- 1Centre for Econics and Ecosystem Management, Eberswalde University for Sustainable Development, Eberswalde, Germany
- 2Biosphere Reserves Institute, Eberswalde University for Sustainable Development, Eberswalde, Germany
Intact forests contribute to the ecosystem functionality of landscapes by storing and sequestering carbon, buffering and cooling the microclimate, and providing a range of related ecosystem functions. Forest fragmentation not only poses a threat to many organisms but also reduces the resistance and resilience of the ecosystem, which is especially relevant to the ongoing climate crisis. The effects of recent extreme heat years on forests in Germany have not been studied in detail for the influence of fragmentation. We investigate the relation of forest fragmentation with temperature and vitality in Germany per ecoregion at the canopy level using satellite imagery at 1-km and 30-m resolution. We compiled and correlated forest maps for connectivity based on Thiessen polygons, canopy temperatures on the hottest days based on land surface temperature, and forest vitality based on the maximum normalized difference vegetation index per growing season. We differentiated between ecoregions and main forest types. In 2022, larger intact tree-covered areas that are less fragmented have relatively low temperatures on hot days and higher overall vitality. Nearly 98% of the almost 1.95 million forest fragments at 30-m resolution in Germany are smaller than 1 km2, which cover nearly 30% of the total forest area. To counteract the forest crisis, forest and landscape management should aim to reduce fragmentation and maintain tree biomass and forest cover in the landscape. Increasing the size of continuous forest fragments contributes to ecosystem-based adaptation to climate change.
1. Introduction
Land use and infrastructure increasingly fragment naturally continuous ecosystems into several smaller fragments more or less isolated from each other (Riitters, 2007; Ibisch et al., 2016). While large unfragmented forest landscapes are considered some of the most vital ecosystems in the world that provide crucial benefits to numerous species (Minnemeyer and Potapov, 2017), fragmentation is a key driver for the loss of ecosystem integrity (Rogers et al., 2022). Large intact forests are the greatest sinks of atmospheric carbon and store disproportionately higher amounts of carbon than fragmented forests, making them an important natural solution in any climate change mitigation and adaptation solution (Potapov et al., 2017; Moomaw et al., 2019). In addition, risks of diminished biodiversity and local extinctions are higher with increasing fragmentation of intact forest landscapes (Betts et al., 2017). Forest fragmentation results in the expansion of forest edges, exposing the forest fragments to higher anthropogenic disturbances (Vieilledent et al., 2018). Higher fragmented and more isolated forest fragments tend to advance changes in local climatic conditions, leading to drier, hotter, and increasingly volatile microclimate (Laurance et al., 2002; Briant et al., 2010; Tuff et al., 2016). Nevertheless, small forest fragments and green canopy cover adjacent to the highly modified anthropogenic landscape also provide substantial benefits for regulating the microclimate (Aalto et al., 2022). However, such effects are more pronounced in large intact forests (Gohr et al., 2021).
The long-term history of forest fragmentation in Central Europe has led to a mosaicked landscape that consists of agricultural lands with scattered fragments of temperate forests. In Central Europe, nearly 40% of the current forested area is located closer than 100-m from the forest edge including the largest continuous mountain forests (Estreguil et al., 2013). Penetration of drought stress and wind into a forest can be measured until several hundred meters from the forest edge, leading to alterations in the forest microclimate and an increase in tree mortality (Laurance et al., 2011). Forest microclimatic changes have been reported at tens to hundreds of meters from the forest edge (Harper et al., 2005; Tuff et al., 2016). This could possibly be one reason for the increased damage due to forest fires every year (Armenteras et al., 2013; Driscoll et al., 2021). The carbon loss that is associated with the edge effects caused by forest fragmentation is another recently recognized factor associated with fragmentation (Silva Junior et al., 2020).
This study refers to all the forest patches as forest fragments irrespective of their size. Smaller forest fragments are largely influenced by the effects of their surrounding edges, and only larger forest fragments with a substantial proportion of interior area can buffer from environmental and biotic changes associated with the edge. The forest edge effect is the outcome of many interacting environmental effects. There are both physio-chemical and biotic impacts from the surroundings on the forest ecosystem. Microclimatic and mesoclimatic impacts are of special relevance for forest vitality as heat and drought stresses have increased over the past decades and are expected to rise substantially in the near future (Jacob et al., 2018). Extreme heat events are more likely to occur with ongoing climate change and contribute to water stress and drought, especially for forest ecosystems (Fisher et al., 2017). In Germany, we speak of a forest crisis based on the severe forest damage in recent years due to droughts, heat waves, pests (especially the bark beetle outbreak in 2018), and mismanagement (Lindner et al., 2014; Schuldt et al., 2020; Blumröder et al., 2021; Ibisch, 2022; Thonfeld et al., 2022). Healthy forests can contribute to landscape cooling, especially on hot days and during heat waves (Gohr et al., 2021).
Although there is a wealth of knowledge on how fragmentation affects temperature and forest vitality in tropical forests (Taubert et al., 2018; Silva Junior et al., 2020), very little research has linked the patterns of fragmentation with temperature and forest vitality altogether in temperate forests, such as forests in Germany. The characteristic features of temperate and tropical forests are inherently different; hence, it is important to understand these linkages specific to temperate forests to develop region or biome-specific forest management strategies. Therefore, it is imperative to understand these effects in temperate forests also. This study aims to expand the current knowledge about the relationship between forest fragments, their sizes, and the associated variations in temperature and forest vitality in Germany. In particular, we addressed the following questions:
(1) Is forest fragmentation associated with spatial variations in landscape temperature and forest vitality?
(2) Are the thermal gradients and variations in forest vitality inside forest fragments influenced by the size and degree of isolation of the forest fragments?
The information obtained in this study provides input to both forest management and landscape planning striving for ecosystem resilience and an ecosystem-based adaptation to climate change. Existing monitoring apps such as the European Forest Condition Monitor (Buras et al., 2021) or the Waldmonitor (Welle et al., 2022) focus on vegetation vitality. Extending this monitoring with analyses of forest fragmentation can contribute to the understanding of forest vulnerability. This study provides evidence-based arguments for reducing forest fragmentation in intensively managed landscapes.
2. Materials and methods
We quantified the relation of forest fragmentation with land surface temperature and forest vitality in Germany per ecoregion at the canopy level using a four-step methodological approach: (i) compilation of annual national forest cover for the year 2022 as well as temperature and vegetation vitality data; (ii) preliminary treatment of all datasets, including standardization of spatial resolution and calculation of annual averages of the hottest days and the maximum vitality within the growing season; (iii) conversion of the forest dataset into a presence–absence forest cover map and calculation of the degree of fragmentation; and (iv) analysis of the relation between forest fragmentation, temperature, and forest vitality using zonal statistics and bivariate choropleth mapping per ecoregion and per forest type. Dataset preprocessing was performed in the code editor of the Google Earth Engine. Post-processing and visualizations were prepared with ArcGIS 10.7 and RStudio version 4.0.3.
2.1. Ecoregions of Germany
To account for the influence of regional characteristics, such as altitude and plant communities, we based our analysis on nine defined ecoregions in Germany (Olson et al., 2001; Bundesamt für Naturschutz [BfN], 2017; Figure 1). The justification of ecoregions as a conglomerate of similar geographical and biological characteristics in terms of the assemblage of species is considered proven (Smith J. R. et al., 2018). The influence of altitudinal effects is represented in the segmentation of the ecoregions in Germany. Hence, we consider the investigation of fragmentation patterns within the ecoregion borders appropriate. Since the two northernmost ecoregions in Germany, the Baltic Sea ecoregion and the North Sea ecoregion, exhibit little forest cover, they were excluded from the analysis.
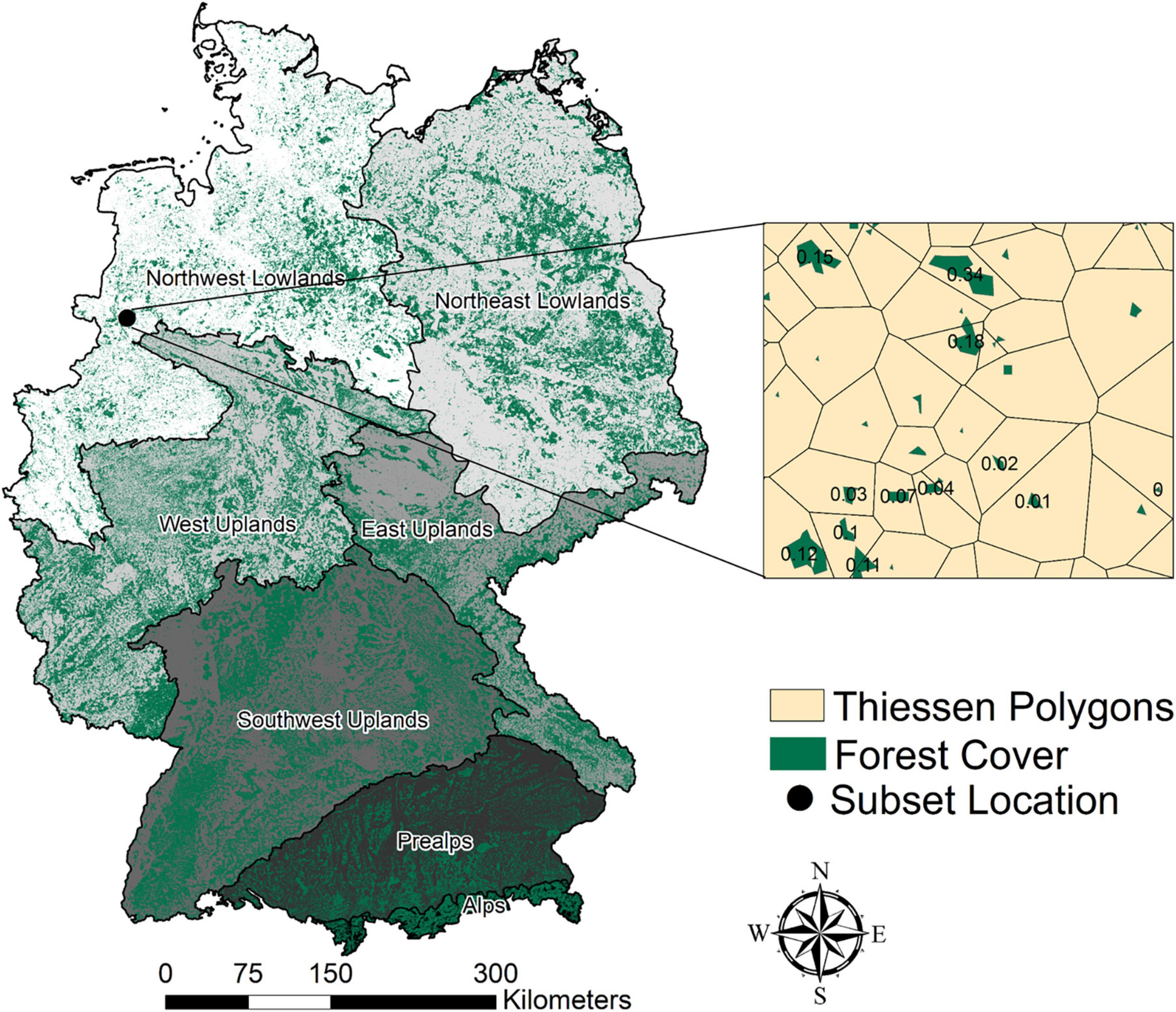
Figure 1. Map of Germany categorized by seven ecoregions (left, Bundesamt für Naturschutz [BfN], 2017). The Baltic Sea and North Sea with low forest cover shares are excluded from the original dataset. Forest cover (green) is based on the Global Forest Change dataset (Hansen et al., 2013) with forest areas of 2021 (defined per pixel as more than 50% forest coverage based on Hansen et al., 2013). An example region of a forest-covered area with corresponding Thiessen polygons and corresponding connectivity values in the range of 0–1 is shown on the right.
2.2. Forest cover and forest types
We created a forest cover map of Germany at a 30-m resolution for 2022 from the Global Tree Cover 2000 dataset, using a canopy cover threshold of 50%, by subtracting the tree cover loss from 2001 to 2021 (Hansen et al., 2013). We did not account for forest cover gain since no reliable data are available to date. The dataset is derived from the Landsat 7ETM + data, and forest cover is characterized as any vegetation taller than 5 m in height (Hansen et al., 2013). This threshold is based on the ability to distinguish tall woody vegetation in multispectral imagery, particularly those present in global-scale earth observation systems such as Landsat and MODIS (Hansen et al., 2010). Despite being criticized for not differentiating native and planted forests, these high-resolution maps are suitable for capturing biophysical features that depend on forest cover across the globe (Tropek et al., 2014). The resulting forest coverage of 27.9% for Germany in 2022 (Supplementary Table 1) differs from the official statistics. The official statistics, with a forest coverage for 2021 of 31.9% (Bundesministerium für Ernährung und Landwirtschaft [BMBL], 2022), include “legal forest” areas without actual tree cover and do not include some woodlands or tree coverage in urban areas. In comparison, in this study, only forest with measurable tree cover is considered and the relatively small forest patches on small islands and at the coastline of the Baltic Sea and the North Sea ecoregions are omitted, which leads to the smaller area of total forest coverage.
We created a forest type map of Germany at 30-m resolution from the latest available CORINE Land Cover product of 2018 at 100-m by first reclassifying the dataset into three forest type classes, namely, broad-leaved, coniferous, and other forests, and secondly reprojecting, resampling (to 30-m), and masking the dataset to the compiled forest cover map at 30-m resolution.
2.3. Forest fragmentation
Thiessen polygon connectivity (refer to, e.g., Ibisch et al., 2016; Mehdipour et al., 2019; Wu et al., 2019) of the forest fragments was used as a proxy to estimate forest fragmentation. The measure combines both fragment size and isolatedness from other forest fragments and is a unitless value ranging between 0 (high fragmentation) and 1 (low fragmentation). It is defined as the ratio between the size of a forest fragment and its surrounding Thiessen polygon. A Thiessen (or Voronoi) polygon describes the area around a sample point/area where any position taken from inside the polygon is closer to the sample point/area than to any of the other sample points/areas. The greater the value of Thiessen connectivity, the closer the neighboring forest fragments are and hence the lower the fragmentation.
The forest cover raster dataset of Germany for 2022 was converted to forest polygons using the centroid method, and one point per forest polygon was generated. Thereafter, Thiessen polygons were created, and Thiessen polygon connectivity values were computed per forest polygon (refer to Figure 1). Using these connectivity values based on the forest cover of Germany for 2022, we generated a forest fragmentation map at 30-m resolution.
2.4. Forest temperature
Extreme heat events are more likely to occur with ongoing climate change and contribute to water stress and drought, especially for forest ecosystems (Fisher et al., 2017). In the temperate biome, healthy forests can contribute to landscape cooling (Gohr et al., 2021). At the same time, the forests are heavily impacted by natural and anthropogenic disturbances. The radiative skin temperature of the land surface is the driving force in the exchange of long-wave radiation and turbulent heat fluxes at the surface–atmosphere interface (Li et al., 2013) and has presented valuable results in several sensible heat flux models (Zhan et al., 1996). Land surface temperature closely resembles air temperature trends when analyzing the effects of forest cover on local temperature even at different latitudinal zones (Li et al., 2016) as even with the heat effect of land surface during the day, there is a heat exchange between air and land surfaces (Jin and Dickinson, 2010; Mildrexler et al., 2011). Therefore, the land surface temperature dataset of Germany qualifies for the assessments of forest canopy temperature and is based on the MODIS Aqua MYD11A1 dataset at 1-km spatial resolution, captured every day at ∼1.30 pm Central European Time (CET). The temperature is measured from the radiation intensity in the infrared range (bands 31 and 32 with 10.8–12.3 μm) (Wan et al., 2015). To create a dataset with the per pixel mean temperature of the hottest days in 2022 from January until October, each day was signatured with the mean temperature of Germany and subsequently the resulting 124 days that exceed 30°C were selected (Supplementary Table 1, refer to Gohr et al., 2021). This way, we generated a map of the mean temperature on the hottest days in German forests for 2022, the warmest year since records began (Deutscher Wetterdienst [DWD], 2022; together with 2019, the summer of 2022 was the third warmest summer since 1881; Imbery et al., 2022). The same procedure was applied to generate means of the temperature on the hottest days for the years 2013–2022 in respect to ecoregions (Supplementary Figure 1).
2.5. Forest vitality
The normalized difference vegetation index (NDVI) is a measure of photosynthetic activity and is commonly used as a proxy for vegetation stress and water balance and therefore indicates vegetation vitality (Lambert et al., 2013; Chakraborty et al., 2022). Furthermore, the NDVI can serve as an explanatory variable for the effects of temperature changes on forest cover (Weng et al., 2004; Deng et al., 2018). The NDVI dataset of Germany is based on the MODIS Aqua MYD13A2 dataset of 16-day composites at 1-km spatial resolution. The composite is preprocessed from MODIS imagery using the near-infrared and visible spectra, is captured every day at ∼1.30 pm CET, and is completed by selecting the best pixel with low clouds, a low view angle, and the highest NDVI (Didan, 2015). For the growing season from May to September, this results in 10 images covering Germany in 2022. We created a map of forest vitality for Germany in 2022 using the maximum value of the growing season. The maximum value per pixel was selected to acknowledge different peaks of “greenness” for different vegetation for the tree-covered areas in 2022. The minimum value was not considered since the influence of fragmentation on healthy forests was the main objective. Minimum values have a diverse range of potential reasons such as outbreaks of pests and diseases, water stress, or other environmental factors. If only the maximum values are taken into account, the loss of vitality may be somewhat underestimated, but this error does not affect the regional assessment of spatial patterns (or temporal changes) substantially. The same procedure was applied to generate means of the greenest NDVI for the years 2013–2022 with respect to forest types and ecoregions (Supplementary Figures 2, 3).
2.6. Analysis
We reclassified the forest fragments into three different categories based on fragment size, that is, small (below 1 km2), medium (between 1 and 5 km2), and large (larger than 5 km2). We resampled the preprocessed temperature and NDVI datasets to 30-m spatial resolution. We extracted the raster datasets using the forest cover mask to prepare temperature, NDVI, Thiessen connectivity, and forest type data for forest cover in Germany. In order to study the spatial relationship between fragmentation, temperature, and forest vitality across different ecoregions in Germany, we prepared bivariate choropleth maps that spatially represent the variation in one variable with respect to another. In addition, we computed statistical information per forest fragment size per ecoregion for Thiessen connectivity, temperature, and vitality, respectively, using the zonal statistics tool in ArcMap 10.7. For a time series of changes in maximum vitality per growing season and changes in the mean temperature on the hottest days, we used a similar approach. For each year from 2013 to 2022 and each ecoregion, we extracted the mean and standard deviation of the temperature and vitality dataset in the corresponding forested areas (by subtracting forest loss of previous years).
3. Results
Conditions regarding fragmentation, temperature, and vitality in the tree-covered areas vary widely across Germany. Larger intact tree-covered areas that are less fragmented (e.g., parts of the Black Forest in the Southwest, Figure 2A) exhibit relatively low temperatures on hot days (Figure 2B) and higher vitality (Figure 2C). Populated areas with low forest coverage and higher fragmentation (e.g., parts of Northwest Germany) feature higher temperatures on hot days and lower vitality in the often very small forest fragments.
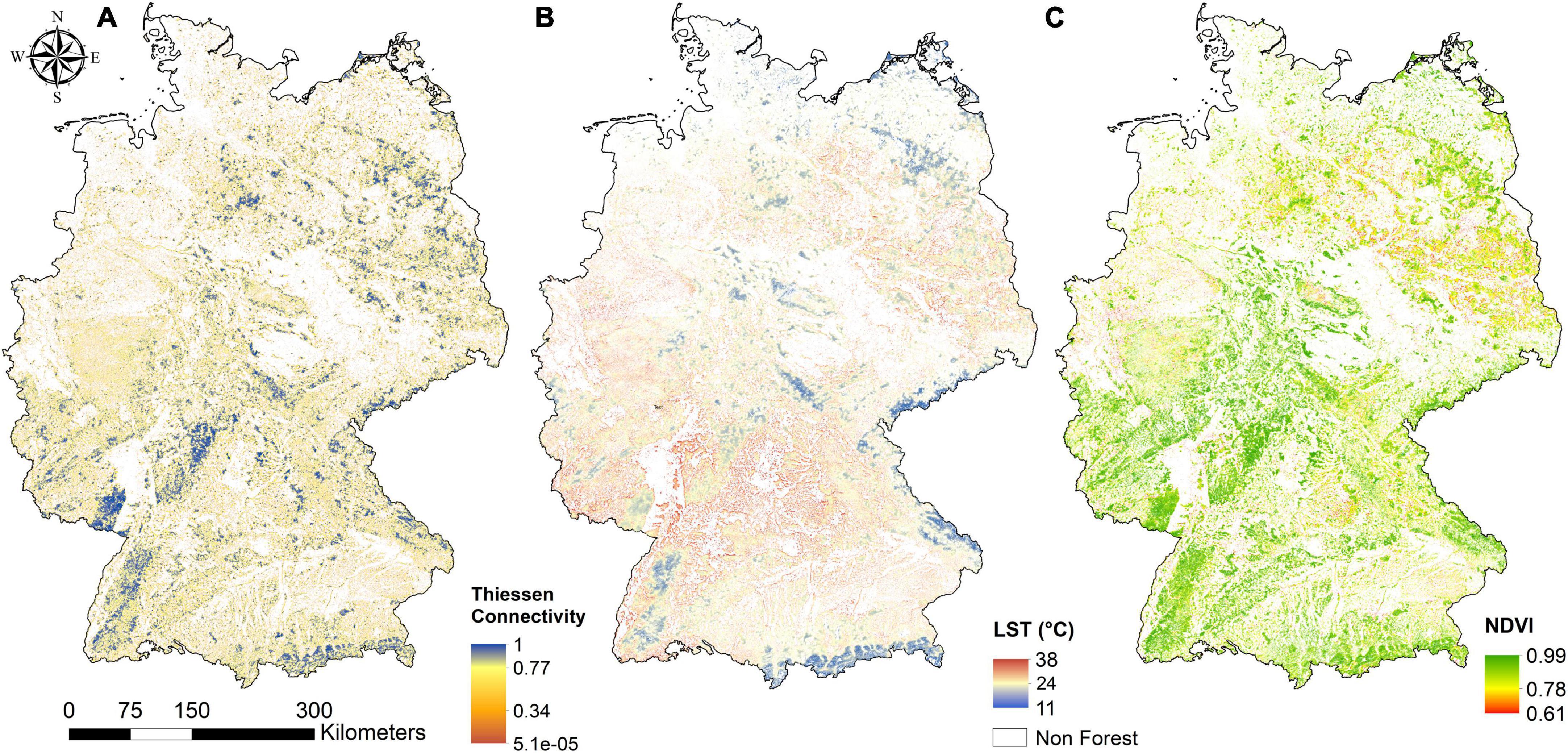
Figure 2. Characteristics of tree-covered areas in Germany 2022 across the terrestrial ecoregions in terms of (A) forest fragmentation (Thiessen connectivity), (B) land surface temperature [land surface temperature (LST); MODIS Aqua MYD11A1] indicated as the per pixel mean of hot days (≥30°C) in 2022, and (C) forest vitality (NDVI; MODIS Aqua MYD13A2) indicated as the maximum value of the growing season May–September.
3.1. Fragmentation, temperature, and vitality
The current forest distribution in Germany is the result of a long history of anthropogenic land use (Kaplan et al., 2009). The current forest cover in Germany accounts for approximately 9.9 million hectares with our study approach [official statistics state 11.4 million hectares (Bundesministerium für Ernährung und Landwirtschaft [BMBL], 2022)] and, at a 30-m resolution, is fragmented into nearly 1.95 million small forest fragments out of which 1.92 million are smaller than 1 km2 and only around 2,000 forest fragments are larger than 5 km2 with a maximum size of 3,800 km2.
With respect to distribution within different ecoregions, 68% (Alps) to 16% (Northwest Lowlands) of Germany is covered by forest (Supplementary Table 1). In terms of total area covered by forests, the West Uplands have the highest forest share of 21,960 km2. A higher value of Thiessen connectivity (closer to 1) indicates higher connectedness of forest fragments and hence less fragmentation. We analyzed the median of Thiessen connectivity values over all the forest fragments in different size classes, and the results indicate that large forest fragments have higher values of Thiessen connectivity in all the ecoregions ranging from 0.75 to 0.87 (Supplementary Table 2), while this range is only 0.58–0.70 in medium-sized forest fragments and 0.31–0.51 in the small-sized forest fragments. When comparing Thiessen connectivity across different ecoregions, forest fragments in all the size classes have the highest values in the Alps. Thiessen connectivity across different forest sizes in all the ecoregions is higher in the large-sized forest fragments than small-sized forests by a magnitude of 0.35–0.49, while this value for large- to medium- and medium- to small-sized fragment comparison lies in the range of 0.13–0.27. The median of Thiessen connectivity values across the ecoregions is high when grouping the values per fragment size.
The 2022 hottest day median temperatures of forests with large fragments were lower compared to small fragments in all the ecoregions by 1.29°C in the Alps to as large as 3.28°C in the Southwest Uplands (Supplementary Table 2). When comparing medium-sized forest fragments with large fragments within these two ecoregions, this difference is between 0.8 and 2.46°C. When comparing small- and medium-sized forest fragments, the difference in temperature ranges between 0.46 and 0.82°C. The highest variation in temperatures between the different-sized forest fragments was found in the Southwest Uplands. The effect of decreasing temperatures with higher elevation is depicted by the ecoregions since the elevation is one classification variable of ecoregions. This pattern was validated on a pixel basis throughout Germany (refer to Supplementary Figure 4). The total of hot days in 2022 varied per ecoregion. In the Alps, 24 days above 30°C were registered, in comparison with the Southwest Highlands 107 days above 30°C in 2022 (full list refer to Supplementary Table 1).
The median vitality values, compiled as medians of the maximum NDVI of the growing season in 2022 in Germany, are generally lower in smaller forest fragments and higher in larger forest fragments (Figure 3 and Supplementary Table 2). Since the areas are not discriminated by forest type (Supplementary Figures 3, 5, 6), a more generalized pattern can be observed. The highest vitality is found in the largest fragments of the Alps, the Western Uplands, and the Southwest Uplands. Vitality values below 0.8 are only observed in the smallest fragments of the Southwest Uplands, and the Northeast and Northwest Lowlands.
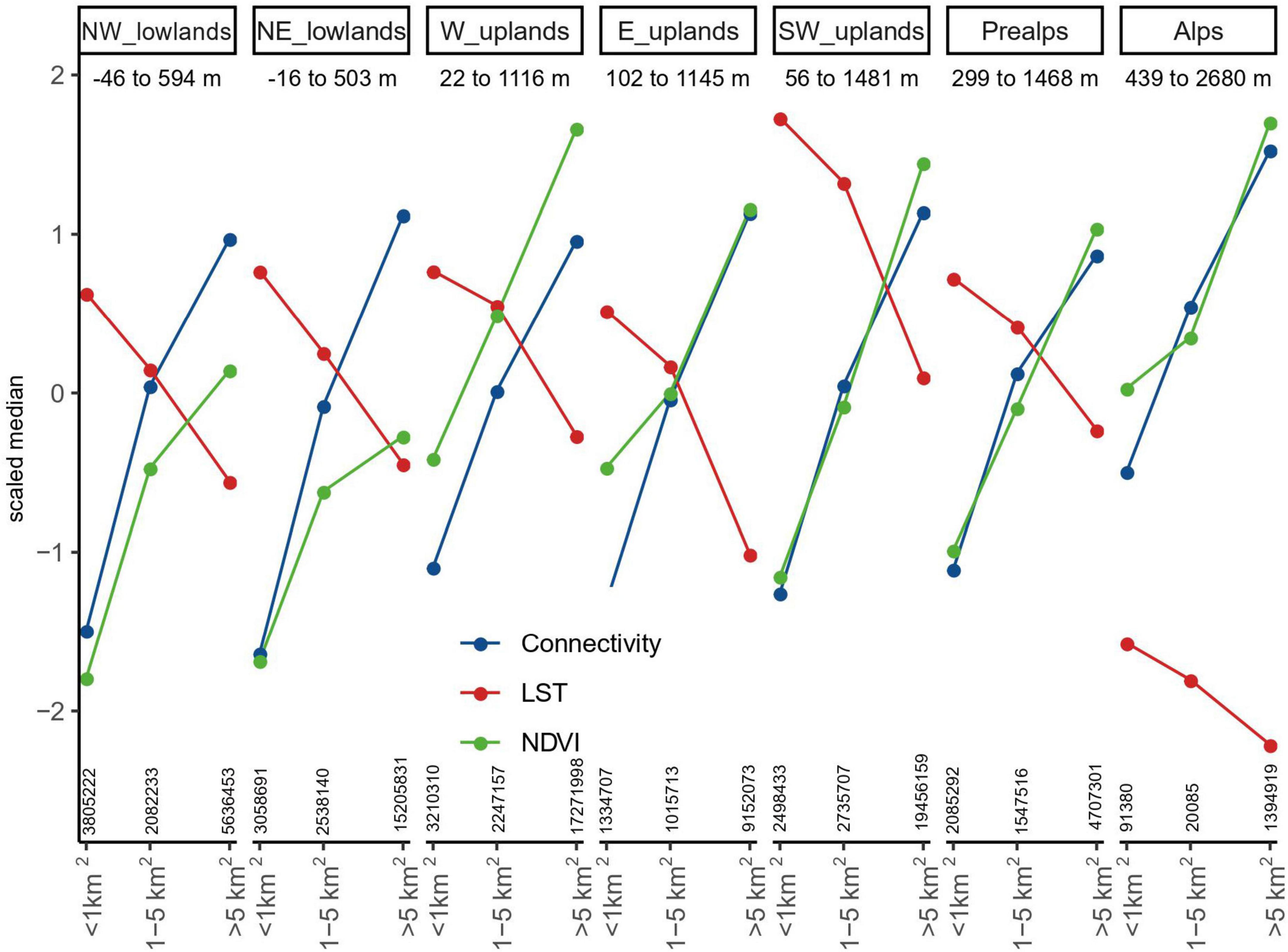
Figure 3. Scaled median (median per indicator for tree-covered areas in Germany 2022 divided by the standard deviation) per ecoregion with respect to different forest fragment sizes (<1 km2, 1–5 km2, and >5 km2). The indicators are Thiessen connectivity for forest-covered areas in Germany in 2022 (blue), land surface temperature [land surface temperature (LST); MODIS Aqua MYD11A1] based on the mean of the hottest days in 2022 (red), and the NDVI (MODIS Aqua MYD13A2) based on the maximum value of the growing season 2022 (green). Altitudinal ranges per ecoregion are depicted below the headings. The coverage of each fragment size group is depicted above the X-axis in km2.
Small forest fragments correspond to low connectivity, low vitality, and higher temperatures throughout all ecoregions and their respective altitudinal ranges when looking at the scaled medians across Germany in 2022 (Figure 3 and Supplementary Table 2). The uncertainty (under- or overestimation due to mixed pixel effect) of values in small fragments below 1 km is high since the temperature and forest vitality are originally captured at 1-km spatial resolution. Nevertheless, there are regional specifics for the temperature and forest vitality. The highest temperatures were observed in the small fragments of the Southwest Uplands, and the lowest temperatures could be found in the largest fragments of the Alps. In the small fragments of the Northeast and Northwest Lowlands, the lowest vitality was observed. The highest vitality was registered in the largest fragments of the Alps and the Western Uplands.
3.2. Spatial variations in fragmentation, temperature, and forest vitality
The bivariate choropleth maps between Thiessen connectivity and land surface temperature (Figure 4A) and Thiessen connectivity and forest vitality (NDVI) (Figure 4B) depict how the studied proxy indicators vary in geographical space with respect to each other. Cyan and magenta color tones on the map indicate a positive association between the two variables, while blue and red color tones indicate a negative association. For instance, the spatial variation between the high connectivity of tree-covered areas in Germany in 2022 and respective low temperatures can be observed in larger, better-connected forest areas (Figure 4A, blue areas). This relationship is not only true for large forest landscapes (e.g., part of the Black Forest or the Alps) but can also be found in areas with smaller forest fragments (e.g., Schorfheide in the Northeast Lowlands). Similar patterns can be found between the high connectivity of tree-covered areas and a high vitality (Figure 4B, magenta areas). However, the spatial pattern is more scattered. Especially the Northeast Lowlands show higher connectivity with a lower vitality signal. In the higher altitudes (e.g., the Alps), temperatures are low when the connectivity is high, but only some areas show a high vitality with high connectivity, while other areas show reduced connectivity and vitality (Figure 4B, Alps ecoregion magenta and green areas). When comparing the bivariate choropleth maps for broad-leaved and coniferous forest types (Supplementary Figures 5, 6), there is a clear indication that broad-leaved forests in Northern and Eastern Germany, representing the natural vegetation, have been severely degraded and fragmented in the past. Coniferous plantations seem to benefit from larger forest blocks and higher connectivity, having a higher NDVI such as the northern Black Forest in the Southwest of Germany.
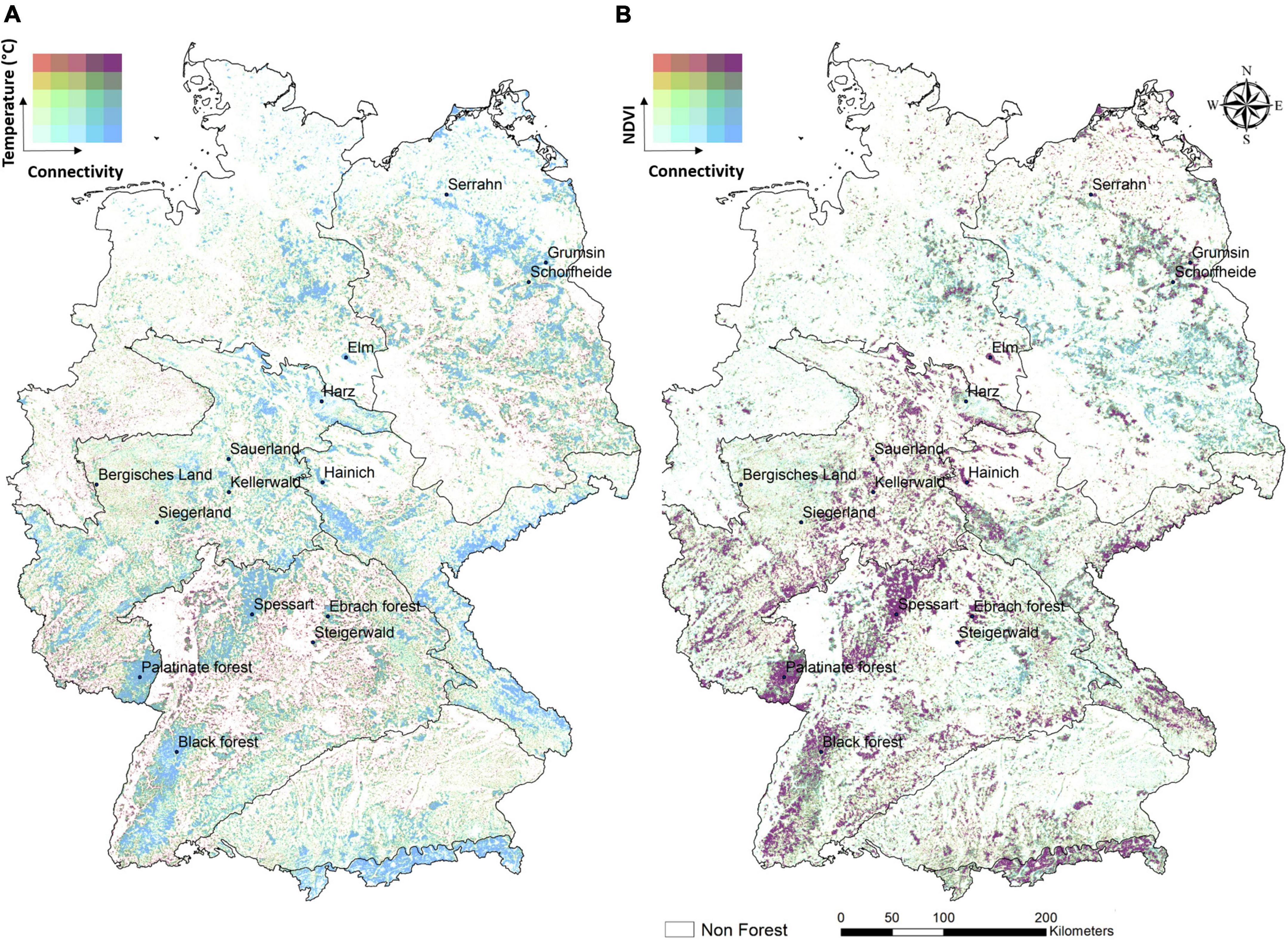
Figure 4. Bivariate maps of tree-covered areas in Germany in 2022 and the relation between the degree of fragmentation with temperatures and vegetation vitality. (A) Thiessen connectivity and land surface temperature of hot days in 2022. (B) Thiessen connectivity and the maximum NDVI per growing season in 2022.
The range of variations taking into account all the forest fragments per ecoregion can be observed in the scaled data ranges of the connectivity in tree-covered areas in their relation to hot day temperatures and maximum vitality (Figure 5). Forest in the Alps is relatively less fragmented than in other ecoregions, is cooler, and has the highest forest vitality. The Lowlands show the lowest median connectivity and lowest vitality, while having similar median temperatures as the Uplands. The highest median temperature is recorded in the Southwest Uplands. In the Western and Eastern Uplands and the Prealps, slightly higher connectivity is accompanied by lower temperatures and higher vitality. When investigating the distribution of Thiessen connectivity per ecoregion regardless of fragment groups, the connectivity is much lower (Figure 5), the reason being that all the ecoregions are dominated by the presence of forest fragments smaller than 1 km2. Hence, lower overall connectivity values are evident.
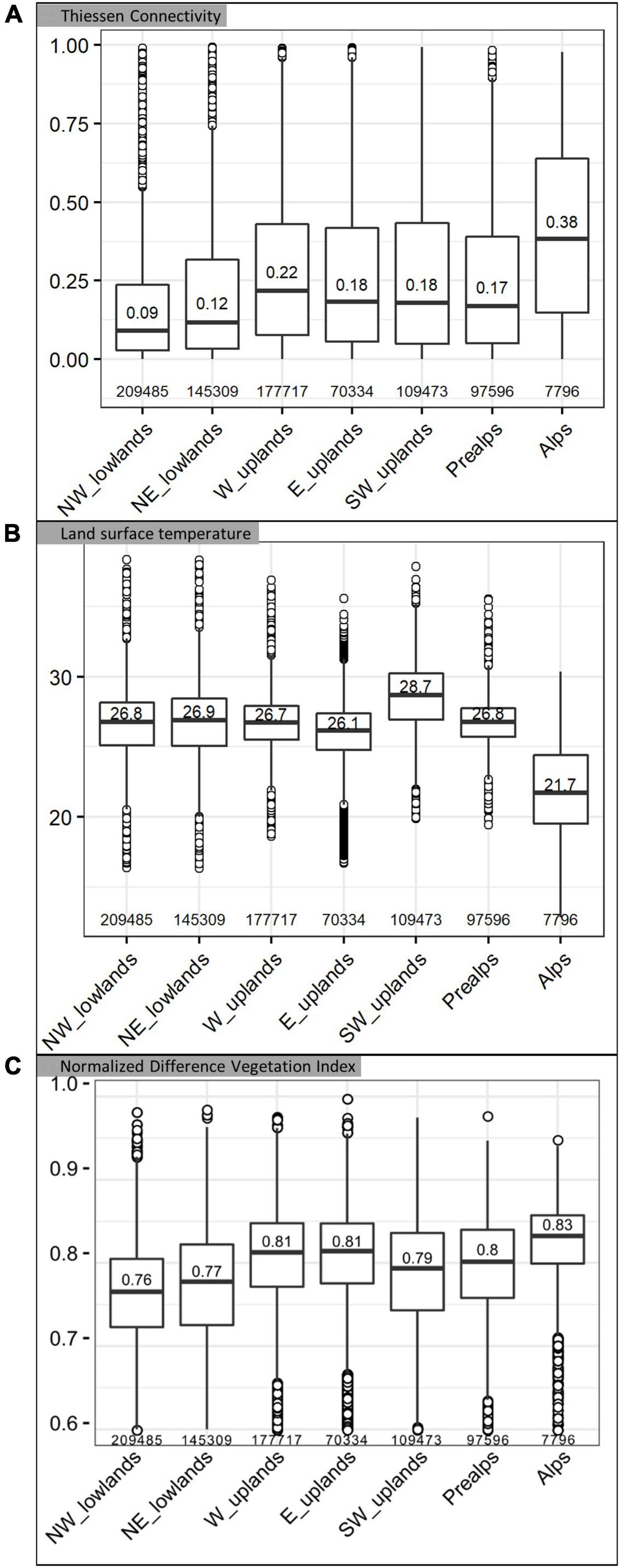
Figure 5. Boxplots of connectivity, temperature, and vitality for tree-covered areas in Germany 2022 per ecoregion including all values regardless of the size of a fragment. The median of each dataset per boxplot is depicted as a bold horizontal line, the line above represents the upper quartile of which 75% of the values fall below and the line below the median represents the lower quartile with 25% of the values below. (A) Thiessen connectivity for forest-covered areas in Germany as of 2022. (B) Land surface temperature (LST) based on the mean of the hottest days in 2022. (C) Vitality (NDVI) based on the maximum value of the growing season in 2022 (for better readability, outliers below 0.6 are not depicted). The count of data points per ecoregion is depicted above the X-axis.
4. Discussion
In the studied year 2022, forest fragmentation clearly impacted local temperatures on hot days and forest vitality. Here, we show that these effects vary by ecoregion and we discuss implications for landscape and forest management.
4.1. Regional implications of fragmentation on forest temperature and vitality
Independent of the ecoregion, larger fragments of tree-covered areas show the highest connectivity, the lowest temperatures, and the highest vitality. Lower values of Thiessen connectivity in all ecoregions result from the dominance of small-sized forest fragments indicating a great degree of fragmentation. The area with the least fragmented forest in Germany are the Alps (highest Thiessen connectivity with a median of 0.38 as compared to the maximum value of 1). In comparison with the other ecoregions, this area is less urbanized due to its topography, and therefore, the effects of fragmentation through infrastructure (Ibisch et al., 2016) or cities are less severe. The overall high forest vitality in the Alps ecoregion is congruent with other studies that measured a growing biomass stock and an expanding forest-covered area, despite intensified natural and human-induced disturbances (Bebi et al., 2017). The Alps are the smallest of all ecoregions with 7,796 data points of the tree-covered areas observed. The high elevation and terrain with steep slopes are most likely a reason for the diverse range of temperature values from low to high. Even though fewer hot days in the year 2022 for the Alps ecoregion were detected, the Alps are also threatened by climate change and more frequent temperature extremes (Seidl et al., 2011; Gobiet et al., 2014). A larger forest cover in mountainous regions can help retain permanent snow cover longer than non-tree-covered areas (Hesslerová et al., 2018).
The Prealps are characterized by the highest altitudinal range of all ecoregions from 299- to 1,468-m elevation (refer to Figure 3). As a consequence of more intensive land use, the tree-covered areas in the Prealps are less connected and less vital as compared to the Alps, and the land surface temperature median is ca. 5°C higher than in the Alps. The foothills of the Alps are also impacted by climate change (e.g., Thrippleton et al., 2020).
The Southwest Uplands are the ecoregion in Germany with the highest measured temperatures of forest canopies in 2022. Therefore, the forest is rarely connected (Thiessen connectivity median 0.18) with a medium vitality (median NDVI 0.79) and high temperatures (median of 28.7°C is 7°C hotter than in the Alps). It is in fact the hottest region in Germany in terms of regional climate and is highly populated. In this ecoregion, there are a few highly connected forests such as the Black Forest in the south-westernmost part but mostly areas with low connectivity and smaller forest fragments. A small-scale study in the area, investigating Scots pine forest edges using dendroecological investigations and close-range remote sensing, found that these forest edges are more vulnerable to drought, showing increased mortality rates and lower tree vitality (Buras et al., 2018). A study conducted in Switzerland using thermal imaging found that the resilience to the drought of broad-leaved forests varies by species (Scherrer et al., 2011). Lower vitality values with a median of 0.71 for the year 2022 can be related to the spruce forest dieback, which is the dominant tree type in the ecoregion. Still, coniferous forests in dense stands seem to benefit from higher connectivity and show higher vitality, especially in the Black Forest (Supplementary Figures 3, 5, 6). Larger broad-leaved dominated forest areas in the northern part of the ecoregion, such as the Spessart, Steigerwald, or Ebrach forests, show relatively high vitality (Supplementary Figure 5).
The Eastern Uplands share a similar value of low connectivity (median 0.18) as the Southwest Uplands but a higher vitality (median 0.81) and 2°C lower temperatures. A strong temperature decline, vitality, and connectivity increase from medium to large forest fragments and can be associated with less intensively managed forests. In the Bavarian forest region, the mainly coniferous forest is relatively vital and connected (Figure 4). However, the vegetation is under stress due to climate-related bark beetle outbreaks of spruce before 2012 (Lausch et al., 2013a,b). The same is true for the Erzgebirge with deforestation due to a recent bark beetle outbreak (Gdulová et al., 2021). The Thuringian Forest still can be recognized as a relatively well-connected area with relatively low temperatures and higher vitality, despite Norway spruce being the main tree species (Thiel et al., 2006). But for some years, it already suffers from heavy storms and bark beetle outbreaks, and it is most likely, that in this area, the effects of climate change will become more frequent, such as a rise in temperatures, more frequent temperature extremes, and a decreased water supply during the growing season (Frischbier et al., 2014).
The Western Uplands are characterized by slightly higher overall connectivity than the Eastern and Southwest Uplands but show higher temperatures and not notably higher vitality (Figure 5A). A high connectivity and high vitality are visible in the Palatinate Forest (Pfälzerwald), on the southern edge of the ecoregion. In general, the vitality is higher than in the other Uplands, especially, because of the relatively high share of broad-leaf forests with a higher vitality range per se (Supplementary Figure 3). At the same time, the ecoregion is dominated by large coniferous plantations and experienced strong Norway spruce dieback in the last years. The Western Uplands are one of the formerly largest forest regions in Western Germany, including the Sauerland, Bergisches Land, Siegerland, and Harz mountains. After years of massive dieback and salvage logging, the remaining forests are in relatively poor condition, fragmented, and with reduced vitality (Popkin, 2021).
In the Northeast Lowlands and the Northwest Lowlands, the small size forest fragments show the lowest connectivity. Even in larger forest fragments with higher connectivity detected, the vitality values are low. This is due to a large number of coniferous plantations in both ecoregions (Supplementary Figure 6). Many Scots pine plantations do not seem to benefit from the generally somewhat lower temperatures in the north. They are often well-connected, but this does not translate into a better vitality signature. In general, there seems to be a gradient toward higher vitality in the north, possibly due to the buffering impact of the sea with its maritime climate, a higher precipitation potential, and a lower drought potential than in the south of Germany (Zink et al., 2016). This effect of continentality is reflected to some extent by the ecoregions. The median connectivity of 0.09 and median vitality of 0.76 are the lowest of all ecoregions in the Northwest Lowlands and therefore can be attested to the less connected forest cover of all ecoregions. The fragmented landscape leads to low connectivity and higher temperatures, and the coniferous plantations (according to ground-truthing) have low vitality. These effects are repercussions, especially of the extreme years from 2018 onward, and consequent bark beetle outbreaks (refer to Supplementary Figure 2). Interestingly, the largest beech forest in Northern Germany, the Elm, lies within the Northwest Lowlands and shows relatively high Thiessen connectivity values with high vitality and low temperatures (refer to Supplementary Figures 5, 7).
4.2. Effects of fragmentation
Independent of the ecoregion, larger fragments of tree-covered areas show the highest connectivity, the lowest temperatures, and the highest vitality (Figure 3). The cooling functions of forest fragments increase with higher connectivity. This cooling effect was also observed in a study on reforestation (Huang et al., 2022). Our results regarding the correlation between high connectivity and increasing forest vitality, based on the NDVI in the temperate forest realm, correspond to other studies with similar results. Others used the NDVI, and for connectivity “vegetation continuous fields,” and found that higher connectivity in protected areas correlates to high NDVI values (Sun et al., 2021). Other factors influencing the NDVI that are not covered in this study would be local and regional characteristics such as climate, soil moisture, dominant tree species, and the degree of disturbance.
This study focuses on regional assessments and could not scrutinize the effects of fragmentation through skidding trails and small-scale edge effects. These patterns potentially increase the fragmentation impacts and most likely have additional effects on the local forest temperature and vitality (Buckley et al., 2003; Sufo Kankeu et al., 2016; Shirvani et al., 2020). The characteristics of native and planted forests in Germany were not covered in this study. To date, no comprehensive and explicit spatial information on the native and planted forests is available, neither for the globe (Grantham et al., 2020) nor for Germany. It is also important to note that in some areas, the NDVI and Thiessen connectivity are not necessarily positively correlated. There are definitely other relevant effects such as the type of forests. For example, in the Scots pine forests of northeastern Germany, relatively low vitality is observed despite high connectivity. However, especially these forests represent mostly planted even-aged monocultures with low structural diversity, relatively open canopies, and many timber extraction roads and trails. Our analysis could not take into account that there is also internal forest fragmentation, which contributes to the vulnerability of the ecosystem in the form of forest roads and skid trails as there are currently no data available. The situation in Germany is currently worsening because the infrastructure for the expansion of renewable energy production is pushing into the forest. Here, wide permanent access roads and openings for wind turbines increase the forest edges and the edge effects in the midst of the forest.
To promote the protection of remaining old-growth broad-leaved forests, UNESCO recognized some old temperate forests in Europe as natural heritage, which are component parts of the serial UNESCO transnational property “Ancient and Primeval Beech Forests of the Carpathians and Other Regions of Europe” (Voloscuk et al., 2013; Ibisch et al., 2017; Jovanović et al., 2019; UNESCO, 2022). In Germany, the Hainich National Park, Kellerwald-Edersee National Park, Serrahn Forest, and the Grumsin Forest are part of this property and show relatively high connectivity, especially in the core zones, despite being of relatively small size. These areas are highly important not only in terms of being a heritage site but also as a cooling factor in the landscape, a remnant habitat, and are of recreational value for humans.
4.3. Recommendations for landscape and forest management
Forest vitality and functionality are not only impacted by climate change and natural disturbances but also by silvicultural management. The management attempts of the last decades to protect forests in Europe did not mitigate climate warming (Naudts et al., 2016) but even led to increased temperatures within forests (Blumröder et al., 2021). Effective ecosystem management must allow ecosystems to mature through the growth of biomass, information, and network and to maintain or enhance working capacity in the best possible way. The production and storage of biomass and biogenic free energy in the ecosystem—including dead wood, humus, or organic molecules in mineral soil—are the physical basis of all possible natural ecological processes in the ecosystem. Linking to the development of biophysical capacity is also an increase in the ecohydrological capacity of forest ecosystems. The conservation of “green water” stored and mobilized by ecosystems (Ellison et al., 2017; Sheil, 2018; Te Wierik et al., 2021) and microclimatic regulation (Blumröder et al., 2021, 2022) deserve the highest priority in management (Ibisch, 2022). To mitigate hot temperature extremes in European forests, the increase in the broad-leaved tree fraction is a necessary measure (Schwaab et al., 2020). German forests are already highly fragmented. In some forest-poor regions, it is recommended to increase tree and forest cover to buffer temperatures and contribute to forest vitality (Gohr et al., 2021). The regulating function of connected forests within the water, energy, and carbon cycles is more needed than ever (Ellison et al., 2017) since highly fragmented forests with more forest edges provoke more carbon loss (Smith et al., 2018).
Remote sensing and especially new datasets like the dominant tree species of German forests (Welle et al., 2022) will support monitoring fragmentation as seen in other countries (Kupfer, 2006). It is an urgent task for the state rapporteurs of the federal states and the federal ministries responsible for forests to ensure that forest fragmentation is included in the forest status reports and the corresponding analyses of forest health.
Fragmentation is caused by infrastructure expansion and land use changes but also by (past) forest management: When plantations collapse by massive tree dieback, they are often salvage-logged. The regeneration capacity decreases under climate change and potentially these areas are then converted into open lands. It is inevitable that forest fragmentation in the near future will further increase due to large-scale tree dieback in monocultures. The ban on salvage logging and clearcutting seems to be a necessary step in forest management. The existing smaller forest fragments have the potential to be transformed into larger core forest areas for enhanced ecosystem development. This can be achieved by the abandonment of timber extraction, implementing reforestation measures such as assisted restoration, planting native trees, natural regrowth, agroforestry solutions, and commercial plantations.
5. Conclusion
The study reflects that the forests in Germany are highly fragmented, which weakens their ecosystem functionality. This study provides observational evidence to show that highly fragmented forests exhibit higher temperatures and less forest vitality. Thereby, the fragmentation in Germany as of 2022 substantially contributes to the current and ongoing forest crises. The existing small forest fragments have immense potential to be transformed into larger core areas for better ecosystem functioning. With increasing forest fires and extreme climatic events in Germany and worldwide in general, there is an urgent need to advance forest management and restoration efforts that can safeguard the benefits of functional forests as much and as long as possible. Reducing the fragmentation of forests is a crucial contribution to ecosystem-based adaptation to climate change.
Data availability statement
The original contributions presented in this study are included in the article/Supplementary material, further inquiries can be directed to the corresponding author.
Author contributions
PI, DM, and CG designed the research. DM and CG performed the analysis, visualization, and wrote the original draft. PI and JB provided comprehensive support in the assessment and interpretation of the results. All authors contributed to the interpretation of the results and the subsequent revisions of the manuscript.
Funding
This study was partly funded by the PYROPHOB project (Grant number: 2219WK50A4, Federal Ministry of Food and Agriculture and the Federal Ministry for the Environment, Nature Conservation, Nuclear Safety, and Consumer Protection) and through the “Gläserner Forstbetrieb” project by the German Federal Ministry for Education and Research (BMBF) via the VDI/VDE, grant number: 16LC1603C. PI conceived and supervised the study within the framework of his long-term research program facilitated by the research professorships “Biodiversity and natural resource management under global change” (2009–2015) and “Ecosystem based sustainable development” (since 2015) granted by the Eberswalde University for Sustainable Development. CG was funded through the Biosphere Reserves Institute and the Innovation and Career Center “ProBio-LaB” by the Ministry of Science, Research and Culture of the federal state of Brandenburg.
Conflict of interest
The authors declare that the research was conducted in the absence of any commercial or financial relationships that could be construed as a potential conflict of interest.
Publisher’s note
All claims expressed in this article are solely those of the authors and do not necessarily represent those of their affiliated organizations, or those of the publisher, the editors and the reviewers. Any product that may be evaluated in this article, or claim that may be made by its manufacturer, is not guaranteed or endorsed by the publisher.
Supplementary material
The Supplementary Material for this article can be found online at: https://www.frontiersin.org/articles/10.3389/ffgc.2023.1099460/full#supplementary-material
References
Aalto, I. J., Maeda, E. E., Heiskanen, J., Aalto, E. K., and Pellikka, P. K. E. (2022). Strong influence of trees outside forest in regulating microclimate of intensively modified afromontane landscapes. Biogeosciences 19, 4227–4247. doi: 10.5194/bg-19-4227-2022
Armenteras, D., Gonzalez, T. M., and Retana, J. (2013). Forest fragmentation and edge influence on fire occurrence and intensity under different management types in Amazon forests. Biol. Conserv. 159, 73–79. doi: 10.1016/j.biocon.2012.10.026
Bebi, P., Seidl, R., Motta, R., Fuhr, M., Firm, D., Krumm, F., et al. (2017). Changes of forest cover and disturbance regimes in the mountain forests of the Alps. For. Ecol. Manag. Ecol. Mount. For. Ecosyst. Eur. 388, 43–56. doi: 10.1016/j.foreco.2016.10.028
Betts, M. G., Wolf, C., Ripple, W. J., Phalan, B., Millers, K. A., Duarte, A., et al. (2017). Global forest loss disproportionately erodes biodiversity in intact landscapes. Nature 547, 441–444. doi: 10.1038/nature23285
Blumröder, J. S., May, F., Härdtle, W., and Ibisch, P. L. (2021). Forestry contributed to warming of forest ecosystems in Northern Germany during the extreme summers of 2018 and 2019. Ecol. Solut. Evid. 2:e12087. doi: 10.1002/2688-8319.12087
Blumröder, J. S., Schmidt, F., Gordon, A., Grosse, S., and Ibisch, P. L. (2022). Ecosystemic resilience of a temperate post-fire forest under extreme weather conditions. Front. For. Glob. Change 5:1070958. doi: 10.3389/ffgc.2022.1070958
Briant, G., Gond, V., and Laurance, S. G. W. (2010). Habitat fragmentation and the desiccation of forest canopies: A case study from Eastern Amazonia. Biol. Conserv. 143, 2763–2769. doi: 10.1016/j.biocon.2010.07.024
Buckley, D. S., Crow, T. R., Nauertz, E. A., and Schulz, K. E. (2003). Influence of skid trails and haul roads on understory plant richness and composition in managed forest landscapes in Upper Michigan, USA. For. Ecol. Manag. 175, 509–520. doi: 10.1016/S0378-1127(02)00185-8
Bundesamt für Naturschutz [BfN] (2017). Naturräume und Großlandschaften Deutschland. Bonn: Federal Agency for Nature Conservation.
Bundesministerium für Ernährung und Landwirtschaft [BMBL] (2022). Ergebnisse der Waldzustandserhebung 2021. Rochusstr: BMBL, 76.
Buras, A., Rammig, A., and Zang, C. S. (2021). The European forest condition monitor: Using remotely sensed forest greenness to identify hot spots of forest decline. Front. Plant Sci. 12:689220. doi: 10.3389/fpls.2021.689220
Buras, A., Schunk, C., Zeiträg, C., Herrmann, C., Kaiser, L., Lemme, H., et al. (2018). Are scots pine forest edges particularly prone to drought-induced mortality? Environ. Res. Lett. 13:025001. doi: 10.1088/1748-9326/aaa0b4
Chakraborty, T., Sarkar, S. K., and Morshed, M. D. M. (2022). Big data and remote sensing for multi-decadal drought impact assessment on shorea robusta. Theor. Appl. Climatol. 148, 1587–1602. doi: 10.1007/s00704-022-04019-2
Deng, Y., Wang, S., Bai, X., Tian, Y., Wu, L., Xiao, J., et al. (2018). Relationship among land surface temperature and LUCC. NDVI in typical karst area. Sci. Rep. 8:641. doi: 10.1038/s41598-017-19088-x
Deutscher Wetterdienst [DWD] (2022). Deutschlandwetter im herbst 2022. Available online at: https://www.dwd.de/DE/presse/pressemitteilungen/DE/2022/20221130_deutschlandwetter_herbst2022_news.html (accessed October 10, 2022).
Didan, K. (2015). MOD13A2 MODIS/Terra vegetation indices 16-Day L3 global 1km SIN Grid V006 [Data Set]. NASA EOSDIS land processes DAAC. Available online at: https://doi.org/10.5067/MODIS/MOD13A2.006 (accessed June 7, 2022).
Driscoll, D. A., Armenteras, D., Bennett, A. F., Brotons, L., Clarke, M. F., Doherty, T. S., et al. (2021). How fire interacts with habitat loss and fragmentation. Biol. Rev. 96, 976–998.
Ellison, D., Morris, C. E., Locatelli, B., Sheil, D., Cohen, J., Murdiyarso, D., et al. (2017). Trees, forests and water: Cool insights for a hot world. Glob. Environ. Change 43, 51–61. doi: 10.1016/j.gloenvcha.2017.01.002
Estreguil, C., Caudullo, G., de Rigo, D., and San-Miguel-Ayanz, J. (2013). Forest Landscape in Europe: Pattern, fragmentation and connectivity. EUR Sci. Tech. Res. 25717.
Fisher, J. B., Melton, F., Middleton, E., Hain, C., Anderson, M., Allen, R., et al. (2017). The future of evapotranspiration: Global requirements for ecosystem functioning, carbon and climate feedbacks, agricultural management, and water resources. Water Resour. Res. 53, 2618–2626. doi: 10.1002/2016WR020175
Frischbier, N., Profft, I., and Hagemann, U. (2014). “Potential impacts of climate change on forest habitats in the biosphere reserve Vessertal-Thuringian forest in Germany,” in Managing protected areas in central and Eastern Europe under climate change, eds S. Rannow and M. Neubert (Dordrecht: Springer), 243–257.
Gdulová, K., Marešová, J., Barták, V., Szostak, M., Červenka, J., and Moudrý, V. (2021). Use of TanDEM-X and SRTM-C data for detection of deforestation caused by bark beetle in central European mountains. Remote Sens. 13:3042. doi: 10.3390/rs13153042
Gobiet, A., Kotlarski, S., Beniston, M., Heinrich, G., Rajczak, J., and Stoffel, M. (2014). 21st century climate change in the European Alps—A review. Sci. Tot. Environ. 493, 1138–1151. doi: 10.1016/j.scitotenv.2013.07.050
Gohr, C., Blumröder, J. S., Sheil, D., and Ibisch, P. L. (2021). Quantifying the mitigation of temperature extremes by forests and wetlands in a temperate landscape. Ecol. Inform. 66:101442. doi: 10.1016/j.ecoinf.2021.101442
Grantham, H. S., Duncan, A., Evans, T. D., Jones, K. R., Beyer, H. L., Schuster, R., et al. (2020). Anthropogenic modification of forests means only 40% of remaining forests have high ecosystem integrity. Nat. Commun. 11, 1–10. doi: 10.1038/s41467-020-19493-3
Hansen, M. C., Potapov, P. V., Moore, R., Hancher, M., Turubanova, S. A. A., Tyukavina, A., et al. (2013). High-resolution global maps of 21st-century forest cover change. Science 342, 850–853. doi: 10.1126/science.1244693
Hansen, M. C., Stehman, S. V., and Potapov, P. V. (2010). Quantification of global gross forest cover loss. Proc. Natl. Acad. Sci. U.S.A. 107, 8650–8655. doi: 10.1073/pnas.0912668107
Harper, K. A., Ellen Macdonald, S., Burton, P. J., Chen, J., Brosofske, K. D., Saunders, S. C., et al. (2005). Edge influence on forest structure and composition in fragmented landscapes. Conserv. Biol. 19, 768–782.
Hesslerová, P., Huryna, H., Pokorný, J., and Procházka, J. (2018). The effect of forest disturbance on landscape temperature. Ecol. Eng. 120, 345–354.
Huang, A., Shen, R., Jia, G., and Xu, X. (2022). Reforestation enhanced landscape connectivity for thermal buffering in China. Environ. Res. Lett. 17:014056. doi: 10.1088/1748-9326/ac3fda
Ibisch, P. L. (2022). Ein ökosystembasierter Ansatz für den Umgang mit der Waldkrise in der Klimakrise. Natur Landschaft 97, 325–333.
Ibisch, P. L., Hoffmann, M. T., Kreft, S., Pe’er, G., Kati, V., Biber-Freudenberger, L., et al. (2016). A global map of roadless areas and their conservation status. Science 354, 1423–1427. doi: 10.1126/science.aaf7166
Ibisch, P. L., Waldherr, M. G., and Knapp, H. D. (2017). Extension nomination of the’Primeval Beech Forests of the Carpathians and Ancient Beech Forests of Germany’as Pan-European UNESCO natural world heritage site. Natur Landschaft 92, 109–118.
Imbery, F., Friedrich, K., Fleckenstein, R., Becker, A., Bissolli, P., Haeseler, S., et al. (2022). Klimatologischer rückblick sommer 2022. Deutscher wetterdienst. Available online at: https://www.dwd.de/DE/leistungen/besondereereignisse/temperatur/20220921_bericht_sommer2022.pdf?__blob=publicationFile&v=6 (accessed October 10, 2022).
Jacob, D., Kotova, L., Teichmann, C., Sobolowski, S. P., Vautard, R., Donnelly, C., et al. (2018). Climate impacts in Europe Under+ 1.5 C global warming. Earth’s Fut. 6, 264–285.
Jin, M., and Dickinson, R. E. (2010). Land surface skin temperature climatology: Benefitting from the strengths of satellite observations. Environ. Res. Lett. 5:044004. doi: 10.1088/1748-9326/5/4/044004
Jovanović, I., Dragišić, A., Ostojić, D., and Krsteski, B. (2019). Beech forests as world heritage in aspect to the next extension of the ancient and primeval beech forests of the carpathians and other regions of Europe world heritage site. Zastita Prirode 69, 15–32. doi: 10.5937/ZasPri1901015J
Kaplan, J. O., Krumhardt, K. M., and Zimmermann, N. (2009). The prehistoric and preindustrial deforestation of Europe. Quaternary Sci. Rev. 28, 3016–3034. doi: 10.1016/j.scitotenv.2006.08.013
Kupfer, J. A. (2006). National assessments of forest fragmentation in the US. Glob. Environ. Change 16, 73–82. doi: 10.1016/j.gloenvcha.2005.10.003
Lambert, J., Drenou, C., Denux, J.-P., Balent, G., and Cheret, V. (2013). Monitoring forest decline through remote sensing time series analysis. GISci. Remote Sens. 50, 437–457. doi: 10.1080/15481603.2013.820070
Laurance, W. F., Camargo, J. L. C., Luizão, R. C. C., Laurance, S. G., Pimm, S. L., Bruna, E. M., et al. (2011). The fate of Amazonian forest fragments: A 32-year investigation. Biol. Conserv. 144, 56–67.
Laurance, W. F., Lovejoy, T. E., Vasconcelos, H. L., Bruna, E. M., Didham, R. K., Stouffer, P. C., et al. (2002). Ecosystem decay of Amazonian forest fragments: A 22-year investigation. Conserv. Biol. 16, 605–618.
Lausch, A., Heurich, M., Gordalla, D., Dobner, H.-J., Gwillym-Margianto, S., and Salbach, C. (2013a). Forecasting potential bark beetle outbreaks based on spruce forest vitality using hyperspectral remote-sensing techniques at different scales. For. Ecol. Manag. 308, 76–89. doi: 10.1016/j.foreco.2013.07.043
Lausch, A., Heurich, M., and Fahse, L. (2013b). Spatio-temporal infestation patterns of Ips typographus (L.) in the Bavarian forest national park, Germany. Ecol. Indicat. Link. Landscape Struc. Biodivers. 31, 73–81. doi: 10.1016/j.ecolind.2012.07.026
Li, Y., Zhao, M., Mildrexler, D. J., Motesharrei, S., Mu, Q., Kalnay, E., et al. (2016). Potential and actual impacts of deforestation and afforestation on land surface temperature. J. Geophys. Res. Atmos. 121, 14,372–14,386.
Li, Z.-L., Tang, B.-H., Wu, H., Ren, H., Yan, G., Wan, Z., et al. (2013). Satellite-derived land surface temperature: Current status and perspectives. Remote Sens. Environ. 131, 14–37.
Lindner, M., Fitzgerald, J. B., Zimmermann, N. E., Reyer, C., Delzon, S., van der Maaten, E., et al. (2014). Climate change and European forests: What do we know, What are the uncertainties, and What are the implications for forest management? J. Environ. Manag. 146, 69–83. doi: 10.1016/j.jenvman.2014.07.030
Mehdipour, N., Fakheran, S., Soffianian, A., and Pourmanafi, S. (2019). Road-induced fragmentation and the environmental value of roadless areas in a partly protected landscape in Central Iran. Environ. Monit. Assess. 191:461. doi: 10.1007/s10661-019-7571-4
Mildrexler, D. J., Zhao, M., and Running, S. W. (2011). A global comparison between station air temperatures and MODIS land surface temperatures reveals the cooling role of forests. J. Geophys. Res. Biogeosci. 116, doi: 10.1029/2010JG001486
Minnemeyer, S., and Potapov, P. (2017). World’s last intact forests are becoming increasingly fragmented. Washington, DC: World Resource Institute.
Moomaw, W. R., Masino, S. A., and Faison, E. K. (2019). Intact forests in the United States: Proforestation mitigates climate change and serves the greatest good. Front. For. Glob. Change 2:27. doi: 10.3389/ffgc.2019.00027
Naudts, K., Chen, Y., McGrath, M. J., Ryder, J., Valade, A., Otto, J., et al. (2016). Europe’s forest management did not mitigate climate warming. Science 351, 597–600. doi: 10.1126/science.aad7270
Olson, D. M., Dinerstein, E., Wikramanayake, E. D., Burgess, N. D., Powell, G. V. N., Underwood, E. C., et al. (2001). Terrestrial ecoregions of the world: A new map of life on earth: A new global map of terrestrial ecoregions provides an innovative tool for conserving biodiversity. BioScience 51, 933–938. doi: 10.1641/0006-35682001051[0933:TEOTWA]2.0.CO;2
Potapov, P., Hansen, M. C., Laestadius, L., Turubanova, S., Yaroshenko, A., Thies, C., et al. (2017). The last frontiers of wilderness: Tracking loss of intact forest landscapes from 2000 to 2013. Sci. Adv. 3:e1600821. doi: 10.1126/sciadv.1600821
Riitters, K. H. (2007). “Forest fragmentation,” in Forest health monitoring: 2005 national technical report. General technical report SRS-104, Vol. 104, (Asheville, NC: US Department of Agriculture, Forest Service, Southern Research Station), 9–15.
Rogers, B. M., Mackey, B., Shestakova, T. A., Keith, H., Young, V., Kormos, C. F., et al. (2022). Using ecosystem integrity to maximize climate mitigation and minimize risk in international forest policy. Front. For. Glob. Change 5:929281. doi: 10.3389/ffgc.2022.929281
Scherrer, D., Bader, M. K. F., and Körner, C. (2011). Drought-sensitivity ranking of deciduous tree species based on thermal imaging of forest canopies. Agric. For. Meteorol. 151, 1632–1640. doi: 10.1016/j.agrformet.2011.06.019
Schuldt, B., Buras, A., Arend, M., Vitasse, Y., Beierkuhnlein, C., Damm, A., et al. (2020). A first assessment of the impact of the extreme 2018 summer drought on central European forests. Basic Appl. Ecol. 45, 86–103. doi: 10.1016/j.baae.2020.04.003
Schwaab, J., Davin, E. L., Bebi, P., Duguay-Tetzlaff, A., Waser, L. T., Haeni, M., et al. (2020). Increasing the broad-leaved tree fraction in European forests mitigates hot temperature extremes. Sci. Rep. 10:14153. doi: 10.1038/s41598-020-71055-1
Seidl, R., Rammer, W., and Lexer, M. J. (2011). Climate change vulnerability of sustainable forest management in the Eastern Alps. Climatic Change 106, 225–254. doi: 10.1007/s10584-010-9899-1
Sheil, D. (2018). Forests, atmospheric water and an uncertain future: The new biology of the global water cycle. For. Ecosyst. 5, 1–22.
Shirvani, Z., Abdi, O., and Buchroithner, M. F. (2020). A new analysis approach for long-term variations of forest loss, fragmentation, and degradation resulting from road-network expansion using landsat time-series and object-based image analysis. Land Degradation Dev. 31, 1462–1481. doi: 10.1002/ldr.3530
Silva Junior, C. H. L., Aragão, L. E. O. C., Anderson, L. O., Fonseca, M. G., Shimabukuro, Y. E., Vancutsem, C., et al. (2020). Persistent collapse of biomass in Amazonian forest edges following deforestation leads to unaccounted carbon losses. Sci. Adv. 6:eaaz8360. doi: 10.1126/sciadv.aaz8360
Smith, I. A., Hutyra, L. R., Reinmann, A. B., Marrs, J. K., and Thompson, J. R. (2018). Piecing together the fragments: Elucidating edge effects on forest carbon dynamics. Front. Ecol. Environ. 16, 213–221. doi: 10.1002/fee.1793
Smith, J. R., Letten, A. D., Ke, P.-J., Anderson, C. B., Hendershot, J. N., Dhami, M. K., et al. (2018). A global test of ecoregions. Nat. Ecol. Evol. 2, 1889–1896. doi: 10.1038/s41559-018-0709-x
Sufo Kankeu, R., Sonwa, D. J., Eba’a Atyi, R., and Nkal, N. M. M. (2016). Quantifying post logging biomass loss using satellite images and ground measurements in Southeast Cameroon. J. For. Res. 27, 1415–1426. doi: 10.1007/s11676-016-0277-3
Sun, J., Zheng, Y., and Shi, J. (2021). Conserving the landscape connectivity of natural forest reserves in tourism development. Prof. Geogr. 73, 573–578. doi: 10.1080/00330124.2021.1898994
Taubert, F., Fischer, R., Groeneveld, J., Lehmann, S., Müller, M. S., Rödig, E., et al. (2018). Global patterns of tropical forest fragmentation. Nature 554, 519–522. doi: 10.1038/nature25508
Te Wierik, S. A., Cammeraat, E. L. H., Gupta, J., and Artzy-Randrup, Y. A. (2021). Reviewing the impact of land use and land-use change on moisture recycling and precipitation patterns. Water Resour. Res. 57:e2020WR029234. doi: 10.1029/2020WR029234
Thiel, C., Drezet, P., Weise, C., Quegan, S., and Schmullius, C. (2006). Radar remote sensing for the delineation of forest cover maps and the detection of deforestation. For. Int. J. For. Res. 79, 589–597. doi: 10.1093/forestry/cpl036
Thonfeld, F., Gessner, U., Holzwarth, S., Kriese, J., Da Ponte, E., and Kuenzer, C. (2022). Recent forest cover loss in Germany after the 2018-2020 drought years. Bonn, Germany. Available online at: https://elib.dlr.de/186889/ (accessed August 23, 2022).
Thrippleton, T., Lüscher, F., and Bugmann, H. (2020). Climate change impacts across a large forest enterprise in the Northern Pre-Alps: Dynamic forest modelling as a tool for decision support. Eur. J. For. Res. 139, 483–498. doi: 10.1007/s10342-020-01263-x
Tropek, R., Sedláček, O., Beck, J., Keil, P., Musilová, Z., Šímová, I., et al. (2014). Comment on ‘High-resolution global maps of 21st-century forest cover change.’ Science 344, 981–981.
Tuff, K. T., Tuff, T., and Davies, K. F. (2016). A framework for integrating thermal biology into fragmentation research. Ecol. Lett. 19, 361–374. doi: 10.1111/ele.12579
UNESCO (2022). Ancient and primeval beech forests of the carpathians and other regions of Europe. UNESCO World Heritage Centre. Available online at: https://whc.unesco.org/en/list/1133/ (accessed October 10, 2022).
Vieilledent, G., Grinand, C., Rakotomalala, F. A., Ranaivosoa, R., Rakotoarijaona, J. R., Allnutt, T. F., et al. (2018). Combining global tree cover loss data with historical national forest cover maps to look at six decades of deforestation and forest fragmentation in Madagascar. Biol. Conserv. 222, 189–197.
Voloscuk, I., Pichler, V., and Pichlerová, M. (2013). The primeval beech forests of the carpathians and ancient beech forests of Germany: Joint natural heritage of Europe. Folia Oecol. 40:295.
Wan, Z., Hook, S., and Hulley, G. (2015). Collection-6MODIS land surface temperature productsusers’ guide. NASA EOSDIS land processes DAAC. Available online at: https://doi.org/10.5067/MODIS/MYD11A1.006 (accessed June 7, 2022).
Welle, T., Aschenbrenner, L., Kuonath, K., Kirmaier, S., and Franke, J. (2022). Mapping dominant tree species of German forests. Remote Sens. 14:3330. doi: 10.3390/rs14143330
Weng, Q., Lu, D., and Schubring, J. (2004). Estimation of land surface temperature–vegetation abundance relationship for urban heat island studies. Remote Sens. Environ. 89, 467–483. doi: 10.1016/j.rse.2003.11.005
Wu, L., Zhou, H., Li, J., Li, K., Sun, X., Lu, S., et al. (2019). Thiessen polygon analysis and spatial pattern evolution of neolithic cultural sites (8.0–4.0 Ka BP) in Huaibei Plain of Anhui, East China. Quaternary Int. 521, 75–84. doi: 10.1016/j.quaint.2019.06.005
Zhan, X., Kustas, W. P., and Humes, K. S. (1996). An intercomparison study on models of sensible heat flux over partial canopy surfaces with remotely sensed surface temperature. Remote Sens. Environ. 58, 242–256.
Keywords: land surface temperature (LST), normalized difference vegetation index (NDVI), Thiessen connectivity, forest fragmentation, forest cover
Citation: Mann D, Gohr C, Blumröder JS and Ibisch PL (2023) Does fragmentation contribute to the forest crisis in Germany? Front. For. Glob. Change 6:1099460. doi: 10.3389/ffgc.2023.1099460
Received: 15 November 2022; Accepted: 02 January 2023;
Published: 20 January 2023.
Edited by:
William R. Moomaw, Tufts University, United StatesReviewed by:
Heather Keith, Griffith University, AustraliaDavid Joseph Mildrexler, Eastern Oregon Legacy Lands, United States
Copyright © 2023 Mann, Gohr, Blumröder and Ibisch. This is an open-access article distributed under the terms of the Creative Commons Attribution License (CC BY). The use, distribution or reproduction in other forums is permitted, provided the original author(s) and the copyright owner(s) are credited and that the original publication in this journal is cited, in accordance with accepted academic practice. No use, distribution or reproduction is permitted which does not comply with these terms.
*Correspondence: Pierre L. Ibisch, Pierre.Ibisch@hnee.de
†These authors have contributed equally to this work and share first authorship