- 1RESTORE: The North Woods, Lincoln, MA, United States
- 2Department of Biological Sciences, Salisbury University, Salisbury, MD, United States
- 3Trinity College, Hartford, CT, United States
- 4Department of Forest Resources, University of Minnesota Center for Forest Ecology, St. Paul, MN, United States
- 5Highstead Foundation, Redding, CT, United States
- 6Department of Wildland Resources, Utah State University Eastern, Price, UT, United States
- 7Harvard Forest, Harvard University, Petersham, MA, United States
A campaign is underway to clear established forests and expand early-successional habitats—also called young forest, pre-forest, early seral, or open habitats—with the intention of benefitting specific species. Coordinated by federal and state wildlife agencies, and funded with public money, public land managers work closely with hunting and forestry interests, conservation organizations, land trusts, and private landowners toward this goal. While forest-clearing has become a major focus in the Northeast and Upper Great Lakes regions of the U.S., far less attention is given to protecting and recovering old-forest ecosystems, the dominant land cover in these regions before European settlement. Herein we provide a discussion of early-successional habitat programs and policies in terms of their origins, in the context of historical baselines, with respect to species’ ranges and abundance, and as they relate to carbon accumulation and ecosystem integrity. Taken together, and in the face of urgent global crises in climate, biodiversity, and human health, we conclude that public land forest and wildlife management programs must be reevaluated to balance the prioritization and funding of early-successional habitat with strong and lasting protection for old-growth and mature forests, and, going forward, must ensure far more robust, unbiased, and ongoing monitoring and evaluation.
1. Introduction
In this paper we conduct a wide-ranging and integrated assessment of the campaign to expand early-successional forest habitats in two regions of the United States: (1) the Northeast, i.e., New England states (Connecticut, Maine, Massachusetts, New Hampshire, Rhode Island, Vermont) and mid-Atlantic states (New York, Pennsylvania, New Jersey, Maryland, Delaware); and (2) the Upper Great Lakes areas of Michigan, Wisconsin, and Minnesota north and east of the prairie-forest border [see Cochrane and Iltis (2000), Frelich and Reich (2010), Anderson et al. (2018)]. We review the history of forest disturbance and biodiversity research, the genesis of the forest-clearing campaign and the conservation rationales, the contrasts between natural old-growth forests and intensively managed forests, the impacts of forest-clearing projects, and the current balance of activity between forest management and protection. We conclude that instead of intensive and costly management to create additional early-successional habitats, a new “natural” alternative should be considered which would protect and allow the dynamic growth of established aggrading, mature, and old-growth forests alongside maintaining existing early-successional habitats, where appropriate, for targeted species and cultural values. Although the focus of our analysis is two regions, we believe it offers useful lessons for many other parts of the U.S. and world experiencing similar situations (DellaSala et al., 2022b).
1.1. History of forest development and disturbance
Every place on Earth has a dynamic ecological trajectory based on temperature, rainfall, soils, natural disturbances, and other conditions. In the Northeast and Upper Great Lakes regions of the United States the predominant ecological trajectory of the landscape in the absence of intensive human activity is toward “old-growth” forests: a resilient, diverse, carbon-dense, and self-sustaining “shifting mosaic” of tree ages, microhabitats, and native species above and below ground (Pelley, 2009; Thom et al., 2019; Raiho et al., 2022).
For thousands of years before European settlement, vast “primary” forests were inhabited by a thriving Native human population and harbored many exceptionally large trees, and ecosystems that would be characterized as “old-growth” today (Lorimer, 1977; Whitney, 1994; Lorimer and White, 2003). Up to 90% of the Northeast was covered by such forests, and dominated by shade-tolerant and moderately shade-tolerant species (Foster, 1995; Cogbill, 2000; Cogbill et al., 2002; Shuman et al., 2004; Thompson et al., 2013; Foster et al., 2017; Oswald et al., 2020b). Approximately 50–60% of the Upper Great Lakes landscape, and 40–50% of the Southern Great Lakes landscape, consisted of old-growth forests (Cottam and Loucks, 1965; Frelich, 2002). These percentages in the Great Lakes regions pertain to older even-aged and multi-aged forests (generally more than 120 years old). Old-growth forests in the East include sites with trees more than 380 years old, established in the 1640s and earlier (Lorimer, 1980; McCarthy and Bailey, 1996; Abrams et al., 1998; Abrams and Copenheaver, 1999; Pederson, 2013; Heeter et al., 2019), and studies of remnant old-growth stands indicate they are adapted to long-intervals between catastrophic disturbances. Young trees of late-successional species (e.g., sugar maple, hemlock, beech) released from suppression combined with new seedlings of mid-tolerant tree species (e.g., white pine, yellow birch, American basswood, black cherry, white ash, northern red oak) after windstorms, and high intensity fires in conifer forests or blown down hardwood forests are followed by early-successional shade-intolerant species (e.g., paper birch, quaking, and bigtooth aspen) with some mid-tolerant species as listed above.
The terms “primary forest,” “old-growth forest,” and “mature forest,” are not standardized (Leverett, 1996; Buchwald, 2005; Mackey et al., 2014; DellaSala et al., 2022a). For this analysis, we use the following definitions:
• Primary forest. A forest composed of native species that has never been logged and has developed following natural disturbances and under natural processes, regardless of its age (Kormos et al., 2018; FAO, 2020).
• Old-growth forest. A forest affected primarily by the forces of nature, with dominant canopy tree species at or beyond half their lifespan, and with structural characteristics such as canopy gaps, pit and mounds, large snags, gnarled tree crowns, a thick duff layer, and accumulated large coarse woody debris (Martin, 1992; Frelich, 1995; Dunwiddie and Leverett, 1996; Mosseler et al., 2003b; D’Amato et al., 2006; Mackey et al., 2014; USDA Forest Service and Bureau of Land Management, 2022).
• Mature forest. A forest with trees of intermediate age and lower levels of old-growth structural characteristics, but from which old-growth conditions are likely to develop over time if allowed to continue to grow (Spies and Franklin, 1991, Frelich, 1995; Strittholt et al., 2006; Keeton et al., 2011).
Old-growth forests not only have a high degree of structural diversity, but also contain a wide variety of tree species, herbaceous plants, insects, mosses and fungi, and deep, carbon-rich soil with an associated soil microbiome (Frelich, 1995; Davis, 1996; Lapin, 2005; D’Amato et al., 2009; Maloof, 2023). Permanent and semi-permanent large openings are rare in old-growth forests of these regions, associated mainly with cliffs and scree slopes, ridge tops, wetlands, peat bogs, serpentine barrens, avalanche tracks, river margins, pond and lake margins, and coastal shrublands and bluffs (Whitney, 1994; Foster and Motzkin, 2003; Fraver et al., 2009). Old-growth forests contain natural gaps of different sizes, which can be location-specific (wet, rocky, sandy) or part of a dynamic ecological trajectory due to disturbances, such as fire, windstorms, beaver activity, and insect outbreaks (Whitney, 1994; Boose et al., 2001; Frelich, 2002; Seymour et al., 2002; D’Amato et al., 2017). As a result the forest ecosystem remains intact and resilient, supporting widespread re-sprouting and recovery of trees.
Openland and early-successional habitats were not common before the arrival of Europeans in the Northeast or Upper Great Lakes (Cooper-Ellis et al., 1999; Foster et al., 2002; Faison et al., 2006; Anderson et al., 2018; Oswald et al., 2020b; Frelich et al., 2021). Early-successional habitats characterized about 1–4.5% of the Northeast, with greater amounts in coastal pine barren communities of Cape Cod, Long Island, and New Jersey (Lorimer and White, 2003). About 32% of the entire states of Minnesota, Wisconsin, and Michigan was represented by early-successional habitats, mostly in the savannas and prairies in the southern and western parts of the region. To the north, early-successional habitats were found in tens of thousands of patches of shorelines, marshes, sloughs, bogs, cliffs, and fire-prone sand plains (Veatch, 1928; Curtis, 1959; Marschner, 1975). Thus, the region had both dense forests and permanently open habitats maintained by the physiography of the landscape, including prairies and savannas maintained before European settlement by frequent fires—now almost absent due to agricultural conversion of the land. It is important to note that these open habitats were not early-successional stages for forests.
Native people living in the Great Lakes and the Northeast practiced subsistence hunting, fishing, and plant gathering, as well as burning and small-scale farming. Their population was less than 1% of the current population and largely centered along the coast and in major river valleys, with localized and modest impacts across most of the region (Whitney, 1994; Lorimer and White, 2003; Milner and Chaplin, 2010; Oswald et al., 2020b; Frelich et al., 2021; Tulowiecki et al., 2022).
The arrival of Europeans generated a radical landscape transformation. Upland areas, densely forested for thousands of years, were cleared for agriculture and kept open by crop cultivation, cattle and sheep grazing, increased burning of (dry) cleared land, and intensive use of remaining woodlands (Foster and Motzkin, 2003; Faison et al., 2006; Rhemtulla and Mladenoff, 2007; Scheller et al., 2008; Curtis and Gough, 2018; Oswald et al., 2020b). By the height of deforestation from 1850 to 80, 30% of northern New England and 40–50% of southern New England had been cleared (Foster et al., 2017), and by 1920 more than 90% of the Upper Great Lakes region was cutover (Greeley, 1925; Frelich, 1995).
Widespread deforestation caused a major shift in vegetation from long-lived and interior forest species to generalist and early-successional species (Thompson et al., 2013; Foster et al., 2017). Many of the latter species had been uncommon before European settlement, others migrated to the region, and some plants that had previously grown only on extreme and rare sites expanded their distribution and became common “old field” species (Marks, 1983). Early naturalists recognized that populations of some wildlife species had increased greatly due to this abundance of human-created early-successional habitats (Peabody, 1839). By the late 19th century, New England agriculture was declining, leaving countless abandoned and overgrown fields, grasslands, heathlands, and shrublands, as well as old-field white pine forests, and dense sprout woodlands. By the mid–20th century, significant areas of cutover forests were acquired by the public and allowed to begin growing back on state and federal lands (Titus, 1945; Jones, 2011; Knowlton, 2017). Today, millions of acres of forest are a globally significant example of ecological recovery, and the extent of early-successional habitats has declined accordingly (McKibben, 1995; Foster et al., 2002; Litvaitis, 2003; Foster et al., 2017). Consequently, species that depend on early-successional habitats have been returning to more historic levels, including the Bobolink (Dolichonyx oryzivorus), Eastern Meadowlark (Sturnella magna), Golden-winged Warbler (Vermivora chrysoptera), Yellow-breasted Chat (Icteria virens), and New England Cottontail (Sylvilagus transitionalis) (Figure 1; Litvaitis, 1993; Foster, 2002; Askins, 2011; Foster, 2017).
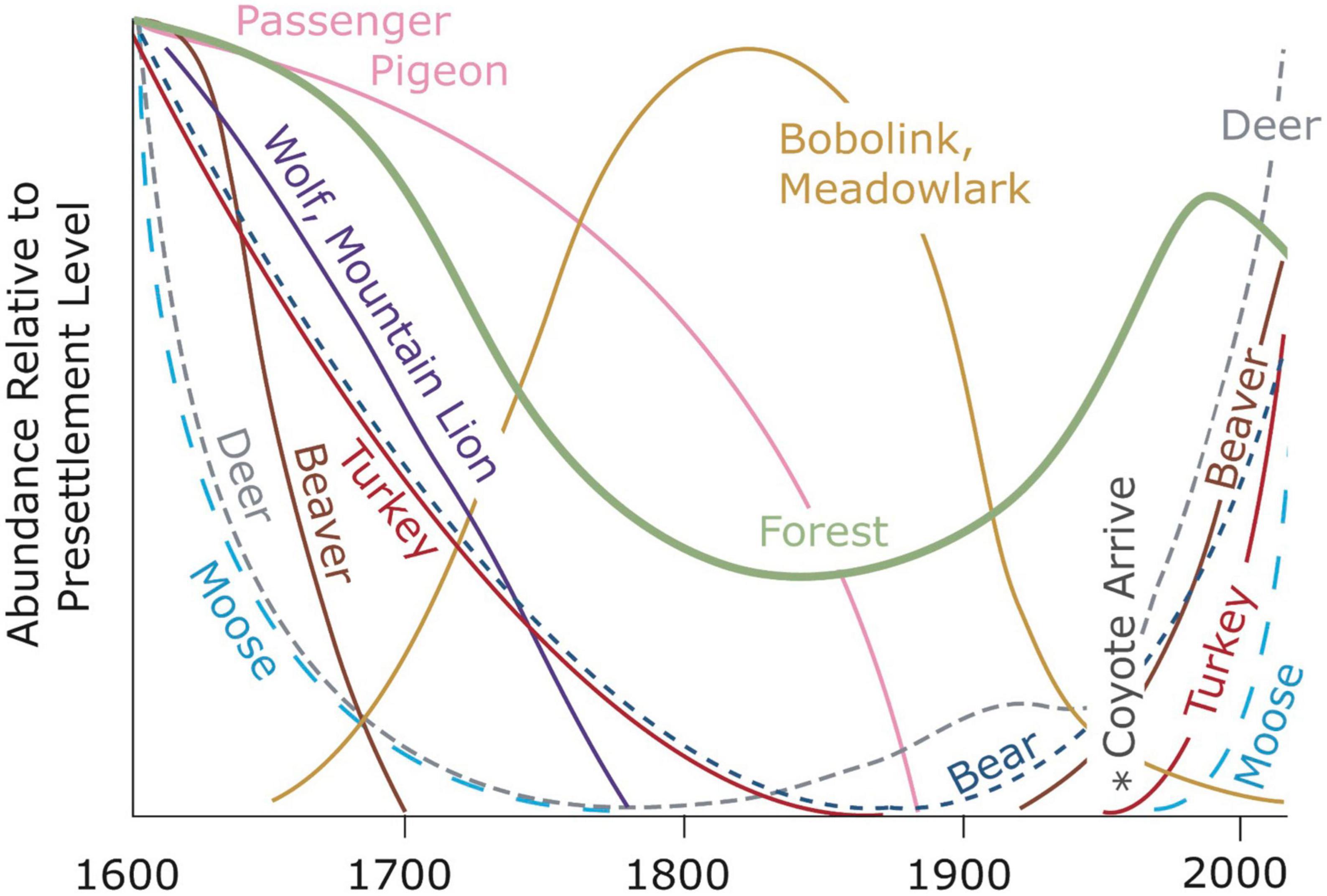
Figure 1. Changes in land cover and wildlife dynamics in New England from ∼1600–2000. The green line shows the abundance, decline and then recovery of forest in New England, which paralleled the population changes in moose, beaver, and deer. The inverse trend is found in openland (early-successional) species, typified by bobolink and meadowlark. The inverted U shows the low population densities of these and other early-successional species before European settlement, increasing populations of these species as forests were cleared, and a return to lower populations as the forests have grown back. *The coyote is not native to New England. Adapted from Foster et al. (2002); also see Figure 2.
Although old-growth forests were the predominant ecological condition before European settlement, they are extremely rare today (Frelich, 1995; Dunwiddie et al., 1996; Davis, 2003; D’Amato et al., 2006; DellaSala et al., 2022b), much less common than younger habitats (Figure 2). A few relatively large tracts of old-growth and protected recovering forests survive in New York, Michigan, and Minnesota, but just small fragments remain across vast regions including all of New England. However, many mature forests are poised to transition to old-growth, and some are undergoing this transition (Ducey et al., 2013; Gunn et al., 2014). This can occur through a straightforward process of forest development and maturation.
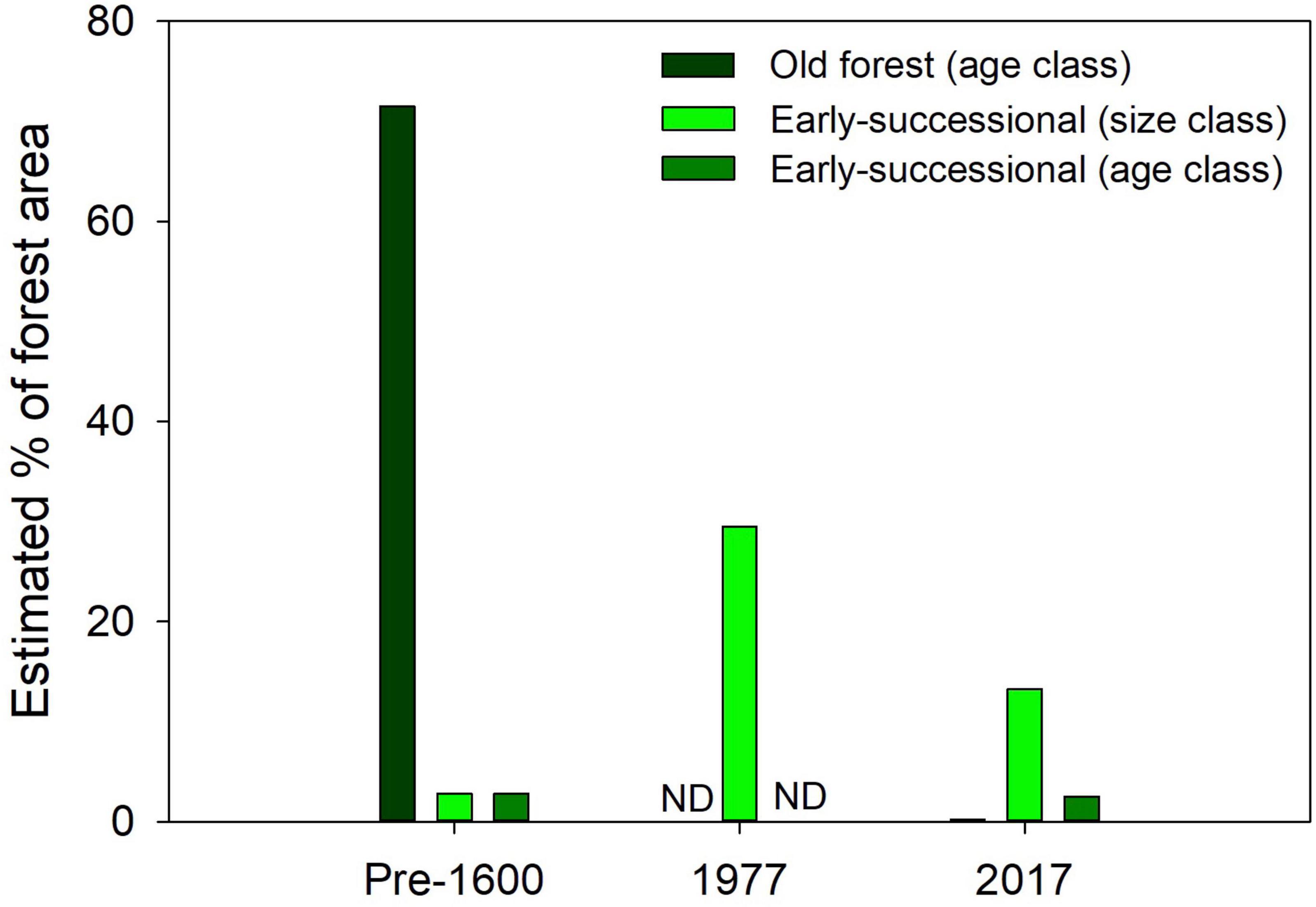
Figure 2. Estimated change in average % of early-successional and old forest habitat from pre-European settlement to current times in the Northeast US as extracted from multiple sources. Old forest is defined > 150 years old. The 1600 estimate for early successional forest is based on “seedling-sapling (1–15 years)” age class (Lorimer and White, 2003). The 1977 estimate is based only on “seedling-sapling” size class as reported in Oswalt et al. (2019); age class data were unavailable (ND = no data). Current estimates (2017) reflect two sources: Oswalt et al. (2019) and USDA Forest Service (2022b) wherein early successional forest (size class) reflects “seedling-sapling,” the smallest class defined by the USDA Forest Service; and early successional forest (age class) reflects the 1–15 year age class. Note that while early-successional forest declined since 1977, it is similar and perhaps multiple times higher than pre-settlement values; and recent accounting is likely an underestimate: it does not include areas such as highway medians, small patches, or corridors (< 0.4 ha or < 36.5 m wide) that may be found on properties such as golf courses, farms, public and private institutions, and private yards. In contrast, old forest habitat has decreased dramatically (old forest data are barely visible in 2017 on this scale).
In the Northeast, forests older than 150 years of age cover only about 0.3% of New England and 0.2% of the Mid-Atlantic region (USDA Forest Service, 2022b). Old-growth forests cover a scant 0.06% of Connecticut (Ruddat, 2022). A Massachusetts survey found a mere 1,100 acres of old-growth forest in 33 small stands, comprising just 0.02% of the land base (D’Amato et al., 2006). Most of the old-growth forest in the Northeast is found in the Adirondack and Catskill parks in New York (Dunwiddie et al., 1996; Davis, 2003; Keeton et al., 2011; New York Department of Environmental Conservation, 2021). In the Upper Great Lakes region, only about 1.9% of the currently forested area remains as primary forest that was never logged. Including secondary forests, approximately 5.5% of the northern hardwood forest type is older than 120 years of age, compared to 89% in the presettlement forest; for red-white pine this is 2.5% versus 55%. For all forest types, about 5.2% is old-growth compared with 68% before European settlement (Frelich, 1995).
1.2. Genesis and rationales of the early-successional habitat campaign
1.2.1. Genesis of the campaign and the “Young Forest Initiative”
A concerted campaign is working to slow and reverse the natural decline in early-successional habitat and species that accompanied the regional reduction in deforestation, intensive forestry, and agriculture. This campaign is promoting early-successional habitat through multiple activities: clearcutting, “group selection,” and other forms of patch clearfelling in established forests; intensive “mechanical treatments” such as brushhogging and mowing; and herbicide application and prescribed fire in successional habitats and younger forests, which are often accompanied by other mechanical treatments (DeGraaf and Yamasaki, 2003; Oehler et al., 2006; American Bird Conservancy, 2007; Schlossberg and King, 2007; King et al., 2011; Yamasaki et al., 2014).
These intensive management activities have long been advocated to benefit popular game species that favor early-successional habitats, such as the American Woodcock (Scolopax minor), Ruffed Grouse (Bonasa umbellus), and White-tailed Deer (Odocoileus virginianus) (Lenarz, 1987; Caron, 2009; Derosier et al., 2015). In the last decade, an expanded management campaign has included popular non-game species that also use these habitats (see Section “1.2.2 Rationale for forest-clearing: halt the decline of specific wildlife species” below). This campaign involves an increasing number and diversity of agencies and organizations, and captures rising amounts of public money from state and federal sources. The goal is to maintain the recent historical and degraded condition of the natural forests of the region.
A key milestone in the genesis of this campaign was the 2008 American Woodcock Conservation Plan (AWCP; see Table 1 for Abbreviations), published by the Wildlife Management Institute (WMI) in collaboration with game management agencies and sportsmen’s organizations (Kelley et al., 2008). The goal is to increase American Woodcock populations to early 1970s levels by clearcutting 11.2 million acres of forest in the Northeast and Upper Great Lakes regions—an area larger than the state of Maryland. WMI also launched the Upper Great Lakes Woodcock and Young Forest Initiative (YFI) to gain public support for the creation of early-successional habitats in Michigan, Minnesota, and Wisconsin (Wildlife Management Institute, 2009, 2010).
Wildlife Management Institute (WMI) soon began expanding the YFI to a national campaign (Gassett, 2018; Weber and Cooper, 2019). Recognizing the controversial nature of such widespread forest-clearing, the organization hired a marketing firm to “shape an overall communications strategy” (Seng and Case, 2019). This firm administered opinion surveys and focus groups that showed most forest landowners value beauty, scenery, nature, and biodiversity far more than logging or financial return. A plan was then devised to promote early-successional habitats through an extensive network of partnerships. Terms which focus group participants found unappealing, such as clearcutting, early-successional habitats, shrub, and scrub, were replaced with the more appealing “young forests.” Simple and positive language emphasized forest “health,” wildlife, habitat diversity, and scientific-sounding outcomes. A pseudo-historical pitch was crafted to emphasize the decline of once common and familiar species without acknowledging the highly artificial and historically anomalous nature of their former abundance (see Table 2 for more details). Numerous publications were produced, such as, “Talking About Young Forests,” to help “natural resource professionals…effectively advocate for creating and managing young forest habitat on public and private lands” (Oehler et al., 2013).
In 2012, YFI inaugurated the “youngforest.org” website, aimed at persuading target audiences to support the campaign (Young Forest Project, 2012). Within a decade, the YFI had recruited more than 100 “partners” (Supplementary material 1, Young Forest Project, 2022a). These are primarily traditional forestry and game species management interests, such as timber companies (Lyme Timber Company, 2017; Weyerhaeuser Company, 2020), federal and state forestry agencies (New York Department of Environmental Conservation, 2015; USDA Forest Service, 2018), federal and state wildlife agencies (U.S. Fish and Wildlife Service, 2015c; Connecticut Department of Energy and Environmental Protection, 2021b), and sportsmen’s organizations (Russell, 2017; Weber and Cooper, 2019). All of these partners benefit from forest-clearing through increased profits from timber sales, larger agency budgets, more staff, direct payments for creating young forest habitat, or elevated populations of desired game species (see Supplementary material 1 for state-by-state examples of forest-clearing).
The YFI has attracted generous financial support from a wide range of public agencies, private organizations, and large corporations such as Richard King Mellon Foundation, U.S. Forest Service, U.S. Fish and Wildlife Service, American Forest Foundation, and Shell Oil Company [see Connecticut Department of Energy and Environmental Protection (2018); New Jersey Audubon (2018); National Fish and Wildlife Foundation (2022b)]. In addition to activities on public lands, money is directed to land trusts (New England Cottontail, 2021) and private landowners (Natural Resources Conservation Service, 2018) through numerous state and federal sources. Much of this activity, supported by the significant money available for forest-clearing for early successional habitats (American Bird Conservancy, 2015; Natural Resources Conservation Service, 2019; Ruffed Grouse Society, 2022), engages broad support by well-intentioned landowners and conservationists by portraying this clearing as “restoration” to retain or save declining species (Smith, 2017; Weidensaul, 2018). There is little acknowledgment that, although these species are truly declining, they were artificially elevated in their abundance by colonial and relatively modern land-use practices that were abandoned in 19th and especially the 20th century.
Currently, every state in the Northeast receives substantial funding for early-successional habitat projects, either through direct federal programs or shared stewardship agreements (Fergus, 2014; USDA Forest Service, 2021b, 2022e; National Fish and Wildlife Foundation, 2022a; Sharon, 2022; Young Forest Project, 2022b). Even as forests are naturally recovering and helping to mitigate climate change in the absence of intensive logging, the momentum and money to clear forests and create open habitats is growing. For instance, the Infrastructure Investment and Jobs Act (2021) authorizes billions of dollars to increase logging for “wildfire risk reduction,” “ecosystem restoration,” and production of “mass timber” buildings (Parajuli, 2022; USDA Forest Service, 2022a). These massive programs will significantly increase early-successional forest habitats across the country, including in the Northeast and Upper Great Lakes regions. In contrast, there appear to be few resources devoted to protecting and expanding mature and old-growth forests.
Meanwhile, forest and wildlife managers–and a surprisingly large number of scientists—contend that the campaign to artificially expand early-successional habitats is vital because: (1) numerous wildlife species that depend on these habitats are declining and potentially endangered (Fergus, 2014), (2) the “restoration” of such habitats is needed to halt and reverse this decline (Young Forest Project, 2022c), and (3) the history of the region includes significant disturbance and presence of early successional habitats (Oehler et al., 2006). However, as noted previously, targeted population increases in specific species are mismatched generally with longer historical trends (Figure 1). Below is a more specific review of the rationales for these assertions, along with questions and concerns that have been raised in response.
1.2.2. Rationale for forest-clearing: Halt the decline of specific wildlife species
The primary justification cited for forest-clearing is that populations of many species needing early-successional habitats are declining (King et al., 2001; King and Schlossberg, 2014; Yamasaki et al., 2014; North American Bird Conservation Initiative, 2019; Rosenberg et al., 2019). Monitoring populations of species and preventing decline is a legitimate concern. Failure to take action in the past has allowed many species to become endangered or go extinct. Therefore, if these assertions are true, if losing species is a possibility, and if there are no plausible alternative explanations, a reasonable conclusion is that some species may need additional early-successional habitat to survive and thrive and would therefore justify habitat experiments and intensive habitat management programs to protect these species.
It is important to recognize that documentation of the decline of early-successional species is almost invariably based on a very recent baseline, generally dating to the 1960s or later (DeGraaf and Yamasaki, 2003; Massachusetts Audubon Society, 2013; North American Bird Conservation Initiative, 2014; Rosenberg et al., 2016, 2017, 2019; Connecticut Department of Energy and Environmental Protection, 2019; Sauer et al., 2020; Littlefield and D’Amato, 2022). This time period is a convenient benchmark because it falls within the lived experience of many of today’s wildlife and forest managers and the landowners and public that they are trying to reach. It also coincides with the first annual North American Breeding Bird Survey (BBS), which took place in 1966 (Sauer et al., 2013). Prior to this time there was little reliable quantitative information on most bird populations (Foster, 1995; Foster et al., 2002; Dunn et al., 2005).
Although useful in many ways, the BBS is flawed as a truly long-term baseline for bird population trends. An ongoing deficiency is that the BBS is not a representative sampling of the broader landscape: it surveys habitats primarily near secondary roads and leaves out a wide range of habitats (Dunn et al., 2000; Dunn et al., 2005; Sauer et al., 2017). Furthermore, the quality of the data is inconsistent because volunteer observers have varying abilities (Dunn et al., 2000), including age-related declines in bird detection abilities and mobility (Farmer et al., 2014).
Beyond these problems, using a mid–1960s baseline for wildlife populations is fundamentally misguided. Every history of the region shows that at the time of the first BBS the Northeast and Upper Great Lakes regions were (and still are) in transition—with unnaturally high amounts of early-successional habitat such as abandoned farmland and forests recovering from intensive clearing and historically anomalous levels of fire, grazing and other human disturbances (Whitney, 1994; Foster et al., 2002; Mladenoff et al., 2008; Mladenoff and Forrester, 2018). As a result, the 1960s populations of wildlife species that occupied and thrived on such habitats were likely inflated well beyond what they would be in natural forests before European settlement (Litvaitis, 1993). This set the stage for a decades-long dramatic downward population trend due to recovering landscapes that are not yet within their true ecological trajectories (Massachusetts Audubon Society, 2013; Connecticut Department of Energy and Environmental Protection, 2019; Rosenberg et al., 2019).
Wildlife population trends since the 1960s need to be viewed in the context of a much longer timeframe (Schulte et al., 2005a,b), as has been provided by many superb studies of changes in major tree species for the region (Mladenoff et al., 2008; Thompson et al., 2016). For examples, Figure 1 spans the period from 1600 to today, displaying dual juxtaposed bell curves—one with forests (and some forest-associated species) steadily declining until the mid–1800s and then recovering through present day, and the other an inverse curve showing early-successional species populations increasing and then declining during that period (Foster et al., 2002). The recovery of the forested landscape may be causing previously inflated early-successional populations to restabilize closer to their natural baseline prior to the arrival of Europeans and under the conditions in which these species evolved.
Despite these caveats, State Wildlife Action Plans (SWAPs) rely heavily on the erroneous 1960s baseline for gauging the status of early-successional species. A SWAP must be filed with the U.S. Fish and Wildlife Service by each state to qualify for a number of major federal grants (The Wildlife Society, 2017). SWAPs include a list of Species of Greatest Conservation Need (SGCN), encompassing species that appear on federal or state lists as threatened or endangered, as well as those which are deemed rare, declining, or vulnerable to decline within that state (Minnesota Department of Natural Resources, 2016). SWAPs are useful sources of information for wildlife managers, but they are limited in scope, focusing on individual species within one state, rather than regional and national biodiversity (Pellerito and Wisch, 2002; Paskus et al., 2015).
With their mid–1900s baseline, SWAPs skew state-level biodiversity policies and programs toward management for conditions of that era. As noted, this is comfortable for wildlife and land managers who grew up during and recently after that time and appeals to many members of the public. However, this has created a false sense of endangerment for early-successional species that: (1) are common and of “least concern” based on International Union for the Conservation of Nature (IUCN) criteria (IUCN, 2012); (2) were historically uncommon (i.e., naturally rare, and at a natural population level); or (3) are non-native (i.e., did not occur in that state prior to European settlement and contribute to under-estimating populations of mature and old-growth forest species). The supposedly grave state of these species is reinforced further by the YFI. For example, its handbook for wildlife managers includes a list of “89 species of wildlife classified as [SGCN] that require young forest habitat to survive and breed” (Oehler et al., 2013). Although these species use early-successional habitats, only a small number of them are listed under the federal Endangered Species Act (U.S. Fish and Wildlife Service, 2022b), and many of them fall into the following categories:
• They are at the edge of their range in a particular state and were temporarily increased in numbers by past forest-clearing, but are now abundant and widely distributed across their range, such as the Yellow-breasted Chat (Icteria virens) in Connecticut or the Prairie Warbler in Massachusetts (Nolan, 1978; Southwell, 2001);
• They were probably rare in, or not native to, a particular state before the arrival of Europeans and moved in as a result of the widespread forest clearing in the 19th century, such as Golden-winged Warbler (Askins, 2011) and Chestnut-sided Warbler (Litvaitis, 1993; Foster et al., 2002) in New England;
• They have declined in population and distribution since the 1960s, but had a limited distribution in the landscape before European settlement, such as the New England Cottontail (Sylvilagus transitionalis) (Figure 3; U.S. Fish and Wildlife Service, 2015a);
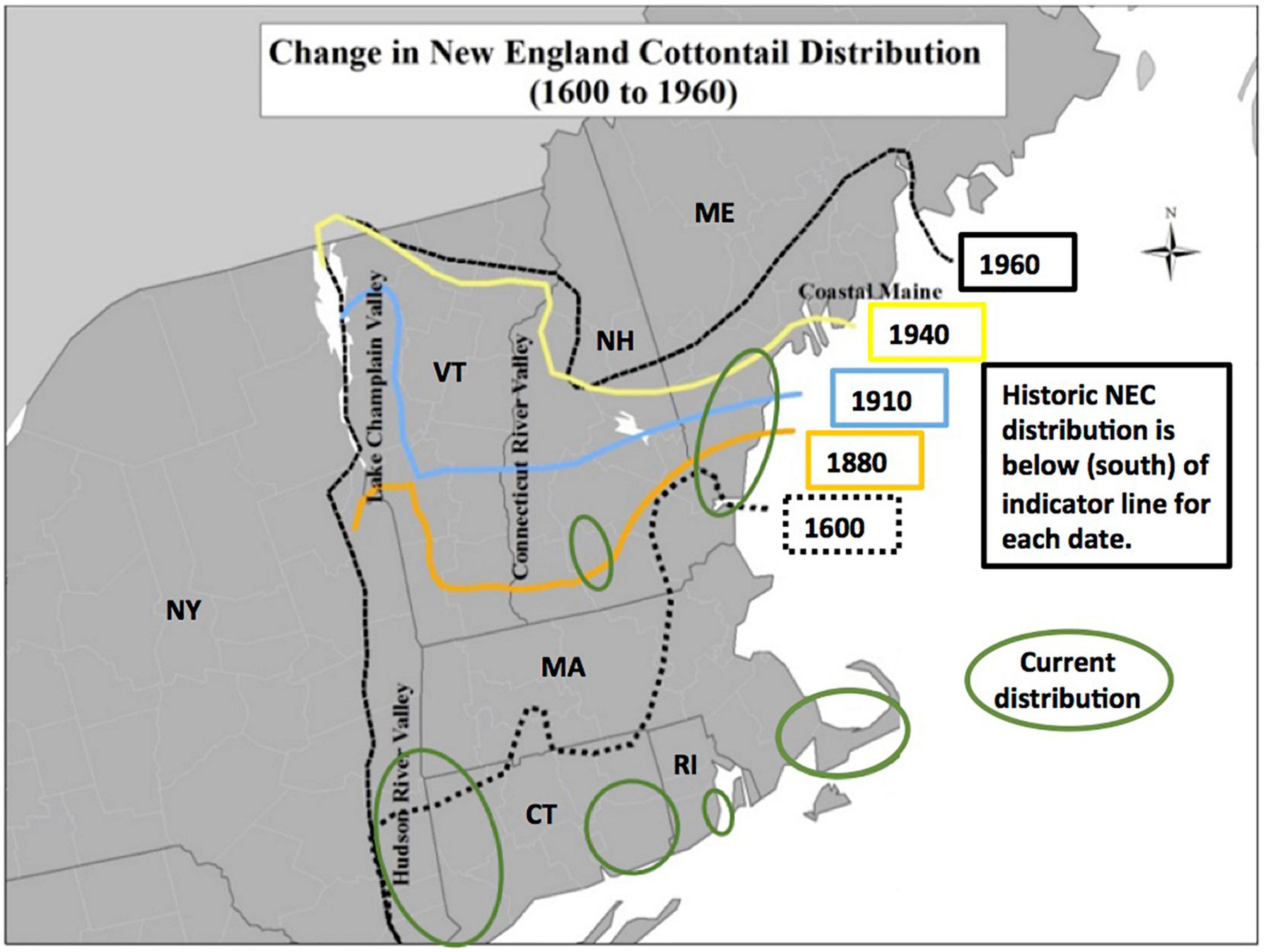
Figure 3. Changes in New England Cottontail (NEC) distribution over time. The estimated range of New England Cottontails (NEC) documented circa ∼1600 (below the dashed line) included primarily Connecticut (CT) and Rhode Island (RI), and part of Massachusetts (MA). The distribution expanded dramatically northward following European settlement and land use (∼1620–1960) to include Vermont (VT), Maine (ME), New Hampshire (NH), and into New York (NY; Hudson River Valley and Lake Champlain Valley). This dramatic expansion was followed by range contraction (∼1960–2022) with forest regrowth and urban and suburban development. Green ovals represent the current documented distribution of NEC. Note that parts of current range still extend outside of pre-European settlement bounds, particularly in ME. NEC distribution map adapted from U.S. Fish and Wildlife Service (2015a,b).
• They have declined from past unnaturally high mid–20th century populations, but continue to be abundant and widely distributed, such as the American Woodcock (Seamans and Rau, 2018), Northern Bobwhite (Colinus virginianus) (Giocomo et al., 2017), Whip-poor-will (Caprimulgus vociferus), Bobcat (Lynx rufus), Smooth Green Snake Opheodrys vernalis), Eastern Buck Moth (Hemileuca maia), and Wild Lupine (Lupinus perennis) (NatureServe, 2022);
• Their declines can be attributed to other causes besides lack of habitat, such as the impact of West Nile virus on Ruffed Grouse populations (Stauffer et al., 2018);
• They benefit from limited, scientifically-backed habitat management, not forest-clearing, as with restoration of Wild Lupine (Lupinus perennis) for the protection of specialist butterflies (Pavlovic and Grundel, 2009; Plenzler and Michaels, 2015).
Including species of questionable “conservation need” on state SGCN lists has helped to validate and encourage forest-clearing and other intensive management to expand early-successional habitats. For instance, a major goal of the Connecticut SWAP is to “keep common species common” (Connecticut Department of Energy and Environmental Protection, 2015), which has been translated into an intensive focus on forest-clearing (Neff, 2017) and is promulgated in agency publications such as “The Clear Cut Advantage” (Connecticut Department of Energy and Environmental Protection, 2013). Many federal and state agencies have goals for significantly expanding early-successional habitats from current levels (USDA Forest Service, 2018; Massachusetts Division of Fisheries and Wildlife, 2022b) without clear plans for monitoring and maintaining the habitat they are creating.
A further problem is that forest-clearing advocates exaggerate the number of species that “require” or “need” early-successional habitat. For instance, the YFI website asserts, without evidence, that, “if we fail to actively create and renew young forest…[m]any songbirds will rarely be seen or heard [and] the New England Cottontail and Appalachian Cottontail could…go extinct (Young Forest Project, 2022c). Another YFI publication claims that, “more than 40…kinds of birds need young forest…” (Fergus, 2014), yet only 12 species of birds in the Northeast are actually considered early-successional forest specialists (Askins, 1993).
Among the species most commonly cited to justify large-scale forest-clearing are the American Woodcock, Ruffed Grouse, Golden-winged Warbler, and New England Cottontail. As discussed in detail in Supplementary 3, whether this strategy is necessary or desirable is open to question for each of these species. For example, the woodcock (Seamans and Rau, 2018), grouse (Wiggins, 2006), and cottontail (Fuller and Tur, 2012) are game species subject to being killed by hunters while the cause and potential solutions to warbler declines are uncertain (Streby et al., 2016).
There is a contention that forest-clearing not only “restores” early-successional species, but also benefits many interior species (Chandler et al., 2012; Stoleson, 2013; King and Schlossberg, 2014; Yamasaki et al., 2014; Schlossberg et al., 2018; New Jersey Department of Environmental Protection, 2018). Yet, these claims are based on a few studies that are limited in their targeted species, timeframe, and geographic scope, and rarely examine alternative hypotheses. For instance, although interior forest bird species may use available early-successional habitats to some extent, there is little evidence that such habitats are favored or necessary for their survival (Vega Rivera et al., 1998; Marshall et al., 2003; Dorazio et al., 2015).
Aside from questions regarding its necessity, long-term effectiveness, and unintended consequences, the intense focus on creating and restoring early-successional habitats diverts resources from exploring strategies to address other factors that are known to impact wildlife populations. These factors include food availability, over-hunting, disease, climate change, environmental toxins, and myriad other reasons that are not connected simply to the areal extent of early-successional habitat.
1.2.3. Rationale for forest-clearing: Halt decline of early-successional habitats
Before European settlement, countless small patches of early-successional habitats were created in the forests of the Northeast and Upper Great Lakes regions on a continuing basis, including by wind and ice storms, insect infestations and disease, drought, floods, fire, and to a lesser extent grazing by large mammals (Runkle, 1982; Peterken, 1996). Contemporary studies of old-growth forests in the eastern U.S. suggest such small gaps are less than 0.1 acre in size. Larger openings were created by beaver impoundments and at intervals of hundreds of years by catastrophic windstorms and tornados. While uncommon in the Northeast outside of coastal pine barren communities, fire occurred every few decades and sometimes created large openings in the Upper Great Lakes region (Frelich, 1995; Lorimer and White, 2003). Native people generally caused minimal forest disturbances except around settlements scattered along coasts and river corridors (Motzkin and Foster, 2002; Parshall and Foster, 2002; Munoz and Gajewski, 2010; Oswald et al., 2020b; Frelich et al., 2021).
Advocates of clearing forests for early-successional habitats assert that natural and pre-European disturbances have been greatly attenuated and, therefore, managers must step in to create them (DeGraaf and Yamasaki, 2003; Oehler et al., 2006; Fergus, 2014; King and Schlossberg, 2014; Littlefield and D’Amato, 2022). While these habitats are reduced from their zenith in the 1800s and early 1900s (Foster et al., 2002; Litvaitis, 2003; Lorimer and White, 2003), extensive early-successional habitats still exist and are continuously produced, naturally and by widespread human activity. Natural disturbances such as storms, insect infestations and disease (including many novel non-native types that were not present when Europeans arrived), floods, and beaver impoundments, continue to create forest openings (Whitney, 1994; Askins, 2000; Frelich, 2002; Zlonis and Niemi, 2014; Wilson et al., 2019). Many types of human disturbances including farming, forest harvesting, and the expansion of electrical transmission lines provide additional extensive areas of early-successional habitats.
About 13% of forest area in the Northeastern United States is currently in the smallest (seedling-sapling) size class (Oswalt et al., 2019), a decline of more than 50% over the past 40 years, but several times higher than estimated presettlement values (Lorimer and White, 2003; Figure 2). Early-successional habitats in the Upper Great Lakes regions today are more difficult to quantify, because much of the southern and western portions of the three states are covered by savannas, prairies, and agricultural land. However, a study found that 4.4% of the area of Michigan north of the prairie-hardwood transition is characterized by forests less than 20 years old (Tavernia et al., 2016), and forests less than 20 years old are estimated to cover 12% of all forested lands in Wisconsin and Minnesota, respectively (Kilgore and Ek, 2013; Wisconsin Department of Natural Resources, 2020; USDA Forest Service, 2022b).
Approximately 65% of timber removals in the Northeast detected in U.S. Forest Service Inventory Data (FIA) are commercial clearcuts, shelterwood, high-grade, group selection, or pre-commercial thinning treatments (Belair and Ducey, 2018)—all major sources of early-successional habitats. In the Northeast and Upper Great Lakes, tens of thousands of acres of these habitats are created each year by the clearcutting of public and private timberlands—more than 10,000 acres in the national forests alone (USDA Forest Service, 2003; USDA Forest Service, 2017). Among the nine Northeast states, almost 19 million acres (16%) are farmland, most of which was formerly forested (U.S. Department of Agriculture, 2020), and about one-third of agricultural lands provide a mosaic of early-successional habitats such as grassland, woodland, wetland, and other open habitats (Brady, 2007; Jeswiet and Hermsen, 2015).
Expansive early-successional habitats are also the byproduct of urban and industrial developments. Examples include pipeline and powerline corridors (King et al., 2009; Askins et al., 2012), highway rights of way (Huijser and Clevenger, 2006; Amaral et al., 2016), golf courses (Tanner and Gange, 2005), greenways (Mason et al., 2007), wind and solar power arrays (South Carolina Department of Natural Resources, 2020; Zaplata and Dullau, 2022), military bases (Young Forest Project, 2022d), airports (Cousineau, 2017), and reclaimed strip mines (Bulluck and Buehler, 2006). Most of these development categories are not included in current inventories of early-successional habitats.
Additional factors are expected to increase the inventory of early-successional habitats. The forests of New England, for example, are rated as “above average” in health, but climate change is projected to have widespread impacts that will expand early-successional habitats (Janowiak et al., 2018; USGCRP, 2018). These impacts include major disturbances from storms (Miller-Weeks et al., 1999; Koches, 2019; Seitz, 2019), increased precipitation and flooding (National Wildlife Federation, 2009; Connecticut Department of Energy and Environmental Protection, 2020; Moustakis et al., 2021), periods of extreme heat and drought (Baca et al., 2018), insect and disease outbreaks (Paradis et al., 2008; Massachusetts Department of Conservation and Recreation, 2018), the introduction of new invasive species (Seidl et al., 2017), and shifts of vegetation and habitats northward (Chen et al., 2011; Toot et al., 2020). SWAPs and the YFI do not take into account such climate impacts.
Another potential source of early-successional habitats is the use of intensive forest management to increase climate “adaptation” and “resilience” of forests, which includes clearcutting, thinning, prescribed burning, and “assisted migration” through tree plantings (Foster and Orwig, 2006; USDA Forest Service, 2021a, 2022c; Climate Change Response Network, 2022a,b, Massachusetts Department of Conservation and Recreation, 2022; Northern Institute of Applied Climate Science, 2022; USDA Forest Service, 2022c). Such intensive forest interventions are, to date, mostly conceptual and experimental (Millar et al., 2007, D’Amato et al., 2011; Sheikh, 2011; Schwartz et al., 2012; Park and Talbot, 2018; Aquilué et al., 2020; Palik et al., 2022). Many questions remain regarding their economic, ecological, and legal and administrative feasibility (Handler et al., 2018). A prudent course would be to move cautiously with such novel strategies while expanding protection for mature and old-growth forests, which have a high degree of ecosystem integrity, genetic diversity, and adaptive capacity (Mosseler et al., 2003a; Thompson et al., 2009; Rogers et al., 2022).
An increasingly common rationale for forest-clearing is that it is necessary to recreate the way that Native people lived in relationship with the land. This is based on the extensively criticized hypothesis that long before European settlement, humans were deliberately managing most of the Northeast and Upper Great Lakes landscape using forest burning and clearing to improve habitat for favored plants and animals (Day, 1953; Mann, 2005; Abrams and Nowacki, 2008; Poulos and Roy, 2015). Some accounts take the idea even further, contending that by 1600, North America was “a humanized landscape almost everywhere” (Denevan, 1992), managed by Native people as a “garden” (Pyne, 2000), with virtually no “natural” plant communities (Williams, 2002). According to this view, the cessation of widespread and frequent pre-European burning and the reforestation of large parts of the region (which had been cleared after European settlement) have resulted in a massive loss of early-successional habitats and species, seriously threatened major plant communities, and reduced native biodiversity (Brose et al., 2001; Poulos and Roy, 2015; Abrams and Nowacki, 2020). The assumed loss of management by Native people is also cited as a major cause of the transition now underway of many oak forests to forests dominated by shade-tolerant species (Abrams, 1992; Brose et al., 2001; Abrams, 2005; Nowacki and Abrams, 2008).
Native burning and other subsistence practices, such as hunting, fishing, plant gathering, and small-scale farming had notable ecological impacts in the immediate vicinity of native encampments and settlements in the Northeast and Upper Great Lakes regions (Whitney, 1994; Lorimer and White, 2003; Oswald et al., 2020b; Frelich et al., 2021; Tulowiecki et al., 2022). However, modern land managers seem to be inappropriately misinterpreting a set of novel landscape conditions created by European land use over the last few centuries as having pre-European origins (Chilton, 2002; Oswald et al., 2020b; Cachat-Schilling, 2021). Extrapolating this misinterpretation to a regional scale has led to claims of widespread and intensive Native manipulation for millennia before European settlement. Unfortunately, these sweeping assumptions are being used to justify large-scale clearing and prescribed burning of established and recovering forests (Pyne, 2000; Brose et al., 2001; Williams, 2002; Oehler et al., 2006; Poulos and Roy, 2015; Abrams and Nowacki, 2020). In 2019 alone, 365,306 acres of forest—an area larger than Rocky Mountain National Park—were burned through prescribed fire in the Northeast and Upper Great Lakes, according to state forestry agencies (Melvin, 2020). Examples of major prescribed fire projects are found in Connecticut (Connecticut Department of Energy and Environmental Protection, 2021a), Massachusetts (Clark and Patterson, 2003), Michigan (Michigan Department of Natural Resources, 2022), and Vermont (USDA Forest Service, 2022d). This is in addition to the significant expanses of forest that are cleared under the premise of creating early-successional habitat.
Beyond the greater risks from mechanized modern forest management, there is significant controversy regarding the hypothesis of intensive and extensive management of the pre-European landscape by Native people (cf., Cachat-Schilling, 2021). For example:
• The presumption that the presettlement landscape was dominated by agriculturally based Native people who regularly burned large areas relies primarily on written or oral accounts by European explorers, travelers, and colonists. The vast majority of these narratives were not objective descriptions, but were vague, subjective, biased, or even meant to promote profit-making enterprises (Russell, 1981; Forman and Russell, 1983; Russell, 1983; Vale, 1998; Vale, 2002; Barrett et al., 2005; Munoz et al., 2014; Foster, 2017).
• Maintenance of the envisioned anthropocentric landscape would have required Native communities to move every 10–20 years, thereby creating extensive early-successional habitat and a wide variety of even-aged forest patches. This scenario is not supported by archeological studies of pollen and charcoal (Chilton, 2002; Oswald et al., 2020b).
• Localized burning and other land use did commonly occur in some population centers along the New England coast where maize agriculture had developed, the estuaries of New York, New Jersey, Delaware, and Maryland, around the eastern Great Lakes, and along major rivers (Russell, 1981; Motzkin and Foster, 2002; Milner and Chaplin, 2010; Munoz and Gajewski, 2010). However, throughout much of the rest of the Northeast and Upper Great Lakes regions, there is no evidence of significant land clearing or agriculture (Chilton, 2002; Parshall and Foster, 2002; Lorimer and White, 2003; Faison et al., 2006; Matlack, 2013; Oswald et al., 2020b). Rather, pollen and charcoal studies show that the vast interior of these regions had a dispersed, low-density population that was seasonally mobile and utilized native resources, not agriculture (Milner and Chaplin, 2010; Foster, 2017; Oswald et al., 2020b; Frelich et al., 2021). Archeological evidence indicates that many Native settlements in these regions are a relatively recent phenomenon—for example, Iroquois settlement began during the last millennium (Warrick, 2000; Bruchac, 2004; Jordan, 2013) and New England coastal settlement was likely encouraged by trade with Europeans (Foster, 2017).
• Pollen and charcoal studies as well as fire records indicate that fire activity before the arrival of Europeans tracked climate and vegetation at broad scales, rather than changes in the size of Native populations (Oswald et al., 2020b; Frelich et al., 2021). Indeed, the period of greatest Native population, shortly before the time of European colonization, was one of relatively low fire activity. At smaller spatial scales, particularly near the coast, some pollen records do show relatively high fire activity just prior to European settlement in areas of higher human population densities (Stevens, 1996; Lorimer and White, 2003; Parshall et al., 2003). Sites on steep slopes in the Appalachians have both a pre-history and a historic pattern of frequent crown and ground fires (Delcourt and Delcourt, 1998; Shumway et al., 2001; Buckley, 2010). Overall fire activity spiked after forest-clearing by European settlers created dry and flammable early-successional habitats, spiked again in the late 19th and early 20th centuries with the expansion of fire-prone abandoned farmlands and cutover forests, and has dramatically declined in the last century (Irland, 2013, 2014; Frelich et al., 2021).
• Long before the first colonization of North America 15,000–18,000 years ago, Northeast and Upper Great Lakes ecosystems had evolved and were maintained by climate and natural disturbances (Foster et al., 2002; McEwan et al., 2011; Noss et al., 2014; Pederson et al., 2014; Oswald et al., 2020b). Historical data and pollen studies indicate that before European settlement, forests were mainly characterized by long-lived shade tolerant and moderately shade tolerant species, not fast growing, early-successional and weedy species that would indicate widespread Native burning (Russell, 1983; Foster et al., 2002; Motzkin and Foster, 2002; Parshall and Foster, 2002; Parshall et al., 2003; Faison et al., 2006; Shuman et al., 2019; Oswald et al., 2020b). Oak savannahs along the prairie-forest border in the Upper Great Lakes region were far more widespread than today and likely maintained at least in part by greater frequencies of fire, including burning by Native people (Whitney, 1994; Frelich et al., 2021; Paciorek et al., 2021). However, the current shift of some forests from disturbance-tolerant species to shade-tolerant species can be explained by changes in climate and other factors rather than a lack of human-caused fires (Foster et al., 2002; McEwan et al., 2011; Noss et al., 2014; Pederson et al., 2014; Oswald et al., 2020b).
• Fire-prone ecosystems occupy about 25% of the forested landscapes of northern Minnesota, Wisconsin, and Michigan (Heinselman, 1973; Frelich, 1995; Frelich and Reich, 1995). However, even with the high occurrence of fires, there was still a much higher proportion of old-growth prior to European settlement than today (Frelich, 1995). Approximately 55% of forests were old growth within the 25% of the landscape that is fire prone (pine and oak forests with some aspen birch and spruce). These areas had 100–250 year return times for severe fires, so that only 55% of the stands would reach an age of 120 years or more. There were both natural and human understory burns, which helped maintain the old multi-aged condition in some stands. Elsewhere, for example in northern hardwood forests, where fires were much less common, the proportion of old-growth was much higher and wind storms were the primary disturbance. Severe fires that set succession back to birch and aspen were quite rare in these areas and were confined largely to blowdown areas. Only small proportions of fire-prone forest landscapes in the Boundary Waters Canoe Area Wilderness and Voyageurs National Park had a long history of regular understory burns (Johnson and Kipfmueller, 2016; Kipfmueller et al., 2017).
• In the Northeast, only limited areas are susceptible to fire, such as coastal pine barrens of Massachusetts, New York, and New Jersey, as well as scattered pavement barrens and sandplain communities in upstate New York and the Connecticut Valley (Forman and Boerner, 1981; Motzkin et al., 1999). Climate change and European land use have been the most important agents of change on these landscapes (Motzkin et al., 1999; Parshall et al., 2003).
In summary, current understanding of the role of fire and other disturbances in the Northeast and Upper Great Lakes regions before the arrival of Europeans is based on uneven, area-specific, and often-inconclusive information (Oswald et al., 2020a; Frelich et al., 2021). Available evidence does not support the hypothesis of widespread, intensive, ongoing burning and other land management over millennia by Native people (Cachat-Schilling, 2021). Instead, the evidence points to human use before European colonization limited to areas near settlements and ultimately constrained by a regional human population that is estimated to be less than 1% of the present population (Milner and Chaplin, 2010).
1.2.4. Rationale for forest-clearing: Reduce the prevalence of “mature” forests
Forest-clearing advocates assert that, in parallel with the presumed lack of “young” forests, there is an overabundance of “mature,” and “even-aged” forests across the landscape. They contend that these forests do not provide an adequate diversity of habitats, and that “active management” can “restore” forest diversity and resiliency by “mimicking” natural forest disturbances and conditions (National Commission on Science for Sustainable Forestry, 2007; Fergus, 2014; King and Schlossberg, 2014; New Jersey Department of Environmental Protection, 2018; Rohrbaugh et al., 2020; Littlefield and D’Amato, 2022). Prior to evaluating this rationale it is important to note that a forest termed “even-aged” can include ages that vary by about 20% of the dominant age, and may also include young trees/advance regeneration, dead trees, and a mosaic of habitats (for example, due to insect damage or storms). “Even-aged” does not mean “even-sized” and tree growth is highly influenced by local site conditions for that species. The term “even-aged” can evoke images of a tree farm or a plantation, but natural forests do not have such a uniform structure, particularly those older than 60–80 years. Although 60–80 year old trees may be termed “mature,” or almost “overmature,” they are at far less than half their natural lifespan and likely at far less than 20% of their potential carbon accumulation (Thompson et al., 2009; Leverett et al., 2021). Most important, forests that are relatively even-aged will transition on naturally toward old-growth and uneven-aged condition if simply left alone (Gunn et al., 2014; Catanzaro and D’Amato, 2019).
With these caveats in mind, it is important to determine if and when removing mature or “even-aged” forests has net benefits. In terms of risks, there is considerable evidence that human-created or -maintained habitats do not provide the complexity, resilience, and diversity over long periods of time that are provided by natural forest ecosystems (Nitschke, 2005; North and Keeton, 2008; Thompson et al., 2009; Lindenmayer and Laurance, 2012; Belair and Ducey, 2018; Thom and Keeton, 2020). Moreover, countless interconnected and long-term ecological variables and processes are not well understood or are still simply unknown—and therefore cannot be “replicated” by human intervention with any confidence.
Taken together, long-term monitoring and further research on these issues should be a top priority. After a natural disturbance a forest can be a chaotic jumble of dead and damaged trees, downed wood, and tip-ups—many involving immense old trees and their associated biodiversity above and below ground (Lain et al., 2008; Santoro and D’Amato, 2019). In a natural forest, snags and downed logs and uproot mounds and pits are large and enduring for 100 years or more, there are no large areas of bare ground or scarified soil, and downed wood and vegetation remains on site (Foster et al., 2003). After an extreme event, such as a hurricane, there may be abundant advance regeneration, understory vegetation, and a mix of damaged and undamaged trees. These building blocks help the forest recover and resist the intrusion of invasive species (Plotkin et al., 2013, D’Amato et al., 2017). Even forests with almost no advance regeneration can regenerate rapidly after a major disturbance (Faison et al., 2016).
To summarize, current programs that create new early-successional forest habitats involve clearing established forested areas. These human-made habitats are dramatically different from the old-growth forest habitats with a mosaic of natural disturbances that dominated the landscape of the Northeast and most of the Upper Great Lakes before European settlement. Early-successional habitats have declined since their peak in the 19th and early 20th centuries but they are still widely represented, actively created by natural and human disturbances, likely undercounted, and expected to increase in the future. In light of the concerns discussed above, there is a compelling argument for re-evaluating the assertion that creating more early-successional habitat is essential for the survival and health of ecosystems, habitats, or species.
2. Impacts of forest clearing projects
2.1. Impacts on biodiversity
Advocates contend that widespread and increased forest-clearing will not have significant negative environmental impacts and can even benefit species associated with mature and old-growth forests (Chandler et al., 2012; Schlossberg et al., 2018; Nareff et al., 2019). Yet, there is ample evidence that this will result in the loss of mature forests and future old-growth habitats, reduced connectivity, an increase in edge habitats, the spread of invasive species, and deleterious effects due to mechanical disruption and species isolation (Wilcove et al., 1986; Small and Hunter, 1988; Franklin, 1989; Askins, 1992; Faaborg et al., 1993).
Meanwhile, and perhaps most important, we have insufficient data on many classes of organisms, and vast numbers of species are still undiscovered (Mora et al., 2011). Numerous moss species need older trees with thicker moisture-holding bark to survive droughts (Zhao et al., 2020). Native snails and insects are more abundant in older forests (Jordan and Black, 2012; Maloof, 2023). These forests host vast networks of plant roots and mycorrhizae, which may link trees to each other and allow the transfer of resources between mature trees (Simard et al., 2012). There is evidence that millions of species of fungi and bacteria swap nutrients between soil and the roots of trees in an interconnected “wood-wide web” of organisms (Steidinger et al., 2019). As scientific methodology evolves, so does our ability to detect tiny organisms and new molecules, including those of critical importance for medicine. In 2018, 16 new species were discovered in a teaspoonful of soil in Massachusetts (Schulz et al., 2018). A study of enchytraeids (a type of annelid worms) in maple forests of northern Minnesota found 9 species new to science (Schlaghamerský et al., 2014). Forest maturity increases the presence of groundwater macroinvertebrates and, in particular, uncommon species (Burch et al., 2022).
Unfortunately, few forests are surveyed for all types of life-forms before clearing to create early-successional habitats. “Resetting” a forest to age “zero” by clearing it reduces ecological complexity immediately because it prevents the full expression of structural and ecological diversity as well as myriad ecosystem services. Recovery is uncertain. Although southeastern U.S. forests are some of the most frequently logged forests in the world (Hansen et al., 2013)—resulting in ample early successional habitat—the region has experienced dramatic long-term declines in early-successional birds (Hanberry and Thompson, 2019). Even less-intensive logging activity can diminish or eliminate disturbance-sensitive and slowly dispersing plant and animal species, with recovery potentially taking many decades, if at all (Duffy and Meier, 1992; Petranka et al., 1994; Hocking et al., 2013).
It is instructive to contrast previously cleared forests that are designated as parks or preserves, where forest ecosystems have been allowed to function and develop predominantly under the influence of natural processes (i.e., GAP 1 areas) with forests subject to clearing of established forests to create early-successional habitats (i.e., some GAP 2 areas) or to commercial logging (i.e., GAP 3 or GAP 4 areas). For more detail on GAP classifications, see Table 1 and U.S. Geological Survey (2022b). Forests that are allowed to recover naturally and develop past the stem-exclusion phase steadily gain structural complexity and biodiversity, in part from ongoing low-to-moderate severity disturbances (Zlonis and Niemi, 2014; Miller et al., 2016; Hilmers et al., 2018). Indeed, the accumulated legacy of a mosaic of natural disturbances is greatest in unmanaged old-growth forests (Oliver and Larson, 1996; Askins, 2000; Lorimer and White, 2003). For instance, the 1-million-acre Boundary Waters Canoe Area Wilderness in Minnesota has taller tree canopies, greater tree species richness, and a larger number of bird species than adjacent managed national forest lands (Zlonis and Niemi, 2014). This wilderness also hosts a similar richness of bird species that favor young forests, such as the Chestnut-sided Warbler (Zlonis and Niemi, 2014). In Maine’s “forever wild” Baxter State Park, natural insect outbreaks create open habitats that benefit early-successional species (Oliveri, 1993). A survey of Michigan habitats concluded that designated wilderness areas had considerable early-successional habitats, even though they were not open to logging or habitat management (Tavernia et al., 2016). As discussed below, findings were similar in New York’s “forever wild” Adirondack and Catskill forest preserves (Widmann et al., 2015).
Numerous rare, threatened, and endangered wildlife species depend upon mature and old-growth forests and their ecosystem services. These species include migratory birds such as the Cerulean Warbler (Setophaga cerulean) (U.S. Fish and Wildlife Service, 2006; Dawson et al., 2012) and Wood Thrush (Hylocichla mustelina) (Bertin, 1977; Hoover et al., 1995; Rosenberg et al., 2003). They include mammals such as the Eastern Spotted Skunk (Spilogale putorius interrupta) (Lombardi et al., 2017; Hassler et al., 2021; Pearce et al., 2021), Appalachian Cottontail (Sylvilagus obscurus) (Chapman et al., 1992), Northern Long-eared Bat (Myotis septentrionalis) (U.S. Fish and Wildlife Service, 2022a), and Allegheny Woodrat (Neotoma magister) (Balcom and Yahner, 1996; Lombardi et al., 2017). They include plants such as Butternut (Juglans cinerea), (Schultz, 2003), Canada Yew (Taxus canadensis) (Dunwiddie et al., 1996; Windels and Flaspohler, 2011), Frasier Sedge (Cymophyllus fraserianus) (Godt et al., 2004), and American Ginseng (Panax quinquefolius) (McGraw et al., 2013). Some species reach their highest densities in old-growth forests, including southern and northern flying squirrels, forest interior birds, and spring ephemeral wildflowers.
The fragmentation of forests, particularly with roads and other human intrusion, can result in the decline of forest interior species. This can have significant impacts on the abundance, species richness, and community dynamics of migratory birds (Small and Hunter, 1988; Askins, 1992; Hagan et al., 1996; Zuckerberg and Porter, 2010; Askins, 2015; Betts et al., 2022). Apex predators can be lost, leading to further biodiversity loss as well as altered dynamics of disease, carbon accumulation, invasive species, and biogeochemical cycles (Terborgh et al., 1999; Anderson et al., 2004; Estes et al., 2011; Terborgh, 2015). Even common forest species are subject to major declines due to loss of natural forest habitats. A global report shows a 69% decrease in monitored wildlife populations between 1970 and 2018, in large part due to habitat fragmentation and degradation (WWF, 2022). Fragmentation can increase prevalence of wildlife diseases including Raccoon Roundworm (Baylisascaris procyonis) (Wolfkill et al., 2021) and may be a factor in oak decline and loss of ecosystem services (Tallamy, 2021) as well as reduced underground biodiversity—a concern that is less explored in the Northeast and Upper Great Lakes than in western forests (Simard, 2021).
Figure 1 reflects biodiversity impacts of habitat changes and hunting over several hundred years. Habitat loss was a factor in the decline of deer, moose, beaver, turkey, wolf, mountain lion, and bear, but intensive hunting and trapping probably had the greatest impact (Foster et al., 2002). Coyotes migrated eastward following wolf extirpation, interbred with wolves, and partially filled the vacant niche left by wolf extirpation. Deer can thrive in disturbed landscapes, which explains their recovery once hunting pressure was relieved (Michigan Department of Natural Resources, 2016). Forest-clearing is widely used today to boost populations of deer and other game species (Lashley et al., 2011; Dechen Quinn et al., 2013; Michigan Department of Natural Resources, 2017). However, high deer population densities can have significant negative effects on forest regeneration, native herbaceous plants—especially charismatic floristic groups such as orchids—and songbirds and their habitats (Alverson et al., 1988; deCalesta, 1994; Rooney and Waller, 2003; Knapp and Wiegand, 2014; Jirinec et al., 2017). Clearing established forests can also introduce and spread invasive and non-native species that ultimately reduce biodiversity (McDonald et al., 2008; Eschtruth and Battles, 2009; LeDoux and Martin, 2013; Coyle et al., 2017). Managed forests have been found to have as much as three times more invasives than fully protected national parks or wilderness (Riitters et al., 2018). Invasive plants can have a negative impact on native animal populations, including birds, mammals and other vertebrates (Fletcher et al., 2019). Invasive earthworms are a serious concern, particularly the new threat of jumping worms (Amynthas spp.) that destroy forest soil very rapidly (Frelich et al., 2019).
2.2. Impacts on the atmosphere
Forests influence water cycles, reduce local and global temperatures, and sequester and accumulate carbon. While carbon receives the most attention, multiple biophysical processes are crucial and interactive (Makarieva et al., 2020; Lawrence et al., 2022). Proponents of forest-clearing assert that carbon emissions are offset by increased sequestration rates of younger forests, by converting trees to wood products, by burning logging “waste” for bioenergy, and by forest carbon accumulation elsewhere—or that the amount of forest removal is so small as to be inconsequential (Hawthorne, 2020; Jenkins and Kroeger, 2020; USDA Forest Service, 2021a). On the contrary, these activities have significant climate costs, including the release of greenhouse gases from the cutting, processing, and transporting of trees for wood products; the disposal of waste and wood products; the release of methane from each log landing; the release of carbon from disturbed soils; and the loss of carbon uptake and accumulation by standing trees (Smith et al., 2006; Nunery and Keeton, 2010; Ingerson, 2011; Mika and Keeton, 2013; Catanzaro and D’Amato, 2019; Cook-Patton et al., 2020; Leturcq, 2020; Vantellingen and Thomas, 2021).
Some studies suggest that younger forests between 30 and 70 years (Catanzaro and D’Amato, 2019) or 40–80 years (Leverett et al., 2021) can sequester carbon at a faster rate than mature or old-growth forests. Other analyses indicate that lands reserved from logging in the Northeast have net carbon sequestration rates that are roughly 33% higher than in logged forests and are projected to sequester more carbon over the next 150 years (Brown et al., 2018). Nevertheless, the climate mitigation value of forest carbon lies not in the sequestration rate but in the total amount that is accumulated and kept out of the atmosphere (Mackey et al., 2013). The power of forests in this process is unparalleled and far greater in old forests than in young forests, both above and below ground; carbon continues to accumulate for centuries (Zhou et al., 2006; Luyssaert et al., 2008; Keeton et al., 2011; Curtis and Gough, 2018; Leverett et al., 2021; Law et al., 2022).
The amount of carbon lost when cutting a mature or old-growth forest is not recovered by fast-growing young forests for many decades to well over a century (Harmon et al., 1990; Aalde et al., 2006; Krebs et al., 2017). One study found almost no net carbon accumulation for 15 years after clearcutting—currently a critical time window for reining in global greenhouse gas emissions (Hamburg et al., 2019). In some cases, older forests are accumulating more carbon as the climate warms (Finzi et al., 2020), they are better able to withstand physiological stress, and they are also more resistant to the stress of climate change than younger forests, particularly regarding carbon storage, timber growth rate, and species richness (Thom et al., 2019). Soil accounts for approximately 50% of total ecosystem carbon storage in the Northeast, with mineral soils comprising the majority (Fahey et al., 2005; Petrenko and Friedland, 2015). Forest-clearing can mobilize and release soil carbon for decades (Nave et al., 2010; Petrenko and Friedland, 2015; Lacroix et al., 2016). It can take from 60 to 100 years for soils on a site to recover from clearcut logging (James and Harrison, 2016).
It is crucial to note that forest carbon stocks in the U.S. are already depleted by about 60% due to past logging and clearing (McKinley et al., 2011) and ongoing timber removals (Gunn et al., 2019). Logging accounts for about 86% of the carbon emitted by U.S. forests each year—far greater than insects, storm damage, fire, development and other uses combined (Harris et al., 2016; Duveneck and Thompson, 2019). Although a small percentage of the carbon in trees that are cut is stored in durable wood products, in the U.S. about 76% of carbon in trees cut for timber is released into the atmosphere each year (Domke et al., 2018), with most of it emitted quickly in processing, waste, and short-lived products (Harmon et al., 1996; Ingerson, 2011; Harmon, 2019; Leturcq, 2020). A logged mature forest stores less than half of the carbon of an uncut mature forest, even if carbon stored in wood products is included in the carbon storage total of the logged areas (Nunery and Keeton, 2010; Law et al., 2022). Impacts are similar for forest-clearing to produce wood bioenergy, which advocates claim is “carbon neutral” (Collins et al., 2015). However, cutting and burning trees releases large amounts of carbon immediately that would take many decades to be recover–if the forest grows back. In addition to other disrupted biophysical processes, this is time we cannot afford in light of the urgent climate crisis (Schulze et al., 2012; Law et al., 2018; Sterman et al., 2022). In short, clearing forests—whether for early-successional habitat or bioenergy—results in serious impacts to the atmosphere. In terms of maximizing carbon accumulation, allowing forests to regrow and remain standing—termed proforestation—is demonstrably preferable to cutting them (Buotte et al., 2019; Moomaw et al., 2019; Mackey et al., 2020; Rogers et al., 2022).
Despite widespread past clearing, the forests of the Northeast and Upper Great Lakes have recovered to the point that they are among the most intact and carbon-dense in the eastern U.S. (Zheng et al., 2008; Zheng et al., 2010; Foster et al., 2017). In addition, because these forests grow vigorously, decay slowly, and are, on average, less than 100 years old, they have centuries of growth ahead and enormous capacity for additional carbon storage (Pan et al., 2011; Williams et al., 2012) and climate stabilization. If allowed to continue growing, these forests can potentially increase in situ carbon storage by a factor of 2.3 to 4.2 (Keeton et al., 2011) and perform crucial ecosystem services (Meyer et al., 2022). For these reasons, the New England Acadian region was identified as a Tier 1 stabilization area in the Global Safety Net (Dinerstein et al., 2020). The potential in the Upper Great Lakes region is also significant, where continued forest recovery in existing forests could add substantial amounts of carbon storage (Rhemtulla et al., 2009).
2.3. Impacts on human health and well-being
With more than 50 million acres of U.S. forests projected to be developed over the next 50 years (Thompson, 2006), forest-clearing for early-successional habitats risks further loss of vital natural green space and threatens the stability of regional temperature and water cycles. All of these have impacts on communities. There is an increasing recognition that natural ecosystems offer the public numerous benefits to physical, mental, and spiritual health, as well as social well-being (Karjalainen et al., 2010; Berman et al., 2012; Buttke et al., 2014; Newman and Cragg, 2016; Hansen et al., 2017; Watson et al., 2018; Connecticut Department of Energy and Environmental Protection, 2020). Adolescents may benefit more from natural woodlands than other types of green space in terms of cognitive development and reduced emotional and behavioral problems (Maes et al., 2021). Natural areas are important places to avoid human-related noise and listen to sounds of the natural world, which can decrease pain, lower stress, improve mood, and enhance cognitive performance (Bratman et al., 2015; Buxton et al., 2021).
Protecting intact habitats as refuges for people—even small areas—aligns with the principles of “harm reduction”—practical strategies and ideas aimed at reducing negative consequences. Increasing the well-being of a community, and avoiding or minimizing negative consequences of heat stress, acute physical and mental stress, and a long-term sense of loss can prevent a more serious or chronic condition, particularly in vulnerable populations such as adolescents, pregnant women, seniors, veterans, and those in recovery (Wang et al., 2019; Tiako et al., 2021). The positive impacts of nature on the promotion of mental health has enormous economic benefits (Bratman et al., 2019) and as does preventing mental illness (The Lancet Global Health, 2020).
In addition to social well-being, nature-based outdoor recreation can be an important factor in diversifying and stabilizing local economies (Power, 1996; Power, 2001; Haefele et al., 2016). Studies have shown that recreationists prefer spending time in forests and other landscapes that are natural and free of human manipulation (Vining and Tyler, 1999; Dwyer, 2003; Eriksson et al., 2012). The positive economic effects of robust ecotourism and increased property values can benefit an entire community (Morton, 1998; Lorah and Southwick, 2003; Holmes and Hecox, 2004; Phillips, 2004; Rasker et al., 2013; Fernandez et al., 2018; Cullinane et al., 2022).
In contrast, clearing forests to expand early-successional habitat can threaten human health. For example, it provides optimal habitat for White-tailed Deer and White-footed Mouse (Peromyscus leucopus)—the most competent hosts for the vector of Lyme disease, the Eastern Blacklegged Tick (Ixodes scapularis) (Allan et al., 2003; LoGiudice et al., 2003; Levi et al., 2012; Telford, 2017; DellaSala et al., 2018; Robertson et al., 2019). There were 185 deaths from auto collisions with animals in 2019 and an estimated 2.1 million animal collision insurance claims in 2020–21, up 7.2 percent from the previous year, with most collisions involving deer (Insurance Information Institute, 2021).
3. Options and alternatives
As discussed above, forest-clearing projects across the Northeast and Upper Great Lakes are proceeding without well-founded consideration of conditions before European settlement, long-term plans for experimental controls and monitoring, or baseline ecological inventories. Assessments made of potential harm to non-target species are cursory, incomplete, or outdated. Quantifiable negative impacts—such as the spread of invasive species, elevated temperatures, increased fire and flood risk, destabilized and decreased climate mitigation and adaptation, degradation of healthy public green spaces, and ongoing expenditures of time and resources—are frequently overlooked. Meanwhile, potentially imperiled interior and old-growth forest species often do not receive adequate attention. Such chronic knowledge gaps render scientific assessment of the impacts of early-successional habitat projects difficult or impossible. Major interdisciplinary reports (Connecticut Department of Energy and Environmental Protection, 2020) offer a strong rationale for addressing these gaps by devoting significant funding to balancing these priorities, to monitoring, comprehensive ecological inventories, and to strengthening management standards and guidelines.
Reassessing the current forest-clearing campaign is an urgent priority: negative impacts are immediate, and once a forest has been cleared or fragmented it takes a century or more to begin to recover a mature or old-growth condition. This is far too late to address the biodiversity, climate, and public health crises that we face in the next critical decades. There are multiple compelling arguments for a new approach that greatly expands wildland preserves while maintaining needed amounts of early-successional habitats and timber production.
3.1. The importance of parks and preserves
There is growing international recognition that the preservation of mature and old-growth forests is essential to address the dual global crises of biodiversity loss and climate change, as well as to promote public health and well-being (Zhou et al., 2006; Luyssaert et al., 2008; Gilhen-Baker et al., 2022; Law et al., 2022). However, in their drive to expand early-successional habitats, land managers have relegated the recovery and protection of old-growth forests to a tiny fraction of their pre-European extent (New Jersey Department of Environmental Protection, 2017; Massachusetts Division of Fisheries and Wildlife, 2022b). The U.S. Forest Service and Bureau of Land Management together administer the largest remaining tracts of mature and old-growth forests in the U.S., yet they are only now beginning a process to inventory these forests (The White House, 2022). Nationally, only about 24% of these forests are protected from logging (DellaSala et al., 2022a).
An extensive system of large, diverse, and connected parks and preserves can help address this challenge (Noss, 1983; Noss et al., 1999; Wuerthner et al., 2015). Studies show that eastern national parks tend to have larger trees, older forests, and more standing deadwood than surrounding managed forests (Miller et al., 2016). They also have greater tree species richness and a higher percentage of rare tree species (Miller et al., 2018). Cool interior forests such as those in parks and other preserves provide shelter for species that are most sensitive to temperature increases (Betts et al., 2017; Betts et al., 2022; Kim et al., 2022; Xu et al., 2022). Protected forests provide important climate benefits in accumulated carbon and avoided greenhouse gas emissions, and the potential to significantly increase carbon storage (Depro et al., 2008; Keeton et al., 2011; Zheng et al., 2013; McGarvey et al., 2015; Brown et al., 2018; Williams et al., 2021; Law et al., 2022). In addition, parks and preserves directly benefit people by producing clean air and water, reducing flooding, preventing soil erosion, cooling surrounding areas, and buffering damage from sea level rise (Luedke, 2019).
Climate scientists and conservation biologists around the world agree that a major expansion of nature preserves is necessary to address the threats of species extinctions and climate change (Di Marco et al., 2019; Yeo et al., 2019; Barber et al., 2020; FAO and UNEP, 2020; Bradshaw et al., 2021). There is a broad consensus that this requires the permanent protection of at least 30% of the Earth by 2030 (Noss et al., 2012; Dinerstein et al., 2019; Rosa and Malcom, 2020; Thompson and Walls, 2021). The U.S. falls far short of meeting this goal. Only about 8% of the U.S. land base now has protection from resource extraction and development equivalent to the U.S. Geological Survey’s GAP 1 level and less than 5% meets GAP 2 standards; the vast majority of these lands are in Alaska and the West (Scott et al., 2001; Aycrigg et al., 2013; Jenkins et al., 2015; Lee-Ashley, 2019; Rosa and Malcom, 2020; Thompson and Walls, 2021; U.S. Geological Survey, 2022a,b). As noted previously, most old-growth forests in the U.S. have no formal protection, even on many GAP 2 public lands, leaving their future uncertain (DellaSala et al., 2022b).
The Northeast and Upper Great Lakes regions are deficient in natural area protection (Scott et al., 2001; Anderson and Olivero Sheldon, 2011; Foster et al., 2023). There are a few notable exceptions, such as the Boundary Waters Canoe Area Wilderness, Isle Royale National Park, Adirondack Forest Preserve, and Baxter State Park, which meet GAP 1 standards (U.S. Geological Survey, 2022a,b). However, less than 1% of the Northeast and Upper Great Lakes regions is estimated to meet this strict level of protection U.S. Geological Survey (2022a). This percentage could be greatly increased through an expanded network of parks and preserves on large tracts of federal and state public lands, and could include key undeveloped private lands acquired from willing sellers (Foster et al., 2017; Meyer et al., 2022; Office of Senator Angus King, 2022). This would have numerous outsized benefits; for example, one study estimated that protected forests cover about 5% of the Northeast (including Virginia) yet store 30% of the aboveground carbon in the region (Lu et al., 2013). New wildland preserves would promote the recovery of mature and old-growth forest ecosystems and provide habitats for wide-ranging imperiled wildlife such as the Gray Wolf (Canis lupus) and Canada Lynx (Lynx Canadensis). They would also offer natural green space to tens of millions of people in major urban communities, reducing pressure on the few existing protected areas (Rhode Island Division of Statewide Planning and Rhode Island Department of Environmental Management, 2019; Reynolds, 2021).
There is ample evidence that expanded wildland preserves governed by natural disturbance regimes would provide early-successional habitats at least equivalent to the natural conditions in which native species evolved. For example, “On reserved forest land in New York [i.e., primarily the “forever wild” Adirondack and Catskill Preserves]… 3 percent [of forest area is] in seedling/sapling and non-stocked stands” (Widmann et al., 2015). Consistent with this, it is estimated that the proportion of the landscape before European settlement “in seedling–sapling forest habitat ranged from 1 to 3% in northern hardwood forests [i.e., beech–birch–maple–hemlock] of the interior upland” (Lorimer and White, 2003).
3.2. Protecting and restoring natural forest ecosystems
The most common strategy for creating early-successional habitats is to clear established forest tracts, purportedly to simulate the continually “shifting mosaic” of patches across a natural landscape (Schlossberg and King, 2007; Smith, 2017; Massachusetts Division of Fisheries and Wildlife, 2022a). However, as discussed above, forest-clearing is not equivalent to natural disturbances; it has significant costs in biodiversity, carbon accumulation, and other ecosystem services; and reduces the possibility of recovering old-growth forest ecosystems dramatically. Moreover, unlike the conservation of mature and old-growth forests, creating and/or maintaining (every 10–12 years) early-successional habitats requires a permanent, resource-consuming commitment of intensive management to replace openings lost to forest succession (DeGraaf and Yamasaki, 2003; Askins, 2011; Bakermans et al., 2011; Yamasaki et al., 2014). This does not take into consideration the mitigation and remediation of unintended environmental side effects: such artificially created “restoration” areas are expensive to maintain (Oehler, 2003; Schlossberg and King, 2007) and there is no assurance that adequate funding will continue to be available. These are serious disadvantages that argue against the current forest-clearing of established natural forest ecosystems.
Among these different perspectives, there is a more balanced alternative: protect and recover mature and old-growth forests wherever possible, quantify the true extent of early-successional habitat and focus maintenance on ecologically suitable lands, including private lands, and encourage efforts to increase protection the full range of natural ecosystems on private lands. At this time there is no indication that this approach is receiving serious consideration from land managers. Yet the likelihood of significant benefits and greatly reduced costs are a compelling argument for such consideration.
4. Discussion
We evaluated peer-reviewed papers, published research, agency reports, and other materials related to a campaign that is focused on expanding early-successional habitats in the Northeast and Upper Great Lakes regions. Each year, this campaign is clearing thousands of acres of established forests. Conversely, the protection of old-growth forests and unmanaged mature forests is currently relegated to a tiny fraction of the land base.
Overall, the forest-clearing campaign is based on two main rationales, which are both open to serious questions and alternative hypotheses:
The primary rationale is that the decline of a number of early-successional species is a pervasive and potentially existential threat. Yet, the baseline for measuring this decline almost invariably begins in the late 1960s, when populations had begun to decrease from abnormally high levels as forests recovered from past clearing. Relying on an artificial baseline that reaches back only 60 years, in an ecosystem where most tree species live for hundreds of years, and during a regional recovery from widespread and intensive land clearing, is fraught with problems. Moreover, it is questionable that any species in these regions needs artificial expansion of early-successional forest habitats to survive and thrive across its multi-state range. Other than limited surveys of birds, game species, and endangered species, there is no reliable information on wildlife populations before the arrival of Europeans, no comprehensive census of forest species even today, and no long-term analysis that systematically estimates wildlife population trends over the last several hundred years.
A second major rationale is that early-successional habitats have dwindled dangerously, have already fallen below the levels that existed before European settlement, and are not being adequately replenished—thereby endangering native biodiversity. However, there is ample evidence that these habitats remain plentiful across these regions (and are likely more prevalent than is accounted for currently), are considerably more abundant than presettlement, and continue to be created by natural and human disturbances—including by mounting climate change impacts. Although early-successional habitats were maintained to some extent by Native people before the arrival of Europeans, these were limited to areas of high population densities near settlements.
Despite its wide-ranging and long-term implications, the campaign for early-successional forest clearing was formulated by a small number of agency, academic, and special interest professionals, with little comprehensive research and analysis, controlled experimentation, strategic planning, monitoring and evaluation, or public involvement and accountability. This organized and aggressive campaign has confused the public and made it challenging for a range of scientists to engage in an open dialogue about an optimal and balanced approach that prioritizes climate stability, ecosystem integrity and public health. Yet, public awareness has grown regarding the evident impacts of forest-clearing projects on biodiversity, climate change, and natural green spaces and, in turn, so has public opposition to these projects (Ketcham, 2022; Potter, 2022; Whitcomb, 2022).
The Gap Analysis Project (GAP) of the U.S. Geological Survey (2022b) can provide the foundation for a balanced alternative to the current costly, intrusive and controversial approach that prioritizes protecting and sustaining natural systems and processes to the greatest extent possible. We suggest the following.
• Establish a significantly expanded system of public parks, wildland preserves, and connecting corridors across the Northeast and Upper Great Lakes with permanent protection under GAP 1 standards. This would preserve old-growth, mature, and recovering forests and allow them to reach their natural maximum ecological potential. Openlands that were deforested in the past, such as grassy areas and farm fields, would be allowed to recover unimpaired, which would provide ample young forest habitats over the next decade. In parallel, new areas of successional habitat would be created by natural disturbance regimes now, and in the future.
• End the clearing of established forests to create early-successional habitats on lands, such as wildlife refuges, under GAP 2 classification. Instead, focus on conserving grassland, shrubland, and savanna habitats where the landform and soil naturally supports their ecological function and species. Examples include coastal landscapes of southern New England and New York, and the Upper Great Lakes prairie-forest transition zone. Re-establish natural disturbance regimes to the extent possible, but allow targeted forest management where appropriate.
• Strengthen the protection of GAP 3 “multiple-use” public lands such as national forests, to maintain natural ecosystems, carbon storage, and public access to green spaces to the extent possible. This includes avoiding intensive resource extraction that destroys or permanently impairs the integrity and productivity of natural systems.
• Regarding public and private lands with no formal protection (GAP 4), encourage the conservation of natural ecosystems and species to the extent possible. This includes agricultural lands and other open space with considerable potential to conserve early-successional habitats. These landowners would continue to determine how they manage their lands, but they would be provided with complete and accurate information on the benefits and costs of habitat management alternatives.
Implementing this “natural” alternative would be prudent, cautious, and low cost, and would permanently sustain the full range of native ecosystems. Allowing deforested lands to recover would accumulate much more carbon and avoid the steep carbon loss associated with cutting established forests (Smith et al., 2006; Cook-Patton et al., 2020).
In the face of many challenges, the people of the Northeast, Upper Great Lakes, and beyond are looking to public lands as a major solution to the loss of biodiversity, the threat of climate change, and the need for healthy public green spaces. We can realize this potential by rebalancing the vision for these lands to ensure the recovery and preservation of the full range of native habitats and the wildlife that depend on them—without ongoing intensive human intervention. There has never been a more appropriate time to make such a transition.
Author contributions
MK, JM, and SM developed the original concept and contributed research, writing, and editing of the manuscript. LF, EF, SB, and DF contributed research, writing, and editing of the manuscript. All authors contributed to its completion and approved the submitted version.
Funding
This work was supported by the Eddy Foundation, Forest Carbon Coalition, and Fund for Wild Nature, and Common Stream.
Conflict of interest
The authors declare that the research was conducted in the absence of any commercial or financial relationships that could be construed as a potential conflict of interest.
Publisher’s note
All claims expressed in this article are solely those of the authors and do not necessarily represent those of their affiliated organizations, or those of the publisher, the editors and the reviewers. Any product that may be evaluated in this article, or claim that may be made by its manufacturer, is not guaranteed or endorsed by the publisher.
Supplementary material
The Supplementary Material for this article can be found online at: https://www.frontiersin.org/articles/10.3389/ffgc.2022.1073677/full#supplementary-material
References
Aalde, H., Gonzalez, P., Gytarsky, M., Krug, T., Kurz, W. A., Ogle, et al. (2006). “Chapter 4. Forest land,” in 2006 IPCC guidelines for national greenhouse gas inventories: agriculture, forestry and other land use, Vol. 4, eds H. S. Eggleston, L. Buendia, K. Miwa, T. Ngara, and K. Tanabe (Kanagawa: IGES).
Abrams, M. D. (1992). Fire and the development of oak forests. Bioscience 42, 346–353. doi: 10.2307/1311781
Abrams, M. D. (2005). Prescribing fire in eastern oak forests: Is time running out? Northern J. Appl. For. 22, 190–196. doi: 10.1093/njaf/22.3.190
Abrams, M. D., and Copenheaver, C. A. (1999). Temporal variation in species recruitment and dendroecology of an old-growth white oak forest in the Virginia Piedmont, USA. For. Ecol. Manag. 124, 275–284. doi: 10.1016/S0378-1127(99)00071-7
Abrams, M. D., and Nowacki, G. J. (2008). Native Americans as active and passive promoters of mast and fruit trees in the eastern USA. Holocene 18, 1123–1137. doi: 10.1177/0959683608095581
Abrams, M. D., and Nowacki, G. J. (2020). Native American imprint in palaeoecology. Nat. Sustain. 3, 896–897.
Abrams, M. D., Ruffner, C. M., and DeMeo, T. E. (1998). Dendroecology and species co-existence in an old-growth Quercus–Acer–Tilia talus slope forest in the central Appalachians, USA. For. Ecol. Manag. 106, 9–18. doi: 10.1016/S0378-1127(97)00234-X
Allan, B. F., Keesing, F., and Ostfeld, R. S. (2003). Effect of forest fragmentation on lyme disease risk. Conserv. Biol. 17, 267–272. doi: 10.1046/j.1523-1739.2003.01260.x
Alverson, W. S., Waller, D. M., and Solheim, S. L. (1988). Forests too deer: Edge effects in Northern Wisconsin. Conserv. Biol. 2, 348–358. doi: 10.1111/j.1523-1739.1988.tb00199.x
Amaral, K. E., Palace, M., O’Brien, K. M., Fenderson, L. E., and Kovach, A. I. (2016). Anthropogenic habitats facilitate dispersal of an early successional obligate: Implications for restoration of an endangered ecosystem. PLoS One 11:e0148842. doi: 10.1371/journal.pone.0148842
American Bird Conservancy (2007). Top 20 most threatened bird habitats in the U.S. The Plains, VA: American Bird Conservancy.
American Bird Conservancy (2015). $10 Mil. Forest restoration project will benefit imperiled golden-winged warbler, 14 January. Available online at: https://abcbirds.org/article/10-mil-forest-restoration-project-will-benefit-imperiled-golden-winged-warbler/ (accessed November 5, 2022).
Anderson, M. G., and Olivero Sheldon, A. (2011). Conservation status of fish, wildlife, and natural habitats in the northeast landscape: implementation of the northeast monitoring framework. Arlington, VA: The Nature Conservancy, Eastern Conservation Science. 289.
Anderson, M. G., Clark, M. M., Cornett, M. W., Hall, K. R., Olivero Sheldon, A., and Prince, J. (2018). Resilient sites for terrestrial conservation in the great lakes and tallgrass prairie. Arlington, VA: The Nature Conservancy, Eastern Conservation Science and North America Region.
Anderson, M., Bernstein, S., Lowenstein, F., Smith, N., and Pickering, S. (2004). Determining the size of eastern forest reserves. Boston, MA: The Nature Conservancy and Sweet Water Trust.
Aquilué, N., Filotas, É, Craven, D., Fortin, M., Brotons, L., and Messier, C. (2020). Evaluating forest resilience to global threats using functional response traits and network properties. Ecol. Appl. 30:e02095. doi: 10.1002/eap.2095
Askins, R. A. (1992). Forest fragmentation and the decline of migratory songbirds. Bird Observer 20, 13–21.
Askins, R. A. (1993). “Population trends in Grassland, Shrubland, and forest birds in Eastern North America,” in. Current ornithology, Vol. 11, ed. D. M. Power (Boston, MA: Springer). doi: 10.1007/978-1-4757-9912-5_1
Askins, R. A. (2000). Restoring North America’s wild birds: lessons from landscape ecology. New Haven, CT: Yale University Press.
Askins, R. A. (2011). The future of blue-winged and golden-winged warblers in Connecticut. Connecticut Woodlands 76, 12–15.
Askins, R. A. (2015). The critical importance of large expanses of continuous 1988 forest for bird conservation in connecticut state of the birds: Protecting and connecting large landscapes. Biol. Faculty Public 25, 24–28.
Askins, R. A., Folsom-O’Keefe, C. M., and Hardy, M. C. (2012). Effects of vegetation, corridor width and regional land use on early successional birds on powerline corridors. PLoS One 7:e31520. doi: 10.1371/journal.pone.0031520
Aycrigg, J. L., Davidson, A., Svancara, L. K., Gergely, K. J., McKerrow, A., and Scott, J. M. (2013). Representation of ecological systems within the protected areas network of the Continental United States. PLoS One 8:e54689. doi: 10.1371/journal.pone.0054689
Baca, A., Larsen, J., Treasure, E., Gavazzi, M., and Walker, N. (2018). Drought impacts in the southern region: a synopsis of presentations and ideas from the drought adaptation workshop in region 8. Atlanta, GA: USDA Forest Service. doi: 10.32747/2018.7280913.ch
Bakermans, M. H., Larkin, J. L., Smith, B. W., Fearer, T. M., and Jones, B. C. (2011). Golden-winged warbler habitat best management practices for forestlands in Maryland and Pennsylvania. The Plains: American Bird Conservancy, 26.
Balcom, B. J., and Yahner, R. H. (1996). Microhabitat and landscape characteristics associated with the threatened allegheny woodrat. Conserv. Biol. 10, 515–525.
Barber, C. V., Petersen, R., Young, V., Mackey, B., and Kormos, C. (2020). Thenexus report: nature based solutions to the biodiversity and climate crisis. F20 foundations, campaign for nature and SEE foundation. Available online at: https://wild-heritage.org/wp-content/uploads/2021/01/The-Nexus-Report.pdf (accessed January 22, 2021).
Barrett, S. W., Swetnam, T. W., and Baker, W. L. (2005). Indian fire use: Deflating the legend. Fire Manag. Today 31–34.
Belair, E. P., and Ducey, M. J. (2018). Patterns in forest harvesting in New England and New York: Using FIA data to evaluate silvicultural outcomes. J. For. 116, 273–282. doi: 10.1093/jofore/fvx019
Berman, M. G., Kross, E., Krpan, K. M., Askren, M. K., Aleah Burson, A., Deldin, P. J., et al. (2012). Interacting with nature improves cognition and affect for individuals with depression. J Affect Disord. 140, 300–305. doi: 10.1016/j.jad.2012.03.012
Bertin, R. (1977). Breeding habitats of the wood thrush and veery. Condor 79, 303–311. doi: 10.1007/s00442-005-0340-9
Betts, M. G., Phalan, B., Frey, S. J. K., Rousseau, J. S., and Yang, Z. (2017). Old-growth forests buffer climate- sensitive bird populations from warming. Divers Distrib. 24, 439–447. doi: 10.1111/ddi.12688
Betts, M. G., Yang, Z., Hadley, A. S., Smith, A. C., Rousseau, J. S., Northrup, J. M., et al. (2022). Forest degradation drives widespread avian habitat and population declines. Nat. Ecol. Evol. 6, 709–719. doi: 10.1038/s41559-022-01737-8
Boose, E. R., Chamberlin, K. E., and Foster, D. R. (2001). Landscape and regional impacts of hurricanes in New England. Ecol. Monogr. 71, 27–48. doi: 10.1890/0012-96152001071[0027:larioh]2.0.co;2
Bradshaw, C. J. A., Ehrlich, P. R., Beattie, A., Ceballos, G., Crist, E., Diamond, J., et al. (2021). Underestimating the challenges of avoiding a ghastly future. Front. Conserv. Sci 13:615419. doi: 10.3389/fcosc.2020.615419
Brady, S. J. (2007). “Chapter 1: Effects of cropland conservation practices on fish and wildlife habitat,” in Fish and wildlife response to farm bill conservation practices, in technical review 07–1 (Bethesda, MD: The Wildlife Society).
Bratman, G. N., Anderson, C. B., Berman, J. G., Cochran, B., de Vries, S., Flanders, J., et al. (2019). Nature and mental health: An ecosystem service perspective. Sci. Adv. 5:eaax0903. doi: 10.1126/sciadv.aax0903
Bratman, G. N., Hamilton, J. P., Hahn, K. S., Daily, G. C., and Gross, J. J. (2015). Nature experience reduces rumination and subgenual prefrontal cortex activation. Proc. Natl. Acad. Sci. USA 112, 8567–8572. doi: 10.1073/pnas.1510459112
Brose, P., Schuler, T., Van Lear, D., and Berst, J. (2001). Bringing fire back the changing regimes of the Appalachian mixed-oak forest. J. For. 99, 30–35.
Brown, M. L., Canham, C. D., Murphy, L., and Donovan, T. M. (2018). Timber harvest as the predominant disturbance regime in northeastern U.S. forests: Effects of harvest intensification. Ecosphere 9:e02062. doi: 10.1002/ecs2.2062
Bruchac, M. (2004). Native land use and settlements in the Northeastern Woodlands. Raid on deerfield: the many stories of 1704. Philadelphia, PA: University of Pennsylvania Department of Anthropology.
Buchwald, E. (2005). “A hierarchical terminology for more or less natural forests in relation to sustainable management and biodiversity conservation,” in Proceedings of the third expert meeting on harmonizing forest-related definitions, (Rome), 11–19.
Buckley, G. L. (2010). America’s conservation impulse. Charlottesville, VA: The University of Virginia Press.
Bulluck, L. P., and Buehler, D. A. (2006). Avian use of early successional habitats: Are regenerating forests, utility right-of-ways and reclaimed surface mines the same? For. Ecol. Manag. 236, 76–84. doi: 10.1016/j.foreco.2006.08.337
Buotte, P. C., Law, B. E., Ripple, W. J., and Berner, L. T. (2019). Carbon sequestration and biodiversity co-benefits of preserving forests in the western United States. Ecol. Appl. 30:e02039. doi: 10.1002/eap.2039
Burch, E., Culver, D. C., Alonzo, M., and Malloy, E. J. (2022). Landscape features and forest maturity promote the occurrence of macroinvertebrates specialized for seepage springs in urban forests in Washington, DC. Aquat. Conserv. Mar. Freshw. Ecosyst. 32, 922–929. doi: 10.1002/aqc.3803
Buttke, D., Allen, D., and Higgins, C. (2014). Benefits of biodiversity to human health and well-being. Park Sci. 31, 24–29.
Buxton, R. T., Pearson, A. L., Allou, C., Fristrup, K., and Wittemyer, G. (2021). A synthesis of health benefits of natural sounds and their distribution in national parks. Proc. Natl. Acad. Sci. USA 118:e2013097118. doi: 10.1073/pnas.2013097118
Cachat-Schilling, N. (2021). Fire and myths about northeast native land stewardship. Massachusetts ethical archaeology Society, 31 March. Available online at: https://www.ethicarch.org/post/fire-and-myths-about-northeast-native-land-stewardship (accessed November 30, 2022).
Caron, S. (2009). Managing your woodland for white-tailed deer. St. Paul, MN: Minnesota Department of Natural Resources.
Catanzaro, P., and D’Amato, A. (2019). Forest carbon: an essential natural solution for climate change. Amherst, MA: University of Massachusetts Amherst and University of Vermont.
Chandler, C. C., King, D. I., and Chandler, R. B. (2012). Do mature forest birds prefer early-successional habitat during the post-fledging period? For. Ecol. Manag. 264, 1–9. doi: 10.1016/j.foreco.2011.09.018
Chapman, J. A., Cramer, K. L., Dippenaar, N. J., and Robinson, T. J. (1992). Systematics and biogeography of the New England cottontail, Sylvilagus transitionalis (Bangs, 1895), with the description of a new species from the Appalachian mountains. Proc. Biol. Soc. Washington 105, 841–866.
Chen, I., Hill, J. K., Ohlemüller, R., Roy, D. B., and Thomas, C. D. (2011). Rapid range shifts of species associated with high levels of climate warming. Science 333, 1024–1026. doi: 10.1126/science.1206432
Chilton, E. S. (2002). “Towns they have none:” diverse subsistence and settlement strategies in native New England,” in Northeast subsistence-settlement change A.D. 700-1300, eds J. P. Hart and C. Reith (Albany, NY: New York State Museum), 289–300.
Clark, K. H., and Patterson, W. A. III. (2003). Fire management plan for montague plain wildlife management area. Amherst, MA: Department of Natural Resources Conservation, University of Massachusetts, 48.
Climate Change Response Network (2022a). U.S. fish and wildlife service and university of vermont: Nulhegan Basin, Silvio O. Conte national fish and wildlife refuge adaptation demonstration project. Houghton, MI: Northern Institute of Applied Climate Science.
Climate Change Response Network (2022b). Chippewa national forest: adaptive silviculture for climate change (ASCC). Houghton, MI: Northern Institute of Applied Climate Science.
Cochrane, T. S., and Iltis, H. H. (2000). Atlas of the wisconsin prairie and savanna flora. Wisconsin department of natural resources technical bulletin No. 191. Madison, WI: 226.
Cogbill, C. V. (2000). Vegetation of the presettlement forests of Northern New England and New York. Rhodora 102, 250–276.
Cogbill, C. V., Burk, J., and Motzkin, G. (2002). The forests of presettlement New England, USA: Spatial and compositional patterns based on town proprietor surveys. J. Biogeogr. 29, 1279–1304. doi: 10.1046/j.1365-2699.2002.00757.x
Collins, S., Merkley, J., Ayotte, K., Baldwin, T., Blunt, A., Brown, S., et al. (2015). Letter to gina mccarthy, ernest moniz, and tom vilsack supporting biomass energy, 30 June. Available online at: http://mediad.publicbroadcasting.net/p/mpbn/files/collinsletter.pdf (accessed November 4, 2022).
Connecticut Department of Energy and Environmental Protection (2013). The clear cut advantage for wildlife and forest health. Connecticut: Connecticut Department of Energy and Environmental Protection.
Connecticut Department of Energy and Environmental Protection (2015). From the director’s desk. Connecticut wildlife, September/October. Connecticut department of energy and environmental protection. Available online at: https://portal.ct.gov/-/media/DEEP/wildlife/pdf_files/outreach/connecticut_wildlife_magazine/cwso15pdf.pdf (accessed November 5, 2022).
Connecticut Department of Energy and Environmental Protection (2018). Return of historic species: young forest initiative. Vol.7, No. 1. Available online at: https://portal.ct.gov/-/media/DEEP/wildlife/pdf_files/habitat/yfshrubinitiative/yfnewsletterissue11pdf.pdf (accessed November 5, 2022).
Connecticut Department of Energy and Environmental Protection (2019). Shrubland bird monitoring. Available online at: https://www.ct.gov/deep/cwp/view.asp?a=2723&q=594738&deepNav_GID=1655 (accessed November 5, 2022).
Connecticut Department of Energy and Environmental Protection (2020). The governor’s council on climate change (GC3) science and technology working group final phase 1 report. Available online at: https://portal.ct.gov/-/media/DEEP/climatechange/GC3/GC3-working-group-reports/GC3-Science-and-Technology-Working-Group-Final-Report-11-19-20.pdf (accessed October 12, 2022).
Connecticut Department of Energy and Environmental Protection (2021b). Connecticut’s young forest habitat initiative. Available online at: https://portal.ct.gov/DEEP/Wildlife/Habitat/Young-Forest-Habitat-Initiative (accessed November 5, 2022).
Connecticut Department of Energy and Environmental Protection (2021a). Native American use of prescribed fire. Available online at: https://portal.ct.gov/DEEP/Forestry/Native-American-Use-of-Prescribed-Fire (accessed November 5, 2022).
Cook-Patton, S. C., Leavitt, S. M., Gibbs, D., Harris, N. L., Lister, K., Anderson-Teixeira, K. J., et al. (2020). Mapping carbon accumulation potential from global natural forest regrowth. Nature 585, 545–550. doi: 10.1038/s41586-020-2686-x
Cooper-Ellis, S., Foster, D. R., Carlton, G., and Lezberg, A. (1999). Forest response to catastrophic wind: Results from an experimental hurricane. Ecology 80, 2683–2696. doi: 10.1890/0012-96581999080[2683:FRTCWR]2.0.CO;2
Cottam, G., and Loucks, O. L. (1965). Early vegetation of Wisconsin University of Wisconsin. Extension Geological and natural history survey department of botany. The University of Wisconsin. Available online at: https://wgnhs.wisc.edu/pubshare/M035.pdf (accessed November 5, 2022).
Cousineau, M. (2017). NH fish and game to take ownership of cottontail habitat. Union Leader, 2 October. Available online at: https://newenglandcottontail.org/news/nh-fish-and-game-take-ownership-cottontail-habitat (accessed November 5, 2022).
Coyle, D. R., Nagendra, U. J., Taylor, M. K., Campbell, J. H., Cunard, C. E., Joslin, A. H., et al. (2017). Soil fauna responses to natural disturbances, invasive species, and global climate change: Current state of the science and a call to action. Soil Biol. Biochem. 110, 116–133. doi: 10.1016/j.soilbio.2017.03.008
Cullinane, T. C., Flyr, M., and Koontz, L. (2022). 2021 national park visitor spending effects: economic contributions to local communities, states, and the nation. Natural resource report NPS/NRSS/EQD/NRR—2022/2395. Fort Collins, CO: National Park Service. doi: 10.36967/nrr-2293346
Curtis, P. S., and Gough, C. M. (2018). Forest aging, disturbance and the carbon cycle. N. Phytol. 219, 1188–1193. doi: 10.1111/nph.15227
D’Amato, A. W., Bradford, J. B., Fraver, S., and Palik, B. J. (2011). Forest management for mitigation and adaptation: Insights from long-term silvicultural experiments. For. Ecol. Manag. 262, 803–816. doi: 10.1016/j.foreco.2011.05.014
D’Amato, A. W., Orwig, D. A., and Foster, D. R. (2006). New estimates of Massachusetts old-growth forests: Useful data for regional conservation and forest reserve planning. Northeastern Naturalist 13, 495–506. doi: 10.1656/1092-6194200613[495:NEOMOF]2.0.CO;2
D’Amato, A. W., Orwig, D. A., and Foster, D. R. (2009). Understory vegetation in old-growth and second-growth tsuga canadensis forests in Western Massachusetts. For. Ecol. Manag. 257, 1043–1052. doi: 10.1016/j.foreco.2008.11.003
D’Amato, A. W., Orwig, D. A., Foster, D. R., Plotkin, A. B., Schoonmaker, P. K., and Wagner, M. R. (2017). Long-term structural and biomass dynamics of virgin Tsuga canadensis–Pinus strobus forests after hurricane disturbance. Ecology 98, 721–733. doi: 10.1002/ecy.1684
Davis, M. B. (2003). Old growth in the east: a survey. Revised edition. Mount Vernon, KY: Appalachia-Science in the Public Interest, 40456.
Davis, M. B. (ed.) (1996). Eastern old-growth forests/prospects for rediscovery and recovery. Washington, DC: Island Press, 383.
Dawson, D. K., Bently Wigley, T., and Keyser, P. D. (2012). Cerulean warbler technical group: Coordinating international research and conservation. Ornitol. Neotropical 23, 273–280.
Day, G. M. (1953). The Indian as an ecological factor in the Northeastern forest. Ecology 34, 329–346. doi: 10.2307/1930900
deCalesta, D. S. (1994). Effect of white-tailed deer on songbirds within managed forests in Pennsylvania. J. Wildl. Manag. 58, 711–718.
Dechen Quinn, A. C., Williams, D. M., and Porter, W. F. (2013). Landscape structure influences space use by white-tailed deer. J. Mammal. 94, 398–407. doi: 10.1644/11-MAMM-A-221.1
DeGraaf, R. M., and Yamasaki, M. (2003). Options for managing early-successional forest and shrubland bird habitats in the Northeastern United States. For. Ecol. Manag. 185, 179–191. doi: 10.1016/S0378-1127(03)00254-8
Delcourt, P. A., and Delcourt, H. R. (1998). The influence of prehistoric human-set fires on oak- chestnut forests in the Southern Appalachians. Castanea 63, 337–345.
DellaSala, D. A., Baker, B. C., Hanson, C. T., Ruediger, L., and Baker, W. (2022b). Have Western USA fire suppression and megafire active management approaches become a contemporary Sisyphus? Biol. Conserv. 268:109499. doi: 10.1016/j.biocon.2022.109499
DellaSala, D. A., Mackey, B., Norman, P., Campbell, C., Comer, P. J., Kormos, C. F., et al. (2022a). Mature and old-growth forests contribute to large-scale conservation targets in the conterminous United States. Front. For. Glob. Change 5:979528. doi: 10.3389/ffgc.2022.979528
DellaSala, D. A., Middelveen, M. J., Liegner, K. B., and Luché-Thayer, J. (2018). Lyme disease epidemic increasing globally due to climate change and human activities. Encycl. Anthropocene 2, 441–451. doi: 10.1016/B978-0-12-809665-9.10516-6
Denevan, W. M. (1992). The pristine myth: The landscape of the Americas in 1492. Ann. Assoc. Am. Geogr. 82, 369–385. doi: 10.1111/j.1467-8306.1992.tb01965.x
Depro, B. M., Murray, B. C., Alig, R. J., and Shanks, A. (2008). Public land, timber harvests, and climate mitigation: Quantifying carbon sequestration potential on U.S. Public Timberlands. For. Ecol. Manag. 255, 1122–1134. doi: 10.1016/j.foreco.2007.10.036
Derosier, A. L., Hanshue, S. K., Wehrly, K. E., Farkas, J. K., and Nichols, M. J. (2015). Michigan’s wildlife action plan: young forests. Lansing: Michigan Department of Natural Resources.
Di Marco, M., Ferrier, S., Harwood, T. D., Hoskins, A. J., and Watson, J. E. M. (2019). Wilderness areas halve the extinction risk of terrestrial biodiversity. Nature 573, 582–585. doi: 10.1038/s41586-019-1567-7
Dinerstein, E., Joshi, A. R., Vynne, C., Lee, A. T. L., Pharand-Deschênes, F., França, M., et al. (2020). A “Global Safety Net” to reverse biodiversity loss and stabilize Earth’s climate. Sci. Adv. 6:eabb2824. doi: 10.1126/sciadv.abb2824
Dinerstein, E., Vynne, C., Sala, E., Joshi, A. R., Fernando, S., Lovejoy, T. E., et al. (2019). A global deal for nature: Guiding principles, milestones, and targets. Sci. Adv. 5:eaaw2869. doi: 10.1126/sciadv.aaw2869
Domke, G., Williams, C. A., Birdsey, R., Coulston, J., Finzi, A., Gough, C., et al. (2018). “Chapter 9: Forests,” in Second state of the carbon cycle report (SOCCR2): A sustained assessment report, eds N. Cavallaro, G. Shrestha, R. Birdsey, M. A. Mayes, R. G. Najjar, S. C. Reed, et al. (Washington, DC: U.S. Global Change Research Program), 365–398. doi: 10.7930/SOCCR2.2018.Ch9
Dorazio, R. M., Connor, E. F., and Askins, R. A. (2015). Estimating the effects of habitat and biological interactions in an avian community. PLoS One 10:e0135987. doi: 10.1371/journal.pone.0135987
Ducey, M. J., Whitman, A. A., and Gunn, J. (2013). Late-successional and old-growth forests in the northeastern United States: Structure, dynamics, and prospects for restoration. Forests 4, 1055–1086. doi: 10.3390/f4041055
Duffy, D. C., and Meier, A. J. (1992). Do appalachian herbaceous understories ever recover from clearcutting? Conserv. Biol. 6, 196–201. doi: 10.1046/j.1523-1739.1992.620196.x
Dunn, E. H., Francis, C. M., Blancher, P. J., Drennan, S. R., Howe, M. A., Lepage, D., et al. (2005). Enhancing the scientific value of the christmas bird count. Auk 122, 338–346. doi: 10.1642/0004-80382005122[0338:ETSVOT]2.0.CO;2
Dunn, E., Johnson, D. H., Jones, S. L., O’Connor, R. J., Petit, D., Pollock, K., et al. (2000). A programmatic review of the North American breeding bird survey: Report of a peer review panel to USGS Patuxent. Laurel, MD: USGS Patuxent Wildlife Research Center.
Dunwiddie, P. W., and Leverett, R. T. (1996). Survey of old-growth forests in Massachusetts. Rhodora 98, 419–444.
Dunwiddie, P. W., Foster, D. R., Leopold, D. J., and Leverett, R. T. (1996). “Old-growth forests of southern New England, New York, and Pennsylvania,” in Eastern old-growth forests: prospects for rediscovery and recovery, ed. M. B. Davis (Washington, D.C: Island Press), 126–143.
Duveneck, M. J., and Thompson, H. R. (2019). Social and biophysical determinants of future forest conditions in New England: Effects of a modern land-use regime. Glob. Environ. Change 55, 115–129. doi: 10.1016/j.gloenvcha.2019.01.009
Dwyer, J. F. (2003). “Urban perceptions of national forests: Three examples from the Northern United States,” in Proceedings of the 2002 northeastern recreation research symposium. Gen. Tech. Rep. NE-302, eds Schuster, Rudy, Comp, (Newtown Square, PA: U.S. Department of Agriculture, Forest Service, Northeastern Research Station), 159–162.
Eriksson, L., Nordlund, A. M., Olsson, O., and Westin, K. (2012). Recreation in different forest settings: A scene preference study. Forests 3, 923–943. doi: 10.3390/f3040923
Eschtruth, A. K., and Battles, J. J. (2009). Assessing the relative importance of disturbance, herbivory, diversity, and propagule pressure in exotic plant invasion. Ecol. Monogr. 265–280. doi: 10.1890/08-0221.1
Estes, J. A., Terborgh, J., Brashares, J. S., Power, M. E., Berger, J., Bond, W. J., et al. (2011). Trophic downgrading of planet earth. Science 333, 301–306. doi: 10.1126/science.1205106
Faaborg, J., Brittingham, M., Donovan, T., and Blake, J. (1993). “Habitat fragmentation in the temperate zone: A perspective for managers,” in Status and management of neotropical migratory birds: September 21-25, 1992, Estes Park, Colorado. Gen. Tech. Rep. RM-229, eds Finch, M. Deborah, Stangel, and W. Peter (Fort Collins, CO: Rocky Mountain Forest and Range Experiment Station, U.S. Dept. of Agriculture, Forest Service), 331–338.
Fahey, T. J., Siccama, T. G., Driscoll, C. T., Likens, G. E., Campbell, J., Johnson, C. E., et al. (2005). The biogeochemistry of carbon at hubbard brook. Biogeochemistry 75, 109–176. doi: 10.1007/s10533-004-6321-y
Faison, E. K., Foster, D. R., and Oswald, W. W. (2006). Early Holocene openlands in southern New England. Ecology 87, 2537–2547. doi: 10.1890/0012-9658200687[2537:EHOISN]2.0.CO;2
Faison, E., DeStefano, S., Foster, D., and Barker Plotkin, A. (2016). Functional response of ungulate browsers in disturbed eastern hemlock forests. For. Ecol. Manag. 362, 177–183. doi: 10.1016/j.foreco.2015.12.006
FAO, and UNEP (2020). The state of the world’s forests 2020. Forests, biodiversity and people. Rome: FAO, doi: 10.4060/ca8642en
Farmer, R. G., Leonard, M. L., Mills Flemming, J. E., and Anderson, S. C. (2014). Observer aging and long-term avian survey data quality. Ecol Evol. 4, 2563–2576. doi: 10.1002/ece3.1101
Fergus, C. (2014). The young forest project: helping wildlife through stewardship and science. Washington, DC: Wildlife Management Institute.
Fernandez, L., Mukherjee, M., and Scott, T. (2018). The effect of conservation policy and varied open space on residential property values: A dynamic hedonic analysis. Land Use Policy 73, 480–487. doi: 10.1016/j.landusepol.2017.12.058
Finzi, A. C., Giasson, M., Barker Plotkin, A. A., Aber, J. D., Boose, E. R., Eric, A., et al. (2020). Carbon budget of the harvard forest long-term ecological research site: Pattern, process, and response to global change. Ecol. Monogr. 90:e01423. doi: 10.1002/ecm.1423
Fletcher, R. A., Brooks, R. K., Lakoba, V. T., Sharma, G., Heminger, A. R., Dickinson, C. C., et al. (2019). Invasive plants negatively impact native, but not exotic, animals. Glob Change Biol. 25, 1–12. doi: 10.1111/gcb.14752
Forman, R. T. T., and Boerner, R. E. (1981). Fire frequency and the pine barrens of New Jersey. Bull. Torrey Botanical Club 108, 34–50. doi: 10.2307/2484334
Forman, R. T. T., and Russell, E. W. B. (1983). Evaluation of historical data in ecology. Bull. Ecol. Soc. Am. 64, 5–7.
Foster, D. R. (1995). “Land-use history and four hundred years of vegetation change in New England,” in Global land use change: a perspective from the columbian encounter, SCOPE publication, eds B. L. Turner, A. G. Sal, F. G. Bernaldez, and F. DiCastri (Madrid: Consejo Superior de Investigaciones Cientificas).
Foster, D. R. (2002). Thoreau’s country: A historical–ecological perspective on conservation in the New England landscape. J. Biogeogr. 29, 1537–1555. doi: 10.1046/j.1365-2699.2002.00786.x
Foster, D. R. (2017). A meeting of land and sea. Nature and the future of Martha’s Vineyard. New Haven, CT: Yale University Press, 352.
Foster, D. R., and Motzkin, G. (2003). Interpreting and conserving the openland habitats of coastal New England. For. Ecol. Manag. 185, 127–150. doi: 10.1016/S0378-1127(03)00251-2
Foster, D. R., and Orwig, D. A. (2006). Preemptive and salvage harvesting of New England forests: When doing nothing is a viable alternative. Conserv. Biol. 20, 959–970. doi: 10.1111/j.1523-1739.2006.00495.x
Foster, D. R., Johnson, E., Hall, B., Leibowitz, J., Thompson, E., Donahue, B., et al. (2023). Wildlands in New England. past, present and future. Petersham, MA: Harvard Forest, Highstead Foundation and Northeast Wilderness Trust.
Foster, D. R., Lambert, K. F., Kittredge, D., Donahue, B., Hart, C., Labich, W., et al. (2017). Wildlands and woodlands, farmlands and communities: broadening the vision for New England. Petersham, MA: Harvard Forest.
Foster, D. R., Motzkin, G., Bernardos, D., and Cardoza, J. (2002). Wildlife dynamics in the changing New England landscape. J. Biogeogr. 29, 1337–1357. doi: 10.1046/j.1365-2699.2002.00759.x
Foster, D., Swanson, F., Aber, J., Burke, I., Brokaw, N., Tilman, D., et al. (2003). The importance of land-use legacies to ecology and conservation. Bioscience 77–88. doi: 10.1641/0006-35682003053[0077:TIOLUL]2.0.CO;2
Fraver, S., White, A. S., and Seymour, R. S. (2009). Natural disturbance in an old-growth landscape of Northern Maine, USA. J. Ecol. 97, 289–298. doi: 10.1111/j.1365-2745.2008.01474.x
Frelich, L. E. (1995). Old forest in the lake states today and before European settlement. Natural Areas J. 15, 157–167.
Frelich, L. E. (2002). Forest dynamics and disturbance regimes. Cambridge: Cambridge University Press.
Frelich, L. E., and Reich, P. B. (1995). Spatial patterns and succession in a Minnesota Southern-boreal forest. Ecol. Monogr. 65, 325–346. doi: 10.2307/2937063
Frelich, L. E., and Reich, P. B. (2010). Will environmental changes reinforce the impact of global warming on the prairie-forest border of central North America? Front. Ecol. Environ. 8:371–378. doi: 10.1890/080191
Frelich, L. E., Blossey, B., Cameron, E. K., Davalos, A., Eisenahuer, N., Fahey, T., et al. (2019). Side swiped: Ecological cascades emanating from earthworm invasion. Front. Ecol. Environ. 17:502–510. doi: 10.1002/fee.2099
Frelich, L. E., Lorimer, C. G., and Stambaugh, M. C. (2021). “History and future of fire in hardwood and conifer forests of the Great Lakes-Northeastern forest region, USA,” in Fire ecology and management: past, present, and future of US forested ecosystems, eds C. H. Greenberg and B. Collins (New York: Springer), 243–285.
Fuller, S., and Tur, A. (2012). Conservation strategy for the New England Cottontail (Sylvilagus transitionalis). US Fish & wildlife publications. Paper 320. Washington, DC: U.S. Fish and Wildlife Service.
Gassett, J. (2018). The young forest initiative - southern appalachian style. Outdoor news bulletin volume 72, Issue 3, 16 Mar. Available online at: https://wildlifemanagement.institute/outdoor-news-bulletin/march-2018/young-forest-initiative-southern-appalachian-style (accessed November 6, 2022).
Gilhen-Baker, M., Roviello, V., Beresford-Kroeger, D., and Roviello, N. (2022). Old growth forests and large old trees as critical organisms connecting ecosystems and human health. A review. Environ. Chem. Lett. 20, 1529–1538. doi: 10.1007/s10311-021-01372-y
Giocomo, J., Vermillion, W., DeMaso, S., and Panjabi, A. (2017). How many are there? Estimating the North American Northern bobwhite population size for conservation planning purposes. Natl Quail Symposium Proc. 8:36.
Godt, M. J. W., Hamrick, J. L., and Meier, A. (2004). Genetic diversity in Cymophyllus fraserianus (Cyperaceae), a rare monotypic Genus. Genetica 122, 207–215. doi: 10.1023/b:gene.0000041049.91375.8c
Greeley, W. B. (1925). The relation of geography to timber supply. Econ. Geogr. 1:1. doi: 10.2307/140095
Gunn, J. S., Ducey, M. J., Andrew, A., and Whitman, A. A. (2014). Late-successional and old-growth forest carbon temporal dynamics in the Northern Forest (Northeastern USA). For. Ecol. Manag. 312, 40–46. doi: 10.1016/j.foreco.2013.10.023
Gunn, J. S., Duceya, M. J., and Belair, E. (2019). Evaluating degradation in a North American temperate forest. For. Ecol. Manag. 432, 415–426. doi: 10.1016/j.foreco.2018.09.046
Haefele, M., Loomis, J., and Bilmes, L. J. (2016). Total economic value of US national park service estimated to be $92 billion: Implications for policy. George Wright Forum 33, 335–345.
Hagan, J. M., Vander Haegen, W. M., and McKinley, P. S. (1996). The early development of forest fragmentation effects on birds. Conserv. Biol. 10, 188–202. doi: 10.1046/j.1523-1739.1996.10010188.x
Hamburg, S. P., Vadeboncoeur, M. A., Johnson, C. E., and Sanderman, J. (2019). Losses of mineral soil carbon largely offset biomass accumulation fifteen years after whole-tree harvest in a northern hardwood forest. Biogeochemistry 144, 1–14. doi: 10.1007/s10533-019-00568-3
Hanberry, B. B., and Thompson, F. R. III. (2019). Open forest management for early successional birds. Wildl. Soc. Bull. 43, 141–151. doi: 10.1002/wsb.957
Handler, S., Pike, C., and St. Clair, B. (2018). Assisted migration. USDA Forest service climate change resource center. Available online at: https://www.fs.usda.gov/ccrc/topics/assisted-migration (accessed November 5, 2011).
Hansen, M. C., Potapov, P. V., Moore, R., Hancher, M., Turubanova, S. A., Tyukavina, et al. (2013). High-resolution global maps of 21st-century forest cover change. Science 342, 850–853. doi: 10.1126/science.1244693
Hansen, M. M., Jones, R., and Tocchini, K. (2017). Shinrin-Yoku (forest bathing) and nature therapy: A state-of-the-art review. Int. J. Environ. Res. Public Health 14:851. doi: 10.3390/ijerph14080851
Harmon, M. E. (2019). Statement From Dr. Mark E. Harmon, Professor Emeritus to the United States house natural resources committee subcommittee on national parks, forests, and public lands concerning the hearing on climate change and public lands: examining impacts and considering adaptation opportunities committee. Testimony date: 21 February. Available online at: https://docs.house.gov/meetings/II/II10/20190213/108911/HHRG-116-II10-20190213-SD012.pdf (accessed November 5, 2022).
Harmon, M. E., Ferrell, W. K., and Franklin, J. F. (1990). Effects on carbon storage of conversion of old-growth forests to young forests. Science 247, 699–702. doi: 10.1126/science.247.4943.699
Harmon, M. E., Harmon, J. M., Ferrell, W. K., and Brooks, D. (1996). Modeling carbon stores in Oregon and Washington forest products: 1900-1992. Climatic Change 33, 1996. doi: 10.1007/BF00141703
Harris, N. L., Hagen, S. C., Saatchi, S. S., Pearson, T. R. H., Woodall, C. W., Domke, G. M., et al. (2016). Attribution of net carbon change by disturbance type across forest lands of the conterminous United States. Carbon Balance Manag. 11:24. doi: 10.1186/s13021-016-0066-5
Hassler, K. N., Waggy, C. D., Spinola, R. M., Oxenrider, K. J., Rodgers, R. E., Pearce, K. J., et al. (2021). Den-site selection by Eastern Spotted Skunks in the central Appalachian mountains of West Virginia. Southeastern Naturalist Southeastern Naturalist 20, 209–224. doi: 10.1656/058.020.0sp1118
Hawthorne, B. (2020). Overview of masswildlife carbon analysis. Massachusetts. Available online at: https://www.mass.gov/doc/overview-of-masswildlife-carbon-analysis/download (accessed November 5, 2022).
Heeter, K. J., Brosi, S. L., and Brewer, G. L. (2019). Dendroecological analysis of xeric, upland, Quercus-dominated old-growth forest within the Ridge and Valley Province of Maryland, USA. Natural Areas J. 39, 319–332. doi: 10.3375/043.039.0304
Heinselman, M. L. (1973). Fire in the virgin forests of the Boundary Waters Canoe Area, Minnesota. Quaternary Res. 3, 329–382. doi: 10.1016/0033-5894(73)90003-3
Hilmers, T., Friess, N., Båssler, C., Heurich, M., Brandl, R., Pretzsch, H., et al. (2018). Biodiversity along temperate forest succession. J. Appl. Ecol. 55, 2756–2766. doi: 10.1111/1365-2664.13238
Hocking, D. J., Babbitt, K. J., and Yamasaki, M. (2013). Comparison of silvicultural and natural disturbance effects on terrestrial salamanders in Northern Hardwood forests. Biol. Conserv. 167, 194–202. doi: 10.1016/j.biocon.2013.08.006
Holmes, F. P., and Hecox, W. E. (2004). Does wilderness impoverish rural regions? Int. J. Wilderness 10, 34–39.
Hoover, J., Brittingham, M., and Goodrich, L. (1995). Effects of forest patch size on nestling success of wood thrushes. Auk 112, 146–155. doi: 10.2307/4088774
Huijser, M. P., and Clevenger, A. P. (2006). “Chapter 11: Habitat and corridor function of rights-of-way,” in The ecology of transportation: managing mobility for the environment, eds J. Davenport and J. L. Davenport (Dordrecht: Springer), 233–254. doi: 10.1007/1-4020-4504-2_11
Infrastructure Investment and Jobs Act (2021). Pub. L. No. 117–58 117 135 Stat. 429. Available online at: https://www.congress.gov/117/plaws/publ58/PLAW-117publ58.pdf (accessed November 5, 2022).
Insurance Information Institute (2021). Facts + statistics: Deer VEHICLE COLLISIONS. Available online at: https://www.iii.org/fact-statistic/facts-statistics-deer-vehicle-collisions (accessed July 29, 2022).
Ingerson, A. (2011). Carbon storage potential of harvested wood: Summary and policy implications. Mitig Adapt Strateg Glob. Change 16, 307–323. doi: 10.1007/s11027-010-9267-5
Irland, L. (2013). Extreme value analysis of forest fires from New York to Nova Scotia, 1950-2010. For. Ecol. Manag. 294, 150–157. doi: 10.1016/j.foreco.2012.09.004
Irland, L. (2014). What happened to S. New England fires? They virtually disappeared over a few decades in the Mid 20th Century. The northern logger and timber processor. Old Forge, NY: Northeastern Loggers’ Association.
IUCN (2012). IUCN Red list categories and criteria, version 3.1, Second Edition. As approved by the 51st meeting of the IUCN Council Gland, Switzerland 9 February 2000. Gland: IUCN, 32.
James, J., and Harrison, R. (2016). The effect of harvest on forest soil carbon: A meta-analysis. Forests 7:308. doi: 10.3390/f7120308
Janowiak, M. K., D’Amato, A. W., Swanston, C. W., Iverson, L., Thompson, F. R. III., Dijak, W., et al. (2018). New England and Northern New York forest ecosystem vulnerability assessment and synthesis: a report from the New England climate change response framework project. Gen. Tech. Rep. NRS-173. Newtown Square, PA: U.S. Department of Agriculture, Forest Service, Northern Research Station, 234. doi: 10.2737/nrs-gtr-173
Jenkins, C. N., Van Houtan, K. S., Pimm, S. L., and Sexton, J. O. (2015). US protected lands mismatch biodiversity priorities. Proc. Natl. Acad. Sci. USA 112, 5081–5086. doi: 10.1073/pnas.1418034112
Jenkins, J., and Kroeger, A. (2020). Seeing the forest: Sustainable wood bioenergy in the Southeast United States. Enviva White Paper 1, 5/2020. Bethesda, MD: Enviva Inc.
Jeswiet, S., and Hermsen, L. (2015). Agriculture and wildlife: a two-way relationship. Catalogue no. 16−002−X ISSN 1913-4320. Ottawa, ON: Statistics Canada.
Jirinec, V., Cristol, D. A., and Leu, M. (2017). Songbird community varies with deer use in a fragmented landscape. Landsc. Urban Plan. 161:1. doi: 10.1016/j.landurbplan.2017.01.003
Johnson, L. B., and Kipfmueller, K. F. (2016). A fire history derived from Pinus resinosa Ait. for the Islands of Eastern Lac La Croix, Minnesota, USA. Ecol. Appl. 26, 1030–1046. doi: 10.1890/15-1151
Jones, J. J. (2011). Transforming the cutover: the establishment of national forests in Northern Michigan. Durham: Forest History Today, Spring/Fall.
Jordan, K. A. (2013). Incorporation and colonization: Postcolumbian iroquois satellite communities and processes of indigenous autonomy. Am. Anthropol. 115, 29–43. doi: 10.1111/j.1548-1433.2012.01533.x
Jordan, S. F., and Black, S. H. (2012). Effects of Forest land management on terrestrial mollusks: a literature review. Portland: The Xerces Society for Invertebrate Conservation.
Karjalainen, E., Sarjala, T., and Raitio, H. (2010). Promoting human health through forests: Overview and major challenges. Environ. Health Prev. Med. 15, 1–8. doi: 10.1007/s12199-008-0069-2
Keeton, W. S., Whitman, A. A., McGee, G. C., and Goodale, C. L. (2011). Late-successional biomass development in northern hardwood-conifer forests of the Northeastern United States. For. Sci. 57:2011. doi: 10.1093/forestscience/57.6.489
Kelley, J. R. Jr., Williamson, S., and Cooper, T. R. (2008). American woodcock conservation plan: a summary of and recommendations for woodcock conservation in North America. U.S. Fish and Wildlife Publications. Washington. D.C: U.S. Fish and Wildlife Service, 430.
Ketcham, C. (2022). Is clear-cutting U.S. forests good for wildlife? National geographic, 24 March. Available online at: https://www.nationalgeographic.com/environment/article/is-clear-cutting-us-forests-good-for-wildlife (accessed November 6, 2022).
Kilgore, M. A., and Ek, A. R. (2013). Minnesota forest age-class distribution, 2011. Minnesota forestry research notes, No. 295. St. Paul, MN: University of Minnesota, Forest Resources Department.
Kim, H., McComb, B. C., Frey, S. J. K., Bell, D. M., and Betts, M. G. (2022). Forest microclimate and composition mediate long-term trends of breeding bird populations. Glob. Change Biol. 28, 6180–6193. doi: 10.1111/gcb.16353
King, D. I., and Schlossberg, S. (2014). Synthesis of the conservation value of the early-successional stage in forests of Eastern North America. For. Ecol. Manag. 324, 186–195. doi: 10.1016/j.foreco.2013.12.001
King, D. I., Chandler, R. B., Collins, J. M., Petersen, W. R., and Lautzenheiser, T. E. (2009). Effects of width, edge and habitat on the abundance and nesting success of scrub–shrub birds in powerline corridors. Biol. Conserv. 2672–2680. doi: 10.1016/j.biocon.2009.06.016
King, D. I., DeGraaf, R. M., and Griffin, C. R. (2001). Productivity of early successional shrubland birds in clearcuts and groupcuts in an eastern deciduous forest. J. Wildl. Manag. 65, 345–350. doi: 10.2307/3802914
King, D. I., Schlossberg, S., Brooks, R. T., and Akresh, M. E. (2011). Effects of fuel reduction on birds in pitch pine–scrub oak barrens of the United States. For. Ecol. Manag. 261, 10–18. doi: 10.1016/j.foreco.2010.08.039
Kipfmueller, K. F., Schneider, E. A., Weyenberg, S. A., and Johnson, L. B. (2017). Historical drivers of a frequent fire regime in the red pine forests of Voyageurs National Park, MN, USA. For. Ecol. Manag. 405, 31–43. doi: 10.1016/j.foreco.2017.09.014
Knapp, W. M., and Wiegand, R. (2014). Orchid (Orchidaceae) decline in the Catoctin Mountains, Frederick County, Maryland as documented by a long-term dataset. Biodivers. Conserv. 23, 1965–1976. doi: 10.1007/s10531-014-0698-2
Knowlton, J. (2017). Continuing the conservation legacy: centennial of the weeks act of 1911. USDA forest service. Available online at: https://www.usda.gov/media/blog/2011/03/02/continuing-conservation-legacy-centennial-weeks-act-1911 (accessed November 6, 2022).
Koches, J. (2019). Hurricane hugo and the woodpeckers: the silver lining of a monster storm. U.S. Fish and Wildlife Service, 21 February. Available online at: https://web.archive.org/web/20220207182013/https://www.fws.gov/southeast/articles/hurricane-hugo-and-the-woodpeckers-the-silver-lining-of-a-monster-storm/ (accessed November 6, 2022).
Kormos, C. F., Mackey, B., DellaSala, D. A., Kumpe, N., Jaeger, T., Mittermeier, R. A., et al. (2018). “Primary forests: definition, status and future prospects for global conservation,” in The Encyclopedia of the Anthropocene, Vol. 2, eds A. Dominick, DellaSala, I. Michael, and Goldstein (Oxford: Elsevier), 31–41.
Krebs, J., Pontius, J., and Schaberg, P. G. (2017). Modeling the impacts of hemlock woolly adelgid infestation and presalvage harvesting on carbon stocks in northern hemlock forests. Can. J. For. Res. 47, 727–734. doi: 10.1139/cjfr-2016-0291
Lacroix, E., Petrenko, C. L., and Friedland, A. J. (2016). Evidence for losses from strongly bound SOM pools after clear cutting in a northern hardwood forest. Soil Sci. 181, 202–207. doi: 10.1097/SS.0000000000000147
Lain, E. J., Haney, A., Burris, J. M., and Burton, J. (2008). Response of vegetation and birds to severe wind disturbance and salvage logging in a southern boreal forest. For. Ecol. Manag. 256, 863–871. doi: 10.1016/j.foreco.2008.05.018
Lapin, M. (2005). Old-growth forests: a literature review of the characteristics of eastern North American forests. Montpelier: Vermont Natural Resources Council.
Lashley, M. A., Harper, C. A., Bates, G. E., and Keyser, P. D. (2011). Forage availability for white-tailed deer following silvicultural treatments in hardwood forests. J. Wildl. Manag. 75, 1467–1476. doi: 10.1002/jwmg.176
Law, B. E., Hudiburg, T. W., Berner, T., Kent, J. J., Buotte, P. C., and Harmon, M. E. (2018). Land use strategies to mitigate climate change in carbon dense temperate forests. Proc. Natl. Acad. Sci. USA 115, 3663–3668. doi: 10.1073/pnas.1720064115
Law, B. E., Moomaw, W. R., Hudiburg, T. W., Schlesinger, W. H., Sterman, J. D., and Woodwell, G. M. (2022). Creating strategic reserves to protect forest carbon and reduce biodiversity losses in the United States. Land 11, 721.
Lawrence, D., Coe, M., Walker, W., Verchot, L. L., and Vandecar, K. (2022). The unseen effects of deforestation: Biophysical effects on climate. Front. For. Glob. Change 5:756115. doi: 10.3389/ffgc.2022.756115
LeDoux, C. B., and Martin, D. K. (2013). Proposed BMPs for invasive plant mitigation during timber harvesting operations. General technical report NRS-118. Washington, DC: USDA Forest Service, Northern Research Station. doi: 10.2737/NRS-GTR-118
Lee-Ashley, M. (2019). How much nature should America keep? Center for American progress public lands team and oceans team. Available online at: https://cdn.americanprogress.org/content/uploads/2019/08/05133927/NatureAmerica-report.pdf (accessed November 6, 2022).
Lenarz, M. S. (1987). Economics of forest openings for white-tailed deer. Wildl. Soc. Bull. 15, 568–573.
Leturcq, P. (2020). GHG displacement factors of harvested wood products: The myth of substitution. Sci. Rep. 10:20752. doi: 10.1038/s41598-020-77527-8
Leverett, R. T. (1996). “Definitions and history,” in Eastern old-growth forests: Prospects for rediscovery and recovery, ed. M. B. Davis (Washington, DC: Island Press), 3–17.
Leverett, R. T., Masino, S. A., and Moomaw, W. R. (2021). Older eastern white pine trees and stands sequester carbon for many decades and maximize cumulative carbon. Front. For. Glob. Change 4:620450. doi: 10.3389/ffgc.2021.620450
Levi, T., Kilpatrick, A. M., Mangel, M., and Wilmers, C. C. (2012). Deer, predators, and the emergence of lyme disease. Proc Natl Acad Sci U S A 109, 10942–10947. doi: 10.1073/pnas.1204536109
Lindenmayer, D. B., and Laurance, W. F. (2012). A history of hubris – cautionary lessons in ecologically sustainable forest management. Biol. Conserv. 151, 11–16. doi: 10.1016/j.biocon.2011.10.032
Littlefield, C. E., and D’Amato, A. W. (2022). Identifying trade-offs and opportunities for forest carbon and wildlife using a climate change adaptation lens. Conserv. Sci. Pract. 2022:e12631. doi: 10.1111/csp2.12631
Litvaitis, J. A. (1993). Response of early successional vertebrates to historic changes in land use. Conserv. Biol. 7, 866–873. doi: 10.1046/j.1523-1739.1993.740866.x
Litvaitis, J. A. (2003). Are pre-Columbian conditions relevant baselines for managed forests in the northeastern United States? For. Ecol. Manag. 185, 113–126. doi: 10.1016/S0378-1127(03)00250-0
LoGiudice, K., Ostfeld, R. S., Schmidt, K. A., and Keesing, F. (2003). The ecology of infectious disease: Effects of host diversity and community composition on Lyme disease risk. Proc. Natl. Acad. Sci. U.S.A. 100, 567–571. doi: 10.1073/pnas.0233733100
Lombardi, J. V., Mengak, M. T., Castleberry, S. B., and Terrell, V. K. (2017). Mammal occurrence in rock outcrops in shenandoah national park: Ecological and anthropogenic factors influencing trap success and co-occurrence. Natural Areas J. 37, 507–514. doi: 10.3375/043.037.0407
Lorah, P., and Southwick, R. (2003). Environmental protection, population change, and economic development in the rural Western United States. Population Environ. 24, 255–272. doi: 10.1023/A:1021299011243
Lorimer, C. G. (1977). The presettlement forest and natural disturbance cycle of northeastern Maine. Ecology 58, 139–148. doi: 10.2307/1935115
Lorimer, C. G. (1980). Age structure and disturbance history of a southern Appalachian virgin forest. Ecology 61, 1169–1184. doi: 10.2307/1936836
Lorimer, C. G., and White, A. S. (2003). Scale and frequency of natural disturbances in the Northeastern US: Implications for early successional forest habitats and regional age distributions. For. Ecol. Manag. 185, 41–64. doi: 10.1016/S0378-1127(03)00245-7
Lu, X., Kicklighter, D. W., Melillo, J. M., Yang, P., Rosenzweig, B., Vörösmarty, C. J., et al. (2013). A contemporary carbon balance for the northeast region of the United States. Environ. Sci. Technol. 47, 13230–13238. doi: 10.1021/es403097z
Luedke, H. (2019). Fact sheet: nature as resilient infrastructure: an overview of nature-based solutions. environmental and energy study institute. Available online at: https://www.eesi.org/files/FactSheet_Nature_Based_Solutions_1016.pdf (accessed November 6, 2022).
Luyssaert, S., Schulze, E. D., Börner, A., Knohl, A., Hessenmöller, D., Beverly, E., et al. (2008). Old-growth forests as global carbon sinks. Nature 455, 213–221. doi: 10.1038/nature07276
Lyme Timber Company (2017). Kunjamuk young forest demonstration project. Available online at: http://lymetimber.com/wp/wp-content/uploads/2017/12/KunjamukYoungForestFactSheet.pdf (accessed November 6, 2022).
Mackey, B., DellaSala, D. A., Kormos, C., Lindenmayer, D., Kumpel, N., Zimmerman, B., et al. (2014). Policy options for the world’s primary forests in multilateral environmental agreements. Conserv. Lett. 8, 139–147. doi: 10.1111/conl.12120
Mackey, B., Kormos, C. F., Keith, H., Moomaw, W. R., Houghton, R. A., Mittermeier, R. A., et al. (2020). Understanding the importance of primary tropical forest protection as a mitigation strategy. Mitig. Adapt Strateg Glob Change 25, 763–787. doi: 10.1007/s11027-019-09891-4
Mackey, B., Prentice, I., Steffen, W., House, J. I., Lindenmayer, D., Keith, H., et al. (2013). Untangling the confusion around land carbon science and climate change mitigation policy. Nat. Clim. Change 3, 552–557. doi: 10.1038/nclimate1804
Maes, M. J. A., Pirani, M., Booth, E. R., Shen, C., Milligan, B., Jones, K. E., et al. (2021). Benefit of woodland and other natural environments for adolescents’ cognition and mental health. Nat. Sustain. 4, 851–858. doi: 10.1038/s41893-021-00751-1
Makarieva, A. M., Nefiodov, A. V., Morozov, V. E., Aleynikov, A. A., and Vasilov, R. G. (2020). Science in the vanguard of rethinking the role of forests in the third millennium: Comments on the draft concept of the federal law “forest code of the russian federation”. For. Clim. Issues 3, 1–25. doi: 10.31509/2658-607x-2020-3-3-1-25
Maloof, J. (2023). Nature’s temples: a natural history of old-growth forests revised and expanded. Princeton, NJ: Princeton University Press, 240.
Mann, C. C. (2005). 1491: new revelations of the Americas before Columbus. New York, NY: Alfred A. Knopf.
Marks, P. L. (1983). On the origin of the field plants of the Northeastern United States. Am. Naturalist 122, 210–228.
Marschner, F. J. (1975). The original vegetation of Minnesota (map). St. Paul, MN: USDA Forest Service, North Central Forest Experiment Station.
Marshall, M., DeCecco, J. A., Williams, A. B., Gale, G. A., and Cooper, R. J. (2003). Use of regenerating clearcuts by late-successional bird species and their young during the post-fledging period. For. Ecol. Manag. 183, 127–135. doi: 10.1016/S0378-1127(03)00101-4
Martin, W. H. (1992). Characteristics of old-growth mixed mesophytic forests. Natural Areas J. 12, 127–135.
Mason, J., Moorman, C., Hess, G., and Sinclair, K. (2007). Designing suburban greenways to provide habitat for forest-breeding birds. Landsc. Urban Plan. 80, 153–164. doi: 10.1016/j.landurbplan.2006.07.002
Massachusetts Audubon Society (2013). State of the birds 2013: Massachusetts breeding birds: a closer look. Massachusetts: Lincoln.
Massachusetts Department of Conservation and Recreation (2018). Sykes mountain forest management proposal. Available online at: https://www.mass.gov/files/documents/2018/02/09/Sykes%20Mountain%20final%20posted.pdf (accessed November 6, 2022).
Massachusetts Department of Conservation and Recreation (2022). Managing our forests. For carbon benefits. Available online at: https://www.mass.gov/info-details/managing-our-forests-for-carbon-benefits (accessed November 5, 2022).
Massachusetts Division of Fisheries and Wildlife (2022a). Wood harvest, mowing, and mulching for habitat management. Available online at: https://www.mass.gov/service-details/wood-harvest-mowing-and-mulching-for-habitat-management (accessed November 6, 2022).
Massachusetts Division of Fisheries and Wildlife (2022b). masswildlife’s habitat goals. Available online at: https://www.mass.gov/service-details/masswildlifes-habitat-goals (accessed November 6, 2022).
Matlack, G. R. (2013). Reassessment of the use of fire as a management tool in deciduous forests of Eastern North America. Conserv. Biol. 27, 916–926. doi: 10.1111/cobi.12121
McCarthy, B. C., and Bailey, D. R. (1996). Composition, structure, and disturbance history of crabtree woods: An old-growth forest of Western Maryland. Bull. Torrey Bot. Club 123, 350–365. doi: 10.2307/2996783
McDonald, R. I., Motzkin, G., and Foster, D. R. (2008). Assessing the influence of historical factors, contemporary processes, and environmental conditions on the distribution of invasive species. J. Torrey Bot. Soc. 135, 260–271. doi: 10.3159/08-RA-012.1
McEwan, R. W., Dyer, J. M., and Pederson, N. (2011). Multiple interacting ecosystem drivers: Towards an encompassing hypothesis of oak forest dynamics across eastern North America. Ecography 34, 244–256. doi: 10.1111/j.1600-0587.2010.06390.x
McGarvey, J. C., Thompson, J. R., Epstein, H. E., and Shugart, H. H. (2015). Carbon storage in old-growth forests of the Mid-Atlantic: Toward better understanding the eastern forest carbon sink. Ecology 96, 311–317. doi: 10.1890/14-1154.1
McGraw, J. B., Lubbers, A. E., Van der Voort, M., Mooney, E. H., Furedi, M. A., Souther, S., et al. (2013). Ecology and conservation of ginseng (Panax quinquefolius) in a changing world. Ann. N.Y. Acad. Sci. 1286, 62–91. doi: 10.1111/nyas.12032
McKinley, D. C., Ryan, M. G., Birdsey, R. A., Giardina, C. P., Harmon, M. E., Heath, L. S., et al. (2011). A synthesis of current knowledge on forests and carbon storage in the United States. Ecol. Applic. 21, 1902–1924. doi: 10.1890/10-0697.1
Melvin, M. A. (2020). National prescribed fire use report. Technical bulletin 04-20. Washington, DC: National Association of State Foresters and the Coalition of Prescribed Fire Councils.
Meyer, S. R., MacLeod, K. K., Thompson, J., Macleod, K. K., Foster, D. R., Perschel, R., et al. (2022). New England’s climate imperative: our forests as a natural climate solution. Redding, CT: Highstead Foundation.
Michigan Department of Natural Resources (2016). Michigan deer management plan, wildlife division report No. 3626. Lansing, MI: Michigan Department of Natural Resources.
Michigan Department of Natural Resources (2017). Deer range improvement program (DRIP) report. Available online at: https://www.michigan.gov/documents/dnr/DRIP_project_report_607688_7.pdf (accessed November 6, 2022).
Michigan Department of Natural Resources (2022). Prescribed burns. Lansing, MI: Michigan Department of Natural Resources.
Mika, A. M., and Keeton, W. S. (2013). Factors contributing to carbon fluxes from bioenergy harvests in the US Northeast: An analysis using field data. GCB Bioenergy 5, 290–305. doi: 10.1111/j.1757-1707.2012.01183.x
Millar, C., Stephenson, N. L., and Stephens, S. L. (2007). Climate change and forests of the future: Managing in the face of uncertainty. Ecol. Applic. 17, 2145–2151. doi: 10.1890/06-1715.1
Miller, K. M., Dieffenbach, F. W., Campbell, J. P., Cass, W. B., Comiskey, J. A., Matthews, E. R., et al. (2016). National parks in the Eastern United States harbor important older forest structure compared with matrix forests. Ecosphere 7:e01404. doi: 10.1002/ecs2.1404
Miller, K. M., McGill, B. J., Mitchell, B. R., Comiskey, J., Dieffenbach, F. W., Matthews, E. R., et al. (2018). Eastern national parks protect greater tree species diversity than unprotected matrix forests. For. Ecol. Manag. 414, 74–84. doi: 10.1016/j.foreco.2018.02.018
Miller-Weeks, M., Eagar, C., and Petersen, C. M. (1999). The Northeastern ice storm 1998, a forest damage assessment for New York, Vermont, New Hampshire, and Maine. Waterbury, VT: North East State Foresters Association, 32.
Milner, G. R., and Chaplin, G. (2010). Eastern North American population at ca. A.D. 1500. Am. Antiquity 75, 707–726. doi: 10.7183/0002-7316.75.4.707
Minnesota Department of Natural Resources (2016). State wildlife action plans: revitalizing conservation in America. St. Paul, MN: Minnesota Department of Natural Resources.
Mladenoff, D. J., and Forrester, J. A. (2018). “Historical patterns and contemporary processes in northern lake states old-growth landscapes,” in Ecology and recovery of eastern old-growth forests, eds A. M. Barton and W. S. Keeton (Washington, DC: Island Press), doi: 10.5822/978-1-61091-891-6_7
Mladenoff, D. J., Schulte, L. A., and Bolliger, J. (2008). “Broad-scale changes in the Northern Forests: From past to present,” in The vanishing present: Wisconsin’s changing lands, waters, and wildlife, eds D. Waller and T. Rooney (Chicago, IL: University of Chicago Press), doi: 10.7208/chicago/9780226871745.003.0005
Moomaw, W. R., Masino, S. A., and Faison, E. K. (2019). Intact forests in the United States: Proforestation mitigates climate change and serves the greatest good. Front. For. Glob. Change 11:27. doi: 10.3389/ffgc.2019.00027
Mora, C., Tittensor, D. P., Adl, S., Simpson, A. G. B., and Worm, B. (2011). How many species are there on earth and in the ocean? PLoS Biol. 9:e1001127. doi: 10.1371/journal.pbio.1001127
Morton, P. (1998). The economic benefits of wilderness: Theory and practice. Denver. Law. Rev 76:465.
Mosseler, A., Major, J. E., and Rajora, O. P. (2003a). Old-growth red spruce forests as reservoirs of genetic diversity and reproductive fitness. Theor. Appl. Genet. 106, 931–937. doi: 10.1007/s00122-002-1156-1
Mosseler, A., Thompson, I., and Pendrel, B. A. (2003b). Overview of old-growth forests in Canada from a science perspective. Environ. Rev. 11, S1–S7. doi: 10.1139/a03-018
Motzkin, G., and Foster, D. R. (2002). Grasslands, heathlands and shrublands in coastal New England: Historical interpretations and approaches to conservation. J. Biogeogr. 29, 1569–1590. doi: 10.1046/j.1365-2699.2002.00769.x
Motzkin, G., Patterson, W. A III., and Foster, D. R. (1999). A historical perspective on pitch pine–scrub oak communities in the Connecticut Valley of Massachusetts. Ecosystems 2, 255–273. doi: 10.1007/s100219900073
Moustakis, Y., Papalexiou, S. M., Onof, C. J., and Paschalis, A. (2021). Seasonality, intensity, and duration of rainfall extremes change in a warmer climate. Earths Fut. 9:e2020EF001824. doi: 10.1029/2020EF001824
Munoz, S. E., and Gajewski, K. (2010). Distinguishing prehistoric human influence on late-Holocene forests in southern Ontario, Canada. Holocene 20, 967–981. doi: 10.1177/0959683610362815
Munoz, S. E., Mladenoff, D. J., Schroeder, S., and Williams, J. W. (2014). Defining the spatial patterns of historical land use associated with the indigenous societies of eastern North America. J. Biogeogr. 41, 2195–2210. doi: 10.1111/jbi.12386
Nareff, G. E., Wood, P. B., Brown, D. J., Fearer, T., Larkin, J. L., and Ford, W. M. (2019). Cerulean Warbler (Setophaga cerulea) response to operational silviculture in the central Appalachian region. For. Ecol. Manag. 448, 409–423. doi: 10.1016/j.foreco.2019.05.062
National Commission on Science for Sustainable Forestry (2007). Conserving biodiversity through sustainable forestry. Available online at: https://www.ddcf.org/globalassets/news-and-publications/imported-news-and-publications/conserving-biodiversity-through-sustainable-forestry.pdf (accessed November 5, 2022).
National Fish and Wildlife Foundation (2022a). New England Forests and Rivers Fund. Available online at: https://www.nfwf.org/programs/new-england-forests-and-rivers-fund (accessed November 5, 2022).
National Fish and Wildlife Foundation (2022b). Central appalachia habitat stewardship program. Available online at: https://www.nfwf.org/programs/central-appalachia-habitat-stewardship-program (accessed November 5, 2022).
National Wildlife Federation (2009). Increased flooding risk: global warming’s wake-up call for riverfront communities. Reston, VA: National Wildlife Federation.
Natural Resources Conservation Service (2018). Private landowner response to NRCS young forest programs. U.S. department of agriculture. Available online at: https://www.nrcs.usda.gov/publications/ceap-wildlife-2018-LandownerResponse-YoungForest.pdf (accessed November 5, 2022).
Natural Resources Conservation Service (2019). The young forest initiative for at-risk species. U.S. department of agriculture. Available online at: https://web.archive.org/web/20210324212730/https://www.nrcs.usda.gov/wps/portal/nrcs/detail/me/programs/farmbill/rcpp/?cid=nrcseprd1322729 (accessed November 5, 2022).
NatureServe (2022). NatureServe network biodiversity location data accessed through NatureServe explorer [web application]. Available online at: https://explorer.natureserve.org/ (accessed November 5, 2022).
Nave, L. E., Vance, E. D., Swanston, C. W., and Curtis, P. S. (2010). Harvest impacts on soil carbon storage in temperate forests. For. Ecol. Manag. 259, 857–866. doi: 10.1016/j.foreco.2009.12.009
Neff, C. (2017). Keeping common species common. New Jersey Audubon. 25 May. Available online at: https://njaudubon.org/keeping-common-species-common/ (accessed November 5, 2022).
New England Cottontail (2021). Monterey preservation land trust, berkshires, Massachusetts: young forest project delivers multiple benefits. Available online at: https://newenglandcottontail.org/demo/monterey-preservation-land-trust-berkshires-massachusetts (accessed November 5, 2022).
New Jersey Audubon (2018). Sparta mountain wildlife management area forest stewardship plan. Available online at: https://njaudubon.org/wp-content/uploads/2018/04/New-Jersey-Audubon-Sparta-Mountain-WMA_handout.pdf (accessed November 5, 2022).
New Jersey Department of Environmental Protection (2017). Sparta mountain wildlife management area stewardship plan. Available online at: https://www.nj.gov/dep/fgw/sparta/smwma_approved_forest_stewardship_plan.pdf (accessed November 5, 2022).
New Jersey Department of Environmental Protection (2018). New Jersey’s wildlife action plan. Trenton, NJ: New Jersey Department of Environmental Protection.
New York Department of Environmental Conservation (2015). A DEC strategic plan for implementing the young forest initiative on wildlife management areas 2015-2020. Albany, NY: New York Department of Environmental Conservation.
New York Department of Environmental Conservation (2021). Assessing old-growth forests in New York state forests and preserves. New York natural heritage program. Available online at: https://www.nynhp.org/ogre/ (accessed November 5, 2022).
Newman, D. J., and Cragg, G. M. (2016). Natural products as sources of new drugs from 1981 to 2014. J. Nat. Prod. 79, 629–661. doi: 10.1021/acs.jnatprod.5b01055
Nitschke, C. R. (2005). Does forest harvesting emulate fire disturbance? A comparison of effects on selected attributes in coniferous-dominated headwater systems. For. Ecol. Manag. 214, 305–319. doi: 10.1016/j.foreco.2005.04.015
Nolan, V. Jr. (1978). The ecology and behavior of the prairie warbler dendroica discolor. Ornithological monographs. No. 26. Washington, DC: American Ornithologists’ Union.
North American Bird Conservation Initiative (2014). The state of the birds 2014 report. Washington, DC: U.S. Department of Interior, 16.
North American Bird Conservation Initiative (2019). The State of the birds 2019 report: America’s birds in crisis. Gatineau, QC: North American Bird Conservation Initiative.
North, M. P., and Keeton, W. S. (2008). “Emulating natural disturbance regimes: An emerging approach for sustainable forest management,” in Patterns and processes in forest landscapes, eds R. Lafortezza, G. Sanesi, J. Chen, and T. Crow (Berlin: Springer), 341–372. doi: 10.1007/978-1-4020-8504-8_19
Northern Institute of Applied Climate Science (2022). U.S. fish and wildlife service and university of vermont: Nulhegan Basin, Silvio O. conte national fish and wildlife refuge adaptation demonstration project. Available online at: https://forestadaptation.org/adapt/demonstration-projects/us-fish-and-wildlife-service-and-university-vermont-nulhegan-basin (accessed November 5, 2022).
Noss, R. F. (1983). A regional landscape approach to maintain diversity. Bioscience 33, 700–706. doi: 10.2307/1309350
Noss, R. F., Dinerstein, E., Gilbert, B., Gilpin, M., Miller, B., Terborgh, J. J., et al. (1999). “Core areas: Where nature reigns,” in Continental conservation: scientific foundations of regional reserve networks, eds M. E. Soulé and J. Terborgh (Washington, DC: Island Press), 99–128.
Noss, R. F., Dobson, A. P., Baldwin, R., Beier, P., Davis, C. R., Dellasala, D. A., et al. (2012). Bolder thinking for conservation. Conserv. Biol. 26, 1–4. doi: 10.1111/j.1523-1739.2011.01738.x
Noss, R. F., Platt, W. J., Sorrie, B. A., Weakley, A. S., Means, B. D., Costanza, J., et al. (2014). How global biodiversity hotspots may go unrecognized: Lessons from the North American Coastal Plain. Divers. Distributions 21, 236–244. doi: 10.1111/ddi.12278
Nowacki, G. J., and Abrams, M. D. (2008). The demise of fire and “mesophication” of forests in the eastern United States. Bioscience 58, 123–138. doi: 10.1641/b580207
Nunery, J. S., and Keeton, W. S. (2010). Forest Carbon Storage in the Northeastern United States: Effects of harvesting frequency and intensity including wood products. For. Ecol. Manag. 259, 13631375. doi: 10.1016/j.foreco.2009.12.029
Oehler, J. D. (2003). State efforts to promote early-successional habitats on public and private lands in the northeastern United States. For. Ecol. Manag. 185, 169–177. doi: 10.1016/s0378-1127(03)00253-6
Oehler, J. D., Covell, D. F., Capel, S., and Long, B. (2006). Managing grasslands, shrublands and young forest habitats for wildlife. Augusta, ME: Northeast Upland Habitat Technical Committee.
Oehler, J., Gifford, N., Fergus, C., Edwards, T., Racey, M., and Allred, S. (2013). Talking about young forests: A communication handbook. Hadley, MA: Northeast Association of Fish and Wildlife Agencies.
Office of Senator Angus King (2022). King introduces bill to improve access to katahdin woods and waters. newsroom/press releases, 10 August. Available online at: https://www.king.senate.gov/newsroom/press-releases/king-introduces-bill-to-improve-access-to-katahdin-woods-and-waters (accessed November 22, 2022).
Oliver, C. D., and Larson, B. A. (1996). Forest Stand dynamics, update edition Yale school of the environment other publications. New York, NY: John Wiley & Sons.
Oliveri, S. F. (1993). Bird responses to habitat changes in baxter state park, Maine. Maine Naturalist 1:145. doi: 10.2307/3858237
Oswald, W. W., Foster, D. R., Shuman, B. N., Chilton, E. S., Doucette, D. L., Deena, L., et al. (2020b). Conservation implications of limited native american impacts in pre-contact New England. Nat. Sustain. 3, 241–246. doi: 10.1038/s41893-019-0466-0
Oswald, W. W., Foster, D. R., Shuman, B. N., Chilton, E. S., Doucette, D. L., and Duranleau, D. L. (2020a). W. W. Oswald et al. reply to M. D. Abrams and G. J. Nowacki. Nature Sustainability. (2020). Nat. Sustain. 3, 900–903. doi: 10.1038/s41893-020-0580-z
Oswalt, S. N., Smith, W. B., Miles, P. D., and Pugh, S. A. (2019). Forest resources of the United States, 2017: a technical document supporting the Forest Service 2020 RPA Assessment. Gen. Tech. Rep. WO-97. Washington, DC: U.S. Department of Agriculture, Forest Service, Washington Office, 223.
Paciorek, C. J., Cogbill, C. V., Peters, J. A., Williams, J. W., Mladenoff, D. J., Dawson, A., et al. (2021). The forests of the midwestern United States at Euro-American settlement: Spatial and physical structure based on contemporaneous survey data. PLoS One 16:e0246473. doi: 10.1371/journal.pone.0246473
Palik, B. J., Clark, P. W., D’Amato, A. W., Swanston, C., and Nagel, L. (2022). Operationalizing forest-assisted migration in the context of climate change adaptation: Examples from the Eastern USA. Ecosphere 13:e4260. doi: 10.1002/ecs2.4260
Pan, Y., Chen, J. M., Birdsey, R., McCullough, K., He, L., and Deng, F. (2011). Age structure and disturbance legacy of North American forests. Biogeosciences 8, 715–732. doi: 10.5194/bg-8-715-2011
Paradis, A., Elkinton, J., Hayhoe, K., and Buonaccorsi, J. (2008). Role of winter temperature and climate change on the survival and future range expansion of the hemlock woolly adelgid (Adelges tsugae) in eastern North America. Mitig. Adapt Strateg Glob. Change 13, 541–554. doi: 10.1007/s11027-007-9127-0
Parajuli, R. (2022). The infrastructure act and forestry: a brief overview. North Carolina state extension, NC State University. Available online at: https://forestry.ces.ncsu.edu/2022/01/iija-forestry/ (accessed November 5, 2022).
Park, A., and Talbot, C. (2018). Information underload: Ecological. Bioscience 68, 251–263. doi: 10.1093/biosci/biy001
Parshall, T., and Foster, D. R. (2002). Fire on the New England landscape: Regional and temporal variation, cultural and environmental controls. J. Biogeogr. 29, 1305–1317. doi: 10.1046/j.1365-2699.2002.00758.x
Parshall, T., Foster, D. R., Faison, E., MacDonald, D., and Hansen, B. C. S. (2003). Long-term history of vegetation and fire in pitch pine–oak forests on cape cod, Massachusetts. Ecology 84, 736–748. doi: 10.1890/0012-96582003084[0736:LTHOVA]2.0.CO;2
Paskus, J. J., Pearsall, D. R., and Ross, J. A. (2015). Facilitating the effectiveness of state wildlife action plans at multiple scales in the upper midwest/great lakes LCC: Findings and recommendations. Report number MNFI 2016-02, 56 pp. + appendices. East Lansing, MI: US Fish and Wildlife Service, Upper Midwest Great Lakes Landscape Conservation Cooperative.
Pavlovic, N. B., and Grundel, R. (2009). Reintroduction of wild lupine (Lupinus perennis L.) depends on variation in canopy, vegetation, and litter cover. Restorat. Ecol. 17, 807–817. doi: 10.1111/j.1526-100X.2008.00417.x
Peabody, W. B. O. (1839). A report on the ornithology of Massachusetts. In reports on the fishes, reptiles and birds of Massachusetts. Published agreeable to an order of the legislature, by the commissioners on the zoological and botanical survey of the state. Boston, MA: Zoological and botanical survey, 255–404.
Pearce, K. J., Serfass, T. L., McCann, J. M., and Feller, D. J. (2021). Status and distribution of the Eastern Spotted Skunk (Spilogale putorius) in Maryland: A historic review and recent assessment. Southeastern Naturalist 20, 52–63. doi: 10.1656/058.020.0sp1106
Pederson, N. (2013). Eastern OLDLIST: a database of maximum ages for Eastern North America. Available online at: https://www.ldeo.columbia.edu/~adk/oldlisteast/ (accessed October 2, 2022).
Pederson, N., D’Amato, A. W., Dyer, J. M., Foster, D. R., Goldblum, D., Hart, J. L., et al. (2014). Climate remains an important driver of post-European vegetation change in the Eastern United States. Glob. Chang. Biol. 21, 2105–2110. doi: 10.1111/gcb.12779
Pellerito, R., and Wisch, R. (2002). State endangered species chart. Animal legal & historical center, Michigan State University College of Law. Available online at: https://www.animallaw.info/article/state-endangered-species-chart (accessed November 5, 2022).
Pelley, J. (2009). Old-growth forests store a treasure trove of carbon. Environ. Sci. Technol. 43, 7602–7603. doi: 10.1021/es902647k
Peterken, G. F. (1996). Natural woodland: ecology and conservation in northern temperate regions. Cambridge: Cambridge University Press.
Petranka, J. W., Brannon, M. P., Hopey, M. E., and Smith, C. K. (1994). Effects of timber harvesting on low elevation populations of southern appalachian salamanders. For. Ecol. Manag. 67, 135–147. doi: 10.1016/0378-1127(94)90012-4
Petrenko, C. L., and Friedland, A. J. (2015). Mineral soil carbon pool responses to forest clearing in northeastern hardwood forests. GCB Bioenergy 7, 1283–1293. doi: 10.1111/gcbb.12221
Phillips, S. (2004). The economic benefits of wilderness: focus on property value enhancement. The Wilderness Society. Avalable at: https://web.archive.org/web/20140809204303if_/http://wilderness.org/sites/default/files/The-Economic-Benefits-of-Wilderness-With-a-Focus-on-Land-Value-Enhancement_low-res.pdf (accessed November 5, 2022).
Plenzler, M. A., and Michaels, H. J. (2015). Seedling recruitment and establishment of Lupinus perennis in a mixed-management landscape. Natural Areas J. 35, 224–234. doi: 10.3375/043.035.0203
Plotkin, A. B., Foster, D., Carlson, J., and Magill, A. (2013). Survivors, not invaders, control forest development following simulated hurricane. Ecology 94, 414–423. doi: 10.1890/12-0487.1
Potter, C. (2022). Forest service pressing ahead with logging around lake. Valley News, 25 April. Available online at: https://www.vnews.com/New-comment-period-opens-on-Lake-Tarleton-proposed-logging-46053192 (accessed November 6, 2022).
Poulos, L. P., and Roy, B. A. (2015). Fire and false brome: How do prescribed fire and invasive Brachypodium sylvaticum affect each other? Invas. Plant Sci. Manag. 8, 122–130. doi: 10.1614/IPSM-D-14-00024.1
Power, T. M. (1996). Wilderness economics must look through the windshield, not the rear-view mirror. Int. J. Wilderness 2, 5–9.
Power, T. M. (2001). The economic impact of the proposed Maine woods national park & preserve. RESTORE. Hallowell, ME: The North Woods.
Pyne, S. J. (2000). Where have all the fires gone? Fire management today, Vol. 60. Washington, DC: USDA Forest Service.
Raiho, A. M., Paciorek, C. J., Dawson, A., Jackson, S. T., Mladenoff, D. J., and Williams, J. W. (2022). 8000-year doubling of Midwestern forest biomass driven by population- and biome-scale processes. Science 376:1491. doi: 10.1126/science.abk3126
Rasker, R., Gude, P. H., and Delorey, M. (2013). The effect of protected federal lands on economic prosperity in the non-metropolitan west. J. Regional Anal. Policy 43, 110–122.
Reynolds, M. T. (2021). National parks overcrowding. Statement before the senate energy and natural resources subcommittee onn national parks, 28 July. Available online at: https://www.doi.gov/ocl/national-parks-overcrowding (accessed October 12, 2022).
Rhemtulla, J. M., and Mladenoff, D. J. (2007). Regional land-cover conversion in the U.S. upper Midwest: Magnitude of change and limited recovery (1850-1935-1993). Landsc. Ecol. 22, 57–75. doi: 10.1007/s10980-007-9117-3
Rhemtulla, J. M., Mladenoff, D. J., and Clayton, M. K. (2009). Historical forest baselines reveal potential for continued carbon sequestration. Proc. Natl. Acad. Sci. U.S.A. 106, 6082–6087. doi: 10.1073/pnas.081007610
Rhode Island Division of Statewide Planning and Rhode Island Department of Environmental Management (2019). Ocean state outdoors: Rhode Island’s comprehensive outdoor recreation plan: State guide plan element 152, Report No. 122. Providence, RI: Rhode Island Division of Statewide Planning and Rhode Island Department of Environmental Management.
Riitters, K. H., Potter, K. M., Iannone, B. V. III., Oswalt, C., Guo, Q., and Fei, S. (2018). Exposure of protected and unprotected forest to plant invasions in the Eastern United States. Forests 9:723. doi: 10.3390/f9110723
Robertson, D. L., Babin, L. M., Krall, J. R., von Fricken, M. E., Baghi, H., and Jacobsen, K. H. (2019). The association between hunter-killed deer and lyme disease in New Jersey, 2000–2014. Ecohealth 16, 330–337. doi: 10.1007/s10393-019-01401-
Rogers, B. M., Mackey, B., Shestakova, T. A., Keith, H., Young, V., Kormos, C. F., et al. (2022). Using ecosystem integrity to maximize climate mitigation and minimize risk in international forest policy. Front. For. Glob. Change 5:929281. doi: 10.3389/ffgc.2022.929281
Rohrbaugh, R. W., Treyger, S., McGinley, K., and Loucks, K. (2020). Healthy forests: A bird-based silvicultural guide for forestry professionals. Audubon, PA: Pennsylvania Chapter, National Audubon Society, 40.
Rooney, T., and Waller, D. (2003). Direct and indirect effects of white-tailed deer in forest ecosystems. For. Ecol. Manag. 181, 165–176. doi: 10.1016/S0378-1127(03)00130-0
Rosa, L., and Malcom, J. (2020). Getting to 30X30: guidelines for decision-makers. Washington, DC: Defenders of Wildlife.
Rosenberg, K. B., Blancher, P. J., Stanton, J. C., and Panjabi, A. O. (2017). Use of North American breeding bird survey data in avian conservation assessments. Condor 119, 594–606. doi: 10.1650/CONDOR-17-57.1
Rosenberg, K. V., Dokter, A. M., Blancher, P. J., Sauer, J. R., Smith, A. C., Smith, P. A., et al. (2019). Decline of the North American Avifauna. Science 366, 120–124. doi: 10.1126/science.aaw1313
Rosenberg, K. V., Hames, R. S., Rohrbaugh, R. W., Swarthout, S. B., Lowe, J. D., and Dhondt, A. A. (2003). A land manager’s guide to improving habitat for forest thrushes. Ithaca, NY: The Cornell Lab of Ornithology.
Rosenberg, K. V., Kennedy, J. A., Dettmers, R., Ford, R. P., Reynolds, D., Alexander, J. D., et al. (2016). Partners in flight landbird conservation plan: 2016 revision for Canada and Continental United States. Partners in flight science committee. Washington, DC: North American Bird Conservation Initiative (NABCI), Association of Fish and Wildlife Agencies, 119.
Ruddat, J. (2022). An inventory of Connecticut’s primeval woodlands. Rhodora. No. 995. Cambridge, MA: New England Botanical Club.
Ruffed Grouse Society (2022). RGS & AWS and partners awarded forest service landscape scale restoration grant in Massachusetts, 19 July. Available online at: https://ruffedgrousesociety.org/rgs-aws-and-partners-awarded-forest-service-landscape-scale-restoration-grant-in-massachusetts/ (accessed November 5, 2022).
Runkle, J. R. (1982). Patterns of disturbance in some old-growth mesic forests of eastern North America. Ecology 63, 1533–1546. doi: 10.2307/1938878
Russell, E. W. B. (1981). Vegetation of Northern New Jersey before European settlement. Am. Midland Naturalist 105, 1–12. doi: 10.2307/2425004
Russell, E. W. B. (1983). Indian-set fires on the forests of the Northeastern United States. Ecology 64, 78–88. doi: 10.2307/1937331
Russell, T. (2017). The 3 biggest obstacles to creating young forest cover. National deer association (aka Quality Deer Management Association), 27 March. Available online at: https://www.deerassociation.com/3-biggest-obstacles-creating-young-forest-cover/ (accessed November 5, 2022).
Santoro, J. A., and D’Amato, A. W. (2019). Structural, compositional, and functional responses to tornado and salvage logging disturbance in southern New England hemlock-hardwood forests. For. Ecol. Manag. 444, 138–150. doi: 10.1016/j.foreco.2019.04.039
Sauer, J. R., Link, W. A., and Hines, J. E. (2020). The North American breeding bird survey, analysis results 1966 – 2019. Laurel, MD: U.S. Geological Survey data release. Eastern Ecological Science Center. doi: 10.5066/P96A7675
Sauer, J. R., Link, W. A., Fallon, J. E., Pardieck, K. L., and Ziolkowski, D. J. (2013). The North American breeding bird survey 1966–2011: Summary analysis and species accounts. North Am. Fauna 79, 1–32. doi: 10.3996/nafa.79.0001
Sauer, J. R., Pardieck, K. L., Ziolkowski, D. J., Smith, A. C., Hudson, M. R., Rodriguez, et al. (2017). The first 50 years of the North American Breeding Bird Survey. Condor 119, 576–593. doi: 10.1650/CONDOR-17-83.1
Scheller, R. M., Van Tuyl, S., Clark, K., Hayden, N. G., Hom, J., and Mladenoff, D. J. (2008). Simulation of forest change in the New Jersey Pine Barrens under current and pre-colonial conditions. For. Ecol. Manag. 255, 1489–1500. doi: 10.1016/j.foreco.2007.11.025
Schlaghamerský, J., Eisenhauer, N., and Frelich, L. E. (2014). Earthworm invasion alters enchytraeid community composition and individual biomass in northern hardwood forests of North America. Appl. Soil Ecol. 83, 159–169. doi: 10.1016/j.apsoil.2013.09.005
Schlossberg, S., and King, D. I. (2007). Ecology and management of scrub-shrub birds in New England: A comprehensive review. Washington, DC: U.S. Department of Agriculture Natural Resource Conservation Service, Resource Inventory and Assessment Division.
Schlossberg, S., King, D. I., Destefano, S., and Hartley, M. (2018). Effects of early-successional shrubland management on breeding wood thrush populations. J. Wildl. Manag. 82, 1572–1581. doi: 10.1002/jwmg.21559
Schulte, L. A., Mladenoff, D. J., Burrows, S. N., Sickley, T. A., and Nordheim, E. V. (2005a). Spatial controls of Pre-Euro-American wind and fire in northern Wisconsin (USA) forest landscapes. Ecosystems 8, 73–94. doi: 10.1007/s10021-004-0052-8
Schulte, L. A., Pidgeon, A. M., and Mladenoff, D. J. (2005b). One hundred fifty years of change in forest bird breeding habitat: Estimates of species distributions. Conserv. Biol. 19, 1944–1956. doi: 10.1111/j.1523-1739.2005.00254.x
Schultz, J. (2003). Conservation assessment for butternut or white walnut (Juglans cinerea) L. Milwaukee, WI: USDA Forest Service, Eastern Region.
Schulz, F., Alteio, L., Goudeau, D., Ryan, E. M., Yu, F. B., Malmstrom, R. S., et al. (2018). Hidden diversity of soil giant viruses. Nat. Commun. 9:4881. doi: 10.1038/s41467-018-07335-2
Schulze, E. D., Korner, C., Law, B., Haberl, H., and Luyssaert, S. (2012). Large-scale bioenergy from additional harvest of forest biomass is neither sustainable nor greenhouse gas neutral. GCB Bioenergy 10, 1–6. doi: 10.1111/j.1757-1707.2012.01169.x
Schwartz, M. W., Hellmann, J. J., McLachlan, J. M., Sax, D. F., Borevitz, J. O., Brennan, J., et al. (2012). Integrating the scientific, regulatory, and ethical challenges. Bioscience 62, 732–743. doi: 10.1525/bio.2012.62.8.6
Scott, J. M., Davis, F. W., McGhie, R. G., Wright, R. G., Groves, C., and Estes, J. (2001). Nature reserves: Do they capture the full range of America’s biological diversity? Ecol. Applic. 11, 999–1007. doi: 10.1890/1051-07612001011[0999:NRDTCT]2.0.CO;2
Seamans, M. E., and Rau, R. D. (2018). American woodcock population status, 2018. Laurel, MD: U.S. Fish and Wildlife Service.
Seidl, R., Thom, D., Kautz, M., Martin-Benito, D., Peltoniemi, M., Vacchiano, G., et al. (2017). Forest disturbances under climate change. Nat. Clim. Change 7, 395–402. doi: 10.1038/nclimate3303
Seitz, G. (2019). Fourth of July marks 20th anniversary of boundary waters blowdown. Quetico superior wilderness news, 1 July. Available online at: https://queticosuperior.org/blog/fourth-of-july-marks-20th-anniversary-of-boundary-waters-blowdown/ (accessed November 6, 2022).
Seng, P. T., and Case, D. J. (2019). “Communicating effectively about young forest management to benefit associated wildlife species,” in Proceedings of the Eleventh American Woodcock Symposium, eds D. G. Krementz, D. E. Andersen, and T. R. Cooper (Minneapolis, MIN: University of Minnesota Libraries Publishing), 67–75. doi: 10.24926/AWS.0109
Seymour, R. S., White, A. S., and deMaynadier, P. G. (2002). Natural disturbance regimes in Northeastern North America — evaluating silvicultural systems using natural scales and frequencies. For. Ecol. Manag. 155, 357–367. doi: 10.1016/S0378-1127(01)00572-2
Sharon, S. (2022). USDA grants $30 million for increased carbon storage in New England forests. Maine Public, 14 September. Lewiston, ME: Maine Public.
Sheikh, P. A. (2011). Forest management for resilience and adaptation (CRS Report No. R41691). Washington, DC: Congressional Research Service.
Shuman, B. N., Marsicek, J., Oswald, J. W., and Foster, D. R. (2019). Predictable hydrological and ecological responses to Holocene North Atlantic variability. Proc. Natl. Acad. Sci. U.S.A. 116, 5985–5990. doi: 10.1073/pnas.1814307116
Shuman, B., Newby, P., Huang, Y., and Webb, T. III. (2004). Evidence for the close climatic control of New England vegetation history. Ecology 85, 1297–1310. doi: 10.1890/02-0286
Shumway, D. L., Abrams, M. D., and Ruffner, C. M. (2001). A 400-year history of fire and oak recruitment in an old-growth oak forest in Western Maryland, USA. Can. J. For. Res. 31, 1437–1443. doi: 10.1139/cjfr-31-8-1437
Simard, S. (2021). Finding the mother tree: discovering the wisdom of the forest. New York, NY: Knopf Doubleday Publishing Group, 368.
Simard, S. W., Beiler, K. J., Bingham, M. A., Deslippe, J. R., Philip, L. J., and Teste, F. P. (2012). Mycorrhizal networks: mechanisms, ecology and modelling. Fungal Biol. Rev. 26, 39–60. doi: 10.1016/j.fbr.2012.01.001
Small, M. F., and Hunter, M. L. (1988). Forest fragmentation and avian nest predation in forested landscapes. Oecologia 76, 62–64. doi: 10.1007/bf00379601
Smith, J. (2017). The American woodcock, and why we should be cutting more trees. the nature conservancy, 28 March. Available online at: https://blog.nature.org/science/2017/03/28/american-woodcock-why-cutting-more-trees-logging-forests/ (accessed November 6, 2022).
Smith, J. E., Heath, L. S., Skog, K. E., and Birdsey, R. A. (2006). Methods for calculating forest ecosystem and harvested carbon with standard estimates for forest types of the United States. Gen. Tech. Rep. NE-343. Newtown Square, PA: U.S. Department of Agriculture, Forest Service, Northeastern Research Station, 216. doi: 10.2737/NE-GTR-343
South Carolina Department of Natural Resources (2020). Technical guidance for the development of wildlife and pollinator habitat at solar farms: South Carolina solar habitat act. Columbia: South Carolina Department of Natural Resources.
Southwell, D. K. (2001). Conservation assessment for prairie warbler (Dendroica discolor). Washington, DC: USDA Forest Service.
Spies, T. A., and Franklin, J. F. (1991). “The structure of natural young, mature, and old-growth Douglas-fir forests in Oregon and Washington,” in Wildlife and vegetation of unmanaged Douglas-fir forests. General technical report PNW-GTR-85, eds L. F. Ruggiero, K. B. Aubry, A. B. Carey, and M. Huff (Portland, OR: U.S. Department of Agriculture Forest Service, Pacific Northwest Research Station), 91–109.
Stauffer, G. E., Miller, D. A. W., Williams, L. M., and Brown, J. (2018). Ruffed grouse population declines after introduction of West Nile virus. J. Wild. Mgmt. 82, 165–172. doi: 10.1002/jwmg.21347
Steidinger, B. S., Crowther, T. W., Liang, J., Van Nuland, M. E., Werner, G. D. A., Reich, P. B., et al. (2019). Climatic controls of decomposition drive the global biogeography of forest-tree symbioses. Nature 569, 404–408. doi: 10.1038/s41586-019-1128-0
Sterman, J., Moomaw, W., Rooney-Varga, J. N., and Siegel, L. (2022). Does wood bioenergy help or harm the climate? Bull. Atomic Sci. 78, 3,128–138. doi: 10.1080/00963402.2022.2062933
Stevens, A. (1996). The paleoecology of coastal sandplain grasslands on Martha’s Vineyard, Massachusetts. Doctoral Dissertations. Amherst, MA: University of Massachusetts Amherst.
Stoleson, S. H. (2013). Condition varies with habitat choice in postbreeding forest birds. Auk 130, 417–428. doi: 10.1525/auk.2013.12214
Streby, H. M., Peterson, S. M., and Andersen, D. E. (2016). “Survival and habitat use of edgling Golden-winged Warblers in the western Great Lakes region,” in Golden-winged warbler ecology, conservation, and habitat management. Studies in Avian Biology (no. 49), eds H. M. Streby, D. E. Andersen, and D. A. Buehler (Boca Raton, FL: CRC Press), 127–140. doi: 10.7717/peerj.4319
Strittholt, J. R., DellaSala, D. A., and Jiang, H. (2006). Status of mature and old-growth forests in the Pacific Northwest. Conserv. Biol. 20, 363–374. doi: 10.1111/j.1523-1739.2006.00384.x
Tallamy, D. W. (2021). The nature of Oaks: the rich ecology of our most essential native trees. Portland: Timber Press.
Tanner, R. A., and Gange, A. C. (2005). Effects of golf courses on local biodiversity. Landsc. Urban Plann. 71, 137–146. doi: 10.1016/j.landurbplan.2004.02.004
Tavernia, B. G., Nelson, M. D., Garner, J. D., and Perry, C. H. (2016). Spatial characteristics of early successional habitat across the upper great lakes states. For. Ecol. Manag. 372, 164–174. doi: 10.1016/j.foreco.2016.04.003
Telford, S. R. (2017). Deer reduction is a cornerstone of integrated deer tick management. J. Integr. Pest Manag. 8, 25;1–5. doi: 10.1093/jipm/pmx024
Terborgh, J. W. (2015). Toward a trophic theory of species diversity. Proc. Natl. Acad. Sci. U.S.A. 11415–11422. doi: 10.1073/pnas.1501070112
Terborgh, J., Estes, J. A., Paquet, P., Ralls, K., Boyd-Heger, D., Miller, B. J., et al. (1999). “The role of top carnivores in regulating terrestrial ecosystems,” in Continental Conservation: Scientific Foundations of Regional Reserve Networks, eds M. E. Soulé and J. Terborgh (Washington, DC: Island Press), 39–64. doi: 10.1111/j.1469-185X.2011.00203.x
The Lancet Global Health (2020). Editorial: Mental health matters. Lancet Glob. Health 8:E1352. doi: 10.1016/S2214-109X(20)30432-0
The White House (2022). Executive order on strengthening the nation’s forests, communities, and local economies. presidential actions, April 22, 2022. Available online at: https://www.whitehouse.gov/briefing-room/presidential-actions/2022/04/22/executive-order-on-strengthening-the-nations-forests-communities-and-local-economies/ (accessed November 6, 2022).
The Wildlife Society (2017). State and tribal wildlife grant program. Available online at: https://wildlife.org/wp-content/uploads/2014/11/Policy-Brief_STWG_FINAL.pdf (accessed November 6, 2022).
Thom, D., and Keeton, W. S. (2020). Disturbance-based silviculture for habitat diversification: Effects on forest structure, dynamics, and carbon storage. For. Ecol. Manag. 469:118132. doi: 10.1016/j.foreco.2020.118132
Thom, D., Golivets, M., Edling, L., Meigs, G. W., Gourevitch, J. D., Sonter, L. J., et al. (2019). The climate sensitivity of carbon, timber, and species richness covaries with forest age in boreal–temperate North America. Glob. Change Biol. 25, 2446–2458. doi: 10.1111/gcb.14656
Thompson, A., and Walls, M. A. (2021). Getting to 30x30: important considerations for the biden administration’s conservation Agenda. Resources for the future. Available online at: https://www.resources.org/common-resources/getting-to-30x30-important-considerations-for-the-biden-administrations-conservation-agenda/ (accessed November 6, 2022).
Thompson, I., Mackey, B., McNulty, S., and Mosseler, A. (2009). Forest resilience, biodiversity, and climate change. A synthesis of the biodiversity/resilience/stability relationship in forest ecosystems. Technical Series no. 43. Montreal: Secretariat of the Convention on Biological Diversity, 67.
Thompson, J. (2006). Society’s choices: land use changes, forest fragmentation, and conservation. Science Findings 88. Portland, OR: U.S. Department of Agriculture, Forest Service, Pacific Northwest Research Station, 5.
Thompson, J. R., Carpenter, D. N., Cogbill, C. V., and Foster, D. R. (2013). Four centuries of change in Northeastern United States forests. PLoS One 8:e72540. doi: 10.1371/journal.pone.0072540
Thompson, J. R., Lambert, K. F., Foster, D. R., Broadbent, E. N., Blumstein, M., Almeyda Zambrano, A. M., et al. (2016). The consequences of four land-use scenarios for forest ecosystems and the services they provide. Ecosphere 7:e01469. doi: 10.1002/ecs2.1469
Tiako, M., Nguemeni, J., McCarthy, C., Meisel, Z. F., Elovitz, M. A., Burris, H. H., et al. (2021). Association between low urban neighborhood greenness and hypertensive disorders of pregnancy. Am. J. Perinatol. doi: 10.1055/s-0041-1733786 [Epub ahead of print].
Titus, H. (1945). The land nobody wanted: the story of Michigan’s public domain. East Lansing, MI: Michigan State College, Agricultural Experiment Station, Section of Conservation.
Toot, R., Frelich, L. E., Butler, E., and Reich, P. B. (2020). Climate-biome envelope shifts create enormous challenges and novel opportunities for conservation. Forests 11:1015. doi: 10.3390/f11091015
Tulowiecki, S. J., Ranney, E. R., Keenan, E. M., Neubert, G. M., and Hogan, M. L. (2022). Localized Native American impacts on past forest composition across a regional extent in north-eastern United States. J. Biogeogr. 49, 1099–1109. doi: 10.1111/jbi.14369
U.S. Department of Agriculture (2020). Farms and land in farms: 2019 summary. National agricultural statistics service. ISSN: 1995-2004. Available online at: https://www.nass.usda.gov/Publications/Todays_Reports/reports/fnlo0220.pdf (accessed November 6, 2022).
U.S. Fish and Wildlife Service (2006). Species of concern: cerulean warbler (Setophaga cerulean). Available online at: https://web.archive.org/web/20220127133641/https://www.fws.gov/midwest/es/soc/birds/cerw/pdf/Cerulean_Warbler_Fact_Sheet.pdf (accessed November 5, 2022).
U.S. Fish and Wildlife Service (2015a). Historical distribution of the New England cottontail (Sylvilagus transitionalis). Supplemental document to the New England cottontail 12-month petition finding, docket number FWS–R5–2015–0136 July 27, 2015. Available online at: https://web.archive.org/web/20220120215800/https://www.fws.gov/northeast/newenglandcottontail/pdf/20150727_NEC_12M_HistoricalDistributionSupplement.pdf (accessed November 7, 2022).
U.S. Fish and Wildlife Service (2015b). Endangered and threatened wildlife and plants; 12-month finding on a petition to list the New England cottontail as an endangered or threatened species. Fed. Reg. Vol. 80 No. 178 55286. (proposed September 15, 2015). Washington, D.C: U.S. Fish and Wildlife Service.
U.S. Fish and Wildlife Service (2015c). New England cottontail (Sylvilagus transitionalis). Available online at: https://web.archive.org/web/20170312201433/https://www.fws.gov/northeast/newenglandcottontail/pdf/NEcottontail2015.pdf (accessed November 5, 2022).
U.S. Fish and Wildlife Service (2022a). Endangered and threatened wildlife and plants; endangered species status for northern long-eared Bat. Fed. Reg. Vol. 87, No. 229 73488 (proposed Wednesday, November 30, 2022). Washington, D.C: U.S. Fish and Wildlife Service.
U.S. Fish and Wildlife Service (2022b). FWS-Listed U.S. species by taxonomic group. ECOS environmental conservation online system. Available online at: https://ecos.fws.gov/ecp/report/species-listings-by-tax-group-totals (accessed November 7, 2022).
U.S. Geological Survey (2022a). Protected areas database of the United States (PAD-US). 3.0: U.S. Geological Survey data release. Reston, VA: U.S. Geological Survey. Available online at: https://www.usgs.gov/programs/gap-analysis-project/science/pad-us-data-download (accessed November 7, 2022).
U.S. Geological Survey (2022b). Gap analysis project (GAP): PAD-US data overview. Available online at: https://www.usgs.gov/programs/gap-analysis-project/science/pad-us-data-overview (accessed October 12, 2022).
USDA Forest Service (2003). Major trend data 1760-2000. Forest inventory & analysis. Available online at: https://web.archive.org/web/20220811180428/https://www.fia.fs.fed.us/slides/major-trends.pdf (accessed October 12, 2022).
USDA Forest Service (2017). Harvest trends on national forest system lands: 1984 to present. Available online at: https://web.archive.org/web/20220120151939/https://www.fs.fed.us/forestmanagement/documents/harvest-trends/NFS-HarvestHistory1984-2017.pdf (accessed November 7, 2022).
USDA Forest Service (2018). Early successional habitat creation project: notice of proposed action and opportunity to comment. Green mountain national forest. Available online at: https://www.wallingfordvt.com/wp-content/uploads/2014/07/GMNF-Scoping-Details-Habitat-Creation.pdf (accessed October 12, 2022).
US Forest Service (2021a) Tarleton integrated resource project grafton county, new hampshire draft environmental assessment and preliminary finding of no significant impact. White Mountain National Forest, – Ranger District. Available online at: https://www.fs.usda.gov/project/?project=56394 (accessed November 6, 2022).
USDA Forest Service (2021b). Fiscal year 2021 S&PF landscape scale restoration funded projects for the Northeast and Midwest. Available online at: https://usfspublic. app.box.com/v/FY21FundedList (accessed November 7, 2022).
USDA Forest Service (2022a). Biden administration announces $32 million to advance climate-smart mass timber construction, expand wood markets, 27 May. [Press release]. Available online at: https://www.usda.gov/media/press-releases/2022/05/27/biden-administration-announces-32-million-advance-climate-smart (accessed November 15, 2022).
USDA Forest Service (2022b). Forest inventory EVALIDator web-application Version 1.8.0.01., Forest inventory and analysis program. St. Paul, MN: Northern Research Station.
USDA Forest Service (2022c). Climate adaptation plan. FS-1196. Washington, D.C: U.S. Department of Agriculture.
USDA Forest Service (2022d). Forest service to use prescribed fire to improve wildlife habitat, 26 April. Available online at: https://www.fs.usda.gov/detail/gmfl/news-events/?cid=FSEPRD1015733 (accessed November 7, 2022).
USDA Forest Service (2022e). Fiscal year 2022 S&PF landscape scale restoration funded projects for the northeast and midwest. Available online at: https://usfs-public.app.box.com/s/1v1o17zgofogxnolgf4jmgm8cltaw49e (accessed November 7, 2022).
USDA Forest Service and Bureau of Land Management (2022). Request for information (RFI) on federal old-growth and mature forests. Fed. Reg. Vol. 87, No. 135, 42493 (proposed Friday, July 15, 2022). Washington, D.C: USDA Forest Service and Bureau of Land Management.
USGCRP (2018). Impacts, risks, and adaptation in the United States: fourth national climate assessment, volume II: report-in-brief, eds D. R. Reidmiller, C. W. Avery, D. R. Easterling, K. E. Kunkel, K. L. M. Lewis, T. K. Maycock, et al. (Washington, DC: U.S. Global Change Research Program), 186. doi: 10.7930/NCA4.2018.RiB
Vale, T. R. (1998). The myth of the humanized landscape: An example from yosemite national park. Natural Areas J. 18, 231–236.
Vale, T. R. (2002). “The pre-European landscape of the United States: pristine or humanized?,” in Fire, native peoples and the natural landscape, ed. T. R. Vale (Washington, D.C: Island Press), 1–39.
Vantellingen, J., and Thomas, S. C. (2021). Log landings are methane emission hotspots in managed forests. Can. J. For. Res. 51, 1916–1925. doi: 10.1139/cjfr-2021-0109
Veatch, J. O. (1928). Reconstruction of forest cover based on soil maps. Q. Bull. Michigan Agric. Exp. Station 10, 116–126.
Vega Rivera, J. H., Rappole, J. H., Mcshea, W. J., and Haas, C. A. (1998). Wood thrush postfledging movements and habitat use in Northern Virginia. The Condor 100, 69–78.
Vining, J., and Tyler, D. E. (1999). Values, emotions and desired outcomes reflected in public responses to forest management plans. Hum. Ecol. Rev. 6, 21–34.
Wang, K., Lombard, J., Rundek, T., Dong, C., Marinovic Gutierrez, C., Byrne, M. M., et al. (2019). Relationship of neighborhood greenness to heart disease in 249 405 US medicare beneficiaries. J. Am. Heart Assoc. 8:e010258. doi: 10.1161/JAHA.118.010258
Warrick, G. (2000). The precontact iroquoian occupation of Southern Ontario. J. World Prehistory 14, 415–466. doi: 10.1023/A:1011137725917
Watson, J. E. M., Evans, T., Venter, O., Williams, B., Tulloch, A., Stewart, C., et al. (2018). The exceptional value of intact forest ecosystems. Nat. Ecol. Evol. 2, 599–610. doi: 10.1038/s41559-018-0490-x
Weber, S., and Cooper, T. R. (2019). “Implementing the American woodcock conservation plan: wildlife management institute’s young forest initiative,” in Proceedings of the eleventh american woodcock symposium, eds D. G. Krementz, D. E. Andersen, and T. R. Cooper (Minneapolis, MN: University of Minnesota Libraries Publishing), 5–8. doi: 10.24926/AWS.0102
Weidensaul, S. (2018). Old-Growth is great, but here’s why we need new-growth forests, too. Living bird magazine, 28 March. The cornell lab of ornithology. Available online at: https://www.allaboutbirds.org/news/old-growth-is-great-but-heres-why-we-need-new-growth-forests-too/ (accessed November 7, 2022).
Weyerhaeuser Company (2020). How we do it: wildlife habitat. Available online at: https://www.weyerhaeuser.com/application/files/3715/9363/0295/WY_How_We_Do_It_Wildlife_Habitat_2020.pdf (accessed November 7, 2022).
Whitcomb, K. Jr. (2022). Vermont logging drawing criticism. The rutland herald, 15 July. Available online at: https://www.eagletimes.com/vermont-logging-drawing-criticism/article_43b9113c-fad7-54e5-a13d-38d92c3e4303.html (accessed November 7, 2022).
Whitney, G. G. (1994). From coastal wilderness to fruited plain: a history of environmental change in temperate North America, 1500 to the present. Cambridge: Cambridge University Press.
Widmann, R. H., Crawford, S., Kurtz, C. M., Nelson, M. D., Miles, P. D., Morin, R. S., et al. (2015). New York forests, 2012. Resource bulletin NRS-98. Newtown Square, PA: U.S Department of Agriculture, Forest Service, Northern Research Station, 128.
Wiggins, D. A. (2006). Ruffed grouse (Bonasa umbellus): A Technical Conservation Assessment. Washington, DC: USDA Forest Service, Rocky Mountain Region.
Wilcove, D. S., McClellan, C. H., and Dobson, A. P. (1986). “Habitat fragmentation in the temperate zone,” in Conservation biology: the science of scarcity and diversity, ed. M. E. Soule (Sunderland, MA: Sinauer Associates), 237–256.
Wildlife Management Institute (2009). Upper great lakes woodcock and young forest initiative: best management practices for woodcock & associated bird species. Washington, DC: Wildlife Management Institute.
Wildlife Management Institute (2010). Implementing the American woodcock conservation plan: progress to date. Washington, DC: Wildlife Management Institute.
Williams, C. A., Collatz, G. J., Masek, J., and Goward, S. N. (2012). Carbon consequences of forest disturbance and recovery across the conterminous United States. Glob. Biogeochem. Cycles 26:GB1005. doi: 10.1029/2010GB003947
Williams, C. A., Hasler, N., and Xi, L. (2021). Avoided deforestation: a climate mitigation opportunity in New England and New York prepared for the United States climate alliance natural and working lands research program. Worcester, MA: Clark University, 1–42.
Williams, G. W. (2002). “Aboriginal use of fire: are there any ‘natural’ plant communities?,” in Wilderness and political ecology: aboriginal land management – myths and reality, eds C. E. Kay and R. T. Simmons (Salt Lake City, UT: University of Utah Press), 48.
Wilson, D. C., Morin, R., Frelich, L. E., and Ek, A. R. (2019). Monitoring disturbance intervals in forests: A case study of increasing forest disturbance in Minnesota. Ann. For. Sci. 76:78. doi: 10.1007/s13595-019-0858-3
Windels, S., and Flaspohler, D. J. (2011). The ecology of Canada Yew (Taxus canadensis Marsh.): A review. Botany 89, 1–17. doi: 10.1139/B10-084
Wisconsin Department of Natural Resources (2020). Wisconsin 2020 Statewide Forest Action Plan. Madison, WI: Wisconsin Department of Natural Resources.
Wolfkill, J., Bejarano, M. E., Serfass, T. L., Turner, G., Brosi, S., Feller, D., et al. (2021). The prevalence of the raccoon roundworm, Baylisascaris procyonis, in allegheny woodrat habitat in the Mid-Atlantic Region, USA. Am. Midland Naturalist 185, 145–147.
Wuerthner, G., Crist, E., and Butler, T. (eds) (2015). Protecting the Wild: parks and wilderness, the foundation for conservation. London: Island Press.
WWF (2022). Living planet report 2022 – Building a nature-positive society, eds R. E. A. Almond, M. Grooten, D. Juffe Bignoli, and T. Petersen (Gland: WWF).
Xu, X., Huang, A., Belle, E., De Frenne, P., and Jia, G. (2022). Protected areas provide thermal buffer against climate change. Sci. Adv. 8:eabo0119. doi: 10.1126/sciadv.abo0119
Yamasaki, M., Costello, C. A., and Leak, W. B. (2014). Effects of clearcutting, patch cutting, and low-density shelterwoods on breeding birds and tree regeneration in New Hampshire northern hardwoods. Res. Pap. NRS- 26. Newtown Square, PA: U.S. Department of Agriculture, Forest Service, Northern Research Station, 15. doi: 10.2737/NRS-RP-26
Yeo, S., Weber, C., Carvalho, F., Clare, L., Woods, M., Merriman, P., et al. (2019). Climate, nature and our 1.5°C future: a synthesis of IPCC and IPBES reports. Gland: WWF International.
Young Forest Project (2012). The solution: a new understanding. Available online at: https://web.archive.org/web/20130427082104/http://youngforest.org/the-solution (accessed October 2, 2022).
Young Forest Project (2022a). Partners. Available online at: https://youngforest.org/partners (accessed October 2, 2022).
Young Forest Project (2022b). Wildlife and sport fish restoration program boosts young forest. Available online at: https://youngforest.org/content/wsfr-funding (accessed November 7, 2022).
Young Forest Project (2022c). The challenge: we’re losing young forest on the land. Available online at: https://youngforest.org/the-challenge (accessed November 7, 2022).
Young Forest Project (2022d). Fort Indiantown Gap, Southeastern Pennsylvania: “Training-scape” helps soldiers, wildlife. Available online at: https://youngforest.org/demo/fort-indiantown-gap-southeastern-pennsylvania (accessed October 2, 2022).
Zaplata, M. K., and Dullau, S. (2022). Applying ecological succession theory to birds in solar parks: An approach to address protection and planning. Land 11:718. doi: 10.3390/land11050718
Zhao, D., Sun, Z., Wang, C., Hao, Z., Sun, B., Zuo, Q., et al. (2020). Using count data models to predict epiphytic bryophyte recruitment in Schima superba Gardn. et Champ. Plantations in urban forests. Forests 11:174. doi: 10.3390/f11020174
Zheng, D., Heath, L. S., and Ducey, M. J. (2008). Spatial distribution of forest aboveground biomass estimated from remote sensing and forest inventory data in New England, USA. J. Appl. Remote Sens. 2:021502. doi: 10.1117/1.2940686
Zheng, D., Heath, L. S., and Ducey, M. J. (2013). Carbon benefits from protected areas in the conterminous United States. Carbon Balance Manag. 8:4. doi: 10.1186/1750-0680-8-4
Zheng, D., Heath, L. S., Ducey, M. J., and Butler, B. (2010). Relationships between major ownerships, forest aboveground biomass distributions, and landscape dynamics in the New England region of USA. Environ. Manag. 45, 377–386. doi: 10.1007/s00267-009-9408-3
Zhou, G., Liu, S., Li, Z., Zhang, D., Tang, X., Zhou, C., et al. (2006). Old-growth forests can accumulate carbon in soils. Science 314:1417. doi: 10.1126/science.1130168
Zlonis, E. J., and Niemi, G. J. (2014). Avian communities of managed and wilderness hemiboreal forests. For. Ecol. Manag. 328, 26–34. doi: 10.1016/j.foreco.2014.05.017
Keywords: natural climate solutions, forest carbon, old-growth forests, young forest, clearcutting, biodiversity, ecosystem services, wildlands
Citation: Kellett MJ, Maloof JE, Masino SA, Frelich LE, Faison EK, Brosi SL and Foster DR (2023) Forest-clearing to create early-successional habitats: Questionable benefits, significant costs. Front. For. Glob. Change 5:1073677. doi: 10.3389/ffgc.2022.1073677
Received: 18 October 2022; Accepted: 12 December 2022;
Published: 09 January 2023.
Edited by:
Alfredo Di Filippo, University of Tuscia, ItalyReviewed by:
Giuliano Maselli Locosselli, University of São Paulo, BrazilHeather Keith, Griffith University, Australia
Copyright © 2023 Kellett, Maloof, Masino, Frelich, Faison, Brosi and Foster. This is an open-access article distributed under the terms of the Creative Commons Attribution License (CC BY). The use, distribution or reproduction in other forums is permitted, provided the original author(s) and the copyright owner(s) are credited and that the original publication in this journal is cited, in accordance with accepted academic practice. No use, distribution or reproduction is permitted which does not comply with these terms.
*Correspondence: Michael J. Kellett, kellett@restore.org