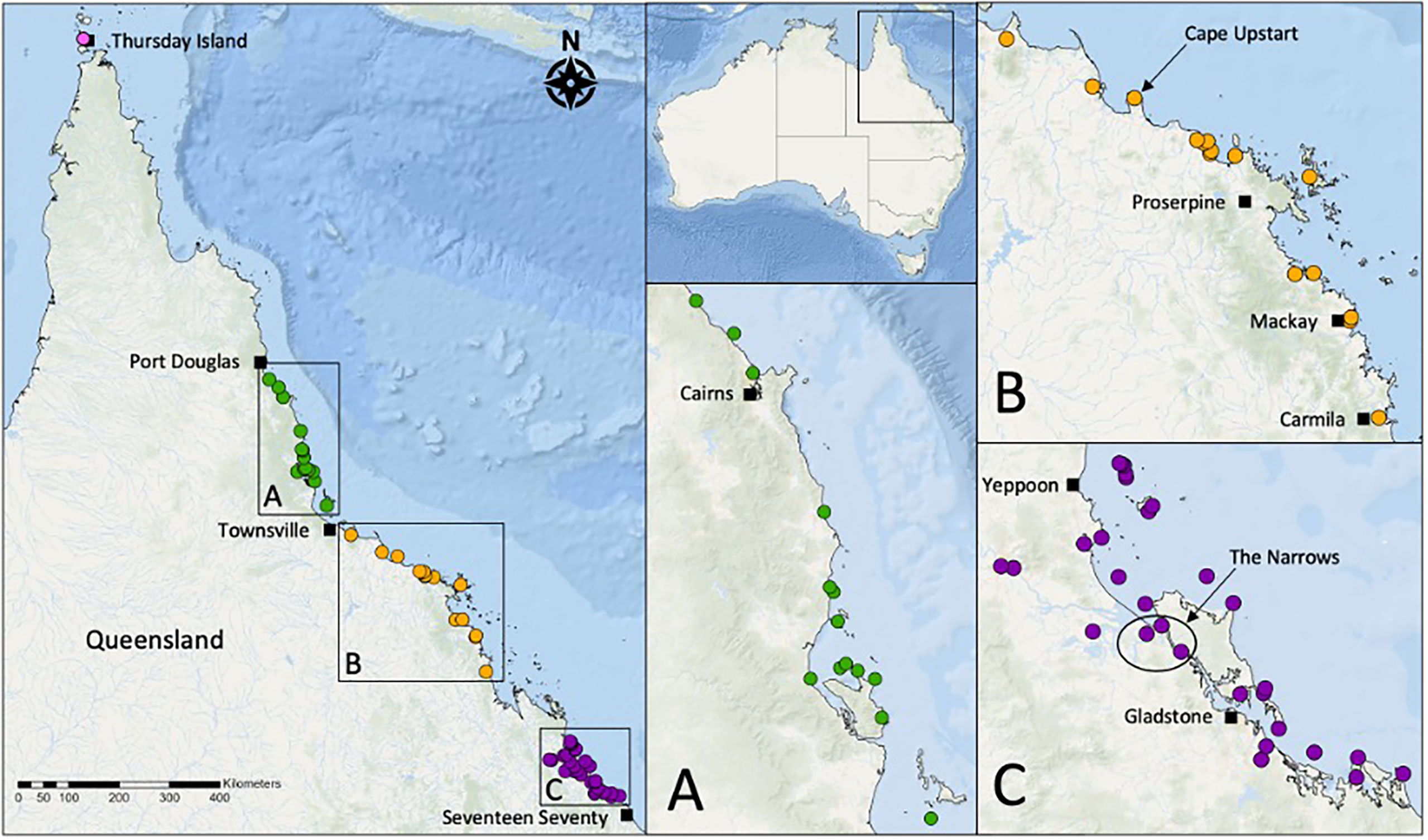
Figure 3 A map of historical oyster reef sites in tropical Queensland. (A) 23 reefs across 14 locations between Townsville and Port Douglas; (B) 22 reefs across 15 locations between Carmila and Townsville; (C) 45 reefs across 29 locations between Seventeen Seventy and Yeppoon. The pink site at the northern tip of Queensland represents at least four reefs comprised of pearl oysters and likely Saccostrea at Thursday Island.
Biology and Ecology of Tropical Reef-Building Oysters
Understanding the biology and ecology of tropical reef-building oysters is important to inform restoration approaches, as differences between temperate and tropical systems are likely which may affect the method of restoration. Knowing these differences will help to guide decision making and avoid potentially costly mistakes. The information in this section is a summary of what is currently known about tropical oyster reefs and reef-building species.
What Types of Reefs Do Tropical Oysters Build?
Like their temperate and subtropical congeners, tropical oysters are capable of building different types of reef structures. The shape of these structures includes high- and low-profile reefs, encrusting veneers on rocks and mangroves, and aggregations in soft sediment (Figure 4). Crassostrea brasiliana, Magallana hongkongensis, Pinctada radiata, Saccostrea cucullata and Striostrea margaritacea are capable of building reefs both intertidally and subtidally (Dye et al., 1994; Lam and Morton, 2004; Al-Khayat and Al-Ansi, 2008; Westphal and Ostrensky, 2016). The eurythermal species Ostrea denselamellosa exists subtidally in Hong Kong but it is unknown whether it builds reefs there (Lam and Morton, 2004). Other tropical species may be capable of building reefs subtidally, but this has not been documented in English literature. By comparison, four of the six temperate species are exclusively subtidal species [Ostrea angasi (Gillies et al., 2020), O. chilensis (Cranfield, 1979), O. edulis (Pogoda et al., 2019) and O. puelchana (Doldan et al., 2018)], and S. glomerata and O. lurida are capable of building reefs both subtidally and intertidally (Pritchard et al., 2015; Gillies et al., 2018). Despite the high diversity of tropical reef-building oysters that we identified, there is little evidence of extant remnant reefs in the tropics. Reported extant remnant reef information including location, reef area, oyster density, abundance, and biomass has been recorded in Table 1 (see Supplementary Material for references), however these systems are likely degraded and therefore may not represent healthy, historic numbers. The identification and characterisation of reference systems (remnant reefs with minimal degradation and healthy populations of oysters) are important for setting and monitoring restoration targets (Fitzsimons et al., 2019), and this represents a large knowledge gap for tropical oyster reefs.
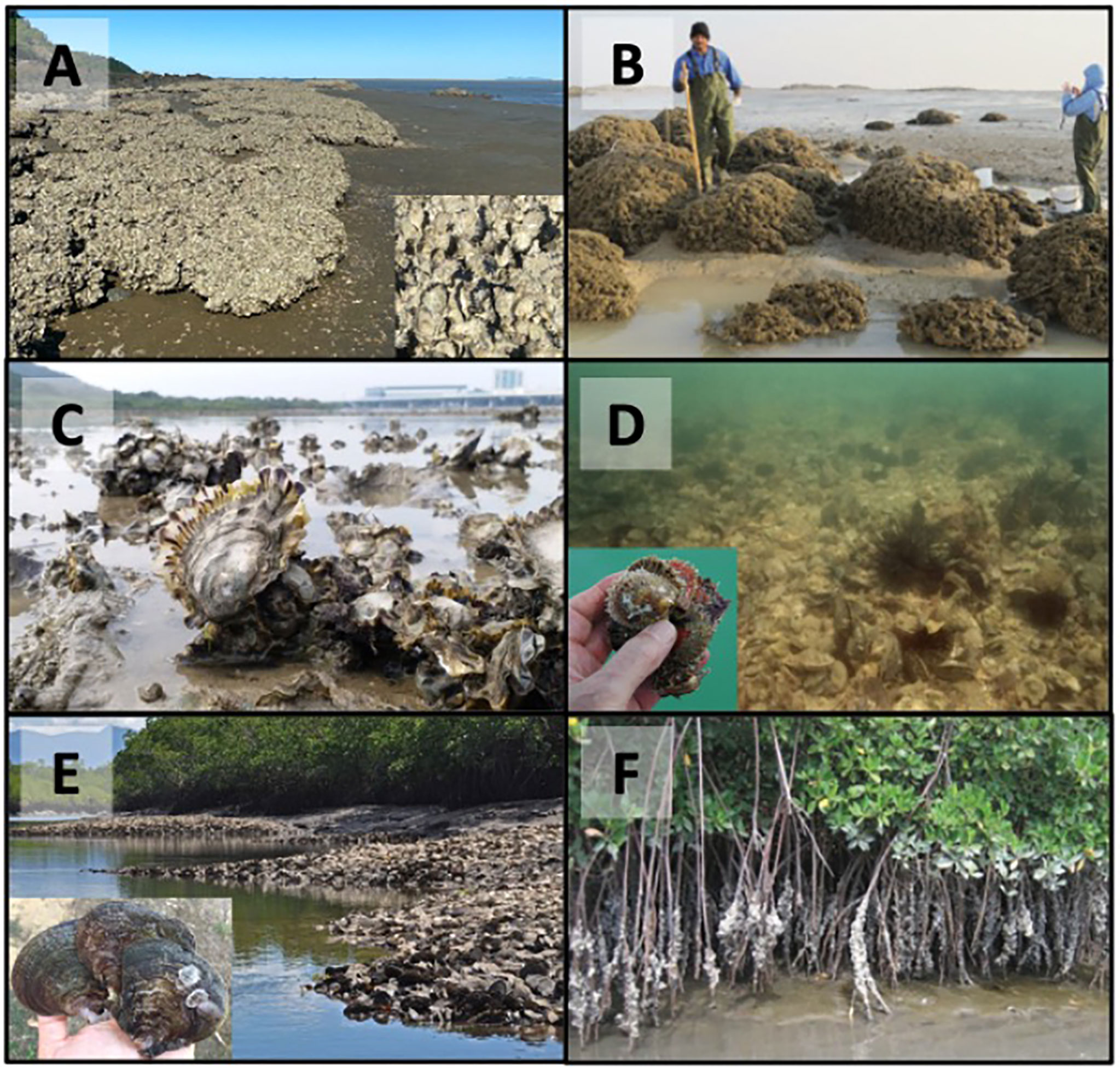
Figure 4 Examples of reef structures built by tropical oysters around the world. (A) Saccostrea sp reefs encrusting rocks in Far North Queensland, Australia; (B) Talonostrea salpinx reefs encrusting rocks in Kuwait (see Al-Kandari et al. (2021)); (C) Low profile intertidal Magallana sp reefs in Hong Kong, China; (D) A low profile subtidal Pinctada radiata oyster reef and gregarious clump near Dubai; (E) Low profile intertidal Isognomon ephippium reefs on muddy banks in the Hinchinbrook Channel, Queensland, Australia; (F) Crassostrea tulipa encrusting mangroves in The Gambia (see Crow and Carney (2013)). Photo credits: (A) – Simon Walker; (B) – Manal Al-Kandari; (C) – Marine Thomas (D) – Henry A Hänni; (E) – Ian McLeod; (F) – Judith Carney).
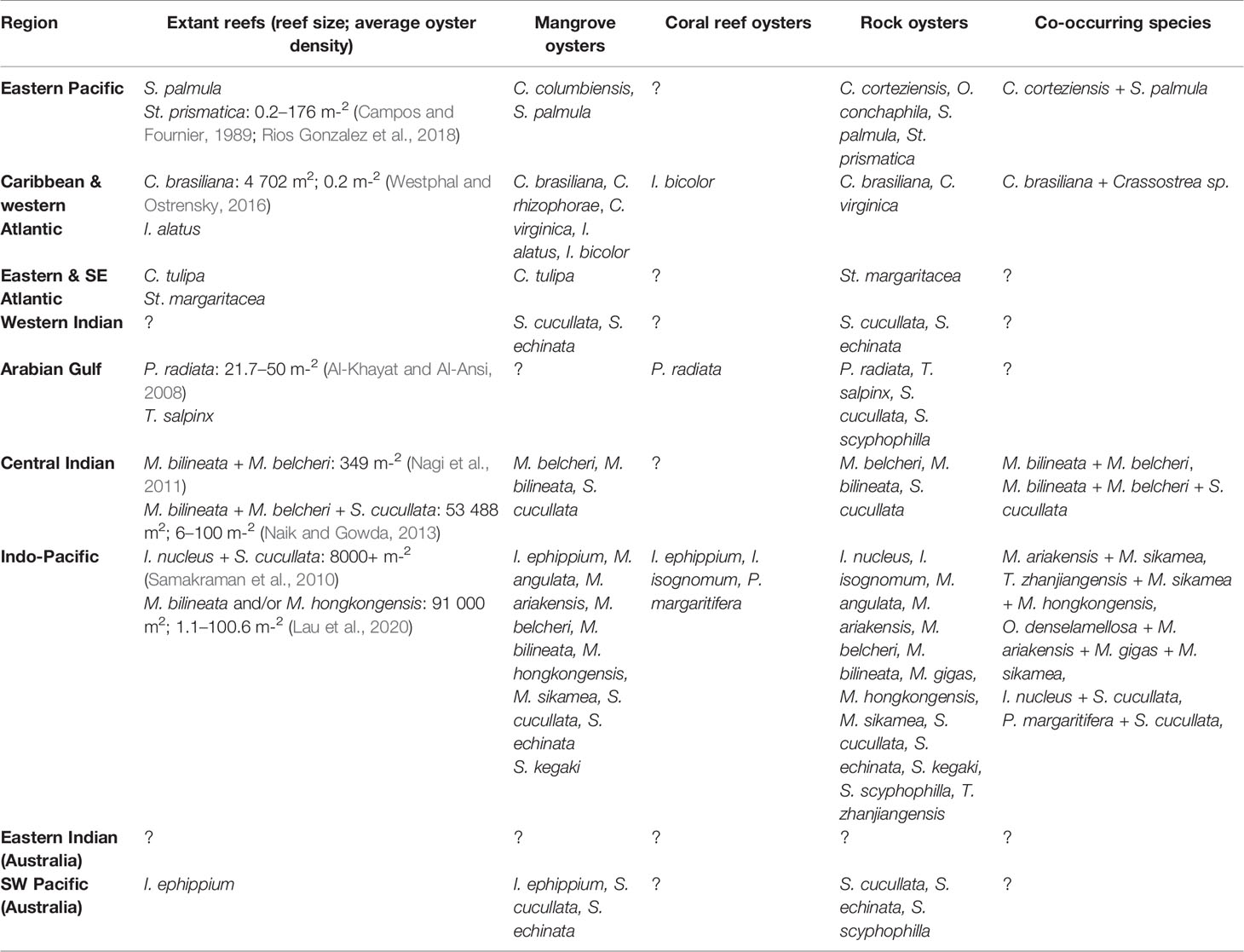
Table 1 Summary of tropical extant reefs, habitat associations and co-occurring tropical and eurythermal reef-building oysters.
Growth, Size and Reproduction
Tropical species of reef-building oysters typically grow and develop faster than temperate species. For example, Crassostrea brasiliana (Legat et al., 2017), Crassostrea tulipa (Crow and Carney, 2013), Crassostrea rhizophorae, Crassostrea corteziensis, Magallana belcheri, Magallana bilineata and Saccostrea cucullata (Angell, 1986) all reach market size, generally between 60 – 70mm in shell height/length, within 12 months or as little as six months in favourable conditions. By comparison all temperate species take a minimum of two years to grow to market size. The fastest growing temperate species is Saccostrea glomerata which takes approximately two years (Dove and O’connor, 2009), followed by Ostrea angasi (Crawford, 2016) and Ostrea edulis (Newkirk and Haley, 1982) which take 2-4 years, and Ostrea chilensis (Cranfield, 1979) and Ostrea lurida (Gillespie, 2009) which take 4-5 years. The fast growth rate of tropical species suggests that tropical oyster reefs may establish faster and provide ecosystem services quicker post restoration than temperate reefs.
Faster growth may be associated with larger adult sizes. Maximum adult size data has been reported for tropical species in Brazil and the American Pacific but is lacking in other parts of the world (Do Amaral and Simone, 2014; Do Amaral and Simone, 2016; Lodeiros et al., 2020). From what is known about the size of wild tropical oysters, there is great variability among species with the largest being Magallana belcheri which can reach 430 mm (Willan et al., 2021) and one of the smallest being Talonostrea salpinx which grows to a maximum of 41 mm (Al-Kandari et al., 2021). Size can be a useful indicator to distinguish between species (Lodeiros et al., 2020), but in areas where knowledge of species diversity and distributions is poor, smaller species can be misidentified as juveniles of larger species (Wu et al., 2013).
Like temperate species, tropical reef-building oysters in the Indo-Pacific and South America tend to follow the trend of summer spawning triggered by increasing water temperatures (Angell, 1986; Wu et al., 2013; Lodeiros et al., 2020). Unlike temperate species however, some tropical oysters can continuously spawn throughout the year. This has been specifically documented for Isognomon alatus, Crassostrea columbiensis, Crassostrea rhizophorae and Saccostrea palmula, and is likely the case for other tropical species (Angell, 1986; Benthotage et al., 2020; Lodeiros et al., 2020). Continuous spatfall throughout the year makes predicting spatfall difficult, as peaks have been correlated with salinity as well as temperature, and these parameters are highly variable in monsoonal areas (Angell, 1986). Reproductive strategies are generally not well documented for tropical reef-building oysters, and basic information about sexual maturity, reproductive cycles, fecundity and larval phases remain a knowledge gap for most species (Benthotage et al., 2020; Lodeiros et al., 2020). Summarizing species-specific information is further complicated by taxonomic ambiguities ( Do Amaral and Simone, 2014; Sekino and Yamashita, 2016), and studies published prior to the utilisation of molecular identification techniques may therefore be inaccurate (Angell, 1986; Lam and Morton, 2003; Wu et al., 2013).
Habitat Associations
Tropical oyster reefs interact with co-occurring habitats in a number of ways. For example, reef-building oysters require hard substrates for settlement, and tropical oysters can be found attached to rocks, mangrove roots and coral reefs, and many species rely on these habitats to form biogenic reef structures (Table 1). Driven by climate change, oyster reefs in parts of the subtropical southeast USA are being replaced by mangroves, primarily Rhizophora mangle, from tropical areas (Mcclenachan et al., 2021). Both the tropical Crassostrea rhizophorae and eurythermal Crassostrea virginica have been documented growing on the roots of R. mangle (C. rhizophorae exclusively so) (Aquino-Thomas and Proffitt, 2014; Lodeiros et al., 2020), however the preferred habitat of C. virginica is hard rocky substrates (Carranza et al., 2009). It is therefore possible that tropical oysters may redistribute into subtropical habitats under a changing climate, impacting temperate and eurythermal species. Assessment of which species are likely to persist under a changing climate should include research on their associated habitats as these relationships in tropical regions are poorly understood. These interactions could impact the broader marine environment as connectivity between oyster reefs and mangroves is an important factor in shaping fish and invertebrate community assemblages (Grabowski et al., 2005; Gilby et al., 2019).
There are likely to be differences in the interactions between oyster reefs and the same co-occurring habitat types between temperate and tropical regions. For instance, oyster reefs and seagrass meadows co-occur in both temperate and tropical systems. Positive and negative interactions have been documented between these ecosystems in subtropical and temperate environments (Booth and Heck, 2009; Wagner et al., 2012; Sharma et al., 2016; Valdez et al., 2020), however in a review of plant-bivalve interactions, positive interactions between plants and bivalves decreased in warmer intertidal areas, likely due to competition for space (Gagnon et al., 2020). Interactions between oyster reefs and seagrass in tropical waters should not be extrapolated from non-tropical studies without further research. Similarly, saltmarsh and oyster reefs co-occur in both tropical and temperate seascapes and positive interactions have been documented in subtropical and temperate regions (Chowdhury et al., 2019; Gagnon et al., 2020), but nothing is known about interactions in tropical regions.
Mixed Species Reefs
We found evidence that 15 species of reef-building oysters co-habited to form mixed-species assemblages in their native distributions; ten of these species were tropical, four were eurythermal, and only one was temperate (Table 1). Documentation of co-occuring reef-building oysters was highest in the Indo-Pacific, where we found the greatest diversity of oyster species. In southern China, Talonostrea zhanjiangensis was previously thought to be juvenile Magallana hongkongensis, a species it co-occurs with, until molecular analysis revealed that it was a new species (Wu et al., 2013). T. zhanjiangensis directly competes for space with M. hongkongensis, and commonly suffers mass mortalities after its first fast growth season. Restoration of M. hongkongensis therefore needs to consider competition for settlement space with T. zhanjiangensis where distributions overlap, and mechanisms to target M. hongkongensis recruitment such as timing substrate deployment with peak spawning times should be considered (Wu et al., 2013). The eurythermal species Magallana sikamea and Magallana ariakensis also form mixed species reefs, however the two species prefer to settle in the upper and lower intertidal zones respectively (Wang et al., 2020). Similar zonation patterns were observed in mixed Isognomon nucleus and Saccostrea sp populations in Thailand, with I. nucleus preferring to settle higher in the intertidal zone than S. cucullata (Samakraman et al., 2010). It is unclear whether these species compete for space or facilitate each other’s recruitment to niche tidal zones via chemical cues (Tamburri et al., 2008) or abiotic stress amelioration (Mcafee et al., 2018; Wang et al., 2020). Positive interactions between conspecific reef-building oysters, including settlement improvement and refugia from physical and biological stress, are well documented (Reeves et al., 2020). Interactions between co-occurring reef-building species however (both positive and negative), are poorly understood and it is unclear which species inhibit or facilitate the settlement of other species, and the mechanisms behind these processes. Similarly, it is unknown whether co-occurring species compete for food or if there are niche partitioning of food particle types and sizes between species, or whether they support unique faunal assemblages.
Oysters are not the only bivalves capable of building habitats. Mussels can also form three-dimensional biogenic reefs by settling gregariously (Gillies et al., 2018; Zu Ermgassen et al., 2020), however no studies in either temperate or tropical regions have investigated interactions between these two reef types. Oysters and mussels can also co-occur to build mixed species reefs (Christianen et al., 2018; Lipcius and Burke, 2018). Interactions in temperate reefs are known to affect ecosystem function; for example, in Chesapeake Bay, C. virginica and the hooked mussel Ischadium recurvum form mixed-species reefs that have double the filtration capacity of single-species reefs (Gedan et al., 2014; Lipcius and Burke, 2018). Habitat-building mussels have been observed on Pinctada radiata reefs in Qatar (Al-Khayat and Al-Ansi, 2008) as well as mixed Magallana bilineata x Magallana belcheri reefs in Pakistan (Aslam et al., 2020), demonstrating that this association occurs in tropical regions. It is unknown whether there are any interactions, positive or negative, between co-occurring mussels and oysters on tropical reefs. Similarly, no research has documented interactions between oysters and corals which are known to occur in the same reef habitats (Figure 5).
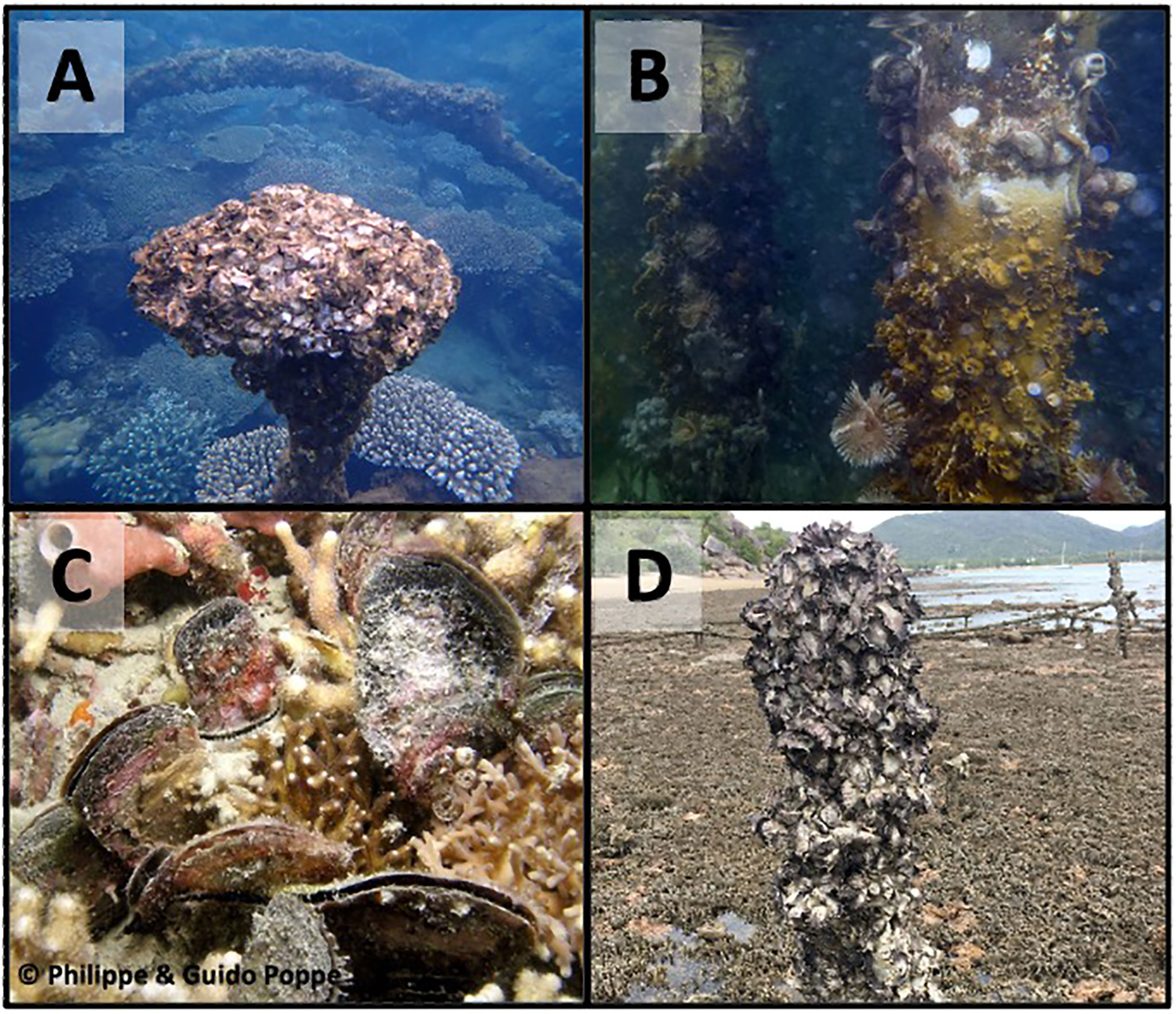
Figure 5 Examples of coral and reef-building oysters co-occurring in reef habitats. (A) Saccostrea sp clustering above coral at Moreton Island, Queensland; (B) Oysters, coral, hydroids and sponges co-habiting a dock at the Smithsonian’s Bocas Del Toro Marine Station in Panama; (C) Isognomon sp and coral co-occurring on a reef in the Philippines; (D) Multiple Saccostrea species clustering on aquaculture furniture on a coral reef at Magnetic Island, Queensland. Photo credits: (A) – Marina Richardson; (B) – Kristina Hall; (C) – Phillippe and Guido Poppe; (D) – Carmel McDougall.
Supported Biological Communities
Subtropical and temperate oyster reefs provide important habitat for fishes. Assessments of fish abundances, community assemblages and habitat use are well documented in the USA (Tolley and Volety, 2005; Hancock and Zu Ermgassen, 2019; La Peyre et al., 2019) and somewhat documented in other countries (Quan et al., 2009; Gilby et al., 2018b), however no research has documented relationships between oyster reefs and fishes in the tropics. This leaves a significant research gap and many questions yet to be answered. For example, to what degree do tropical oyster reefs support fish diversity and abundance? What types of fish community assemblages do tropical oyster reefs support? How do fish use tropical oyster reef habitats? What are fish movement patterns between intertidal reefs and other habitats? Do tropical oyster reefs support juvenile coral reef fish the way that mangroves and seagrasses do (Nagelkerken et al., 2001; Dorenbosch et al., 2005)? Given that habitat for fish, especially commercially important species, is a key ecosystem service of oyster reefs and driver of restoration in other parts of the world (Coen et al., 2007; Grabowski et al., 2012; Karp et al., 2018), this is possibly one of the most significant knowledge gaps for tropical oyster reefs.
Tropical oyster reefs provide habitat for invertebrate communities (Al-Khayat and Al-Ansi, 2008; Aslam et al., 2020), which can be greater in diversity, abundance and biomass compared to adjacent soft sediment habitats (Chan et al., 2022). The effects of oyster reef habitats on invertebrate communities, however, may vary depending on oyster traits and environmental settings (Bateman and Bishop, 2017). For example, on the subtropical and temperate east coast of Australia, the provision of predation refuge played a more important role in cooler estuaries, whereas shading by oysters had a larger effect on biodiversity in warmer estuaries (Mcafee and Bishop, 2019). Similarly, oyster habitat on rocky shores in tropical Hong Kong ameliorated environmental and organismal temperatures during summer low tides through the provision of cooler microclimates (Mcafee et al., 2018). Temperate and tropical oyster reefs are likely to support invertebrate communities dominated by different taxa and, given that invertebrate biodiversity is generally higher in the tropics, higher biodiversity of tropical reefs might be expected. While this question has not been explored in tropical zones, comparisons between temperate and subtropical oyster reefs and aquaculture sites do indicate some trends. In the USA, amphipods are often reported as one of the most abundant invertebrate taxa in temperate reefs and oyster farms, and gastropod densities are low (Rodney and Paynter, 2006; Karp et al., 2018; Ziegler et al., 2018; Ayvazian et al., 2020). At subtropical remnant and restored reefs, however, gastropods are reported as one of the dominant taxa while amphipods are one of the least dominant taxa (Boudreaux et al., 2006; Brown et al., 2014; De Santiago et al., 2019). Similar patterns were found between temperate and subtropical Saccostrea glomerata reefs on the east coast of Australia (Mcleod et al., 2020), and high gastropod and low amphipod abundances were also observed in mixed M. bilineata x M. belcheri reefs in Pakistan, Pinctada radiata beds in Qatar and M. belcheri aquaculture in Thailand (Al-Khayat and Al-Ansi, 2008; Kritsanapuntu and Chaitanawisuti, 2018; Aslam et al., 2020). It is unclear whether temperature is the only driver of these differences. Salinity can also shape invertebrate community assemblages (Karp et al., 2018) and other factors such as reef shape, size, location, or connection to adjacent habitats may also play a part.
Ecosystem Benefits of Tropical Oyster Reefs
We found reported evidence for three environmental and three social ecosystem services provided by oyster reefs in tropical systems. Global quantifications of oyster reef ecosystem services have been garnered primarily from studies in the USA, none of which are tropical (Coen et al., 2007; Grabowski and Peterson, 2007). Although it is likely that tropical oyster reefs provide similar benefits, we did not find any evidence for several potential services including enhanced fish and crustacean production (especially commercially important species), carbon sequestration, and positive effects on co-occurring habitats. Re-establishing ecosystem services is the main driver of oyster reef restoration, and there is therefore a need to quantify the ecosystem services provided by tropical oyster reefs.
Environmental Ecosystem Services
Tropical oyster reefs have been reported to protect shorelines, provide habitat, and act as bioindicators of water quality. They offer nature-based solutions to sea level rise and severe tropical storms by ameliorating wave action and shoreline erosion (Chowdhury et al., 2019; Rodolfo and Lapus, 2021). In Bangladesh, growth of 2 cm per year on installed oyster breakwater reefs kept pace with sea level rise while simultaneously allowing for the harvest of 5.6 kg oysters per m2 per year (Tangelder et al., 2015). Tropical oyster reefs also provide important habitat for marine invertebrates (Al-Khayat and Al-Ansi, 2008; Aslam et al., 2020) and shelter from heat stress for associated intertidal organisms during low tide (Mcafee et al., 2018). By filtering large volumes of water and particulates, oysters are capable of assimilating and bioaccumulating nutrient loads in the water (Kellogg et al., 2013) and, in developed areas, pollutants, microplastics and other contaminants can be traced by using tropical oysters as bio-indicators (Patterson et al., 2019; Patra et al., 2021). The tropical species Magallana hongkongensis and M. bilineata have some of the highest known filtration rates in the world and can filter 30 L/hour when they are only 90 mm in size (Lau et al., 2020). Filtration rates of other tropical species is missing from literature, but given that M. hongkongensis can reach adult sizes of 160 mm under current high harvest pressures (Lam and Morton, 2003), though individuals >300 mm have been recorded, and that tropical species experience warm conditions year-round thus avoiding slower feeding rates in cool temperate conditions (Gray and Langdon, 2018; Eymann et al., 2020), it is likely that mature tropical oysters could have higher rates of filtration than temperate species.
Social Ecosystem Services
Coastal communities in developing countries provide an important social context when assessing the benefits of tropical oyster reefs, with many heavily reliant on marine resources for food and income (Bell et al., 2009; Haque et al., 2009; Crow and Carney, 2013). Almost 100% of oyster farming in the tropics rely on wild caught spat (Nowland et al., 2020), meaning that dependent coastal communities are reliant on the health of wild oyster populations. In particular, harvesting wild and farmed oysters provides employment opportunities for women with low levels of education and economic opportunity (Szuster et al., 2008; Crow and Carney, 2013; Fao, 2020). In The Gambia, for example, there is an expectation for women to provide for their families and oyster harvesting is primarily driven by an aversion to stigmatised waged labour (Lau and Scales, 2016). Oyster farming can also provide broader cultural benefits, for example, in Vietnam oyster farming gives local people a sense of place by providing incomes and a community where people can practice culture (Pierce and O’connor, 2014).
Addressing Tropical Oyster Reef Knowledge Gaps to Guide Restoration
Oyster reef restoration requires the consideration of historical presence, proximity to existing reefs, and environmental and physical parameters to select appropriate restoration sites (Zu Ermgassen et al., 2016; Fitzsimons et al., 2019), however for many tropical species this information is lacking. In low recruitment areas where oyster spat seeding may be necessary, or where multiple reef-building oysters exist, species selected or targeted for restoration should be chosen based on project goals, desired ecosystem services, and resilience to stressors and climate change (Howie and Bishop, 2021). Species selection is likely to be of greater importance in the tropics due to the higher diversity of reef-building oyster species, and therefore restoration candidates, compared to temperate regions where there may only be one or two species to choose from. Current taxonomic ambiguities in the tropics may lead to the misidentification of species with overlapping distributions, and the accidental selection of unsuitable restoration candidates, particularly the superspecies Saccostrea cucullata (Lam and Morton, 2006). Significant background research is needed to fill basic oyster biology knowledge gaps to support successful restoration projects.
Oyster reef restoration that complements the restoration of co-occurring seagrasses, saltmarshes and mangroves is likely to yield greater ecosystem benefits and greater restoration success (Gilby et al., 2018a; Mcleod et al., 2019), however this information is also lacking in the tropics. Equally, it is important to consider possible deleterious effects of oyster reef restoration on co-occurring ecosystems. Oyster harvesting in The Gambia has resulted in the destruction of mangroves when roots are slashed to collect Crassostrea tulipa, and wood is harvested to smoke and preserve oysters (Crow and Carney, 2013). Restoring oyster reefs in regions with widespread poverty where people are reliant on marine resources therefore needs to identify and mitigate unintentional deleterious effects on co-occurring habitats. Similarly, restoration in these regions will likely need to account for harvesting by local communities.
To guide research and remove barriers to successful tropical oyster reef restoration, we identified nine key tropical oyster reef knowledge gaps: 1) current extent and loss of tropical oyster reefs, including the identification of existing threats; 2) taxonomic ambiguities of reef-building oysters, particularly in the Indo-Pacific and Africa; 3) the identification and characterisation of tropical oyster reef reference systems, including reef size and oyster density, abundance and biomass; 4) tropical reef-building oyster reproduction, including sexual maturity, reproductive cycles, fecundity and larval phases; 5) susceptibility of different reef-building species to a changing climate; 6) relationships between tropical oyster reefs and co-occurring habitats, specifically seagrass, saltmarsh, mussel beds and coral reefs; 7) interactions between tropical reef-building oysters, mussels and corals; 8) supported biological communities and reef features that shape them; 9) quantified ecosystem services, particularly the provision of habitat for harvestable fish and crustaceans, filtration, and carbon sequestration.
Key Biological Differences Between Temperate and Tropical Oyster Reefs
We identified four key differences between temperate and tropical oyster reefs; 1) the diversity of reef-building oysters is over four times higher in tropical compared to temperate regions; 2) tropical reef-building oysters can have continuous spatfall throughout the year whereas temperate species have a defined season; 3) tropical reef-building oysters are generally faster growing than temperate reef-building oysters; and 4) tropical oysters commonly create mixed-species oyster reefs whereas temperate oyster reefs are generally formed by a single oyster species. These differences have implications for restoration and the provision of ecosystem services, as novel management strategies will likely be required or be more appropriate in the tropics. For example, temporary closures to allow oysters to reach sexual maturity before harvesting is more feasible for fast growing tropical oysters than it is for slower growing temperate species (Angell, 1986; Crow and Carney, 2013; Lau et al., 2020). In the USA, Grabowski et al. (2012) estimated that oyster reef restoration costs could be recovered in as little as 2-14 years. Given that oysters can grow faster in the tropics, it is possible that restoration costs could be recovered even faster. Naturally occurring mixed-species reefs in the tropics also provides the potential for restoring reefs using multiple species of oysters where known interactions occur, should the anticipated benefits outweigh any additional costs in doing so. Tropical oyster aquaculture is currently reliant on wild caught spat (Nowland et al., 2020), which is likely to be the case for restoration as well (at least initially). Future studies that identify biological differences between tropical species of reef-building oysters will be key to designing future reefs that target the recruitment of multiple species.
Conclusion
Oyster reefs are biodiverse habitats in tropical coastal areas that benefit people through the provision of environmental and social ecosystem services. There is limited evidence in the scientific literature that tropical reefs have suffered large declines similar to temperate reef systems, although this is likely due to under recording in the scientific (English) literature rather than an accurate account of their conservation status. We found evidence of oyster reefs throughout the tropics in the past through other means such as place name proxies and historical accounts, suggesting that tropical oyster reefs were once much more common than they are today. A proper assessment of historical distributions of oyster reefs in the tropics, including the identification of present threats, would be valuable for future research and conservation. Taxonomic ambiguities for tropical species remain, and there is a need for research that documents and describes the biology of remnant tropical reefs and the ecosystem services that they provide. Filling the knowledge gaps highlighted in this review will produce foundational knowledge and remove barriers to tropical oyster reef restoration. Research to address these gaps could be co-ordinated among research institutions, non-governmental organisations, governments, and local communities to ensure that all stakeholders are represented. No large-scale oyster reef restoration has occurred in the tropics to date and, moving forward, careful consideration is needed in regions where the social context, biology and ecology of tropical oyster reefs differs to that of temperate reefs.
Author Contributions
MR, RC, CG and CM conceptualized the study. MR undertook the review, analysed the data, and produced figures. YZ identified and translated Chinese place names and literature. MR wrote the initial draft and all authors edited and reviewed the manuscript. All authors contributed to the article and approved the submitted version.
Funding
Open access funds provided by Griffith University.
Conflict of Interest
The authors declare that the research was conducted in the absence of any commercial or financial relationships that could be construed as a potential conflict of interest.
Publisher’s Note
All claims expressed in this article are solely those of the authors and do not necessarily represent those of their affiliated organizations, or those of the publisher, the editors and the reviewers. Any product that may be evaluated in this article, or claim that may be made by its manufacturer, is not guaranteed or endorsed by the publisher.
Acknowledgments
Thank you to Manal Al-Kandari, Kristina Hall, Henry A. Hänni, Judith Carney, Ian McLeod, Phillippe and Guido Poppe, Marine Thomas and Simon Walker for providing images for use in this publication, and to Michael Traurig for assistance with Figures 2, 3. We would also like to thank the reviewers for their suggestions that have improved this manuscript.
Graphical abstract Image attribution: Integration and Application Network, University of Maryland Center for Environmental Science (ian.umces.edu/imagelibrary/) and BioRender (app.biorender.com). This figure was created in BioRender.
Supplementary Material
The Supplementary Material for this article can be found online at: https://www.frontiersin.org/articles/10.3389/fmars.2022.873768/full#supplementary-material
References
Alagarswami K., Narasimham K. (1973). “Proceedings of the Symposium on Living Resources of the Seas Around India,” in Indian Council of Agricultural Research (ICAR), Special Publication Cochin: Central Marine Fisheries Research Institute), 648–658.
Al-Kandari M., Oliver P. G., Salvi D. (2021). Molecular and Morphological Systematics of a New, Reef Forming, Cupped Oyster From the Northern Arabian Gulf: Talonostrea Salpinx New Species. ZooKeys 1043, 1–20. doi: 10.3897/zookeys.1043.66992
Al-Khayat J. A., Al-Ansi M. A. (2008). Ecological Features of Oyster Beds Distribution in Qatari Waters, Arabian Gulf. Asian J. Sci. Res. 1, 544–561. doi: 10.3923/ajsr.2008.544.561
Alleway H. K., Crawford C., Creighton C., Diggles B., Ford J., Gillies C. L., et al. (2015). “Shellfish Reef Habitats: A Synopsis to Underpin the Repair and Conservation of Australia’s Environmentally, Socially and Economically Important Bays and Estuaries”, in Report to the National Environmental Science Programme, Marine Biodiversity Hub. Eds. Gillies C. L., Creighton C., Mcleod I. M. (James Cook University, Townsville: Centre for Tropical Water and Aquatic Ecosystem Research (TropWATER) Publication).
Angell C. L. (1986). “The Biology and Culture of Tropical Oysters,” in ICLARM Studies and Reviews (Manila, Philippines: International Center for Living Aquatic Resources Management).
Aquino-Thomas J., Proffitt C. E. (2014). Oysters Crassostrea Virginica on Red Mangrove Rhizophora Mangle Prop Roots: Facilitation of One Foundation Species by Another. Mar. Ecol. Prog. Ser. 503, 177–194. doi: 10.3354/meps10742
Aslam S., Dekker H., Siddiqui G., Mustaquim J., Kazmi S. J. H. (2020). Biodiversity on Intertidal Oyster Reefs in the Hab River Mouth: 35 New Records From Pakistan. Regional Stud. Mar. Sci. 39, 101415. doi: 10.1016/j.rsma.2020.101415
Ayvazian S., Gerber-Williams A., Grabbert S., Miller K., Hancock B., Helt W., et al. (2020). Habitat Benefits of Restored Oyster Reefs and Aquaculture to Fish and Invertebrates in a Coastal Pond in Rhode Island, United States. J. Shellfish Res. 39, 563–587. doi: 10.2983/035.039.0306
Barbier E. B. (2017). Marine Ecosystem Services. Curr. Biol. 27, R507–R510. doi: 10.1016/j.cub.2017.03.020
Bateman D. C., Bishop M. J. (2017). The Environmental Context and Traits of Habitat−Forming Bivalves Influence the Magnitude of Their Ecosystem Engineering. Mar. Ecol. Prog. Ser. 563, 95–110. doi: 10.3354/meps11959
Beck M. W., Brumbaugh R. D., Airoldi L., Carranza A., Coen L. D., Crawford C., et al. (2009). Shellfish Reefs at Risk: A Global Analysis of Problems and Solutions (Arlington VA: The Nature Conservancy).
Beck M. W., Brumbaugh R. D., Airoldi L., Carranza A., Coen L. D., Crawford C., et al. (2011). Oyster Reefs at Risk and Recommendations for Conservation, Restoration, and Management. BioScience 61, 107–116. doi: 10.1525/bio.2011.61.2.5
Bell J. D., Kronen M., Vunisea A., Nash W. J., Keeble G., Demmke A., et al. (2009). Planning the Use of Fish for Food Security in the Pacific. Mar. Policy 33, 64–76. doi: 10.1016/j.marpol.2008.04.002
Benthotage C., Cole V. J., Schulz K. G., Benkendorff K. (2020). A Review of the Biology of the Genus Isognomon (Bivalvia; Pteriidae) With a Discussion on Shellfish Reef Restoration Potential of Isognomon Ephippium. Molluscan Res. 40, 286–307. doi: 10.1080/13235818.2020.1837054
Booth D. M., Heck K. L. (2009). Effects of the American Oyster Crassostrea Virginica on Growth Rates of the Seagrass Halodule Wrightii. Mar. Ecol. Prog. Ser. 389, 117–126. doi: 10.3354/meps08163
Boudreaux M. L., Stiner J. L., Walters L. J. (2006). Biodiversity of Sessile and Motile Macrofauna on Intertidal Oyster Reefs in Mosquito Lagoon, Florida. J. Shellfish Res. 25, 1079–1089. doi: 10.2983/0730-8000(2006)25[1079:BOSAMM]2.0.CO;2
Brown L. A., Furlong J. N., Brown K. M., La Peyre M. K. (2014). Oyster Reef Restoration in the Northern Gulf of Mexico: Effect of Artificial Substrate and Age on Nekton and Benthic Macroinvertebrate Assemblage Use. Restor. Ecol. 22, 214–222. doi: 10.1111/rec.12071
Campos J. A., Fournier M. L. (1989). El Banco De Ostrea Iridescens (Pterioida: Ostreidae) De Bahía Curú, Costa Rica. Rev. Biología Trop., 331–333.
Carranza A., Defeo O., Beck M. (2009). Diversity, Conservation Status and Threats to Native Oysters (Ostreidae) Around the Atlantic and Caribbean Coasts of South America. Aquat. Conserv. 19, 344–353. doi: 10.1002/aqc.993
Chan S. S. W., Wong H. T., Thomas M., Alleway H. K., Hancock B., Russell B. D. (2022). Increased Biodiversity Associated With Abandoned Benthic Oyster Farms Highlight Ecosystem Benefits of Both Oyster Reefs and Traditional Aquaculture. Front. Mar. Sci. 9. doi: 10.3389/fmars.2022.862548
Cheung S. C. (2019). Floating Mountain in Pearl River: A Study of Oyster Cultivation and Food Heritage in Hong Kong. Asian Educ. Dev. Stud 8 (4), 433–442. doi: 10.1108/AEDS-02-2018-0048
Chowdhury M. S. N., Walles B., Sharifuzzaman S., Hossain M. S., Ysebaert T., Smaal A. C. (2019). Oyster Breakwater Reefs Promote Adjacent Mudflat Stability and Salt Marsh Growth in a Monsoon Dominated Subtropical Coast. Sci. Rep. 9, 1–12. doi: 10.1371/journal
Christianen M. J. A., Lengkeek W., Bergsma J. H., Coolen J. W. P., Didderen K., Dorenbosch M., et al. (2018). Return of the Native Facilitated by the Invasive? Population Composition, Substrate Preferences and Epibenthic Species Richness of a Recently Discovered Shellfish Reef With Native European Flat Oysters (Ostrea Edulis) in the North Sea. Mar. Biol. Res. 14, 590–597. doi: 10.1080/17451000.2018.1498520
Coen L. D., Brumbaugh R. D., Bushek D., Grizzle R., Luckenbach M. W., Posey M. H., et al. (2007). Ecosystem Services Related to Oyster Restoration. Mar. Ecol. Prog. Ser. 341, 303–307. doi: 10.3354/meps341303
Cook P. A., Warnock B., Gillies C. L., Hams A. (2021). Historical Abundance and Distribution of the Native Flat Oyster (Ostrea Angasi) in Estuaries of the Great Southern Region of Western Australia Help to Prioritise Potential Sites for Contemporary Oyster Reef Restoration. Mar. Freshw. Res. 73, 48–56. doi: 10.1071/MF21058
Cranfield H. J. (1979). The Biology of the Oyster, Ostrea Lutaria, and the Oyster Fishery of Foveaux Strait. Rapports Proces-Verbaux Des. Reunions (Denmark) 175, 44–49.
Crawford C. (2016). National Review of Ostrea Angasi Aquaculture: Historical Culture, Current Methods and Future Priorities (Hobart, Tasmania: University of Tasmania Institute for Marine and Antarctic Studies).
Crow B., Carney J. (2013). Commercializing Nature: Mangrove Conservation and Female Oyster Collectors in The Gambia. Antipode 45, 275–293. doi: 10.1111/j.1467-8330.2012.01015.x
Cui Z., Hu L., Li C., Zhang Z., Guo X., Wang H. (2021). Identification of Saccostrea Mordax and a New Species Saccostrea Mordoides Sp. Nov. (Bivalvia: Ostreidae) From China. J. Shellfish Res. 40, 63–75. doi: 10.2983/035.040.0107
De’ath G., Fabricius K. E., Sweatman H., Puotinen M. (2012). The 27–Year Decline of Coral Cover on the Great Barrier Reef and its Causes. Proc. Natl. Acad. Sci. 109, 17995–17999. doi: 10.1073/pnas.1208909109
De Santiago K., Palmer T. A., Dumesnil M., Pollack J. B. (2019). Rapid Development of a Restored Oyster Reef Facilitates Habitat Provision for Estuarine Fauna. Restor. Ecol. 27, 870–880. doi: 10.1111/rec.12921
Do Amaral V. S., Simone L. R. L. (2014). Revision of Genus Crassostrea (Bivalvia: Ostreidae) of Brazil. J. Mar. Biol. Assoc. United Kingdom 94, 811–836. doi: 10.1017/s0025315414000058
Do Amaral V. S., Simone L. R. L. (2016). Comparative Anatomy of Five Species of Saccostrea Dollfus and Dautzenberg 1920 (Bivalvia: Ostreidae) From the Pacific Ocean. Nautilus 130, 53–71.
Doldan M. D. S., De Rafélis M., Kroeck M. A., Pascual M. S., Morsan E. M. (2018). Age Estimation of the Oyster Ostrea Puelchana Determined From the Hinge Internal Growth Pattern. Mar. Biol. 165, 1–13. doi: 10.1007/s00227-018-3375-2
Dorenbosch M., Grol M., Christianen M., Nagelkerken I., van der Velde G. (2005). Indo-Pacific Seagrass Beds and Mangroves Contribute to Fish Density and Diversity on Adjacent Coral Reefs. Mar. Ecol. Prog. Ser. 302, 63–76. doi: 10.3354/meps302063
Dove M. C., O’connor W. A. (2009). Commercial Assessment of Growth and Mortality of Fifth-Generation Sydney Rock Oysters Saccostrea Glomerata (Gould 1850) Selectively Bred for Faster Growth. Aquacult. Res. 40, 1439–1450. doi: 10.1111/j.1365-2109.2009.02243.x
Dye A., Schleyer M., Lambert G., Lasiak T. (1994). “Intertidal and Subtidal Filter-Feeders in Southern Africa,” in Rocky Shores: Exploitation in Chile and South Africa (Springer, Berlin, Heidelberg), 57–74.
Eymann C., Götze S., Bock C., Guderley H., Knoll A. H., Lannig G., et al. (2020). Thermal Performance of the European Flat Oyster, Ostrea Edulis (Linnaeus 1758)—Explaining Ecological Findings Under Climate Change. Mar. Biol. 167, 1–15. doi: 10.1007/s00227-019-3620-3
Fao (2020). The State of World Fisheries and Aquaculture 2020: Sustainability in Action (Rome: Food and Agriculture Organization of the United Nations).
Filbee-Dexter K., Wernberg T. (2018). Rise of Turfs: A New Battlefront for Globally Declining Kelp Forests. Bioscience 68, 64–76. doi: 10.1093/biosci/bix147
Fitzsimons J., Branigan S., Brumbaugh R. D., Mcdonald T., Zu Ermgassen P. S. E. (2019). Restoration Guidelines for Shellfish Reefs (Arlington VA, USA: The Nature Conservancy).
Fitzsimons J. A., Branigan S., Gillies C. L., Brumbaugh R. D., Cheng J., Deangelis B. M., et al. (2020). Restoring Shellfish Reefs: Global Guidelines for Practitioners and Scientists. Conserv. Sci. Pract. 2, e198. doi: 10.1111/csp2.198
Gagnon K., Rinde E., Bengil E. G. T., Carugati L., Christianen M. J. A., Danovaro R., et al. (2020). Facilitating Foundation Species: The Potential for Plant–Bivalve Interactions to Improve Habitat Restoration Success. J. Appl. Ecol. 57, 1161–1179. doi: 10.1111/1365-2664.13605
Gedan K. B., Kellogg L., Breitburg D. L. (2014). Accounting for Multiple Foundation Species in Oyster Reef Restoration Benefits. Restor. Ecol. 22, 517–524. doi: 10.1111/rec.12107
Gilby B. L., Olds A. D., Connolly R. M., Henderson C. J., Schlacher T. A. (2018a). Spatial Restoration Ecology: Placing Restoration in a Landscape Context. BioScience 68, 1007–1019. doi: 10.1093/biosci/biy126
Gilby B. L., Olds A. D., Duncan C. K., Ortodossi N. L., Henderson C. J., Schlacher T. A. (2019). Identifying Restoration Hotspots That Deliver Multiple Ecological Benefits. Restor. Ecol. 28, 222–232. doi: 10.1111/rec.13046
Gilby B. L., Olds A. D., Peterson C. H., Connolly R. M., Voss C. M., Bishop M. J., et al. (2018b). Maximizing the Benefits of Oyster Reef Restoration for Finfish and Their Fisheries. Fish Fish. 19, 931–947. doi: 10.1111/faf.12301
Gillespie G. E. (2009). Status of the Olympia Oyster, Ostrea Lurida Carpenter 1864, in British Columbia, Canada. J. Shellfish Res. 28, 59–68. doi: 10.2983/035.028.0112
Gillies C. L., Castine S. A., Alleway H. K., Crawford C., Fitzsimons J. A., Hancock B., et al. (2020). Conservation Status of the Oyster Reef Ecosystem of Southern and Eastern Australia. Global Ecol. Conserv. 22, e00988. doi: 10.1016/j.gecco.2020.e00988
Gillies C. L., Mcleod I. M., Alleway H. K., Cook P., Crawford C., Creighton C., et al. (2018). Australian Shellfish Ecosystems: Past Distribution, Current Status and Future Direction. PloS One 13, e0190914. doi: 10.1371/journal.pone.0190914
Goldberg L., Lagomasino D., Thomas N., Fatoyinbo T. (2020). Global Declines in Human-Driven Mangrove Loss. Global Change Biol. 26, 5844–5855. doi: 10.1111/gcb.15275
Grabowski J. H., Brumbaugh R. D., Conrad R. F., Keeler A. G., Opaluch J. J., Peterson C. H., et al. (2012). Economic Valuation of Ecosystem Services Provided by Oyster Reefs. BioScience 62, 900–909. doi: 10.1525/bio.2012.62.10.10
Grabowski J. H., Hughes A. R., Kimbro D. L., Dolan M. A. (2005). How Habitat Setting Influences Restored Oyster Reef Communities. Ecology 86, 1926–1935. doi: 10.1890/04-0690
Grabowski J. H., Peterson C. H. (2007). Restoring Oyster Reefs to Recover Ecosystem Services. Ed. Cuddington K, Byers JE, Wilson WG, Hastings A (Elsevier: Ecosystem Engineers: Plants to Protists).
Gray J. S. (1997). Marine Biodiversity: Patterns, Threats and Conservation Needs. Biodiversity Conserv. 6, 153–175. doi: 10.1023/A:1018335901847
Gray M. W., Langdon C. J. (2018). Ecophysiology of the Olympia Oyster, Ostrea Lurida, and Pacific Oyster, Crassostrea Gigas. Estuaries Coasts 41, 521–535. doi: 10.1007/s12237-017-0273-7
Guo X., Li C., Wang H., Xu Z. (2018). Diversity and Evolution of Living Oysters. J. Shellfish Res. 37, 755–771. doi: 10.2983/035.037.0407
Hancock B., Zu Ermgassen P. (2019). Enhanced Production of Finfish and Large Crustaceans by Bivalve Reefs. Ed. A.C. Smaal, J.G. Ferreira, J. Grant, J.K.Petersen, and Ø. Strand. (Cham: Goods and Services of Marine Bivalves, Springer).
Haque C. E., Deb A. K., Medeiros D. (2009). Integrating Conservation With Livelihood Improvement for Sustainable Development: The Experiment of an Oyster Producers' Cooperative in Southeast Brazil. Soc. Natural Resour. 22, 554–570. doi: 10.1080/08941920802271761
Hashim R., Kamali B., Tamin N. M., Zakaria R. (2010). An Integrated Approach to Coastal Rehabilitation: Mangrove Restoration in Sungai Haji Dorani, Malaysia. Estuarine Coast. Shelf Sci. 86, 118–206. doi: 10.1016/j.ecss.2009.10.021
Hernández A. B., Brumbaugh R. D., Frederick P., Grizzle R., Luckenbach M. W., Peterson C. H., et al. (2018). Restoring the Eastern Oyster: How Much Progress has Been Made in 53 Years? Front. Ecol. Environ. 16, 463–471. doi: 10.1002/fee.1935
Howie A. H., Bishop M. J. (2021). Contemporary Oyster Reef Restoration: Responding to a Changing World. Front. Ecol. Evol. 518. doi: 10.3389/fevo.2021.689915
Karp M. A., Seitz R. D., Fabrizio M. C. (2018). Faunal Communities on Restored Oyster Reefs: Effects of Habitat Complexity and Environmental Conditions. Mar. Ecol. Prog. Ser. 590, 35–51. doi: 10.3354/meps12470
Kellogg M. L., Cornwell J. C., Owens M. S., Paynter K. T. (2013). Denitrification and Nutrient Assimilation on a Restored Oyster Reef. Mar. Ecol. Prog. Ser. 480, 1–19. doi: 10.3354/meps10331
Kritsanapuntu S., Chaitanawisuti N. (2018). Effects of Extensive Bottom Cultivation of Tropical Oyster Crassostrea Belcheri on Benthic Invertebrate Community Structure in Ban Don Bay, Suratthani Province, Southern Thailand. Aquacult. Int. 26, 433–449. doi: 10.1007/s10499-017-0227-x
Lam K., Morton B. (2003). Mitochondrial DNA and Morphological Identification of a New Species of Crassostrea (Bivalvia: Ostreidae) Cultured for Centuries in the Pearl River Delta, Hong Kong, China. Aquaculture 228, 1–13. doi: 10.1016/s0044-8486(03)00215-1
Lam K., Morton B. (2004). The Oysters of Hong Kong (Bivalvia: Ostreidae and Gryphaeidae). Raffles Bull. Zoology 52, 11–28.
Lam K., Morton B. (2006). Morphological and Mitochondrial-DNA Analysis of the Indo-West Pacific Rock Oysters (Ostreidae: Saccostrea Species). J. Molluscan Stud. 72, 235–245. doi: 10.1093/mollus/eyl002
La Peyre M. K., Aguilar Marshall D., Miller L. S., Humphries A. T. (2019). Oyster Reefs in Northern Gulf of Mexico Estuaries Harbor Diverse Fish and Decapod Crustacean Assemblages: A Meta-Synthesis. Front. Mar. Sci. 6. doi: 10.3389/fmars.2019.00666
Lau J. D., Scales I. R. (2016). Identity, Subjectivity and Natural Resource Use: How Ethnicity, Gender and Class Intersect to Influence Mangrove Oyster Harvesting in The Gambia. Geoforum 69, 136–146. doi: 10.1016/j.geoforum.2016.01.002
Lau S. C. Y., Thomas M., Hancock B., Russell B. D. (2020). Restoration Potential of Asian Oysters on Heavily Developed Coastlines. Restor. Ecol. 28, 1643–1653. doi: 10.1111/rec.13267
Legat J. F. A., Legat A., Fogaça F. D. S., Tureck C. R., Suhnel S., De Melo C. (2017). Growth and Survival of Bottom Oyster Crassostrea Gasar Cultured in the Northeast and South of Brazil. Embrapa Meio-Norte-Artigo em periódico indexado (ALICE) 43, 172–184. doi: 10.20950/1678-2305.2017v43n2p172
Linnaeus C. V. (1758). Systema Naturae Per Regna Tria Naturae. Secundum Classes, Ordines, Genera, Species, Cum Characteribus, Differentiis, Synonymis, Locis, vol. I. (Stockholm: Laurentii Salvii).
Lipcius R. N., Burke R. P. (2018). Successful Recruitment, Survival and Long-Term Persistence of Eastern Oyster and Hooked Mussel on a Subtidal, Artificial Restoration Reef System in Chesapeake Bay. PloS One 13, e0204329. doi: 10.1371/journal.pone.0204329
Liu T.-S. (1995). Becoming Marginal: A Fluid Community and Shamanism in the Pearl River Delta of South China (University of Pittsburgh, Ann Arbor, MI).
Lodeiros C., Valentich-Scott P., Chávez-Villalba J., Mazón-Suástegui J. M., Grijalva-Chon J. M. (2020). Tropical and Subtropical Ostreidae of the American Pacific: Taxonomy, Biology, Ecology, and Genetics. J. Shellfish Res. 39, 181–206. doi: 10.2983/035.039.0202
Mcafee D., Bishop M. J. (2019). The Mechanisms by Which Oysters Facilitate Invertebrates Vary Across Environmental Gradients. Oecologia 189, 1095–1106. doi: 10.1007/s00442-019-04359-3
Mcafee D., Bishop M. J., Yu T. N., Williams G. A. (2018). Structural Traits Dictate Abiotic Stress Amelioration by Intertidal Oysters. Funct. Ecol. 32, 2666–2677. doi: 10.1111/1365-2435.13210
Mcclenachan G., Witt M., Walters L. J. (2021). Replacement of Oyster Reefs by Mangroves: Unexpected Climate-Driven Ecosystem Shifts. Glob Chang Biol. 27, 1226–1238. doi: 10.1111/gcb.15494
Mcdougall C., Nenadic N., Healy J. (2020). Guide to Queensland’s Intertidal Oysters (Brisbane, QLD, Australia: Griffith University).
Mcleod I. M., Boström-Einarsson L., Creighton C., D'anastasi B., Diggles B., Dwyer P. G., et al. (2020). Habitat Value of Sydney Rock Oyster (Saccostrea Glomerata) Reefs on Soft Sediments. Mar. Freshw. Res. 71, 771–781. doi: 10.1071/mf18197
Mcleod I. M., Le Port A., Sheaves M. (2015). Protection and Repair of Australia’s Shellfish Reefs: Great Barrier Reef Region Report (James Cook University, Townsville: Centre for Tropical Water & Aquatic Ecosystem Research (TropWATER) Publication), 18.
Mcleod I. M., Zu Ermgassen P. S. E., Gillies C. L., Hancock B., Humphries A. (2019). Can Bivalve Habitat Restoration Improve Degraded Estuaries? Ed. Wolanski E., Day J. W., Elliott M., Ramesh R.. Coasts and Estuaries: the Future Elsevier.
Meesters H. W. G., Smith S. R., Becking L. E. (2015). A Review of Coral Reef Restoration Techniques, Institute for Marine Resources (IMARES), Bremerhaven.
Nagelkerken I., Kleijnen S., Klop T., Van Den Brand R., de la Moriniere E. C., van der Velde G. (2001). Dependence of Caribbean Reef Fishes on Mangroves and Seagrass Beds as Nursery Habitats: A Comparison of Fish Faunas Between Bays With and Without Mangroves/Seagrass Beds. Mar. Ecol. Prog. Ser. 214, 225–235. doi: 10.3354/meps214225
Nagi H. M., Shenai-Tirodkar P. S., Jagtap T. G. (2011). Dimensional Relationships in Crassostrea Madrasensis (Preston) and C. Gryphoides (Schlotheim) in Mangrove Ecosystem. Indian J. Geo-Marine Sci. 40, 559–566.
Naik M. G., Gowda G. (2013). Survey of Oyster Beds of Mulky Estuary, South-West Coast of India. J. Acad. Ind. Res. 1, 601–605.
Newkirk G., Haley L. (1982). Progress in Selection for Growth Rate in the European Oyster Ostrea Edulis. Mar. Ecol. Prog. series. Oldendorf 10, 77–79. doi: 10.3354/meps010077
Nowland S. J., O’connor W. A., Osborne M. W. J., Southgate P. C. (2020). Current Status and Potential of Tropical Rock Oyster Aquaculture. Rev. Fish. Sci. Aquacult. 28, 57–70. doi: 10.1080/23308249.2019.1670134
Ostroff S. (2020). “Can the Oyster Speak? Pearling Empires and the Marine Environments of South India and Sri Lanka, C. 1600–1900,” in In Animal Trade Histories in the Indian Ocean World. Eds. Chaiklin M., Gooding P., Campbell G. (Cham: Springer International Publishing), 65–98.
Palandro D. A., Andréfouët S., Hu C., Hallock P., Müller-Karger F. E., Dustan P., et al. (2008). Quantification of Two Decades of Shallow-Water Coral Reef Habitat Decline in the Florida Keys National Marine Sanctuary Using Landsat Data, (1984–2002). Remote Sens. Environ. 112, 3388–3399. doi: 10.1016/j.rse.2008.02.015
Paling E. I., Fonseca M., Van Katwijk M. M., Van Keulen M. (2009). Seagrass Restoration. Coast. wetlands: integrated Ecosyst. approach, 687–713.
Patra P., Mohandass C., Chakraborty P., Seleyi S. C. (2021). Biochemical Responses of Oysters in Evaluating Environmental Quality of Tropical Indian Estuarine Systems. Chemosphere 278, 130338. doi: 10.1016/j.chemosphere.2021.130338
Patterson J., Jeyasanta K. I., Sathish N., Booth A. M., Edward J. P. (2019). Profiling Microplastics in the Indian Edible Oyster, Magallana Bilineata Collected From the Tuticorin Coast, Gulf of Mannar, Southeastern India. Sci. Total Environ. 691, 727–735. doi: 10.1016/j.scitotenv.2019.07.063
Pierce J., O’connor W. (2014). “Impact of Oyster Farming on Rural Community Sustainability in North Vietnam,” in Linking Local and Global Sustainability. Eds. Sandhu S., Mckenzie S., Harris H. (Dordrecht: Springer Netherlands), 207–224.
Pogoda B., Brown J., Hancock B., Preston J., Pouvreau S., Kamermans P., et al. (2019). The Native Oyster Restoration Alliance (NORA) and the Berlin Oyster Recommendation: Bringing Back a Key Ecosystem Engineer by Developing and Supporting Best Practice in Europe. Aquat. Living Resour. 32, 13. doi: 10.1051/alr/2019012
Preston J., Gamble C., Debney A., Helmer L. D., Hancock B., Zu Ermgassen P. (2020). European Native Oyster Habitat Restoration Handbook (London: Zoological Society of London).
Pritchard C., Shanks A., Rimler R., Oates M., Rumrill S. (2015). The Olympia Oyster Ostrea Lurida: Recent Advances in Natural History, Ecology, and Restoration. J. Shellfish Res. 34, 259–271. doi: 10.2983/035.034.0207
Quan W.-M., Zhu J.-X., Ni Y., Shi L.-Y., Chen Y.-Q. (2009). Faunal Utilization of Constructed Intertidal Oyster (Crassostrea Rivularis) Reef in the Yangtze River Estuary, China. Ecol. Eng. 35, 1466–1475. doi: 10.1016/j.ecoleng.2009.06.001
Reeves S. E., Renzi J. J., Fobert E. K., Silliman B. R., Hancock B., Gillies C. L. (2020). Facilitating Better Outcomes: How Positive Species Interactions can Improve Oyster Reef Restoration. Front. Mar. Sci. 7. doi: 10.3389/fmars.2020.00656
Rios Gonzalez K., Lopez Uriarte E., Chong Carrillo O., Vega Villasante F., Chavez Villalba J. (2018). Rock Oyster Striostrea Prismatica (Gray 1825): Biology, Exploitation and Conservation. Latin Am. J. Aquat. Res. 46, 626–644. doi: 10.3856/vol46-issue4-fulltext-1
Roberts C. M., Mcclean C. J., Veron J. E., Hawkins J. P., Allen G. R., Mcallister D. E., et al. (2002). Marine Biodiversity Hotspots and Conservation Priorities for Tropical Reefs. Science 295, 1280–1284. doi: 10.1126/science.1067728
Rodney W. S., Paynter K. T. (2006). Comparisons of Macrofaunal Assemblages on Restored and Non-Restored Oyster Reefs in Mesohaline Regions of Chesapeake Bay in Maryland. J. Exp. Mar. Biol. Ecol. 335, 39–51. doi: 10.1016/j.jembe.2006.02.017
Rodolfo R. S., Lapus M. R. (2021). “Business Continuity as a Means to Strengthen Disaster Risk Reduction in a Coastal Community of Oyster Farmers,” in Strengthening Disaster Risk Governance to Manage Disaster Risk. (Elsevier), 133–141.
Romero A. (2003). Death and Taxes: The Case of the Depletion of Pearl Oyster Beds in Sixteenth-Century Venezuela. Conserv. Biol. 17, 1013–1023. doi: 10.1046/j.1523-1739.2003.01406.x
Sala E., Knowlton N. (2006). Global Marine Biodiversity Trends. Annu. Rev. Environ. Resour. 31, 93–122. doi: 10.1146/annurev.energy.31.020105.100235
Salvi D., Mariottini P. (2016). Molecular Taxonomy in 2D: A Novel ITS2 rRNA Sequence-Structure Approach Guides the Description of the Oysters' Subfamily Saccostreinae and the Genus Magallana(Bivalvia: Ostreidae). Zoological J. Linn. Soc. 179, 263–276. doi: 10.1111/zoj.12455
Samakraman S., Williams G. A., Monthon G. (2010). Spatial and Temporal Variability of Intertidal Rocky Shore Bivalves and Gastropods in Sichang Island, East Coast of Thailand. Pub. Seto Mar. Biol. Lab. Special Publ. Ser. 10, 35–46.
Sekino M., Yamashita H. (2016). Mitochondrial and Nuclear DNA Analyses of Saccostrea Oysters in Japan Highlight the Confused Taxonomy of the Genus. J. Molluscan Stud. 82, 492–506. doi: 10.1093/mollus/eyw022
Sharma S., Goff J., Moody R. M., Byron D., Heck K. L., Powers S. P., et al. (2016). Do Restored Oyster Reefs Benefit Seagrasses? An Experimental Study in the Northern Gulf of Mexico. Restor. Ecol. 24, 306–313. doi: 10.1111/rec.12329
Silliman B. R., Grosholz E. D., Bertness M. D. (2009). Human Impacts on Salt Marshes: A Global Perspective (Berkley, CA: Univ of California Press).
Smyth D., Al-Maslamani I., Chatting M., Giraldes B. (2016). Benthic Surveys of the Historic Pearl Oyster Beds of Qatar Reveal a Dramatic Ecological Change. Mar. Pollut. Bull. 113, 147–155. doi: 10.1016/j.marpolbul.2016.08.085
Su J., Friess D. A., Gasparatos A. (2021). A Meta-Analysis of the Ecological and Economic Outcomes of Mangrove Restoration. Nat. Commun. 12, 1–13. doi: 10.1038/s41467-021-25349-1
Szuster B. W., Chalermwat K., Flaherty M., Intacharoen P. (2008). Peri-Urban Oyster Farming in the Upper Gulf of Thailand. Aquacult. Economics Manage. 12, 268–288. doi: 10.1080/13657300802494438
Tamburri M. N., Luckenbach M. W., Breitburg D. L., Bonniwell S. M. (2008). Settlement of Crassostrea Ariakensis Larvae: Effects of Substrate, Biofilms, Sediment and Adult Chemical Cues. J. Shellfish Res. 27, 601–608. doi: 10.2983/0730-8000(2008)27[601:SOCALE]2.0.CO;2
Tangelder M., Ysebaert T., Chowdhury S., Reinhard A. J., Doorn F., Hossain M. S., et al. (2015). Eco-Engineered Coastal Defense Integrated With Sustainable Aquatic Food Production in Bangladesh (ECOBAS) (Project Report Number C048/15 IMARES, Wageningen University, The Netherlands).
Tolley S. G., Volety A. K. (2005). The Role of Oysters in Habitat Use of Oyster Reefs by Resident Fishes and Decapod Crustaceans. J. Shellfish Res. 24, 1007–1012. doi: 10.2983/0730-8000(2005)24[1007:TROOIH]2.0.CO;2
Toone T. A., Hunter R., Benjamin E. D., Handley S., Jeffs A., Hillman J. R. (2021). Conserving Shellfish Reefs—a Systematic Review Reveals the Need to Broaden Research Efforts. Restor. Ecol. 29 (4), e13375. doi: 10.1111/rec.13375
Valdez S. R., Zhang Y. S., van der Heide T., Vanderklift M. A., Tarquinio F., Silliman B. R. (2020). Positive Ecological Interactions and the Success of Seagrass Restoration. Restor. Front. Mar. Sci. 7. doi: 10.3389/fmars.2020.00091
Wagner E., Dumbauld B. R., Hacker S. D., Trimble A. C., Wisehart L. M., Ruesink J. L. (2012). Density-Dependent Effects of an Introduced Oyster, Crassostrea Gigas, on a Native Intertidal Seagrass, Zostera Marina. Mar. Ecol. Prog. Ser. 468, 149–160. doi: 10.3354/meps09952
Wang T., Fan R., Cheng Q., Sun Z., Fan X., Li N., et al. (2020). Intertidal Zonation of the Suminoe Oyster Crassostrea Ariakensis and the Kumamoto Oyster Crassostrea Sikamea on the Coast of the Northern East China Sea. J. Shellfish Res. 39, 31–41. doi: 10.2983/035.039.0104
Watson J. L. (2022). Guarding the Shoreline: Oyster Farming, Salt Production, and Fishing Along the South China Coast, (1667–1978). J. Chin. History, 6, 1–29. doi: 10.1017/jch.2021.8
Weng X., Zhang B., Zheng X. (2021). Detection and Analysis of Sources of Lime Mineral in Ancient Buildings. New J. Chem. 45 (36), 16490–16499. doi: 10.1039/D1NJ02587B
Westphal G. G. C., Ostrensky A. (2016). Use of Side-Scan Sonar for Estimations of Crassostrea Brasiliana (Lamarck 1819) Stocks in Subtidal Banks on the South Coast of Brazil. Braz. J. Oceanography 64, 49–56. doi: 10.1590/s1679-87592016104506401
Willan R. C., Nenadic N., Ramage A., Mcdougall C. (2021). Detection and Identification of the Large, Exotic, Crassostreine Oyster Magallana Bilineata (Röding 1798) in Northern Queensland, Australia. Molluscan Res. 41 (1), 1–11. doi: 10.1080/13235818.2020.1865515
Wu X., Xiao S., Yu Z. (2013). Mitochondrial DNA and Morphological Identification of Crassostrea Zhanjiangensis Sp. Nov. (Bivalvia: Ostreidae): A New Species in Zhanjiang, China. Aquat. Living Resour. 26, 273–280. doi: 10.1051/alr/2013065
Ziegler S. L., Grabowski J. H., Baillie C. J., Fodrie F. J. (2018). Effects of Landscape Setting on Oyster Reef Structure and Function Largely Persist More Than a Decade Post-Restoration. Restor. Ecol. 26, 933–942. doi: 10.1111/rec.12651
Zu Ermgassen P. S. E., Hancock B., Deangelis B., Greene J., Elisabeth S., Spalding M., et al. (2016). Setting Objectives for Oyster Habitat Restoration Using Ecosystem Services: A Manager’s Guide (Arlington VA: The Nature Conservancy), 76pp.
Keywords: oyster reef restoration, oyster diversity, ecosystem services, tropical restoration, reef-building oysters, multi-species restoration, tropical oyster reefs, tropical oysters
Citation: Richardson MA, Zhang Y, Connolly RM, Gillies CL and McDougall C (2022) Some Like it Hot: The Ecology, Ecosystem Benefits and Restoration Potential of Oyster Reefs in Tropical Waters. Front. Mar. Sci. 9:873768. doi: 10.3389/fmars.2022.873768
Received: 11 February 2022; Accepted: 24 May 2022;
Published: 23 June 2022.
Edited by:
Susana Carvalho, Center for Marine and Environmental Sciences (MARE), PortugalReviewed by:
Romuald Lipcius, William & Mary’s Virginia Institute of Marine Science, United StatesJose M. Fariñas-Franco, Galway-Mayo Institute of Technology, Ireland
Bayden D. Russell, University of Hong Kong, China
Copyright © 2022 Richardson, Zhang, Connolly, Gillies and McDougall. This is an open-access article distributed under the terms of the Creative Commons Attribution License (CC BY). The use, distribution or reproduction in other forums is permitted, provided the original author(s) and the copyright owner(s) are credited and that the original publication in this journal is cited, in accordance with accepted academic practice. No use, distribution or reproduction is permitted which does not comply with these terms.
*Correspondence: Marina A. Richardson, marina.richardson@griffithuni.edu.au; Carmel McDougall, c.mcdougall@griffith.edu.au