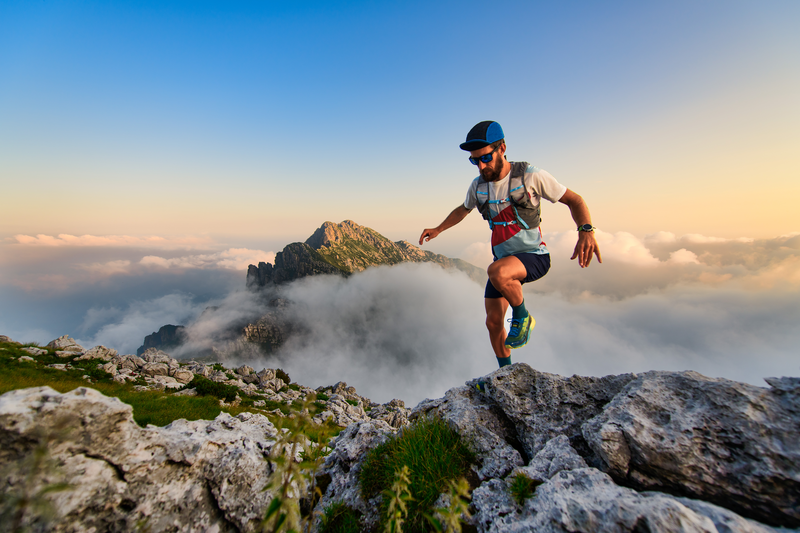
95% of researchers rate our articles as excellent or good
Learn more about the work of our research integrity team to safeguard the quality of each article we publish.
Find out more
MINI REVIEW article
Front. Immunol. , 02 April 2025
Sec. Cancer Immunity and Immunotherapy
Volume 16 - 2025 | https://doi.org/10.3389/fimmu.2025.1581098
This article is part of the Research Topic Harnessing Molecular Insights for Enhanced Drug Sensitivity and Immunotherapy in Cancer View all 27 articles
Hepatocellular carcinoma (HCC) remains a leading cause of cancer-related mortality globally. The tumor microenvironment (TME) plays a pivotal role in HCC progression, characterized by dynamic interactions between stromal components, immune cells, and tumor cells. Key immune players, including tumor-associated macrophages (TAMs), tumor-infiltrating lymphocytes (TILs), cytotoxic T lymphocytes (CTLs), regulatory T cells (Tregs), MDSCs, dendritic cells (DCs), and natural killer (NK) cells, contribute to immune evasion and tumor progression. Recent advances in immunotherapy, such as immune checkpoint inhibitors (ICIs), cancer vaccines, adoptive cell therapy (ACT), and combination therapies, have shown promise in enhancing anti-tumor responses. Dual ICI combinations, ICIs with molecular targeted drugs, and integration with local treatments or radiotherapy have demonstrated improved outcomes in HCC patients. This review highlights the evolving understanding of the immune microenvironment and the therapeutic potential of immunotherapeutic strategies in HCC management.
HCC constitutes 80-90% of Liver cancer cases, alongside intrahepatic cholangiocarcinoma and mixed-type carcinoma (1, 2). The tumor microenvironment (TME), a key driver of cancer progression, dynamically evolves from premalignant stages to advanced tumor development (3, 4). This evolution is characterized by the transition from a premalignant microenvironment to a tumor-promoting microenvironment, both of which adapt as the tumor progresses (5).
The immune microenvironment, comprising TILs, CTLs, Tregs, TAMs, and MDSCs, plays a pivotal role in the development and progression of HCC (6). HCC is frequently asymptomatic in its early stages, leading to the majority of cases being diagnosed at advanced phases, which significantly limits surgical intervention. Traditional therapeutic approaches often exhibit limited efficacy and are associated with high rates of recurrence. In contrast, immunotherapy has emerged as a transformative strategy, leveraging advances in immunobiology to enhance anti-tumor immune responses. This review summarizes the roles of immune cells within the TME and recent advancements in immunotherapy for HCC.
TAMs exhibit M1 and M2 phenotypes, with M2 promoting immune evasion and tumor progression. Glycolytic pathway inhibition suppresses M2 polarization (7). Receptor-interacting serine/threonine-protein kinase 3 (RIPK3)-deficient TAMs enhance M2 polarization via fatty acid oxidation, whereas decitabine modulates metabolism to boost anti-tumor immunity (8). TAMs drive HCC invasion, metastasis, and recurrence, correlating with poor prognosis (9). Hypoxia induces IL-1β release via TLR4/TIR-TRIF/NF-κB, promoting epithelial-mesenchymal transition (EMT) (10, 11). TAMs produce inflammatory cytokines, including TNF-α, IL-β, IL-6, and IL-23. They also expand Th17 cells and suppress immunity by upregulating PD-1, CTLA-4, and GITR (12–15). TGF-β upregulates TIM-3 on TAMs, enhancing tumor growth via NF-κB/IL-6 (16). Mitochondrial fission in HCC activates TLR9/NF-κB, increasing CD163+ TAM infiltration and CCL2, linked to poor prognosis (17). Furthermore, TAMs interact with cancer-associated fibroblasts (CAFs) via cytokines such as TGF-β and stromal cell-derived factor-1 (SDF-1), promoting M2 polarization, fibrosis, and immunosuppression, enhancing tumorigenesis and immune evasion (18).
TILs, comprising T, B, and NK cells, are crucial in antitumor immunity (19–22). FOXP3+ regulatory T cells (Tregs) act as tumor suppressors, while immunohistochemical studies demonstrate that immune cells regulate both antitumor responses and tumor-promoting conditions within the TME (23, 24). In HCC, the role of TILs in immune surveillance varies, with the balance between regulatory and cytotoxic T cells significantly influencing tumor progression (25). TILs are emerging as potential prognostic biomarkers in HCC, although findings on the impact of FOXP3+ and CD8+ T cells on prognosis remain conflicting (26–29).
CTLs, guided by CD4+ T helper (Th) cells, target abnormal cells and initiate cytotoxic responses to eliminate tumors. Activated DCs support CTL function by providing CXCL16 and IL-15, which promote CTL accumulation and survival (30, 31). Despite immune suppression via hypoxia, metabolic competition, insufficient CD4+ T cells, and high expression of regulatory molecules (e.g., VEGF, CXCL17, IL-10, IDO), the presence of CD8+ CTLs in HCC correlates with improved survival (32–37). However, Fas/FasL expression in CD8+ T cells and endothelial cells, induced by VEGF-A and PGE2, accelerates CD8+ turnover, diminishing antitumor response (32, 38, 39). Additionally, CTLs are further suppressed by IL-2 and indoleamine 2,3-dioxygenase (IDO) secreted by CD14+ DCs (40).
Tregs, marked by CD25 and FOXP3, maintain immune tolerance but promote tumor progression in the TME. They infiltrate cancers like melanoma, suppressing anti-tumor responses via CCL6/CCL20 and TCR/IL-10/TGF-β pathways (41, 42). In HCC, Tregs are elevated in tumor tissues and blood, with increased CD4+CD25+ cells (43, 44). LncRNAs and pro-inflammatory signals enhance Treg differentiation; lnc-Epidermal growth factor receptor (EGFR) overexpression inhibits ubiquitination via AP-1/NFAT1, aiding immune suppression (45). Tregs are key immunotherapy targets, with sorafenib reducing Tregs by blocking TGF-β (46). Various microRNAs, including miR-150-5p and miR-142-3p, are implicated in immunosuppression mediated by Treg cell-derived extracellular vesicles, which foster a tolerogenic state in dendritic cells (47).
MDSCs exhibit immunosuppressive functions by inhibiting T cell activation, inducing T cell anergy, suppressing NK cell cytotoxicity, and polarizing macrophages towards a pro-tumor phenotype (48). MDSCs are broadly categorized into two subsets: monocytic (M-MDSCs) and polymorphonuclear (PMN-MDSCs). Under physiological conditions, MDSCs differentiate into macrophages, granulocytes, and dendritic cells; however, during inflammation or tumorigenesis, they undergo expansion and transform into immune-suppressive TAMs (49). Within the TME, MDSCs exert their immunosuppressive effects by depleting cysteine, upregulating inducible nitric oxide synthase (iNOS) and arginase-1 (ARG-1), impairing T cell function, promoting regulatory T cell (Treg) expansion, and generating reactive oxygen species (ROS) to inhibit NK cell activity (50). Notably, KRAS mutations in M-MDSCs suppress interferon regulatory factor 2 (IRF2), leading to the release of CXCL3, which recruits additional MDSCs and inhibits cytotoxic T cells (51). Clinical studies have demonstrated that elevated M-MDSC populations in HCC patients are closely associated with the induction of CD4+CD25+Foxp3+ Tregs and the suppression of NK cell activity through NKp30-dependent cell contact (52, 53). Furthermore, CCRK expression in tumors promotes MDSC accumulation, with high CCRK/IL-6/CD11b/CD33 levels linked to poor prognosis (54). Preclinical HCC models show CCRK signaling recruits MDSCs to establish an immune-suppressive TME, reversible by targeting CCRK or IL-6 (55). Besides, Treg-derived exosomes amplify the immunosuppressive capacity of MDSCs (56).
DCs is recognized as the most potent antigen-presenting cells, predominantly exist in an immature state in vivo, where they exhibit exceptional efficiency in antigen uptake. They activate T lymphocytes for anti-tumor responses, stimulate B cell maturation, and activate Th and NK cells. DCs are classified into cDC1 (myeloid-derived) and cDC2 (lymphoid-derived) subtypes. They present antigens via MHC-I/II pathways and induce co-stimulatory signals (e.g., B7/CD28) to activate CTLs for anti-tumor immunity (57). Antigen delivery by DCs may involve exosome vesicles (58). In HCC, LAMP3+ DCs, expressing CD80/83 and CCR7, migrate to liver lymph nodes, interacting with Tregs via CD86-CD28 and exhausted T cells via CD86-CTLA4 (5). HCC patients show reduced circulating pDCs and cDCs, with decreased co-stimulatory molecule expression, negatively correlating with IL-10 levels (59, 60). CD303+ pDCs in HCC tumors co-localize with type 1 Tregs and correlate with poor prognosis (61, 62).
NK cells, innate lymphocytes, mediate tumor cell killing via perforin/granzyme exocytosis, FASL/RAILR binding, and IFN-γ/TNFα secretion. Classified into CD56dim (mature, cytotoxic, blood-dominant) and CD56bright (immature, immunomodulatory, lymphoid organ-resident) subsets (63). In the TME, NK infiltration is limited, with suppressed function due to altered chemokine profiles, fructose-1,6-bisphosphatase-induced glycolysis suppression, and adenosine-mediated maturation inhibition (64, 65). High NK and CD8+ T cell presence correlates with favorable prognosis and tumor apoptosis in early HCC (66). However, in stage III HCC (67), CD56dim NK frequency declines, while immunoregulatory NKs expand, with tumor-infiltrating CD56dim NKs expressing PD-1/NKG2A, linked to poor prognosis (68, 69). Sorafenib enhances NK activity via macrophage-derived cytokines (IL-12, IL-18, IL-1β), suggesting NK activation contributes to its anti-tumor efficacy (70, 71).
TANs are guided into tumors by chemokines (CXCL1/2/5/6/8, CCL3/5) binding to CXCR1/2 receptors (72). TANs exhibit dual roles: promoting tumor growth via angiogenesis, ECM remodeling, and immunosuppression, or mediating anti-tumor responses through ROS, RNS, and direct cytotoxicity. Polarization to N1 (anti-tumor, IFNβ/IL-1β-driven) or N2 (pro-tumor, TGF-β/IL-6/7/8-driven) phenotypes influences tumor dynamics (73). The neutrophil-lymphocyte ratio is a prognostic marker in liver cancer (74). Neutrophil-tumor cell clusters enhance metastasis via upregulated cell cycle and DNA replication genes (75). N2 TANs induce stem cell-like phenotypes in HCC through BMP2/TGF-β2/miR-301b-3p signaling and CXCL5 feedback loops (76, 77). They recruit macrophages/Tregs via CCL2/CCL17, inducing sorafenib resistance (78), and form NETs, promoting HCC progression (79) (Figure 1).
The PD-1/PD-L1 axis, expressed on immune and tumor cells respectively, suppresses immune activation and cytotoxic function, particularly in liver malignancies (80). Clinical trials demonstrate PD-1 inhibitors’ efficacy: nivolumab (CheckMate-040) showed 23% ORR and 28.6-month median OS in sorafenib-naïve HCC (81), while pembrolizumab (KEYNOTE-240) improved survival versus placebo (82). Camrelizumab achieved 14.7% ORR and 14.2-month median OS in Chinese HCC patients (83). with comparable outcomes among PD-1 inhibitors (81, 83–85). PD-L1 inhibitors show promise, with atezolizumab plus bevacizumab (IMbrave 150) demonstrating superior 12-month survival versus sorafenib in unresectable HCC (86).
CTLA-4, a homolog of CD28 expressed on activated CD4+ and CD8+ T lymphocytes, competes with CD28 for binding to B7 ligands, thus inhibiting T lymphocyte activation (87). CTLA-4 inhibitors relieve this inhibitory signal, activating specific effector T cells to induce or enhance anti-tumor immune responses. Ipilimumab is currently the only approved CTLA-4 inhibitor. A study of 21 HCC patients treated with ipilimumab showed a partial response rate (PR) of 17.6% and a disease control rate (DCR) of 76.4%, demonstrating its significant therapeutic effect (88).
GITR agonists enhance anti-tumor immune responses through co-stimulation, making them a novel target in cancer immunotherapy. Preclinical studies have shown that GITR agonists promote the activation of CD8+ and CD4+ effector T cells while inhibiting the activity of tumor-infiltrating T cells. A phase I clinical trial of the GITR agonist monoclonal antibody TRx518 demonstrated promising safety and immune effects in patients with advanced cancer (NCT01239134) (89).
Cancer vaccines are therapeutic vaccines targeting tumor-associated antigens, stimulating the body’s immune response to target and kill tumor cells. The main types of cancer vaccines include peptide vaccines, DC vaccines, and oncolytic virus vaccines.
Tumor peptide vaccines, utilizing surface antigens or intracellular peptides, activate T cell-mediated anti-tumor immunity (90–92). Glypican-3, overexpressed in HCC, demonstrated clinical efficacy, with Sawada et al. (93) showing reduced recurrence and improved survival when combined with surgery versus surgery alone. Multi-drug resistance protein 3 vaccines also enhanced survival in advanced HCC (94, 95). Glypican-3 trials induced CTL responses in 30/33 patients, with partial/stable disease and improved OSR objective survival rates (OSR) (96, 97). Phase II studies confirmed reduced post-surgical recurrence (93). Alpha-fetoprotein (AFP) vaccines activated HCC immunity (98), with hAFP-DCs enhancing anti-tumor effects in mice (99). AFP-derived peptides (AFP357,403) showed safety and efficacy, including complete remission (100), while AFP158-specific TCR genes enabled T cells to target HCC cells (101).
Dendritic cells (DCs), as highly potent antigen-presenting cells, can be effectively sensitized using tumor antigen extracts to elicit robust and specific immune responses. Accumulating evidence demonstrates that DC-based vaccines significantly enhance the proliferation and activation of CD8+ T lymphocytes while elevating serum interferon-gamma (IFN-γ) levels in patients with hepatocellular carcinoma (HCC), thereby contributing to improved overall survival (OS) (102). Meta-analyses confirm DC immunotherapy as an effective adjuvant for HCC, boosting antitumor immunity, survival rates, and reducing recurrence, with good safety (103). Notably, MIZUKOSHI et al. (104) provided compelling evidence that the combination of transcatheter arterial embolization (TAE) with DC infusion significantly amplifies tumor-specific T lymphocyte responses and enhances antitumor immunity compared to TAE monotherapy, highlighting the synergistic potential of this combined therapeutic approach. Post-surgery, RFA, TACE, or PEI followed by DC immunotherapy reduced recurrence risk and enhanced tumor-specific immunity (105). Combining DC vaccines or CIK with conventional treatments improved prognosis, OSR, and reduced recurrence (106). DC monotherapy significantly improved 1-year OSR, demonstrating its clinical utility as an HCC adjuvant therapy.
Oncolytic viruses proliferate only within tumor cells, causing tumor cell lysis, and exert dual effects by specifically killing tumor cells and activating the body’s antitumor immune response. JX-594, an oncolytic vaccinia virus, has been shown to have therapeutic effects in liver cancer. Heo et al. (107) injected the JX-594 virus vaccine into liver cancer tissue, confirming its therapeutic effect and demonstrating early efficacy. Additionally, Pexa-Vec, another oncolytic virus, has garnered widespread attention in HCC treatment. A clinical trial combining Pexa-Vec with sorafenib for advanced HCC patients showed that the combination treatment slowed disease progression and prolonged survival in patients with advanced HCC (108).
Cytokines, including IFN, IL, colony-stimulating factors, and TNF are immune cell-secreted signaling molecules. IFN exhibits antiviral, immune-regulating, anti-angiogenic, and pro-apoptotic effects, crucial in tumor treatment. Lee et al. (109) found PEGylated IFN reduced recurrence in post-surgery liver cancer patients. Bertelli et al. (110) showed IL-2 prolonged survival in inoperable HCC patients, highlighting cytokines’ role in liver cancer therapy.
CAR-T therapy involves genetically modifying T lymphocytes to express receptors targeting tumor antigens, which recognize specific ligands to exert antitumor effects (111). Target antigens, overexpressed in tumors but minimally in normal tissues, are crucial for efficacy. Common targets in liver cancer include GPC3, AFP, hepatocyte growth factor receptor, and MUC1. GPC3 CAR-T delayed disease progression in HCC with good safety (112). GPC3-CAR T cells showed tumor-clearing effects in HCC patient-derived xenograft models (113). A phase I trial (NCT02395250, NCT03146234) reported 2 of 13 advanced HCC patients achieved PR, supporting CAR-GPC3 T cell therapy’s safety and efficacy (114).
Previous clinical studies have shown that CIK cell therapy enhances immune function, clears residual tumor foci, and reduces tumor recurrence. Currently, DC and CIK cells are co-cultured to form DC-CIK cells. By presenting tumor antigens to CIK cells, DCs make the immune response more specific, enhancing CIK cell-mediated tumor cell killing. Wang et al. (115) showed that after co-culturing GPC3-transfected DCs with CIK cells, the differentiation and IFN-γ secretion of CIK cells were promoted, inducing a specific killing effect against GPC3-expressing liver cancer cells. DC-CIK cell immunotherapy has broad application prospects in liver cancer treatment.
TCR-T therapy targets tumor antigen peptides presented by the major histocompatibility complex (MHC), with its antitumor activity primarily depending on CD8+ T lymphocytes. As the hepatitis B virus (HBV) genetic fragments can integrate into the chromosomes of liver cells during chronic infection, TCR-T therapy can target HBV antigens in HCC cells, making TCR-T therapy particularly advantageous in HBV-related HCC (116).
NK cells, comprising 30%-50% of intrahepatic lymphocytes, are crucial in immune defense against viral infections and tumor development. Dysfunction in NK cells, including reduced numbers and functional defects, facilitates tumor immune evasion, contributing to HCC (117). Allogeneic NK cell immunotherapy is increasingly used in clinical trials. Combining irreversible electroporation with NK cell therapy significantly improved median OS in stage IV HCC patients (NCT03008343) (118). Another trial (NCT01147380) infused donor-derived activated NK cells into liver transplant recipients, showing safety and feasibility in preventing metastasis and recurrence (119). Genetic modification of NK cells is also explored to enhance specificity and efficacy.
Dual ICI combination therapy demonstrates synergistic effects, enhancing response rates and efficacy. Studies on PD-1/PD-L1 and CTLA-4 inhibitors in melanoma have informed HCC trials. In CheckMate 040, nivolumab and ipilimumab in sorafenib-treated HCC patients yielded an ORR of 34%, DCR of 51.2%, and 60-month survival of 29% (120). The CheckMate 9DW trial provided updated evidence supporting the significant OS benefits over sorafenib (121). The HIMALAYA trial established the combination of tremelimumab and durvalumab as an effective first-line therapy, achieving a median OS of 16.4 months compared to 13.8 months with sorafenib (122). GITR agonist antibodies with PD-1 inhibitors may overcome monotherapy resistance (89, 123). They enhance TIL responses in advanced HCC (124), which indicates improved anti-tumor efficacy.
Molecular targeted drugs inhibiting tumor angiogenesis can suppress the tumor’s immune-suppressive microenvironment and enhance immunotherapy sensitivity (125, 126). Combining immunotherapy with targeted treatment yields synergistic anti-tumor effects. Atezolizumab plus bevacizumab showed superior efficacy in advanced unresectable HCC than sorafenib alone (86). KEYNOTE-524 (127) and LEAP-002 trial (128) reported elevated ORR or OS in advanced HCC. These findings highlight the efficacy of ICI combined with targeted drugs, overcoming monotherapy limitations.
HCC treatments like ablation and interventional therapies release tumor antigens, induce necrosis, and activate immune cells (CD8+ T-cells, NK cells). Combined with immunotherapy, they enhance anti-tumor effects. A study showed ablation with tremelimumab increased CD8+ T-cells, with median OS of 12.3 months (129). TACE, a non-surgical HCC treatment, induces tumor necrosis. DC-CIK with TACE prolonged PFS than TACE alone, suggesting immunotherapy combined with local treatments benefits non-surgical HCC patients (130).
Combining 131I-Metoxymab, a radioactive fragment targeting HCC-associated CD147, with radiofrequency ablation (RFA) significantly lowered 1- and 2-year recurrence rates compared to RFA alone (131). This finding highlights the therapeutic potential of McAb-mediated radiotherapy through dual cytotoxic mechanisms in liver cancer treatment. In HCC murine models, combined Radiotherapy and anti-PD-L1 therapy enhanced survival and tumor control versus monotherapies, with Radiotherapy -induced PD-L1 upregulation via IFN-gamma/STAT3 potentially boosting immunotherapy (132). A matched analysis showed Radiotherapy addition to anti-PD-1 and anti-angiogenic therapy improved ORR, OS, and PFS (133) (Table 1).
The immune microenvironment plays a pivotal role in the progression of hepatocellular carcinoma, with immune cells both promoting tumor growth and suppressing anti-tumor responses. The intricate interplay between TAMs, TILs, and MDSCs shapes the immune landscape, influencing the effectiveness of therapies. Immunotherapy, particularly the use of immune checkpoint inhibitors, has revolutionized treatment strategies for HCC, offering new hope for patients with advanced disease. The combination of ICIs with molecular targeted drugs, local treatments, or radiotherapy holds significant promise for improving treatment outcomes. However, HCC immunotherapy faces limitations due to low response rates and resistance, driven by TME-induced immune suppression, T-cell exhaustion, and immunosuppressive cells. Combination therapies show variable efficacy and pose risks of immune-related adverse events, necessitating deeper insights into resistance mechanisms and predictive biomarkers.
DX: Writing – original draft. YL: Writing – original draft. FX: Writing – original draft. ZBD: Writing – original draft. ML: Writing – original draft. QZ: Writing – original draft. ZQD: Writing – original draft, Writing – review & editing.
The author(s) declare that financial support was received for the research and/or publication of this article. National Inheritance Studio Construction Project for Renowned Traditional Chinese Medicine Experts (Document No. Guo Zhong Yi Yao Ren Jiao Han [2022] No. 75 issued by the National Administration of Traditional Chinese Medicine); Special Research Project on Traditional Chinese Medicine in Henan Province (2023ZY2062).
The authors declare that the research was conducted in the absence of any commercial or financial relationships that could be construed as a potential conflict of interest.
The author(s) declare that no Generative AI was used in the creation of this manuscript.
All claims expressed in this article are solely those of the authors and do not necessarily represent those of their affiliated organizations, or those of the publisher, the editors and the reviewers. Any product that may be evaluated in this article, or claim that may be made by its manufacturer, is not guaranteed or endorsed by the publisher.
1. Llovet JM, Kelley RK, Villanueva A, Singal AG, Pikarsky E, Roayaie S, et al. Hepatocellular carcinoma. Nat Rev Dis Primers. (2021) 7:6. doi: 10.1038/s41572-020-00240-3
2. Li X, Ramadori P, Pfister D, Seehawer M, Zender L, Heikenwalder M. The immunological and metabolic landscape in primary and metastatic liver cancer. Nat Rev Cancer. (2021) 21:541–57. doi: 10.1038/s41568-021-00383-9
3. Hanahan D, Coussens LM. Accessories to the crime: functions of cells recruited to the tumor microenvironment. Cancer Cell. (2012) 21:309–22. doi: 10.1016/j.ccr.2012.02.022
4. Zhang X, Zhuge J, Liu J, Xia Z, Wang H, Gao Q, et al. Prognostic signatures of sphingolipids: Understanding the immune landscape and predictive role in immunotherapy response and outcomes of hepatocellular carcinoma. Front Immunol. (2023) 14:1153423. doi: 10.3389/fimmu.2023.1153423
5. Affo S, Yu LX, Schwabe RF. The role of cancer-associated fibroblasts and fibrosis in liver cancer. Annu Rev Pathol. (2017) 12:153–86. doi: 10.1146/annurev-pathol-052016-100322
6. Chew V, Lai L, Pan L, Lim CJ, Li J, Ong R, et al. Delineation of an immunosuppressive gradient in hepatocellular carcinoma using high-dimensional proteomic and transcriptomic analyses. Proc Natl Acad Sci U.S.A. (2017) 114:E5900–9. doi: 10.1073/pnas.1706559114
7. Wilson JL, Nagele T, Linke M, Demel F, Fritsch SD, Mayr HK, et al. Inverse data-Driven modeling and multiomics analysis reveals phgdh as a metabolic checkpoint of macrophage polarization and proliferation. Cell Rep. (2020) 30:1542–1552 e1547. doi: 10.1016/j.celrep.2020.01.011
8. Wu L, Zhang X, Zheng L, Zhao H, Yan G, Zhang Q, et al. RIPK3 orchestrates fatty acid metabolism in tumor-associated macrophages and hepatocarcinogenesis. Cancer Immunol Res. (2020) 8:710–21. doi: 10.1158/2326-6066.CIR-19-0261
9. Ding T, Xu J, Wang F, Shi M, Zhang Y, Li SP, et al. High tumor-infiltrating macrophage density predicts poor prognosis in patients with primary hepatocellular carcinoma after resection. Hum Pathol. (2009) 40:381–9. doi: 10.1016/j.humpath.2008.08.011
10. Zhang J, Zhang Q, Lou Y, Fu Q, Chen Q, Wei T, et al. Hypoxia-inducible factor-1alpha/interleukin-1beta signaling enhances hepatoma epithelial-mesenchymal transition through macrophages in a hypoxic-inflammatory microenvironment. Hepatology. (2018) 67:1872–89. doi: 10.1002/hep.29681
11. Gong X, Chi H, Strohmer DF, Teichmann AT, Xia Z, Wang Q. Exosomes: A potential tool for immunotherapy of ovarian cancer. Front Immunol. (2022) 13:1089410. doi: 10.3389/fimmu.2022.1089410
12. Kuang DM, Peng C, Zhao Q, Wu Y, Chen MS, Zheng L. Activated monocytes in peritumoral stroma of hepatocellular carcinoma promote expansion of memory T helper 17 cells. Hepatology. (2010) 51:154–64. doi: 10.1002/hep.23291
13. Zhang H, Xia T, Xia Z, Zhou H, Li Z, Wang W, et al. KIF18A inactivates hepatic stellate cells and alleviates liver fibrosis through the TTC3/Akt/mTOR pathway. Cell Mol Life Sci. (2024) 81:96. doi: 10.1007/s00018-024-05114-5
14. Xiao J, Lin H, Liu B, Xia Z, Zhang J, Jin J. Decreased S1P and SPHK2 are involved in pancreatic acinar cell injury. biomark Med. (2019) 13:627–37. doi: 10.2217/bmm-2018-0404
15. Wang JF, Wang JS, Liu Y, Ji B, Ding BC, Wang YX, et al. Knockdown of integrin beta1 inhibits proliferation and promotes apoptosis in bladder cancer cells. Biofactors. (2025) 51:e2150. doi: 10.1002/biof.2150
16. Yan W, Liu X, Ma H, Zhang H, Song X, Gao L, et al. Tim-3 fosters HCC development by enhancing TGF-beta-mediated alternative activation of macrophages. Gut. (2015) 64:1593–604. doi: 10.1136/gutjnl-2014-307671
17. Bao D, Zhao J, Zhou X, Yang Q, Chen Y, Zhu J, et al. Mitochondrial fission-induced mtDNA stress promotes tumor-associated macrophage infiltration and HCC progression. Oncogene. (2019) 38:5007–20. doi: 10.1038/s41388-019-0772-z
18. Gunaydin G. CAFs interacting with TAMs in tumor microenvironment to enhance tumorigenesis and immune evasion. Front Oncol. (2021) 11:668349. doi: 10.3389/fonc.2021.668349
19. Ding W, Xu X, Qian Y, Xue W, Wang Y, Du J, et al. Prognostic value of tumor-infiltrating lymphocytes in hepatocellular carcinoma: A meta-analysis. Med (Baltimore). (2018) 97:e13301. doi: 10.1097/MD.0000000000013301
20. Xie H, Xi X, Lei T, Liu H, Xia Z. CD8(+) T cell exhaustion in the tumor microenvironment of breast cancer. Front Immunol. (2024) 15:1507283. doi: 10.3389/fimmu.2024.1507283
21. Xiong J, Chi H, Yang G, Zhao S, Zhang J, Tran LJ, et al. Revolutionizing anti-tumor therapy: unleashing the potential of B cell-derived exosomes. Front Immunol. (2023) 14:1188760. doi: 10.3389/fimmu.2023.1188760
22. Wang Y, Zhu H, Wang X. Prognosis and immune infiltration analysis of endoplasmic reticulum stress-related genes in bladder urothelial carcinoma. Front Genet. (2022) 13:965100. doi: 10.3389/fgene.2022.965100
23. Schreiber RD, Old LJ, Smyth MJ. Cancer immunoediting: integrating immunity’s roles in cancer suppression and promotion. Science. (2011) 331:1565–70. doi: 10.1126/science.1203486
24. Wang Y, Wang J, Liu J, Zhu H. Immune-related diagnostic markers for benign prostatic hyperplasia and their potential as drug targets. Front Immunol. (2024) 15:1516362. doi: 10.3389/fimmu.2024.1516362
25. Prieto J, Melero I, Sangro B. Immunological landscape and immunotherapy of hepatocellular carcinoma. Nat Rev Gastroenterol Hepatol. (2015) 12:681–700. doi: 10.1038/nrgastro.2015.173
26. Katz SC, Bamboat ZM, Maker AV, Shia J, Pillarisetty VG, Yopp AC, et al. Regulatory T cell infiltration predicts outcome following resection of colorectal cancer liver metastases. Ann Surg Oncol. (2013) 20:946–55. doi: 10.1245/s10434-012-2668-9
27. Wang F, Jing X, Li G, Wang T, Yang B, Zhu Z, et al. Foxp3+ regulatory T cells are associated with the natural history of chronic hepatitis B and poor prognosis of hepatocellular carcinoma. Liver Int. (2012) 32:644–55. doi: 10.1111/j.1478-3231.2011.02675.x
28. Chew V, Chen J, Lee D, Loh E, Lee J, Lim KH, et al. Chemokine-driven lymphocyte infiltration: an early intratumoural event determining long-term survival in resectable hepatocellular carcinoma. Gut. (2012) 61:427–38. doi: 10.1136/gutjnl-2011-300509
29. Ramzan M, Sturm N, Decaens T, Bioulac-Sage P, Bancel B, Merle P, et al. Liver-infiltrating CD8(+) lymphocytes as prognostic factor for tumour recurrence in hepatitis C virus-related hepatocellular carcinoma. Liver Int. (2016) 36:434–44. doi: 10.1111/liv.2016.36.issue-3
30. Di Pilato M, Kfuri-Rubens R, Pruessmann JN, Ozga AJ, Messemaker M, Cadilha BL, et al. CXCR6 positions cytotoxic T cells to receive critical survival signals in the tumor microenvironment. Cell. (2021) 184:4512–4530 e4522. doi: 10.1016/j.cell.2021.07.015
31. Zhao Y, Wei K, Chi H, Xia Z, Li X. IL-7: A promising adjuvant ensuring effective T cell responses and memory in combination with cancer vaccines? Front Immunol. (2022) 13:1022808. doi: 10.3389/fimmu.2022.1022808
32. Motz GT, Santoro SP, Wang LP, Garrabrant T, Lastra RR, Hagemann IS, et al. Tumor endothelium FasL establishes a selective immune barrier promoting tolerance in tumors. Nat Med. (2014) 20:607–15. doi: 10.1038/nm.3541
33. Moreno-Cubero E, Larrubia JR. Specific CD8(+) T cell response immunotherapy for hepatocellular carcinoma and viral hepatitis. World J Gastroenterol. (2016) 22:6469–83. doi: 10.3748/wjg.v22.i28.6469
34. Xia Z, Chen S, He M, Li B, Deng Y, Yi L, et al. Editorial: Targeting metabolism to activate T cells and enhance the efficacy of checkpoint blockade immunotherapy in solid tumors. Front Immunol. (2023) 14:1247178. doi: 10.3389/fimmu.2023.1247178
35. Li Z, Zhou H, Xia Z, Xia T, Du G, Franziska SD, et al. HMGA1 augments palbociclib efficacy via PI3K/mTOR signaling in intrahepatic cholangiocarcinoma. biomark Res. (2023) 11:33. doi: 10.1186/s40364-023-00473-w
36. Zhang J, Peng G, Chi H, Yang J, Xie X, Song G, et al. CD8 + T-cell marker genes reveal different immune subtypes of oral lichen planus by integrating single-cell RNA-seq and bulk RNA-sequencing. BMC Oral Health. (2023) 23:464. doi: 10.1186/s12903-023-03138-0
37. Soltani M, Zhao Y, Xia Z, Ganjalikhani Hakemi M, Bazhin AV. The importance of cellular metabolic pathways in pathogenesis and selective treatments of hematological Malignancies. Front Oncol. (2021) 11:767026. doi: 10.3389/fonc.2021.767026
38. Guo CL, Yang XH, Cheng W, Xu Y, Li JB, Sun YX, et al. Expression of Fas/FasL in CD8+ T and CD3+ Foxp3+ Treg cells–relationship with apoptosis of circulating CD8+ T cells in hepatocellular carcinoma patients. Asian Pac J Cancer Prev. (2014) 15:2613–8. doi: 10.7314/APJCP.2014.15.6.2613
39. Zhai X, Xia Z, Du G, Zhang X, Xia T, Ma D, et al. LRP1B suppresses HCC progression through the NCSTN/PI3K/AKT signaling axis and affects doxorubicin resistance. Genes Dis. (2023) 10:2082–96. doi: 10.1016/j.gendis.2022.10.021
40. Han Y, Chen Z, Yang Y, Jiang Z, Gu Y, Liu Y, et al. Human CD14+ CTLA-4+ regulatory dendritic cells suppress T-cell response by cytotoxic T-lymphocyte antigen-4-dependent IL-10 and indoleamine-2,3-dioxygenase production in hepatocellular carcinoma. Hepatology. (2014) 59:567–79. doi: 10.1002/hep.26694
41. Cioplea M, Nichita L, Georgescu D, Sticlaru L, Cioroianu A, Nedelcu R, et al. FOXP3 in melanoma with regression: between tumoral expression and regulatory T cell upregulation. J Immunol Res. (2020) 2020:5416843. doi: 10.1155/2020/5416843
42. Chen KJ, Lin SZ, Zhou L, Xie HY, Zhou WH, Taki-Eldin A, et al. Selective recruitment of regulatory T cell through CCR6-CCL20 in hepatocellular carcinoma fosters tumor progression and predicts poor prognosis. PloS One. (2011) 6:e24671. doi: 10.1371/journal.pone.0024671
43. Yang XH, Yamagiwa S, Ichida T, Matsuda Y, Sugahara S, Watanabe H, et al. Increase of CD4+ CD25+ regulatory T-cells in the liver of patients with hepatocellular carcinoma. J Hepatol. (2006) 45:254–62. doi: 10.1016/j.jhep.2006.01.036
44. Ormandy LA, Hillemann T, Wedemeyer H, Manns MP, Greten TF, Korangy F. Increased populations of regulatory T cells in peripheral blood of patients with hepatocellular carcinoma. Cancer Res. (2005) 65:2457–64. doi: 10.1158/0008-5472.CAN-04-3232
45. Zhang X, Zhang P, Cong A, Feng Y, Chi H, Xia Z, et al. Unraveling molecular networks in thymic epithelial tumors: deciphering the unique signatures. Front Immunol. (2023) 14:1264325. doi: 10.3389/fimmu.2023.1264325
46. Wang Q, Yu T, Yuan Y, Zhuang H, Wang Z, Liu X, et al. Sorafenib reduces hepatic infiltrated regulatory T cells in hepatocellular carcinoma patients by suppressing TGF-beta signal. J Surg Oncol. (2013) 107:422–7. doi: 10.1002/jso.v107.4
47. Smyth LA, Boardman DA, Tung SL, Lechler R, Lombardi G. MicroRNAs affect dendritic cell function and phenotype. Immunology. (2015) 144:197–205. doi: 10.1111/imm.2015.144.issue-2
48. Deng Y, Shi M, Yi L, Naveed Khan M, Xia Z, Li X. Eliminating a barrier: Aiming at VISTA, reversing MDSC-mediated T cell suppression in the tumor microenvironment. Heliyon. (2024) 10:e37060. doi: 10.1016/j.heliyon.2024.e37060
49. Talmadge JE, Gabrilovich DI. History of myeloid-derived suppressor cells. Nat Rev Cancer. (2013) 13:739–52. doi: 10.1038/nrc3581
50. Tian X, Shen H, Li Z, Wang T, Wang S. Tumor-derived exosomes, myeloid-derived suppressor cells, and tumor microenvironment. J Hematol Oncol. (2019) 12:84. doi: 10.1186/s13045-019-0772-z
51. Liao W, Overman MJ, Boutin AT, Shang X, Zhao D, Dey P, et al. KRAS-IRF2 axis drives immune suppression and immune therapy resistance in colorectal cancer. Cancer Cell. (2019) 35:559–572 e557. doi: 10.1016/j.ccell.2019.02.008
52. Hoechst B, Ormandy LA, Ballmaier M, Lehner F, Kruger C, Manns MP, et al. A new population of myeloid-derived suppressor cells in hepatocellular carcinoma patients induces CD4(+)CD25(+)Foxp3(+) T cells. Gastroenterology. (2008) 135:234–43. doi: 10.1053/j.gastro.2008.03.020
53. Hoechst B, Voigtlaender T, Ormandy L, Gamrekelashvili J, Zhao F, Wedemeyer H, et al. Myeloid derived suppressor cells inhibit natural killer cells in patients with hepatocellular carcinoma via the NKp30 receptor. Hepatology. (2009) 50:799–807. doi: 10.1002/hep.23054
54. Zhou J, Liu M, Sun H, Feng Y, Xu L, Chan AWH, et al. Hepatoma-intrinsic CCRK inhibition diminishes myeloid-derived suppressor cell immunosuppression and enhances immune-checkpoint blockade efficacy. Gut. (2018) 67:931–44. doi: 10.1136/gutjnl-2017-314032
55. Hochst B, Schildberg FA, Sauerborn P, Gabel YA, Gevensleben H, Goltz D, et al. Activated human hepatic stellate cells induce myeloid derived suppressor cells from peripheral blood monocytes in a CD44-dependent fashion. J Hepatol. (2013) 59:528–35. doi: 10.1016/j.jhep.2013.04.033
56. Xie Q-H, Zheng J-Q, Ding J-Y, Wu Y-F, Liu L, Yu Z-L, et al. Exosome-mediated immunosuppression in tumor microenvironments. Cells. (2022) 11:1946. doi: 10.3390/cells11121946
57. Constantino J, Gomes C, Falcao A, Neves BM, Cruz MT. Dendritic cell-based immunotherapy: a basic review and recent advances. Immunol Res. (2017) 65:798–810. doi: 10.1007/s12026-017-8931-1
58. Szczerba BM, Castro-Giner F, Vetter M, Krol I, Gkountela S, Landin J, et al. Neutrophils escort circulating tumour cells to enable cell cycle progression. Nature. (2019) 566:553–7. doi: 10.1038/s41586-019-0915-y
59. Kunitani H, Shimizu Y, Murata H, Higuchi K, Watanabe A. Phenotypic analysis of circulating and intrahepatic dendritic cell subsets in patients with chronic liver diseases. J Hepatol. (2002) 36:734–41. doi: 10.1016/S0168-8278(02)00062-4
60. Beckebaum S, Zhang X, Chen X, Yu Z, Frilling A, Dworacki G, et al. Increased levels of interleukin-10 in serum from patients with hepatocellular carcinoma correlate with profound numerical deficiencies and immature phenotype of circulating dendritic cell subsets. Clin Cancer Res. (2004) 10:7260–9. doi: 10.1158/1078-0432.CCR-04-0872
61. Pedroza-Gonzalez A, Zhou G, Vargas-Mendez E, Boor PP, Mancham S, Verhoef C, et al. Tumor-infiltrating plasmacytoid dendritic cells promote immunosuppression by Tr1 cells in human liver tumors. Oncoimmunology. (2015) 4:e1008355. doi: 10.1080/2162402X.2015.1008355
62. Zhou ZJ, Xin HY, Li J, Hu ZQ, Luo CB, Zhou SL. Intratumoral plasmacytoid dendritic cells as a poor prognostic factor for hepatocellular carcinoma following curative resection. Cancer Immunol Immunother. (2019) 68:1223–33. doi: 10.1007/s00262-019-02355-3
63. Cozar B, Greppi M, Carpentier S, Narni-Mancinelli E, Chiossone L, Vivier E. Tumor-infiltrating natural killer cells. Cancer Discovery. (2021) 11:34–44. doi: 10.1158/2159-8290.CD-20-0655
64. Harmon C, Robinson MW, Fahey R, Whelan S, Houlihan DD, Geoghegan J, et al. Tissue-resident Eomes(hi) T-bet(lo) CD56(bright) NK cells with reduced proinflammatory potential are enriched in the adult human liver. Eur J Immunol. (2016) 46:2111–20. doi: 10.1002/eji.201646559
65. Poznanski SM, Singh K, Ritchie TM, Aguiar JA, Fan IY, Portillo AL, et al. Metabolic flexibility determines human NK cell functional fate in the tumor microenvironment. Cell Metab. (2021) 33:1205–1220 e1205. doi: 10.1016/j.cmet.2021.03.023
66. Chew V, Tow C, Teo M, Wong HL, Chan J, Gehring A, et al. Inflammatory tumour microenvironment is associated with superior survival in hepatocellular carcinoma patients. J Hepatol. (2010) 52:370–9. doi: 10.1016/j.jhep.2009.07.013
67. Cai L, Zhang Z, Zhou L, Wang H, Fu J, Zhang S, et al. Functional impairment in circulating and intrahepatic NK cells and relative mechanism in hepatocellular carcinoma patients. Clin Immunol. (2008) 129:428–37. doi: 10.1016/j.clim.2008.08.012
68. Liu Y, Cheng Y, Xu Y, Wang Z, Du X, Li C, et al. Increased expression of programmed cell death protein 1 on NK cells inhibits NK-cell-mediated anti-tumor function and indicates poor prognosis in digestive cancers. Oncogene. (2017) 36:6143–53. doi: 10.1038/onc.2017.209
69. Sun C, Xu J, Huang Q, Huang M, Wen H, Zhang C, et al. High NKG2A expression contributes to NK cell exhaustion and predicts a poor prognosis of patients with liver cancer. Oncoimmunology. (2017) 6:e1264562. doi: 10.1080/2162402X.2016.1264562
70. Sprinzl MF, Reisinger F, Puschnik A, Ringelhan M, Ackermann K, Hartmann D, et al. Sorafenib perpetuates cellular anticancer effector functions by modulating the crosstalk between macrophages and natural killer cells. Hepatology. (2013) 57:2358–68. doi: 10.1002/hep.26328
71. Hage C, Hoves S, Strauss L, Bissinger S, Prinz Y, Poschinger T, et al. Sorafenib induces pyroptosis in macrophages and triggers natural killer cell-mediated cytotoxicity against hepatocellular carcinoma. Hepatology. (2019) 70:1280–97. doi: 10.1002/hep.30666
72. Wen ZF, Liu H, Gao R, Zhou M, Ma J, Zhang Y, et al. Tumor cell-released autophagosomes (TRAPs) promote immunosuppression through induction of M2-like macrophages with increased expression of PD-L1. J Immunother Cancer. (2018) 6:151. doi: 10.1186/s40425-018-0452-5
73. Zhao W, Cao L, Ying H, Zhang W, Li D, Zhu X, et al. Endothelial CDS2 deficiency causes VEGFA-mediated vascular regression and tumor inhibition. Cell Res. (2019) 29:895–910. doi: 10.1038/s41422-019-0229-5
74. Wang D, Bai N, Hu X, OuYang XW, Yao L, Tao Y, et al. Preoperative inflammatory markers of NLR and PLR as indicators of poor prognosis in resectable HCC. PeerJ. (2019) 7:e7132. doi: 10.7717/peerj.7132
75. Ruhland MK, Roberts EW, Cai E, Mujal AM, Marchuk K, Beppler C, et al. Visualizing synaptic transfer of tumor antigens among dendritic cells. Cancer Cell. (2020) 37:786–799 e785. doi: 10.1016/j.ccell.2020.05.002
76. Zhou SL, Dai Z, Zhou ZJ, Wang XY, Yang GH, Wang Z, et al. Overexpression of CXCL5 mediates neutrophil infiltration and indicates poor prognosis for hepatocellular carcinoma. Hepatology. (2012) 56:2242–54. doi: 10.1002/hep.25907
77. Zhou SL, Yin D, Hu ZQ, Luo CB, Zhou ZJ, Xin HY, et al. A positive feedback loop between cancer stem-like cells and tumor-associated neutrophils controls hepatocellular carcinoma progression. Hepatology. (2019) 70:1214–30. doi: 10.1002/hep.30630
78. Zhou SL, Zhou ZJ, Hu ZQ, Huang XW, Wang Z, Chen EB, et al. Tumor-associated neutrophils recruit macrophages and T-regulatory cells to promote progression of hepatocellular carcinoma and resistance to sorafenib. Gastroenterology. (2016) 150:1646–1658 e1617. doi: 10.1053/j.gastro.2016.02.040
79. van der Windt DJ, Sud V, Zhang H, Varley PR, Goswami J, Yazdani HO, et al. Neutrophil extracellular traps promote inflammation and development of hepatocellular carcinoma in nonalcoholic steatohepatitis. Hepatology. (2018) 68:1347–60. doi: 10.1002/hep.29914
80. Li Z, Li N, Li F, Zhou Z, Sang J, Chen Y, et al. Immune checkpoint proteins PD-1 and TIM-3 are both highly expressed in liver tissues and correlate with their gene polymorphisms in patients with HBV-related hepatocellular carcinoma. Med (Baltimore). (2016) 95:e5749. doi: 10.1097/MD.0000000000005749
81. El-Khoueiry AB, Sangro B, Yau T, Crocenzi TS, Kudo M, Hsu C, et al. Nivolumab in patients with advanced hepatocellular carcinoma (CheckMate 040): an open-label, non-comparative, phase 1/2 dose escalation and expansion trial. Lancet. (2017) 389:2492–502. doi: 10.1016/S0140-6736(17)31046-2
82. Finn RS, Ryoo BY, Merle P, Kudo M, Bouattour M, Lim HY, et al. Pembrolizumab as second-line therapy in patients with advanced hepatocellular carcinoma in KEYNOTE-240: A randomized, double-blind, phase III trial. J Clin Oncol. (2020) 38:193–202. doi: 10.1200/JCO.19.01307
83. Qin S, Ren Z, Meng Z, Chen Z, Chai X, Xiong J, et al. Camrelizumab in patients with previously treated advanced hepatocellular carcinoma: a multicentre, open-label, parallel-group, randomised, phase 2 trial. Lancet Oncol. (2020) 21:571–80. doi: 10.1016/S1470-2045(20)30011-5
84. Zhu AX, Finn RS, Edeline J, Cattan S, Ogasawara S, Palmer D, et al. Pembrolizumab in patients with advanced hepatocellular carcinoma previously treated with sorafenib (KEYNOTE-224): a non-randomised, open-label phase 2 trial. Lancet Oncol. (2018) 19:940–52. doi: 10.1016/S1470-2045(18)30351-6
85. Kudo M, Finn RS, Edeline J, Cattan S, Ogasawara S, Palmer DH, et al. Updated efficacy and safety of KEYNOTE-224: a phase II study of pembrolizumab in patients with advanced hepatocellular carcinoma previously treated with sorafenib. Eur J Cancer. (2022) 167:1–12. doi: 10.1016/j.ejca.2022.02.009
86. Finn RS, Qin S, Ikeda M, Galle PR, Ducreux M, Kim TY, et al. Atezolizumab plus bevacizumab in unresectable hepatocellular carcinoma. N Engl J Med. (2020) 382:1894–905. doi: 10.1056/NEJMoa1915745
87. Brown C, Sekhavati F, Cardenes R, Windmueller C, Dacosta K, Rodriguez-Canales J, et al. CTLA-4 immunohistochemistry and quantitative image analysis for profiling of human cancers. J Histochem Cytochem. (2019) 67:901–18. doi: 10.1369/0022155419882292
88. Lombardi A, Mondelli MU. Review article: immune checkpoint inhibitors and the liver, from therapeutic efficacy to side effects. Aliment Pharmacol Ther. (2019) 50:872–84. doi: 10.1111/apt.15449
89. Zappasodi R, Sirard C, Li Y, Budhu S, Abu-Akeel M, Liu C, et al. Rational design of anti-GITR-based combination immunotherapy. Nat Med. (2019) 25:759–66. doi: 10.1038/s41591-019-0420-8
90. Couzinet A, Suzuki T, Nakatsura T. Progress and challenges in glypican-3 targeting for hepatocellular carcinoma therapy. Expert Opin Ther Targets. (2024) 28:895–909. doi: 10.1080/14728222.2024.2416975
91. Li Y, Wang Y, Wang Y, Huang J, Guo Z. Development and evaluation of (68)Ga-labeled TMTP1-based cyclic peptide probes for targeting hepatocellular carcinoma. Mol Pharm. (2025). doi: 10.1021/acs.molpharmaceut.4c01123
92. Wang Y, Wang X. A pan-cancer analysis of heat-shock protein 90 beta1(HSP90B1) in human tumours. Biomolecules. (2022) 12:1377. doi: 10.3390/biom12101377
93. Sawada Y, Yoshikawa T, Ofuji K, Yoshimura M, Tsuchiya N, Takahashi M, et al. Phase II study of the GPC3-derived peptide vaccine as an adjuvant therapy for hepatocellular carcinoma patients. Oncoimmunology. (2016) 5:e1129483. doi: 10.1080/2162402X.2015.1129483
94. Mizukoshi E, Nakagawa H, Kitahara M, Yamashita T, Arai K, Sunagozaka H, et al. Phase I trial of multidrug resistance-associated protein 3-derived peptide in patients with hepatocellular carcinoma. Cancer Lett. (2015) 369:242–9. doi: 10.1016/j.canlet.2015.08.020
95. Wang Y, Zhu H, Xu H, Qiu Y, Zhu Y, Wang X. Senescence-related gene c-Myc affects bladder cancer cell senescence by interacting with HSP90B1 to regulate cisplatin sensitivity. Aging (Albany NY). (2023) 15:7408–23. doi: 10.18632/aging.204863
96. Sawada Y, Sakai M, Yoshikawa T, Ofuji K, Nakatsura T. A glypican-3-derived peptide vaccine against hepatocellular carcinoma. Oncoimmunology. (2012) 1:1448–50. doi: 10.4161/onci.21351
97. Sawada Y, Yoshikawa T, Nobuoka D, Shirakawa H, Kuronuma T, Motomura Y, et al. Phase I trial of a glypican-3-derived peptide vaccine for advanced hepatocellular carcinoma: immunologic evidence and potential for improving overall survival. Clin Cancer Res. (2012) 18:3686–96. doi: 10.1158/1078-0432.CCR-11-3044
98. Butterfield LH, Ribas A, Meng WS, Dissette VB, Amarnani S, Vu HT, et al. T-cell responses to HLA-A*0201 immunodominant peptides derived from alpha-fetoprotein in patients with hepatocellular cancer. Clin Cancer Res. (2003) 9:5902–8.
99. Pang XH, Chen MS, Jia WH, Zhou XX. Inhibitory effects of human AFP-derived peptide-pulsed dendritic cells on mouse hepatocellular carcinoma. Ai Zheng. (2008) 27:1233–8.
100. Nakagawa H, Mizukoshi E, Kobayashi E, Tamai T, Hamana H, Ozawa T, et al. Association between high-avidity T-cell receptors, induced by alpha-fetoprotein-derived peptides, and anti-tumor effects in patients with hepatocellular carcinoma. Gastroenterology. (2017) 152:1395–1406 e1310. doi: 10.1053/j.gastro.2017.02.001
101. Zhu W, Peng Y, Wang L, Hong Y, Jiang X, Li Q, et al. Identification of alpha-fetoprotein-specific T-cell receptors for hepatocellular carcinoma immunotherapy. Hepatology. (2018) 68:574–89. doi: 10.1002/hep.29844
102. El Ansary M, Mogawer S, Elhamid SA, Alwakil S, Aboelkasem F, Sabaawy HE, et al. Immunotherapy by autologous dendritic cell vaccine in patients with advanced HCC. J Cancer Res Clin Oncol. (2013) 139:39–48. doi: 10.1007/s00432-012-1298-8
103. Chen C, Ma YH, Zhang YT, Zhang F, Zhou N, Wang X, et al. Effect of dendritic cell-based immunotherapy on hepatocellular carcinoma: A systematic review and meta-analysis. Cytotherapy. (2018) 20:975–89. doi: 10.1016/j.jcyt.2018.06.002
104. Mizukoshi E, Nakamoto Y, Arai K, Yamashita T, Mukaida N, Matsushima K, et al. Enhancement of tumor-specific T-cell responses by transcatheter arterial embolization with dendritic cell infusion for hepatocellular carcinoma. Int J Cancer. (2010) 126:2164–74. doi: 10.1002/ijc.v126:9
105. Lee JH, Tak WY, Lee Y, Heo MK, Song JS, Kim HY, et al. Adjuvant immunotherapy with autologous dendritic cells for hepatocellular carcinoma, randomized phase II study. Oncoimmunology. (2017) 6:e1328335. doi: 10.1080/2162402X.2017.1328335
106. Cao J, Kong FH, Liu X, Wang XB. Immunotherapy with dendritic cells and cytokine-induced killer cells for hepatocellular carcinoma: A meta-analysis. World J Gastroenterol. (2019) 25:3649–63. doi: 10.3748/wjg.v25.i27.3649
107. Heo J, Reid T, Ruo L, Breitbach CJ, Rose S, Bloomston M, et al. Randomized dose-finding clinical trial of oncolytic immunotherapeutic vaccinia JX-594 in liver cancer. Nat Med. (2013) 19:329–36. doi: 10.1038/nm.3089
108. Breitbach CJ, Burke J, Jonker D, Stephenson J, Haas AR, Chow LQ, et al. Intravenous delivery of a multi-mechanistic cancer-targeted oncolytic poxvirus in humans. Nature. (2011) 477:99–102. doi: 10.1038/nature10358
109. Lee D, Chung YH, Kim JA, Park WH, Jin YJ, Shim JH, et al. Safety and efficacy of adjuvant pegylated interferon therapy for metastatic tumor antigen 1-positive hepatocellular carcinoma. Cancer. (2013) 119:2239–46. doi: 10.1002/cncr.v119.12
110. Bertelli R, Neri F, Tsivian M, Ruhrman N, Cavallari G, Beltempo P, et al. Endolymphatic immunotherapy in inoperable hepatocellular carcinoma. Transplant Proc. (2008) 40:1913–5. doi: 10.1016/j.transproceed.2008.05.049
111. June CH, O’Connor RS, Kawalekar OU, Ghassemi S, Milone MC. CAR T cell immunotherapy for human cancer. Science. (2018) 359:1361–5. doi: 10.1126/science.aar6711
112. Fu Q, Zheng Y, Fang W, Zhao Q, Zhao P, Liu L, et al. RUNX-3-expressing CAR T cells targeting glypican-3 in patients with heavily pretreated advanced hepatocellular carcinoma: a phase I trial. EClinicalMedicine. (2023) 63:102175. doi: 10.1016/j.eclinm.2023.102175
113. Jiang Z, Jiang X, Chen S, Lai Y, Wei X, Li B, et al. Anti-GPC3-CAR T cells suppress the growth of tumor cells in patient-derived xenografts of hepatocellular carcinoma. Front Immunol. (2016) 7:690. doi: 10.3389/fimmu.2016.00690
114. Tampaki M, Ionas E, Hadziyannis E, Deutsch M, Malagari K, Koskinas J. Association of TIM-3 with BCLC stage, serum PD-L1 detection, and response to transarterial chemoembolization in patients with hepatocellular carcinoma. Cancers (Basel). (2020) 12:212. doi: 10.3390/cancers12010212
115. Wang Y, Wang Y, Mu H, Liu T, Chen X, Shen Z. Enhanced specific antitumor immunity of dendritic cells transduced with the glypican 3 gene and co-cultured with cytokine-induced killer cells against hepatocellular carcinoma cells. Mol Med Rep. (2015) 11:3361–7. doi: 10.3892/mmr.2015.3239
116. Rochigneux P, Chanez B, De Rauglaudre B, Mitry E, Chabannon C, Gilabert M. Adoptive cell therapy in hepatocellular carcinoma: biological rationale and first results in early phase clinical trials. Cancers (Basel). (2021) 13:271. doi: 10.3390/cancers13020271
117. Liu P, Chen L, Zhang H. Natural killer cells in liver disease and hepatocellular carcinoma and the NK cell-based immunotherapy. J Immunol Res. (2018) 2018:1206737. doi: 10.1155/2018/1206737
118. Alnaggar M, Lin M, Mesmar A, Liang S, Qaid A, Xu K, et al. Allogenic natural killer cell immunotherapy combined with irreversible electroporation for stage IV hepatocellular carcinoma: survival outcome. Cell Physiol Biochem. (2018) 48:1882–93. doi: 10.1159/000492509
119. Muntasell A, Ochoa MC, Cordeiro L, Berraondo P, Lopez-Diaz-de-Cerio A, Cabo M, et al. Targeting NK-cell checkpoints for cancer immunotherapy. Curr Opin Immunol. (2017) 45:73–81. doi: 10.1016/j.coi.2017.01.003
120. Melero I, Yau T, Kang YK, Kim TY, Santoro A, Sangro B, et al. Nivolumab plus ipilimumab combination therapy in patients with advanced hepatocellular carcinoma previously treated with sorafenib: 5-year results from CheckMate 040. Ann Oncol. (2024) 35:537–48. doi: 10.1016/j.annonc.2024.03.005
121. Kudo M. Nivolumab plus ipilimumab: A novel first-line combination immunotherapy for unresectable hepatocellular carcinoma. Cancer. (2024) 13:459–67. doi: 10.1159/000540801
122. Sangro B, Chan S, Kelley R, Lau G, Kudo M, Sukeepaisarnjaroen W, et al. Four-year overall survival update from the phase III HIMALAYA study of tremelimumab plus durvalumab in unresectable hepatocellular carcinoma. Ann Oncol. (2024) 35:448–57. doi: 10.1016/j.annonc.2024.02.005
123. Buzzatti G, Dellepiane C, Del Mastro L. New emerging targets in cancer immunotherapy: the role of GITR. ESMO Open. (2020) 4:e000738. doi: 10.1136/esmoopen-2020-000738
124. van Beek AA, Zhou G, Doukas M, Boor PPC, Noordam L, Mancham S, et al. GITR ligation enhances functionality of tumor-infiltrating T cells in hepatocellular carcinoma. Int J Cancer. (2019) 145:1111–24. doi: 10.1002/ijc.v145.4
125. Tian L, Goldstein A, Wang H, Ching Lo H, Sun Kim I, Welte T, et al. Mutual regulation of tumour vessel normalization and immunostimulatory reprogramming. Nature. (2017) 544:250–4. doi: 10.1038/nature21724
126. Jin W, Yang Q, Chi H, Wei K, Zhang P, Zhao G, et al. Ensemble deep learning enhanced with self-attention for predicting immunotherapeutic responses to cancers. Front Immunol. (2022) 13:1025330. doi: 10.3389/fimmu.2022.1025330
127. Finn RS, Ikeda M, Zhu AX, Sung MW, Baron AD, Kudo M, et al. Phase ib study of lenvatinib plus pembrolizumab in patients with unresectable hepatocellular carcinoma. J Clin Oncol. (2020) 38:2960–70. doi: 10.1200/JCO.20.00808
128. Llovet JM, Kudo M, Merle P, Meyer T, Qin S, Ikeda M, et al. Lenvatinib plus pembrolizumab versus lenvatinib plus placebo for advanced hepatocellular carcinoma (LEAP-002): a randomised, double-blind, phase 3 trial. Lancet Oncol. (2023) 24:1399–410. doi: 10.1016/S1470-2045(23)00469-2
129. Duffy AG, Ulahannan SV, Makorova-Rusher O, Rahma O, Wedemeyer H, Pratt D, et al. Tremelimumab in combination with ablation in patients with advanced hepatocellular carcinoma. J Hepatol. (2017) 66:545–51. doi: 10.1016/j.jhep.2016.10.029
130. He G, Zheng C, Huo H, Zhang H, Zhu Z, Li J, et al. TACE combined with dendritic cells and cytokine-induced killer cells in the treatment of hepatocellular carcinoma: A meta-analysis. Int Immunopharmacol. (2016) 40:436–42. doi: 10.1016/j.intimp.2016.09.015
131. Bian H, Zheng JS, Nan G, Li R, Chen C, Hu CX, et al. Randomized trial of [131I] metuximab in treatment of hepatocellular carcinoma after percutaneous radiofrequency ablation. J Natl Cancer Inst. (2014) 106:dju239. doi: 10.1093/jnci/dju239
132. Du SS, Chen GW, Yang P, Chen YX, Hu Y, Zhao QQ, et al. Radiation Therapy Promotes Hepatocellular Carcinoma Immune Cloaking via PD-L1 Upregulation Induced by cGAS-STING Activation. Int J Radiat Oncol Biol Phys. (2022) 112:1243–55. doi: 10.1016/j.ijrobp.2021.12.162
Keywords: immune microenvironment, immunotherapy, HCC, NK cell therapy, CAR-T therapy
Citation: Xie D, Liu Y, Xu F, Dang Z, Li M, Zhang Q and Dang Z (2025) Immune microenvironment and immunotherapy in hepatocellular carcinoma: mechanisms and advances. Front. Immunol. 16:1581098. doi: 10.3389/fimmu.2025.1581098
Received: 21 February 2025; Accepted: 17 March 2025;
Published: 02 April 2025.
Edited by:
Minghua Ren, First Affiliated Hospital of Harbin Medical University, ChinaReviewed by:
Liying Ren, Peking University People’s Hospital, ChinaCopyright © 2025 Xie, Liu, Xu, Dang, Li, Zhang and Dang. This is an open-access article distributed under the terms of the Creative Commons Attribution License (CC BY). The use, distribution or reproduction in other forums is permitted, provided the original author(s) and the copyright owner(s) are credited and that the original publication in this journal is cited, in accordance with accepted academic practice. No use, distribution or reproduction is permitted which does not comply with these terms.
*Correspondence: Zhongqin Dang, ZHpocW5AMTI2LmNvbQ==
Disclaimer: All claims expressed in this article are solely those of the authors and do not necessarily represent those of their affiliated organizations, or those of the publisher, the editors and the reviewers. Any product that may be evaluated in this article or claim that may be made by its manufacturer is not guaranteed or endorsed by the publisher.
Research integrity at Frontiers
Learn more about the work of our research integrity team to safeguard the quality of each article we publish.