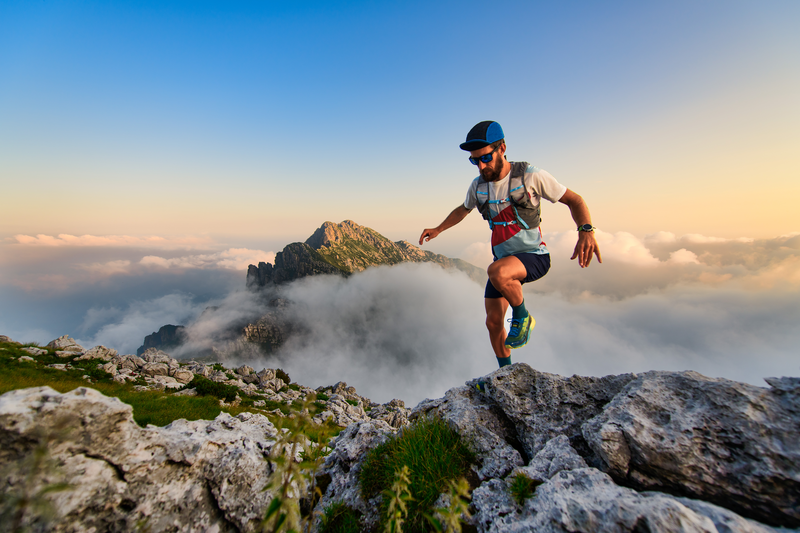
94% of researchers rate our articles as excellent or good
Learn more about the work of our research integrity team to safeguard the quality of each article we publish.
Find out more
ORIGINAL RESEARCH article
Front. Hortic. , 30 August 2024
Sec. Sustainable Pest and Disease Management
Volume 3 - 2024 | https://doi.org/10.3389/fhort.2024.1440410
This article is part of the Research Topic The Use of Volatile Organic Compounds in Sustainable Management of Pests and Diseases View all 4 articles
Conservation biological control (CBC) is a sustainable measure for ecological intensification in agriculture to establish and maintain robust natural enemy populations. CBC is contributing to integrated pest management with reduced use of pesticides and support of native biodiversity in agroecosystems. Despite rapidly expanding research on CBC during the last decades, its application in pest management at the farm level is very limited. Here, we tested a CBC strategy in a 5-year on-farm study at three locations in East Norway. This CBC strategy combined two tools to increase biological control of aphids in spring barley; 1-ATTRACT, the application of a volatile organic compound (VOC) attractant that increases lacewing egg laying, and 2-HABITAT, the maintenance of natural border vegetation. We found that the VOC attractants recruited natural enemies and guided them to the right place at the right time from the border vegetation into the cropping area to control the aphid population efficiently and reliably. The results also showed that the VOC attractants combined with periodical maintained natural border vegetation provided a higher lacewing activity and aphid suppression than with annual sown floral buffer strips. We found that maintained natural border vegetation supported by VOC attractants provided lacewing populations that controlled aphids up to 100 m into the cropping area. Without VOC attractants we recorded lacewing activity up to 50 m from the border into the cropping area if natural border vegetation was available, and up to 25 m if no border vegetation existed. The overall results demonstrated the feasibility of this CBC approach under Norwegian farming conditions leading to the successful adoption of this CBC-strategy by the farming community.
Conservation biological control (CBC) is a promising tool for ecological intensification in agriculture (Tscharntke et al., 2005, Tscharntke et al., 2012; Shields et al., 2019). CBC aims to establish resilient natural enemy populations for pest management and reduced use of pesticides by supporting native biodiversity in agroecosystems (Eilenberg et al., 2001). CBC is an auspicious way to reduce the dependence on pesticides and consequentially reduces the risk to human health, loss of biodiversity and ecosystem services, while still maintaining high yields (Geiger et al., 2010; Aune et al., 2018; Sánchez-Bayo and Wyckhuys, 2019). However, despite rapidly expanding research on CBC over the last decades, the implementation in applied pest management is still very limited (Griffith et al., 2008; González-Chang et al., 2020). CBC measures are often effective in supporting natural enemy populations, but suppression of the target pests cannot be guaranteed (Begg et al., 2017; Cloyd, 2020). Other key factors for the poor integration of CBC into existing farmers practices today is the lack of understanding of the logistically and economically feasibility of CBC approaches, but also communication and perceptual barriers and thus, the acceptance by growers (Cullen et al., 2008; Naranjo et al., 2015; Shields et al., 2019; Johnson et al., 2020).
In Norway only 3% of the country’s territory can be used for food production. From this area is around 30% suitable for cereal production. Barley, wheat and oats are most important cereals in Norway. In 2022, there was around 280,880 hectares used for cereal production and half from this area used for barley production, with a yield of around 5.7 tons of barley per hectare (Statistics Norway, 2024). Based on climatic and topographic conditions, most of the barley is produced in the counties Viken, Innlandet and Trøndelag. Norwegian cereal production is characterized by relatively small fields, producing cereal for the domestic market. Aphids are the main pest insects in cereals in Norway, mainly the two species Sitobion avenae Fabricius and Rhopalosiphum padi L. (Hemiptera: Aphididae). They can cause serious yield losses in some years, while they are of minor importance in other years. It is unpredictable when these aphids become a serious problem for cereals production and when they can be tolerated. A smart and sustainable control of S. avenae and R. padi is therefore a challenge.
In a previous study in East Norway (Viken, 2015–2018), we developed a CBC strategy combining a volatile organic compound (VOC) attractant for lacewings, annual floral buffer strips, and insect hotels for increased lacewing overwintering survival to support aphid biological control in barley. This CBC strategy resulted in a significant increase in lacewing activity and significant aphid reduction below the economic damage threshold in the fields over 4 years (Thöming and Knudsen, 2021). However, the practical feasibility of this CBC strategy and adoption by farmers was not addressed.
Parallel to the previous study, a survey was conducted to understand how Norwegian cereal farmers implement integrated pest management (IPM) (Kvakkestad et al., 2021). The use of IPM measures have become mandatory in Norway for professional plant growers in 2015 and the farmers must follow the eight general principles of IPM since then (EU Directive, 2009). For IPM of pest insect in cereal production in Norway this includes mainly the following measures; the use of preventive measures, mainly crop rotation (P1), monitoring of pest insects (P2), the use of economic thresholds to decide whether or when to apply plant-protection measures (P3), choosing non-chemical plant protection measures (P4), the use of pesticides that have the fewest side effects for the environment and human health (P5), reduce the use of pesticides as much as possible, e.g. due to reduced application frequency and dose (P6), and checking the success of the applied pest control measures (P8). Most Norwegian farmers involved in the study understood the IPM beyond economic aspects and were motivated to implement it (Kvakkestad et al., 2021). As an outcome of both studies (Kvakkestad et al., 2021; Thöming and Knudsen, 2021), barriers and incentives to the adoption of the CBC strategy as an IPM-tool in Norwegian cereal production have been evaluated from the farmer’s perspective. The following issues were identified to be crucial for implementing a CBC strategy in Norway by farmers; 1) the CBC using natural field edges had to be better in controlling aphids than sown annual floral buffer strips combined with insect hotels. 2) The active range of the CBC strategy using VOCs and flowering field edges to control the aphids in the cropping area had to be sufficient. 3) The effects of flower border vegetation and VOC attractants must be clear. The concerns by the farmers have been addressed in some earlier studies. It is known that semi-natural habitats are part of most agricultural landscapes and have potential to support ecosystem services, such as biological pest control (Holland et al., 2017). Several studies stated that restoration of native, perennial plants back into farm systems gives a better effect in biodiversity and with it in biological control than growing of annual flower strips, as the continuity of trophic (food) and structural (shelter) resources existing in native habitats affect natural enemy conservation to a greater extent than annual flower strips can (Norderhaug et al., 2000; Auestad et al., 2008; Hamre et al., 2010; Iuliano and Gratton, 2020; Tooker et al., 2020). In addition, farmers can take advantage of these already existing natural vegetation habitats for biological pest management with little input and without losing land needed for production (Fiedler et al., 2008). However, we still see that natural border vegetation used as food and shelter resources support beneficial insect populations but can also reduce natural enemy movement from the border vegetation into the crop (Holland et al., 2008; Begg et al., 2017; Gontijo, 2019). In this context, VOCs like herbivore induced plant volatiles (HIPVs) play an important role (Blassioli-Moraes et al., 2022). HIPVs are a part of the inducible defense system of plants which are released from damaged plants to the environment. These VOCs communicate that the plant is attacked by herbivores to surrounding tissue, neighboring plants, and natural enemies from a distance (Heil and Ton, 2008; Braasch and Kaplan, 2012; Ninkovic et al., 2016). Natural enemies use these signals to find good places for feeding or for their offspring. HIPVs can also be used to recruit natural enemies and guide them to the right place at the right time from the border vegetation into the crop for effective pest control. Recent research indicates that VOCs like HIPVs can help to increase the functional response of natural enemies in CBC approaches (Khan et al., 2008; Ayelo et al., 2021; Kansman et al., 2023). Semiochemicals and habitat manipulation combined in a CBC strategy have shown synergy effects to enhance biological control in different crops. However, practical relevance and implementation in applied pest management of such CBC approaches is still very limited (Simpson et al., 2011; Jaworski et al., 2019; Pålsson et al., 2022).
The feedback from the farmers (Kvakkestad et al., 2021; Thöming and Knudsen, 2021) and the lack of practical field studies were the bases for the 5-years field study presented here, where a specific VOC lacewing attractant was tested on-farm in cereal production in East Norway. We compared natural flowering border vegetation with annual sown floral buffer strips with and without using VOC attractants for lacewings regarding impact in biological aphid control, the active range of the CBC strategy in the cropping area and the feasibility of this approach for farmers.
This is a study on the implementation and practicability of a previously developed CBC strategy, performed in close cooperation with Norwegian farmers. The aim is to deliver a proof of concept on the feasibility of a CBC approach based on a VOC attractant for lacewings combined with maintained natural border vegetation to promote the acceptance of this approach by growers. In addition, we aimed to show that VOCs can be used as a tool in CBC to deliver a more robust and unerring pest control. We tested the hypothesis of whether a CBC strategy including a VOC attractant for lacewings combined with maintained natural border vegetation can establish a robust lacewing population in an agroecosystem to reduce aphid infestations in cereals, grown in crop rotation according to farmers practices in Norway. In addition, we were interested in the dispersion distance lacewings cover from the border area of a field into the crop depending on availability of natural border vegetation and VOCs applied in the field.
The field trials were conducted in close cooperation with farmers over a period of 5 years (2019–2023) in commercial cereals fields at three different locations in Viken county in East Norway (Hobøl, Våler, Vestby). Based on farmers practices spring barley (Hordeum vulgare L.) was grown in three of the five years in all three locations (2019, 2020, 2023). Whereas, in 2021 and 2022 other crops were grown depending on the respective crop rotation (oats Avena sativa L.; oilseed rape Brassica napus L.; broad beans Vicia faba L.; pea Lathyrus oleraceus Lam.). Thus, data analyzed and presented in this study are from the years 2019, 2020 and 2023 only, while the natural border vegetation of the three locations were maintained all five years (2019–2023). In this field study, we assessed the population level of common green lacewings Chrysoperla carnea s.l. Stephens (Neuroptera: Chrysopidae), and the two most common aphid species in cereals in Norway, S. avenae and R. padi while testing a CBC strategy including the two tools ATTRACT and HABITAT to increase aphid biological control by lacewings.
As lacewing attractant, a paste containing the three components methyl salicylate, phenylacetaldehyde, and acetic acid at a ratio of 1:1:1 (100 mg of each compound) was applied. Previous studies have shown that these components attract lacewings, increase their egg laying and are able to improve aphid biological control (Tóth et al., 2009; Koczor et al., 2015; Pålsson et al., 2019; Thöming and Knudsen, 2021). The odor paste was produced by ISCA Technologies (ISCA Technologies Inc., Riverside, CA, USA) and Bio-Innovate AB (Lund, Sweden). The product is based on a biologically inert biodegradable wax-water emulsion releasing paste loaded with the above-described blend at a total load of active ingredients of 300 mg/mL. A single release point for this product consisted of 0.75 mL droplet applied with a plastic syringe on one leaf in the field. There was one application date for the VOC attractant paste at the beginning of the field experiments, respectively. Data on the release properties of the paste were described by Pålsson et al. (2019). The paste was stored at –18°C until used.
To provide food and shelter resources for beneficial insects we used natural field edges adjoining the fields used in this study. The border vegetation was grass dominated, interspersed with local flowering herbs. As a maintenance measure to improve local flowering herbs as food source, the natural border vegetation was cut once in the season (approx. end of July), but not too short (plants were allowed to stand with approx. 15 cm height). The cut plant material was allowed to dry on the ground for some days, to ensure seed dispersal and was removed thereafter. These measures were based on traditional management of semi-natural meadows in Norway (Norderhaug et al., 2000). In addition, all field edges used in this study contained structures that provided shelter resources for insects such as bushes, trees, dry wood, piles of stones or rocks.
Annual floral buffer strips (field experiment 1 only) were sown at field edges around end of April each year at a density of 10 g seeds/m2, with 30% flower and 70% grass seeds. The flower seed mix was based on a commercial product containing 33 different species (Blomstereng, Nelson Garden AS, Bergen, Norway; 10% flower seeds) as described in a previous study (Thöming and Knudsen, 2021). The floral buffer strips and the crop were sown at the same time. Under the experimental conditions in our study, the applied flower seed mix provided flowering vegetation as food and habitat resources for insects in all 5 years (2019–2023) from approx. beginning of June until the first frost in autumn (September/October), when the lacewing adults started to search for overwintering shelter. The maintained natural border vegetation provided flowering vegetation for a longer period of time, starting already in the beginning of May.
In our 5-year field study (2019–2023), we established each year three experimental fields of approximately 0,5 ha at the three different locations in East Norway, respectively (= nine experimental fields in total). At each location one field were established with annual floral buffer strip, one field with natural border vegetation which has been regularly maintained each year as described above, and as a control, one field with no border vegetation at all. Two plots were established in each experimental field, one plot with VOC treatment and one without. Thus, six treatments were tested in field plots of 30 x 30 m, respectively: (1) as a control, with neither border vegetation nor VOCs; (2) without border vegetation but with VOCs; (3) with natural border vegetation, but without VOCs; (4) with natural border vegetation and with VOCs; (5) with annual flower strips, but without VOCs; (6) with annual flower strips and with VOCs. The isolation distance was at least 50 m between the plots with and without VOC treatment, and at least 500 m between the fields with different types of border vegetation. The fields with border vegetation (treatments 3–6) were bordered by a 0.7–1 m vegetation strip on one side. In the plots treated with VOCs (treatment 2, 4, 6) paste-droplets (0.75 mL/plant) were applied with a plastic syringe on leaves in the upper third of the barley plants with 4 droplets/100 m2 (= one droplet on one plant per plot). No insecticides were used during the 5-year period in any of the locations. All stages of lacewings including eggs, larvae, pupae and adults, as well as nymphs and adults of aphids were counted and recorded on five plants closest to five registration points. The five registration points were evenly distributed along a diagonal line across each plot. Since the occurrence of C. carnea s.l., as well as the suppression of aphids were evaluated in the same field experiment, we did not trap insects nor remove counted individuals. Instead, we marked counted lacewing eggs (drawing a dot close to the egg) to avoid double counting. Considering that other stages of lacewings (i.e., lacewing larvae) are mobile, double counting may have occurred. Data on the insects were recorded in June/July (calendar week 26 with BBCH 39–59; 27, BBCH 55–65; 28, BBCH 60–69; 31, BBCH 75–89) in 2019, 2020, 2023. The economic damage threshold for aphids in cereal crops in Norway were checked at the four registration dates (number of aphids per plant, registering 100 plants on a 100 m-diagonal through the field). This is for S. avenae more than 3 aphids per plant at BBCH 51–59 and ten or more aphids per plant at BBCH 67–69, and for R. padi ten or more aphids per plant at BBCH 51–59.
In 2023, we tested in an additional field experiment the active range of the VOCs and the patterns of insect movement in response of the lacewing attractant, with and without natural border vegetation.
Four experimental fields of approximately 1 ha (including experimental area of 30 m x 100 m) each were established at the three different locations in East Norway (= twelve experimental fields in total). At each location two fields were established with natural border vegetation which had been regularly maintained since 2019 as described above, and as a control, two fields with no border vegetation, one field of each type with VOC treatment and one without (Figure 1). Four treatments were tested: (1) control, neither border vegetation nor VOCs; (2) no border vegetation, but with VOCs; (3) with natural border vegetation, but no VOCs; (4) with natural border vegetation and with VOCs. The isolation distance was at least 500 m between the fields with the four treatments. The fields with border vegetation (treatments 3 and 4) were bordered by 30 m length and approx. 1 m width natural vegetation on one side of the field. In the fields treated with VOCs (treatment 2 and 4) a row of five paste-droplets (0.75 mL/plant) were applied in the field as described above. The first droplet was applied 1 m off the field border and the other four droplets were applied following a straight line into the field, at intervals of 25 m (1 m, 25 m, 50 m, 75 m, 100 m) (Figure 1). No insecticides were used in the experiments. Lacewings (eggs, larvae, pupae, adults) and aphids (nymphs, adults) were recorded in a registration grid in the experimental area of 30 m x 100 m as shown in Figure 1. The insect counts were performed as described above in June/July (calendar week 26, 27, 28, 31) in 2023.
Figure 1. Schematic representation of the field set up in Experiment 2 used to test the dispersion distance lacewings cover from the border area of a field into the crop depending on availability of natural border vegetation and VOCs applied in the field, and the resulting impact on biological control of aphids by green lacewings. Four treatments were tested: neither border vegetation nor VOCs (1) −BV − VOC; no border vegetation, but with VOCs (2) −BV + VOC; with natural border vegetation, but no VOCs (3) +BV − VOC; with natural border vegetation and with VOCs (4) +BC + VOC. The green bars represent the natural border vegetation, the red frame represents the experimental area in the fields, the yellow dots represent the registration points, and the blue crosses represent the release points for the VOCs.
To assess the practicability of our CBC strategy and the acceptance by growers we performed the field experiments described above on-farm and in close cooperation with the farmers, who contributed with space in their commercial fields. In addition, a focus group of 12 farmers (5 from Hobøl, 4 from Våler, and 3 from Vestby; Viken county, East Norway) was established to explore how farmers experience the use of our tested CBC strategy in their cereal production. The focus group consisted of farmers which were interested in the CBC strategy and used it in the study period but had no experience with CBC from before. The farmers were advised on how to use the CBC strategy and supervised during the study period. In addition, the focus group regularly met, at least three times yearly (2019–2023), to discuss their experiences. These meetings lasted on average 2–3 h. They included field visits, open debates with all participants as well as individually discussions with each participant and qualitative interviews. The dialogues of the focus group meetings were transcribed, and qualitative content analysis was used to locate descriptions of how farmers rate the feasibility of the CBC approach in their production, and how they accept it as part of their IPM strategy.
For Experiment 1, the average number of lacewings (eggs and larvae combined) and aphids (S. avenae and R. padi, nymphs and adults, combined) per plot and each registration date, respectively, were analyzed using generalized linear mixed models (GLMMs) with a Poisson or a negative binomial distribution and a log link (PROC GLIMMIX, SAS 9.4). The numbers counted for each of the two groups were used as the response variables, with treatment, registration date, year, interaction between treatment and year as fixed factors and location as a random effect. In addition, the average numbers counted for each of the two groups per plot were used as the response variables, with treatment, year, interaction between treatment and year as fixed factors and location as a random effect. The choice of the most fitting distribution was based on a test for overdispersion.
For Experiment 2, the average number of lacewings and aphids were analyzed using GLMMs with an exponential distribution and a ln link (PROC GLIMMIX, SAS 9.4). The total average of the two groups registered in the five rows of the registration grid (1, 25, 50, 75, 100 m distance from the field border, Figure 1) were used as the response variables, with treatment, registration date (day), distance from the field border (distance), interaction between treatment and registration date as fixed factors and location as a random effect. In both experiments, after establishing the significance of the fixed factors, Tukey’s tests were performed for pairwise comparisons between levels of each factor when necessary. A significance level of α = 0.05 was selected in all analyses.
Over the 5-year experimental period, the general occurrence of insects in our field studies in East Norway varied as observed in previous studies (Thöming and Knudsen, 2021). Incidences of insects in general were at a similar level in 2019 and 2023 but were quite low in 2020 throughout the region and for most insect species. This trend was reflected in our aphid and lacewing counting results (Figures 2–5). In all, 8,715, 1,447, and 7,120 aphids and 142, 61, and 121 lacewings (on average per location) were recorded in the barley fields in June/July of the years 2019, 2020 and 2023, respectively.
Figure 2. Average number of aphids and lacewings (± SE) per registration in experiment 1 in the experimental years 2019 (first year of border vegetation maintenance), 2020 (second year of border vegetation maintenance) and 2023 (fifth year of border vegetation maintenance) in cereal fields with sown annual flower strips, maintained natural border vegetation or without border vegetation, with and without the use of volatiles attracting lacewings, respectively. Different letters above bars indicate significant differences between treatments in 2019 (uppercase), 2020 (lowercase), and 2023 (lowercase bold italic), (A) for aphids and (B) for lacewings (GLMM, Tukey’s test p < 0.05).
Figure 3. Mean number of aphids and lacewings per 5 plants (± SE) in cereal fields of experiment 1 with sown annual flower strips, natural border vegetation or without border vegetation, with and without the use of VOC attracting lacewings, respectively, registered the day before volatile application (day 0), one (day 7), two (day 14) and five weeks (day 35) after volatile application in the first experimental years 2019 (A), 2020 (B) and 2023 (C). Different letters above bars indicate significant differences between treatments for each registration date for aphids (uppercase), and lacewings (lowercase) (GLMM, Tukey’s test p < 0.05).
Figure 4. Mean number of aphids (A) and lacewings (B) per 5 plants (± SE) in cereal fields of experiment 2 registered at 5 different distances (1, 25, 50, 75, 100 m) from the border area of the field into the crop for the four treatments (1) neither border vegetation nor VOCs; (2) no border vegetation, but with VOCs; (3) with natural border vegetation after four years of maintenance, but no VOCs; (4) with natural border vegetation after four years of maintenance and with VOCs. Registrations were performed the day before volatile application (day 0), one (day 7), two (day 14) and five weeks (day 35) after volatile application in 2023. Different letters above bars indicate significant differences between treatments for each registration date for aphids (uppercase), and lacewings (lowercase) (GLMM, Tukey’s test p < 0.05).
Figure 5. Graphic representation of the spatial distribution of aphids and lacewings in cereal fields of experiment 2 related to the distance to the field border (with natural border vegetation or without border vegetation) and related to the use of VOCs attracting lacewings (±VOC), respectively. The white bars at the y-axis of the single plots represent the position of the natural border vegetation, the white dots in the plots represent the position of the five volatile droplets applied in the field. The insects were registered the day before volatile application (A; day 0), one (B; day 7), two (C; day 14) and five weeks (D; day 35) after volatile application in the fifth experimental year 2023.
The lowest number of aphids was recorded in the plots with VOC treatment and with natural border vegetation (natural border vegetation with VOC; Figure 2A, Table 1). In 2023, i.e. in the fifth year of maintained natural border vegetation, the aphid occurrence in the plots with natural border vegetation and VOC treatment was at a very low level during all 4 registration dates (day 0, 7, 14, 35), whereas, a higher aphid occurrence was observed in fields with annual flower strips and without border vegetation (with and without VOCs; Figure 3C, Table 1). In general, overall years, we observed an increased number of aphids in the plots without volatiles compared to plots with VOC treatment, for all types of border vegetation, although this difference was not always significant (Figure 3, Table 1).
The opposite effect was found regarding the lacewings. The significantly highest number of lacewings were recorded in the plots with VOC treatment and with natural border vegetation (natural border vegetation with VOC; Figure 2B, Table 1), especially in 2023 (Figure 3C, Table 1). Higher numbers of lacewings were recorded in plots with VOC treatment compared to the plots without VOCs, for all types of border vegetation (Figures 2B, 3, Table 1). Without VOC treatment the lacewing occurrence was similar in all fields, independent of the type of border vegetation (with annual flowers strips, natural border vegetation and without border vegetation). There was a significant increase in lacewing occurrence in plots with VOC treatment in fields with natural border vegetation, compared to the VOC treated plots in fields with annual flower strips and without border vegetation (Figures 2B, 3C, Table 1).
In this field experiment we recorded lacewings up to 100 m into the crop in fields with maintained natural border vegetation supported by volatile attractants at all registration dates (Figures 4, 5). Without VOCs, we recorded lacewing activity up to 50 m from the field border into the field if natural border vegetation was available, and up to 25 m if no border vegetation existed (Figures 4, 5). We found in general a decrease in number of insects (lacewings and aphids) with increasing distance between field border and registration point. Most insects were recorded 1 m off the field border and fewest 100 m off the field border, independent of type of border vegetation and VOC treatment (Figure 4, Table 1). Lowest numbers of aphids (Figure 4A) and highest number of lacewings (Figure 4B) were recorded in fields with natural border vegetation supported by VOC treatment. Whereas highest numbers of aphids (Figure 4A) and lowest numbers of lacewings (Figure 4B) were found in fields with neither border vegetation nor VOC treatment.
The spatial distribution of aphids and lacewings in cereal fields related to the distance to the field border (with natural border vegetation or without border vegetation) and related to the use of VOCs (with or without VOCs), depending on time (registration day 0, 7, 14, 35) is shown in Figure 5. The color pattern highlights the occurrence of aphids in fields with neither border vegetation nor VOCs up to 100 m into the field (Figures 5C, D). In contrast, lowest numbers of aphids were recorded in fields with both, natural border vegetation and VOC treatment (Figures 5C, D). Likewise, the color patterns illustrates the dispersion of lacewings, up to 100 m into the field and clearly guided by the volatile droplets, in fields with VOC treatment, compared to very low numbers of lacewings in fields without border vegetation and without VOC treatment (Figure 5).
The focus group meetings and qualitative content analysis provided information about how the Norwegian cereal farmers at our locations experienced and accepted the tested CBC approach. The general picture from the focus group was that at the beginning of the experimental period (2019) most of the farmers were concerned about the efficiency of the CBC approach in pest management (1), yield risks (2), the practical feasibility and workload of the CBC approach (3), and the economic feasibility (4) (Table 2A). Whereas, at the end of the experimental period (2023) most of the farmers were not concerned any longer about these four issues (Table 2B). As a result, all twelve farmers were willing to use the CBC approach based on VOC-attractants for natural enemies of pest insects and natural border vegetation as a part of IPM also in future.
Table 2. Responded perceptions about the four most arguable issues for the farmers (N = 12) based on qualitative interviews in the focus group meetings in the beginning of the experimental period (A) and at the end of the experimental period (B).
During the focus group meetings, we registered that the farmers were motivated to learn how CBC works and how they can use our approach, to integrate it into their particularly IPM practices permanently. The maintenance of the natural border vegetation as tool to support biological pest control, was not a new concept for the farmers. Most of the farmers knew the concept from their fathers and grandfathers already. However, the approach to use volatiles to support the natural border vegetation tool and to achieve a more robust and unerring biological pest control, was new for the farmers and needed to be proofed and explained broadly. The farmers needed an elaborated supervision on the use of the CBC strategy. In the beginning of the experimental period (2019), most arguable issues for the farmers were the efficiency of the approach in pest management (1), yield risks (2), the practical feasibility and workload of the CBC approach (3), and the economic feasibility (4) (Table 2A, Figure 6A). At the end of the experimental period (2023) all farmers of our focus group (12 from 12 farmers) were confident that our CBC approach can work as reliable IPM tool, also on their farm. The efficiency in pest management was assessed by the farmers as equivalent to previous used methods (= use of chemical pesticides) (Figure 6B). The farmers did not see an important difference in yield after using our CBC approach as IPM tool in their farming practice, compared to previous used IPM measures (= use of chemical pesticides) (Figure 6B). In the first two years (2019/2020) the workload was slightly bigger compared to previous used IPM measures. The higher workload was mainly based on restoration of the natural border vegetation, which is more work intense in the first years, and the learning on how to use VOCs in pest management and how to integrate the CBC approach in farmers practices. In the following years (2021–2023), most of the farmers (11 from 12 farmers) stated that the workload was not higher than with previous IPM measures (Figure 6B). The comparison of the costs (time, consumables, equipment) and yield using our CBC approach with costs/yield using previous IPM measures showed equivalent cost effectiveness over the five years (Figure 6B). The farmers (12 from 12 farmers) saw a considerable advantage in our CBC approach compared to previous used pest management tools as it reduced pesticide use to a large extent. In addition, farmers could take advantage of already existing natural border vegetation for biological pest management with little input and without losing land needed for crop production. There was no need to establish flower strips on arable land (Figure 6C). At the end of the experimental period, all farmers involved in the focus groups agreed on the economic and practicable feasibility of the CBC approach (Figure 6D).
Figure 6. Summary of the results gained from the focus group meetings and qualitative content analysis regarding Norwegian cereal farmers experience and acceptance of the tested CBC approach. The figure is showing farmers main concerns in the beginning of the study (A), farmers conclusions after four years on-farm testing (B), the main benefits of the tested CBC approach the farmers saw (C), and farmers decision regarding their acceptance of the tested CBC approach at the end of the study (D).
Our results showed that it is possible to avoid aphid infestations in spring barley by combining different natural mechanisms as pest management tools in a deliberately compiled CBC strategy. Our first field experiment indicated that lacewing attracting volatiles combined with regular maintained natural field vegetation provided a higher lacewing activity and aphid suppression than annual sown floral buffer strips. The use of VOCs always increased the lacewing activity and aphid suppression, independent from the type of border vegetation used in the experiments. This is showing synergistic effects of the two pest control tools used, VOCs attracting adult green lacewings to the fields and stimulating their egg laying (ATTRACT), combined with maintained natural border vegetation to enhance food and habitat resources for adult lacewings throughout the field season (HABITAT). By using these two tools, natural enemies were recruited and guided at the right time and to the right place from the border vegetation into the crop to better control the target pest. The results of the second field experiment showed that maintained natural border vegetation supported by volatile attractants for lacewings provided biological control of aphids up to 100 m into the field. Whereas, without the use of VOC attractants, we recorded lacewing activity up to 50 m from the field border into the field if natural border vegetation was available, and up to 25 m if no border vegetation existed. This clearly indicates how VOCs can help to increase the functional response of natural enemies in CBC approaches based on vegetation elements in the agricultural landscape to enhance food and habitat resources of the beneficials. In addition, our on-farm study provided an indication on the logistically and economically feasibility of this CBC approach, as well as the adoption by growers.
This ATTRACT–HABITAT strategy seems to work equally effective in biological aphid control as a previous tested CBC strategy, using the three tools ATTRACT (same lacewing attractant and egg laying stimulator as used here), FOOD – annual floral buffer strips established at field edges to enhance resources for adult lacewings, and SHELTER – insect hotels equipped with the ternary attractant as hibernation shelters to support the overwintering survival of adult lacewings (Thöming and Knudsen, 2021). In the present 5-year field study, we observed that a simpler CBC strategy comprising ATTRACT and HABITAT only (instead of ATTRACT–FOOD–SHELTER) facilitated a significant increase in biological control of aphids by establishing a resilient lacewing population in the agroecosystem. As previously shown (Thöming and Knudsen, 2021), it is possible to keep the aphid infestations below the damage threshold set for aphids in cereal crops in Norway (Andersen, 2003; Heggen et al., 2005) at all three locations in East Norway and during all years of the field experiments. In contrast, the aphid infestations in the control plots were above the economic damage threshold at all locations in 2019 and 2023. In commercial barley production this implies that it would be necessary to apply pesticides to avoid economic loss due to aphid infestation. These results show that by using two CBC tools combined in one strategy, the aphid pest population can be kept below the damage threshold and the use of synthetic pesticides can be avoided. At the same time, by using the ATTRACT–HABITAT strategy the farmers had the advantage of using already existing natural vegetation habitats for biological pest management instead of establishing flower strips and insect hotels on their production areas using the ATTRACT–FOOD–SHELTER strategy (Thöming and Knudsen, 2021). That means for the farmers less input (work and expenses), without losing land needed for production (Fiedler et al., 2008). In addition, several studies have stated that restoration and management of native vegetation in a farm system, such as natural border vegetation, delivers a better effect in biological pest control than growing of annual flower strips, as the continuity of food and shelter resources existing in native habitats affect natural enemy conservation to a greater extent than annual flower strips can (Norderhaug et al., 2000; Auestad et al., 2008; Hamre et al., 2010; Iuliano and Gratton, 2020; Tooker et al., 2020). Our results indicate the same. In our first field experiment in the current study, VOCs in combination with regular maintained natural field vegetation provided a higher lacewing activity and aphid suppression than combined with annual sown floral buffer strips. The continuity of food and shelter resources was given by native vegetation (e.g. flowering and non-flowering herbes, bushes, trees) and other structures in the border areas of our fields such as dead wood, piles of stones and rocks. The regular maintenance measures we applied in the natural border vegetation clearly improved the flowering herbs from year to year, as it is known for a long time from traditional management of semi-natural meadows in Norway (Norderhaug et al., 2000). In the natural border vegetation, we recorded flowering plants from beginning of May until the first frost in autumn. In contrast, our grown floral buffer strips provided the flowering vegetation not until June. Food and shelter resources provided by bushes, trees, dead wood, stones, and rocks in the natural border areas were not available in the artificial floral buffer strips. In autumn the floral buffer strips, and with it all remaining food and shelter resources, were removed. These limitations of resources for natural enemies given by grown floral buffer strips compared to natural border vegetation might have a negative impact on the population development of natural enemies and their services for agriculture (Iuliano and Gratton, 2020; Tooker et al., 2020).
As in previous studies (Tóth et al., 2009; Koczor et al., 2010, Koczor et al., 2015; Jones et al., 2016; Pålsson et al., 2019, Pålsson et al., 2022), we strengthened the existing lacewing population in the agricultural landscapes by using a VOC attractant and oviposition stimulator consisting of common floral volatiles and herbivore-induced plant volatiles (HIPVs); methyl salicylate, acetic acid, and phenylacetaldehyde. These three plant volatiles are naturally used by plants for their defense. Methyl salicylate is emitted by plants when they are attacked by pests to recruit natural enemies of those pests (Molleman et al., 1997; Gadino et al., 2012). Acetic acid is used to signal that nectar is present as food source (Landolt and Alfaro, 2000; Tóth et al., 2009; Knight et al., 2014). Phenylacetaldehyde operates both as food and as a SOS signal (Tóth et al., 2006; El-Sayed et al., 2016; Jones et al., 2016). As formulation in the present 5-year field study we used a biologically inert biodegradable wax-water emulsion releasing paste loaded with the above-described volatile blend (Teixeira et al., 2010; Pålsson et al., 2019). This formulation has the benefit that it is easier to apply in a cereal field compared to commercially available dispensers used in previous studies (Pålsson et al., 2019; Thöming and Knudsen, 2021). These dispensers were developed for fruit production and are consisting of a cotton wick placed into a sealed polyethylene bag through which volatiles were slowly released. To facilitate its use in the crop, these dispensers are delivered with a pre-attached plastic strip. If applied in cereal fields, the dispensers must be stapled to a holding stick which has to be removed after the season and costs extra work time (Pålsson et al., 2019).
Another advantage of the odor paste compared to dispensers is, that with the odor paste it is easy to fine-tune the application of volatiles (by both dose and lure density) in a crop as required. This is important to adjust the volatile release to the respective agricultural landscape with its specific entomological and vegetation resources. In the present 5-year field study we used a reduced dose and lure density (0.75 mL per droplet and 4 droplets per 100 m2) compared to our previous 4-year field study (1 mL per droplet and 24 droplets per 100 m2; Thöming and Knudsen, 2021) to avoid possible negative side effects on non-target beneficials. Findings from Thöming and Knudsen (2021) indicated that non-target beneficials (ladybirds, hoverflies, parasitoids), may be affected by the application of the lacewing VOC attractant. The study found evidence that applying the lacewing attractant boosted the green lacewings at the cost of ladybirds, hoverflies, and parasitized aphid mummies, even though flower strips were used as alternative resources for prey, nectar, pollen, and habitat (Thöming and Knudsen, 2021). As known from previous studies (Pålsson et al., 2019), lacewings are arriving earlier than ladybirds, hoverflies, and parasitoids in the fields. By using VOCs we are strengthening the lacewings in addition, making them the predominant beneficial insect species in the crops. The combination of early arrival and predominance of lacewings make the lacewings highly competitive with other beneficials. It is known that the type of early predation supported by the VOCs is crucial for avoiding aphid infestations that surpass the economic damage thresholds (Bianchi et al., 2006; Zhang and Swinton, 2009; Dedryer et al., 2010; Porcel et al., 2018). However, applying VOCs while prey to feed natural enemies in an agricultural system are lacking may lead to starvation with unpredictable consequences for the agroecosystem. Furthermore, promoting a particular species of beneficials in an agricultural system over time may influence the population dynamics of other species in the second, third and/or even fourth tropic level in the ecosystem (Turlings and Erb, 2018). As discussed previously (Thöming and Knudsen, 2021), another important issue to consider is the specificity of the VOCs used. The same compound or blend might have attractant or repellent effects on insects other than the target species (Pettersson et al., 1994; James and Price, 2004; Bengtsson et al., 2006; Snoeren et al., 2010; Rodriguez-Saona et al., 2011). To reduce or even avoid undesired effects on non-target species in the agricultural ecosystem and at the same time support and stabilize the effects on the target species, it is smart to combine VOCs with allocation of food and shelter resources, e.g. by providing natural border vegetation (Simpson et al., 2011; Hatt et al., 2018). We have not studied effects of our VOCs on non-target beneficials in the present study and it remains to be investigated whether there will be effects on non-target species in the long-term using our ATTRACT-HABITAT strategy. However, we used a reduced dose and lure density compared to previous field studies (Thöming and Knudsen, 2021) and we found that maintained natural border vegetation supported by VOCs still resulted in lacewing activity and biological control of aphids up to 100 m into the field. Without the volatile attractant we recorded lacewing activity up to 50 m from the field border into the field only if natural border vegetation was available, and up to 25 m if no border vegetation existed. It remains to be investigated how long into the field we will be able to find robust biological control of aphids by using our ATTRACT-HABITAT strategy. Studies on patterns of insect movement in response of semiochemicals, particularly plant volatiles, and the active range of such semiochemicals in pest control, particularly used to support beneficial insects are still rare (Larsson, 2016; Smart et al., 2017; Conchou et al., 2019; Ninkovic et al., 2020) and need to be investigated.
Finally, as an important result of this on-farm study, we demonstrated the logistically and economically feasibility of this CBC approach. Here, the involvement of the farmers in implementation and development of the field experiments, the establishment of a focus group with supervised testing of the CBC strategy by the farmers themselves on their own farms, and a good communication with the growers over the years accounted substantially for the farmers’ adoption of the tested CBC-strategy. Contrary to many studies (Begg et al., 2017; Cloyd, 2020; Johnson et al., 2020), the farmers involved in our study experienced over time that CBC measures can be effective in both supporting natural enemy populations and suppressing pests, and at the same time be logistically and economically feasible. As a result, the farmers were accepting this new CBC approach as part of their IPM concept, and they were willing to implement it into applied pest management on their farm permanently. An action which is still very rare in agriculture today (Griffith et al., 2008; González-Chang et al., 2020). This is just one case study for a relatively small group of farmers, but it clearly shows that a good demonstration of the CBC concept, close collaboration with the farmers and a continuous communication can be an important key for acceptance and implementation of CBC into existing farming practices. There are several scientific approaches how to perform economic assessments of CBC to show economics and adoptions of CBC (e.g. Cullen et al., 2008; Naranjo et al., 2015), which remains to be investigated for our CBC strategy. However, to start an adoption process of CBC in farming practices, we chose our approach to combine on-farm experiments in very close cooperation with the farmers, and work with a local focus group with simple qualitative content analysis, deliberately. This practical CBC approach is easily communicated, and the farmers involved in our study can serve as demonstration cases for other farmers to follow. Especially for farmers who are motivated to change their farming practices towards CBC, starting implementing CBC approaches within small focus groups might be a first step to new agro-ecological practices in the future.
The raw data supporting the conclusions of this article will be made available by the authors, without undue reservation.
The manuscript presents research on animals that do not require ethical approval for their study.
GT: Conceptualization, Data curation, Formal analysis, Funding acquisition, Investigation, Methodology, Project administration, Resources, Software, Supervision, Validation, Visualization, Writing – original draft, Writing – review & editing.
The author(s) declare financial support was received for the research, authorship, and/or publication of this article. This research was funded by basic funding of the Norwegian Institute of Bioeconomy Research (Research Council of Norway, Contract No. 342631/L10).
We are very grateful to the farmers who hosted our field experiments and to the farmers who participated in the focus group work. We thank Torfinn Torp for statistical advice, and the colleagues at NIBIO and the Norwegian Agricultural Extension Service (NLR) for valuable discussion throughout the project. Special thanks got to the local farmer association “Farge i felt” for supporting the work on conservation biological control in East Norway since 2017 by spreading the message among the farmers, for their helping hands during experiments and meetings and constructive discussions.
The authors declare that the research was conducted in the absence of any commercial or financial relationships that could be construed as a potential conflict of interest.
All claims expressed in this article are solely those of the authors and do not necessarily represent those of their affiliated organizations, or those of the publisher, the editors and the reviewers. Any product that may be evaluated in this article, or claim that may be made by its manufacturer, is not guaranteed or endorsed by the publisher.
Auestad I., Rydgren K., Økland R. H. (2008). Scale-dependence of vegetation-environment relationships in semi-natural grassland. J. Vegetation Sci. 19, 139–148. doi: 10.3170/2007-8-18344
Aune S., Bryn A., Hovstad K. A. (2018). Loss of semi-natural grassland in a boreal landscape: impact of agricultural intensification and abandonment. J. Land Use Sci. 13, 375–390. doi: 10.1080/1747423X.2018.1539779
Ayelo P. M., Pirk C. W. W., Yusuf A. A., Chailleux A., Mohamed S. A., Deletre E. (2021). Exploring the kairomone-based foraging behavior of natural enemies to enhance biological control: a review. Front. Ecol. Evol. 9, 641974. doi: 10.3389/fevo.2021.641974
Begg G., Cook S. M., Dye R., Ferrante M., Franck P., Lavigne C., et al. (2017). A functional overview of conservation biological control. Crop Prot. 97, 145–158. doi: 10.1016/j.cropro.2016.11.008
Bengtsson M., Jaastad G., Knudsen G., Kobro S., Backman A. C., Pettersson E., et al. (2006). Plant volatiles mediate attraction to host and non-host plant in apple fruit moth, Argyresthia conjugella. Entomologia Experimentalis Applicata 118, 77–85. doi: 10.1111/j.1570-7458.2006.00359.x
Bianchi F. J. J. A., Booij C. J. H., Tscharntke T. (2006). Sustainable pest regulation in agricultural landscapes: a review on landscape composition, biodiversity and natural pest control. Proc. R. Soc. B Biol. Sci. 273, 1715–1727. doi: 10.1098/rspb.2006.3530
Blassioli-Moraes M. C., Venzon M., Silveira L. C. P., Gontijo L. M., Togni P. H. B., Sujii E. R., et al. (2022). Companion and smart plants: scientific background to promote conservation biological control. Neotropical Entomology 51, 171–187. doi: 10.1007/s13744-021-00939-2
Braasch J., Kaplan I. (2012). Over what distance are plant volatiles bioactive? Estimating the spatial dimension of attraction in an arthropod assemblage. Entomologia Experimentalis Applicata 145, 115–123. doi: 10.1111/j.1570-7458.2012.01317.x
Cloyd R. A. (2020). How effective is conservation biological control in regulating insect pest populations in organic crop production systems? Insects 11, 744. doi: 10.3390/insects11110744
Conchou L., Lucas P., Meslin C., Proffit M., Staudt M., Renou M. (2019). Insect odorscapes: from plant volatiles to natural olfactory scenes. Front. Physiol. 10, 972. doi: 10.3389/fphys.2019.00972
Cullen R., Warner K. D., Johnsson M., Wratten S. D. (2008). Economics and adoption of conservation biological control. Biol. Control 45, 272–280. doi: 10.1016/j.biocontrol.2008.01.016
Dedryer C.-A., Le Ralec A., Fabre F. (2010). The conflicting relationships between aphids and men: A review of aphid damage and control strategies. C. R. Biologies 333, 539–553. doi: 10.1016/j.crvi.2010.03.009
Eilenberg J., Hajek A., Lomer C. (2001). Suggestions for unifying the terminology in biological control. BioControl 46, 387–400. doi: 10.1023/A:1014193329979
El-Sayed A. M., Knight A. L., Byers J. A., Judd G. J. R., Suckling D. M. (2016). Caterpillar-induced plant volatiles attract conspecific adults in nature. Sci. Rep. 6, 1–14. doi: 10.1038/srep37555
EU (2009). “Directive 2009/128/EC of the European Parliament and the Council of 21 October 2009 establishing a framework for community action to achieve the sustainable use of pesticides,” in Official Journal of the European Union L 309. Available online at: https://eur-lex.europa.eu/eli/dir/2009/128/oj
Fiedler A. K., Landis D. A., Wratten S. D. (2008). Maximizing ecosystem services from conservation biological control: the role of habitat management. Biol. Control 45, 254–271. doi: 10.1016/j.biocontrol.2007.12.009
Gadino A. N., Walton V. M., Lee J. C. (2012). Evaluation of methyl salicylate lures on populations of Typhlodromus pyri (Acari: Phytoseiidae) and other natural enemies in western Oregon vineyards. Biol. Control 63, 48–55. doi: 10.1016/j.biocontrol.2012.06.006
Geiger H., Bengtsson J., Berendse F., Weisser W. W., Emmerson M., Morales M. B., et al. (2010). Persistent negative effects of pesticides on biodiversity and biological control on European farmland. Basic Appl. Ecol. 11, 97–105. doi: 10.1016/j.baae.2009.12.001
Gontijo L. M. (2019). Engineering natural enemy shelters to enhance conservation biological control in field crops. Biol. control 130, 155–163. doi: 10.1016/j.biocontrol.2018.10.014
González-Chang M., Wratten S., Shields M. W., Costanza R., Dainese M., Gurr G. M., et al. (2020). Understanding the pathways from biodiversity to agro-ecological outcomes: A new, interactive approach. Agriculture Ecosyst. Environ. 301, 107053. doi: 10.1016/j.agee.2020.107053
Griffith G. J. K., Holland J. M., Bailey A., Thomas M. B. (2008). Efficacy and economics of shelter habitats for conservation biological control. Biol. Control 45, 200–209. doi: 10.1016/j.biocontrol.2007.09.002
Hamre L. N., Halvorsen R., Edvardsen A., Rydgren K. (2010). Plant species richness, composition and habitat specificity in a Norwegian agricultural landscape. Agriculture Ecosyst. Environ. 138, 189–196. doi: 10.1016/j.agee.2010.04.018
Hatt S., Boeraeve F., Artru S., Dufrêne M., Francis F. (2018). Spatial diversification of agroecosystems to enhance biological control and other regulating services: An agroecological perspective. Sci. Total Environ. 621, 600–611. doi: 10.1016/j.scitotenv.2017.11.296
Heggen H. E., Hofsvang T., Ørpen H. M. (2005). Plantevern i korn – integrert bekjempelse (Oslo, Norway: Landbruksforlaget), 97pp.
Heil M., Ton J. (2008). Long-distance signaling in plant defense. Trends Plant Sci. 13, 264–272. doi: 10.1016/j.tplants.2008.03.005
Holland J. M., Douma J. C., Crowley L., James L., Kor L., Stevenson D. R. W., et al. (2017). Semi-natural habitats support biological control, pollination and soil conservation in Europe. A review. Agron. Sustain. Dev. 37, 31. doi: 10.1007/s13593-017-0434-x
Holland J. M., Oaten H., Southway S., Moreby S. (2008). The effectiveness of field margin enhancement for cereal aphid control by different natural enemy guilds. Biol. control 47, 71–76. doi: 10.1016/j.biocontrol.2008.06.010
Iuliano B., Gratton C. (2020). Temporal resource (dis)continuity for conservation biological control: from field to landscape scales. Front. Sustain. Food Syst. 4, 127. doi: 10.3389/fsufs.2020.00127
James D. G., Price T. S. (2004). Field-testing of methyl salicylate for recruitment and retention of beneficial insects in grapes and hops. J. Chem. Ecol. 30, 1613–1628. doi: 10.1023/B:JOEC.0000042072.18151.6f
Jaworski C. C., Xiao D., Xu Q., Ramirez-Romero R., Guo X., Wang S., et al. (2019). Varying the spatial arrangement of synthetic herbivore-induced plant volatiles and companion plants to improve conservation biological control. J. Appl. Ecol. 56, 1176–1188. doi: 10.1111/1365-2664.13353
Johnson A. C., Liu J., Reynolds O., Furlong M. J., Mo J., Rizvi S., et al. (2020). Conservation biological control research is strongly uneven across trophic levels and economic measures. Pest Manage. Sci. 77, 2165–2169. doi: 10.1002/ps.6162
Jones V. P., Horton D. R., Mils N. J., Unruh T. R., Baker C. C., Melton T. D., et al. (2016). Evaluating plant volatiles for monitoring natural enemies in apple, pear and walnut orchards. Biol. Control 102, 53–65. doi: 10.1016/j.biocontrol.2015.03.009
Kansman J. T., Jaramillo J. L., Ali J. G., Hermann S. L. (2023). Chemical ecology in conservation biocontrol: new perspectives for plant protection. Trends Plant Sci. 28, 1166–1177. doi: 10.1016/j.tplants.2023.05.001
Khan Z., James D. G., Midega C. A. O., Pickett J. (2008). Chemical ecology and conservation biological control. Biol. Control 45, 2010–2224. doi: 10.1016/j.biocontrol.2007.11.009
Knight A. L., Hilton R., Basoalto E., Stelinski L. L. (2014). Use of glacial acetic acid to enhance bisexual monitoring of tortricid pests with kairomone lures in pome fruits. Environ. Entomology 43, 1628–1640. doi: 10.1603/EN14153
Koczor S., Knudsen G. K., Hatleli L., Szentkirályi F., Tóth M. (2015). Manipulation of oviposition and overwintering site choice of common green lacewings with synthetic lure (Neuroptera: Chrysopidae). J. Appl. Entomology 139, 201–206. doi: 10.1111/jen.12150
Koczor S., Szentkirályi F., Birkett M., Pickett J. A., Voigt E., Tóth M. (2010). Attraction of Chrysoperla carnea complex and Chrysopa spp. lacewings (Neuroptera: Chrysopidae) to aphid sex pheromone components and a synthetic blend of floral compounds in Hungary. Pest Manage. Sci. 66, 1347–1379. doi: 10.1002/ps.v66:12
Kvakkestad V., Steiro Å.L., Vatn A. (2021). Pesticide policies and farm behavior: the introduction of regulations for integrated pest management. Agriculture 11, 828. doi: 10.3390/agriculture11090828
Landolt P. J., Alfaro J. F. (2000). Trapping Lacanobia subjuncta, Xestia c-nigrum, and Mamestra configurata (Lepidoptera: Noctuidae) with acetic acid and 3-Methyl-1-butanol in controlled release dispensers. Environ. Entomology 30, 656–662. doi: 10.1603/0046-225X-30.4.656
Larsson M. C. (2016). Pheromones and other semiochemicals for monitoring rare and endangered species. J. Chem. Ecol. 42, 853–866. doi: 10.1007/s10886-016-0753-4
Molleman F., Drukker B., Blommers L. (1997). “A trap for monitoring pear psylla predators using dispensers with the synomone methysalicyate,” in Proceedings of the section Experimental and Applied Entomology of the Netherlands Entomological Society (N.E.V.), Vol. 8. 177–182.
Naranjo S. E., Ellsworth P. C., Frisvold G. B. (2015). Economic value of biological control in integrated pest management of managed plant systems. Annu. Rev. Entomology 60, 621–645. doi: 10.1146/annurev-ento-010814-021005
Ninkovic V., Markovic D., Dahlin M. (2016). Decoding neighbor volatiles in preparation for future competition and implications for tritrophic interactions. Perspect. Plant Ecology Evol. Systematics 23, 11–17. doi: 10.1016/j.ppees.2016.09.005
Ninkovic V., Markovic D., Rensing M. (2020). Plant volatiles as cues and signals in plant communication. Plant Cell Environ. 44, 1030–1043. doi: 10.1111/pce.13910
Norderhaug A., Ihse M., Pedersen O. (2000). Biotope patterns and abundance of meadow plant species in a Norwegian rural landscape. Landscape Ecol. 15, 201–218. doi: 10.1023/A:1008141400166
Pålsson J., Porcel M., Dekker T., Tasin M. (2022). Attract, reward and disrupt: responses of pests and natural enemies to combinations of habitat manipulation and semiochemicals in organic apple. J. Pest Sci. 95, 619–631. doi: 10.1007/s10340-021-01410-2
Pålsson J., Thöming G., Silva R., Porcel M., Dekker T., Tasin M. (2019). Recruiting on the spot: a biodegradable formulation for lacewings to trigger biological control of aphids. Insects 10, 6. doi: 10.3390/insects10010006
Pettersson J., Pickett J. A., Pye B. J., Quiroz A., Smart L. E., Wadhams L. J., et al. (1994). Winter host component reduces colonization by bird-cherry-oat aphid, Rhopalosiphum padi (L.) (Homoptera, Aphididae), and other aphid species in cereal fields. J. Chem. Ecol. 20, 2565–2574. doi: 10.1007/BF02036192
Porcel M., Andersson G. K. S., Pålsson J., Tasin M. (2018). Organic management in apple orchards: Higher impacts on biological control than on pollination. J. Appl. Ecol. 55, 2779–2789. doi: 10.1111/1365-2664.13247
Rodriguez-Saona C., Kaplan I., Braasch J., Chinnasamy D., Williams L. (2011). Field responses of predaceous arthropods to methyl salicylate: a meta-analysis and case study in cranberries. Biol. Control 59, 294–303. doi: 10.1016/j.biocontrol.2011.06.017
Sánchez-Bayo F., Wyckhuys K. A. G. (2019). Worldwide decline of entomofauna: a review of its drivers. Biol. Conserv. 232, 8–27. doi: 10.1016/j.biocon.2019.01.020
Shields M. W., Johnson A. C., Pandey S., Cullen R., González-Chang M., Wratten S. D., et al. (2019). History, current situation and challenges for conservation biological control. Biol. Control 131, 25–35. doi: 10.1016/j.biocontrol.2018.12.010
Simpson M., Gurr G. M., Simmons A. T., Wratten S. D., James D. G., Leeson G., et al. (2011). Attract and reward: Combining chemical ecology and habitat manipulation to enhance biological control in field crops. J. Appl. Ecol. 48, 580–590. doi: 10.1111/j.1365-2664.2010.01946.x
Smart L. E., Aradottir G. I., Bruce T. J. A. (2017). “Role of semiochemicals in integrated pest management,” in Integrated Pest Management: Current Concepts and Ecological Perspectives (Elsevier Science, San Diego), 93–109.
Snoeren T. A., Mumm R., Poelman E. H., Yang Y., Pichersky E., Dicke M. (2010). The herbivore-induced plant volatile methyl salicylate negatively affects attraction of the parasitoid Diadegma semiclausum. J. Chem. Ecol. 36, 479–489. doi: 10.1007/s10886-010-9787-1
Statistics Norway (2024). Available online at: https://www.ssb.no/en (Accessed 28.06.2024).
Teixeira L. A. F., Mason K., Mafra-Neto A., Isaacs R. (2010). Mechanically-applied wax matrix (SPLAT-GBM) for mating disruption of grape berry moth (Lepidoptera: Tortricidae). Crop Prot. 29, 1514–1520. doi: 10.1016/j.cropro.2010.08.013
Thöming G., Knudsen G. K. (2021). Semiochemicals and habitat manipulation to support green lacewing activity to reduce aphid infestations in agroecosystems. Basic Appl. Ecol. 51, 30–42. doi: 10.1016/j.baae.2021.01.004
Tooker J. F., O’Neal M. E., Rodriguez-Saona C. (2020). Balancing disturbance and conservation in agroecosystems to improve biological control. Annu. Rev. Entomology 65, 81–100. doi: 10.1146/annurev-ento-011019-025143
Tóth M., Bozsik A., Szentkirályi F., Letardi A., Tabilio M. R., Verdinelli M., et al. (2006). Phenylacetaldehyde: a chemical attractant for common green lacewings (Chrysoperla carnea s.l., Neurotera: Chrysopidae). Eur. J. Entomology 103, 267–271. doi: 10.14411/eje.2006.033
Tóth M., Szentkirályi F., Vuts J., Letardi A., Tabilio M. R., Jaastad G., et al. (2009). Optimization of a phenylacetaldehyde-based attractant for common green lacewings (Chrysoperla carnea s.l.). J. Chem. Ecol. 35, 449–458. doi: 10.1007/s10886-009-9614-8
Tscharntke T., Clough Y., Wanger T. C., Jackson L., Motzke I., Perfecto I., et al. (2012). Global food security, biodiversity conservation and the future of agricultural intensification. Biol. Conserv. 151, 53–59. doi: 10.1016/j.biocon.2012.01.068
Tscharntke T., Klein A. M., Kruess A., Steffan-Dewenter I., Thies C. (2005). Landscape perspectives on agricultural intensification and biodiversity – ecosystem service management. Ecol. Lett. 8, 857–874. doi: 10.1111/j.1461-0248.2005.00782.x
Turlings T. C. J., Erb M. (2018). Tritrophic interactions mediated by herbivore-induced plant volatiles: mechanisms, ecological relevance, and application potential. Annu. Rev. Entomology 63, 433–452. doi: 10.1146/annurev-ento-020117-043507
Keywords: semiochemicals, green lacewings, semi-natural habitats, ecological intensification, ecosystem services, feasibility of CBC, growers’ acceptance
Citation: Thöming G (2024) Conservation biological control on-farm, using VOCs combined with maintained natural border vegetation for a more unerring and resilient biological control of aphids in cereals. Front. Hortic. 3:1440410. doi: 10.3389/fhort.2024.1440410
Received: 29 May 2024; Accepted: 19 July 2024;
Published: 30 August 2024.
Edited by:
Jörg-Peter Schnitzler, Helmholtz Association of German Research Centers (HZ), GermanyCopyright © 2024 Thöming. This is an open-access article distributed under the terms of the Creative Commons Attribution License (CC BY). The use, distribution or reproduction in other forums is permitted, provided the original author(s) and the copyright owner(s) are credited and that the original publication in this journal is cited, in accordance with accepted academic practice. No use, distribution or reproduction is permitted which does not comply with these terms.
*Correspondence: Gunda Thöming, Z3VuZGEudGhvZW1pbmdAbmliaW8ubm8=
Disclaimer: All claims expressed in this article are solely those of the authors and do not necessarily represent those of their affiliated organizations, or those of the publisher, the editors and the reviewers. Any product that may be evaluated in this article or claim that may be made by its manufacturer is not guaranteed or endorsed by the publisher.
Research integrity at Frontiers
Learn more about the work of our research integrity team to safeguard the quality of each article we publish.